- 1Department of Material Science and Engineering, Promotion Center for Global Materials Research (PCGMR), National Cheng Kung University, Tainan, Taiwan
- 2School of Energy Science and Engineering, Vidyasirimedhi Institute of Science and Technology, Rayong, Thailand
We report the development of a simple, one-pot solution-processing synthesis of nitrogen (N) and boron (B) co-doped in few-layered graphene by submerged liquid plasma exfoliation (SLPE). Simultaneous graphite exfoliation and N, and B co-doping in few layered graphene nanosheets (NB-GNs) were produced by micro-plasma discharge at the graphite tip immersed in the acetonitrile (ACN) solvent containing sodium tetraphenylborate. Higher boron contents led to an amorphous nano-carbon, while a few-layered NB-GNs was obtained at low boron content in the ACN reaction mixture. To analyze the structural defect, nanosheet morphology, N and B co-doping in a few-layered graphene, X-ray diffraction (XRD), Raman spectroscopy, transmission electron microscopy coupled with energy-dispersive X-ray spectroscopy (TEM-EDS) and X-ray photoelectron spectroscopy (XPS) were used. This solution-processing strategy will be useful for the practical option of exploring a large family of two-dimensional (2D) materials.
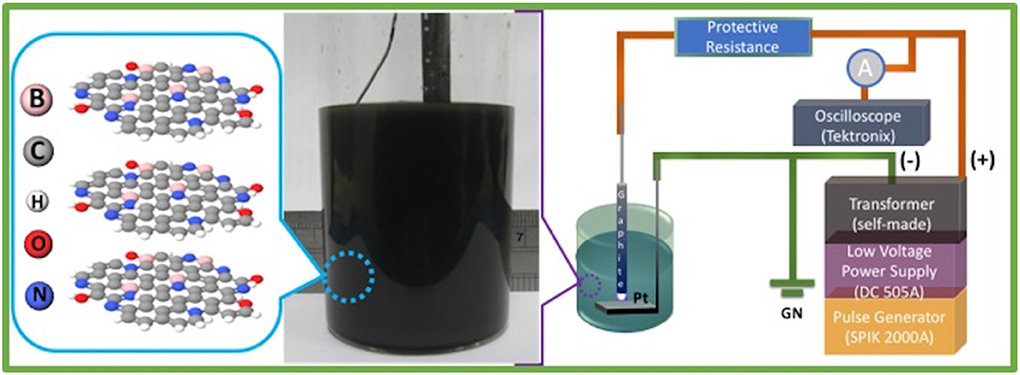
Graphical Abstract. The graphical representation on in situ, one-pot solution-processing synthesis of nitrogen (N) and boron (B) co-doped in few-layered graphene via submerged liquid plasma exfoliation (SLPE) approach.
Introduction
Chemically functionalized graphene-based hybrid materials with specific morphology emerged as an attractive material for the various applications due to the specific surface area of graphene, electrical, thermal, optical properties (Kuila et al., 2012). Covalent bonds with specific functional groups and chemical doping are the simplest way for modification of graphene that involves the chemical modification of the carbon sp2 lattice, by introducing heteroatoms, such as S, P, Se, Si, N, and B (Panchakarla et al., 2009; Lazar et al., 2014; Agnoli and Favaro, 2016). Such modified graphene demonstrates the enhanced chemical and electrochemical catalytic activity in the oxygen reduction reaction (ORR), sensors, biomedicine, and photovoltaics applications (Usachov et al., 2011; Kuila et al., 2012; Agnoli and Favaro, 2016).
