- 1Department of Industrial Engineering, University of Salerno, Fisciano, Italy
- 2Nice Filler s.r.l., Naples, Italy
- 3National Research Council, Institute for Research on Combustion, Naples, Italy
The present work reports a study aimed at investigating the recovery and the upgrade of tomato processing residues through the preparation of innovative green composites based on tomato peels (TPs) and natural halloysite nanotubes (HNTs) loaded with carvacrol, as a natural antibacterial agent. The loading of carvacrol into HNTs was successfully achieved through a simple procedure that does not involve any chemical modifications of HNTs. Composite films with different amount of HNTs (i.e., 2.5, 5, 10, 20%wt) were prepared using mechanical milling in presence of water, followed by solvent casting method. The structural organization and the main physical properties of the obtained bio-based active composites have been deeply investigated. In particular, thermal and mechanical properties along with the films' interaction with water vapor were assessed and then correlated to the filler loading. Results show that the thermal behaviour of bio-composites improves with the filler loading. Conversely, the mechanical properties get worse, especially at higher loading, due to the poor interaction between HNTs and matrix. The presence of hydrophilic HNTs was found to not dramatically increase the degree of hydrophilicity of the of the materials, most likely due to the presence of hydrophobic carvacrol that acts as an hydrophobic coating for HNTs. The release of carvacrol was also analyzed. Results show that the obtained composites exhibit a long-term release of the antimicrobial agent, which suggests them to be promising candidate materials for food packaging application.
Introduction
The concern of the society about environmental issues and sustainability has increased greatly over the last decades. The replacement of fossil fuels with renewable biomass and bio-wastes as raw feedstocks, for the production of bio-chemicals and bio-materials, is an interesting option to respond to these concerns (Brachi et al., 2014).
Food packaging is currently one of the major source of plastic wastes (Kirwan and Strawbridge, 2003). The largest fraction of the materials used in the packaging industry comes from fossil fuels and is non-degradable, thus representing a serious environmental issue worldwide. The demand for the more eco-friendly and sustainable films and/or coatings for effective food packaging has, therefore, generated considerable interest in recent years. In particular, the development of new bio-materials from largely available and cheap residual biomass resources, such as agro-industrial residues, to produce green/edible packaging manufactures that meet aesthetic, mechanical and barrier requirements for food applications is one of the major challenges currently facing scientists and technologists from different areas of expertise (Zhiguo et al., 2015; Eghbal and Choudhary, 2018; Kam et al., 2018). Edible packaging materials are, in fact, not just biodegradable in nature, thus avoiding the environmental damage and concerns about their disposal (Giosafatto et al., 2014a,b), but also have the characteristic of being eaten along with the food they contain, thereby offering greater opportunities for commercial application. For example, green films based on proteins and/or polysaccharides can be filled with a wide range of functional additives, thus acting as carriers for antioxidants, antimicrobials, flavoring agents, colorants and vitamins in more specific applications (Gorrasi et al., 2012; Gorrasi and Bugatti, 2016; Saha et al., 2016; Silva et al., 2016).
Tomato (Lycopersicon esculentum) represents the second most diffused vegetable crop worldwide, next to potato (Brachi et al., 2016a,b). Each year about 150 million tons are produced and consumed, and 40 million are processed products (i.e., peeled or unpeeled, tomato paste, whole or un-whole tomatoes) (Tomato News, 2014). The tomato processing typically generates two residual fractions, which compose 3–5 wt% of the whole tomato: (i) tomato peels, that is the byproduct of the peeling of tomatoes used for canning and (ii) tomato pomace that is a mixture crushed seeds, tomato peels and a small residue of pulp remaining after the processing for soup, juice or ketchup (Al Khraisat, 2015). Such wastes, due to the high organic content molecules (carbohydrates, proteins, fats, oils, etc.), if not opportunely managed, not only represent increasing disposal and potentially severe pollution problems, but they can also be a considerable loss of potentially valuable biomass and nutrients. In this context, the use of cheap and largely available wastes from tomato processing industry as a major component of edible films has been proposed in this work since it may offer a more sustainable and eco-friendly option to produce novel prototypes for food packaging application. In more detail, it may contribute to effectively reduce the environmental impact from both packaging and food industries simultaneously.
Despite aforementioned advantages, green/edible packaging manufactures have only experienced a limited development and use so far (Trends et al., 2013). Regarding bio-based food packaging, the main challenge is related to their inherent characteristics such as the poor barrier and mechanical properties. In addition, the difficulty in introducing and retaining for long periods any bacteriostatic or bacteriolitic substances able to preserve the food quality, because of the fast release from the material's bulk, has also hampered their diffusion (Gorrasi, 2015). In this regard, the possibility to encapsulate such substances within natural, biocompatible and non-toxic nano-containers, which allow for their sustained release, could offer a viable option to overcome the above drawback. Halloysite nanotubes (HNTs) are aluminumsilicate nanoparticles, having a hollow tubular shape, high aspect ratio and high strength, which make them suitable nanocarriers for biologically active molecules. Moreover, they can be effectively dispersed in polymeric matrices (biocompatible). Because of these properties, HNTs have proved to be very effective in the production of high-performance polymeric nanocomposites, specifically for the controlled release of active agents (Lvov et al., 2008; Cavallaro et al., 2011, 2013; Gorrasi and Vertuccio, 2016; Scarfato et al., 2016; Bugatti et al., 2017; Gorrasi et al., 2017). In particular, their non-toxic nature (Vergaro et al., 2010; Fakhrullina et al., 2015), and effective encapsulation capacity make HNTs ideal nano-containers for antibacterial agents. In addition, Halloysites has been proven to be a biocompatible material in several recent reports on cell cultures (Lvov et al., 2016), and invertebrate models (Fakhrullina et al., 2015). In particular, studies by Lvov et al. (2016), where the toxicity of HNT was tested after 48 h of incubation with fibroblast and human breast cells, showed that it is much less harmful than ordinary sodium chloride salt (Massaro et al., 2017).
