- 1Department of Chemistry, University of Hull, Hull, UK
- 2Sporomex Ltd., Driffield, UK
- 3Weill Cornell Medical College Qatar, Doha, Qatar
- 4Hull York Medical School, University of Hull, Hull, UK
Sporopollenin is highly cross-linked polymer composed of carbon, hydrogen, and oxygen that is extraordinarily stable and has been found chemically intact in sedimentary rocks some 500 million years old. It makes up the outer shell (exine) of plant spores and pollen and when extracted it is in the form of an empty exine or microcapsule. The exines resemble the spores and pollen from which they are extracted, in size and morphology. Also, from any one plant such characteristics are incredible uniform. The exines can be used as microcapsules or simply as micron-sized particles due to the variety of functional groups on their surfaces. The loading of a material into the chamber of the exine microcapsule is via multi-directional nano-diameter sized channels. The exines can be filled with a variety of polar and non-polar materials. Enzymes can be encapsulated within the shells and still remain active. In vivo studies in humans have shown that an encapsulated active substance can have a substantially increased bioavailability than if it is taken alone. The sporopollenin exine surface possesses phenolic, alkane, alkene, ketone, lactone, and carboxylic acid groups. Therefore, it can be derivatized in a number of ways, which has given rise to applications in areas, such as solid supported for peptide synthesis, catalysis, and ion-exchange chromatography. Also, the presence of the phenolic groups on sporopollenin endows it with antioxidant activity.
The Oxford English Dictionary defines a biopolymer as “any of various polymers occurring in living organisms.” They are often categorized simply as carbohydrates, proteins, nucleic acids, polyisoprenoid lipids, and lignin. Sporopollenin seems to fall off the list or appears much lower in profile, despite its extraordinary properties that can exceed those of more widely known biopolymers. Sporopollenin makes up the fabric of the outer shell (exine) of plant spores and pollens and is arguably the toughest plant substance known. It has been referred to as the “diamond of the plant world” (Kesseler, 2004), as “probably one of the most extraordinary resistant materials known in the organic world” (Feagri and Iverson, 1964) and, in the plural sense, “the most resistant organic materials of direct biological origin found in nature and in geological samples” (Brooks and Shaw, 1978). In support of these statements, intact exines have been found in some of the most ancient sedimentary rocks found on the Earth’s surface (Wellman et al., 2003). In 1814, John (1814) was first to comment on the inertness of tulip pollen wall material, which he called “pollenin.” Such inertness was later reported in 1829 by Braconnot (1829) in the pollen wall of bullrush (Scirpoides holoschoenus), which he named “sporonin.” Almost a century later Zetzsche et al. made a systematic study to characterize the material from the asexual spores of Lycopodium clavatum L. (club moss) (Zetzsche and Kälin, 1931; Zetzsche and Vicari, 1931). He combined the previous names to form the word “sporopollenin,” as the generic term for the resistant exine material forming both spore and pollen grain walls, since they appeared to be of the same or have a very similar chemical character. More simply, sporopollenin has been defined as “the resistant non-soluble material left after acetolysis” (Heslop-Harrison and Shaw, 1971; Shaw, 1971) (using a hot mixture acetic anhydride and concentrated sulfuric acid) (Erdtman, 1960) since it readily digests most other biopolymers. Perhaps because of this resilience, the structure and biosynthesis of sporopollenin has yet to be fully elucidated although significant progress has been made over the years (Brooks and Shaw, 1978; Hemsley et al., 1992; Shaw and Apperley, 1996; Ahlers et al., 2000; Fraser et al., 2012; Watson et al., 2012). There is evidence (Hemsley et al., 1998; Gabarayeva and Hemsley, 2006) for the involvement of self-assembly in the formation of exines and more recently evidence for genetic and molecular regulation in sporopollenin biosynthesis (Basketter et al., 