- 1Department of Macromolecular Science and Engineering, Case Western Reserve University, Cleveland, OH, USA
- 2School of Materials Science and Engineering, East China University of Science and Technology, Shanghai, China
High-performance thermosets via amide–imide functional benzoxazine resins as precursors have been synthesized. The structures of synthesized monomers have been confirmed by 1H NMR and Fourier transform infrared. Among these two benzoxazine monomers, the ortho-amide–imide functional benzoxazine resin shows powerful features both in the synthesis of benzoxazine monomers and the properties of the corresponding thermosets. For the cross-linked poly(amide-co-imide) based on ortho-amide–imide functional benzoxazine, a smart route is adopted to develop a more thermally stable cross-linked poly(benzoxazole-co-imide). Besides, the poly(benzoxazole-co-imide) can also undergo a further thermal treatment to form polybenzoxazole. Furthermore, a main-chain type ortho-functional polybenzoxazine with amide-co-imide and benzoxazine groups as repeating units has also been prepared. Both the ortho-amide–imide functional benzoxazine and main-chain type polybenzoxazine resins show the possibility to form high-performance thermosets with low cost and easy processability.
Introduction
Polybenzoxazoles (PBOs) are a class of heterocyclic high-performance polymers possessing high mechanical strength, excellent thermal stability, and interesting photophysical properties (Jaffe et al., 1992; Meador, 1998). However, the very high rigidity of PBOs also brings the difficulties in synthesis and fabrication. The traditional approach to prepare the PBOs is the use of the strong acid, such as poly(phosphoric acid) (PPA), which can dissolve PBOs (Feng et al., 2004). However, the use of such strong acid is environmentally unfriendly. Besides, the acid residues can catalyze the hydrolysis reaction of the oxazole rings at moderately elevated temperature under humid environment. The recent approach is to develop a PBOs precursor that is soluble in organic solvent (Fukumaru et al., 2012, 2014), or fusible at low temperature.
Benzoxazine is a newly developed thermosetting resin, which can be synthesized by Mannich condensation from phenol, amine, and formaldehyde (Holly and Cope, 1944; Ning and Ishida, 1994). The major advantages of polybenzoxazine include excellent mechanical and thermal properties, low water absorption, high char yield, and low surface free energy (Ishida and Allen, 1996; Ishida and Low, 1997; Kim et al., 1999a,b; Wang et al., 2006; Velez-Herrera and Ishida, 2009; Yagci et al., 2009; Zhang et al., 2012, 2013). The most interesting and unique characteristics of this class of polymers is their extraordinarily rich molecular design flexibility that allows designing a variety of molecular structures to tailor the desired properties. With the advantage of design flexibility of benzoxazine, we already developed an approach to prepare PBOs by benzoxazine resins as precursors via o-amide structure formed upon polybenzoxazine formation (Agag et al., 2012). The cross-linked PBOs show further advantages including outstanding flexibility in the molecular design and cost effectiveness. However, this method also brought some problems during the fabrication. Although the ortho-amide benzoxazines have the very good solubility in normal solvents, some water has to be released between 250 and 300°C for the thermal conversion to form PBOs.
Poly(amide-co-imide)s (PAIs) have been developed as alternative materials, which offer a compromise between excellent processability and thermal stability. Aromatic PAIs possess balanced characteristics between polyamides and polyimides such as high thermal stability and good mechanical properties together with ease of processability (Imai, 1996). Besides, Mathias and others reported the possible thermal conversion of ortho-hydroxypolyimides into PBOs with loss of carbon dioxide at temperature around 400°C (Tullos et al., 1999; Park et al., 2007). The above features inspired the idea to incorporate amide and imide groups into one benzoxazine compound, which can be developed as a precursor for PBOs with better processability. The number of moles of water produced per chemical repeat unit in the current system is the same as our previous o-amide benzoxazine mentioned above. However, the molecular weight of the chemical repeat unit in the current paper is much larger, thus reducing the amount of water generated per unit weight, minimizing the chance of gas bubble formation. Furthermore, the thermal conversion to form PBOs from ortho-amide–imide functional benzoxazines can be realized by releasing water and CO2 gradually at a broad temperature range of 250–400°C, which further avoids forming porous structures during the processing.