The preparation of bio-based nano-composites via the traditional melt mixing techniques is not a viable option since their melting temperatures are typically too close to the temperatures at which the thermal degradation of the natural matrices takes place. Mechanical milling (MM), as a solid state mixing, has turned out to be an economic and ecological alternative for achieving an efficient dispersion of nanofillers into bio-based and biodegradable matrices (Gorrasi and Sorrentino, 2015), as well as in oil derived polymers (Delogu et al., 2017). In addition to this, the advantage of working in dry conditions and with almost any type of polymer matrix at ambient temperature, opened new and unexpected routes for the preparation of advanced functional composites (Gorrasi et al., 2007). The use of mechanical energy contains several inherent advantages including the control of degradation processes associated with high temperatures, the strong reduction of environmental impact and the possibility of compatibilizing (recycle) immiscible blends derived from end used materials. The simultaneous dispersion of nanoparticles, the promotion of mechano-chemical reactions and the proper manipulation of thermo-sensitive active molecules (i.e., antimicrobials, oxygen scavengers and drugs, represent further fundamental advantages of this process in the framework of the formulation and preparation of novel polymer nanocomposites with functional properties.
In this context, mechanical milling was selected in this work for the preparation of novel green/edible composites consisting of tomato peels (TPs) as a bio-based matrix and carvacrol encapsulated into HNTs as a natural antibacterial agent. The structural organization and the main physical properties of the obtained active bio-based composites were investigated; in particular, thermal and mechanical properties along with the films' interaction with water vapor were assessed and then correlated to the filler loading. The sustained release of carvacrol was also analyzed. Finally, the study was complemented with in-depth characterization of tomato peels residues (i.e., ultimate and proximate analyses, calorific values measures, and cellulose, hemicellulose and lignin content determination), which may be useful to identify further suitable application routes.
Experimental Section
Raw Materials Sampling and Characterization
Tomato peels (TPs) used in this work were collected from a tomato processing industry located in Salerno (40°47′24.5′′N, 14°46′15.8′′E), Campania region (IT). Prior to use, the raw feedstock was subjected to some pretreatments. In particular, it was first placed in a ventilated fume hood for approximately 48 h of exposure to fresh air, which reduced its moisture content from about 80%wt to about 6%wt. Then, it was processed to reduce its particles size by using a batch knife mill (Grindomix GM 300 by Retsch) for 20 s at a speed as high as 3,200 rpm for three times. The milled sample was finally manually sieved and the size fraction smaller than 500 μm was kept for the following utilization. Halloysite nanoclay powders (CAS 1332-58-7) and carvacrol (CAS 499-75-2) were supplied from Sigma Aldrich (Italy) and were used as received.
The determination of volatile matter, moisture, ash content and fixed carbon in raw tomato peels was performed by using a TGA 701 LECO thermogravimetric analyzer by following the ASTM D5142. Elemental analysis was carried out according to ASTM D 5291-92 by using a CHN 2000 LECO analyzer for carbon, hydrogen and nitrogen. The oxygen content was finally calculated by subtracting the ash content and CHN from the total. All these analyses were performed in triplicate at least and the average values are reported in Table 1. The higher heating value (HHV, MJ/kg on a dry basis) of the dried samples was measured using an oxygen bomb calorimeter (Parr 6200 Calorimeter). The measurements were repeated twice and the average value was used for subsequent calculation of the low calorific value (LHV) according to the Equation (1):
where Hdry represents the weight percent of hydrogen resulting from elemental analysis, and LHV and HHV are given in MJ/kg. The results are also presented in Table 1. The contents of extractives, hemicellulose, cellulose and lignin in raw tomato peels were also determined according to a previously reported procedure (Brachi et al., 2016a,b). Briefly, about 10 g of air-dried TPs passing through a 40 mesh screen were first submitted to Soxhlet extraction with an ethanol/toluene (1:2, v:v) solution in order to remove the extractives (i.e., fats, resin, wax, phenol, pigments, oils, and other organic compounds), which could affect the subsequent fiber analysis. In more details, the extraction was conducted for 24 h at the rate of about 8 siphon cycles per hour. Then, the resulting oven-dried, extractive-free solid sample (about 3 g) was submitted to a delignification process by treating it with sodium chlorite (NaClO2, technical grade, 80%) and acetic acid (reagent grade) for about 6 h in a water bath at 70°C. The extractive- and lignin-free white holocellulose sample was subsequently treated with two sodium hydroxide solutions (17.5 and 8.3 wt%, respectively) and acetic acid (10 wt%) to isolate the α-cellulose fraction. The soluble fraction remaining after this treatment represents hemicellulose and pectins. Finally the lignin content was calculated by difference. The organic polymeric composition of TPs obtained through the above procedures, is shown in Table 1. The content of inorganic elements in raw tomato peels was determined by inductively coupled plasma-mass spectrometry (ICP-MS) by using an Agilent 7500 CE instrument. The results obtained from these analyses are shown in Table 2.
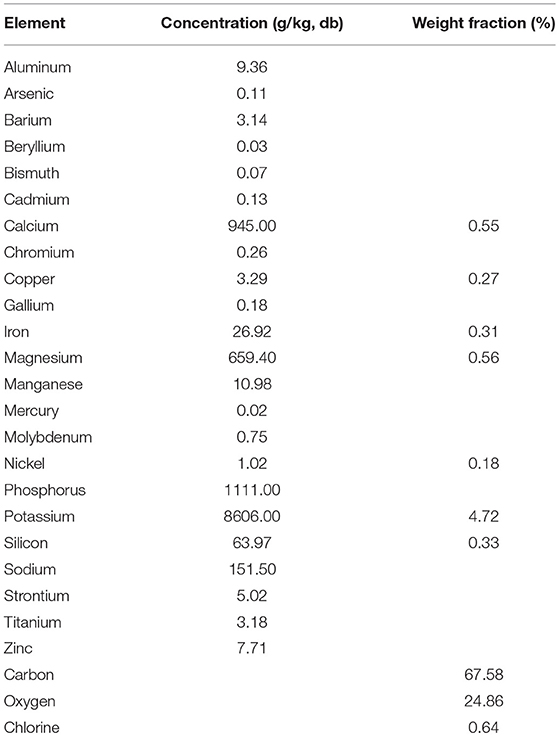
Table 2. Content of chemical elements in raw tomato peels by ICP-MS analysis (left hand column), and EDX relative to SEM micrograph in Figure 1C (right hand column).