1997; Ariizumi and Toriyama, 2011). Also, there is strong support for sporopollenin being built up via catalytic enzyme reactions present in the tapetum. In spite of the widely divergent exine morphology and patterning across plant taxa, the developmental processes of sporopollenin formation appear, surprisingly, not to vary significantly, which suggests the preservation of a common molecular mechanism (Fraser et al., 2012). The quest to unravel the structure of sporopollenin has attracted several workers and a variety of approaches over the years (Shaw, 1971; Schulze Osthoff and Wiermann, 1987; Guilford et al., 1988; Wehling et al., 1989; Shaw and Apperley, 1996). Cumulative evidence is in support of sporopollenin being a highly cross-linked polymer composed of carbon, hydrogen, and oxygen. The building blocks appear to be varied and complex, comprising straight- and branched-aliphatic chains, some of which are saturated, unsaturated, and polyhydroxylated (Ahlers et al., 2000). Other important building blocks involve oxygenated aromatic rings and phenylpropanoid moieties. Cross-linking of the blocks has been shown to involve ether cross-linking (Domínguez et al., 1998; Ahlers et al., 2000) but carbon–carbon cross-linking is likely. Exines can be extracted from spores or pollen by a variety of methods, all of which rely upon and demonstrate the extreme stability of sporopollenin to harsh chemical treatment and a wide range of digestive enzymes. The early extraction work was performed to provide a source material for structural characterization. Chemical methods have included successive treatments with hot acetone, potassium hydroxide, and phosphoric acid (Zetzsche and Huggler, 1928; Zetzsche and Kälin, 1931; Zetzsche and Vicari, 1931; Zetzsche et al., 1937) although sulfuric acid was suggested (Shaw and Apperley, 1996) to be a better alternative to phosphoric acid. Acetolysis, introduced by Erdtman (1960) is often used as a cleaning method for palynological sample preparation and has been used frequently since it is rapid and efficient. Interestingly, when used under relatively mild conditions acetolysis has relatively little impact on sporopollenin chemistry (Jardin et al., 2015). Domínguez et al. (1998) developed a method involving anhydrous hydrofluoric acid in pyridine in one-step and claimed that it isolated unaltered sporopollenin. An alternative one-step method used aqueous 4-methylmorpholine-N-oxide and sucrose in alkaline conditions (Loewus et al., 1985; Espelie et al., 1989; Couderchet et al., 1996). Most of the extraction methods produce sporopollenin that is nitrogen free by combustion elemental analysis, which is indicative of sporopollenin being protein free. However, exines treated with sequential hot alkali and acid treatments were also shown to be protein free by mass spectrometry (MALDI-TOF-MS and ESI-QqToF-MS) and electrophoresis (SDS-PAGE), which are techniques in keeping with the Advisory Committee on Novel Foods and Processes (ACNFP) guidelines for the detection of trace protein in foods. It was proposed by Wiermann and Gubatz (1992) that all the harsh chemical methods denature sporopollenin in spore or pollen wall. For this reason, they embarked upon a detailed investigation using an extensive sequence of digestive enzymes to remove all biopolymers and leave only the natural sporopollenin intact (Schulze Osthoff and Wiermann, 1987). Clearly, it is important that sporopollenin be biologically degraded in nature “otherwise we would quite simply be submerged in these materials,” as Faegri (1971) stated. Various bacteria are known to degrade sporopollenin under certain conditions (e.g., pH or aerobic milieu) (Faegri, 1971; Stanley and Linskens, 1974; Edlund et al., 2004). There is surprisingly little in the literature pertaining to characterized enzymes that are capable of degrading sporopollenin. Following pollination, the intine secretes an enzyme cocktail (acid phosphatase, ribonuclease, esterase, and amylase) to break up the exine, but the precise characterization of such enzymes not been made (Edlund et al., 2004). Similarly, an uncharacterized pollen esterase has been found in Hordeum vulgare L. (barley) pollen that hydrolyzes sporopollenin, in pore formation (Ahokas, 1976).