In this study, both para- and ortho-amide–imide functional benzoxazine monomers were prepared. The ortho-amide–imide functional benzoxazine shows great advantages both in the synthesis of benzoxazine monomers, ease of processing which is rare for high-performance resins, and the properties of the corresponding polybenzoxazines compared with the para-amide–imide functional benzoxazine. This polybenzoxazine derived from ortho-functional benzoxazine monomer is a subject of very recent paper where unexpected superior properties were observed for ortho-functional benzoxazine in comparison of para-functional benzoxazine (Liu and Ishida, 2014). For the cross-linked PAIs based on ortho-amide–imide functional benzoxazine, subsequent in situ thermal conversion can provide an alternative method for preparing a more thermally stable cross-linked poly(benzoxazole-co-imide). Besides, the poly(benzoxazole-co-imide) can also undergo a further thermal conversion to form PBO. Compared with the conversion of ortho-hydroxypolyimides and ortho-hydroxypolyamides to PBOs, it starts from rather inexpensive benzoxazine compounds than very expensive aromatic bisaminophenols. As such, the compound is easy to synthesize, inexpensive, and easy to process. Moreover, a main-chain type ortho-functional polybenzoxazine with amide–imide and benzoxazine groups as repeating units in the main-chain has also been prepared. Additionally, the structure of these new benzoxazines has been characterized, and the thermal and mechanical properties of the corresponding thermosets are discussed.
Materials and Methods
Materials
o-Aminophenol (98%), p-aminophenol (97%), trimellitic anhydride chloride (98%), and paraformaldehyde (95%), were used as received from Sigma-Aldrich. 4,4′-Diaminodiphenylmethane (DDM) (98%), was purchased from Aldrich. Aniline was purchased from Aldrich and purified by distillation. Dimethylformamide, hexane, xylenes, and sodium sulfate were obtained from Fisher scientific and used as received.
Characterization
Proton nuclear magnetic resonance (1H NMR) spectra were acquired on a Varian Oxford AS300 at a proton frequency of 300 MHz. The average number of transients for 1H MNR measurement was 64. A relaxation time of 10 s was used for the integrated intensity determination of 1H NMR spectra. Fourier transform infrared (FTIR) spectra were obtained using a Bomem Michelson MB100 FTIR spectrometer, which was equipped with a deuterated triglycine sulfate (DTGS) detector and a dry air purge unit. Co addition of 32 scans was recorded at a resolution of 4 cm−1. Transmission spectra were obtained by casting a thin film on a KBr plate for partially polymerized samples. A TA Instruments differential scanning calorimeter (DSC) model 2920 was used with a heating rate of 10°C/min and a nitrogen flow rate of 60 mL/min for all tests. All samples were sealed in hermetic aluminum pans with lids. Thermogravimetric analyses (TGA) were performed on a TA Instrument Q500 TGA with a heating rate of 10°C/min in a nitrogen atmosphere at a flow rate of 40 mL/min. Dynamic mechanical analysis (DMA) was done on a TA Instruments Model Q800 DMA applying controlled strain tension mode with amplitude of 10 μm and a ramp rate of 3°C/min.
Preparation of N,2-Bis(4-Hydroxyphenyl)-1,3-Dioxoisoindoline-5-Carboxamide (Abbreviated as pAI)
Into a 250 mL round flask were placed trimellitic anhydride chloride (6.32 g, 0.03 mol), p-aminophenol (6.54 g, 0.06 mol), and 60 ml of dimethylformamide. The mixture was stirred at room temperature for 2 h, and then refluxed for 6 h. After cooling to room temperature, the reaction mixture was poured into 500 mL of 2 mol L−1 NaHCO3 aqueous solution to give a powder-like precipitate. Then the precipitate was filtered and washed several times with de-ionized water. The products were dried under vacuum to obtain a brown powder (yield 82%). 1H NMR (DMSO-d6), ppm: δ = 6.72–8.47 (11H, Ar), 9.34 (s, NH), 9.78 (s, OH), 10.41 (s, OH). IR spectra (KBr, cm−1) = 3322 (O-H stretching), 1782, 1726 (Imide I), 1709, 1651 (amide I), 1384 (imide II, C-N stretching), 730 (C =O bending).
Preparation of N,2-Bis(2-Hydroxyphenyl)-1,3-Dioxoisoindoline-5-Carboxamide (Abbreviated as oAI)
Exactly the same amounts and procedures as pAI were used to synthesize oAI. The product was dried under vacuum to obtain a yellow powder (yield 85%). 1H NMR (DMSO-d6), ppm: δ = 6.79–8.65 (11H, Ar), 9.71 (s, OH), 9.90 (s, OH), 10.01 (s, NH). IR spectra (KBr, cm−1) = 3334 (O-H stretching), 1775, 1705 (Imide I), 1705, 1657 (amide I), 1387 (imide II, C-N stretching), 751 (C =O bending).