Preparation of HNTs-Carvacrol Nano-Hybrid
0.5 g of HNTs and 10 mL of carvacrol were firstly mixed and ultrasonicated by using an ultrasonicator UP200S for 30 min in an ice bath. The HNT-carvacrol mixture was then transferred into a vacuum jar connected to a vacuum pump; 1 mbar pressure was applied into the jar for 30 min to remove air inside HNTs, followed by the application of atmospheric pressure for 10 min to allow carvacrol molecules enter evacuated HNTs. The cycle was repeated three times to increase loading efficiency. The content of carvacrol (%wt) in the HNT-carvacrol hybrid was evaluated by the thermogravimetric analysis (Figure 2A) according to the following Equation (2):
where X is the loaded carvacrol, Rmix is the weight loss percentage of the mixture, RH and Rs are the weight loss percentages of halloysite and carvacrol, respectively. This equation assumes that the total weight loss of the single component is not affected by the other components present in mixture. The carvacrol amount detected with this method was ≅76%.
Preparation of Composites TPs/HNTs-Carvacrol
Composites TPs/HNTs-carvacrol were prepared by the following procedure. One gram of tomato peels was dissolved in 20 mL of distilled water and 2 mL of glycerol, as plasticizer. The obtained solution was stirred for 3 h at 70°C. The nano-hybrid was added to the tomato peels/glycerol/water solution in different amounts, in order to obtain nano-hybrid concentrations equal to 2.5, 5, 10, and 20 wt%. To allow the best intimate mixing between the different phases, after stirring the composite solution for 30 min, each composition was submitted to high energy ball milling at ambient temperature for 60 min. The apparatus used was a Retsh (Germany) planetary ball milling (PM 100), with a cylindrical steel jar of 50 cm3 containing 5 tungsten carbide balls of 10 mm of diameter. The rotation speed was 640 rpm. The mixtures obtained were poured into Petri dishes and dried in an oven at 70°C for 7 days. Films of pure tomato peels were obtained using the same experimental conditions. All films, having the thickness of about 600 μm, were then characterized. Composite samples will be coded as follows: TPsxHNTs-Carvy where x is the tomato peel amount and y is the HNTs-carvacrol load.
Methods of Investigation
SEM/EDX measurements were performed by a Fei Inspect Microscope. X-ray diffraction (XRD) patterns were taken, in reflection, with an automatic Bruker diffractometer (equipped with a continuous scan attachment and a proportional counter), using nickel-filtered Cu Kα radiation (Kα = 1.54050 Å) and operating at 40 kV and 40 mA, step scan 0.05° of 2Ï' and 3 s of counting time.
Thermogravimetric analyses (TGA) were carried out from 30 to 600°C (heating rate of 10°C/min) under air flow, using a Mettler TC-10 thermo-balance.
The mechanical properties of the samples were evaluated from stress-strain curves obtained using a dynamometric apparatus INSTRON 4301. The experiments were conducted at room temperature with the deformation rate of 5 mm/min. The initial length of the samples was about 10 mm. Elastic moduli were derived from the linear part of the stress-strain curves, giving to the sample a deformation of 0.1%. Data were averaged on five samples.
Sorption of water, in controlled atmosphere and humidity on both TPs films and film composites (approximately 600 μm thickness), was investigated through the determination of moisture uptake of samples exposed at the same conditions of temperature and relative humidity (HR). The conventional static desiccator technique (Bellur et al., 2009; Vasquez and Coronella, 2009), which employs a glass desiccator containing a supersaturated salt water solution as a humidity control chamber, was employed. Specifically, three different salts, i.e., KBr, Mg(NO3)2, and CH3COOK, were used in this work, which allow exposing samples to 80 ± 2, 53 ± 2 and 23 ± 2% of RH, respectively, at room temperature (i.e., 25 ± 2°C). In order to minimize temperature changes, the desiccator was partially submerged in a water bath. Approximately 100 mg of oven-dried samples were put into an open weighing bottle and then placed into the desiccator for testing. The humidity and temperature in the glass desiccator were checked using a digital thermo-hygrometer (30.5005 TFA Dostmann). Equilibrium moisture content (ECM) of each sample up to an exposure time of 60 days was measured by using a Kern DBS Halogen Moisture analyzer.
The release kinetics of carvacrol were performed by ultraviolet spectrometric measurement at ambient temperature, using a Spectrometer UV-2401 PC Shimadzu (Japan). The tests were performed using rectangular specimens of 4 cm2 and same thickness (600 μm), placed into 25 mL of ethanol and stirred at 100 rpm in an orbital shaker (VDRL MOD. 711+, Asal S.r.l.). The medium release was withdrawn at fixed time intervals and replenished with fresh medium. The considered band was at 275 nm.
Results and Discussion
Experimental results regarding composition (proximate analysis, fiber composition and ultimate) and calorific content of fresh TPs, as well as their comparison with the values found in the literature, are shown in Table 1. Comparing the obtained data with literature references (Rossini et al., 2013; Toscano et al., 2015; Mangut et al., 2016) some inconsistence emerge regarding the fiber composition of tomato peel, which conversely are not observed among the data from the proximate, the ultimate and the calorific-content analyses. In this regard, it is worth noting that a different analytical approach (van Soest method) has been used by Toscano et al. (2015), to quantify the individual components in tomato peels with respect that used in this research, following the Laboratory Analytical Procedures (LAP) established by National Renewable Energy Laboratory (NREL). It is well-known that methods that utilize different chemical means to separate and quantify the biomass components can give different compositional results for the same substrate (Templeton et al., 2014). Accordingly, the comparison of the analyzed values in this paper with the literature references confirms the consistency of the data shown here.
Figures 1A–D show the SEM micrographs of tomato peel samples obtained at different magnifications. It results that tomato peels are very thin, having a thickness of approximately 50 μm (Figure 1C) and exhibit a well-organized 3D honeycomb-like structure with large cavities, which provides a larger specific surface area (C-D). The Energy Dispersive X-ray (EDX) analysis of the tomato peel surface relative to the SEM micrograph in Figure 1C confirms that TPs contain a large amount of carbon (C), oxygen (O), and potassium (K) (Table 2), in agreement with data obtained by ultimate (Table 1) and ICP-MS analyses (Table 2). The presence of chlorine (Cl) in tomato peels is in accordance with the literature (Rossini et al., 2013), where it is reported that chlorine present in the tomato processing residues is mainly concentrated in the peel fraction.
