- 1Department of Mechanical and Manufacturing Engineering, Universiti Putra Malaysia, Serdang, Malaysia
- 2Laboratory of Biocomposite Technology, Institute of Tropical Forestry and Forest Products, Universiti Putra Malaysia, Serdang, Malaysia
- 3Department of Aerospace Engineering, Faculty of Engineering, Universiti Putra Malaysia, Serdang, Malaysia
- 4Aerospace Manufacturing Research Centre (AMRC), Faculty of Engineering, Universiti Putra Malaysia, Serdang, Malaysia
- 5Science and Technology Research Institute for Defence, Kuala Lumpur, Malaysia
Kevlar 29 is the most widely used synthetic fiber for body armor applications and they have been derived from petroleum based resources. Depletion of petroleum resources and the increase in awareness about the eco-friendly materials encouraged the researchers to explore the potential use of natural fiber as an alternative for synthetic fibers. Hybridization of natural fiber with synthetic fiber will result in unique properties which is difficult to obtain from the individual fibers. In this research Analytical Hierarchy Process (AHP) was used to identify the most suitable natural fiber to be hybridized with Kevlar 29 fiber as a reinforcement in the polymer composites for personal body armor. Fourteen natural fibers and seven criteria's were selected and analyzed for hybridization with respect to the personal body armors design specification. Cocos nucifera sheath which is a naturally woven fiber yields the highest priority vector and it was selected as a most promising natural fiber for hybridization with Kevlar 29 for personal body armor. Eventually, sensitivity analysis was carried out to check the stability of the priority ranking.
Introduction
In olden days, the soldiers wore defensive covering to protect the body from physical attack. Ancient body armors were made by using animal hide or plant fiber (made from cotton, thatch, and silk in woven mat). After the arrival of metallic materials, the personal body armors were manufactured as a plate by using iron, copper, and steel (Hani et al., 2016). The twentieth century innovations in material science and manufacturing advancement led to the discovery of man-made synthetic textile materials (such as fiberglass, nylon, Kevlar, etc.) that have provided personal body armor with improved ballistic resistance. Also the density of these advanced synthetic fibers is less than the metallic materials (Cavallaro, 2011). Now a day's aramid fiber (Kevlar 29) reinforced polymer composites have been widely used in personal body armor against ballistic and blast threats (Yahaya et al., 2014). Though aramid fiber composites have higher specific strength, corrosion resistance and impact strength, most of the fibers are manufactured from petroleum based resources. Depletion of petroleum resources encouraged the researchers to explore the potential use of plant fiber as an alternative natural reinforcement to polymeric composites. Natural fibrous material is a potential resource in textile and other industries (Jiang et al., 2017). As researchers focused on green composites, more number of researches focused on replacement of synthetic fibers with natural fibers. The main advantage of hybrid composites lie in the ability to combine the advantages of their individual constituents. Moreover, it is an effective way of reducing the overall material cost.
In advanced materials research, selection of suitable candidate material for a particular application has become increasingly crucial to achieve both customer satisfaction and eco-friendly design. Multi criteria decision making technique is widely used to identify the most suitable materials for a particular application (Al-oqla et al., 2015a,b). Among the multi-criteria decision-making techniques (WPIM, TOPSIS, and AHP), AHP was extensively used in various material selection scenarios (Mansor et al., 2013). It was developed in the 70's by Thomas (Da silva neves and Camanho, 2015). Generally, AHP is used to select the most suitable alternative for a particular application or project. AHP process starts with goal setting followed by criteria's and alternatives selection. The final ranking of the alternatives mainly depends upon the criteria weightage and the relative importance of the alternatives. The plant fiber for vehicle spall liner was selected using AHP (Yahaya et al., 2014). Seven criteria's and 14 natural fibers were taken into account. They have chosen kenaf as a potential natural fiber for hybridization with Kevlar fiber for vehicle spall liner. The natural fiber for automotive dash board application was selected using AHP (Sapuan et al., 2011). They have considered mechanical and physical properties of the natural fiber as the criteria during the selection process. Apart from material selection, AHP was utilized to select the best design among the innovative design concepts for the polymer composites for automotive brake lever applications (Mansor et al., 2014). Some studies have utilized both AHP and TOPSIS to choose the best ceramic waste as a replacement for conventional concrete with respect to compressive strength and environmental impacts (Rashid et al., 2017). AHP was used to choose the best alternative fuel with respect to cost and policy aspect for the Greek road transport (Tsita and Pilavachi, 2012). Dweiri et al. (2016) proposed a decision support model to identify the efficient supplier in the automobile industry using AHP. Moreover, they have evaluated their decision using sensitivity analysis. Sensitivity analysis validates the AHP results with different criteria weightage (Chang et al., 2007). AHP can be implemented effectively by using the “Expert Choice” software which is available commercially (Dalalah et al., 2010; Hani et al., 2012; Erdogan et al., 2017).
In this research, AHP Method was employed to select the most suitable natural or plant fiber for hybridization with Kevlar 29 for personal body armor application. Commercially available AHP software “expert choice 11.5” (Expert choice, Inc. Arlington, VA) was used for fiber selection. The product design specification for the personal body armor were formulated based on the sustainability requirement and performance. The criteria for selection are as follows: fiber orientation, cellulose, cost, availability, density, tensile strength and young's modulus. During the material selection stage, 14 natural fibers have been chosen. The pairwise comparison matrices of 14 alternatives have been formulated with respect to seven criteria's. Then pairwise comparison matrix (PCM) of seven criteria's was formulated with respect to the main goal. The pairwise comparison data were synthesized to obtain the rank of candidate materials with respect to different criteria's and the overall rank with respect to the objective. The consistency ratio were also verified to check the degree of consistency for the judgments as it should not exceed 0.10. Eventually, sensitivity analysis was performed to simulate the results while varying the criteria's weightage. Sensitivity analysis verifies the AHP results with different criteria weightage.
