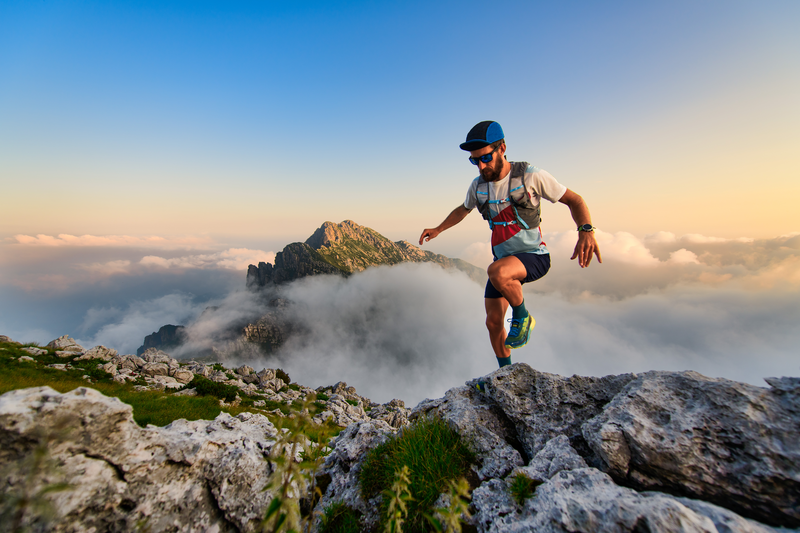
95% of researchers rate our articles as excellent or good
Learn more about the work of our research integrity team to safeguard the quality of each article we publish.
Find out more
REVIEW article
Front. Mar. Sci. , 24 March 2025
Sec. Marine Affairs and Policy
Volume 12 - 2025 | https://doi.org/10.3389/fmars.2025.1576661
This article is part of the Research Topic Challenges and Opportunities for Decarbonizing the Maritime Industry View all 7 articles
In recent years, the International Maritime Organization (IMO) has set strict emission standards for the shipping industry, which has raised high demands for ship emission reduction technologies. This review analyzes the research status of ship emission reduction technologies using bibliometric methods based on 714 publications from the Social Science Citation Index (SSCI) and SCI-Expanded (SCIE) databases from 2000 to 2024, and use VOSviewer software for the visualization of publications. It identifies key trends, productive entities and key contributors, and research hotspots in the field. The core findings are that green power technologies, digital intelligence technologies, and emission reduction technologies are current focal points. Future research should focus on finding optimal solutions for alternative marine fuels, clarifying the low-carbon transformation pathways for ships, and promoting effective follow-up actions from all relevant parties.
Shipping is currently the primary mode of transportation in international trade, carrying nearly 90% of global goods trade (Xiao et al., 2024, 2025). However, the majority of vessels currently used in shipping rely on fossil fuels and have low energy efficiency, leading to environmental pollution. In response to pollution issues, the International Maritime Organization (IMO) has successively implemented measures such as establishing Emission Control Areas (ECA), enforcing a global sulfur limit, and introducing the existing Energy Efficiency Existing Ship Index (EEXI) and Carbon Intensity Indicator (CII) rating system to restrict ship emissions, leading to increasing policy pressure on the shipping industry. Therefore, ship emission reduction technology has become a focus of scholarly attention and an urgent issue that needs to be addressed. This review focuses on ship emission reduction technology and analyzes this field using bibliometric methods.
This review is based on literature selected from the Social Science Citation Index (SSCI) and SCI-Expanded (SCIE) databases of Web of Science related to ship emission reduction technologies. The literature search results show that the number of literature reviews related to ship emission reduction technologies has been increasing year by year. It has been observed that the current research hotspots in this field mainly focus on alternative fuels, speed and route optimization, green hull design, and ship waste heat recovery. Parris et al. (2024) discusses the production methods, advantages, and challenges faced in the current development of methanol as a marine alternative fuel, asserting that methanol has the advantages of high efficiency, good cost-effectiveness. Shi et al. (2023) reviewed the production methods of green power and analyzed the potential of green fuels in the shipping industry. Deng et al. (2021) summarized the emission reduction technologies of marine diesel engines through three pathways: fuel optimization, pre-combustion control, and exhaust gas treatment, and comprehensively assessed the application of LNG in marine diesel engines. Wang et al. (2022) comprehensively summarized alternative fuels available for ships in the short and long term, including LNG, and emphasized that the use of alternative fuels still faces some challenges. Lion et al. (2020) reviewed the development of medium and low-speed diesel engines, with a focus on the potential of diesel engines in the application of waste heat recovery systems. Nuchturee et al. (2020) introduced technologies suitable for the onboard energy systems of fully electric vessels and provided recommendations for the development of new energy vessels, including fully electric ships. Risso et al. (2023) reviewed the development of onboard carbon capture and storage technology.
At present, there are fewer reviews on ship emission reduction technologies, especially lacking a systematic bibliometric analysis. Therefore, this paper aims to use bibliometric methods to identify the most active authors, journals, and countries in the field of ship emission reduction technology research from 2000 to 2024, outline the development trajectory of ship emission reduction technology, accurately reveal the current research hotspots and future development trends in this field, and help researchers quickly familiarize themselves with the area. Additionally, this paper also outlines the difficulties currently faced in ship emission reduction and provides some meaningful examples and practical suggestions to serve as strong references for subsequent policy formulation, technological research and development, and industrial development, promoting ship emission reduction technology to new heights.
This section introduces bibliometric methods. Section 2.1 provides an overall introduction to bibliometric methods, while sections 2.2 and 2.3 demonstrate the processes of data collection and information retrieval.
Bibliometrics is a method for conducting quantitative analysis of various characteristics of academic literature. It focuses on literature and bibliometric characteristics as the research objects, revealing the distribution structure of literature in a specific research field through statistical analysis of indicators such as authors, journal sources, citations, and citation counts (Mukherjee et al., 2022). Bibliometric methods have important economic implications. For example, the quantitative analysis reduces the interference of subjective factors, and provide important references for subsequent research, avoid redundant studies, optimize the allocation of academic resources, and the research results can provide data support and decision-making references for the government and policymakers. This paper constructs a comprehensive analytical framework and uses VOSviewer version 1.6.20 to analyze and visualize academic literature on ship emission reduction technologies published from 2000 to 2024 (The period from 2000 to 2024 is a reasonable base period as it comprehensively covers the evolution of ship emission reduction technologies, aligns with policy changes, benefits from abundant and reliable data, and reflects the industry’s transformation and the development of research methods), as shown in Figure 1. This paper will include the following aspects: (1) annual publication trend analysis; (2) source journal analysis; (3) collaboration network analysis; (4) keyword analysis.
Web of Science (WoS) is a globally leading academic literature retrieval platform developed and maintained by Clarivate. It enjoys a high reputation in the academic community, with strict inclusion criteria, and the literature it indexes is highly authoritative, widely recognized by researchers, making it a primary platform for academic literature retrieval. Therefore, we use the SSCI and SCIE databases of WoS as the data sources for this review.
This article focuses on ship emission reduction technologies, thus the selection of keywords (such as ships, boats, environmental protection, energy conservation, etc.) revolves around the development and application of ship emission reduction technologies in the context of sustainable development. According to the survey, there are various types of ship emission reduction technologies, which are currently mainly divided into three categories: the first is green power technologies for ships, the second is digital and intelligent technologies, and the third is ship emission reduction technologies. Therefore, this review aims to comprehensively cover the three aforementioned technologies when selecting keywords (such as alternative fuels, shore power, optimization, etc.) in order to enhance the breadth and depth of the search data as much as possible.
The following will provide a detailed explanation of the selection of keywords for this review. Core basic keywords: “ships,” “boats,” and “vessels” clearly define the research subject as ships, ensuring the relevance of the search scope and excluding unrelated topics.
Topic keywords: “energy conservation,” “environmental protection,” and “emission” clearly define the search scope related to environmental protection, energy saving, and emission reduction. The use of multiple keywords in parallel can also expand the search range for academic publications, incorporating more relevant literature as references.
Keywords related to ship emission reduction technologies: “design,” “engine,” “propulsion system” summarize green power technologies for ships; “technologies,” “alternative fuels” emphasize alternative fuel technologies for ships; “shore power,” “sulfur,” “optimization” correspond to shore power, desulfurization towers, scrubbers, and speed and route optimization for emission reduction technologies. The above keywords cover the most popular directions in the field of ship emission reduction technologies, expanding the search scope.