It has been reported that B-doped graphene (BG) and N-doped graphene (NG) can be synthesized to exhibit p- and n-type semiconducting electronic properties that can be systematically tuned with the concentration of B and N for the various applications (Panchakarla et al., 2009; Muhammad et al., 2017, 2018; Rafique et al., 2019). The NG and BG hybrids are synthesized from the molecular carbon and boron/nitrogen as a starting precursor. Though, the graphene oxide (GO) has been used as a common precursor for the chemical functionalization/doping (Panchakarla et al., 2009; Wang et al., 2012; Agnoli and Favaro, 2016). In post-synthesis, hetero-atoms doping in graphene has been performed by two-step modification; (1) GO/reduced-GO synthesized by chemical, electrochemical and liquid phase exfoliation, and (2) doping step by solid-state reaction, hydrothermal/solvothermal, electrochemical and chemical vapor deposition method (Agnoli and Favaro, 2016). Although, there have been numerous reports on the synthesizing of NGs and BGs (Usachov et al., 2011; Wang et al., 2012; Senthilnathan et al., 2013, 2014a,b,c, 2015; Lazar et al., 2014; Sanjeeva Rao et al., 2014), the simultaneous exfoliated graphite and multiple-heteroatom doping (N and B) into the graphene framework could be practically challenged at one-pot synthesis.
Herein, we propose a simple strategy on simultaneous graphite exfoliation, and N and B co-doping into a few-layered GNs by SLPE, as a proof of concept proposed by Yoshimura et al. (Senthilnathan et al., 2013, 2014a,b,c, 2015; Sanjeeva Rao et al., 2014). Therefore, the strategy presented here will be practically a useful option for exploring the multiple-heteroatom doping into a large family of two-dimensional (2D) material manufacturing such as, transition metal carbides and nitrides (MXenes), transition metal dichalcogenides (TMDs), h-BN and borophene (Gogotsi, 2015; Satheeshkumar et al., 2016a,b; Anasori et al., 2017; Sarycheva et al., 2017) by the non-conventional method.
Experimental Section
In submerged liquid plasma exfoliation (SLPE), different amounts of sodium tetraphenylborate-NaBPh4 (99.5%) was added into the anhydrous acetonitrile (ACN, 99.95%) solvent; both are purchased from the Sigma-Aldrich. The concentration of ACN was fixed in 0.77 mol (40 mL), as a nitrogen source and 10 min of the graphite exfoliation for all experiments. To study the effect of the addition of boron concentration on graphite exfoliation by SLPE, the sodium tetraphenylborate is 0.1, 1, 2.5, and 5 mM were added into the ACN solvent, before the exfoliation. The distance between cathode and anode is ~2.0 mm kept as constant for all experiments by using a moving stage assembly (Translation Stage Triple-Divide Series 9064 and 9065) operated by a computer. A discharge voltage was applied between the two electrodes with respect to the concentration of boron in the solvents (the applied discharge voltage of 300–400 V, 275–350 V, 245–320 V, and 208–300 V for the reaction mixtures containing the concentration of 5, 2.5, 1.0, and 0.1 mM boron in 0.77 mol of ACN solvent, respectively), a pulse delay of 250 μs, and a pulse width of 10 μs using a pulse generator (AVTECH AV-1022-C) connected to a high-voltage amplifier (TREK Model 609E-6), which can generate 0.1–5 kV (Senthilnathan et al., 2013, 2014a,b,c, 2015; Sanjeeva Rao et al., 2014). The powder X-ray diffraction (Bruker new D8 ADVANCE instrument equipped with a monochromatic Cu Kα radiation with a wavelength of 1.5418 Å, which is operated at 40 kV and 40 mA measurement.
Characterization
The morphology of NB-GNs was examined using transmission electron microscopy (TEM) (HT7700, Hitachi, Tokyo, Japan). The Raman spectra were measured on a RamanScope SENTERRA II (Bruker) with 532 nm excitation wavelength laser. For Raman measurements, the samples were deposited onto the glass substrate and recorded using a thermoelectric-cooled CCD array detector (Andor, DU420A-OE-152). The Raman signal was transferred through a 50 μm slit of a spectrometer and the spectral resolution of the spectrograph at 4 cm−1 across the Raman data acquired. The spectral acquisition time was 5 s for all the samples with the 2 accumulations averaged with a laser-power of 3 mW, 20× objective lens. The high-resolution X-ray photoelectron spectroscopy (XPS) measurements were carried out on a PHI Quantera SXM spectrometer (ULVAC Inc., Kanagawa, Japan), and PF4 software was used for the deconvolution of the narrow-scan XPS spectra. XPS was performed with a monochromatic Al Ka source (25 W, hυ = 1486.6 eV) and an energy resolution of 1 eV.