Exines are unique as microcapsules due to their resilience, consistency in size and characteristic morphology, the latter two features being peculiar to the plant taxa. The exines (Figure 1, left) are penetrated by a myriad of nano-diameter sized channels that provide access to the internal chamber (Figure 1, centre) for hydration and dehydration processes (Rowley et al., 2003). The biological role of the exines is to protect and transport the delicate genetic and nutrient payloads as part of the plant replication process. Therefore, the exines have evolved to be light shielding (Rozema et al., 2001; Atkin et al., 2011), antioxidant, elastic, and resilient, both chemically and physically. Such a wide range of properties endowed upon sporopollenin exines has attracted interest toward a wide range of applications, particularly where the raw pollen or spores can be obtained in bulk commercial amounts. Examples include club moss (L. clavatum) (Figure 1), sunflower (Helianthus annuus), pine (Pinus), and green algae (Chlorella vulgaris). The foremost of these is the club moss, which is perhaps the most robust and plentiful since it is used in herbal remedies (Orhan et al., 2007; Mandal et al., 2010) and pyrotechnics (Ellern, 1968). However, the use of pollen from cereal crops, such as rye, maize, and wheat, holds great potential due to the large amounts of such crops being grown worldwide. Exines have served in two roles: (i) as simple “microparticles” that are resilient, uniform, and have a multifunctional and highly decorated surface and (ii) resilient microcapsules with an accessible chamber that can be filled with a variety of polar and non-polar actives with relatively favorable weight-for-weight loadings (Figure 1, right). Initially, applications used only the former capability.
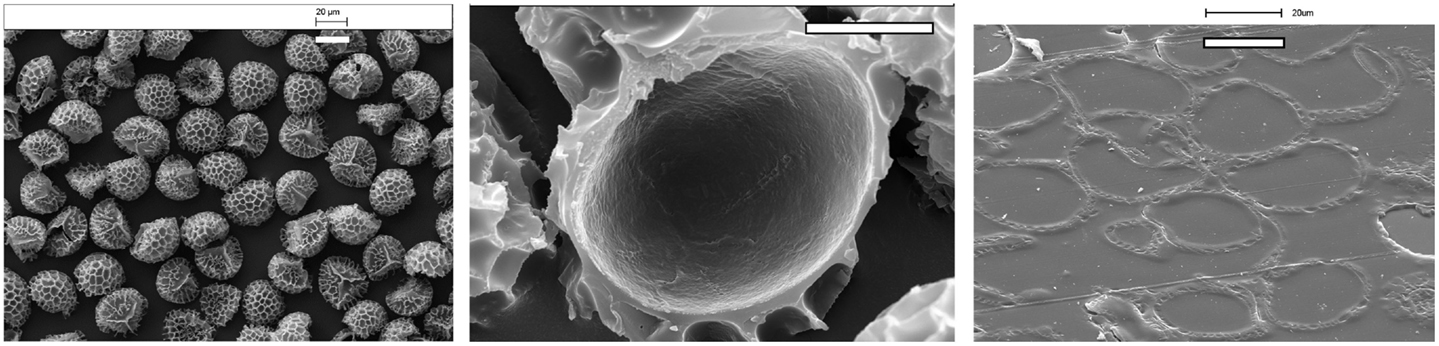
Figure 1. Scanning electron microscopy (SEM) micrographs of Lycopodium clavatum L exines [left (bar=20 μm)] exterior view of a cluster of exines [center (bar=10 μm)], microtome section of a single exine showing the empty internal cavity, and [left (bar=20 μm)] a microtome section of exines embedded in acrylic resin with internal cavities filled with the resin.
Applications as microparticles: the first reported application used exines extracted from L. clavatum using sequential treatment with hot alkali and acid (Mackenzie and Shaw, 1980). The aromatic moieties of the exines were chloromethylated and used as a Merrifield-type resin to synthesize a tripeptide. Conveniently, the loading of the chloromethyl linker was similar to that found on commercial synthetic resins. The chemical resilience and consistency in particle size of exines, coupled with available aromatic and carboxylic acid functions, were put to use as an immobile phase in chromatography and ion-exchange processes (Shaw et al., 1988). The former could be sulfonated with chlorosulfonic acid to produce a strongly acid material and the carboxylic acid could be converted into basic amino functionalities, either by using different types of diamines or reductive amination using ammonia (Barrier et al., 2010). Various chemically modified and unmodified exines from L. clavatum have been used for metal remediation (Archibald et al., 2014). Modifications have also been reported to demonstrate the use of sporopollenin exines as a support for use in heterogeneous catalysis. Examples include sporopollenin-supported Schiff bases (Sahin et al., 2012) and palladium catalysts for use in Heck coupling reactions (Keles, 2013). More recently, the exines have been demonstrated to act as an efficient support for enzyme-catalyzed reactions (de Souza et al., 2015). The exine surface possesses ionizable groups (phenols and carboxylic acids) that become increasingly negatively charged with an increase in pH (Binks et al., 2011). This has facilitated their being able to adsorb at both air–water and oil–water interfaces, forming stabilized liquid marbles and emulsions, respectively. The surface conjugated phenol functionalities have been shown by cyclic voltammetry to cause either a two-electron two-proton, or a two-electron one-proton process, which suggests that a rapid electron transfer takes place over the over the surface. This is in accordance with the observed antioxidant behavior of sporopollenin for protecting encapsulated omega-3 oils. Furthermore, the exines have been shown to adsorb ultra-violet and visible light (Atkin et al., 2011; Lomax and Fraser, 2015), which might be expected in terms of their biological role of protecting the genetic material within the pollen or spore.