Preparation of 1,3-Dioxo-N,2-Bis(3-Phenyl-3,4-Dihydro-2H-Benzo[e][1,3]Oxazin-6-yl)Isoindoline-5-Carboxamide (Abbreviated as pAI-a)
Into a 100 mL round flask were added 40 mL of mixed isomer xylenes, aniline (1.86 g, 0.02 mol), pAI (3.74 g, 0.01 mol), and excess of paraformaldehyde (1.81 g, 0.06 mol). The mixture was stirred at 120°C for 48 h. The mixture was subsequently cooled to room temperature and filtered off. Then the solution was precipitated into 100 mL of hexane. Removal of solvent afforded a yellow powder (yield 52%). 1H NMR (DMSO-d6), ppm: δ = 4.61, 4.69 (s, Ar-CH2-N, oxazine), 5.45, 5.52 (s, O-CH2-N, oxazine), 6.51–8.48 (19H, Ar), 10.18 (s, NH). IR spectra (KBr), cm−1: 1771, 1721 (imide I), 1721, 1675 (amide I),1500 (stretching of trisubstituted benzene ring), 1372 (imide II), 1229 (C-O-C asymmetric stretching), 951 (out-of-plane C-H).
Preparation of 1,3-Dioxo-N,2-Bis(3-Phenyl-3,4-Dihydro-2H-Benzo[e][1,3]Oxazin-8-yl)Isoindoline-5-Carboxamide (Abbreviated as oAI-a)
Into a 100 mL round flask were added 40 mL of mixed isomer xylenes, aniline (1.86 g, 0.02 mol), oAI (3.74 g, 0.01 mol), and paraformaldehyde (1.21 g, 0.04 mol). The mixture was stirred at 120°C for 6 h. The mixture was cooled to room temperature. Then the solution was precipitated into 100 mL of hexane. Removal of solvent afforded a yellow powder (yield 95%). 1H NMR (DMSO-d6), ppm: δ = 4.70, 4.74 (s, Ar-CH2-N, oxazine), 5.43, 5.54 (s, O-CH2-N, oxazine), 6.54–8.57 (19H, Ar), 9.95 (s, NH). IR spectra (KBr), cm−1: 1780, 1724 (imide I), 1724, 1675 (amide I), 1498 (stretching of trisubstituted benzene ring), 1380 (imide II), 1234 (C-O-C asymmetric stretching), 926 (out-of-plane C-H).
Preparation of Amide-Co-Imide Main-Chain Type Benzoxazine Polymer (oAI-ddm)
Into a 250 mL round flask were added 60 mL of xylenes, DDM (1.98 g, 0.01 mol), oAI (3.74 g, 0.01 mol), and paraformaldehyde (1.21 g, 0.04 mol). The mixture was stirred at 120°C for 12 h. The mixture was cooled to room temperature and filtered off. Then the solution was precipitated into 100 mL of hexane. Removal of solvent afforded a yellow powder (yield 75%).
Preparation of Poly(Benzoxazole-Co-Imide) and Polybenzoxazole Thermosets
A solutions of 30% solid content of the oAI-a and oAI-ddm in DMF was prepared. Then, the solution was cast over a dichlorodimethylsilane-pretreated glass plate. The films were dried in an air circulating oven at 100°C for 3 days to remove the solvent. The film as fixed on a glass plate was polymerized stepwise at 200°C for 2 h and 300°C for 1 h to obtain poly(benzoxazole-co-imide) thermosets. The poly(benzoxazole-co-imide) thermosets are defined as poly(oAI-a)-300 and poly(oAI-ddm)-300 from the monomeric and main-chain precursors, respectively. The poly(benzoxazole-co-imide) thermosets were further treated at 400°C for 1 h to obtain PBO thermosets. The PBO thermosets are defined as poly(oAI-a)-400 and poly(oAI-ddm)-400 from the monomeric and main-chain precursors, respectively. All film showed dark brown color with thickness ranging from 0.1 to 0.3 mm.
Results and Discussion
Preparation of Amide-Co-Imide Functional Benzoxazine Monomers
A successful synthesis of amide–imide functional benzoxazine monomers has been achieved using primary amine (aniline), formaldehyde, and amide-co-imide functional phenol (ortho- and para-isomers) as indicated in Scheme 1.
We chose trimellitic anhydride chloride to prepare amide–imide functional phenols (pAI and oAI) as the first step, and then pAI and oAI reacted with aniline and paraformaldehyde to prepare amide–imide difunctional benzoxazine monomers. At the second step, the synthesis method and the reaction time of para-amide–imide functional benzoxazine monomer (pAI-a) exhibited great differences from ortho-isomer (oAI-a). The synthesis of pAI-a was difficult because of the poor solubility of pAI in ordinary solvent. Besides, the pAI did not disappear despite applying long reaction time, leading to poor yield. Thus, excess paraformaldehyde and the reaction time of 48 h were applied to obtain at least 80% yield of benzoxazine monomer. However, the synthesis of oAI-a completed in just 6 h, and a transparent solution was subsequently formed, leading to a high yield. We assumed that the intramolecular hydrogen bond between the phenolic OH group and the carbonyl group decreases the polarity of oAI. As a result, it improves the solubility of oAI in aprotic solvent. Additionally, the intramolecular hydrogen bond also accelerates the ionization of phenolic OH during the initial stage for forming benzoxazine ring. Thus, the ring-closing of benzoxazine ring for ortho-isomer becomes much easier than para-isomer.
