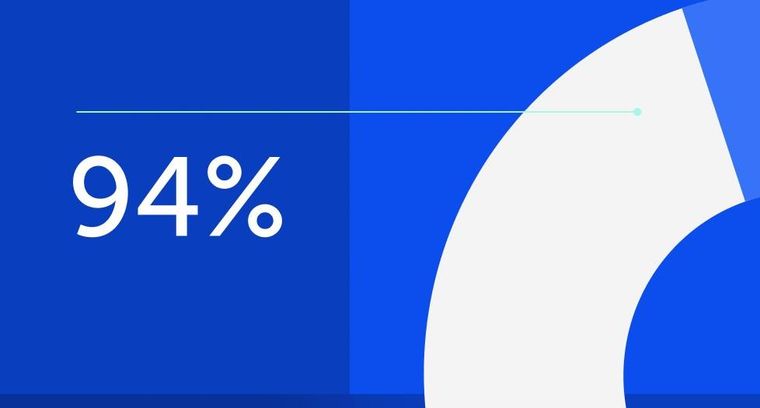
94% of researchers rate our articles as excellent or good
Learn more about the work of our research integrity team to safeguard the quality of each article we publish.
Find out more
REVIEW article
Front. Mater., 18 June 2019
Sec. Polymeric and Composite Materials
Volume 6 - 2019 | https://doi.org/10.3389/fmats.2019.00137
This review article provides an overview of the research and applications of soft self-healing polymers and their nanocomposites. A number of concepts based on physical and chemical interactions have been explored to create dynamic and reversible gel and elastomer networks, each strategy presenting its own advantages and drawbacks. Physical interactions include supramolecular interactions, ionic bonding, hydrophobic interactions, and multiple intermolecular interactions. Such networks do not require external stimulus and are capable of multiple self-healing cycles. They are generally characterized by a rapid but limited healing efficiency. The addition of nanofillers enhances the mechanical strength of the soft networks as in conventional gels and elastomers, and do not compromise the network healing dynamics. In certain cases, nanofillers moreover trigger the healing process through e.g., multi-complexation processes between each component. Chemical interactions include Diels-Alder reactions and disulphide, imine, boronate ester, or acylhydrazones bonding, and are usually triggered with an external stimulus. The resulting healing is efficient, leading to good mechanical properties, but is generally slow at ambient temperatures, and dynamic chemical interactions are only reversible at higher temperatures. Conductive nanofillers were reported to speed up the healing process in such systems owing to their energy absorption properties. The challenges with nanofillers remain their functionalization and dispersion within the self-healing formulations. Soft self-healing gels and nanocomposites find applications in engineering such as coatings, sensors, actuators and soft robotics, and in the bio-medical field, including drug delivery, adhesives, tissue engineering and wound healing.
Material's research has traditionally focused on the design of new materials that are intrinsically resistant to chemical and mechanical damage; however, material properties degrade with time, and during service owing to unavoidable impacts and stresses inducing cracks. Since few decades, nature has inspired scientists to develop new chemistries and processes to restore material properties after mechanical and functional damage has occurred. Small wounds can generally be healed in biological systems (Harrington et al., 2016), but large body parts such as limbs can also be regenerated, such as in the axolotl, a type of Mexican salamander (Kragl et al., 2009). This inspiration has led to the development of new materials, in particular polymers having self-healing properties (Binder, 2013; Herbst et al., 2013; Yang and Urban, 2013; Campanella et al., 2018; Dahlke et al., 2018; Diba et al., 2018; Ding F. et al., 2018; Kim et al., 2018; Wang et al., 2018). Compared to conventional polymers, self-healing polymers demonstrate the ability to reform broken bonds via a physical process or a symbiosis of physical and chemical processes. The design of materials having self-healing abilities requires a multidisciplinary process including mechanics, process dynamics, and chemistry. Several realistic strategies have been formulated over the past two decades (Chen et al., 2002; White et al., 2002; Gould, 2003), including elastomers (Sordo et al., 2015), coatings (Samadzadeh et al., 2010; Bailey et al., 2015; Zhang et al., 2018), and composite materials (Cho et al., 2009; Cohades et al., 2018), which not long ago appeared a creation of vivid imagination. A number of review articles comparing different healing concepts in bulk as well as composite materials is available (Caruso et al., 2009; Blaiszik et al., 2010; Binder, 2013; Herbst et al., 2013; Yang and Urban, 2013; Bekas et al., 2016; Cohades et al., 2018).
In practice, two interactive effects are observed in self-healing systems. First, in order to close the damage cavity a certain physical flow of a “mobile phase” has to occur within the network structure (Yang et al., 2015b). Second, in order to ensure the restoration of the functional and mechanical integrity, the polymer network needs to be rebuilt. One of the earliest self-healing techniques relies on an extrinsic capsule/vascule based concept, whereby a microencapsuled healing agent is incorporated within the polymer. The stress-induced rupture promotes monomer delivery to the cracks, in turn inducing an appropriate polymerization reaction between the components, often involving a catalyst (White et al., 2002; Brown et al., 2005; Wilson et al., 2008; Guadagno et al., 2010; Coope et al., 2011). However, capsules act as voids in the materials, the encapsulated agent is consumed after a first damage event, thus reducing the ability to heal multiple times, and the stability of catalyst or healing agent is often limited (Brown et al., 2004; Blaiszik et al., 2008; Jin et al., 2013).
The present review focuses on the alternative intrinsic self-healing approach, where the polymer itself repairs after damage by the utilization of dynamic/reversible interactions that can be broken and renewed under different physicochemical conditions. Intrinsic self-healing presents several advantages in comparison to extrinsic self-healing due to multiple times of healing at the same location without help of any healing agent and catalyst. Two main strategies, based on physical interactions and chemical interactions have been implemented to create intrinsic self-healing polymers, as sketched in Figure 1. Reversible bonding can be either non-covalent, including host-guest interactions, π-π stacking, hydrogen bonds, ionic and hydrophobic interactions, metal coordination, or covalent, such as imine bonds, disulphide and diselenide bridging, Diels-Alder reaction, reversible boronate ester, and acylhydrazones bonds. As detailed in the following, the reversibility of covalent bonds between different functional groups can be induced by external stimuli, and can be influenced by a change in temperature, pH, catalytic activity, irradiation, moisture, etc. (Garcia, 2014).
Figure 1. Various strategies used to synthesize physical and chemical interaction based soft self-healing materials.
Focus of the review is more specifically on soft materials (gels, elastomers) and their nanocomposites, that have gained strong interest in recent years. Application examples include actuators, sensors (Wang et al., 2014; Chortos et al., 2017; Han et al., 2017d), flexible conductors (Wu and Chen, 2016b), tissue engineering scaffolds, drug delivery systems (Bowden et al., 1998; Geckil et al., 2010; Vig et al., 2013). Conventional soft materials have low stiffness and ultimate strength of a few MPa or tenths of MPa in the case of tough hydrogels, which greatly limits their application. The addition of nanofillers is thus highly advantageous not only for enhancing their mechanical properties by introducing higher stiffness reinforcement (Tee et al., 2012) but also to improve their functional (for example thermal or electrical conduction) and biological (Annabi et al., 2014; Lau and Kiick, 2015) properties. Traditional nanofillers include carbon nanotubes (CNTs) (Gopal et al., 2010; Qi et al., 2013), graphene (Potts et al., 2011; Huang et al., 2012; Shaygan Nia et al., 2015), ceramic oxides (Mera et al., 2013), nano silica (Engel and Kickelbick, 2013), and nanocellulose (Galland et al., 2014). As an example, the tensile strength of polyvinyl alcohol was increased up to 60 times with the addition of cellulose nano-whiskers (Ding Q. et al., 2018). Nanofillers have also been exploited to promote self-healing properties. For instance, conducting nanofillers with high thermal conductivity (graphene, CNT, metals) are used as nanoscale heaters to accelerate the self-healing mechanisms within the polymer matrix (Bai and Shi, 2017; Dai et al., 2018). In fact, nanocomposite materials feature very large specific interfacial areas, usually above 100 m2/g. Careful design and control of the polymer-nanofiller interfacial bond dynamics (including through functionalization of the particles and/or the polymer), which remain challenging to optimize nanofiller dispersion and process rheology, represents a further option toward intrinsic self-healing.
Keeping in mind the properties and applications of soft materials, this article reviews the strategies for synthesizing self-healing soft materials based on the concept of constitutional dynamic chemistry (intrinsic self-healing) relying on physical and chemical interactions. For each case, the effect of nanofillers addition as a tool to enhance the self-healing properties of the soft materials is discussed in details. We also illustrate the emerging applications of self-healing soft nanocomposites by highlighting their usage in various fields.
This section reviews the strategies for the preparation of physical self-healing gels and elastomers based upon dynamic networks of non-covalent interactions, including supramolecular interactions, ionic bonding, hydrophobic interactions, multiple intermolecular interactions, and nanocomposite interactions. An overview of the reaction types, which are discussed herein, is provided in Table 1. Please note that it is difficult to compare the various materials as the methods to assess their mechanical properties and healing efficiency are different. Relying on transient bonds, physical interaction based materials are capable of multiple self-healing cycles, where the mobility of the polymer chains and the density of the network influences the life time of the reversible bonds (Binder and Zirbs, 2007; de Greef and Meijer, 2008). For example, poly(ethylene glycol) based hydrogels were synthesized while functionalizing the prepolymer with quadruple hydrogen bond (UPy groups); due to their hydrophilic nature PEG-moieties can accommodate water, whereas the UPy groups give strength and elasticity while assembling into hydrogen-bonded arrays (Van Gemert et al., 2012). A heart shaped object demonstrated a shape persistent and self-healing behavior when cut into two pieces, which was mended by simply pressing both halves together (Figure 2).
Figure 2. (a) UPy-containing polymer. (b) Supramolecular hydrogel made of PEG-UPy polymer 15 wt% in water (Van Gemert et al., 2012). Reproduced with the permission of 2012 WILEY-VCH Verlag GmbH & Co. KGaA Weinheim, SupraPolix BV, 2010.
In another example, an ionic self-healing mechanism involves charged polymer chains cross-linked with oppositely charged ions or polymer chains. Relative to organogels, ion gels exhibit high chemical stability and ionic conductivity, and are thus advantageous for a wide range of applications from soft actuators (Imaizumi et al., 2012) to electrolytes for lithium ion batteries (Kitazawa et al., 2018). A self-healing and adhesive ionic gel was prepared by the mixing of polycation poly(allylamine hydrochloride) (PAH) with pyrophosphate (PPi) and tripolyphosphate (TPP) (Huang et al., 2014). Due to highly dense ionic crosslinking, a high storage modulus was observed (G'∞ ≈ 4 × 105 Pa). Due to stabilization and functional groups rearrangement, the self-healing performance of physically crosslinked hydrogels decreases when increasing the time to place back together the cut surfaces (Herbst et al., 2013). A time independent single-component zwitterionic hydrogel with a capability for spontaneous healing under physiological conditions has been reported (Bai et al., 2014), where carboxylbetaine acrylamide (AAZ) was physically crosslinked via electrostatic interactions among zwitterionic moieties, resulting in a time-independent healing behavior. In addition, their self-healing process does not require any external stimulus; however, a decrease in self-healing was observed with increase in crosslinking density (Bai et al., 2014).