Results and Discussion
In the SLPE experiment, graphite rod was used as a point high-voltage electrode and a Pt sheet was used as a planar ground electrode, as shown in Figure 1A. The powder XRD pattern of graphite and exfoliated graphite as shown in Figure 1B. The exfoliated amorphous nano-carbon (Figure 1B, spectrum b-d) shows the increasing the 2θ value of (002) with contraction of the interlayer space when compared with that of pristine graphite (Figure 1B spectrum a). This contraction of the interlayer spacing was observed only for the amorphous nano-carbon samples (Figure 1B, spectrum b-d) because of the high plasma voltage (refer experimental section) were applied during the exfoliation. The plasma was produced only at this high discharge voltage due to the presence of high boron concentration in the precursor mixture solution, which certainly increases the conductivity of the reaction mixture (Gupta, 1981; Sokolov et al., 1984; Ka and Oh, 2008). Such a high-applied discharge plasma voltage could cause the contraction in the interlayer of as produced amorphous nano-carbon. The XRD peak at around 26.4 (2θ) is due to (002) lattice plane of graphite structure it goes lattice contraction with decreasing intensity of (002) plane as the concentration of boron decreased from 5 to 1 mM in 0.77 mol ACN (Figure 1B spectrum b to d). Also, the full width at a half maximum of 002 peak decreases with the decreasing concentration of boron in the ACN mixtures, as a result the intensity of 002 planes at 26.9 (2θ) gradually diminished and, in turn, showed a broad XRD feature between the 20-30 (2θ) where the graphitic peak at 26.9 (2θ) was absent, suggesting that a few-layered NB-NGs were produced, as the concentration of boron was 0.1 mM.
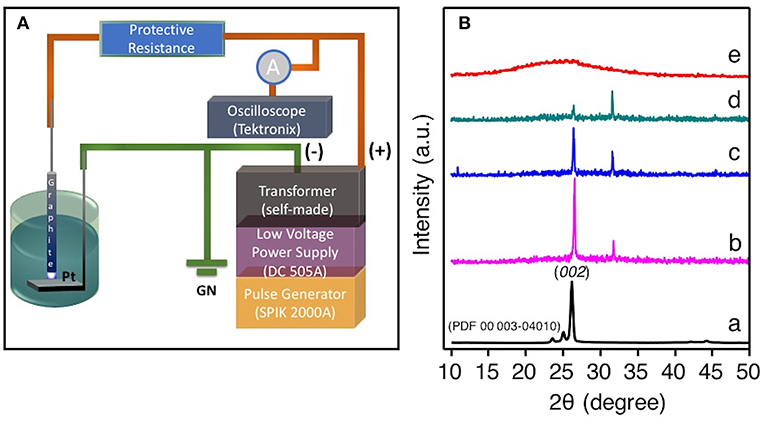
Figure 1. (A) The systematic representation of graphite exfoliation in ACN by SLEP setup. (B) The powder XRD pattern of pristine graphite (a), Exfoliated graphite obtained by SLPE in the presence of various concentration ranging from 5 mM (b), 2.5 mM (c), 1 mM (d), and (e) 0.1 mM sodium tetraphenylborate-NaBPh4 in 0.77 mol ACN.
TEM analysis on the morphology of few-layered NB-NGs studied in the presence of a various concentration of boron in ACN by SLPE (Figure 2). The low and high-resolution images revealed the amorphous-like nano-carbons are produced, as the concentration of boron was 5 mM (Figures 2A–C). In contrast, multilayers, sponge-like graphene-network (Figures 2D–F) and few-layered graphene nanosheets (Figures 2G–I) were obtained upon the use of 1 and 0.1 mM boron, respectively. The SAED pattern analysis on few layered NB-GNs (Figure 2I), which reveal the graphitic-like honey-comb lattice nanostructure and the EDX mapping analysis showed the presence of nitrogen (green), boron (red) and carbon (blue) in few layered NB-GNs (Figure 2). Note that, the concentration of boron plays a vital role in the graphite exfoliation in ACN by SLPE and concluded that lower boron-content in the precursor mixture would keep the nanosheets morphology.