Applications as microcapsules: the capability of exines from L. clavatum to act as microcapsules (Figure 1) was demonstrated (Barrier et al., 2011) for a range of polar and non-polar materials, over a wide range of molecular weights, from water to proteins up to 2000 kDa (Atwe et al., 2014). Notably, neither the sporopollenin nor the encapsulation procedure was significantly deleterious to the enzymes’ activity, thus offering the possibility of the exines to act as micro-reaction vessels or transporters of enzymes. This concept was also shown to be effective for the encapsulation of a lipase and subsequent use as catalyst in a variety of reactions (Tutar et al., 2009; Yilmaz, 2012). L. clavatum exines were also shown to work as micro-reactors for inorganic reactions. Magnetite nanoparticles and low-soluble salts, such as calcium phosphates, were formed within the exines’ chambers. The relatively large available space in the L. clavatum exine’s chamber, coupled with the exine’s amphiphilic surface properties have shown application by being able to sequester oil very efficiently from oil-in-water emulsions. Not surprisingly, the efficiency could be controlled by modifying the polarity of the exines by converting the exine’s surface hydroxyl groups (alcohols, phenols, and carboxylic acids), into polar salts (Na+ and K+) or non-polar derivatives (ethers, esters, and acetates). The ester forms were shown to sequester oils in near quantitative fashion. The elasticity of the exines permitted encapsulated oils to be released from the microcapsules in a stepwise manner simply by repeated rubbing. It is of note that the exines can be obtained with the cellulose intine (under) layer remaining intact, by hydrolysis of the spores or pollen with strong base, such as aqueous potassium hydroxide (Diego-Taboada et al., 2012). These double layered microcapsules (intine plus exine) can also be alkylated and acylated to sequester oil efficiently from emulsions. Normally, encapsulation into the exine’s available chamber is via the nano-diameter channels that penetrate them. However, it would appear that compression of the dry exines under some 5–10 tonnes/cm2 can open the trilete scar feature of the exines, allowing entry of living yeast cells into the exines’ chambers (Barrier et al., 2011; Hamad et al., 2011). After this process, the cells still remain viable within the chamber and the exines undamaged, which further illustrate the remarkable elasticity and physical robustness of the exines. The potential for sporopollenin exines to be used in food and pharmaceutical applications has been demonstrated by their having taste-masking properties to the tongue when encapsulated with, such as fish oil and ibuprofen (Diego-Taboada et al., 2013). Also, the exines were shown to encapsulate a commercial gadolinium(III) MRI contrast agent and allow slow release over 8 h in blood plasma (Lorch et al., 2009). In vitro studies have shown exines to have the potential to act as an effective drug delivery vector in which release can be triggered by pH (Beckett et al., 2009; Diego-Taboada et al., 2013). However, more importantly, the potential for exines to be used to deliver specialized foods and drugs has been demonstrated by the significantly enhanced bioavailability of the omega oil eicosapentaenoic acid (EPA) in a double cross-over study in human volunteers. It was shown that the taking the EPA in the encapsulated form provided a circa 10-fold increase in bioavailability over taking the EPA alone. The mechanism involved may be associated with bioadhesion of the exine capsules in the gastrointestinal tract. A later in vivo study in mouse showed that the sporopollenin exines filled with ovalbumin as a model antigen could be used for oral immunization and which demonstrates the potential of the exines to be used for oral vaccination (Atwe et al., 2014). The authors offered evidence by confocal microscopy that the exines translocate across the mouse intestinal epithelium, which, the authors proposed, might be involved in the stimulation of the immune system. This work further supports the potential of such microcapsules to have universality toward the delivery of polar and non-polar payloads. The unique three-dimensional shapes and surface topographies of pollens have attracted interest to synthesis inorganic mimics for use in drug delivery and adhesive microparticles with tuneable short- and long-range attractive forces (Goodwin et al., 2013).