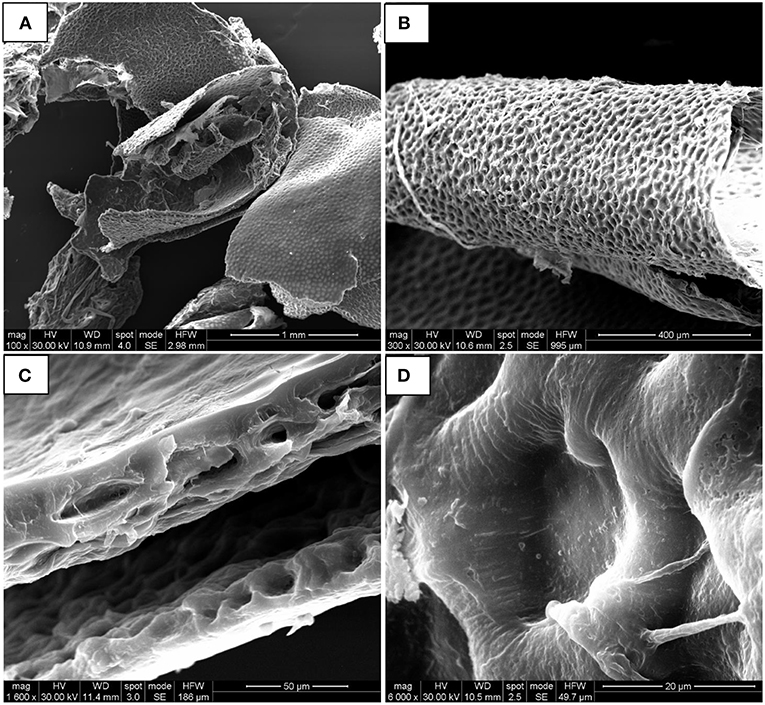
Figure 1. Scanning electron microscopy (SEM) micrographs of tomato peels at 100× (A), 300× (B), 1,600× (C), and 6,000× (D) magnification.
Properties of Pure and Composite TP-Based Films
Carvacrol molecules inside and/or outside HNTs are very difficult to be accurately determined. The evaluation of the degradation temperatures of the HNTs, carvacrol and HNTs-carvacrol, through TGA analysis, can be used to give an indication about the molecules' intercalation, as elsewhere demonstrated (Gorrasi, 2015; Biddeci et al., 2016; Hendessi et al., 2016). Figure 2 reports the weight (%) (TG) (A) and derivative weight (DTG) (B) analysis performed on the HNTs, carvacrol and the nano-hybrid HNTs-carvacrol. Data show that halloysite exhibits a degradation step at about 500°C, which can be attributed to the dehydroxylation of its matrix (Horvath et al., 2003). The thermal degradation of carvacrol occurs in one step centered at 170°C. A shift toward higher values for the carvacrol degradation temperature, centered at 195°C, is clearly evident for the nano-hybrid. The observed delay in the thermo-oxydative degradation of carvacrol molecules could be attributed to two different phenomena, namely: (i) a reduced oxygen permeability due to the barrier effect produced by fillers building a tortuous path (Monsiváis-Barrón et al., 2014), or (ii) the fact that large part of carvacrol was successfully entrapped into the nanotubes, and the degradation of the active molecule probably occurs at higher temperature, with respect to the free molecules only after a spillage from the tubes.
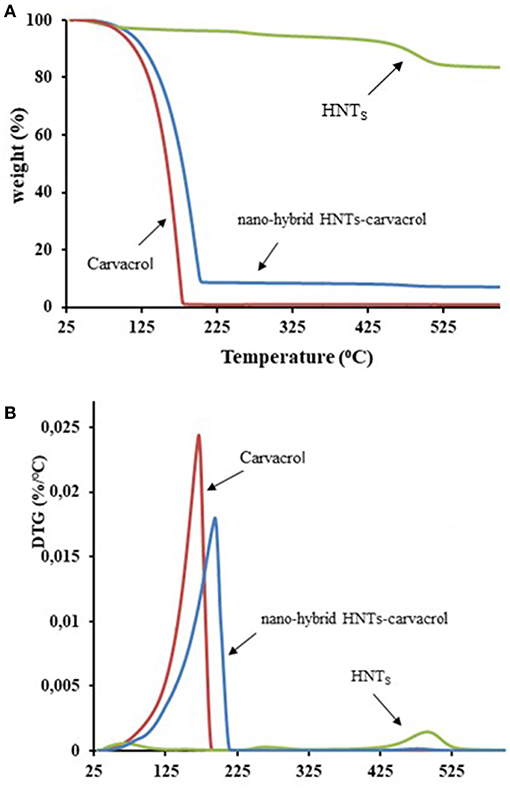
Figure 2. Weight (%) (TG) (A) and derivative weight (%/°C) DTG (B) on the HNTs (), carvacrol (
), and the nano-hybrid HNTs-carvacrol (
).
Figure 3 shows the XRD of composites' films and unfilled film based on TPs. Data reveal the presence of an amorphous structure in all of the sample, as evidenced by the presence of a broad halo centered at 2θ ≅ 21°. The amorphous structure of the matrix is retained in all the composites obtained at different filler load. The inset of Figure 3 shows the XRD spectrum of dehydrated HNTs, with the typical d-spacing at 7.3 Å, that represents the first order (001) basal reflection (Hillier et al., 2016). Such peak is not visible in the composites. It may be possible that during the milling process, in the reported experimental conditions, a part of the first order basal reflection of HNTs is partially de-structured, or such peak may be hidden under the main peak of the TPs' matrix.