Electrostatic interaction based physically cross-linked zwitterionic hydrogels were developed using carboxybetaine acrylamide, 7-acrylamidoheptanoate acid and hydroxyhexylacrylamide (Bai et al., 2014). Interestingly the reported hydrogel demonstrates a time-independent healing behavior, in that the separation time of cut fragments does not compromise healing efficiency. Wei et al. reported electrostatic interactions based self-healable supramolecular luminescent hydrogels from ABA triblock copolymers [Poly(2-(2-guanidinoethoxy) ethyl methacrylate)-b-poly(ethylene oxide)-b-poly(2-(2-guanidinoethoxy) ethyl methacrylate)] and polyoxometalates (Wei et al., 2014), where the mechanical strength of the hydrogel can be simply tuned by altering the ionic strength, concentrations, and copolymer composition. A reversible electrostatic interaction promoted ionic self-healing mechanism was emphasized by Varghese et al., where a charged polymer chain crosslinks with an oppositely charged polymer chain or with oppositely charged ions. However, based on experimental conditions the materials also illustrate the hindrance in self-healing due to electrostatic repulsion when the polymeric ions were deprotonated (Phadke et al., 2012). Recent years have observed a growing attention in the development of nature inspired dopa-modified polymeric structures, in particular to explore the biomimetic adhesives for tissue engineering (Barrett et al., 2013; Kim et al., 2014). Catechol induced polyelectrolyte coacervate bioadhesive has been developed using a poly(acrylic acid) backbone decorated with catechol appendants in presence of weak divalent cross-linker Zn2+ (Wang et al., 2015), where negative charged carboxylic groups from poly(acrylic acid) electrostatically interact with zinc chelated mono-catechol group, thus leading to a quick generation of the dense coacervates.
A double-network (DN) based self-healing material with improved mechanical properties has been reported, where a symbiosis of covalent and non-covalent crosslinking network had been employed (Gong, 2010). However, as the chemically covalent-cross-linked network cannot reversibly recover to its original state, the DN soft materials exhibit poor self-healing efficiency (Chen et al., 2015, 2016). Thus, a multiple non-covalent cross-linked network could be more advantageous for designing self-healing materials with high mechanical properties. A double network based poly(N-acryloyl glycinamide-co-N-benzyl acrylamide) containing a triple amide in one side group was reported by Feng et al. The triple amide based soft material demonstrated a good shape memory ability, high mechanical strength (1.1 MPa) and about 95% self-healing efficiency at room temperature (Feng et al., 2018).
Most of the widely explored soft materials are also now often combined with nanosized inorganic components to form nanocomposites, as summarized in Table 2. The addition of stiff nanofillers is strategic not only for enhancing the mechanical properties of the soft matrix (Tee et al., 2012) but also to improve their self-healing properties (Bai and Shi, 2017; Dai et al., 2018). Zhong et al. (2015a) proposed a tough and highly stretchable self-healing hydrogel based on silica nanoparticles, ferric ions and poly(acrylic acid) (PAA). The hydrogel demonstrated excellent toughness (tensile strength 860 kPa, elongation at break ~2,300%). It was suggested that the stretchability and toughness arose from the reversible cross-linking interactions between polymer chains, helpful for energy dissipation through stress triggered dynamic process. Exfoliated sodium montmorillonite based self-healing hydrogel was formed by in situ polymerization of acrylamide monomers (Gao et al., 2015). These hydrogels demonstrated good stretchability with a high fracture toughness (10.1 MJ m−3) and fracture strain up to 11,800%, however, exhibited slow recovery of mechanical properties (RT, 5 days) and required multiple dry/re-swell handlings.
Table 2. Physical interaction based self-healing mechanism, healing efficiency, recovery, mechanical/electrical properties of soft nanocomposites.
A host-guest interaction based healable, stable, and recoverable nanocomposite hydrogel was developed using a three-component architecture consisting of poly(vinyl alcohol) (PVA), cucurbit[8]uril (CB) and cellulose nanocrystals (McKee et al., 2014). These nanocomposite hydrogels exhibit significant mechanical strength and stiffness with a storage modulus of 10 MPa. Immediate self-recovery was shown via step-strain measurements at RT and rapid self-healing abilities were observed (within 30 s, RT). Also the hydrogel was able to rapidly self-heal (within 10 s) once re-joined even after 4 months.
In another example, self-recovering hydrogels have been formed based on a viscoelastic polyvinyl alcohol (PVA)-borax (PB) gel matrix and nanostructured CNFs–PPy (cellulose nanofibrils-polypyrrole) complexes that synergizes the bio-template role of CNFs and the conductive nature of PPy. The CNF-PPy complexes not only tangle with PVA chains though hydrogen bonds, but also form reversibly cross-linked complexes with borate ions (Ding Q. et al., 2018). The multi-complexation between each component leads to the formation of a hierarchical three-dimensional network (Figure 3). Owing to a combined reinforcing and conductive network inside the hydrogel (2 wt.% CNFs-PPy), it demonstrated high storage modulus (~0.1 MPa) and nominal compression stress (~22 MPa), about 60 and 2,240 times higher than those of pure PVA hydrogel, respectively.
Figure 3. (a) Schematic illustration of preparation and synthesis process of CNF–PPy/PB hydrogels. (b) CNF–PPy/PB hydrogels molded into various shapes. (c) Formation mechanism of the integrated 3D network within CNF–PPy/PB hydrogels. (d) Multi-complexation between PVA, CNF–PPy, and borax. (e) Schematic illustration of in situ self-healing and dynamic reversible cross-links of hydrogels (Ding Q. et al., 2018). Reproduced with the permission of 2018 American Chemical Society.
Mussel inspired, metal coordination bonds based on reversible dynamics have been demonstrated to function as dynamic cohesive cross-linkers in bulk polymer materials (Amstad et al., 2009). Niels-Andersen et al. incorporated iron oxide nanoparticles (Fe3O4 NP) in catechol modified polymer networks to obtain hydrogel cross-linked via reversible metal-coordination bonds at Fe3O4 NP surface (Li et al., 2016b). The structure provides solid-like yet reversible hydrogel mechanics. Zirconium hydroxide has been utilized as nanofiller in a poly(acrylamide) to produce hydrogen bonding interaction based self-healing gel. The gels exhibited good mechanical properties with 114 KPa-404 KPa tensile strength and 284–723% elongation at break (Jiang et al., 2017). A metal-ligand network based highly stretchable self-healing elastomer was developed consisting of 2,6-pyridinedicarboxamide ligands that coordinate to Fe(III) centers through weak carboxamido-iron ones, a strong iron-pyridyl one. The material demonstrated self-healing at a temperature as low as −20 °C (Figure 4) (Li et al., 2016a).
Figure 4. (A,B) Schematic structure of material design and single-molecule force spectroscopy characterization. (C) Stress–strain curves of a film healed at room temperature (r.t.) for different lengths of time show an increase of the stretching ability when the film is allowed to heal for longer. (D) The stress–strain curves of the film healed at −20 and 0°C for 72 h, and the stress–strain curves of the film healed at room temperature for 48 h after surface aging for 24 h (Li et al., 2016a). Reproduced with the permission of 2016 American Chemical Society.
Carbon nanomaterials (CNT, graphene), which show good compatibility with polymers due to their large π-conjugated system, have been widely used to produce mechanically enhanced composite materials. Such nanofillers moreover improve the chemical stability, the electrical and thermal conductivity (Balandin et al., 2008), and the microwave and infrared (IR) absorbing capacity of polymers (Sui et al., 2011). CNT based supramolecular hydrogels directed by hierarchical hydrogen bond (a mixture of strong and weak bond) interaction has been reported (Du et al., 2014b), where the gel was obtained by mixing multiwall carbon nanotubes (MWCNT) with polyethylene polyamine (PAA). The properties of the gel can be easily tailored by controlling the ratio of strong and weak hydrogen bonds. The autonomous healing gel demonstrated a multiple responsiveness (NIR light, pH, and thermal) including temperature dependent reversible adhesion behavior. A host-guest interaction based self-healable conducting elastomer consisting of singe-walled carbon nanotubes (SWCNT) and poly(2-hydroxyethyl methacrylate) (PHEMA) was reported (Guo et al., 2015). The elastomers sustain a high conductivity up to 180% stretching, however, under large strain the conductivity decreases. This might be due to partial breakdown the SWCNT network that leads to a decrease in the total number of conduction paths. Wang et al. have developed a rapid room temperature self-healing elastic nanocomposite material by combining the hydrogen-bonded polymer and graphene oxide as a cross-linker (Wang et al., 2013), where hydrogen bonding network in polymer chain provides self-healing capability, while GO enabled good mechanical properties.
A symbiosis of shape memory and self-healing was also achieved for graphene oxide based polyurethane (GO-PU) nanocomposites (Thakur et al., 2015), where fracture of the composite was effectively healed by exposure to direct sunlight and MW. The nanocomposites were effectively healed within 30–50 s under low MW power (360 W) and within 5–7 min under direct sunlight. During the healing process, GO absorbed energy from the stimulus, and then transferred this energy to the HPU matrix, where the soft segment of the HPU melted (low Tm ~50°C). Thus, the crack could repair with a higher mobility of the soft segment of HPU. To achieve combined attributes of shape memory, self-healing and self-cleaning properties, hyperbranched polyurethane (HPU)-TiO2/reduced graphene oxide (TiO2/RGO) nanocomposites were reported (Thakur and Karak, 2015), where the fabricated nanocomposite exhibited composition and dose-dependent mechanical properties with excellent shape recovery ratio (91–95%) as well as shape recovery rate (1–3 min) under exposure to sunlight. The presence of a high amount of RGO (0.5–1 wt.%) in the nanocomposite helps in rapid and efficient healing, whereas a high amount of TiO2 nanoparticles (5–10 wt.%) aids in achieving good self-cleaning properties.
This section covers the strategies for the preparation of chemical self-healing soft materials based upon dynamic networks of covalent interactions, including Diels-Alder reactions, disulphide, imine bond, boronate ester bond, acylhydrazones, and nanocomposite interactions. An overview of the reaction types, which are discussed herein, is provided in Table 3. Depending on the conditions, dynamic permanent covalent bonds can break and reform. For instance, a disulfide and acylhrazone bonds based self-healing network was developed, where in PEO based hydrogel, disulfide exchange reaction occurs at basic pH, whereas acidic pH is helpful for acylhydrazone exchange reactions (Deng et al., 2012). The material did not show self-healing at neutral pH as the covalent bonds were not dynamic. In general, the broadly investigated temperature triggered reversible diene and dienophile based [4+2] cycloaddition and retro Diels-Alder (DA) mechanism make good candidates for healing networks.