Product Detail
Design Criteria
Personal body armor can be classified into two categories such as soft and hard body armor (Azrin hani abdul et al., 2011). Soft body armor contain multiple layers of fabrics up to 50 layers (Weigh below 4.5 kg). Soft body armor has to withstand the impact of projectile up to 500 m/s (According to National Institute of Justice (NIJ) armor standard). Further, the hard armor has to withstand the impact of the projectile more than 500 m/s (NIJ level IIIA) when worn in conjunction with soft armor vest (NIJ Standards, 2008; Hani et al., 2012).
In order to design the body armor ergonomically, the following factors are important such as: mobility, weight and comfort. Equal distribution of the weight of the body armor over the human body avoids the user fatigue and it will not cause any breathing troubles, especially when the temperature varies abruptly. The challenging task while designing the personal body armor is that the designer need to balance the level of protection required with respect to the particular threat type. Further, the most important factor to be considered during the personal body armor design process is, identification of the specific threat type such as ballistic, stab, etc. For example, body armors designed for ballistic protection required enough yarn mobility in the weave to avoid the premature failure and it is not recommended for stab protection. Whereas, in stab protection the textile should have dense weave pattern so that it can resist the sharp pointed objects.
At present, the soft body armor for ballistic protection contains packs or panels with woven, nonwoven or combination of woven/nonwoven fiber reinforced with polymer matrix. Generally, woven architecture is preferred for ballistic application. This is due to the ballistic resistance for woven arrangement is superior to nonwoven architecture. Further decrease in fiber diameter increases the tenacity of fiber. The stiffness of a fiber is designated by its young's modulus (NIJ Standards, 2008).
The ballistic packs can prevent penetrations according to NIJ threat categories such as: level IIA, II, IIIA, III, and level IV with enough layers of fabrics. The standard armor test velocity for different categories are as follows: Level IIA (373 m/s), level II (436 m/s), level III (448 m/s), level IIIA (847 m/s), and level IV (878 m/s). Ballistic testing determines the materials “ballistic limit” or “limit velocities.” The varieties of ballistic limit velocities include V0, V50, and V100. V0 is the maximum velocity at which no complete penetration will occur. V50 indicates the 50% probability of complete penetration, whereas V100 represents the minimum velocity with 100% probability of complete penetration.
The ballistic performance of the body armor material can be evaluated by using energy absorption (Eabs) and ballistic limit (V50) (Yahaya et al., 2016). Whenever a projectile of mass “m” hits the target with velocity “v” it possess kinetic energy. The kinetic energy of the projectile before and after impact would be termed as impact energy and residual energy. The difference between impact energy and residual energy is called energy absorption. V50 and Eabs can be calculated using the following relation
Where Vs is the striking velocity; Vr is the residual velocity
Energy absorption could be maximized by considering the following fiber factors such as fiber architecture (unidirectional, woven), stacking sequence and stitching patterns. Twenty to Thirty layers of fabric can be used to stop the projectiles fired from handguns. Four plates were used in the releasable body armor vest for the US special operations command as shown in Figure 1.
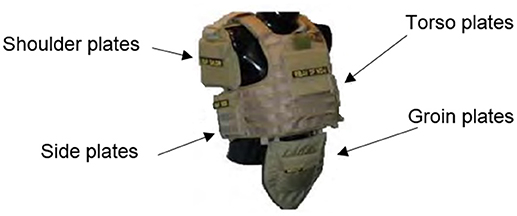
Figure 1. Releasable body armor vest (with permission Cavallaro, 2011).
Overall the primary design criteria or the factors to be considered for the personal body armor material selection are as follows: performance, weight, standards, cost and environmental issues as shown in Table 1.
The main objective of this research is choosing the best alternative natural fiber for hybridization with kevlar29. The limitation of using plant fiber as a hybridizing material is moisture absorption. Cellulose content is the main reason for the hydrophilic nature of the plant fiber. The plant fiber which has more cellulose content will absorb more moisture. Low cellulose content declines the hydrophilic nature of the natural fiber and forms a rough and hard surface which is suitable to absorb the kinetic energy of the projectile.
Hence for this particular personal body armor application while forming the pairwise comparison matrix (PCM) more priority weightage has been given for cellulose content than other criteria's. The next priority has been given for fiber orientation. Because, woven fiber reinforced polymer composites exhibited higher ballistic performance than nonwoven fiber reinforced polymer composites. The next priority was given to density, tensile strength and modulus. Finally, cost and availability was also considered during the natural fiber selection process. Regarding the environmental issues, incorporation of natural fiber will increase the reuse and recyclability, also natural fibers are safer during the disposal of the product.
AHP Methodology
Goal Setting and Hierarchical Frame Work
The Figure 2 shows the general analytical hierarchy process. Initially, the hierarchical framework for the selection of natural fiber was formulated as shown in Figure 3. Level 1 in the hierarchy is the overall goal of the AHP. Seven criteria's were selected based on the personal body amor's design specification as shown in level 2. Fourteen natural fibers were selected as candidate materials for hybridization with Kevlar 29 as shown in level 3.
PCM of Criteria and Synthesizing the Criteria Based on the Natural Fiber Selection
The second stage in the AHP process is formulation of PCM for criteria's based on the relative intensity scale values as shown in Table 2. Then the Eigen vector (w) or the priority vector for the criteria's were synthesized. The Eigen vector can be calculated by using the following relation (Hambali et al., 2010).
Where, w is the Eigen vector or priority vector, ai,j is the relative intensity scale and n is the number of criteria.
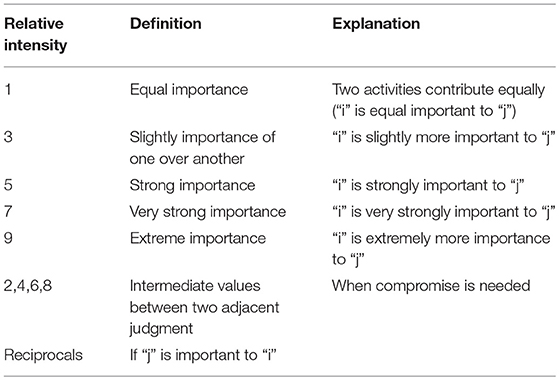
Table 2. Relational data for constructing pair-wise comparisons (Chang et al., 2007).