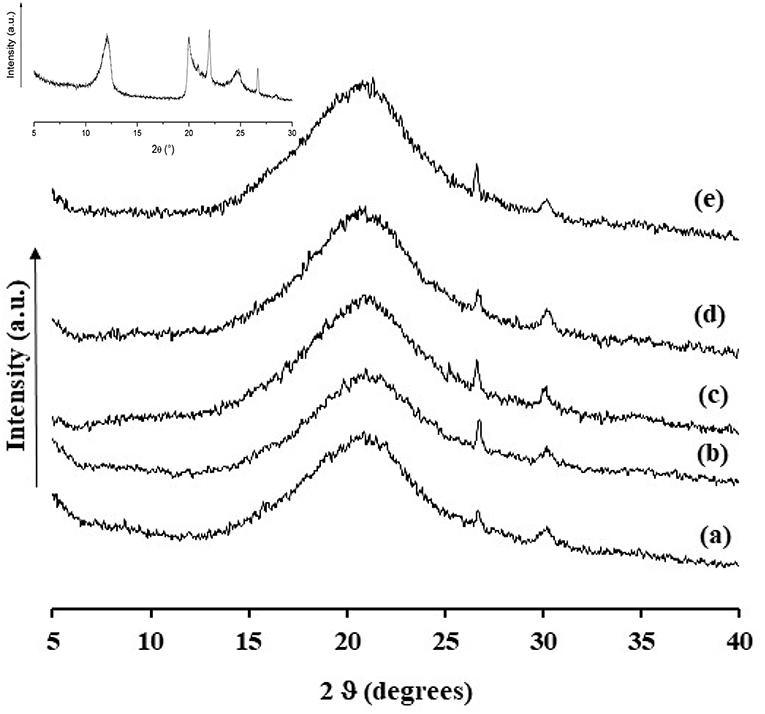
Figure 3. XRD analysis evaluated on films of TPs100HNTs-carv0 (a), TPs97.5HNTs-carv2.5 (b), TPs95HNTs-carv5 (c), TPs90HNTs-carv10 (d), TPs80HNTs-carv20 (e); Inset: XRD of HNTs.
Figure 4A shows the TGA and DTG (Figure 4B) analysis evaluated on composites films. Unfilled film made of tomato peels was analyzed for comparison. It is suggested that the pyrolysis of the investigated tomato processing residue is a rather complex process occurring in several stages as it is clearly reflected by the presence of several DTG peaks (Figure 4B), which are also reflected in less noticeable changes in the slope of the TG curves. The complicated thermal behavior exhibited by tomato peels is a likely consequence of its very complex chemical composition, which is characterized by the presence of several macro-components (i.e., cellulose, hemicelluloses, and lignin, see Table 1) and minor constituents (e.g., lipids, waxes, proteins, oil, etc.) in different percentages (Lazos and Kalathenos, 1988; Knoblich et al., 2005). The negligible weight loss (i.e., approximately 7 wt%) observed at a temperature lower than 200°C can be attributed to the removal of moisture and to the start of polysaccharides hydrolysis, whereas, the second and third decomposition stage can be most likely ascribed to the thermal degradation of hemicelluloses and cellulose. However, a clear attribution of all the decomposition events or peaks to a specific chemical species appeared to be hardly achieved when analyzing the DTG profiles only, because mass losses of several minor chemical components probably occurred during each step at the same time. It is evident that all degradation temperatures decrease with the filler loading. As already found in the case of pectins/HNTs-essential oil (Gorrasi, 2015), we hypothesize that the decomposition of volatile products due to oxidation phenomena, can have a synergistic effect in determining the thermal decomposition of the materials.
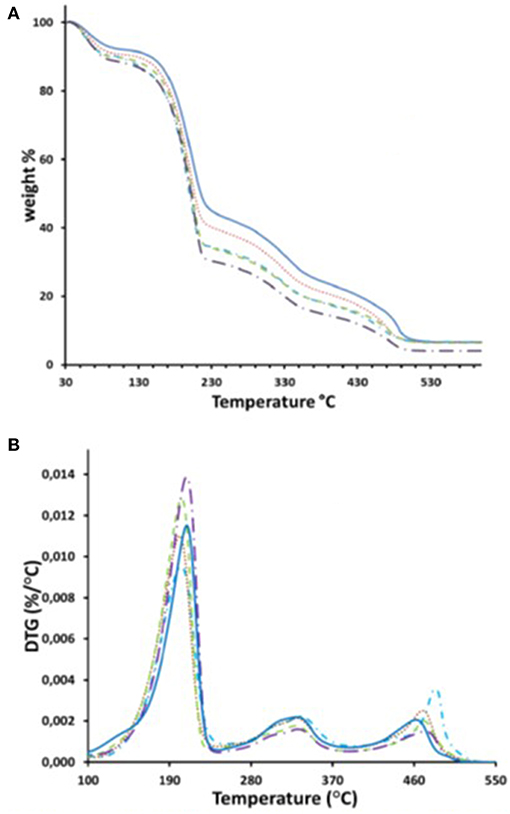
Figure 4. (A) TGA and (B) DTG analysis evaluated on TPs100HNTs-carv0 (), TPs97.5HNTs-carv2.5 (
), TPs95HNTs-carv5 (
), TPs90HNTs-carv10 (
), TPs80HNTs-carv20 (
).
The stress-strain curves of tomato peels film and composites, which were not reported, allowed for evaluation of the mechanical parameters of the samples. In particular, the elastic modulus, E (MPa), the stress at break point, σbreak (MPa), and the elongation at break, εbreak (mm/mm%) were evaluated. The mechanical parameters are reported in Figure 5. The elastic modulus (MPa) tends to decrease with the filler content, and also the stress at break (MPa). HNTs filler, having an inorganic nature, is incompatible with the organic matrix. It is worth to note that they were dispersed into the organic matrix with no chemical treatment on the surface. The lowering of the mechanical properties is mainly due to this difference in nature of both components. A “disconnection” between the two phases is then evidenced from the mechanical response. Experimental results evidenced that the higher is the filler loading, the higher the degree of separation appears between the two phases.
The values of elongation at break point (mm/mm%) is not significantly modified with the filler loading, also at high percentages (i.e., 10 and 20 wt%). This can be due to a plasticizing effect of the carvacrol oil with respect to the matrix, which helps to prevent the loss of mechanical consistence of the samples.
Figure 6 reports the sorption of water vapor, as Ceq (wt%), as a function of the filler loading for all the obtained composites and the unfilled sample at relative moistures 24, 55, and 80%. Data are reported in Table 3. As expected, the sorption of water increases with the moisture increasing. The presence of hydrophilic HNTs, even at a high percentage, does not dramatically increase the hydrophilicity of the materials, as found for other biodegradable materials filled with HNTs (Gorrasi, 2015; Bugatti et al., 2017). This may be due to the presence of hydrophobic carvacrol around the HNTs that can act as hydrophobic coating.