A comprehensive study by Garcia et al. demonstrated the effect of reversible DA bonds in covalently cross-linked networks (Garcia et al., 2014). The study shows that due to retro-DA reaction at high temperature, an increased mobility in the network leads to the self-healing of the system. However, the required high temperature for DA/retro DA damages the properties of the parent material. A switchable “on” and “off” based photo-switchable DA dynamic network was developed by Hecht et al., where the incorporation of furan crosslinker allows reversible (de- and re-crosslinking) in short and mobile maleimide-substituted poly(lauryl methacrylate) chains (Figure 5) (Fuhrmann et al., 2016). In general, the common dynamic chemical interactions based self-healing are reversible upon temperature usage, thus limiting their application areas.
Figure 5. Schematic representation of (A) photo-switchable, dynamically crosslinked polymer network and (B) the chemical structures of the crosslinkers, indicating the switchable reactivity for the reversible DA reaction and healability of the network (Fuhrmann et al., 2016). Reproduced with the permission of 2016 Springer Nature, United Kingdom.
The incorporation of nanofillers with this type of self-healing mechanism is also widely described, as highlighted in Table 4. In particular conductive nanofillers are used to trigger and catalyze the self-repair performance, owing to their superior heat absorption properties and resulting local heating under sunlight and microwave radiation. Indeed, due to their high photo-thermal conversion, conductive nanofillers enable to increase the temperature up to 200°C in a very short time (Kohlmeyer et al., 2012). Shi et al. developed a dynamic reversible cross-linked organic-inorganic network via DA reaction between poly(styrene-butadiene-styrene) and CNT. The material demonstrated DA based self-healing with complete recovery of the mechanical properties in a time as short as 10 s due to the photo-thermal effect of CNT under laser irradiation (Figure 6) (Bai and Shi, 2017) whereas no self-healing occurred in absence of CNT.
Table 4. Chemical interaction based self-healing mechanism, healing efficiency, recovery, mechanical/electrical properties of soft nanocomposites.
Figure 6. (A) Healing Process of the Sample; (B) Reaction Occurring during the Healing Process (Bai and Shi, 2017). Reproduced with the permission of 2017 American Chemical Society.
Self-healing soft materials find applications in engineering and bio-medical fields. The major uses of self-healing materials so far are in surface coating (Canadell et al., 2011; Yoon et al., 2012; Yang et al., 2015a), drug delivery (Huebsch et al., 2014; Liu et al., 2016; Wang et al., 2016, 2017a; Xing et al., 2016; Xia et al., 2017; Yavvari et al., 2017; Hong et al., 2018), tissue engineering (Dankers et al., 2012; Bastings et al., 2014; Gaffey et al., 2015; Rodell et al., 2015; Loebel et al., 2017), wound healing (Gaharwar et al., 2014; Han et al., 2016; Zhao et al., 2017b; Zhu et al., 2017; Li et al., 2018c), and soft robotics (Shi et al., 2015; Darabi et al., 2017; Han et al., 2017b; Liu et al., 2018b). Herein, some applications of soft self-healing nanocomposites in biomedical fields including drug delivery tissue adhesive, as well as in industrial fields including actuators, sensors, and coatings are gathered (Table 5).
Table 5. Demonstrated applications for soft self-healing nanocomposites and associated healing features.
A large variety of concepts based on physical and chemical interactions have been explored to design soft self-healing materials, each strategy presenting its own advantages and drawbacks. In general, materials based on chemical interactions require an ad-hoc external stimulus to trigger the healing reactions, whereas those based on physical interactions enjoy an autonomous and fast repair. As a matter of fact and as highlighted in many reviews, no standard measurement technique is available to quantify self-healing, so it is difficult to compare the merits of each material in terms of healing efficiency as easily as it is for their strength or toughness. According to the summarized data, soft self-healing materials based on ionic bonding and hydrogen interactions demonstrate a fast but moderate healing efficiency, whereas a slow but high healing efficiency is observed in case of supramolecular bonding. When considering applications, it is important to keep in mind that mechanical robustness and self-healing properties are often opposite, except in some cases where chemical interactions triggered with external stimulus can be relied upon. However, even if there is no straightforward technique to compare the mechanical properties of soft materials, generally, the modulus followed the usual trend, with covalent bonding > ionic bonding > hydrophobic bonding > supramolecular interactions. The addition of nanofillers enables enhancing the mechanical and other physical properties such as thermal and electrical conductivity (Ding Q. et al., 2018). The addition of conducting nanofiller was found more effective for not only enhancing the conductivity but also mechanical properties of the soft materials. As for self-healing, conducting nanofillers are used as triggers/catalysts for photo-thermal activation and resulting enhanced self-repair performance. Laser-induced photo-thermal activation was for instance found to speed up the healing process, but this also led to the partial breakdown of the conductive network, thereby preventing multiple healing. Efficient healing combined with desirable mechanical properties seems at this stage to be reached using a chemical interaction-based concept. Certainly, the selective functionalization and dispersion of nanofillers is quite challenging, and perhaps that is the reason why most of the soft self-healing nanocomposites are nowadays based on physical interactions, rather than on chemical interactions.
RS and BK performed the literature search and tables as well as first drafts. YL, SR, and VM organized the outline and wrote the text. All authors have contributed to the review article.
This work is funded by the Swiss National Foundation Scientific Exchange grant, which financed a visit of SR at EPFL.
The authors declare that the research was conducted in the absence of any commercial or financial relationships that could be construed as a potential conflict of interest.
We gratefully acknowledge the financial support from the Swiss National Foundation Scientific Exchange Grant Switzerland (IZSEZO_178681/1).
Akiyama, T., Ushio, A., Itoh, Y., Kawaguchi, Y., Matsumoto, K., and Jikei, M. (2017). Synthesis and healing properties of poly (arylether sulfone)–poly (alkylthioether) multiblock arylether sulfone–poly (alkylthioether) multiblock copolymers. J. Polym. Sci. Part A Polym. Chem. 55, 3545–3553. doi: 10.1002/pola.28736
Amaral, A. J. R., Emamzadeh, M., and Pasparakis, G. (2018). Transiently malleable multi-healable hydrogel nanocomposites based on responsive boronic acid copolymers. Polym. Chem. 9, 525–537. doi: 10.1039/C7PY01202K
Amstad, E., Gillich, T., Bilecka, I., Textor, M., and Reimhult, E. (2009). Ultrastable iron oxide nanoparticle colloidal suspensions using dispersants with catechol-derived anchor groups. Nano Lett. 9, 4042–4048. doi: 10.1021/nl902212q
Annabi, N., Tamayol, A., Uquillas, J. A., Akbari, M., Bertassoni, L. E., Cha, C., et al. (2014). 25th anniversary article: rational design and applications of hydrogels in regenerative medicine. Adv. Mater. 26, 85–124. doi: 10.1002/adma.201303233
Bai, J., He, Q., Shi, Z., Tian, M., Xu, H., Ma, X., et al. (2017). Self-assembled elastomer nanocomposites utilizing C60and poly(styrene-b-butadiene-b-styrene) via thermally reversible Diels-Alder reaction with self-healing and remolding abilities. Polymer 116, 268–277. doi: 10.1016/j.polymer.2017.03.080
Bai, J., Li, H., Shi, Z., and Yin, J. (2015). An eco-friendly scheme for the cross-linked polybutadiene elastomer via thiol-ene and Diels-Alder click chemistry. Macromolecules 48, 3539–3546. doi: 10.1021/acs.macromol.5b00389
Bai, J., and Shi, Z. (2017). Dynamically cross-linked elastomer hybrids with light-induced rapid and efficient self-healing ability and reprogrammable shape memory behavior. ACS Appl. Mater. Interfaces 9, 27213–27222. doi: 10.1021/acsami.7b06407
Bai, T., Liu, S., Sun, F., Sinclair, A., Zhang, L., Shao, Q., et al. (2014). Biomaterials Zwitterionic fusion in hydrogels and spontaneous and time-independent self-healing under physiological conditions. Biomaterials 35, 3926–3933. doi: 10.1016/j.biomaterials.2014.01.077
Bailey, B. M., Leterrier, Y., Garcia, S. J., Van Der Zwaag, S., and Michaud, V. (2015). Electrically conductive self-healing polymer composite coatings. Prog. Org. Coatings 85, 189–198. doi: 10.1016/j.porgcoat.2015.04.013
Balandin, A. A., Ghosh, S., Bao, W., Calizo, I., Teweldebrhan, D., Miao, F., et al. (2008). Superior thermal conductivity of single-layer graphene. Nano Lett. 8, 902–907. doi: 10.1021/nl0731872
Banerjee, S. L., Bhattacharya, K., Samanta, S., and Singha, N. K. (2018). Self-healable antifouling zwitterionic hydrogel based on synergistic phototriggered dynamic disulfide metathesis reaction and ionic interaction. ACS Appl. Mater. Interfaces 10, 27391–27406. doi: 10.1021/acsami.8b10446
Barrett, D. G., Fullenkamp, D. E., He, L., Holten-Andersen, N., Lee, K. Y. C., and Messersmith, P. B. (2013). pH-based regulation of hydrogel mechanical properties through mussel-inspired chemistry and processing. Adv. Funct. Mater. 23, 1111–1119. doi: 10.1002/adfm.201201922
Bastings, M. M. C., Koudstaal, S., Kieltyka, R. E., Nakano, Y., Pape, A. C. H., Feyen, D. A. M., et al. (2014). A fast pH-switchable and self-healing supramolecular hydrogel carrier for guided, local catheter injection in the infarcted myocardium. Adv. Healthc. Mater. 3, 70–78. doi: 10.1002/adhm.201300076
Bekas, D. G., Tsirka, K., Baltzis, D., and Paipetis, A. S. (2016). Self-healing materials: a review of advances in materials, evaluation, characterization and monitoring techniques. Compos. Part B Eng. 87, 92–119. doi: 10.1016/j.compositesb.2015.09.057
Binder, W. H. (2013). Self-Healing Polymers: From Principles to Applications. Weinheim: WILEY-VCH Verlag GmbH & Co. KGaA.
Binder, W. H., and Zirbs, R. (2007). “Supramolecular polymers and networkswith hydrogen bonds in the main- and side-chain,” in Hydrogen Bonded Polymers, ed W. Binder (Berlin: Springer, 1–78.