The structures of the monomers were confirmed using 1H NMR spectra, as depicted in Figures 1 and 2. The typical resonances attributed to the benzoxazine structures, Ar-CH2-N- and -O-CH2-N- for pAI-a are observed at 4.61, 4.69, and 5.45, 5.52 ppm, respectively. Also the resonances for oAI-a are observed at 4.70, 4.74, and 5.43, 5.54 ppm, respectively. Ordinarily, the characteristic benzoxazine resonance pair for Ar-CH2-N- and -O-CH2-N- consists of singlets with frequency separation of around 0.8–0.9 ppm (Ishida and Agag, 2011). However, due to the asymmetric nature of the magnetic environment of pAI-a and oAI-a, those singlets split into doublets. Besides, the 1H NMR spectra confirm the presence of amide group (-NH-) at 10.18 and 9.95 ppm for pAI-a and oAI-a, respectively.
There are a number of infrared absorption bands, highlighted in Figure 3, which are used to verify the formation of amide, imide, and oxazine rings in each monomer. For example, imide is presented by the characteristic bands at 1771, 1780 cm−1, 1721, 1724 cm−1, and 1372, 1380 cm−1 for pAI-a and oAI-a, respectively. The characteristic doublet at 1771, 1780 cm−1 and 1721, 1724 cm−1 are the typical bands for imide, which are attributed to the imide C-C(=O)-C asymmetric and symmetric stretching, respectively. The bands at 1372 and 1380 cm−1 are due to the axial stretching of C-N bonding (Ishida et al., 1980). Besides, the characteristic band at 1675 cm−1 is the typical band for amide, which is attributed to the amide I mode. Bands characteristic of asymmetric trisubstituted benzene that appear at 1500 and 1498 cm−1 support the incorporation of imide and amide group into benzoxazine monomers. Furthermore, the presence of the benzoxazine ring aromatic ether in the monomers is indicated by bands centered at 1229 and 1234 cm−1 for the pAI-a and oPP-a, respectively, which are due to the C-O-C asymmetric stretching modes. The characteristic out-of-plane absorption modes of benzene with an attached oxazine ring are located at 951 and 926 cm−1 for the pAI-a and oAI-a, respectively (Dunkers and Ishida, 1995).
Polymerization Behavior of Benzoxazine Monomers
The polymerization profiles of imide functional benzoxazine monomers were studied by DSC as depicted in Figure 4. The thermograms show that the maxima of the ring-opening polymerization of pAI-a and oAI-a centered at 219 and 215°C, respectively. The polymerization behavior of amide containing benzoxazines further support our previous finding that the presence of amide (-NH-CO-) linkage accelerated the polymerization in comparison with ordinary benzoxazines (Agag et al., 2010). The ortho-functional benzoxazine monomer oAI-a shows lower ring-opening polymerization temperature compared with the para-isomer. The presence of intramolecular hydrogen bonding between an amide linkage and the adjacent oxazine ring acts as an internal incentive to stimulate the ring-opening polymerization in a smart way, like a self-complementary initiator. However, the exotherm maxima separation between the para and ortho species is smaller than expected. This is likely due to the poorer purity of the para species and show lower exotherm maximum temperature than the pure sample as shown in the lack of melting endotherm in the para species. This lack of melting endotherm is caused by the difficulty in synthesis, as described previously, and as a consequence, the presence of oligomers that is difficult to be eliminated. The NMR spectra show the oxazine contents of pAI-a and oAI-a to be 90 and 98%, respectively. In the case of synthesizing pAI-a, the solubility of starting material of pAI is very poor in the reaction solvent. As a result, the longer reaction time leads the phenol group catalyzing the ring-opening of oxazine ring during the reaction, forming about 10% oligomers.
Thermal Properties of Amide-Co-Imide Functional Polybenzoxazines
The thermal stability of the polybenzoxazine derived from para- and ortho-benzoxazine isomers has been studied by TGA (Figure 5) after thermal treatment at 200°C for 2 h. The derivative weight-loss curves are also shown in Figure 5. From the comparison of these two polybenzoxazines, we observed that poly(pAI-a) shows two high weight-loss stages in the range of 250–400°C and 450–600°C, which shows a degradation similar to typical polybenzxoazines. Figure 6 shows FTIR spectra of pAI-a after various thermal treatment. As shown in Figure 6, the amide band at 1675 cm−1 decreases significantly from 300 to 400°C treatment, which suggests the degradation of amide groups in poly(pAI-a). Meanwhile, the characteristic band for imide at 1771 cm−1 does not change at all during the heating. Besides, it is well known that the polybenzoxazines derived from benzoxazine monomers contain terminal Schiff base groups and secondary amides as defect structures, which can be decomposed around 250–300°C (Hamerton et al., 2013). Thus, the first weight-loss stages in the range of 250–400°C for poly(pAI-a) is due to the degradation of defect structures and amide groups. On the contrary, the polybenzoxazine of ortho-isomer (oAI-a) shows different weight change profiles. Poly(oAI-a) does not show the obvious weight-loss stage in the range of 450–600°C. The second weight-loss stage of poly(oAI-a) is in the range of 550–750°C, which is much higher than poly(pAI-a). As a result, the char yield of the polybenzoxazines based on ortho-monomer is much higher than the polybenzoxazine based on para-isomers.