Consistency Analysis and Consistency Ratio (CR)
The third stage is to calculate the consistency ratio (CR) which measures the consistency of the judgments. If the consistency ratio is less than 0.1 then the judgments are consistent. However, if the Consistency ratio is more than 0.1 then the judgments are untrustworthy and it should be reviewed and improved. Hambali et al. (2010) determined the consistency ratio by using the equations as follows.
Calculation of Principal Eigen Value (λmax)
Where, ai,j is the relative intensity scale and w is the Eigen vector.
Calculation of Consistency Index (CI)
Where, n is the no of criterion.
Calculation of Consistency Ratio(CR)
Where, CI is the consistency index and RI is the Random consistency index. The RI value can be obtained from Table 3.
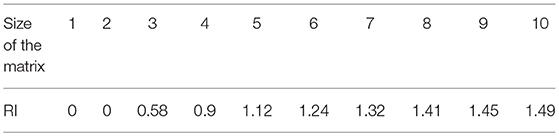
Table 3. Average Random Consistency (Al-harbi, 2001; Dweiri and Al-oqla, 2006).
Formulating PCM of Candidate Materials and Synthesizing the Candidate Materials With Respect to Criteria
The Fourth stage in the AHP process is formulation of PCM for the candidate materials with respect to all the criteria's. Then the Eigen vector (w) for the alternatives for each the criteria's were calculated.
Global Priority Vector
The fifth stage in the analytical hierarchical process is formulation of global priority vector by multiplying priority vector matrix for the candidate materials and priority vector for the criteria.
Evaluation and Selection of Best Alternative
The final stage is identification and selection of best candidate material based on the ranking of global priority vector. The priority vector which scores highest value will be a best alternative material for the proposed personal body armor application.
Importance of Criteria in the Selection of Natural Fiber
Cellulose
Plant fibers would be termed as “natural composites” which contains cellulose fibrils incorporated in the lignin matrix. Cellulose is a natural chemical and it is available in all the natural fibers (Sathishkumar et al., 2014). It is a linear homo polymer made up of D-glucopyranose units interlinked with β- 1, 4 glycosidic bonds. It consists of carbon, hydrogen and oxygen in the following mass percentages 44.44, 6.17, and 49.39%, respectively, (C6H10O5) n is the chemical formula of cellulose. “n” represents the degree of polymerization which indicates the amount of glucose groups ranging from hundreds to tens of thousands or even more. It has been proven that the cellulose consist of repeated dehydrated glucose units. As shown in Figure 4 the repeating unit of cellulose is termed as cellobiose (Chen, 2014).
Among the natural fibers cotton fiber has the maximum cellulose content while the cocos nucifera sheath possess minimum cellulose content as shown in Table 4. All the natural fibers contains cellulose which is the main reason for its hydrophilic nature. Each anhydro-d-glucose element of cellulose contains three alcohol hydroxyls. These hydroxyls forms hydrogen bonding in between the cellulose macromolecules and with the hydroxyl groups which is present in the air (Bledzki et al., 1996). Highest “cellulose content” would increase the hydrophilic nature since the cellulose forms a strong hydrogen bond with the atmospheric air molecules. The natural fiber which has lowest cellulose could not make a strong hydrogen bond with the adjacent cellulose molecules and with the moisture. This makes the surface of the natural fiber rough and exhibits moderate interfacial interactions with the matrix. Moreover, it declines the hydrophilic nature. Higher ballistic limit and energy absorption could be achieved by moderate fiber/matrix adhesion (Yahaya et al., 2016). Hence, moderate fiber/matrix adhesion will efficiently absorb the kinetic energy of the projectile and dissipates the impact energy rapidly away from the impact zone. While formulating PCM maximum relative intensity scale value has been given for the fiber which is having low cellulose content which can absorb the kinetic energy of the projectile and resist the moisture absorption.
Fiber Orientation
Fiber orientation plays a vital role in the ballistic performance of the fiber reinforced polymer composites. It has been proven that woven fibers exhibited higher ballistic performance than the unidirectional and short fiber reinforced polymer composites (Yahaya et al., 2015). Moreover, dense and tightly woven natural fibers would efficiently prevent perforation during ballistic impact (Cavallaro, 2011). Hence, more intensity scale value was given for naturally woven dense fibers like cocos nucifera sheath, sugar palm fibers followed by long fibers (Sisal, banana) (Bisanda and Ansell, 1991) and short fiber (Coir).
Density
Density is one of the major criteria in the fiber selection as it is directly related to the comfort behavior of the personal body armor. Density of the natural fiber significantly affects the strength to weight ratio of the composites. Higher strength to weight ratio of the composites has led to superior mechanical properties. The fibers which had higher density enhances the overall weight of the personal body armor. It severely affects the soldier's movability and needs a lot of human potential to hold the body armor. Hence, it is imperative to identify the alternative natural fiber which is having density almost similar to or less than Kevlar fiber. Table 4 shows the density of the candidate natural fibers and the Kevlar 29. Maximum intensity scale value was given for the fiber which has lowest density. Whereas, least priority value was given to the fiber which has maximum density.
Tensile Strength
The rear side of the ballistic panel is subjected to tensile mode of failure during ballistic impact. Hence, tensile strength is also an important factor in the fiber selection for personal body armor. It is the resistance offered by the material against the externally applied tensile load with respect to area. Generally, the tensile strength of the polymers could be improved with the addition of fiber in the matrix because the fiber has higher strength and stiffness than the matrix. The highest intensity scale value was awarded to the fiber which possess maximum tensile strength. Pineapple leaf fiber holds the maximum tensile strength among the candidate materials which is shown in Table 4.
Tensile Modulus
Tensile modulus is a measure of elasticity. According to hook's law stress is directly proportional to strain within the elastic limit. Moreover, tensile modulus or young's modulus (E) is the ratio between stress and strain. Flax and pine apple leaf fiber had an excellent tensile modulus than other natural fibers and they have been awarded with the highest priority scale value.