Table 1 provides the specific search parameters.
This section analyzing the annual publication volume and trends in this field to better understand the evolution of research. Figure 2 shows the number of publications in this research area and the changing trends since 2009. Prior to 2009, due to a relatively weak awareness of sustainable development in academia, there was insufficient innovation awareness and motivation for ship emission reduction technologies, resulting in a low volume of related publications. From Figure 2, it can be observed that the publication volume of related literature maintained a steady upward trend from 2009 to 2016. Starting in 2017, the publication volume began to accelerate, showing a year-on-year increasing trend, and this rapid growth continued until it peaked in 2023. In 2024, there was a slight decline, but the volume remained at a high level. From 2021 to 2024, the total publication volume reached 430 articles, accounting for 60% of the total number, indicating that ship emission reduction technologies emission reduction technologies for ships have become an important issue in recent years.
The reasons for this trend are as follows: Firstly, the IMO confirmed in October 2016 that a global shipping sulfur limit of 0.50% would be implemented in 2020. In response to this policy, ship emission reduction technologies became a research hotspot, leading to a rapid increase in literature after 2016 (Yang et al., 2023). Secondly, technological advancement plays a crucial role in this context, with the application of new energy technologies in the shipping sector becoming increasingly widespread. In particular, continuous breakthroughs in technologies such as LNG fuel, electric vessels, and hydrogen fuel cell ships have opened up broad prospects for the development of ship emission reduction technologies. Finally, the strong funding from governments and international organizations for research projects, along with the deepening of the industry-university-research cooperation model, has provided solid financial support and an efficient collaborative platform for research in this field.
This review involves 714 publications from 166 different journals. Table 2 lists the top 15 journals in order of publication volume, from highest to lowest. These 15 journals published a total of 348 publications, accounting for 48.7% of the total. From the table, it can be seen that the three journals with the highest publication volumes are the “Journal of Marine Science and Engineering” (73 publications), “Ocean Engineering” (48 publications), and “Journal of Cleaner Production” (28 publications), which together published 149 publications, accounting for 20.8% of the total. Notably, the “Journal of Marine Science and Engineering” alone published 73 publications, accounting for 10.2% of the total, indicating that this journal places significant emphasis on research related to ship emission reduction technologies. The publications published in these journals provide important references for academic work in this field.
The Journal Citation Indicator (JCI) is a journal evaluation metric launched by Clarivate Analytics in 2021, used to measure the citation impact of recent publications in journals. The calculation of JCI is based on the Category Normalized Citation Impact (CNCI), which is a metric in the analysis and benchmarking tool Incites. JCI takes the average CNCI of all publications published in a given journal over the past three years (Torres-Salinas et al., 2022). From the perspective of JCI, journals such as “Energy Conservation and Management” (1.97), “ Transportation Research Part D: Transport and Environment “ (1.63), “Applied Energy” (1.62), and “Energy” (1.59) stand out, with JCI indices exceeding 1.5. These journals have significant academic influence in the field and ensure the high quality of the published literature through a strict review mechanism, which can directly promote the development of ship emission reduction technology.
Table 2 also includes several journals closely related to the field of environmental protection, such as “Sustainability,” “ Transportation Research Part D: Transport and Environment,” “Environment,” and “Environmental Science & Technology.” This indicates a strong correlation between research on ship emission reduction technologies and environmental protection. The aforementioned journals pay more attention to the environmental benefits brought by the use of ship emission reduction technologies. Additionally, there are some journals in the energy field, such as “Energies,” “Energy Conservation and Management,” “Energy,” and “Fuel,” which indicates that the application of new energy in shipping has become a research hotspot.
This section will analyze the collaborative relationships in the publications from three perspectives: the country, the institution, and the author.
By analyzing the national information from the literature selected in this review, we can understand the current state of research on ship emission reduction technologies in various countries and the cooperative relationships between them. From 2000 to 2024, scholars from 58 different countries have published publications in the SSCI and SCIE databases, contributing to the development of ship emission reduction technologies. Table 3 lists the top 10 countries with the most published publications in this field from 2000 to 2024. It can be observed that China is the country with the most publications, totaling 287 publications, while South Korea and the United Kingdom rank second and third with 58 and 44 publications, respectively. Together, China, South Korea, and the United Kingdom have published 389 publications, accounting for 54.48% of the total, with more than half of the publications originating from these three countries.
This review utilizes VOSviewer version 1.6.20 software to construct a cooperation network map. The specific search steps are shown in Table 4. Figure 3 displays the cooperation network among countries that have collaborated on at least four publications. There are a total of 33 projects, 5 groups, and a total link strength of 274. Ranked by the number of cooperating countries, China’s cooperation network is in the lead, having collaborated with 24 countries, with a total link strength of 94, followed by the United States (16 cooperating countries, total link strength of 34) and Sweden (16 cooperating countries, total link strength of 32). The thickness of the lines connecting the nodes in Figure 3 indicates the strength of the connections between countries. It was observed that China has closer cooperation with countries such as Singapore and the United Kingdom, and the top five countries with the highest publication numbers have formed a relatively extensive cooperation network. However, it appears that, apart from China, other countries have relatively few intercontinental collaborations. For example, the United Kingdom has 12 cooperating countries, 8 of which are European countries.
As shown in Figure 3, in addition to the 10 countries listed in Table 4, there are also some countries with relatively few published documents, such as Portugal, Belgium, and Lithuania, which have made significant contributions in this field. For example, research teams from the University of Lisbon and the University of Porto have achieved a series of results in optimizing LNG fuel ship propulsion systems, but their collaboration with other countries is relatively limited, and the intensity of cooperation is low, resulting in comparatively fewer outcomes. In the future, it is necessary to further expand the international cooperation network, strengthen communication between different countries, and promote the development of ship emission reduction technologies. The main reason for China’s significant achievements in this field lies in the strategic support and policy drive at the national level, as well as the deep cooperation between universities and enterprises, forming a complete chain of “university technology research - enterprise engineering application,” which can effectively promote the implementation of academic results. These experiences are worth learning from for other countries.
This review covers 714 publications from 712 different organizations. Table 5 lists the 10 most active organizations worldwide in the field of low-carbon emission reduction for ships, which collectively published 270 publications, accounting for nearly 38% of the total. The top five organizations are all from China, while the remaining four are from Europe, and one organization is from South Korea. Specifically, Shanghai Maritime University ranks first with 51 publications, followed by Wuhan University of Technology (45 publications) and Dalian Maritime University (38 publications) in second and third places, respectively. The fourth place is held by Harbin Engineering University (27 publications), and the fifth is Hong Kong Polytechnic University (23 publications). A large number of academic achievements have emerged in China in the field of ship emission reduction technology, thanks to the Chinese government’s high regard and strong support for research in this area, which has greatly enhanced the enthusiasm of Chinese scholars for scientific research. Another Asian institution mentioned in the table—Korea Maritime and Ocean University—has published 16 related publications, ranking jointly with the Technical University of Denmark in 8th place.
This review utilizes VOSviewer version 1.6.20 software to construct an organizational collaboration network. The specific search process is detailed in Table 6. Figure 4 presents the inter-organizational collaboration network with a minimum document count of 7, which encompasses 32 projects, forming 7 groups, and shows a total connection strength of 288. In Figure 4, the size of the projects visually reflects the number of publications published by each organization; the larger the project, the greater the number of publications. The thickness of the lines between organizations indicates the degree of collaboration among them.
Observations reveal that Shanghai Maritime University (5 cooperative organizations, total link strength 12) leads in the number of published publications. Despite its abundant publication achievements, it is somewhat lacking in the breadth of its cooperative network, having established collaborative relationships only with five organizations, including Harbin Engineering University and Fudan University (Guo H. et al., 2024). Shanghai Maritime University should actively expand its academic cooperation network in the future, sharing resources and complementing advantages with other organizations, in order to achieve more outstanding results in the field of scientific research.