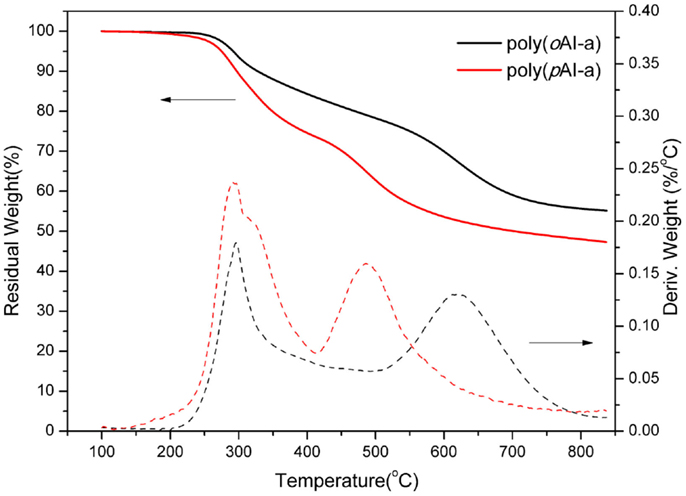
Figure 5. Thermogravimetric analysis of amide-co-imide functional benzoxazine isomers after thermal polymerization at 200°C.
In order to qualitatively study the structural evolution of poly(oAI-a), FTIR analysis of oAI-a after various thermal treatments was carried out and the spectra are shown in Figure 7. The characteristic absorption band at 926 cm−1 (the out-of-plane bending vibration of C-H) and 1234 cm−1 (C-O-C asymmetric stretching modes) gradually disappear from room temperature to 200°C, suggesting the ring-opening of the benzoxazine. Besides, the cyclization to poly(o-hydroxyamide) was observed by the decrease of the amide I band at 1675 cm−1 and appearance of benzoxazole structure at 1615 cm−1 (C =N stretching) after 200°C. As a result, the first weight-loss stage in the range of 200–300°C is due to the formation of benzoxazole through intramolecular cyclization between the neighboring hydroxyl and amide groups. Moreover, the characteristic peak for imide at 1780 cm−1 gradually disappears after 350°C, which is in agreement with the possible thermal conversion of ortho-hydroxypolyimides into benzoxazole structure.
In order to further support the benzoxazole formation, the TGA analyses with the isothermal conditions at 300 and 400°C for each 1 h of poly(oAI-a) has been studied. As shown in Figure 8, a weight-loss of 6.42 wt% is observed in the first weight-loss stage, which is higher than the theoretical weight loss from complete release of water (2.96 wt%). As mentioned above, the difference between the observed and theoretically expected weight-loss amount by 3.46 wt% might originate from the degradation of the defect structures. Besides, the weight loss for the second weight-loss stage is 7.95 wt% since the theoretical weight loss from complete release of CO2 should be 7.24 wt%, which is nearly identical to the theoretically expected amount, implying that no significant additional degradation mechanism exists. The previous approaches for developing PBO precursors that are soluble in organic solvents were realized by incorporating flexible groups, such as tert-butyldimethylsilyl, methyl, and isopropyl. As a result, the degradation of those flexible groups occurred during the thermally induced ring-closing reaction of benzoxazole formation, which leads to a high weight loss (Fukumaru et al., 2012, 2014). The trade-off generally exists between the solubility and thermal stability of PBO precursors. However, only around 14.5 wt% total weight loss was observed in this study, which is much lower compared with other methods for obtaining PBO s with the weight loss of 33–36 wt% (Fukumaru et al., 2014). Moreover, the third weight-loss stage of poly(oAI-a) is in the range of 550–700°C, which is in accordance with the degradation of PBO. Therefore, the thermal behavior of pAI-a and oAI-a can be described as Scheme 2.