Cost
The raw material cost was considered from the literature (Yahaya et al., 2014) and from the commercial online supplier database. Highest priority scale value was given for the natural fiber which possess low cost. However, minimum relative intensity scale value was awarded for “cost” criteria due to the primary design requirement of personal body armor.
Availability
Higher intensity scale value has been given for agro wastes and widely available natural fibers. But while giving the relative intensity sale value, “availability” has been awarded with minimum intensity scale value. This is due to the fact that the technical criteria's such as cellulose, fiber orientation, density, tensile strength and modulus were given more weightage than availability.
Pairwise Comparison Matrix (PCM) of Criteria's and Alternatives
The PCM was formulated using Expert choice v.11.5 software. The aim of the PCM is to identify the relative importance of criteria's as well as alternatives which affects the overall goal of the analytical hierarchical model. The following section will clearly discuss about the methodology of formulating PCM in detail.
PCM of Criteria's
Figure 5A shows the PCM of criteria's with respect to the goal whereas the red colored numbers indicate that the column member (Cellulose) is relatively more important than row member (fiber orientation). Black colored number indicates that the row member (fiber orientation) is relatively more important than column member (cost).The diagonal is kept as 1, since their corresponding row and column remains same. Moreover, the consistency ratio is less than 0.1 which shows that the judgments are consistent.
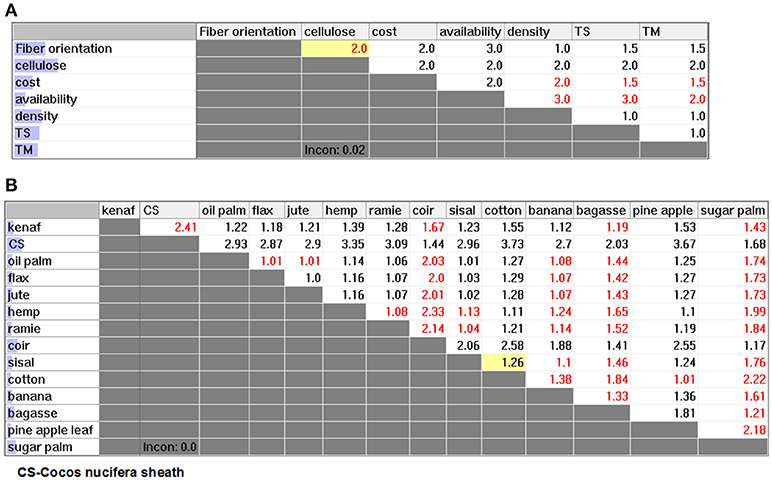
Figure 5. (A) Pairwise comparison matrix of criteria. (B) Pairwise comparison matrix of alternatives with respect to cellulose.
PCM of Alternatives With Respect to Criteria's
Figure 5B, shows the PCM of candidate materials with respect to cellulose. For personal body armor application fibers which possess lowest cellulose content was given more weightage. In the Figure 5B all the diagonals are assigned with 1, since their relative importance remains same. The cellulose content of the candidate materials are given in the Table 4. The process of assigning relative importance scale in the PCM can be explained as follows. The cellulose content of kenaf and cocos nucifera sheaths are 53.4 and 22.2% respectively, and their corresponding ratio is (53.4/22.3) 2.41, which indicates that cocos nucifera sheath is 2.41 times better than kenaf. Similarly other values were assigned in the PCM based on their relative importance with respect to cellulose. Moreover, the consistency ratio is less than 0.1 which proves that the judgments are consistent.
Figure 6A shows the PCM of density. The density of candidate materials are shown in Table 4. All the diagonals are assigned with 1, since their relative importance remains same. The relative importance scale in the matrix were assigned based on their corresponding ratio with respect to density (For example: Density of kenaf is 1.4 kg/m3 and density of Cocos nucifera sheath is 1.3 kg/m3, the density ratio is 1.08). The red colored 1.08 in the pairwise comparison matrix indicate that the column member (Cocos nucifera sheath) has been given more weightage than row member (Kenaf). Similarly, all the values in the PCM were assigned based on their relative importance with respect to density. Moreover, the consistency ratio is less than 0.1, which shows that the judgments are consistent for the alternatives with respect to density.
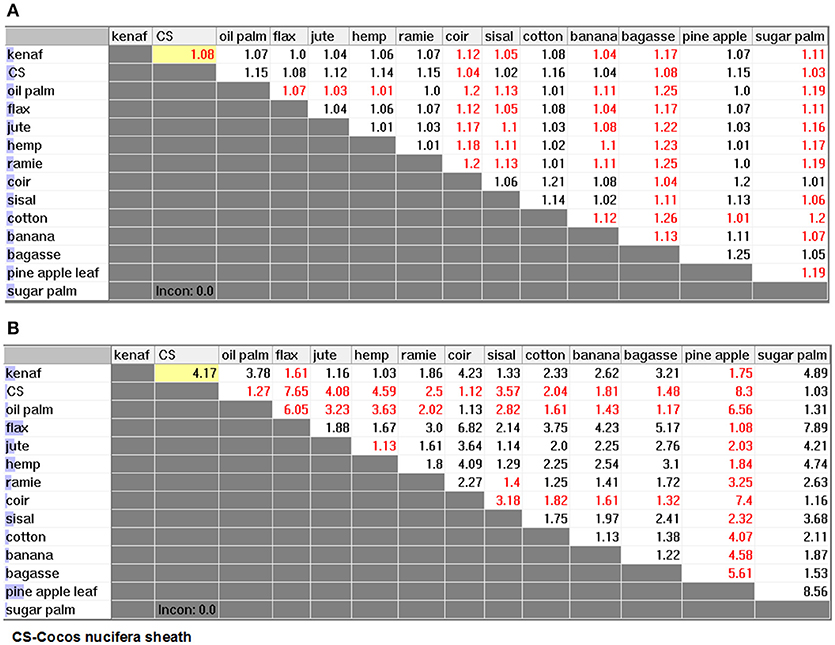
Figure 6. (A) Pairwise comparison matrix of alternatives with respect to density. (B) Pairwise comparison matrix of alternatives with respect to tensile strength.