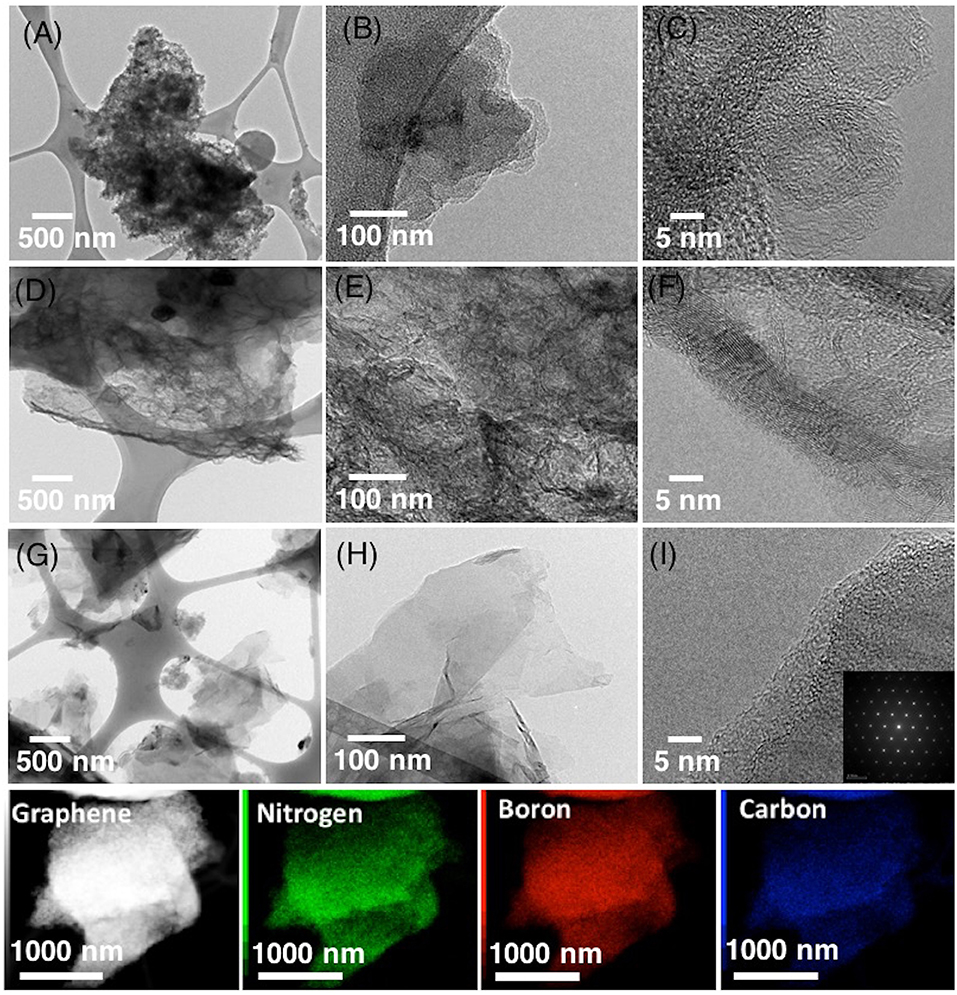
Figure 2. The low and corresponding high-resolution TEM images of few-layered NB-GNs synthesized by SLPE in the presence of 5 mM (A–C), 2.5 mM (D–F), and 0.1 mM sodium tetraphenylborate-NaBPh4 (G–I) in 0.77 mol ACN. The EDX mapping analysis showing the presence of N, B, and C in NB-GNs by SLPE, with a precursor containing 0.1 mM NaBPh4 in 0.77 mol ACN.