For many years, sporopollenin has been studied as a curiosity due its robust nature and role in nature; however, it is only since 1980 that there has been a steady rise of interest in the potential uses of sporopollenin, particularly from L. clavatum. The authors believe that there will be more applications and uses in the years to come of this unique renewable polymer that is plentiful in nature from a wide variety of plant sources.
Conflict of Interest Statement
Some of the work cited was sponsored by the company Sporomex Ltd. Grahame Mackenzie is a Director of Sporomex Ltd., and Dr. Alberto Diego Taboada has received sponsorship from the company.
References
Ahlers, F., Bubert, H., Steuernagel, S., and Wiermann, R. (2000). The nature of oxygen in sporopollenin from the pollen of typha angustifolia L. Z. Naturforsch. C 55, 129–136. doi:10.1515/znc-2000-3-401
Ahokas, H. (1976). Evidence of a pollen esterase capable of hydrolysing sporopollenin. Experientia 32, 175–177. doi:10.1007/BF01937750
Archibald, S. J., Atkin, S. L., Bras, W., Diego-Taboada, A., Mackenzie, G., Mosselmans, J. F. W., et al. (2014). How does iron interact with sporopollenin exine capsules? An X-ray absorption study including microfocus XANES and XRF imaging. J. Mater. Chem. B 2, 945–959. doi:10.1039/C3TB21523G
Ariizumi, T., and Toriyama, K. (2011). Genetic regulation of sporopollenin synthesis and pollen exine development. Annu. Rev. Plant Biol. 62, 437–460. doi:10.1146/annurev-arplant-042809-112312
Atkin, S. L., Barrier, S., Cui, Z., Fletcher, P. D. I., Mackenzie, G., Panel, V., et al. (2011). UV and visible light screening by individual sporopollenin exines derived from Lycopodium clavatum (club moss) and Ambrosia trifida (giant ragweed). J. Photochem. Photobiol. B 102, 209–217. doi:10.1016/j.jphotobiol.2010.12.005
Atwe, S. U., Ma, Y., and Gill, H. S. (2014). Pollen grains for oral vaccination. J. Control. Release 194, 45–52. doi:10.1016/j.jconrel.2014.08.010
Barrier, S., Diego-Taboada, A., Thomasson, M. J., Madden, L., Pointon, J. C., Wadhawan, J. D., et al. (2011). Viability of plant spore exine capsules for microencapsulation. J. Mater. Chem. 21, 975–981. doi:10.1039/C0JM02246B
Barrier, S., Loebbert, A., Boasman, A. J., Boa, A. N., Lorch, M., Atkin, S. L., et al. (2010). Access to a primary aminosporopollenin solid support from plant spores. Green Chem. 12, 234–240. doi:10.1039/B913215E
Basketter, D. A., Chamberlain, M., Griffiths, H. A., Rowson, M., Whittle, E., and York, M. (1997). The classification of skin irritants by human patch test. Food Chem. Toxicol. 35, 845–852. doi:10.1016/S0278-6915(97)00053-7
Binks, B. P., Boa, A. N., Kibble, M. A., Mackenzie, G., and Rocher, A. (2011). Sporopollenin capsules at fluid interfaces: particle-stabilised emulsions and liquid marbles. Soft Matter 7, 4017–4024. doi:10.1039/c0sm01516d
Braconnot, H. (1829). Recherches chimiques sur le pollen de Typha latifolia L., famille des typhacées. Ann. Chim. Phys. 2, 91–105.