In contrast, Dalian Maritime University (with 11 cooperative organizations and a total link strength of 23) and Hong Kong Polytechnic University (also with 11 cooperative organizations and a total link strength of 22) have both established relatively extensive cooperation networks, and there is also a close cooperative relationship between the two (Guo Y. et al., 2024). The collaboration among these top organizations has undoubtedly injected strong momentum into the research and development in this field. Looking ahead, organizations should further strengthen their cooperation, not only between higher education institutions but also actively consider deep collaboration with diverse entities such as governments and non-governmental organizations.
Author level analysis can help us identify influential authors in this research field and gain a deep understanding of the research dynamics of leading figures in the field. A total of 2,327 authors participated in the 714 publications selected for this review. Table 7 shows the number of publications, the percentage of total publications, and the individual H-index of the 15 authors with the highest number of published publications. The H-index is a comprehensive indicator that measures a scholar’s academic output and impact, indicating that they have h papers, each cited at least h times, balancing quantity and quality. Overall, 8 out of the 15 authors are from China. Most authors in the table have a high H-index, indicating their authority.
This review constructed an author collaboration network using VOSviewer software version 1.6.20, with the specific search process shown in Table 8. Figure 5 illustrates the author collaboration network with a minimum document count of 4, comprising 72 items, 23 clusters, and a total link strength of 502. The size of the items in the figure represents the number of published publications, the thickness of the lines connecting the items indicates the strength of collaboration between authors, and the color of the items reflects the results of the clustering analysis. Scholars with the same hue belong to the same research cluster. It can be observed from the figure that a considerable number of research clusters have formed in this field, and there are some inter-cluster collaboration relationships.
Specifically, Shuaian Wang, who leads in both the number of published publications and H-index (with 4 co-authors and a total link strength of 11), has four co-authors: Lu Zhen, Ran Yan, Qiang Meng, and Harilaos Psaraftis, all of whom belong to the lower central red cluster and jointly research topics such as ship scheduling, speed and range optimization, and fuel consumption optimization (Qi et al., 2023; Yan et al., 2024). In the deep yellow cluster on the right side of the center, Nikola Vladimir from the University of Zagreb (5 co-authors, total link strength of 16) is the main author, who has collaborated multiple times with Ailong Fan from the Wuhan University of Technology on topics related to the life cycle cost assessment (LCCA) of marine alternative fuels and the decarbonization of marine power systems (Fan et al., 2023, 2022). Ibrahim Seddiek from the Arab Academy for Science, Technology & Maritime Transport (with 2 co-authors and a total link strength of 6) has published 10 publications, with his research primarily focusing on marine alternative fuels, ship energy systems, and new energy propulsion technologies, achieving a series of outstanding results (Ammar and Seddiek, 2023; Elkafas and Seddiek, 2024). Although the author has published a relatively large number of publications, they have not formed a broad collaborative network. Song Zhou from Harbin Engineering University (4 co-authors, total link strength of 12) is the main author in the deep green cluster positioned in the upper middle. He has conducted systematic research on the emission reduction design of ship engines and the waste heat recovery systems for ships. In 2020, he proposed a waste heat recovery system that combines a supercritical CO2 Brayton cycle power generation system with a Kalina cycle power generation system in the journal “Energy Conversion and Management,” demonstrating strong innovation and significant emission reduction effects (Feng et al., 2020; Guo et al., 2020; Zhou et al., 2023). It is worth mentioning that the figure shows that many scholars have few co-authors, limited to issues within their research teams. Ship emission reduction technology is interdisciplinary. To ensure the scientific and objective nature of the research, future collaborations with other scholars should be moderately increased.
This review utilizes VOSviewer software version 1.6.20 to conduct co-occurrence analysis of keywords, helping to quickly understand the current hot research areas in ship emission reduction technology. Table 9 lists the 20 most frequently occurring keywords from 714 pieces of literature, along with their corresponding frequencies and total link strength. “Ships,” as the main subject of research, ranks second with a frequency of 108 occurrences. Terms related to emissions such as “Emissions,” “Ship emissions,” “Emission reduction,” and “Exhaust emissions” also appear in the table; these keywords are fundamental vocabulary in the fields of environmental protection, low carbon, and emission reduction. Additionally, the appearance of keywords like “optimization” and “speed optimization” indicates that using optimization methods for ship speed and range to reduce pollutant emissions has become a relatively popular topic in academia. The presence of keywords such as “energy efficiency,” “alternative fuels,” and “natural gas” reflects scholars’ emphasis on alternative fuels for ships, clean energy, and enhancing energy efficiency. Keywords like “design” and “system” are related to the green optimization design of ships.
Figure 6 shows the co-occurrence network of all keywords. Each element in the figure represents a keyword, and the size of the element indicates the frequency of the keyword’s occurrence. When two or more keywords appear in the context of the same publication, it is referred to as keyword co-occurrence. The distance between elements represents the strength of the association between different keywords; the closer the distance between two elements, the stronger their association. The color of the elements represents clusters; elements of the same color belong to the same cluster, indicating a strong association, while different colors signify that they come from different clusters. The thickness of the lines between elements represents the robustness of the association. In the co-occurrence network of keywords in Figure 6, the minimum occurrence threshold is set to 10, resulting in 71 elements, 5 clusters, and a total link strength of 13,213.
Observations indicate that keywords appearing 10 times or more are divided into five clusters, namely red, green, purple, blue, and yellow. The main keywords in the red and purple clusters include “optimization,” “design,” and “model,” with a focus on optimizing navigation speed, routes, and fleet design, as well as specific ship emission reduction technologies such as desulfurization towers, shore power, and new energy propulsion technologies. The main keywords in the green cluster are “alternative fuels,” “performance,” and “diesel engine,” focusing on the use of alternative fuels for ships and the green optimization of marine diesel engines. The main keywords in the blue cluster are “ship emissions,” “exhaust emissions,” and “air pollution,” which primarily study the monitoring of ship pollution emissions and the hazards posed by these emissions. The aforementioned four clusters summarize the current popular research hotspots in this field and provide directions for future research. The main keywords in the yellow cluster are “energy efficiency,” “emissions reduction,” and “technologies,” which are common terms frequently appearing in most publications. In the following fourth section, this review categorizes ship emission reduction technologies into (1) green power technologies, (2) digital intelligence technologies, and (3) emission reduction technologies, with a focus on introducing these three categories in order.
This review focuses on two main aspects of green power technology for ships: alternative fuels and fuel cells, and ship energy systems.
Table 10 lists the main alternative fuels currently entering the industry’s view. The alternative fuels such as LNG, methanol, and ammonia fuel have very low sulfur content, and the sulfur oxide emissions produced during combustion are significantly reduced compared to traditional fuels. Currently, the industry is actively researching various feasible alternative fuels. Radonja et al. (2019) introduced the advantages and disadvantages of methanol and ethanol as alternative fuels for ships based on their physical properties, concluding that methanol and ethanol are not the optimal fuel choices for future ships, but rather alternative solutions at this stage. Gore et al. (2022) studied the cost-effectiveness of four alternative fuels—LNG, methanol, green hydrogen, and green ammonia, concluding that green hydrogen is the best choice for future alternative fuels. Atilhan et al. (2021) comprehensively assessed the feasibility of green hydrogen as an alternative fuel from the perspectives of production, economic performance, storage conditions, and safety. In addition, the diversity of alternative fuels has raised a new question: how should alternative fuels be selected, and according to what criteria (Zwaginga et al., 2024)? To address this issue, Moshiul et al. (2023) proposed a comprehensive assessment framework that identifies the best alternatives based on the Technique for Order Preference by Similarity to Ideal Solution (TOPSIS) method. In April 2023, the China Classification Society (CCS) launched a comprehensive assessment system for ship clean energy, which provides consulting services to offer decision-making references for governments and enterprises in selecting alternative fuels.
A fuel cell is an advanced energy conversion device that can directly and efficiently convert the rich chemical energy contained in fuel into electrical energy through electrochemical reactions. This process reduces energy loss and lowers carbon emissions (Lee, 2022). In the exploration and application of fuel cell technology, many scholars are committed to promoting its development toward a more environmentally friendly and economical direction. Percic et al. (2022) regard green hydrogen and green ammonia as zero-carbon fuels, identifying alternatives to diesel-powered vessels by considering the environmental and economic indicators of the power systems in question, utilizing life cycle assessment (LCA) and LCCA. Baldi et al. (2020) studied the impact of solid oxide fuel cells (SOFC) on energy savings and emission reductions in ships using an optimization-based approach. In October 2023, the hydrogen fuel cell-powered vessel “Three Gorges Hydrogen Boat 1” completed its maiden voyage in Hubei, China. Compared to traditional fuel-powered ships, it is expected that this vessel can replace 103.16 tons of fuel annually, reducing CO2 emissions by 343.67 tons.