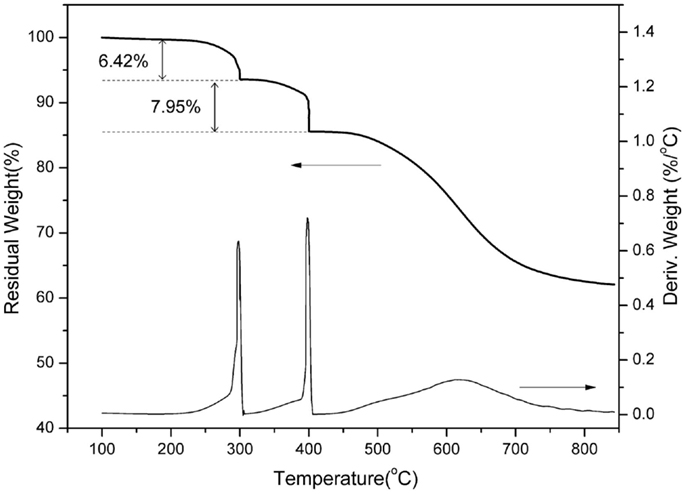
Figure 8. Thermogravimetric analysis of poly(oAI-a). At 300 and 400°C, isothermal heating was applied for 1 h each.
Preparation of Amide-Co-Imide Main-Chain Type Benzoxazine Polymer
It is well known that the thermal and mechanical performance of polybenzoxazine thermosets derived from main-chain benzoxazine polymers proved to be excellent in comparison to those derived from monomers (Chernykh et al., 2006). In this study, a main-chain type ortho-functional polybenzoxazine with amide–imide and benzoxazine groups as repeating units in the main-chain (oAI-ddm) has also been prepared. The structure of the main-chain oAI-ddm was confirmed using 1H NMR spectrum, as depicted in Figure 9. The typical resonances attributed to the benzoxazine structure, Ar-CH2-N- and -O-CH2-N- for main-chain oAI-ddm are observed at 4.65, 4.63 and 5.35, 5.46 ppm, respectively. Also the 1H NMR spectra confirm the presence of amide group (-NH-) at 9.89 ppm and methylene group (-CH2-) at 3.68 ppm. Moreover, the intensity ratio of O-CH2-N to -NH- is 3.66:1, which is lower than the ideal main-chain polybenzoxazine with complete ring-closure (4:1). Thus, the concentration of ring-closure can be calculated as 3.66/4 = 91.5%.
DSC Analysis of oAI-ddm as a Functional of Heat Treatment
The crosslinking behavior of the main-chain (oAI-ddm) was also studied by DSC as shown in Figure 10 after thermal treatment at 150, 200, 250, and 300°C for 1 h at each temperature.
It is well known that 1,3-benzoxazine exhibits an exothermic ring-opening polymerization reaction around 250°C (Ishida and Agag, 2011). However, the DSC results of oAI-ddm show a different thermal behavior compared with the traditional benzoxazines. The thermograms not only show an exothermic ring-opening behavior of benzoxazine but also an endothermic cyclodehydration behavior of o-hydroxyamide to give benzoxazole. It is observed that the exothermic peak gradually decreases after each treatment temperature. The exotherm and endotherm practically disappeared at 300°C, thereby showing the completion of the ring-opening and cyclodehydration reaction. Besides, no Tg can be observed after the thermal treatment at 300°C of oAI-ddm before 350°C, which means the excellent thermal property of poly(oAI-ddm)-300.
Thermal Properties of Poly(Benzoxazole-Co-Imide) Thermosets
The results of DMA of the thermosets are shown in Figure 11 for the storage (E′) modulus, loss (E″) modulus, and tan δ. In this work, the peak of tan δ is used to mark the glass transition temperature (Tg) due to the difficulty of determining the peak position for the E″ spectrum, which is normally used as the definition of Tg by DMA. As shown in Figure 11A, the Tg determined as the peak temperature of tan δ curve is around 332°C for poly(oAI-a)-300. However, the poly(oAI-ddm)-300 shows no Tg before 400°C as shown in Figure 11B, which is in agreement with the DSC results, suggesting that this material can be used at very high temperatures. We did not run the analysis at a higher temperature to find the Tg because the degradation took place after 400°C. The flatness of the E′ spectrum in a very wide temperature range from room temperature to almost 400°C suggests that this material is ideally suited for application in a variable temperature where constant mechanical properties throughout the temperature range is desired. The broad and weak transitions centered around 125°C is likely due to the β transition of the polymer.
The thermal stability of poly(benzoxazole-co-imide) thermosets (poly(oAI-a)-300 and poly(oAI-ddm)-300 has been studied by TGA as shown in Figure 12. The 5 and 10% weight-loss temperatures (Td5 and Td10) for poly(oAI-a)-300 are 406 and 476°C, respectively. For poly(oAI-ddm)-300, the Td5 and Td10 are 410 and 473°C, respectively. Besides, the char yield for poly(oAI-a)-300 and poly(oAI-ddm)-300 are as high as 63 and 52%, respectively. The thermal property data of poly(oAI-a)-300 and poly(oAI-ddm)-300 are summarized in Table 1. The data demonstrate high thermal stability of both monomer and main-chain benzoxazine based cross-linked poly(benzoxazole-co-imide) thermosets.