Brooks, J., and Shaw, G. (1978). Sporopollenin a review of its chemistry paleochemistry and geochemistry. Grana 17, 91–98. doi:10.1080/00173137809428858
Couderchet, M., Schmalfuß, J., and Böger, P. (1996). Incorporation of oleic acid into sporopollenin and its inhibition by the chloroacetamide herbicide metazachlor. Pestic. Biochem. Physiol. 55, 189–199. doi:10.1006/pest.1996.0048
de Souza, S. P., Bassut, J., Marquez, H. V., Junior, I. I., Miranda, L. S. M., Huang, Y., et al. (2015). Sporopollenin as an efficient green support for covalent immobilization of a lipase. Catal. Sci. Technol. 5, 3288–3295. doi:10.1039/C4CY01682C
Diego-Taboada, A., Cousson, P., Raynaud, E., Huang, Y., Lorch, M., Binks, B. P., et al. (2012). Sequestration of edible oil from emulsions using new single and double layered microcapsules from plant spores. J. Mater. Chem. 22, 9767–9773. doi:10.1039/c2jm00103a
Diego-Taboada, A., Maillet, L., Banoub, J. H., Lorch, M., Rigby, A. S., Boa, A. N., et al. (2013). Protein free microcapsules obtained from plant spores as a model for drug delivery: ibuprofen encapsulation, release and taste masking. J. Mater. Chem. B 1, 707–713. doi:10.1039/C2TB00228K
Domínguez, E., Mercado, J. A., Quesada, M. A., and Heredia, A. (1998). Isolation of intact pollen exine using anhydrous hydrogen fluoride. Grana 37, 93–96. doi:10.1080/00173139809362649
Edlund, A. F., Swanson, R., and Preuss, D. (2004). Pollen and stigma structure and function: the role of diversity in pollination. The Plant Cell 16, S84–S97. doi:10.1105/tpc.015800
Ellern, H. (1968). Military and Civilian Pyrotechnics. New York, NY: Chemical Publishing Company Inc.
Erdtman, G. (1960). The acetolysis method. A revised description. Svensk Botanisk Tidskrift 54, 561–564.
Espelie, K. E., Loewus, F. A., Pugmire, R. J., Woolfenden, W. R., Baldi, B. G., and Given, P. H. (1989). Structural-analysis of lilium-longiflorum sporopollenin by C-13 NMR-spectroscopy. Phytochemistry 28, 751–753. doi:10.1016/0031-9422(89)80108-6
Faegri, K. (1971). “The preservation of sporopollenin membranes under natural conditions,” in Sporopollenin, eds J. Brooks, P. R. Grant, M. Muir, P. Van Gijzel and G. Shaw (London: Academic Press), 256–272.
Fraser, W. T., Scott, A. C., Forbes, A. E. S., Glasspool, I. J., Plotnick, R. E., Kenig, F., et al. (2012). Evolutionary stasis of sporopollenin biochemistry revealed by unaltered Pennsylvanian spores. New Phytol. 196, 397–401. doi:10.1111/j.1469-8137.2012.04301.x
Gabarayeva, N., and Hemsley, A. R. (2006). Merging concepts: the role of self-assembly in the development of pollen wall structure. Rev. Palaeobot. Palynol. 138, 121–139. doi:10.1016/j.revpalbo.2005.12.001
Goodwin, W. B., Gomez, I. J., Fang, Y., Meredith, J. C., and Sandhage, K. H. (2013). Conversion of pollen particles into three-dimensional ceramic replicas tailored for multimodal adhesion. Chem. Mater. 25, 4529–4536. doi:10.1021/cm402226w
Guilford, W. J., Schneider, D. M., Labovitz, J., and Opella, S. J. (1988). High-resolution solid-state C-13 NMR-spectroscopy of sporopollenins from different plant taxa. Plant Physiol. 86, 134–136. doi:10.1104/pp.86.1.134
Hamad, S. A., Dyab, A. F. K., Stoyanov, S. D., and Paunov, V. N. (2011). Encapsulation of living cells into sporopollenin microcapsules. J. Mater. Chem. 21, 18018–18023. doi:10.1039/c1jm13719k
Hemsley, A. R., Chaloner, W. G., Scott, A. C., and Groombridge, C. J. (1992). C-13 Solid-state nuclear-magnetic-resonance of sporopollenins from modern and fossil plants. Ann. Bot. 69, 545–549.