Blaiszik, B. J., Kramer, S. L. B., Olugebefola, S. C., Moore, J. S., Sottos, N. R., and White, S. R. (2010). Self-healing polymers and composites. Annu. Rev. Mater. Res. 40, 179–211. doi: 10.1146/annurev-matsci-070909-104532
Blaiszik, B. J., Sottos, N. R., and White, S. R. (2008). Nanocapsules for self-healing materials. Compos. Sci. Technol. 68, 978–986. doi: 10.1016/j.compscitech.2007.07.021
Bowden, N., Brittain, S., Evans, A. G., Hutchinson, J. W., and Whitesides, G. M. (1998). Spontaneous formationof ordered structures in thin films of metals supported on an elastomeric polymer. Nature 393, 146–149. doi: 10.1038/30193
Brown, E. N., White, S. R., and Sottos, N. R. (2004). Microcapsule induced toughening in a self-healing polymer composite. J. Mater. Sci. 39, 1703–1710. doi: 10.1023/B:JMSC.0000016173.73733.dc
Brown, E. N., White, S. R., and Sottos, N. R. (2005). Retardation and repair of fatigue cracks in a microcapsule toughened epoxy composite - Part II: In situ self-healing. Compos. Sci. Technol. 65, 2474–2480. doi: 10.1016/j.compscitech.2005.04.053
Cai, G., Wang, J., Qian, K., Chen, J., Li, S., and Lee, P. S. (2017). Extremely stretchable strain sensors based on conductive self-healing dynamic cross-links hydrogels for human-motion detection. Adv. Sci. 4:1600190. doi: 10.1002/advs.201600190
Campanella, A., Döhler, D., and Binder, W. H. (2018). Self-healing in supramolecular polymers. Macromol. Rapid Commun. 39:1700739. doi: 10.1002/marc.201700739
Canadell, J., Goossens, H., and Klumperman, B. (2011). Self-healing materials based on disulfide links. Macromolecules 44, 2536–2541. doi: 10.1021/ma2001492
Caruso, M. M., Davis, D. A., Shen, Q., Odom, S. A., Sottos, N. R., White, S. R., et al. (2009). Mechanically-induced chemical changes in polymeric materials. Chem. Rev. 109, 5755–5798. doi: 10.1021/cr9001353
Chang, R., An, H., Li, X., Zhou, R., Qin, J., Tian, Y., et al. (2017). Self-healable polymer gels with multi-responsiveness of gel–sol–gel transition and degradability. Polym. Chem. 8, 1263–1271. doi: 10.1039/C6PY02122K
Chao, A., Negulescu, I., and Zhang, D. (2016). Dynamic covalent polymer networks based on degenerative imine bond exchange: tuning the malleability and self-healing properties by solvent. Macromolecules 49, 6277–6284. doi: 10.1021/acs.macromol.6b01443
Chen, J., Zhang, Y., Hu, C., Deng, Y., Shi, L., and Ran, R. (2018a). Robust and self-healing hydrophobic association hydrogels using poly(styrene-co-acrylonitrile) macromolecule microspheres as cross-linking centers. Chem. Select 3, 418–427. doi: 10.1002/slct.201702582
Chen, P., Wang, X., Wang, G. Y., Duo, Y. R., Zhang, X. Y., Hu, X. H., et al. (2014). Double network self-healing graphene hydrogel by two step method for anticancer drug delivery. Mater. Technol. 29, 210–213. doi: 10.1179/1753555714Y.0000000128
Chen, Q., Chen, H., Zhu, L., and Zheng, J. (2015). Fundamentals of double network hydrogels. J. Mater. Chem. B 3, 3654–3676. doi: 10.1039/C5TB00123D
Chen, Q., Chen, H., Zhu, L., and Zheng, J. (2016). Engineering of tough double network hydrogels. Macromol. Chem. Phys. 217, 1022–1036. doi: 10.1002/macp.201600038
Chen, X., Dam, M. A., Ono, K., Mal, A., Shen, H., Nutt, S. R., et al. (2002). A thermally re-mendable cross-linked polymeric material. Science 295, 1698–1702. doi: 10.1126/science.1065879
Chen, Y., Cheng, W., Teng, L., Jin, M., Lu, B., Ren, L., et al. (2018b). Graphene oxide hybrid supramolecular hydrogels with self-healable, bioadhesive and stimuli-responsive properties and drug delivery application. Macromol. Mater. Eng. 303, 1–11. doi: 10.1002/mame.201700660
Cho, S. H., White, S. R., and Braun, P. V. (2009). Self-healing polymer coatings. Adv. Mater. 21, 645–649. doi: 10.1002/adma.200802008
Chortos, A., Zhu, C., Oh, J. Y., Yan, X., Pochorovski, I., To, J. W.-F., et al. (2017). Investigating limiting factors in stretchable all-carbon transistors for reliable stretchable electronics. ACS Nano 11, 7925–7937. doi: 10.1021/acsnano.7b02458
Cohades, A., Branfoot, C., Rae, S., Bond, I., and Michaud, V. (2018). Progress in self-healing fiber-reinforced polymer composites. Adv. Mater. Interfaces 5:1800177. doi: 10.1002/admi.201800177
Cong, H. P., Wang, P., and Yu, S. H. (2013). Stretchable and self-healing graphene oxide-polymer composite hydrogels: a dual-network design. Chem. Mater. 25, 3357–3362. doi: 10.1021/cm401919c
Coope, T. S., Mayer, U. F. J., Wass, D. F., Trask, R. S., and Bond, I. P. (2011). Self-healing of an epoxy resin using scandium(III) triflate as a catalytic curing agent. Adv. Funct. Mater. 21, 4624–4631. doi: 10.1002/adfm.201101660
Cordier, P., Tournilhac, F., Soulié-Ziakovic, C., and Leibler, L. (2008). Self-healing and thermoreversible rubber from supramolecular assembly. Nature 451, 977–980. doi: 10.1038/nature06669
Cui, J., and del Campo, A. (2012). Multivalent H-bonds for self-healing hydrogels. Chem. Commun. 48, 9302–9304. doi: 10.1039/c2cc34701f
Cui, W., Ji, J., Cai, Y., Li, H., and Ran, R. (2015). Robust, anti-fatigue, and self-healing graphene oxide/hydrophobically associated composite hydrogels and their use as recyclable adsorbents for dye wastewater treatment. J. Mater. Chem. A 3, 17445–17458. doi: 10.1039/C5TA04470G
Dahlke, J., Zechel, S., Hager, M. D., and Schubert, U. S. (2018). How to design a self-healing polymer: general concepts of dynamic covalent bonds and their application for intrinsic healable materials. Adv. Mater. Interfaces 5, 1–14. doi: 10.1002/admi.201800051
Dai, S., Zhou, X., Wang, S., Ding, J., and Yuan, N. (2018). A self-healing conductive and stretchable aligned carbon nanotube/hydrogel composite with a sandwich structure. Nanoscale 10, 19360–19366. doi: 10.1039/C8NR05897K
Dankers, P. Y. W., Hermans, T. M., Baughman, T. W., Kamikawa, Y., Kieltyka, R. E., Bastings, M. M. C., et al. (2012). Hierarchical formation of supramolecular transient networks in water: a modular injectable delivery system. Adv. Mater. 24, 2703–2709. doi: 10.1002/adma.201104072
Darabi, M. A., Khosrozadeh, A., Mbeleck, R., Liu, Y., Chang, Q., Jiang, J., et al. (2017). Skin-inspired multifunctional autonomic-intrinsic conductive self-healing hydrogels with pressure sensitivity, stretchability, and 3D printability. Adv. Mater. 29:1700533. doi: 10.1002/adma.201700533
de Greef, T. F. A., and Meijer, E. W. (2008). Supramolecular polymers. Nature 453, 171–173. doi: 10.1038/453171a
Deng, G., Li, F., Yu, H., Liu, F., Liu, C., Sun, W., et al. (2012). Dynamic hydrogels with an environmental adaptive self-healing ability and dual responsive sol – gel transitions. ACS Macro Lett. 1, 275–279. doi: 10.1021/mz200195n
Deng, G., Tang, C., and Li, F. (2010). Covalent cross-linked polymer gels with reversible sol - gel transition and self-healing properties. Macromolecules 43, 1191–1194. doi: 10.1021/ma9022197
Diba, M., Spaans, S., Ning, K., Ippel, B. D., Yang, F., Loomans, B., et al. (2018). Self-healing biomaterials: from molecular concepts to clinical applications. Adv. Mater. Interfaces 5:1800118. doi: 10.1002/admi.201800118
Ding, F., Li, H., Du, Y., and Shi, X. (2018). Recent advances in chitosan-based self-healing materials. Res. Chem. Intermed. 44, 4827–4840. doi: 10.1007/s11164-018-3339-7
Ding, Q., Xu, X., Yue, Y., Mei, C., Huang, C., Jiang, S., et al. (2018). Nanocellulose-mediated electroconductive self-healing hydrogels with high strength, plasticity, viscoelasticity, stretchability, and biocompatibility toward multifunctional applications. ACS Appl. Mater. Interfaces 10, 27987–28002. doi: 10.1021/acsami.8b09656
Dong, P., Cui, K., Xu, F., Jiang, T., and Ma, Z. (2018). Synthesis of new ionic crosslinked polymer hydrogel combining polystyrene and poly(4-vinyl pyridine) and its self-healing through a reshuffling reaction of the trithiocarbonate moiety under irradiation of ultraviolet light. Polym. Int. 67, 868–873. doi: 10.1002/pi.5571
Du, P., Wu, M., Liu, X., Zheng, Z., Wang, X., Joncheray, T., et al. (2014a). Diels-Alder-based crosslinked self-healing polyurethane/urea from polymeric methylene diphenyl diisocyanate. J. Appl. Polym. Sci. 131, 1–7. doi: 10.1002/app.40234
Du, R., Wu, J., Chen, L., Huang, H., Zhang, X., and Zhang, J. (2014b). Hierarchical hydrogen bonds directed multi-functional carbon nanotube-based supramolecular hydrogels. Small 10, 1387–1393. doi: 10.1002/smll.201302649
Engel, T., and Kickelbick, G. (2013). Thermoreversible reactions on inorganic nanoparticle surfaces: Diels–Alder reactions on sterically crowded surfaces. Chem. Mater. 25, 149–157. doi: 10.1021/cm303049k
Feng, Z., Zuo, H., Gao, W., Ning, N., Tian, M., and Zhang, L. (2018). A robust, self-healable, and shape memory supramolecular hydrogel by multiple hydrogen bonding interactions. Macromol. Rapid Commun. 39, 1–7. doi: 10.1002/marc.201800138