Thermal Properties of Polybenzoxazole Thermosets
The thermal stability of PBO thermosets poly(oAI-a)-400 and poly(oAI-ddm)-400 has also been studied by TGA as shown in Figure 13. The 5 and 10% weight-loss temperatures (Td5 and Td10) for poly(oAI-a)-400 are 536 and 589°C, respectively. For poly(oAI-ddm)-400, the Td5 and Td10 are 503 and 555°C, respectively. The maxima of weight-loss rates for poly(oAI-a)-400 and poly(oAI-ddm)-400 are as high as 633 and 636°C, respectively. Besides, the char yield for poly(oAI-a)-400 and poly(oAI-ddm)-400 are as high as 71 and 60%, respectively. The data demonstrate extremely high thermal stability of both monomer and main-chain benzoxazine based cross-linked PBO thermosets. However, it was very difficult to measure mechanical properties since they were brittle.
Conclusion
A new class of amide-co-imide functional benzoxazines has been successfully synthesized. Among these new benzoxazine monomers, ortho-amide–imide functional benzoxazine resins show powerful advantages both in the synthesis of benzoxazine monomer or main-chain type polybenzoxazine and the properties of the corresponding thermosets. Besides, the poly(benzoxazole-co-imide) derived from the main-chain benzoxazine shows the Tg as high as over 400°C, and PBO thermosets based on the ortho-(amide-co-imide) functional benzoxazine monomer shows the Td5 as high as 536°C, appointing these materials as a good candidate for high-performance applications.
Conflict of Interest Statement
The authors declare that the research was conducted in the absence of any commercial or financial relationships that could be construed as a potential conflict of interest.
Acknowledgment
Kan Zhang is indebted to the partial support of Chinese Scholarship Council (CSC) Program.
References
Agag, T., Arza, C. R., Maurer, F. H., and Ishida, H. (2010). Primary amine-functional benzoxazine monomers and their use for amide-containing monomeric benzoxazines. Macromolecules 43, 2748–2758. doi:10.1021/ma902556k
Agag, T., Liu, J., Graf, R., Spiess, H. W., and Ishida, H. (2012). Benzoxazole resin: a novel class of thermoset polymer via smart benzoxazine resin. Macromolecules 45, 8991–8997. doi:10.1021/ma300924s
Chernykh, A., Liu, J., and Ishida, H. (2006). Synthesis and properties of a new crosslinkable polymer containing benzoxazine moiety in the main chain. Polymer 47, 7664–7669. doi:10.1016/j.polymer.2006.08.041
Dunkers, J., and Ishida, H. (1995). Vibrational assignments of 3-alkyl-3, 4-dihydro-6-methyl-2H-1, 3-benzoxazines in the fingerprint region. Spectrochim. Acta A. 51, 1061–1074. doi:10.1016/0584-8539(94)00114-Q
Feng, D., Wang, S., Zhuang, Q., Guo, P., Wu, P., and Han, Z. (2004). Joint theoretical and experimental study of the UV absorption spectra of polybenzoxazoles. J. Mol. Struct. 707, 169–177. doi:10.1016/j.molstruc.2004.07.018
Fukumaru, T., Fujigaya, T., and Nakashima, N. (2012). Extremely high thermal resistive poly (p-phenylene benzobisoxazole) with desired shape and form from a newly synthesized soluble precursor. Macromolecules 45, 4247–4253. doi:10.1021/ma3006526
Fukumaru, T., Saegusa, Y., Fujigaya, T., and Nakashima, N. (2014). Fabrication of poly (p-phenylenebenzobisoxazole) film using a soluble poly(o-alkoxyphenylamide) as the precursor. Macromolecules 47, 2088–2095. doi:10.1021/ma4024526
Hamerton, I., Thompson, S., Howlin, B. J., and Stone, C. A. (2013). New method to predict the thermal degradation behavior of polybenzoxazines from empirical data using structure property relationships. Macromolecules 46, 7605–7615. doi:10.1021/ma401444z
Holly, F. W., and Cope, A. C. (1944). Condensation products of aldehydes and ketones with o-aminobenzyl alcohol and o-hydroxybenzylamine. J. Am. Chem. Soc. 66, 1875–1879. doi:10.1021/ja01239a022
Imai, Y. (1996). “Synthesis of polyamideimides,” in Polyimide: Fundamentals and Applications, eds M. K. Ghosh and K. L. Mittal (New York, NY: Marcel Dekker Press), 49–70.