Figure 6B shows the PCM of tensile strength. Tensile strength of the alternatives are shown in Table 4.The tensile strength of 1st row member (kenaf) and 1st column member (kenaf) remains same, and it was filled with 1(similarly all the diagonals). Tensile strength of 1st row (kenaf) member is 930 MPa and second column member (cocos nucifera sheath) is 196 MPa. Their ratio is 4.17, which indicates that kenaf has been given more weightage than cocos nucifera sheath with respect to tensile strength. Similarly, all other cells were filled with their corresponding ratios. Further, the consistency ratio is less than 0.1, which indicates that the judgments are consistent for the candidate materials with respect to tensile strength.
Similarly, PCM of 14 alternatives with respect to the remaining criteria's (fiber orientation, tensile modulus, cost and availability) were formulated based on the relative intensity values.
Results and Discussion
The Analytical hierarchy process has been carried out using “Expert choice” v.11.5 software. Initially PCM of criteria was formulated. Then pairwise comparison matrix of 14 alternatives with respect to seven criteria's were made. Synthesizing PCM exhibited the priority vector of criteria, and alternatives with respect to seven criteria's. The overall results of the analytical hierarchy process have been verified and validated using sensitivity analysis by changing the weights of the main criteria on the priority ranking of candidate materials.
Synthesizing Pairwise Judgments
Synthesizing Pairwise Judgments of Criteria's
Figure 7A shows the synthesis of criteria with 0.02 consistency. The Eigen vector or priority vector for cellulose is 0.245, which is higher than the other criteria's. The criteria ranking based on the Eigen vector is in the following order, Cellulose > fiber orientation > density > tensile strength > tensile modulus > Cost > availability. From these ranking it is clear that for personal body armor application energy absorption is a primary factor which is directly related to the cellulose content of the natural fiber. Lower cellulose content of the plant fiber would improve the energy absorption and ballistic limit of the ballistic panel. Cost and availability were assigned with least priority vector.
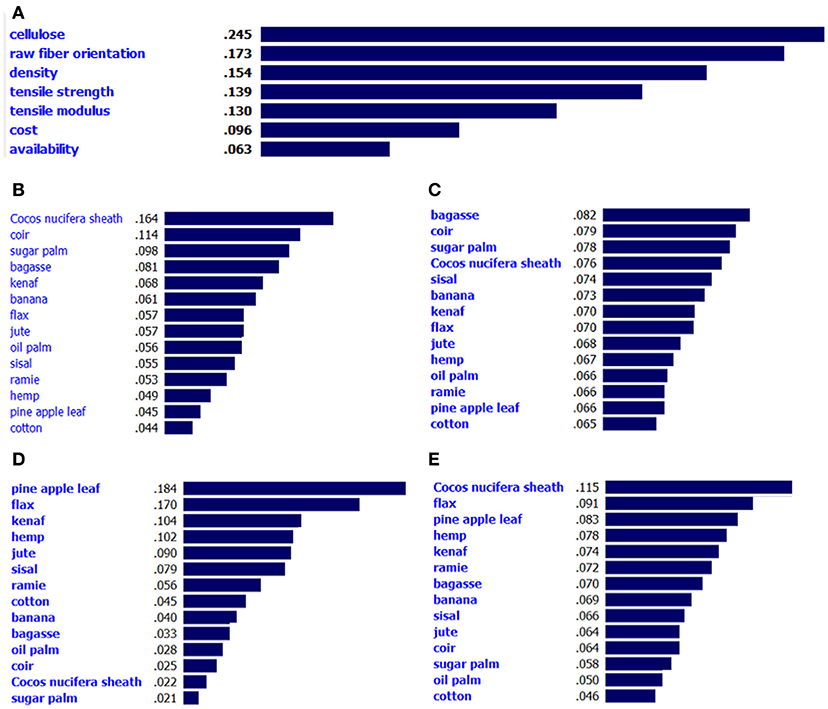
Figure 7. (A) Synthesis with respect to the criteria. (B) Synthesis of alternatives with respect to the cellulose. (C) Synthesis of alternatives with respect to the density. (D) Synthesis of alternatives with respect to the tensile strength. (E) Overall synthesis with respect to the selection of natural fiber.
Synthesizing Pairwise Judgments of Alternatives With Respect to Criteria
Figure 7B shows the synthesis of alternatives with respect to cellulose. Cocos nucifera sheath scored the highest priority value and cotton had the least priority value due to its highest cellulose content. The alternate natural fibers ranking based on the eigen vector with respect to cellulose, is in the following order: Cocos nucifera sheath > Coir > Sugar palm > Bagasse > Kenaf > Banana > Flax > Jute > Oil palm > Sisal > Ramie > Hemp > Pine Apple > Cotton. From this synthesis it has been understood that the fibers which scored least eigen vector seems unfit for the body armor application.
Figure 7C shows the synthesis of candidate material with respect to density. Bagasse fiber scored the highest priority vector and cotton had the least priority vector. But the difference in density of the candidate material is minimum. The alternate natural fibers ranking with respect to density, is in the following order: Bagasse > Coir > Sugar palm > Cocos nucifera sheath > Sisal >Banana > Kenaf >Flax > Jute > Hemp > Oil palm > Ramie > Pine Apple > Cotton. The candidate natural fiber which scored least priority vector with respect to density, seems unfit for personal body armor.
Figure 7D shows the synthesis of candidate materials with respect to tensile strength. Pine apple and flax scored the maximum eigen vector. Sugar palm had minimum tensile strength among the candidate materials. Due to the less cellulose content, oil palm, coir and cocos nucifera sheath has moderate tensile strength than pine apple. The candidate materials ranking with respect to tensile strength, is in the following order: Pine Apple > Flax > Kenaf > Hemp > Jute > Sisal > Ramie > Cotton > Banana > Bagasse > Oil palm > Coir > Cocos nucifera sheath > Sugar palm.
Similarly synthesis of the candidate materials with respect to the remaining criteria's has been evaluated and the overall synthesis results are shown in Figure 7E.