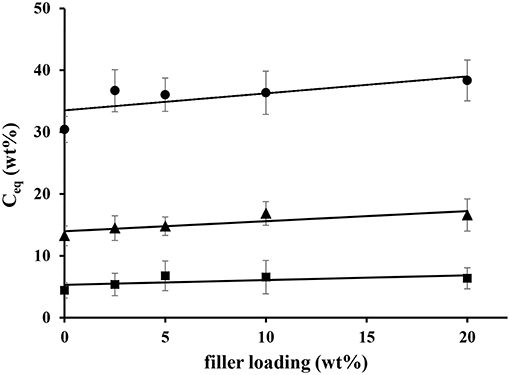
Figure 6. Sorption of water vapor, as Ceq (wt%), as function of the filler loading at relative moistures 23% (), 55% (
), and 80% (
).
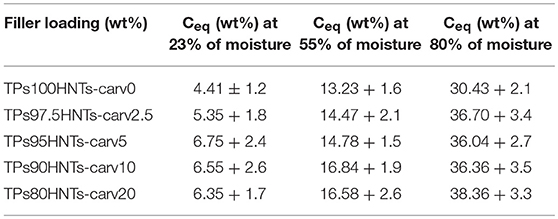
Table 3. Ceq (wt%) of water vapor sorbed at different moisture for unfilled TPs film and composites.
To study the ability of the considered composites to release carvacrol, measurements of controlled release in ethanol were conducted. In particular, Figure 7 reports the release fraction of carvacrol (wt%), as function of contact time (h). It is evident that, in all cases, after 1 month no complete release occurred for any sample. A faster release occurs within the first hour, probably due to the release of carvacrol on the surface of the films, then the release rate decreases over time. The carvacrol release fraction (wt%) decreases with filler loading. This result can be interpreted in terms of lowered counter-diffusion of the carvacrol molecules from inside the matrix to the release medium. The dispersed HNTs increases to a large extent the tortuosity of the system, leading to a decrease in the rate of diffusion of carvacrol molecules. This result demonstrates that HNTs loaded carvacrol, embedded into the considered bio-based matrix, act as nano-containers that allow the sustained release of the active molecule. This could result in a longer-lasting antibacterial effect.
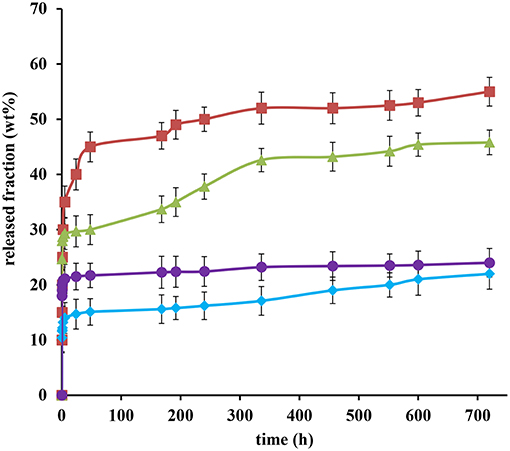
Figure 7. Release fraction of carvacrol (wt%), as function of contact time (h) for TPs97.5HNTs-carv2.5 (), TPs95HNTs-carv5 (
), TPs90HNTs-carv10 (
), TPs80HNTs-carv20 (
).
Concluding Remarks
This paper demonstrated how mechanical milling is a valuable technology to obtain green nanocomposites based on a largely available waste material, like tomato peels (TPs), and halloysite nanotubes (HNTs) filled with a natural antimicrobial, like carvacrol.
• XRD analysis on the composites showed that the TPs matrix presents an amorphous structure, which is retained in the composites at any filler loading. The basal peak of dehydrated HNTs tends to disappear and/or to be expanded after the intercalation of carvacrol, and the ball milling treatment with TPs in presence of water.
• TG-DTG analysis enabled us to hypothesize that the carvacrol molecules inside HNTs delay the thermo-oxydation, either for a barrier effect to oxygen inducted from the HNTs, or because some molecules were successfully loaded into the nanotubes. The degradation of the TPs matrix into the composites decreases with the filler loading. It was hypothesized that the decomposition of volatile products, due to oxidation phenomena, can have a synergistic effect in determining the thermal decomposition of the materials.
• Mechanical properties showed a decrease, or at least a maintenance, of all the mechanical parameters. It worth to note that natural HNTs were incorporated into the TPs matrix with no chemical treatment.
• The sorption of water vapor was evaluated at relative moistures 24, 55, and 80%. Data show that it increases with increasing the moisture. The presence of highly hydrophilic HNTs, even at high percentage, does not dramatically increase the hydrophilicity of the materials. This may be due to the presence of hydrophobic carvacrol around the HNTs that can act like an hydrophobic coating.
• The sustained release of carvacrol, in vitro, was studied as function of time. The carvacrol release fraction (wt%) decreases with filler loading and after 1 month no complete release is evident for any composite. The presence of the dispersed HNTs increasing the tortuosity of the system, led to a large decrease in the rate of counter-diffusion of carvacrol molecules from the TPs matrix. This result allows us to hypothesize the application of such materials in food packaging field, where it is needed longer-lasting antibacterial effect.
Author Contributions
GG conceived the paper. GV, VB, and PB performed the experiments. GG, GV, VB, and PB interpreted the results. GG and PB wrote the paper.
Funding
This work was supported by the project High Performing Advanced Material Platform for Active and Intelligent Food Packaging: Cronogard™ (H2020-SMEINST-2-2016-2017). Grant agreement n. 783696.
Conflict of Interest Statement
The authors declare that the research was conducted in the absence of any commercial or financial relationships that could be construed as a potential conflict of interest.
Acknowledgments
We are grateful to Dr. Luciano Cortese (IRC-CNR) and Dr. Fernando Stanzione for providing expertise and access to their SEM/EDX and ICP-MS equipments.