The ship energy system refers to the systems used on ships to provide power and electricity, including propulsion systems, power systems, etc. It integrates these systems into one in the form of electrical energy, providing power for ship propulsion, communication and navigation, special operations, and daily equipment through the power network, achieving comprehensive utilization of energy on the entire ship (Cheng et al., 2022; Ma et al., 2023). Scholars have also conducted research on the energy systems of ships. Gabbar et al. (2021) evaluated the “Nuclear-Renewable Energy Hybrid Energy System (N-R HES)” based on Key Performance Indicators (KPI) including the Cost of Energy (COE) and Net Present Cost (NPC), and compared it with other energy systems, concluding that N-R HES may be the optimal energy system for emission reduction. Pan et al. (2021) examined the progress of integrating renewable energy into traditional ship power systems, as well as the basic working principles and application methods of renewable energy, including solar energy, wind energy, and fuel cells. They introduced the core technologies required for the development of existing renewable energy vessels and revealed the immense potential of renewable energy power systems for ships. In April 2024, the world’s first pure electric-powered container ship, “COSCO SHIPPING GREENWATER 01,” docked at Nanjing Port in China. It is equipped with 36 mobile box-type lithium iron phosphate batteries, totaling 50,000 kWh capacity, addressing the issue of short voyage distances for electric ships. This ship sets a new benchmark for the shipping industry’s low-carbon and environmentally friendly transformation, achieving “zero emission, zero pollution, zero noise.”
In recent years, the development of intelligent digital technologies has been rapid, and their application in the shipping industry has also expanded quickly, changing the landscape of the shipping industry at an unprecedented pace Peng et al. (2025). These technologies have greatly promoted the greening process of the shipping industry (Wang et al., 2023; Xue and Lai, 2024).
Artificial intelligence, big data, machine learning, and AIS are widely used in shipping (Luo et al., 2024a; Luo et al., 2025). These technologies enable vessels to identify real-time weather and traffic conditions, and automatically plan optimal routes and speeds (Luo et al., 2024b). This helps ships avoid congested areas, shorten distances, and utilize favorable conditions like tailwinds and currents, significantly reducing energy consumption. Navigational speed is crucial for energy and emissions. While reducing speed can cut emissions, it must balance efficiency and safety. Choosing the optimal economic speed can reduce costs and emissions, especially in long-distance shipping (Liu H. et al., 2025; Xiang et al., 2025). Chen et al. (2023) proposed a multi-objective path optimization algorithm based on field theory, applied to Arctic route design. The algorithm determines the navigation area based on the vessel’s navigational capabilities and utilizes the gradient descent principle between the starting point and destination for path optimization, providing a reference for route design. Zheng et al. (2019) introduced artificial neural networks to predict fuel consumption of cruise ships, setting the minimization of fuel consumption as the objective based on historical data. They combined the neural network with four improved particle swarm optimization algorithms to select the optimal sailing speed between stations. Zhang et al. (2022) proposed a modeling approach for optimizing the navigation speed of inland vessels on the Yangtze River in China, along with a heuristic algorithm - the Adaptive Lagrangian Differential Evolution method (ALDE). They constructed a comprehensive discrete model and a fuel consumption model, establishing an optimization function for the target navigation speed of inland vessels. In April 2022, China’s Zhifei intelligent navigation container ship was put into operation, and as of now, it has completed over 400 voyages covering more than 20,000 nautical miles, with stable autonomous planning of both route and speed. In June 2022, South Korea’s “Prism Courage,” an ultra-large LNG autonomous navigation vessel, completed transoceanic autonomous navigation testing, during which the ship autonomously planned the optimal route. Fuel efficiency improved by 7%, and greenhouse gas emissions were reduced by 5%. These examples demonstrate the significant potential of using intelligent technology to optimize route.
With the implementation of IMO sulfur emission limitation regulations and the establishment of ECAs, shipowners mainly choose to use low-sulfur fuels or install ship desulphurization towers to meet the requirements (Zhong et al., 2021; Shu et al., 2023). The use of low-sulfur oil, which is obtained by reducing the sulfur content of crude oil through refining, can significantly reduce sulfur oxide emissions. In recent years, the price differential between high-sulfur oil and low-sulfur oil has remained high overall, which makes it a mainstream choice for ships to install desulfurization towers and then continue to use high-sulfur oil to gain an advantage in terms of cost. Marine desulphurization towers, also known as Exhaust Gas Cleaning Systems (EGCS), are devices installed on the exhaust pipes of ships’ main engines and generators. They promote full contact between exhaust gases containing sulfur oxides and scrubber water through spray scrubbing, so that the sulfur oxides in the exhaust gases are absorbed by the scrubber water and converted into harmless substances. Currently, they are categorized into three main types: open, closed, and hybrid (Endres et al., 2018). Scrubbers are usually located in front of or as part of a desulfurization tower for the initial treatment of flue gases. Long et al. (2021) proposed an advanced square scrubber that is small in size, light in weight, and high in efficiency. Qi et al. (2023) developed a mixed-integer nonlinear model to explore the fleet cost issue of installing and using a scrubber. The results showed that the use of scrubbers can effectively reduce operating costs and suggested that the installation of scrubbers should be divided into a number of years to reduce the financial pressure of installing scrubbers. In the future, the market for low-sulfur oil and desulfurization towers has a wide range of prospects.
Shore power, also known as cold ironing, is related to the energy supply when ships dock. When a ship docks at a port, all onboard thermal power sources are shut down, allowing the engine to cool completely. However, electrical power remains necessary for onboard operations. To address this, the ship’s shore power system is utilized: once the vessel is moored, the port’s power supply system transmits electricity through a ship-to-shore connection to the vessel’s onboard system, replacing fuel-based power generation (Sun et al., 2022; Wang et al., 2024). Currently, due to the high-cost constraints of installation and ship modification, shore power technology is still an under-utilized technology, and the diverse power requirements of different types of ships and different operational scenarios have posed a great challenge to the diffusion of cold ironing technology. Bakar et al. (2023) outline the operation, power requirements, and challenges faced by the cold ironing technology and discuss how to achieve the ultimate goal of zero carbon emissions from shipping through the synergy of cold ironing and synergy between port microgrids to achieve the ultimate goal of zero carbon emissions from shipping. Peddi et al. (2024) Estimates potential reductions in pollutant emissions from the large-scale use of the cold iron process in Europe, identifies challenges to the large-scale use of the cold iron process, and provides solutions. Nguyen et al. (2021) evaluated the reduction of four air pollutant emissions after the adoption of shore power in the port of Kaohsiung, Taiwan, China, estimating vessel emissions based on auxiliary engine operating loads, average berthing time, and emission factors, and the results showed that the highest emission reduction benefit of shore power was 8.70% +/- 2.10% for Nox and 11.74% for SO2 +/-2.95%. Rolán et al. (2019) innovatively suggest that the Port of Barcelona use cold ironing technology that is entirely powered by renewable energy systems to further reduce polluting emissions. In 2024, China’s State Grid built the world’s first near-zero carbon emission port microgrid in Lianyungang, Jiangsu Province, which contains 16 sets of port shore power systems with a total capacity of 36,200 kVA, generating more than 6,000,000 kWh of clean energy annually, saving 21,000 tons of standard coal consumption and reducing 56,000 tons of CO2 emissions, making it a replicable and popularized sample for zero-carbon port construction.