Raman spectroscopy analysis was carried out to evaluate the degrees of boron doping (as co-doping) in a structural distortion of graphene (Figure 3A). In graphite, the two Raman peaks at 1,578 and 2,667 cm−1 for the G and 2D peak, respectively, while the weak Raman peak shown at 1,342 cm−1 is attributed to D peak due to the breathing modes of sp2 rings (A1g symmetry). The position of a D peak in all exfoliated graphite was blue-shifted to 1,334 cm−1 when compared to that of graphite (1,342 cm−1), as the concentration of boron decreased from 5 to 0.1 mM. This peak shifting occurs due to the sp3 carbon content in graphene and the increasing D peak intensity (from red to jade color) due to the degree of short-range disorder, such as in-plane substitutional heteroatoms, vacancies, and grain boundaries (Lazar et al., 2014). Note that the G peak position for exfoliated graphite showing red-shifted at 1,586 cm−1 (Dresselhaus et al., 2008; Panchakarla et al., 2009) reveals the doping of N and B-atom into graphene framework, since the G peak of graphene responds to doping results in phonon stiffening (Dresselhaus et al., 2008; Panchakarla et al., 2009). Besides, the exfoliated graphite (exclude graphite) has exhibit the Raman D'-band which represent the intravalley double resonance scattering processes due to the N-doping in grapheme (Dresselhaus et al., 2008; Ferrari and Basko, 2013).
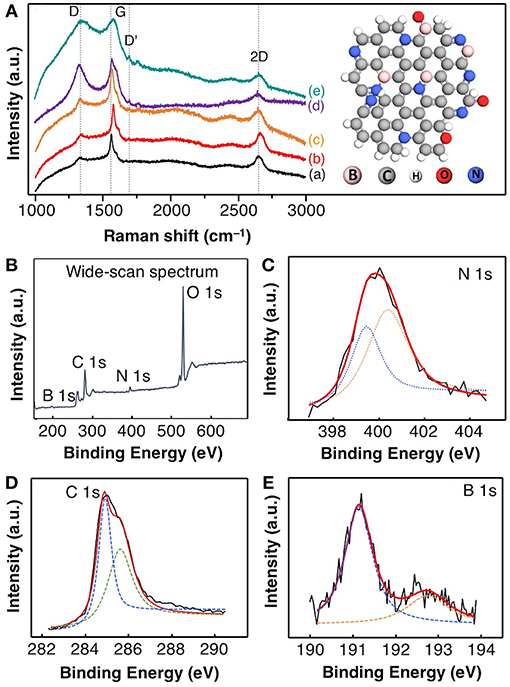
Figure 3. (A) The Raman spectra of (a) pristine graphite and NB-GNs synthesized by SLPE in the presence of (b) 5 mM, (c) 2.5 mM, (d) 1 mM, and (e) 0.1 mM NaBPh4 in 0.77 mol ACN. The high-resolution XPS analysis of individual deconvolution core-level (B) wide-scan, (C) N 1 s, (D) C 1 s, and (E) B 1s spectra of NB-GNs (0.1 mM NaBPh4 in 0.77 mol ACN). The insert (on top) represents the ball-stick model of N and B co-doped graphene based on Raman spectroscopy and XPS analysis.
It is interesting to note that the Raman 2D peak has shown red-shifted from at 2,667 to 2,671 cm−1 for graphite and exfoliated graphite, respectively, indeed became a more symmetric 2D band. From these results, the red-shifted 2D peak can be explained by the enlarged graphite interlayer upon exfoliation and N, and B-doping in the graphene framework (Panchakarla et al., 2009; Ferrari and Basko, 2013). The intensity ID/IG ratio can be used as an indication of the degree of disorder in a carbon structure. Thus, the ID/IG ratios of graphite were compared to that of the exfoliated graphite (1 mM boron), ID/IG are 0.593 and 0.812, respectively. The higher ID/IG ratio is a result of structural defects caused by both, the N and B-heteroatoms were implanted at the radicalized graphene site (Dresselhaus et al., 2008; Panchakarla et al., 2009; Ferrari and Basko, 2013). The insertion of N and B-groups increases the sp3 carbon character in the synthesized graphene, resulting in changes in the hybridization and symmetry of few-layered NB-GNs.