Fuhrmann, A., Göstl, R., Wendt, R., Kötteritzsch, J., Hager, M. D., Schubert, U. S., et al. (2016). Conditional repair by locally switching the thermal healing capability of dynamic covalent polymers with light. Nat. Commun. 7:13623. doi: 10.1038/ncomms13623
Gaffey, A. C., Chen, M. H., Venkataraman, C. M., Trubelja, A., Rodell, C. B., Dinh, P. V., et al. (2015). Injectable shear-thinning hydrogels used to deliver endothelial progenitor cells, enhance cell engraftment, and improve ischemic myocardium. J. Thorac. Cardiovasc. Surg. 150, 1268–1277. doi: 10.1016/j.jtcvs.2015.07.035
Gaharwar, A. K., Avery, R. K., Assmann, A., Paul, A., McKinley, G. H., Khademhosseini, A., et al. (2014). Shear-thinning nanocomposite hydrogels for the treatment of hemorrhage. ACS Nano 8, 9833–9842. doi: 10.1021/nn503719n
Galland, S., Leterrier, Y., Nardi, T., Plummer, C. J. G., Månson, J. A. E., and Berglund, L. A. (2014). UV-cured cellulose nanofiber composites with moisture durable oxygen barrier properties. J. Appl. Polym. Sci. 131:40604. doi: 10.1002/app.40604
Gantar, A., Drnovšek, N., Casuso, P., Pérez-San Vicente, A., Rodriguez, J., Dupin, D., et al. (2016). Injectable and self-healing dynamic hydrogel containing bioactive glass nanoparticles as a potential biomaterial for bone regeneration. RSC Adv. 6, 69156–69166. doi: 10.1039/C6RA17327F
Gao, G., Du, G., Sun, Y., and Fu, J. (2015). Self-healable, tough, and ultrastretchable nanocomposite hydrogels based on reversible polyacrylamide/montmorillonite adsorption. ACS Appl. Mater. Interfaces 7, 5029–5037. doi: 10.1021/acsami.5b00704
Garcia, S. J. (2014). Effect of polymer architecture on the intrinsic self-healing character of polymers. Eur. Polym. J. 53, 118–125. doi: 10.1016/j.eurpolymj.2014.01.026
Garcia, S. J., Hager, M. D., Bose, R. K., Julia, K., Schubert, U. S., and van der Zwaag, S. (2014). A rheological and spectroscopic study on the kinetics of self-healing in a single-component Diels–Alder copolymer and its underlying chemical reaction. J. Polym. Sci. Part A Polym. Chem. 52, 1669–1675. doi: 10.1002/pola.27164
Gaulding, J. C., Spears, M. W., and Lyon, L. A. (2013). Plastic deformation{,} wrinkling{,} and recovery in microgel multilayers. Polym. Chem. 4, 4890–4896. doi: 10.1039/c3py00173c
Geckil, H., Xu, F., Zhang, X., Moon, S., and Demirci, U. (2010). Engineering hydrogels as extracellular matrix mimics. Nanomedicine 5, 469–484. doi: 10.2217/nnm.10.12
Gong, J. P. (2010). Why are double network hydrogels so tough? Soft Matter 6, 2583–2590. doi: 10.1039/b924290b
Gopal, N., Rana, S., Whan, J., Li, L., and Hwa, S. (2010). Polymer nanocomposites based on functionalized carbon nanotubes. Prog. Polym. Sci. 35, 837–867. doi: 10.1016/j.progpolymsci.2010.03.002
Guadagno, L., Longo, P., Raimondo, M., Naddeo, C., Mariconda, A., Sorrentino, A., et al. (2010). Cure behavior and mechanical properties of structural self-healing epoxy resins. J. Polym. Sci. Part B Polym. Phys. 48, 2413–2423. doi: 10.1002/polb.22139
Guo, K., Zhang, D.-L., Zhang, X.-M., Zhang, J., Ding, L.-S., Li, B.-J., et al. (2015). Conductive elastomers with autonomic self-healing properties. Angew. Chemie Int. Ed. 54, 12127–12133. doi: 10.1002/anie.201505790
Guo, Z., Ma, W., Gu, H., Feng, Y., He, Z., Chen, Q., et al. (2017). pH-Switchable and self-healable hydrogels based on ketone type acylhydrazone dynamic covalent bonds. Soft Matter 13, 7371–7380. doi: 10.1039/C7SM00916J
Haldar, U., Bauri, K., Li, R., Faust, R., and De, P. (2015). Polyisobutylene-based pH-responsive self-healing polymeric gels. ACS Appl. Mater. Interfaces 7, 8779–8788. doi: 10.1021/acsami.5b01272
Han, L., Lu, X., Liu, K., Wang, K., Fang, L., Weng, L. T., et al. (2017a). Mussel-inspired adhesive and tough hydrogel based on nanoclay confined dopamine polymerization. ACS Nano 11, 2561–2574. doi: 10.1021/acsnano.6b05318
Han, L., Lu, X., Wang, M., Gan, D., Deng, W., Wang, K., et al. (2017b). A mussel-inspired conductive, self-adhesive, and self-healable tough hydrogel as cell stimulators and implantable bioelectronics. Small 13:1601916. doi: 10.1002/smll.201601916
Han, L., Yan, L., Wang, K., Fang, L., Zhang, H., Tang, Y., et al. (2017c). Tough, self-healable and tissue-adhesive hydrogel with tunable multifunctionality. NPG Asia Mater. 9:e372. doi: 10.1038/am.2017.33
Han, L., Zhang, Y., Lu, X., Wang, K., Wang, Z., and Zhang, H. (2016). Polydopamine nanoparticles modulating stimuli-responsive PNIPAM hydrogels with cell/tissue adhesiveness. ACS Appl. Mater. Interfaces 8, 29088–29100. doi: 10.1021/acsami.6b11043
Han, S.-T., Peng, H., Sun, Q., Venkatesh, S., Chung, K.-S., Lau, S. C., et al. (2017d). An overview of the development of flexible sensors. Adv. Mater. 29:1700375. doi: 10.1002/adma.201700375
Han, X., Meng, X., Wu, Z., Wu, Z., and Qi, X. (2018). Dynamic imine bond cross-linked self-healing thermosensitive hydrogels for sustained anticancer therapy via intratumoral injection. Mater. Sci. Eng. C 93, 1064–1072. doi: 10.1016/j.msec.2018.08.064
Harrington, M. J., Speck, O., Speck, T., Wagner, S., and Weinkamer, R. (2016). “Biological archetypes for self-healing materials BT - self-healing materials,” in Advances in Polymer Science, eds M. D. Hager, S. van der Zwaag, and U. S. Schubert (Cham: Springer International Publishing, 307–344.
He, L., Fullenkamp, D. E., Rivera, J. G., and Messersmith, P. B. (2011). PH responsive self-healing hydrogels formed by boronate-catechol complexation. Chem. Commun. 47, 7497–7499. doi: 10.1039/c1cc11928a
Herbst, F., Döhler, D., Michael, P., and Binder, W. H. (2013). Self-healing polymers via supramolecular forces. Macromol. Rapid Commun. 34, 203–220. doi: 10.1002/marc.201200675
Herbst, F., Seiffert, S., and Binder, W. H. (2012). Dynamic supramolecular poly(isobutylene)s for self-healing materials. Polym. Chem. 3:3084. doi: 10.1039/c2py20265d
Hernández, M., Grande, A. M., Dierkes, W., Bijleveld, J., Van Der Zwaag, S., and García, S. J. (2016). Turning vulcanized natural rubber into a self-healing polymer: effect of the disulfide/polysulfide ratio. ACS Sustain. Chem. Eng. 4, 5776–5784. doi: 10.1021/acssuschemeng.6b01760
Hong, S. H., Kim, S., Park, J. P., Shin, M., Kim, K., Ryu, J. H., et al. (2018). Dynamic bonds between boronic acid and alginate: hydrogels with stretchable, self-healing, stimuli-responsive, remoldable, and adhesive properties. Biomacromolecules 19, 2053–2061. doi: 10.1021/acs.biomac.8b00144
Hou, C., Huang, T., Wang, H., Yu, H., Zhang, Q., and Li, Y. (2013). A strong and stretchable self-healing film with self-activated pressure sensitivity for potential artificial skin applications. Sci. Rep. 3, 21–25. doi: 10.1038/srep03138
Hu, X., Liang, R., Li, J., Liu, Z., and Sun, G. (2018). Highly stretchable self-healing nanocomposite hydrogel reinforced by 5 nm particles. ES Mater. Manuf. 2, 1–8. doi: 10.30919/esmm5f158
Huang, X., Qi, X., Boey, F., and Zhang, H. (2012). Graphene-based composites. Chem. Soc. Rev. 41, 666–686. doi: 10.1039/C1CS15078B
Huang, Y., Lawrence, P. G., and Lapitsky, Y. (2014). Self-assembly of stiff, adhesive and self-healing gels from common polyelectrolytes. Langmuir 30, 7771–7777. doi: 10.1021/la404606y
Huebsch, N., Kearney, C. J., Zhao, X., Kim, J., Cezar, C. A., Suo, Z., et al. (2014). Ultrasound-triggered disruption and self-healing of reversibly cross-linked hydrogels for drug delivery and enhanced chemotherapy. Proc. Natl. Acad. Sci. U.S.A. 111, 9762–9767. doi: 10.1073/pnas.1405469111
Imaizumi, S., Kokubo, H., and Watanabe, M. (2012). Polymer actuators using ion-gel electrolytes prepared by self-assembly of ABA-triblock copolymers. Macromolecules 45, 401–409. doi: 10.1021/ma2022138
Jeon, I., Cui, J., Illeperuma, W. R. K., Aizenberg, J., and Vlassak, J. J. (2016). Extremely stretchable and fast self-healing hydrogels. Adv. Mater. 28, 4678–4683. doi: 10.1002/adma.201600480
Jiang, G., Liu, C., Liu, X., Zhang, G., Yang, M., and Liu, F. (2009). Construction and properties of hydrophobic association hydrogels with high mechanical strength and reforming capability. Macromol. Mater. Eng. 294, 815–820. doi: 10.1002/mame.200900160
Jiang, H., Zhang, G., Feng, X., Liu, H., Li, F., Wang, M., et al. (2017). Room-temperature self-healing tough nanocomposite hydrogel crosslinked by zirconium hydroxide nanoparticles. Compos. Sci. Technol. 140, 54–62. doi: 10.1016/j.compscitech.2016.12.027
Jin, H., Miller, G. M., Pety, S. J., Griffin, A. S., Stradley, D. S., Roach, D., et al. (2013). Fracture behavior of a self-healing, toughened epoxy adhesive. Int. J. Adhes. Adhes. 44, 157–165. doi: 10.1016/j.ijadhadh.2013.02.015
Jing, X., Mi, H. Y., Napiwocki, B. N., Peng, X. F., and Turng, L. S. (2017). Mussel-inspired electroactive chitosan/graphene oxide composite hydrogel with rapid self-healing and recovery behavior for tissue engineering. Carbon N. Y. 125, 557–570. doi: 10.1016/j.carbon.2017.09.071