Shipboard Carbon Capture System refers to the separation and capture of CO2 from ship exhaust gas or fuel, and then transporting it to the destination by means of transportation to be utilized for resource utilization or injected into the seabed/strata for storage to prevent it from entering into the atmosphere, so as to realize the permanent emission reduction of CO2 emitted from the ship, and the core process includes four steps: capture, separation, compression, liquefaction, and storage and unloading of CO2 (Oh et al., 2024a; Oh et al., 2024b). The advantage of the shipboard carbon capture system is that the system does not require alternative fuels, and ships can achieve carbon emission compliance based on the use of the original traditional fossil fuels, which saves a lot of investment and time for ship retrofitting. Jiang et al. (2024) introduced the current status of the combination of onboard carbon capture systems and low-carbon fuels, concluding that based on existing onboard carbon capture technologies, further utilization of the ship’s waste heat and cold energy should be pursued to meet the requirements of the IMO Energy Efficiency Design Index (EEDI), which can also significantly reduce the economic costs of onboard carbon capture systems. Wohlthan et al. (2024) explored carbon capture technologies on board ships based on oxyfuel combustion, and the results showed that the competitiveness of carbon capture from pure oxyfuel combustion is limited due to the reduced engine efficiency combined with the large demand for oxygen on board, and that the use of liquefied oxygen, a byproduct of the onshore electrolysis process, can be considered to avoid direct oxygen supply on board ships to improve energy efficiency. Stec et al. (2021) proposed the feasibility of using an amine-based carbon capture system on board a ship, and the publication analyzed the energy demand of the whole system and simulated the operational efficiency of the carbon capture system under tropical and Arctic conditions, respectively, and the results showed that this system can be used as a method to reduce EEDI. In January 2024, China Evergreen Marine’s 14,000 TEU container ship “EVERTOP” was put back into operation after being retrofitted with a desulphurization + carbon capture system by China State Shipbuilding Corporation (CSSC), which is the world’s first full-process carbon capture system for ships, marking the first time that the marine carbon capture technology realizes “CO2 capture - liquefaction storage - disembarkation - transshipment - reuse” in the whole process in a global scale. It is expected that 44,000 tons of carbon emission reduction can be achieved annually, extending the life of the ship for at least 12 years, and bringing more than 8 million US dollars of economic benefits for the ship owner.
Ship Waste Heat Recovery System is a system that recovers and utilizes all kinds of waste heat generated by a ship in the course of its operation. The system can convert waste heat into electricity or thermal energy needed for ship operation, enhancing energy utilization efficiency and reducing resource waste (Bashan and Kokkulunk, 2020). The advantages of waste heat recovery systems are that the technology is more mature, the equipment is simple to install and maintain, and the payback period is short, which can bring great economic and environmental benefits to ships by improving energy efficiency to reduce energy consumption and pollution emissions. Lu et al. (2022) proposed an adaptive waste heat recovery system based on CO2 mixtures, which can change its operating mode according to the energy demands of different seasons and working conditions, thereby reducing fuel consumption rates. Although the shore power technology mentioned in the previous section can avoid pollution during ship calls, it is not applicable to ships with short-term calls, to address this issue, Baldasso et al. (2020) proposed a waste heat recovery system that combines thermal energy storage and organic Rankine cycle (ORC) based technology to address the power needs of ships during short port calls, the literature based on a hypothetical ferry case (which requires 1 MW of auxiliary power during the berth period) The literature investigates the technical feasibility of the system based on a hypothetical ferry case (which requires 1 MW of auxiliary power during port calls) and compares its cost-effectiveness with the alternative of using batteries during the calls. The results show that, although the proposed system requires the installation of an 82 m³ storage tank, it is still economically competitive and is more suitable for installation, especially for newly built ships, and it is expected that the system will reduce the ferry’s CO emissions from ferries by 8%. Alfa Laval, a Swedish company, has developed the E-PowerPack system, an innovative waste heat recovery solution for ships based on ORC technology. Following the installation of this system on a particular vessel, it operates for 7200 hours annually, generating an output of 1080 MWh per year. With an electricity cost of $0.10 per kWh, the system leads to an annual cost saving of up to $115,000. The E-PowerPack system not only enhances the energy efficiency of ships but also significantly reduces fuel consumption and greenhouse gas emissions, thereby providing crucial support for the sustainable development of the shipping industry.
High Cost Challenge: According to Citibank’s predictions, decarbonization in the shipping industry will require approximately $2 trillion in investment, which is undoubtedly a significant financial burden for shipping companies (Tan et al., 2023; Xue et al., 2024). Most shipping companies prefer to adopt new technologies only after they have matured and costs have decreased. Taking alternative fuels as an example, many alternative fuels face difficulties in production and supply, with their output far below the quantities needed to achieve decarbonization targets. The imbalance between supply and demand leads to higher prices (Nguyen et al., 2023).
Vicious Cycle Challenge: Due to the uncertainty of the low-carbon transformation path for ships, there is a lack of confidence in the shipping industry’s innovation and application of new technologies. If companies do not use new technologies, ports are also reluctant to invest in infrastructure that matches these new technologies, and the lack of infrastructure further discourages companies from investing, creating a vicious cycle. Taking shore power as an example, due to the uncertainty of whether ships can use shore power, ports are often unwilling to invest in the construction of shore power facilities to prevent resource waste. According to statistics, currently, out of 6,000 ports worldwide, fewer than 200 provide shore power facilities.
Talent Shortage Challenge: The global shipbuilding industry is currently facing a severe talent shortage. As the shipbuilding sector accelerates its transition toward low-carbon practices, the market’s demand for composite talents who are proficient in ship manufacturing technology and familiar with green intelligent technology is becoming increasingly urgent. However, such composite talents are extremely scarce, and some existing crew members still need to improve their professional skills. When new emission reduction technologies are installed on ships, these crew members often struggle to quickly master the relevant operations, thereby limiting the effective promotion and application of emission reduction technologies. Furthermore, although ship emission reduction technologies are rapidly developing, talent cultivation is a time-consuming process that requires substantial resource investment. The education system’s adjustments in terms of professional settings, training objectives, and the provision of practical opportunities are lagging behind, making it difficult for students receiving traditional professional education to adapt to the new trends in industry development.
Firstly, although LNG technology is relatively mature and costs are relatively low, LNG has issues with methane leakage and has not fully achieved net-zero carbon emissions. The shipping industry generally views it as a transitional fuel for low-carbon transformation of vessels, rather than the optimal solution for future ship fuels. The industry should actively explore the possibility of applying other alternative fuels, including methanol, ammonia, and hydrogen, which have lower carbon emissions and higher energy density. After fully understanding the drawbacks of these fuels, a comprehensive approach should be taken to overcome them through technological innovation, infrastructure development, safety management, and other methods. Methanol has significant drawbacks such as high toxicity, strong corrosiveness, and demanding storage conditions. To tackle these issues, future solutions could focus on developing corrosion-resistant ship materials and creating comprehensive methanol storage systems. These systems would optimize storage facility design, improve efficiency, and enhance leak monitoring and prevention capabilities, thereby enhancing overall safety management (Brown and Hampp, 2023).
In addition, in recent years, the rapid development of intelligent technologies such as big data and artificial intelligence has driven the shipping industry toward digitalization and intelligence (Shu et al., 2024, 2024). Researchers should keenly grasp and adapt to this development trend. They should continue to explore the application of intelligent technologies in areas such as ship route planning, intelligent collision avoidance, emission monitoring, and energy management (Hao et al., 2024; Luo et al., 2022; Shu et al., 2024; Xin et al., 2025). The acquisition, processing, and analysis of data are currently key factors limiting the development of this technology. In the future, consideration can be given to how to enhance the reliability and stability of intelligent processors through technological innovation and structural optimization, such as employing cloud computing and edge computing technologies to improve data processing efficiency. Furthermore, to reduce the learning costs for crew members, the operating system should be simplified and optimized, using artificial intelligence technologies to provide crew members with warning alerts, operational recommendations, and other auxiliary functions. Maritime education should also develop talent training plans and curriculum systems in response to the digital and intelligent trends emerging in maritime development, exploring and developing interdisciplinary and cross-professional curriculum systems of “Intelligent + Navigation,” and utilizing AI technology to create virtual training scenarios to enhance students’ overall quality.