Interestingly, the G peak is the usual response upon doping in the graphene, results in the phonon stiffening occurs due to the high-hole doping, while phonon softening caused high-electron doping in the grapheme (Panchakarla et al., 2009). Since the G peak always stiffens, the 2D peak responds differently to holes and electron doping (Dresselhaus et al., 2008; Panchakarla et al., 2009; Usachov et al., 2011; Wang et al., 2012; Lazar et al., 2014). Based on the above facts, it is possible to estimate the doping level by monitoring both the G and 2D peaks ratio. In principle, the doping causes the decreasing the I2D/IG ratio value, accordingly, we have estimated the I2D/IG ratio, they are 0.572 and 0.261 for the graphite and NB-GNs (0.1 mM boron), respectively.
To further investigate the doping of N and B in exfoliated graphite, the XPS analysis was carried out to estimate the atom% N and B species present in the exfoliated graphite. The wide-scan XPS spectrum reveals the presence of B, C, N, and O (Figure 3B). The deconvoluted N 1s spectrum indicates the chemically distinguished two nitrogen components (Figure 3C) which have shown the binding energy (BE) at 398.8 and 400.6 eV for the pyridinic nitrogen (sp2 hybridization) and N-atom in the exfoliated graphite, respectively (Gammon et al., 2003). The C 1s spectrum (Figure 3D), which could be deconvoluted into C–C and C–N with BE at 284.7 and 285.7 eV, respectively. In addition, the B 1s spectra were deconvoluted into two peaks at 191.2 eV and 192.8 eV for N–B–C and B–O species, respectively (Figure 3E). Note that strong BE at 191.2 eV peak suggests that B and N are mainly bonding as B–N because the pure boron BE peak usually appears at 188 eV (Panchakarla et al., 2009). This implies the existence of an N atom neighboring with B in the carbon domains and also suggesting that the B bonded to carbon in the sp2 carbon network of graphene. Based on XPS analysis, the doping up to 12.4 atom% and 6.9 atom% for the N and B, respectively, in the exfoliated graphite produced by SLPE in the presence of 0.1 mM of NaBPh4 in 0.77 mol ACN. Herein, our proposed method could be able to obtain the quality of exfoliated graphite-hybrid in a very short time, paving the way for large scalable synthesis of NB-GNs in high yield.
Conclusion
In conclusion, we have successfully demonstrated the effectiveness of the presence of boron concentration in the ACN precursor on scalable, one-pot production of few-layered NB-GNs by SLPE at ambient temperature and pressure. By combining XRD and TEM analysis, we found that the morphology of NB-GNs depends on the concentration of boron used in the precursor solution. The chemical doping of N and B (as co-doped) in the few-layered of graphene nanosheets was ideally monitored by Raman spectroscopy and XPS analysis. The advantage of the reported method is in-situ functionalization of N and B into the few-layered graphene, as well as the rapid exfoliation by submerged liquid plasma process in ACN. Moreover, the simplicity of this solution-processing approach strategy offers new insight into SLPE not only graphite exfoliation but also other graphene analogs of layered 2D transition metal dichalcogenide including transition metal carbides and nitrides (MXenes), h-BN and borophenes.
Data Availability
All datasets generated for this study are included in the manuscript/supplementary files.
Author Contributions
SE developed the synthesis concept and synthesized the materials. SE and C-YS initiated and performed all the experiments. SE, C-YS, and MY analyzed the data and wrote the paper.
Funding
SE was supported by a postdoctoral fellowship from Vidyasirimedhi Institute of Science and Technology (VISTEC), Thailand. MY was supported by Hi-GEM project.
Conflict of Interest Statement
The authors declare that the research was conducted in the absence of any commercial or financial relationships that could be construed as a potential conflict of interest.
Acknowledgments
SE, C-YS, and MY would like to thank the support from National Cheng Kung University, Taiwan.