Hemsley, A. R., Vincent, B., Collinson, M., and Griffiths, P. C. (1998). Simulated self-assembly of spore exines. Ann. Bot. 82, 105–109. doi:10.1006/anbo.1998.0653
Heslop-Harrison, J., and Shaw, G. (1971). “Sporopollenin in the biological context,” in Sporopollenin, eds J. Brooks, P. R. Grant, M. Muir and P. Van Gijzel (London: Academic Press), 1–30.
Jardin, P. E., Fraser, W. T., Lomax, B. H., and Gosling, W. D. (2015). The impact of oxidation on spore and pollen chemistry. J. Micropalaeontolog 34, 139–149. doi:10.1144/jmpaleo2014-022
John, J. F. (1814). Über den befruchtungsstaub, nebst einer analyse des tulpenpollens. J. Chem. Phys. 12, 244–252.
Keles, M. (2013). Preparation of heterogeneous palladium catalysts supported on sporopollenin for heck coupling reactions. Synthesis and Reactivity in Inorganic Metal-Organic and Nano-Metal Chemistry 43, 575–579. doi:10.1080/15533174.2012.749895
Loewus, F. A., Baldi, B. G., Franceschi, V. R., Meinert, L. D., and McCollum, J. J. (1985). Pollen sporoplasts – dissolution of pollen walls. Plant Physiol. 78, 652–654. doi:10.1104/pp.78.3.652
Lomax, B. H., and Fraser, W. T. (2015). Palaeoproxies: botanical monitors and recorders of atmospheric change. Palaeontology 58, 759–768. doi:10.1111/pala.12180
Lorch, M., Thomasson, M. J., Diego-Taboada, A., Barrier, S., Atkin, S. L., Mackenzie, G., et al. (2009). MRI contrast agent delivery using spore capsules: controlled release in blood plasma. Chem. Commun. 42, 6442–6444. doi:10.1039/b909551a
Mackenzie, G., and Shaw, G. (1980). Sporopollenin. A novel, naturally occurring support for solid phase peptide synthesis. Int. J. Pept. Protein Res. 15, 298–300. doi:10.1111/j.1399-3011.1980.tb02580.x
Mandal, S. K., Biswas, R., Bhattacharyya, S. S., Paul, S., Dutta, S., Pathak, S., et al. (2010). Lycopodine from Lycopodium clavatum extract inhibits proliferation of HeLa cells through induction of apoptosis via caspase-3 activation. Eur. J. Pharmacol. 626, 115–122. doi:10.1016/j.ejphar.2009.09.033
Orhan, I., Kupeli, E., Sener, B., and Yesilada, E. (2007). Appraisal of anti-inflammatory potential of the clubmoss, Lycopodium clavatum L. J. Ethnopharmacol. 109, 146–150. doi:10.1016/j.jep.2006.07.018
Rowley, J. R., Skvarla, J. J., and El-Ghazaly, G. (2003). Transfer of material through the microspore exine – from the loculus into the cytoplasm. Can. J. Bot. 81, 1070–1082. doi:10.1139/b03-095
Rozema, J., Broekman, R. A., Blokker, P., Meijkamp, B. B., de Bakker, N., van de Staaij, J., et al. (2001). UV-B absorbance and UV-B absorbing compounds (para-coumaric acid) in pollen and sporopollenin: the perspective to track historic UV-B levels. J. Photochem. Photobiol. B 62, 108–117. doi:10.1016/S1011-1344(01)00155-5
Sahin, M., Gubbuk, I. H., and Kocak, N. (2012). Synthesis and characterization of sporopollenin-supported schiff bases and ruthenium(III) sorption studies. J. Inorg. Organomet. Polym. Mater. 22, 1279–1286. doi:10.1007/s10904-012-9739-z
Schulze Osthoff, K., and Wiermann, R. (1987). Phenols as integrated compounds of sporopollenin from Pinus pollen. J. Plant Physiol. 131, 5–15. doi:10.1016/S0176-1617(87)80262-6
Shaw, G. (1971). “The chemistry of sporopollenin,” in Sporopollenin, eds J. Brooks, P. R. Grant, M. Muir, P. V. Gijzel and G. Shaw (London: Academic Press), 305–334.