Finally, regarding the issue of shipping companies’ lack of confidence in investing in ship emission reduction technologies, the economics community needs to consider how to formulate appropriate investment subsidies or incentive mechanisms to stimulate the investment enthusiasm of shipping companies (Li et al., 2024; Liu M. et al., 2025; Qi et al., 2022). The reasons hindering corporate investment are the high risks, low return on investment, and long return periods. The government could consider providing certain tax incentives to shipping companies that invest in ship emission reduction technologies to alleviate the tax burden on enterprises, or offer projects with lower interest loans based on the scale of investment. By providing green credit, green bonds, and other financial instruments, the government can offer shipping companies more diversified financing channels, reduce financing costs, and enhance corporate investment enthusiasm (Metzger and Schinas, 2019). Green credit and bonds can provide shipping companies with funding for ship energy efficiency retrofitting, with broad application prospects: In June 2022, the International Finance Corporation (IFC) of the World Bank Group reached a cooperation agreement with Qingdao Bank in China to provide a $150 million blue syndicated loan specifically for marine-friendly projects. This loan has a lower interest rate, which can effectively promote the low-carbon retrofitting process of ships. In addition, large shipping companies can issue bonds linked to sustainable development, using corporate carbon emission intensity as a reference standard for the bond interest rate increase, which not only addresses funding issues but also provides impetus for ship energy efficiency retrofitting.
Table 11 shows Prioritization of Ship Emission Reduction Technologies.
This review examines ship emission reduction technologies by analyzing 714 publications from 2000 to 2024 sourced from the SSCI and SCIE databases. The analysis shows a significant increase in publications since 2017, indicating growing research interest. The top three journals in this field are the Journal of Marine Science and Engineering, Ocean Engineering, and Journal of Cleaner Production.
China leads in publications (287), followed by South Korea (58) and the UK (44), with these three countries accounting for 54.48% of the total. China’s dominance is attributed to strong government policies, high research funding, and extensive international cooperation. European countries have a closer cooperation network compared to Asian countries. Key institutions like Shanghai Maritime University and Wuhan University of Technology have made significant contributions, highlighting the importance of collaboration.
Shuaian Wang from Hong Kong Polytechnic University is the top author, reflecting the need for broader cross-team collaborations to address future challenges. Keyword analysis reveals current research hotspots, emphasizing the importance of continued global cooperation to tackle climate challenges.
Reviewing the research and challenges faced in the field of ship emission reduction technologies, this paper proposes the following policy recommendations: First, in line with the emission reduction targets set by the IMO, governments should formulate national-level emission reduction plans and timelines tailored to their specific circumstances, providing guidance for the research and application of ship emission reduction technologies. Second, governments should plan the clean fuel refueling network in advance, particularly by establishing refueling stations at major ports to ensure the normal supply of clean fuels, alleviating concerns for ships using clean fuels. Finally, the government should fully play a leading role by promoting technology sharing through international platforms such as the IMO, advocating for win-win cooperation, and uniting global efforts to jointly address the challenges of global shipping emission reduction.
This review acknowledges its limitations. The literature is sourced solely from the SSCI and SCIE databases, potentially omitting important works. The selected keywords, while covering mainstream technologies, might miss some cutting-edge research. Additionally, bibliometric methods provide a macro view through quantitative analysis, which may not deeply explore the working principles and impacts of specific technologies or their synergies. To address these limitations, future research could expand data retrieval sources and keyword selection, conduct more targeted studies, and integrate different research methods to offer a more comprehensive analysis and scientific guidance for the development of shipping emission reduction technologies.
JB: Writing – original draft, Writing – review & editing. YY: Writing – original draft, Writing – review & editing. XB: Writing – original draft, Writing – review & editing.
The author(s) declare that financial support was received for the research and/or publication of this article. This research was funded by National Natural Science Foundation of China, grant number 52472323, 72404022, Science and Technology Planning Projects of Yunnan Provincial Science and Technology Agency grant 202101BA070001-236.
The authors declare that the research was conducted in the absence of any commercial or financial relationships that could be construed as a potential conflict of interest.
The author(s) declare that no Generative AI was used in the creation of this manuscript.
All claims expressed in this article are solely those of the authors and do not necessarily represent those of their affiliated organizations, or those of the publisher, the editors and the reviewers. Any product that may be evaluated in this article, or claim that may be made by its manufacturer, is not guaranteed or endorsed by the publisher.
Ammar N. R., Seddiek I. S. (2023). Hybrid/dual fuel propulsion systems towards decarbonization: case study container ship. Ocean Eng. 281, 114962. doi: 10.1016/j.oceaneng.2023.114962
Atilhan S., Park S., El-Halwagi M. M., Atilhan M., Moore M., Nielsen R. B. (2021). Green hydrogen as an alternative fuel for the shipping industry. Curr. Opin. Chem. Eng. 31, 100668. doi: 10.1016/j.coche.2020.100668
Bakar N. N. A., Bazmohammadi N., Vasquez J. C., Guerrero J. M. (2023). Electrification of onshore power systems in maritime transportation towards decarbonization of ports: a review of the cold ironing technology. Renewable Sustain. Energy Rev. 178, 113243. doi: 10.1016/j.rser.2023.113243
Baldasso E., Gilormini T. J. A., Mondejar M. E., Andreasen J. G., Larsen L. K., Fan J. H., et al. (2020). Organic rankine cycle-based waste heat recovery system combined with thermal energy storage for emission-free power generation on ships during harbor stays. J. Cleaner Production 271, 122394. doi: 10.1016/j.jclepro.2020.122394
Baldi F., Moret S., Tammi K., Maréchal F. (2020). The role of solid oxide fuel cells in future ship energy systems. Energy 194, 116811. doi: 10.1016/j.energy.2019.116811
Bashan V., Kokkulunk G. (2020). Exergoeconomic and air emission analyses for marine refrigeration with waste heat recovery system: a case study. J. Mar. Eng. Technol. 19, 147–160. doi: 10.1080/20464177.2019.1656324
Brown T., Hampp J. (2023). Ultra-long-duration energy storage anywhere: methanol with carbon cycling. Joule 7, 2414–2420. doi: 10.1016/j.joule.2023.10.001
Chen A. W., Chen W. Q., Zheng J. (2023). Arctic route planning and navigation strategy: the perspective of ship fuel costs and carbon emissions. J. Mar. Sci. Eng. 11, 1308. doi: 10.3390/jmse11071308
Cheng P., Pan T., Li R. Y., Liang N. (2022). Research on optimal matching of renewable energy power generation system and ship power system. Iet Renewable Power Generation 16, 1649–1660. doi: 10.1049/rpg2.12470
Deng J. J., Wang X. C., Wei Z. L., Wang L., Wang C. Y., Chen Z. B. (2021). A review of NOx and SOx emission reduction technologies for marine diesel engines and the potential evaluation of liquefied natural gas fuelled vessels. Sci. Total Environ. 766, 144319. doi: 10.1016/j.scitotenv.2020.144319
Elkafas A. G., Seddiek I. S. (2024). Application of renewable energy systems in seaports towards sustainability and decarbonization: energy, environmental and economic assessment. Renewable Energy 228, 120690. doi: 10.1016/j.renene.2024.120690
Endres S., Maes F., Hopkins F., Houghton K., Mårtensson E. M., Oeffner J., et al. (2018). A new perspective at the ship-air-sea-interface: the environmental impacts of exhaust gas scrubber discharge. Front. Mar. Sci. 5. doi: 10.3389/fmars.2018.00139
Fan A. L., Li Y. P., Liu H. Y., Yang L., Tian Z. Q., Li Y. R., et al. (2023). Development trend and hotspot analysis of ship energy management. J. Cleaner Production 389, 135899. doi: 10.1016/j.jclepro.2023.135899
Fan A. L., Yang J., Yang L., Wu D., Vladimir N. (2022). A review of ship fuel consumption models. Ocean Eng. 264, 112405. doi: 10.1016/j.oceaneng.2022.112405
Feng Y. M., Du Z. Q., Shreka M., Zhu Y. Q., Zhou S., Zhang W. P. (2020). Thermodynamic analysis and performance optimization of the supercritical carbon dioxide brayton cycle combined with the kalina cycle for waste heat recovery from a marine low-speed diesel engine. Energy Conversion Manage. 206, 112483. doi: 10.1016/j.enconman.2020.112483
Gabbar H. A., Adham M. I., Abdussami M. R. (2021). Analysis of nuclear-renewable hybrid energy system for marine ships. Energy Rep. 7, 2398–2417. doi: 10.1016/j.egyr.2021.04.030
Gore K., Rigot-Mueller P., Coughlan J. (2022). Cost assessment of alternative fuels for maritime transportation in Ireland. Transportation Res. Part D:Transport Environ. 110, 103416. doi: 10.1016/j.trd.2022.103416
Guo Y. H., Wang Y. Y., Chen Y. H., Wu L. X., Mao W. G. (2024b). Learning-based pareto-optimum routing of ships incorporating uncertain meteorological and oceanographic forecasts. Transportation Res. Part E:Logistics Transportation Rev. 192, 103786. doi: 10.1016/j.tre.2024.103786
Guo H., Wang Z. C., Zhou S., Zhang M., Shreka M. (2024a). The influence of pre-chamber parameters on the performance of a two-stroke marine dual-fuel low-speed engine. J. Mar. Sci. Eng. 12, 1232. doi: 10.3390/jmse12071232
Guo H., Zhou S., Zou J. X., Shreka M. (2020). A numerical study on the pilot injection conditions of a marine 2-stroke lean-burn dual fuel engine. Processes 8, 1396. doi: 10.3390/pr8111396
Hao G. Z., Xiao W. H., Huang L. W., Chen J. H., Zhang K., Chen Y. J. (2024). The analysis of intelligent functions required for inland ships. J. Mar. Sci. Eng. 12, 836. doi: 10.3390/jmse12050836
Jiang K. J., Yang L., Fang Z. C., Ma N., Li X. L., Huang Z. (2024). Navigating towards a zero-carbon world: research progress of chemical solvent-based shipboard carbon capture system integration and renewable energy coupling. Ocean Eng. 309, 118585. doi: 10.1016/j.oceaneng.2024.118585
Lee C.-Y. (2022). A study on the development trends of polymer electrolyte membrane fuel cells and application to ships. J. Korean Soc. Mar. Environ. Saf. 28, 657–666. doi: 10.7837/kosomes.2022.28.4.657
Li Z., Wang L., Wang G., Xin X., Chen K., Zhang T. (2024). Investment and subsidy strategy for low-carbon port operation with blockchain adoption. Ocean Coast. Manage. 248, 106966. doi: 10.1016/j.ocecoaman.2023.106966
Lion S., Vlaskos I., Taccani R. (2020). A review of emissions reduction technologies for low and medium speed marine Diesel engines and their potential for waste heat recovery. Energy Conversion Manage. 207, 112553. doi: 10.1016/j.enconman.2020.112553
Liu H., Wu C., Li B., Zong Z., Shu Y. (2025). Research on ship anomaly detection algorithm based on transformer-GSA encoder. doi: 10.1109/TITS.2025.3536483. 15580016.