Overall Synthesizing Results
From the overall synthesis as shown in Figure 7E, it was concluded that the cocos nucifera sheath is the most suitable and promising natural fiber to be hybridized with Kevlar 29 for personal body armor, as it scored the maximum priority vector (0.115). Cotton had the least priority vector (0.046). The overall ranking of the alternate natural fibers is in the following order: Cocos nucifera sheath > Flax > Pine Apple > Hemp > Kenaf > Ramie > Bagasse > Banana > Sisal> Jute > Coir > Sugar palm > Oil palm > Cotton. Apart from that, the overall consistency ratio for the natural fiber selection was found to be 0.01 which is within the recommended CR value (<0.10). It has proven that the judgments made during the natural fiber selection process is very consistent and the suggested analysis results are acceptable.
Sensitivity Analysis
The sensitivity analysis of the natural fiber selection was performed using “Expert Choice v 11.5” software. The aim of the sensitivity analysis is to understand the effect of changing weights of the main criteria on the ranking of alternate candidate materials. The overall ranking of the candidate natural fibers are mainly depends on the eigen vectors assigned to the main criteria. Thus, changing the eigen vector of the main criteria (either decreasing and increasing) will change the ranks of the alternative candidate materials (Mansor et al., 2013).
In this research, the priority vector of each criteria was increased by 20% to check the variation on the ranking scenarios of the alternatives. For example the Figures 8–10 shows the change in ranking of the alternatives when the priority vector of the cellulose, density and tensile strength was increased by 20% respectively. Summarized sensitivity analysis results are shown in Table 5. From the sensitivity analysis it has been found that among the seven criteria's except tensile strength and tensile modulus, the remaining five criteria's showed that Cocos nucifera sheath is the most promising natural material for hybridization with Kevlar 29. Therefore, the sensitivity analysis results validates the earlier results.
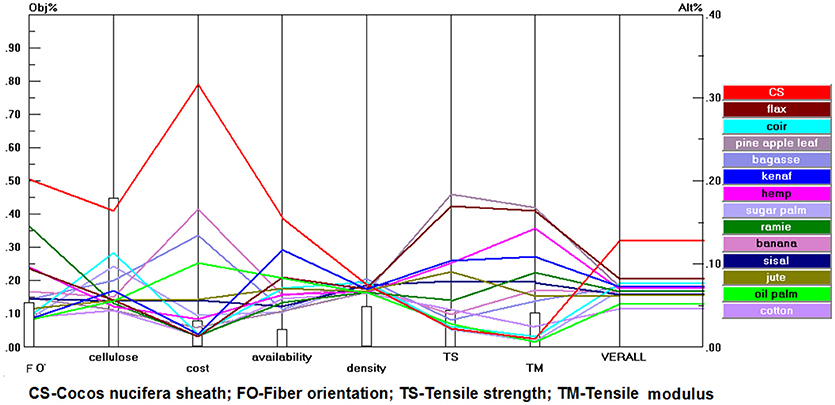
Figure 8. Sensitivity graph of criteria with respect to goal when the priority vector of cellulose is increased by 20% (24.5–44.5%).
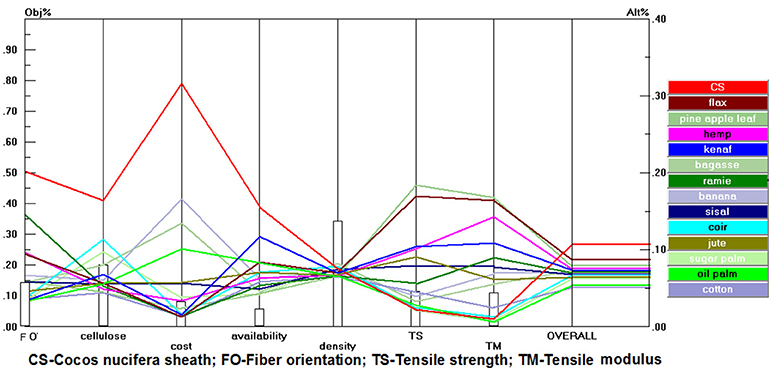
Figure 9. Sensitivity graph of criteria with respect to goal when the priority vector of density is increased by 20% (15.4–35.4%).
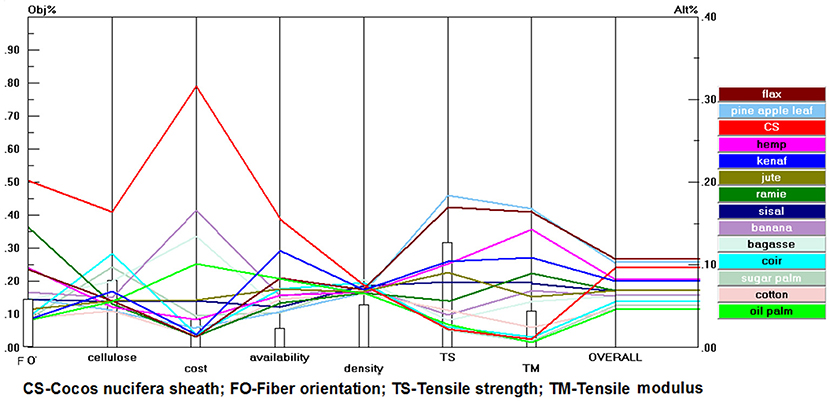
Figure 10. Sensitivity graph of criteria with respect to goal when the priority vector of tensile strength is increased by 20% (13.9–33.9%).
Conclusion
• Selection of the most suitable plant fiber to be hybridized with Kevlar 29 in the polymer composites for personal body armor application was performed using AHP based on the design requirement of the product.
• Through AHP method, naturally woven novel Cocos nucifera sheath was identified as a potential natural fiber which satisfies the design objective and the requirements of personal body armor.
• Consistency analysis was performed to evaluate the degree of consistency of the obtained results and the judgments made during pairwise comparison analysis. The overall consistency ratio for the natural fiber selection was 0.01 which is less than the maximum allowable CR value (<0.10), and it proves that the judgments made during the fiber selection process is very consistent and the suggested analysis results are acceptable.