References
Agnoli, S., and Favaro, M. (2016). Doping graphene with boron: a review of synthesis methods, physicochemical characterization, and emerging applications. J. Mater. Chem. A 4, 5002–5025. doi: 10.1039/C5TA10599D
Anasori, B., Lukatskaya, M. R., and Gogotsi, Y. (2017). 2D metal carbides and nitrides (MXenes) for energy storage. Nat. Rev. Mat. 2:16098. doi: 10.1038/natrevmats.2016.98
Dresselhaus, M. S., Dresselhaus, G., and Hofmann, M. (2008). Raman spectroscopy as a probe of graphene and carbon nanotubes. Philos. Trans. A Math. Phys. Eng. Sci. 366, 231–236. doi: 10.1098/rsta.2007.2155
Ferrari, A. C., and Basko, D. M. (2013). Raman spectroscopy as a versatile tool for studying the properties of graphene. Nat. Nanotech. 8, 235–246. doi: 10.1038/nnano.2013.46
Gammon, W. J., Kraft, O., Reilly, A. C., and Holloway, B. C. (2003). Experimental comparison of N(1s) X-ray photoelectron spectroscopy binding energies of hard and elastic amorphous carbon nitride films with reference organic compounds. Carbon 41, 1917–1923. doi: 10.1016/S0008-6223(03)00170-2
Gogotsi, Y. (2015). Transition metal carbides go 2D. Nat. Mater. 14, 1079–1080. doi: 10.1038/nmat4386
Gupta, R. P. (1981). Lattice relaxation at a metal surface. Phys. Rev. B 23, 6265–6270. doi: 10.1103/PhysRevB.23.6265
Ka, B. H., and Oh, S. M. (2008). Electrochemical activation of expanded graphite electrode for electrochemical capacitor. J. Electrochem. Soc. 155, A685–A692. doi: 10.1149/1.2953525
Kuila, T., Bose, S., Mishra, A. K., Khanra, P., Kim, N. H., and Lee, J. H. (2012). Chemical functionalization of graphene and its applications. Prog. Mater. Sci. 57, 1061–1105. doi: 10.1016/j.pmatsci.2012.03.002
Lazar, P., Zboril, R., Pumera, M., and Otyepka, M. (2014). Chemical nature of boron and nitrogen dopant atoms in graphene strongly influences its electronic properties. Phys. Chem. Chem. Phys. 16, 14231–14235. doi: 10.1039/C4CP01638F
Muhammad, R., Shuai, Y., Irfan, A., and Ping, T. H. (2018). First-principles investigations of manganese oxide (MnOx) complex-sandwiched bilayer graphene systems. RSC Adv. 8, 23688–23697. doi: 10.1039/C8RA03484B
Muhammad, R., Shuai, Y, and Tana, H. P. (2017). A first-principles study on alkaline earth metal atom substituted monolayer boron nitride (BN). J. Mat. Chem. C 5, 8112–8127. doi: 10.1039/C7TC02894F
Panchakarla, L. S., Subrahmanyam, K. S., Saha, S. K., Govindaraj, A., Krishnamurthy, H. R., Waghmare, U. V., et al. (2009). Synthesis, structure, and properties of boron- and nitrogen-doped graphene. Adv. Mater. 21, 4726–4730. doi: 10.1002/adma.200901285
Rafique, M., Shuai, Y., Ahmed, I., Shaikh, R., and Tunio, M. A. (2019). Tailoring electronic and optical parameters of bilayer graphene through boron and nitrogen atom co-substitution; an ab-initio study. Appl. Sur. Sci. 480, 463–471. doi: 10.1016/j.apsusc.2019.02.240
Sanjeeva Rao, K., Senthilnathan, J., Ting, J. M., and Yoshimura, M. (2014). Continuous production of nitrogen-functionalized graphene nanosheets for catalysis applications. Nanoscale 6, 12758–12768. doi: 10.1039/C4NR02824D
Sarycheva, A., Makaryan, T., Maleski, K., Satheeshkumar, E., Melikyan, A., Minassian, H., et al. (2017). Two-dimensional titanium carbide (MXene) as surface-enhanced raman scattering substrate. J. Phy. Chem. C 121, 19983–19988. doi: 10.1021/acs.jpcc.7b08180