Liu M., Xin X., Wang X., Zhang T., Chen K. (2025). Dual-channel slot sales strategy for container liner shipping companies with blockchain technology adoption. Transport Policy 162, 200–220. doi: 10.1016/j.tranpol.2024.12.006
Long N. V. D., Lee D. Y., Kim M. J., Kwag C., Lee Y. M., Kang K. J., et al. (2021). Desulfurization scrubbing in a squared spray column for a 720 kW marine diesel engine: design, construction, simulation, and experiment. Chem. Eng. Processing-Process Intensification 161, 108317. doi: 10.1016/j.cep.2021.108317
Lu B. W., Shi L. F., Tian H., Wang X., Shu G. Q. (2022). CO2-mixture-based ship waste heat recovery system (SWHRS) with multiple energy outputs and composition-adjustable mixture. Energy Conversion Manage. 251, 114994. doi: 10.1016/j.enconman.2021.114994
Luo J. A., Geng X. F., Li Y. B., Yu Q. C. (2022). Study on the risk model of the intelligent ship navigation. Wireless Commun. Mobile Computing 2022, 3437255. doi: 10.1155/2022/3437255
Luo X., Yan R., Wang S. A. (2024a). Ship sailing speed optimization considering dynamic meteorological conditions. Transportation Res. Part C:Emerging Technol. 167, 104827. doi: 10.1016/j.trc.2024.104827
Luo X., Yan R., Xu L., Wang S. A. (2024b). Accuracy and applicability of ship’s fuel consumption prediction models: a comprehensive comparative analysis. Energy 310, 133187. doi: 10.1016/j.energy.2024.133187
Luo X., Zhang M. Y., Han Y., Yan R., Wang S. N. (2025). Ship fuel consumption prediction based on transfer learning: models and applications. Eng. Appl. Artif. Intell. 141, 109769. doi: 10.1016/j.engappai.2024.109769
Ma W. H., Zhang J. F., Han Y. Y., Mao T. Y., Ma D. F., Zhou B., et al. (2023). A decision-making optimization model for ship energy system integrating emission reduction regulations and scheduling strategies. J. Ind. Inf. Integration 35, 100506. doi: 10.1016/j.jii.2023.100506
Metzger D., Schinas O. (2019). Fuzzy real options and shared savings: investment appraisal for green shipping technologies. Transportation Res. Part D:Transport Environ. 77, 1–10. doi: 10.1016/j.trd.2019.09.016
Moshiul A. M., Mohammad R., Hira F. A. (2023). Alternative fuel selection framework toward decarbonizing maritime deep-sea shipping. Sustainability 15, 5571. doi: 10.3390/su15065571
Mukherjee D., Lim W. M., Kumar S., Donthu N. (2022). Guidelines for advancing theory and practice through bibliometric research. J. Business Res. 148, 101–115. doi: 10.1016/j.jbusres.2022.04.042
Nguyen D. H., Lin C., Cheruiyot N. K., Hsu J. Y., Cho M. Y., Hsu S. H., et al. (2021). Reduction of NOx and SO2 emissions by shore power adoption. Aerosol Air Qual. Res. 21, 200100. doi: 10.4209/aaqr.210100
Nguyen V., Rudzki K., Dzida M., Pham N. D. K., Pham M. T., Nguyen P. Q. P., et al. (2023). Understanding fuel saving and clean fuel strategies towards green maritime. Polish Maritime Res. 30, 146–164. doi: 10.2478/pomr-2023-0030
Nuchturee C., Li T., Xia H. P. (2020). Energy efficiency of integrated electric propulsion for ships - a review. Renewable Sustain. Energy Rev. 134, 110145. doi: 10.1016/j.rser.2020.110145
Oh J., Kim D., Roussanaly S., Anantharaman R., Lim Y. (2024a). Optimal capacity design of amine-based onboard CO2 capture systems under variable marine engine loads. Chem. Eng. J. 483, 149136. doi: 10.1016/j.cej.2024.149136
Oh J., Kim D., Roussanaly S., Lim Y. (2024b). Greenhouse gas emissions of shipping with onboard carbon capture under the FuelEU Maritime regulation: A well-to-wake evaluation of different propulsion scenarios. Chem. Eng. J. 498, 155407. doi: 10.1016/j.cej.2024.155407
Pan P. C., Sun Y. W., Yuan C. Q., Yan X. P., Tang X. J. (2021). Research progress on ship power systems integrated with new energy sources: a review. Renewable Sustain. Energy Rev. 144, 111048. doi: 10.1016/j.rser.2021.111048
Parris D., Spinthiropoulos K., Ragazou K., Giovou A., Tsanaktsidis C. (2024). Methanol, a plugin marine fuel for green house gas reduction-a review. Energies 17, 605. doi: 10.3390/en17030605
Peddi K. P., Ricci S., Rizzetto L. (2024). Reduction potential of gaseous emissions in european ports using cold ironing. Appl. Sciences-Basel 14, 6837. doi: 10.3390/app14156837
Peng P., Xie X. W., Claramunt C., Lu F., Gong F. Z., Yan R. (2025). Bibliometric analysis of maritime cybersecurity: research status, focus, and perspectives. Transportation Res. Part E:Logistics Transportation Rev. 195, 103971. doi: 10.1016/j.tre.2025.103971
Percic M., Frkovic L., Puksec T., Cosic B., Li O. L., Vladimir N. (2022). Life-cycle assessment and life-cycle cost assessment of power batteries for all-electric vessels for short-sea navigation. Energy, 251, 13. doi: 10.1016/j.energy.2022.123895
Qi J. W., Feng R. J., Wang S. A. (2023). Optimization of fleet scrubber installation and utilization considering sulfur emission control areas and marine fuel switching. J. Mar. Sci. Eng. 11, 1849. doi: 10.3390/jmse11101849
Qi J. W., Wang H., Zheng J. F. (2022). Promoting liquefied natural gas (LNG) bunkering for maritime transportation: should ports or ships be subsidized? Sustainability 14, 6647. doi: 10.3390/su14116647
Radonja R., Bebic D., Glujic D. (2019). Methanol and ethanol as alternative fuels for shipping. Promet-Traffic Transportation 31, 321–327. doi: 10.7307/ptt.v31i3.3006
Risso R., Cardona L., Archetti M., Lossani F., Bosio B., Bove D. (2023). A review of on-board carbon capture and storage techniques: solutions to the 2030 IMO regulations. Energies 16, 6748. doi: 10.3390/en16186748
Rolán A., Manteca P., Oktar R., Siano P. (2019). Integration of cold ironing and renewable sources in the barcelona smart port. IEEE Trans. Industry Appl. 55, 7198–7206. doi: 10.1109/tia.2019.2910781
Shi J., Zhu Y. Q., Feng Y. M., Yang J., Xia C. (2023). A prompt decarbonization pathway for shipping: green hydrogen, ammonia, and methanol production and utilization in marine engines. Atmosphere 14, 584. doi: 10.3390/atmos14030584
Shu Y., Han B., Song L., Yan T., Gan L., Zhu Y., et al. (2024). Analyzing the spatio-temporal correlation between tide and shipping behavior at estuarine port for energy-saving purposes. Appl. Energy 367, 123382. doi: 10.1016/j.apenergy.2024.123382