Kakuta, T., Takashima, Y., Nakahata, M., Otsubo, M., Yamaguchi, H., and Harada, A. (2013). Preorganized hydrogel: self-healing properties of supramolecular hydrogels formed by polymerization of host–guest-monomers that contain cyclodextrins and hydrophobic guest groups. Adv. Mater. 25, 2849–2853. doi: 10.1002/adma.201205321
Kavitha, A. A., and Singha, N. K. (2009). “Click Chemistry” in tailor-made polymethacrylates bearing reactive furfuryl functionality: a new class of self-healing polymeric material. ACS Appl. Mater. Interfaces 1, 1427–1436. doi: 10.1021/am900124c
Kim, B. J., Oh, D. X., Kim, S., Seo, J. H., Hwang, D. S., Masic, A., et al. (2014). Mussel-mimetic protein-based adhesive hydrogel. Biomacromolecules 15, 1579–1585. doi: 10.1021/bm4017308
Kim, S.-M., Jeon, H., Shin, S.-H., Park, S.-A., Jegal, J., Hwang, S. Y., et al. (2018). Superior toughness and fast self-healing at room temperature engineered by transparent elastomers. Adv. Mater. 30:1705145. doi: 10.1002/adma.201705145
Kitazawa, Y., Iwata, K., Kido, R., Imaizumi, S., Tsuzuki, S., Shinoda, W., et al. (2018). Polymer electrolytes containing solvate ionic liquids: a new approach to achieve high ionic conductivity, thermal stability, and a wide potential window. Chem. Mater. 30, 252–261. doi: 10.1021/acs.chemmater.7b04274
Kohlmeyer, R. R., Lor, M., and Chen, J. (2012). Remote, local, and chemical programming of healable multishape memory polymer nanocomposites. Nano Lett. 12, 2757–2762. doi: 10.1021/nl2044875
Kragl, M., Knapp, D., Nacu, E., Khattak, S., Maden, M., Epperlein, H. H., et al. (2009). Cells keep a memory of their tissue origin during axolotl limb regeneration. Nature 460:60. doi: 10.1038/nature08152
Lau, H. K., and Kiick, K. L. (2015). Opportunities for multicomponent hybrid hydrogels in biomedical applications. Biomacromolecules 16, 28–42. doi: 10.1021/bm501361c
Li, C., Chen, P., Shao, Y., Zhou, X., Wu, Y., Yang, Z., et al. (2015). A writable polypeptide-DNA hydrogel with rationally designed multi-modification sites. Small 11, 1138–1143. doi: 10.1002/smll.201401906
Li, C.-H., Wang, C., Keplinger, C., Zuo, J.-L., Jin, L., Sun, Y., et al. (2016a). A highly stretchable autonomous self-healing elastomer. Nat. Chem. 8:618. doi: 10.1038/nchem.2492
Li, J., Liu, Q., Ho, D., Zhao, S., Wu, S., Ling, L., et al. (2018a). Three-dimensional graphene structure for healable flexible electronics based on Diels–Alder chemistry. ACS Appl. Mater. Interfaces 10, 9727–9735. doi: 10.1021/acsami.7b19649
Li, J., Zhang, G., Sun, R., and Wong, C.-P. (2017). A covalently cross-linked reduced functionalized graphene oxide/polyurethane composite based on Diels–Alder chemistry and its potential application in healable flexible electronics. J. Mater. Chem. C 5, 220–228. doi: 10.1039/C6TC04715G
Li, Q., Barrett, D. G., Messersmith, P. B., and Holten-Andersen, N. (2016b). Controlling hydrogel mechanics via bio-inspired polymer-nanoparticle bond dynamics. ACS Nano 10, 1317–1324. doi: 10.1021/acsnano.5b06692
Li, W., Lu, S., Zhao, M., Lin, X., Zhang, M., Xiao, H., et al. (2018b). Self-healing cellulose nanocrystals-containing gels via reshuffling of thiuram disulfide bonds. Polymers 10:1392. doi: 10.3390/polym10121392
Li, Y., Chen, S., Wu, M., and Sun, J. (2012). Polyelectrolyte multilayers impart healability to highly electrically conductive films. Adv. Mater. 24, 4578–4582. doi: 10.1002/adma.201201306
Li, Y., Wang, X., Fu, Y., Wei, Y., Zhao, L., and Tao, L. (2018c). Self-adapting hydrogel to improve the therapeutic effect in wound-healing. ACS Appl. Mater. Interfaces 10, 26046–26055. doi: 10.1021/acsami.8b08874
Liu, J., Song, G., He, C., and Wang, H. (2013). Self-healing in tough graphene oxide composite hydrogels. Macromol. Rapid Commun. 34, 1002–1007. doi: 10.1002/marc.201300242
Liu, J., Zhang, X., Chen, X., Qu, L., Zhang, L., Li, W., et al. (2018a). Stimuli-responsive dendronized polymeric hydrogels through Schiff-base chemistry showing remarkable topological effects. Polym. Chem. 9, 378–387. doi: 10.1039/C7PY01865G
Liu, Q., Zhan, C., Barhoumi, A., Wang, W., Santamaria, C., McAlvin, J. B., et al. (2016). A supramolecular shear-thinning anti-inflammatory steroid hydrogel. Adv. Mater. 28, 6680–6686. doi: 10.1002/adma.201601147
Liu, S., Kang, M., Li, K., Yao, F., Oderinde, O., and Fu, G. (2018b). Polysaccharide-templated preparation of mechanically-tough, conductive and self-healing hydrogels. Chem. Eng. J. 334, 2222–2230. doi: 10.1016/j.cej.2017.11.103
Liu, X., He, B., Wang, Z., Tang, H., Su, T., and Wang, Q. (2014). Tough nanocomposite ionogel-based actuator exhibits robust performance. Sci. Rep. 4, 1–7. doi: 10.1038/srep06673
Liu, Y.-J., Cao, W.-T., Ma, M.-G., and Wan, P. (2017). Ultrasensitive wearable soft strain sensors of conductive, self-healing, and elastic hydrogels with synergistic “Soft and Hard” hybrid networks. ACS Appl. Mater. Interfaces 9, 25559–25570. doi: 10.1021/acsami.7b07639
Loebel, C., Rodell, C. B., Chen, M. H., and Burdick, J. A. (2017). Shear-thinning and self-healing hydrogels as injectable therapeutics and for 3D-printing. Nat. Protoc. 12:1521. doi: 10.1038/nprot.2017.053
Lu, B., Lin, F., Jiang, X., Cheng, J., Lu, Q., Song, J., et al. (2017). One-pot assembly of microfibrillated cellulose reinforced PVA–borax hydrogels with self-healing and pH-responsive properties. ACS Sustain. Chem. Eng. 5, 948–956. doi: 10.1021/acssuschemeng.6b02279
Lv, C., Zhao, K., and Zheng, J. (2018). A highly stretchable self-healing poly(dimethylsiloxane) elastomer with reprocessability and degradability. Macromol. Rapid Commun. 39, 1–6. doi: 10.1002/marc.201700686
Martin, R., Rekondo, A., Ruiz De Luzuriaga, A., Cabañero, G., Grande, H. J., and Odriozola, I. (2014). The processability of a poly(urea-urethane) elastomer reversibly crosslinked with aromatic disulfide bridges. J. Mater. Chem. A 2, 5710–5715. doi: 10.1039/c3ta14927g
McKee, J. R., Appel, E. A., Seitsonen, J., Kontturi, E., Scherman, O. A., and Ikkala, O. (2014). Healable, stable and stiff hydrogels: combining conflicting properties using dynamic and selective three-component recognition with reinforcing cellulose nanorods. Adv. Funct. Mater. 24, 2706–2713. doi: 10.1002/adfm.201303699
Mera, G., Navrotsky, A., Sen, S., Kleebe, H.-J., and Riedel, R. (2013). Polymer-derived SiCN and SiOC ceramics – structure and energetics at the nanoscale. J. Mater. Chem. A 1, 3826–3836. doi: 10.1039/c2ta00727d
Mukhopadhyay, P., Fujita, N., Takada, A., Kishida, T., Shirakawa, M., and Shinkai, S. (2010). Regulation of a real-time self-healing process in organogel tissues by molecular adhesives. Angew. Chemie Int. Ed. 49, 6338–6342. doi: 10.1002/anie.201001382
Nasresfahani, A., and Zelisko, P. M. (2017). Synthesis of a self-healing siloxane-based elastomer cross-linked via a furan-modified polyhedral oligomeric silsesquioxane investigation of a thermally reversible silicon-based cross-link. Polym. Chem. 8, 2942–2952. doi: 10.1039/C7PY00215G
Nia, A. S., Rana, S., Döhler, D., Osim, W., and Binder, W. H. (2015). Nanocomposites via a direct graphene-promoted “click”-reaction. Polymer 79, 21–28. doi: 10.1016/j.polymer.2015.10.013
Ozdemir, T., and Sozmen, F. (2016). BODIPY based self-healing fluorescent gel formation via acylhydrazone linkage. RSC Adv. 6, 10601–10605. doi: 10.1039/C5RA22993F
Phadke, A., Zhang, C., Arman, B., Hsu, C., Mashelkar, R. A., and Lele, A. K. (2012). Rapid self-healing hydrogels. Proc. Natl. Acad. Sci. U.S.A. 109, 4383–4388. doi: 10.1073/pnas.1201122109
Piest, M., Zhang, X., Trinidad, J., and Engbersen, J. F. J. (2011). PH-responsive, dynamically restructuring hydrogels formed by reversible crosslinking of PVA with phenylboronic acid functionalised PPO-PEO-PPO spacers (Jeffamines®). Soft Matter 7, 11111–11118. doi: 10.1039/c1sm06230a
Potts, J. R., Dreyer, D. R., Bielawski, C. W., and Ruoff, R. S. (2011). Graphene-based polymer nanocomposites. Polymer 52, 5–25. doi: 10.1016/j.polymer.2010.11.042
Pramanik, N. B., Nando, G. B., and Singha, N. K. (2014). Self-healing polymeric gel via RAFT polymerization and Diels-Alder click chemistry. Polymer 69, 349–356. doi: 10.1016/j.polymer.2015.01.023
Qi, H., Liu, J., Gao, S., and Mäder, E. (2013). Multifunctional films composed of carbon nanotubes and cellulose regenerated from alkaline–urea solution. J. Mater. Chem. A 1, 2161–2168. doi: 10.1039/C2TA00882C
Rao, Z., Inoue, M., Matsuda, M., and Taguchi, T. (2011). Quick self-healing and thermo-reversible liposome gel. Colloids Surf. B Biointerfaces 82, 196–202. doi: 10.1016/j.colsurfb.2010.08.038