References
Al Khraisat, A. A. A. (2015). Evaluation of metabolizable nutrient values of Tomato Pomace (TP) in Nile tilapia (Oreochromis niloticus). Int. J. Fisc. Aquat. Sci. 2, 164–167. Available online at: http://www.fisheriesjournal.com/vol2issue4/Pdf/2-4-7.1.pdf
Bellur, S. R., Coronella, C. J., and Vàsquez, V. R. (2009). Analysis of biosolids equilibrium moisture and drying. Environ. Prog. Sustain. Energy 28, 291–298. doi: 10.1002/ep.10353
Biddeci, G., Cavallaro, G., Di Blasi, F., Lazzara, G., Massaro, M., Milioto, S., et al. (2016). Halloysite nanotubes loaded with peppermint essential oil as filler forfunctional biopolymer film. Carbohydr. Polym. 152, 548–557. doi: 10.1016/j.carbpol.2016.07.041
Brachi, P., Chirone, R., Miccio, F., Miccio, M., Picarelli, A., and Ruoppolo, G. (2014). Fluidized bed co-gasification of biomass and polymeric wastes for a flexible end-use of the syngas: focus on bio-methanol. Fuel 128, 88–98. doi: 10.1016/j.fuel.2014.02.070
Brachi, P., Miccio, F., Miccio, M., and Ruoppolo, G. (2016a). Pseudo-component thermal decomposition kinetics of tomato peels via isoconversional methods. Fuel Process. Technol. 154, 243–250. doi: 10.1016/j.fuproc.2016.09.001
Brachi, P., Miccio, F., Miccio, M., and Ruoppolo, G. (2016b). Torrefaction of tomato peel residues in a fluidized bed of inert particles and a fixed-bed reactor. Energy Fuel 30, 4858–4868. doi: 10.1021/acs.energyfuels.6b00328
Bugatti, V., Sorrentino, A., and Gorrasi, G. (2017). Encapsulation of Lysozyme into halloysite nanotubes and dispersion in PLA: structural and physical properties and controlled release analysis. Europ Polymer J. 93, 495–506. doi: 10.1016/j.eurpolymj.2017.06.024
Cavallaro, G., Donato, D. I., Lazzara, G., and Milioto, S. (2011). Films of halloysite nanotubes sandwiched between two layers of biopolymer: from themorphology to the dielectric, thermal, transparency, and wettability properties. J. Phys. Chem. C 115, 20491–20498. doi: 10.1021/jp207261r
Cavallaro, G., Lazzara, G., and Milioto, S. (2013). Sustainable nanocomposites based on halloysite nanotubes and pectin/polyethylene glycol blend. Polymer Degrad. Stab. 98, 2529–2536. doi: 10.1016/j.polymdegradstab.2013.09.012
Delogu, F., Gorrasi, G., and Sorrentino, A. (2017). Fabrication of polymer nanocomposites via ball milling: present status and future perspectives. Prog. Mater. Sci. 86, 75–126. doi: 10.1016/j.pmatsci.2017.01.003
Eghbal, N., and Choudhary, R. (2018). Complex coacervation: encapsulation and controlled release of active agents in food systems. LWT-Food Sci. Tech. 90, 254–264. doi: 10.1016/j.lwt.2017.12.036
Fakhrullina, G. I., Akhatova, F. S., Lvov, Y. M., and Fakhrullin, R. F. (2015). Toxicity of halloysiteclay nanotubes in vivo: a Caenorhabditis elegans study. Environ. Sci. Nano 2, 54–59. doi: 10.1039/C4EN00135D
Giosafatto, C. V., Di Pierro, P., Gunning, A. P., Mackie, A., Porta, R., and Mariniello, L. (2014b). Trehalose-containing hydrocolloid edible films prepared in the presence of transglutaminase. Biopolymer 101, 931–937. doi: 10.1002/bip.22477
Giosafatto, C. V., Di Pierro, P., Gunning, P., Mackie, A., Porta, R., and Mariniello, L. (2014a). Characterization of citrus pectin edible films containing transglutaminase-modified phaseolin. Carbohydrate Polym. 106, 200–208. doi: 10.1016/j.carbpol.2014.02.015
Gorrasi, G. (2015). Dispersion of halloysite loaded with natural antimicrobials intopectins: characterization and controlled release analysis. Carbohydrate Polym. 127, 47–53. doi: 10.1016/j.carbpol.2015.03.050
Gorrasi, G., Attanasio, G., Izzo, L., and Sorrentino, A. (2017). Controlled release mechanisms of sodium benzoate from a biodegradable polymer and halloysite nanotube composite. Polymer Intern. 66, 690–698. doi: 10.1002/pi.5309
Gorrasi, G., and Bugatti, V. (2016). Edible bio-nano-hybrid coatings for food protection based on pectins and LDH-salicylate: preparation and analysis of physical properties. LWT-Food Sci. Technol. 69, 139–145. doi: 10.1016/j.lwt.2016.01.038
Gorrasi, G., Bugatti, V., and Vittoria, V. (2012). Pectins filled with LDH-antimicrobial molecules: preparation, characterization and physical properties. Carbohydrate Polym. 89, 132–137. doi: 10.1016/j.carbpol.2012.02.061
Gorrasi, G., Romeo, V., Sannino, D., Sarno, M., Ciambelli, P., Vittoria, V., et al. (2007). Carbon nanotube induced structural and physical property transitions of syndiotactic polypropylene. Nanotechnology 18:275703. doi: 10.1088/0957-4484/18/27/275703
Gorrasi, G., and Sorrentino, A. (2015). Mechanical milling as a technology to produce structural and functional bio-nanocomposites. Green Chem. 17, 2610–2625. doi: 10.1039/C5GC00029G
Gorrasi, G., and Vertuccio, L. (2016). Evaluation of zein/halloysite nano-containers as reservoirs of active molecules for packaging applications: preparation and analysis of physical properties. J. Cereal Sci. 70, 66–71. doi: 10.1016/j.jcs.2016.05.008
Hendessi, S., Sevinis, E. B., Unal, S., Cebecia, F. C., Menceloglu, Y. Z., and Unal, H. (2016). Antibacterial sustained-release coatings from halloysite nanotubes/waterborne polyurethanes. Pro. Org. Coat. 101, 253–261. doi: 10.1016/j.porgcoat.2016.09.005
Hillier, S., Brydson, R., Delbos, E., Fraser, T., Gray, N., Pendlowski, H., et al. (2016). Correlations among the mineralogical and physical properties of halloysite nanotubes (HNTs). Clay Minerals 51, 325–350. doi: 10.1180/claymin.2016.051.3.11
Horvath, E., Frost, R. L., Mako, E., Kristof, J., and Cseh, T. (2003). Thermal treatment of mechano-chemically activated kaolinite. Thermochim. Acta 404, 227–235. doi: 10.1016/S0040-6031(03)00184-9
Kam, W. Y. J., Mirhosseini, H., Abas, F., Hussain, N., Hedayatnia, S., and Chong, H. L. F. (2018). Antioxidant activity enhancement of biodegradable film as active packaging utilizing crude extract from durian leaf waste. Food Control 90, 66–72. doi: 10.1016/j.foodcont.2018.02.036
Kirwan, M. J., and Strawbridge, J. W. (2003). “Plastics in food packaging,” in Food Packaging Technology, eds R. Coles, D. McDowell, and M. J. Kirwan (Oxford: Blackwell Publishing, CRC Press), 158–160.