Shu Y., Hu A., Zheng Y., Gan L., Xiao G., Zhou C., et al. (2023). Evaluation of ship emission intensity and the inaccuracy of exhaust emission estimation model. Ocean Eng. 287, 115723. doi: 10.1016/j.oceaneng.2023.115723
Stec M., Tatarczuk A., Iluk T., Szul M. (2021). Reducing the energy efficiency design index for ships through a post-combustion carbon capture process. Int. J. Greenhouse Gas Control 108, 103333. doi: 10.1016/j.ijggc.2021.103333
Sun L., Ding P. T., Xiong Y. X., Liu W., Hu Z. J. (2022). Carbon emission reduction of shore power from power energy structure in China. Front. Mar. Sci. 9. doi: 10.3389/fmars.2022.1077289
Tan Z. J., Zeng X. Y., Wang T. S., Wang Y. D., Chen J. H. (2023). Capacity investment of shore power berths for a container port: environmental incentive and infrastructure subsidy policies. Ocean Coast. Manage. 239, 106582. doi: 10.1016/j.ocecoaman.2023.106582
Torres-Salinas D., Valderrama-Baca P., Arroyo-MaChado W. (2022). Is there a need for a new journal metric? correlations between JCR impact factor metrics and the journal citation indicator -JCI. J. Informetrics 16, 101315. doi: 10.1016/j.joi.2022.101315
Wang Y., Cao Q., Liu L., Wu Y., Liu H. Y., Gu Z. Y., et al. (2022). A review of low and zero carbon fuel technologies: achieving ship carbon reduction targets. Sustain. Energy Technol. Assessments 54, 102762. doi: 10.1016/j.seta.2022.102762
Wang J. G., Li H. H., Yang Z. L., Ge Y. E. (2024). Shore power for reduction of shipping emission in port: a bibliometric analysis. Transportation Res. Part E: Logistics Transportation Rev. 188, 103639. doi: 10.1016/j.tre.2024.103639
Wang H. Q., Yan R., Wang S. A., Zhen L. (2023). Innovative approaches to addressing the tradeoff between interpretability and accuracy in ship fuel consumption prediction. Transportation Res. Part C:Emerging Technol. 157, 104361. doi: 10.1016/j.trc.2023.104361
Wohlthan M., Thaler B., Helf A., Keller F., Kaub V., Span R., et al. (2024). Oxyfuel combustion based carbon capture onboard ships. Int. J. Greenhouse Gas Control 137, 104234. doi: 10.1016/j.ijggc.2024.104234
Xiang Z., Xin X., Zhang T., Chen K., Liu M. (2025). Asia−Europe liner shipping network design model considering arctic route and black carbon tax. Ocean Coast. Manage. 261, 107492. doi: 10.1016/j.ocecoaman.2024.107492
Xiao G. N., Pan L., Lai F. B. (2025). Application, opportunities, and challenges of digital technologies in the decarbonizing shipping industry: a bibliometric analysis. Front. Mar. Science., 1523267. doi: 10.3389/fmars.2025.1523267
Xiao G. N., Wang Y. Q., Wu R. J., Li J. P., Cai Z. Y. (2024). Sustainable maritime transport: a review of intelligent shipping technology and green port construction applications. J. Mar. Sci. Eng. 12, 1728. doi: 10.3390/jmse12101728
Xin X., Zhang T., Wang X., He F., Wu L. (2025). Risk-averse distributionally robust optimization for construction waste reverse logistics with a joint chance constraint. Comput. Operations Res. 173, 106829. doi: 10.1016/j.cor.2024.106829
Xue Y. M., Lai K. H. (2024). Digital green shipping innovation: conception, adoption, and challenges. J. Global Inf. Manage. 32, 349929. doi: 10.4018/jgim.349929
Xue Y. M., Lai K. H., Wang C. Y. (2024). How to invest decarbonization technology in shipping operations? evidence from a game-theoretic investigation. Ocean Coast. Manage. 251, 107076. doi: 10.1016/j.ocecoaman.2024.107076
Yan R., Yang D., Wang T. Y., Mo H. Y., Wang S. A. (2024). Improving ship energy efficiency: models, methods, and applications. Appl. Energy 368, 123132. doi: 10.1016/j.apenergy.2024.123132
Yang W. M., Chen X. D., Liu Y. (2023). Review and reflections of legislation and policies on shipping decarbonization under China’s “dual carbon” target. Front. Mar. Sci. 10. doi: 10.3389/fmars.2023.1131552
Zhang L. H., Peng X. Y., Liu Z. F., Wei N. X., Wang F. (2022). An application of augmented lagrangian differential evolution algorithm for optimizing the speed of inland ships sailing on the yangtze river. Int. J. Naval Architecture Ocean Eng. 14, 100488. doi: 10.1016/j.ijnaoe.2022.100488
Zheng J. Q., Zhang H. R., Yin L., Liang Y. T., Wang B. H., Li Z. B., et al. (2019). A voyage with minimal fuel consumption for cruise ships. J. Cleaner Production 215, 144–153. doi: 10.1016/j.jclepro.2019.01.032
Zhong H. L., Guo C. H., Yip T. L., Gu Y. M. (2021). Bi-perspective sulfur abatement options to mitigate coastal shipping ships emissions: a case study of chinese coastal zone. Ocean Coast. Manage. 209, 105658. doi: 10.1016/j.ocecoaman.2021.105658
Zhou J. X., Zhou S., Wang Z. G. (2023). Emission characteristics of particulate matter emitted from 4-and 2-stroke marine diesel engines under the background of sulfur emission reduction. Environ. Sci. pollut. Res. 30, 33660–33673. doi: 10.1007/s11356-022-24594-1
Keywords: bibliometric analysis, ship emission reduction technology, sustainability, maritime transport, green shipping technologies
Citation: Bai J, Yan Y and Bai X (2025) A comprehensive review of ship emission reduction technologies for sustainable maritime transport. Front. Mar. Sci. 12:1576661. doi: 10.3389/fmars.2025.1576661
Received: 14 February 2025; Accepted: 05 March 2025;
Published: 24 March 2025.
Edited by:
Xu Xin, Tongji University, ChinaReviewed by:
Ran Yan, Nanyang Technological University, SingaporeCopyright © 2025 Bai, Yan and Bai. This is an open-access article distributed under the terms of the Creative Commons Attribution License (CC BY). The use, distribution or reproduction in other forums is permitted, provided the original author(s) and the copyright owner(s) are credited and that the original publication in this journal is cited, in accordance with accepted academic practice. No use, distribution or reproduction is permitted which does not comply with these terms.
*Correspondence: Yutong Yan, eWFueXV0b25nQGd6bXR1LmVkdS5jbg==
Disclaimer: All claims expressed in this article are solely those of the authors and do not necessarily represent those of their affiliated organizations, or those of the publisher, the editors and the reviewers. Any product that may be evaluated in this article or claim that may be made by its manufacturer is not guaranteed or endorsed by the publisher.
Research integrity at Frontiers
Learn more about the work of our research integrity team to safeguard the quality of each article we publish.