Rekondo, A., Martin, R., Ruiz De Luzuriaga, A., Cabañero, G., Grande, H. J., and Odriozola, I. (2014). Catalyst-free room-temperature self-healing elastomers based on aromatic disulfide metathesis. Mater. Horizons 1, 237–240. doi: 10.1039/C3MH00061C
Rengui, P., Yu, Y., Chen, S., Yang, Y., and Tang, Y. (2014). Conductive nanocomposite hydrogels with self-healing property. RSC Adv. 4, 35149–35155. doi: 10.1039/C4RA05381H
Rodell, C. B., MacArthur, J. W. Jr., Dorsey, S. M., Wade, R. J., Wang, L. L., Woo, Y. J., et al. (2015). Shear-thinning supramolecular hydrogels with secondary autonomous covalent crosslinking to modulate viscoelastic properties in vivo. Adv. Funct. Mater. Mater. 25, 636–644. doi: 10.1002/adfm.201403550
Roy, S., Baral, A., and Banerjee, A. (2013). An amino-acid-based self-healing hydrogel: modulation of the self-healing properties by incorporating carbon-based nanomaterials. Chem. A Eur. J. 19, 14950–14957. doi: 10.1002/chem.201301655
Sabzi, M., Babaahmadi, M., Samadi, N., Mahdavinia, G. R., Keramati, M., and Nikfarjam, N. (2017). Graphene network enabled high speed electrical actuation of shape memory nanocomposite based on poly(vinyl acetate). Polym. Int. 66, 665–671. doi: 10.1002/pi.5303
Samadzadeh, M., Boura, S. H., Peikari, M., Kasiriha, S. M., and Ashrafi, A. (2010). A review on self-healing coatings based on micro/nanocapsules. Prog. Org. Coatings 68, 159–164. doi: 10.1016/j.porgcoat.2010.01.006
Seyed Shahabadi, S. I., Kong, J., and Lu, X. (2017). Aqueous-only, green route to self-healable, UV-resistant, and electrically conductive polyurethane/graphene/lignin nanocomposite coatings. ACS Sustain. Chem. Eng. 5, 3148–3157. doi: 10.1021/acssuschemeng.6b02941
Shao, C., Wang, M., Chang, H., Xu, F., and Yang, J. (2017). A self-healing cellulose nanocrystal-poly(ethylene glycol) nanocomposite hydrogel via Diels-Alder click reaction. ACS Sustain. Chem. Eng. 5, 6167–6174. doi: 10.1021/acssuschemeng.7b01060
Shao, C., Wang, M., Meng, L., Chang, H., Wang, B., Xu, F., et al. (2018). Mussel-inspired cellulose nanocomposite tough hydrogels with synergistic self-healing, adhesive, and strain-sensitive properties. Chem. Mater. 30, 3110–3121. doi: 10.1021/acs.chemmater.8b01172
Shi, Y., Wang, M., Ma, C., Wang, Y., Li, X., and Yu, G. (2015). A conductive self-healing hybrid gel enabled by metal–ligand supramolecule and nanostructured conductive polymer. Nano Lett. 15, 6276–6281. doi: 10.1021/acs.nanolett.5b03069
Sordo, F., Mougnier, S.-J., Loureiro, N., Tournilhac, F., and Michaud, V. (2015). Design of self-healing supramolecular rubbers with a tunable number of chemical cross-links. Macromolecules 48, 4394–4402. doi: 10.1021/acs.macromol.5b00747
Spoljaric, S., Salminen, A., Luong, N. D., and Seppälä, J. (2014). Stable, self-healing hydrogels from nanofibrillated cellulose, poly(vinyl alcohol) and borax via reversible crosslinking. Eur. Polym. J. 56, 105–117. doi: 10.1016/j.eurpolymj.2014.03.009
Sui, D., Huang, Y., Huang, L., Liang, J., Ma, Y., and Chen, Y. (2011). Flexible and transparent electrothermal film heaters based on graphene materials. Small 7, 3186–3192. doi: 10.1002/smll.201101305
Sun, C., Jia, H., Lei, K., Zhu, D., Gao, Y., Zheng, Z., et al. (2019). Self-healing hydrogels with stimuli responsiveness based on acylhydrazone bonds. Polymer 160, 246–253. doi: 10.1016/j.polymer.2018.11.051
Tarus, D., Hachet, E., Messager, L., Catargi, B., Ravaine, V., and Auzély-Velty, R. (2014). Readily prepared dynamic hydrogels by combining phenyl boronic acid-and maltose-modifi ed anionic polysaccharides at neutral pH. Macromol. Rapid Commun. 35, 2089–2095. doi: 10.1002/marc.201400477
Tee, B. C.-K., Wang, C., Allen, R., and Bao, Z. (2012). An electrically and mechanically self-healing composite with pressure- and flexion-sensitive properties for electronic skin applications. Nat. Nanotechnol. 7, 825–832. doi: 10.1038/nnano.2012.192
Thakur, S., Barua, S., and Karak, N. (2015). Self-healable castor oil based tough smart hyperbranched polyurethane nanocomposite with antimicrobial attributes. RSC Adv. 5, 2167–2176. doi: 10.1039/C4RA11730A
Thakur, S., and Karak, N. (2015). Tuning of sunlight-induced self-cleaning and self-healing attributes of an elastomeric nanocomposite by judicious compositional variation of the TiO2-reduced graphene oxide nanohybrid. J. Mater. Chem. A 3, 12334–12342. doi: 10.1039/C5TA02162F
Tuncaboylu, D. C., Sahin, M., Argun, A., Oppermann, W., and Okay, O. (2012). Dynamics and large strain behavior of self-healing hydrogels with and without surfactants. Macromolecules 45, 1991–2000. doi: 10.1021/ma202672y
Tuncaboylu, D. C., Sari, M., Oppermann, W., and Okay, O. (2011). Tough and self-healing hydrogels formed via hydrophobic interactions. Macromolecules 44, 4997–5005. doi: 10.1021/ma200579v
Van Gemert, G. M. L., Peeters, J. W., Söntjens, S. H. M., Janssen, H. M., and Bosman, A. W. (2012). Self-healing supramolecular polymers in action. Macromol. Chem. Phys. 213, 234–242. doi: 10.1002/macp.201100559
Vig, B. S., Huttunen, K. M., Laine, K., and Rautio, J. (2013). Amino acids as promoieties in prodrug design and development. Adv. Drug Deliv. Rev. 65, 1370-−1385. doi: 10.1016/j.addr.2012.10.001
Wang, C., Liu, N., Allen, R., Tok, J. B.-H., Wu, Y., Zhang, F., et al. (2013). A rapid and efficient self-healing thermo-reversible elastomer crosslinked with graphene oxide. Adv. Mater. 25, 5785–5790. doi: 10.1002/adma.201302962
Wang, J., Lv, C., Li, Z., and Zheng, J. (2018). Facile preparation of polydimethylsiloxane elastomer with self-healing property and remoldability based on Diels–Alder chemistry. Macromol. Mater. Eng. 303, 1–6. doi: 10.1002/mame.201800089
Wang, J., Wang, D., Yan, H., Tao, L., Wei, Y., Li, Y., et al. (2017a). An injectable ionic hydrogel inducing high temperature hyperthermia for microwave tumor ablation. J. Mater. Chem. B 5, 4110–4120. doi: 10.1039/C7TB00556C
Wang, Q., Gossweiler, G. R., Craig, S. L., and Zhao, X. (2014). Cephalopod-inspired design of electro-mechano-chemically responsive elastomers for on-demand fluorescent patterning. Nat. Commun. 5:4899. doi: 10.1038/ncomms5899
Wang, W., Narain, R., and Zeng, H. (2018). Rational design of self-healing tough hydrogels: a mini review. Front. Chem. 6:497. doi: 10.3389/fchem.2018.00497
Wang, W., Xu, Y., Li, A., Li, T., Liu, M., von Klitzing, R., et al. (2015). Zinc induced polyelectrolyte coacervate bioadhesive and its transition to a self-healing hydrogel. RSC Adv. 5, 66871–66878. doi: 10.1039/C5RA11915D
Wang, Y., Park, J. P., Hong, S. H., and Lee, H. (2016). Biologically inspired materials exhibiting repeatable regeneration with self-sealing capabilities without external stimuli or catalysts. Adv. Mater. 28, 9961–9968. doi: 10.1002/adma.201603290
Wang, Y. M., Pan, M., Liang, X. Y., Li, B. J., and Zhang, S. (2017b). Electromagnetic wave absorption coating material with self-healing properties. Macromol. Rapid Commun. 38, 1–8. doi: 10.1002/marc.201700447
Wang, Z., Tian, H., He, Q., and Cai, S. (2017c). Reprogrammable, reprocessible, and self-healable liquid crystal elastomer with exchangeable disulfide bonds. ACS Appl. Mater. Interfaces 9, 33119–33128. doi: 10.1021/acsami.7b09246
Wei, D., Yang, J., Zhu, L., Chen, F., Tang, Z., Qin, G., et al. (2018). Semicrystalline hydrophobically associated hydrogels with integrated high performances. ACS Appl. Mater. Interfaces 10, 2946–2956. doi: 10.1021/acsami.7b15843
Wei, H., Du, S., Liu, Y., Zhao, H., and Chen, C. (2014). Tunable, luminescent, and self-healing hybrid hydrogels of polyoxometalates and triblock copolymers based on electrostatic assembly. Chem. Commun. 50, 1447–1450. doi: 10.1039/C3CC48732F
Wei, Z., He, J., Liang, T., Oh, H., Athas, J., Tong, Z., et al. (2013a). Autonomous self-healing of poly(acrylic acid) hydrogels induced by the migration of ferric ions. Polym. Chem. 4, 4601–4605. doi: 10.1039/c3py00692a
Wei, Z., Yang, J. H., Du, X. J., Xu, F., Zrinyi, M., Osada, Y., et al. (2013b). Dextran-based self-healing hydrogels formed by reversible Diels–Alder reaction under physiological conditions. Macromol. Rapid Commun. 34, 1464–1470. doi: 10.1002/marc.201300494
White, S. R., Sottos, N. R., Geubelle, P. H., Moore, J. S., Kessler, M. R., Sriram, S. R., et al. (2002). Correction: autonomic healing of polymer composites. Nature 415, 817–817. doi: 10.1038/415817a
Wilson, G. O., Moore, J. S., White, S. R., Sottos, N. R., and Andersson, H. M. (2008). Autonomic healing of epoxy vinyl esters via ring opening metathesis polymerization. Adv. Funct. Mater. 18, 44–52. doi: 10.1002/adfm.200700419
Wu, S., Li, J., Zhang, G., Yao, Y., Li, G., Sun, R., et al. (2017). Ultrafast self-healing nanocomposites via infrared laser and their application in flexible electronics. ACS Appl. Mater. Interfaces 9, 3040–3049. doi: 10.1021/acsami.6b15476