Knoblich, M., Anderson, B., and Latshaw, D. (2005). Analyses of tomato peel and seed byproducts and their use and a source of carotenoids. J. Sci. Food Agric. 85, 1166–1177. doi: 10.1002/jsfa.2091
Lazos, E. S., and Kalathenos, P. (1988). Technical note: composition of tomato processing wastes. Int. J. Food Sci. Technol. 23, 649–665. doi: 10.1111/j.1365-2621.1988.tb01052.x
Lvov, Y., Wang, W., Zhang, L., and Fakhrullin, R. (2016). Halloysite clay nanotubes for loading and sustained release of functional compounds. Adv. Mater. 28, 1227–1250. doi: 10.1002/adma.201502341
Lvov, Y. M., Shchukin, D. G., Möhwald, H., and Price, R. R. (2008). Halloysite clay nanotubesfor controlled release of protective agents. ACS Nano 2, 814–820. doi: 10.1021/nn800259q
Mangut, V., Sabio, E., Gañán, J., González, J. F., Ramiro, A., González, C. M., et al. (2016). Thermogravimetric study of the pyrolysis of biomass residues from tomato processing industry. Fuel Process. Technol. 87, 109–115. doi: 10.1016/j.fuproc.2005.08.006
Massaro, M., Lazzara, G., Milioto, S., Noto, R., and Riela, S. (2017). Covalently modified halloysite clay nanotubes: synthesis, properties, biological and medical applications. J. Mater. Chem. B 5, 2867–2882. doi: 10.1039/C7TB00316A
Monsiváis-Barrón, A. J., Bonilla, J., and Sánchez-Fernández, A. (2014). Property relationship in organosilanes and nanotubes filled hybrid composites. Materials 7, 7073–7092. doi: 10.3390/ma7107073
Rossini, G., Toscano, G., Duca, D., Corinaldesi, F., Foppa Pedretti, E., and Riva, G. (2013). Analysis of the characteristics of the tomato manufacturing residues finalized to the energy recovery. Biomass Bioenergy 51, 177–182. doi: 10.1016/j.biombioe.2013.01.018
Saha, N. R., Sarkar, G., Roy, I., Rana, D., Bhattacharyya, A., Adhikari, A., et al. (2016). Studies on methylcellulose/pectin/montmorillonite nanocomposite films and their application possibilities. Carbohydrate Polym. 136, 1218–1227. doi: 10.1016/j.carbpol.2015.10.046
Scarfato, P., Avallone, E., Incarnato, L., and Di Maio, L. (2016). Development and evaluation of halloysite nanotube-based carrier for biocide activity in construction materials protection. Appl. Clay Sci. 132–133, 336–342. doi: 10.1016/j.clay.2016.06.027
Silva, Â., Duarte, A., Sousa, S., Ramos, A., and Domingues, F. C. (2016). Characterization and antimicrobial activity of cellulose derivatives films incorporated with a resveratrol inclusion complex. LWT-Food Sci. Technol. 73, 481–489. doi: 10.1016/j.lwt.2016.06.043
Templeton, D. W., Wolfrum, E. J., Yen, J. H., and Sharpless, K. E. (2014). Compositional analysis of biomass reference materials: results from an interlaboratory study. Bioenergy Res. 9, 303–314. doi: 10.1007/s12155-015-9675-1
Tomato News (2014). Tomato Processing Industry. Available online at: http://www.tomatonews.com/resources.html (Accessed June 26, 2015).
Toscano, G., Pizzi, A., Foppa Pedretti, E., Rossini, G., Ciceri, G., Martignon, G., et al. (2015). Torrefaction of tomato industry residues. Fuel 143, 89–97. doi: 10.1016/j.fuel.2014.11.039
Trends, N., Rhim, J. W., Park, H. M., and Ha, C. S. (2013). Bio-nanocomposites for food packaging applications. Prog. Polymer Sci. 38, 1629–1652. doi: 10.1016/j.progpolymsci.2013.05.008
Vasquez, V. R., and Coronella, C. J. (2009). A simple model for vapor-moisture equilibrium in biomass substrates. AIChE J. 55, 1595–1603. doi: 10.1002/aic.11762
Vergaro, V., Abdullayev, E., Lvov, Y. M., Zeitoun, A., Cingolani, R., Rinaldi, R., et al. (2010). Cytocompatibility uptake of halloysite clay nanotubes. Biomacromolecules 11, 820–826. doi: 10.1021/bm9014446
Keywords: tomato peels, halloysite, green composites, carvacrol, packaging
Citation: Bugatti V, Brachi P, Viscusi G and Gorrasi G (2019) Valorization of Tomato Processing Residues Through the Production of Active Bio-Composites for Packaging Applications. Front. Mater. 6:34. doi: 10.3389/fmats.2019.00034
Received: 19 December 2018; Accepted: 14 February 2019;
Published: 08 March 2019.
Edited by:
Yu Dong, Curtin University, AustraliaReviewed by:
Kenan Song, Massachusetts Institute of Technology, United StatesLiqing Wei, Forest Products Laboratory, United States Department of Agriculture USDA, United States
Copyright © 2019 Bugatti, Brachi, Viscusi and Gorrasi. This is an open-access article distributed under the terms of the Creative Commons Attribution License (CC BY). The use, distribution or reproduction in other forums is permitted, provided the original author(s) and the copyright owner(s) are credited and that the original publication in this journal is cited, in accordance with accepted academic practice. No use, distribution or reproduction is permitted which does not comply with these terms.
*Correspondence: Giuliana Gorrasi, ggorrasi@unisa.it