• From the sensitivity analysis results, it was found that cocos nucifera sheath is the most promising natural fiber to be hybridized with Kevlar 29 fiber as it scored the highest priority vector in five out of seven simulated scenario's, which further validates the results obtained through AHP.
• Overall, AHP method provides a platform to the researchers to find the solution for the multi criteria decision making problems effectively, though it consumes more time while formulating PCM of the alternatives with respect to the criteria as it requires systematic and detailed rating values.
Author Contributions
JN initiated and performed the analytical hierarchy process and wrote the manuscript with input from MJ. MJ collected the candidate materials' properties and provided the analytical tool. EZ collected the design criteria and standards for personal body armor. MS contributed to the sensitivity analysis. RY corrected the manuscript and contributed to making the PCM.
Conflict of Interest Statement
The authors declare that the research was conducted in the absence of any commercial or financial relationships that could be construed as a potential conflict of interest.
Acknowledgments
We are thankful to Ministry of Higher Education for Providing HICOE Grant No: 6369108 to INTROP, UPM for doing this work. The authors are grateful to Malaysian Industry-Government Group for High Technology (MIGHT) for Financial support of this work Under Newton-Ungku Omar Fund Grant No: 6300873 and 6300896.
Abbreviations
PCM, Pairwise comparison matrix; WPIM, Weighted property index method; TOPSIS, Technique for Order Preference by Similarity to Ideal Solution; AHP, Analytical Hierarchy Process; CR, Consistency Ratio; CI, Consistency Index; TS, Tensile strength; TM, Tensile modulus.
References
Al-harbi, K. M. A. (2001). Application of the ahp in project management. Int. J. Proj. Manag. 19, 19–27. doi: 10.1016/S0263-7863(99)00038-1
Al-oqla, F. M., Salit, M. S., Ishak, M. R., and Aziz, N. A. (2015a). Selecting natural fibers for bio-based materials with conflicting criteria. Am. J. Appl. Sci. 12, 64–71. doi: 10.3844/ajassp.2015.64.71
Al-oqla, F. M., Sapuan, S., Ishak, M., and Nuraini, A. (2015b). Decision making model for optimal reinforcement condition of natural fiber composites. Fiber. Polym. 16, 153–163. doi: 10.1007/s12221-015-0153-3
Azrin hani abdul, R., Roslan, A., Jaafar, M., Roslan, M. N., and Ariffin, S. (2011). Mechanical properties evaluation of woven coir and kevlar reinforced epoxy composites. Adv. Mat. Res. 277, 36–42. doi: 10.4028/www.scientific.net/AMR.277.36
Bisanda, E., and Ansell, M. P. (1991). The effect of silane treatment on the mechanical and physical properties of sisal-epoxy composites. Compos. Sci. Technol. 41, 165–178. doi: 10.1016/0266-3538(91)90026-L
Bledzki, A., Reihmane, S., and Gassan, J. (1996). Properties and modification methods for vegetable fibers for natural fiber composites. J. Appl. Polym. Sci. 59, 1329–1336. doi: 10.1002/(SICI)1097-4628(19960222)59:8<1329::AID-APP17>3.0.CO;2-0
Cavallaro, P. V. (2011). Soft Body Armor: An Overview of Materials, Manufacturing, Testing, and Ballistic Impact Dynamics. NUWC-NPT Technical Report. 12,057. Naval Undersea Warfare Center Division Newport.
Chang, C.W., Wu, C.R., Lin, C.T., and Chen, H.C. (2007). An application of ahp and sensitivity analysis for selecting the best slicing machine. Comput. Ind. Eng. 52, 296–307. doi: 10.1016/j.cie.2006.11.006
Chen, H. (ed.) (2014). “Chemical composition and structure of natural lignocellulose,” in Biotechnology of Lignocellulose (Beijing: Springer), 25–71.
Da silva neves, A. J., and Camanho, R. (2015). The use of ahp for it project priorization–a case study for oil & gas company. Proc. Comput. Sci. 55, 1097–1105. doi: 10.1016/j.procs.2015.07.076
Dalalah, D., Al-oqla, F., and Hayajneh, M. (2010). Application of the analytic hierarchy process (ahp) in multi-criteria analysis of the selection of cranes. jordan, J. Mech. Indust. Eng. 4, 567–578
Devi, L. U., Bhagawan, S., and Thomas, S. (1997). Mechanical properties of pineapple leaf fiber-reinforced polyester composites. J. Appl. Polym. Sci. 64, 1739–1748. doi: 10.1002/(SICI)1097-4628(19970531)64:9<1739::AID-APP10>3.0.CO;2-T
Dweiri, F., and Al-oqla, F. M. (2006). Material selection using analytical hierarchy process. Int. J. Comput. Appl. Tech. 26, 182–189. doi: 10.1504/IJCAT.2006.010763
Dweiri, F., Kumar, S., Khan, S. A., and Jain, V. (2016). Designing an integrated ahp based decision support system for supplier selection in automotive industry. Expert Syst. Appl. 62, 273–283. doi: 10.1016/j.eswa.2016.06.030
Erdogan, S. A., Šaparauskas, J., and Turskis, Z. (2017). Decision making in construction management: ahp and expert choice approach. Proc. Eng. 172, 270–276. doi: 10.1016/j.proeng.2017.02.111
Gassan, J., and Bledzki, A. (1996). “Modification methods on nature fibers and their influence on the properties of the composites,” in Technical Papers of the Annual Technical Conference-Society of Plastics Engineers Incorporated (Indianapolis, IN: Society of Plastics Engineers Inc.), 2552–2557.