Shaw, G., and Apperley, D. C. (1996). C-13-NMR spectra of Lycopodium clavatum sporopollenin and oxidatively polymerised beta-carotene. Grana 35, 125–127. doi:10.1080/00173139609429483
Shaw, G., Sykes, M., Humble, R. W., Mackenzie, G., Marsden, D., and Pehlivan, E. (1988). The use of modified sporopollenin from Lycopodium clavatum as a novel ion- or ligand-exchange medium. React. Polym. Ion Exchangers Sorbents 9, 211–217. doi:10.1016/0167-6989(88)90034-7
Stanley, R. G., and Linskens, H. F. (eds) (1974). Pollen: Biology, Biochemistry, Management. Berlin: Springer-Verlag, 307.
Tutar, H., Yilmaz, E., Pehlivan, E., and Yilmaz, M. (2009). Immobilization of Candida rugosa lipase on sporopollenin from Lycopodium clavatum. Int. J. Biol. Macromol. 45, 315–320. doi:10.1016/j.ijbiomac.2009.06.014
Watson, J. S., Fraser, W. T., and Sephton, M. A. (2012). Formation of a polyalkyl macromolecule from the hydrolysable component within sporopollenin during heating/pyrolysis experiments with Lycopodium spores. J. Anal. Appl. Pyrolysis 95, 138–144. doi:10.1016/j.jaap.2012.01.019
Wehling, K., Niester, C., Boon, J. J., Willemse, M. T. M., and Wiermann, R. (1989). p-Coumaric acid – a monomer in the sporopollenin skeleton. Planta 179, 376–380. doi:10.1007/BF00391083
Wellman, C. H., Osterloff, P. L., and Mohiuddin, U. (2003). Fragments of the earliest land plants. Nature 425, 282–285. doi:10.1038/nature01884
Wiermann, R., and Gubatz, S. (1992). Pollen wall and sporopollenin. Int. Rev. Cytol. 140, 35–72. doi:10.1016/S0074-7696(08)61093-1
Yilmaz, E. (2012). Enantioselective enzymatic hydrolysis of racemic drugs by encapsulation in sol-gel magnetic sporopollenin. Bioprocess Biosyst. Eng. 35, 493–502. doi:10.1007/s00449-011-0622-z
Zetzsche, F., and Huggler, K. (1928). Untersuchungen über die membran des sporen und pollen I. 1. Lycopodium clavatum L. Justus Liebigs. Ann. Chem. 461, 89–108. doi:10.1002/jlac.19284610105
Zetzsche, F., and Kälin, O. (1931). Untersuchungen über die membran der sporen und pollen v. 4. Zur autoxydation der sporopollenine. Helv. Chim. Acta 14, 517–519. doi:10.1002/hlca.19310140151
Zetzsche, F., Kalt, P., Lietchi, J., and Ziegler, E. (1937). Zur konstitution des lycopodium-sporonins, des Tasmanins und des lange-sporonins. J. Prakt. Chem. 148, 267–286. doi:10.1002/prac.19371480903
Keywords: sporopollenin, pollen, spores, exines, microcapsules
Citation: Mackenzie G, Boa AN, Diego-Taboada A, Atkin SL and Sathyapalan T (2015) Sporopollenin, the least known yet toughest natural biopolymer. Front. Mater. 2:66. doi: 10.3389/fmats.2015.00066
Received: 11 August 2015; Accepted: 02 October 2015;
Published: 19 October 2015
Edited by:
José Alejandro Heredia-Guerrero, Istituto Italiano di Tecnologia, ItalyReviewed by:
Frederik Roman Wurm, Max Planck Institute for Polymer Research, GermanyWesley Toby Fraser, Oxford Brookes University, UK
Copyright: © 2015 Mackenzie, Boa, Diego-Taboada, Atkin and Sathyapalan. This is an open-access article distributed under the terms of the Creative Commons Attribution License (CC BY). The use, distribution or reproduction in other forums is permitted, provided the original author(s) or licensor are credited and that the original publication in this journal is cited, in accordance with accepted academic practice. No use, distribution or reproduction is permitted which does not comply with these terms.
*Correspondence: Grahame Mackenzie, g.mackenzie@hull.ac.uk