Ishida, H., and Allen, D. J. (1996). Mechanical characterization of copolymers based on benzoxazine and epoxy. Polymer 37, 4487–4495. doi:10.1016/0032-3861(96)00303-5
Ishida, H., and Low, H. Y. (1997). A study on the volumetric expansion of benzoxazine-based phenolic resin. Macromolecules 30, 1099–1106. doi:10.1021/ma960539a
Ishida, H., Wellinghoff, S. T., Baer, E., and Koenig, J. L. (1980). Spectroscopic studies of poly [N, N’-bis (phenoxyphenyl) pyromellitimide]. 1. Structures of the polyimide and three model compounds. Macromolecules 13, 826–834. doi:10.1021/ma60076a011
Jaffe, M., Haider, M. I., Menczel, J., and Rafalko, J. (1992). Thermal characterization of high performance PBI and 6F polymers and their alloys. Polym. Eng. Sci. 32, 1236–1241. doi:10.1002/pen.760321709
Kim, H. J., Brunovska, Z., and Ishida, H. (1999a). Dynamic mechanical analysis on highly thermally stable polybenzoxazines with an acetylene functional group. J. Appl. Polym. Sci. 73, 857–862. doi:10.1002/(SICI)1097-4628(19990808)73:6<857:AID-APP1>3.0.CO;2-C
Kim, H. J., Brunovska, Z., and Ishida, H. (1999b). Molecular characterization of the polymerization of acetylene-functional benzoxazine resins. Polymer 40, 1815–1822. doi:10.1016/S0032-3861(98)00393-0
Liu, J., and Ishida, H. (2014). Anomalous isomeric effect on the properties of bisphenol F-based benzoxazines: toward the molecular design for higher performance. Macromolecules 47, 5682–5690. doi:10.1021/ma501294y
Meador, M. A. (1998). Recent advances in the development of processable high-temperature polymers. Annu. Rev. Mater. Sci. 28, 599–630. doi:10.1146/annurev.matsci.28.1.599
Ning, X., and Ishida, H. (1994). Phenolic materials via ring-opening polymerization: synthesis and characterization of bisphenol-A based benzoxazines and their polymers. J. Polym. Sci. A Polym. Chem. 32, 1121–1129. doi:10.1002/pola.1994.080320614
Park, H. B., Jung, C. H., Lee, Y. M., Hill, A. J., Pas, S. J., and Mudie, S. T. (2007). Polymers with cavities tuned for fast selective transport of small molecules and ions. Science 318, 254–258. doi:10.1126/science.1146744
Pubmed Abstract | Pubmed Full Text | CrossRef Full Text | Google Scholar
Tullos, G. L., Powers, J. M., Jeskey, S. J., and Mathias, L. J. (1999). Thermal conversion of hydroxy-containing imides to benzoxazoles: polymer and model compound study. Macromolecules 32, 3598–3612. doi:10.1021/ma981579c
Velez-Herrera, P., and Ishida, H. (2009). Low temperature polymerization of novel, monotropic liquid crystalline benzoxazines. J. Polym. Sci. A Polym. Chem. 47, 5871–5881. doi:10.1002/pola.23632
Wang, C. F., Su, Y. C., Kuo, S. W., Huang, C. F., Sheen, Y. C., and Chang, F. C. (2006). Low-surface-free-energy materials based on polybenzoxazine. Angew. Chem. Int. Ed. 45, 2248–2251. doi:10.1002/anie.200503957
Yagci, Y., Kiskan, B., and Ghosh, N. N. (2009). Recent advancement on polybenzoxazine – a newly developed high performance thermoset. J. Polym. Sci. A Polym. Chem. 47, 5565–5576. doi:10.1002/pola.23597
Zhang, K., Zhuang, Q., Liu, X., Yang, G., Cai, R., and Han, Z. (2013). A new benzoxazine containing benzoxazole-functionalized polyhedral oligomeric silsesquioxane and the corresponding polybenzoxazine nanocomposites. Macromolecules 46, 2696–2704. doi:10.1021/ma400243t
Keywords: benzoxazine, polybenzoxazine, amide–imide, benzoxazole, thermoset, high performance
Citation: Zhang K and Ishida H (2015) Smart synthesis of high-performance thermosets based on ortho-amide–imide functional benzoxazines. Front. Mater. 2:5. doi: 10.3389/fmats.2015.00005
Received: 03 December 2014; Accepted: 19 January 2015;
Published online: 02 February 2015.
Edited by:
Miroslav Slouf, Institute of Macromolecular Chemistry, Czech RepublicReviewed by:
Jaroslav Mosnacek, Polymer Institute of the Slovak Academy of Sciences, SlovakiaTsutomu Takeichi, Toyohashi University of Technology, Japan
Copyright: © 2015 Zhang and Ishida. This is an open-access article distributed under the terms of the Creative Commons Attribution License (CC BY). The use, distribution or reproduction in other forums is permitted, provided the original author(s) or licensor are credited and that the original publication in this journal is cited, in accordance with accepted academic practice. No use, distribution or reproduction is permitted which does not comply with these terms.
*Correspondence: Hatsuo Ishida, Department of Macromolecular Science and Engineering, Case Western Reserve University, Cleveland, OH 44106, USA e-mail: hxi3@cwru.edu