Hambali, A., Sapuan, S., Ismail, N., and Nukman, Y. (2010). Material selection of polymeric composite automotive bumper beam using analytical hierarchy process. J. Cent. South Univ. 17, 244–256. doi: 10.1007/s11771-010-0038-y
Hani, A., Roslan, A., Mariatti, J., and Maziah, M. (2012). Body armor technology: a review of materials, construction techniques and enhancement of ballistic energy absorption. Adv. Mat. Res. 806–812. doi: 10.4028/www.scientific.net/AMR.488-489.806
Hani, A. A., Azman, N., Ahmad, R., Mariatti, M., Roslan, M., and Marsi, N. (2016). “Ballistic impact response of woven hybrid coir/kevlar laminated composites,” in MATEC Web of Conferences (Phuket), Vol. 78, 01048. doi: 10.1051/matecconf/20167801048
Ishak, M., Leman, Z., Sapuan, S., Rahman, M., and Anwar, U. (2013). Chemical composition and ft-ir spectra of sugar palm (Arenga pinnata) fibers obtained from different heights. J. Nat. Fibers 10, 83–97. doi: 10.1080/15440478.2012.733517
Jawaid, M., and Khalil, H. A. (2011). Cellulosic/synthetic fibre reinforced polymer hybrid composites: a review. Carbohydr. Polym. 86, 1–18. doi: 10.1016/j.carbpol.2011.04.043
Jiang, W., Zhou, C., Han, G., Via, B., Swain, T., Fan, Z., et al. (2017). Classification and identification of plant fibrous material with different species using near infrared technique—a new way to approach determining biomass properties accurately within different species. Front. Plant Sci. 7:2000. doi: 10.3389/fpls.2016.02000
Khalil, H. A., Yusra, A. I., Bhat, A. and Jawaid, M. (2010). Cell wall ultrastructure, anatomy, lignin distribution, and chemical composition of malaysian cultivated kenaf fiber. Ind. Crops Prod. 31, 113–121. doi: 10.1016/j.indcrop.2009.09.008
Mansor, M. R., Sapuan, S., Zainudin, E. S., Nuraini, A., and Hambali, A. (2013). Hybrid natural and glass fibers reinforced polymer composites material selection using analytical hierarchy process for automotive brake lever design. Mater. Des. 51, 484–492. doi: 10.1016/j.matdes.2013.04.072
Mansor, M. R., Sapuan, S., Zainudin, E. S., Nuraini, A., and Hambali, A. (2014). Conceptual design of kenaf fiber polymer composite automotive parking brake lever using integrated triz–morphological chart–analytic hierarchy process method. Mater. Des. 54, 473–482. doi: 10.1016/j.matdes.2013.08.064
NIJ Standards (2008). Ballistic Resistance of Body Armor NIJ Standard-0101.06. Washington, DC: US Department of Justice, Office of Justice Programs, National Institute of Justice.
Rashid, K., Razzaq, A., Ahmad, M., Rashid, T., and Tariq, S. (2017). Experimental and analytical selection of sustainable recycled concrete with ceramic waste aggregate. Constr. Build Mater. 154, 829–840. doi: 10.1016/j.conbuildmat.2017.07.219
Reddy, K. O., Reddy, G. S., Maheswari, C. U., Rajulu, A. V., and Rao, K. M. (2010). Structural characterization of coconut tree leaf sheath fiber reinforcement. J. For. Res. 21, 53–58. doi: 10.1007/s11676-010-0008-0
Reddy, N., and Yang, Y. (2005). Biofibers from agricultural byproducts for industrial applications. Trends. Biotechnol. 23, 22–27. doi: 10.1016/j.tibtech.2004.11.002
Sapuan, S., Kho, J., Zainudin, E., Leman, Z., Ali, B., and Hambali, A. (2011). Materials selection for natural fiber reinforced polymer composites using analytical hierarchy process. Ind. J. Eng Mater. Sci. 18, 255–226
Sathishkumar, T., Naveen, J., and Satheeshkumar, S. (2014). Hybrid fiber reinforced polymer composites–a review. J. Reinf. Plast. Comp. 33, 454–471. doi: 10.1177/0731684413516393
Saw, S. K., and Datta, C. (2009). Thermo mechanical properties of jute/bagasse hybrid fibre reinforced epoxy thermoset composites. Bioresources 4, 1455–1475.
Tsita, K. G., and Pilavachi, P. A. (2012). Evaluation of alternative fuels for the greek road transport sector using the analytic hierarchy process. Ener. Policy 48, 677–686. doi: 10.1016/j.enpol.2012.05.079
Yahaya, R., Sapuan, S., Jawaid, M., Leman, Z., and Zainudin, E. (2015). Effects of kenaf contents and fiber orientation on physical, mechanical, and morphological properties of hybrid laminated composites for vehicle spall liners. Polym. Compos. 36, 1469–1476. doi: 10.1002/pc.23053
Yahaya, R., Sapuan, S., Jawaid, M., Leman, Z., and Zainudin, E. (2016). Investigating ballistic impact properties of woven kenaf-aramid hybrid composites. Fiber. Polym. 17, 275–281. doi: 10.1007/s12221-016-5678-6
Keywords: synthetic fiber, natural fiber, hybrid composites, analytical hierarchy process, personal body armor
Citation: Naveen J, Jawaid M, Zainudin ES, Sultan MTH and Yahaya RB (2018) Selection of Natural Fiber for Hybrid Kevlar/Natural Fiber Reinforced Polymer Composites for Personal Body Armor by Using Analytical Hierarchy Process. Front. Mater. 5:52. doi: 10.3389/fmats.2018.00052
Received: 05 June 2018; Accepted: 03 August 2018;
Published: 27 August 2018.
Edited by:
Johnny Beaugrand, INRA Centre Angers-Nantes Pays de la Loire, FranceReviewed by:
Philippe Evon, National Polytechnic Institute of Toulouse, FranceLuigi Sorrentino, Istituto Per i Polimeri, Compositi e Biomateriali (IPCB), Italy
Copyright © 2018 Naveen, Jawaid, Zainudin, Sultan and Yahaya. This is an open-access article distributed under the terms of the Creative Commons Attribution License (CC BY). The use, distribution or reproduction in other forums is permitted, provided the original author(s) and the copyright owner(s) are credited and that the original publication in this journal is cited, in accordance with accepted academic practice. No use, distribution or reproduction is permitted which does not comply with these terms.
*Correspondence: M. Jawaid, amF3YWlkX21kQHlhaG9vLmNvLmlu; amF3YWlkQHVwbS5lZHUubXk=