Wu, T., and Chen, B. (2016a). A mechanically and electrically self-healing graphite composite dough for stencil-printable stretchable conductors. J. Mater. Chem. C 4, 4150–4154. doi: 10.1039/C6TC01052K
Wu, T., and Chen, B. (2016b). Synthesis of multiwalled carbon nanotube-reinforced polyborosiloxane nanocomposites with mechanically adaptive and self-healing capabilities for flexible conductors. ACS Appl. Mater. Interfaces 8, 24071–24078. doi: 10.1021/acsami.6b06137
Wu, X., Li, J., Li, G., Ling, L., Zhang, G., Sun, R., et al. (2018). Heat-triggered poly(siloxane-urethane)s based on disulfide bonds for self-healing application. J. Appl. Polym. Sci. 135, 1–9. doi: 10.1002/app.46532
Xia, L.-Y., Zhang, X., Cao, M., Chen, Z., and Wu, F.-G. (2017). Enhanced fluorescence emission and singlet oxygen generation of photosensitizers embedded in injectable hydrogels for imaging-guided photodynamic cancer therapy. Biomacromolecules 18, 3073–3081. doi: 10.1021/acs.biomac.7b00725
Xing, R., Liu, K., Jiao, T., Zhang, N., Ma, K., Zhang, R., et al. (2016). An injectable self-assembling collagen – gold hybrid hydrogel for combinatorial antitumor photothermal/photodynamic therapy. Adv. Mater. 28, 3669–3676. doi: 10.1002/adma.201600284
Xu, C., Cao, L., Lin, B., Liang, X., and Chen, Y. (2016). Design of self-healing supramolecular rubbers by introducing ionic cross-links into natural rubber via a controlled vulcanization. ACS Appl. Mater. Interfaces 8, 17728–17737. doi: 10.1021/acsami.6b05941
Xu, C., Cui, R., Fu, L., and Lin, B. (2018). Recyclable and heat-healable epoxidized natural rubber/bentonite composites. Compos. Sci. Technol. 167, 421–430. doi: 10.1016/j.compscitech.2018.08.027
Xu, J., Yang, D., Li, W., Gao, Y., Chen, H., and Li, H. (2011). Phenylboronate-diol crosslinked polymer gels with reversible sol-gel transition. Polymer (52, 4268–4276. doi: 10.1016/j.polymer.2011.07.015
Xu, Y., Wu, Q., Sun, Y., Bai, H., and Shi, G. (2010). Three-dimensional self-assembly of graphene oxide and DNA into multifunctional hydrogels. ACS Nano 4, 7358–7362. doi: 10.1021/nn1027104
Xu, Z., Peng, J., Yan, N., Yu, H., Zhang, S., Liu, K., et al. (2013). Simple design but marvelous performances: molecular gels of superior strength and self-healing properties. Soft Matter 9, 1091–1099. doi: 10.1039/C2SM27208C
Yan, M., Tang, J., Xie, H., Lou, N.i, B., Zhang, H. L., and Chen, E. Q. (2015). Self-healing and phase behavior of liquid crystalline elastomer based on a block copolymer constituted of a side-chain liquid crystalline polymer and a hydrogen bonding block. J. Mater. Chem. C 3, 8526–8534. doi: 10.1039/C5TC01603G
Yang, H., Tang, J., Shang, C., Miao, R., Zhang, S., and Liu, K. (2018). Calix [4] arene-based dynamic covalent gels: marriage of robustness, responsiveness, and self-healing. Macromol. Rapid Commun. 39:1700679. doi: 10.1002/marc.201700679
Yang, W. J., Tao, X., Zhao, T., Weng, L., Kang, E.-T., and Wang, L. (2015a). Antifouling and antibacterial hydrogel coatings with self-healing properties based on a dynamic disulfide exchange reaction. Polym. Chem. 6, 7027–7035. doi: 10.1039/C5PY00936G
Yang, X., Liu, G., Peng, L., Guo, J., Tao, L., and Yuan, J. (2017). Highly efficient self-healable and dual responsive cellulose-based hydrogels for controlled release and 3D cell culture. Adv. Funct. Mater. 27:1703174. doi: 10.1002/adfm.201703174
Yang, Y., Ding, X., and Urban, M. W. (2015b). Chemical and physical aspects of self-healing materials. Prog. Polym. Sci. 49, 34–59. doi: 10.1016/j.progpolymsci.2015.06.001
Yang, Y., and Urban, M. W. (2013). Self-healing polymeric materials. Chem. Soc. Rev. 42, 7446–7467. doi: 10.1039/c3cs60109a
Yavvari, P. S., Pal, S., Kumar, S., Kar, A., Awasthi, A. K., Naaz, A., et al. (2017). Injectable, self-healing chimeric Catechol-Fe(III) hydrogel for localized combination cancer therapy. ACS Biomater. Sci. Eng. 3, 3404–3413. doi: 10.1021/acsbiomaterials.7b00741
Yoon, J. A., Kamada, J., Koynov, K., Mohin, J., Nicolaý, R., Zhang, Y., et al. (2012). Self-healing polymer films based on Thiol–Disulfide exchange reactions and self-healing kinetics measured using atomic force microscopy. Macromolecules 45, 142–149. doi: 10.1021/ma2015134
Yu, H., Wang, Y., Yang, H., Peng, K., and Zhang, X. (2017). Injectable self-healing hydrogels formed via thiol/disulfide exchange of thiol functionalized F127 and dithiolane modified PEG. J. Mater. Chem. B 5, 4121–4127. doi: 10.1039/C7TB00746A
Zhang, D. D., Ruan, Y. B., Zhang, B. Q., Qiao, X., Deng, G., Chen, Y., et al. (2017). A self-healing PDMS elastomer based on acylhydrazone groups and the role of hydrogen bonds. Polymer 120, 189–196. doi: 10.1016/j.polymer.2017.05.060
Zhang, E., Wang, T., Zhao, L., Sun, W., Liu, X., and Tong, Z. (2014). Fast self-healing of graphene oxide-hectorite clay-poly(N,N -dimethylacrylamide) hybrid hydrogels realized by near-infrared irradiation. ACS Appl. Mater. Interfaces 6, 22855–22861. doi: 10.1021/am507100m
Zhang, F., Ju, P., Pan, M., Zhang, D., Huang, Y., Li, G., et al. (2018). Self-healing mechanisms in smart protective coatings: a review. Corros. Sci. 144, 74–88. doi: 10.1016/j.corsci.2018.08.005
Zhang, Y., Tao, L., Li, S., and Wei, Y. (2011). Synthesis of multiresponsive and dynamic chitosan-based hydrogels for controlled release of bioactive molecules. Biomacromolecules 12, 2894–2901. doi: 10.1021/bm200423f
Zhao, L., Huang, J., Wang, T., Sun, W., and Tong, Z. (2017a). Multiple shape memory, self-healable, and supertough PAA-GO-Fe3+hydrogel. Macromol. Mater. Eng. 302, 1–9. doi: 10.1002/mame.201600359
Zhao, X., Wu, H., Guo, B., Dong, R., Qiu, Y., and Ma, P. X. (2017b). Biomaterials antibacterial anti-oxidant electroactive injectable hydrogel as self- healing wound dressing with hemostasis and adhesiveness for cutaneous wound healing. Biomaterials 122, 34–47. doi: 10.1016/j.biomaterials.2017.01.011
Zhao, Y., Zhang, Y., Sun, H., Dong, X., Cao, J., Wang, L., et al. (2016). A self-healing aqueous lithium-ion battery. Angew. Chemie Int. Ed. 55, 14384–14388. doi: 10.1002/anie.201607951
Zheng, Q., Ma, Z., and Gong, S. (2016). Multi-stimuli-responsive self-healing metallo-supramolecular polymer nanocomposites. J. Mater. Chem. A 4, 3324–3334. doi: 10.1039/C5TA10694J
Zhong, M., Liu, X.-Y., Shi, F.-K., Zhang, L.-Q., Wang, X.-P., Cheetham, A. G., et al. (2015a). Self-healable, tough and highly stretchable ionic nanocomposite physical hydrogels. Soft Matter 11, 4235–4241. doi: 10.1039/C5SM00493D
Zhong, M., Liu, Y.-T., and Xie, X.-M. (2015b). Self-healable, super tough graphene oxide–poly(acrylic acid) nanocomposite hydrogels facilitated by dual cross-linking effects through dynamic ionic interactions. J. Mater. Chem. B 3, 4001–4008. doi: 10.1039/C5TB00075K
Zhong, Y., Wang, X., Zheng, Z., and Du, P. (2015c). Polyether-maleimide-based crosslinked self-healing polyurethane with Diels-Alder bonds. J. Appl. Polym. Sci. 132, 1–9. doi: 10.1002/app.41944
Zhu, M., He, S., Dai, Y., Han, J., Gan, L., Liu, J., et al. (2018). Long-lasting sustainable self-healing ion gel with triple-network by trigger-free dynamic hydrogen bonds and ion bonds. ACS Sustain. Chem. Eng. 6, 17087–17098. doi: 10.1021/acssuschemeng.8b04452
Keywords: nanocomposites, self-healing, elastomers, gels, soft matter, interfacial interactions
Citation: Sanka RVSP, Krishnakumar B, Leterrier Y, Pandey S, Rana S and Michaud V (2019) Soft Self-Healing Nanocomposites. Front. Mater. 6:137. doi: 10.3389/fmats.2019.00137
Received: 31 January 2019; Accepted: 28 May 2019;
Published: 18 June 2019.
Edited by:
Alfonso Maffezzoli, University of Salento, ItalyReviewed by:
Xuhong Guo, East China University of Science and Technology, ChinaCopyright © 2019 Sanka, Krishnakumar, Leterrier, Pandey, Rana and Michaud. This is an open-access article distributed under the terms of the Creative Commons Attribution License (CC BY). The use, distribution or reproduction in other forums is permitted, provided the original author(s) and the copyright owner(s) are credited and that the original publication in this journal is cited, in accordance with accepted academic practice. No use, distribution or reproduction is permitted which does not comply with these terms.
*Correspondence: Sravendra Rana, c3JhbmFAZGRuLnVwZXMuYWMuaW4=; Véronique Michaud, dmVyb25pcXVlLm1pY2hhdWRAZXBmbC5jaA==
Disclaimer: All claims expressed in this article are solely those of the authors and do not necessarily represent those of their affiliated organizations, or those of the publisher, the editors and the reviewers. Any product that may be evaluated in this article or claim that may be made by its manufacturer is not guaranteed or endorsed by the publisher.
Research integrity at Frontiers
Learn more about the work of our research integrity team to safeguard the quality of each article we publish.