Satheeshkumar, E., Bandyopadhyay, A., Sreedhara, M. B., Pati, S. K., Rao, C. N. R., and Yoshimura, M. (2016a). One-step simultaneous exfoliation and covalent functionalization of MoS2 by amino acid induced solution processes. ChemNanoMat 3, 172–177. doi: 10.1002/cnma.201600363
Satheeshkumar, E., Makaryan, T., Melikyan, A., Minassian, H., Gogotsi, Y., and Yoshimura, M. (2016b). One-step solution processing of Ag, Au and Pd@MXene hybrids for SERS. Sci. Rep. 6:32049. doi: 10.1038/srep32049
Senthilnathan, J., Liu, Y. F., Rao, K. S., and Yoshimura, M. (2014a). Submerged liquid plasma for the synchronized reduction and functionalization of graphene oxide. Sci. Rep. 4:4395. doi: 10.1038/srep04395
Senthilnathan, J., Rao, K. S., Lin, W.-H., Ting, J.-M., and Yoshimura, M. (2015). Formation of reusable Au-acetonitrile polymers and N-doped graphene catalyst under UV light via submerged liquid plasma process. J. Mater. Chem. A 3, 3035–3043. doi: 10.1039/C4TA05586A
Senthilnathan, J., Rao, K. S., and Yoshimura, M. (2014b). Submerged liquid plasma – low energy synthesis of nitrogen-doped graphene for electrochemical applications. J. Mater. Chem. A 2, 3332–3337. doi: 10.1039/C3TA14946C
Senthilnathan, J., Sanjeeva Rao, K., Lin, W.-H., Liao, J.-D., and Yoshimura, M. (2014c). Low energy synthesis of nitrogen functionalized graphene/nanoclay hybrid via submerged liquid plasma approach. Carbon 78, 446–454. doi: 10.1016/j.carbon.2014.07.025
Senthilnathan, J., Weng, C. C., Liao, J. D., and Yoshimura, M. (2013). Submerged liquid plasma for the synthesis of unconventional nitrogen polymers. Sci. Rep. 3:2414. doi: 10.1038/srep02414
Sokolov, J., Jona, F., and Marcus, P. M. (1984). Trends in metal surface relaxation. Solid State Commun. 49, 307–312. doi: 10.1016/0038-1098(84)90575-1
Usachov, D., Vilkov, O., Grüneis, A., Haberer, D., Fedorov, A., Adamchuk, V. K., et al. (2011). Nitrogen-doped graphene: efficient growth, structure, and electronic properties. Nano Lett. 11, 5401–5407. doi: 10.1021/nl2031037
Keywords: graphene, exfoliation, boron—containing compounds, nitrogen, solution processing
Citation: Elumalai S, Su C-Y and Yoshimura M (2019) Scalable One-Pot Synthesis of Nitrogen and Boron Co-doped Few Layered Graphene by Submerged Liquid Plasma Exfoliation. Front. Mater. 6:216. doi: 10.3389/fmats.2019.00216
Received: 22 May 2019; Accepted: 21 August 2019;
Published: 06 September 2019.
Edited by:
Wee-Jun Ong, Xiamen University, MalaysiaReviewed by:
Alberto Ansaldo, Istituto Italiano di Tecnologia, ItalyYong Shuai, Harbin Institute of Technology, China
Copyright © 2019 Elumalai, Su and Yoshimura. This is an open-access article distributed under the terms of the Creative Commons Attribution License (CC BY). The use, distribution or reproduction in other forums is permitted, provided the original author(s) and the copyright owner(s) are credited and that the original publication in this journal is cited, in accordance with accepted academic practice. No use, distribution or reproduction is permitted which does not comply with these terms.
*Correspondence: Satheeshkumar Elumalai, analyticalsathi@gmail.com; Masahiro Yoshimura, yoshimur@mail.ncku.edu.tw