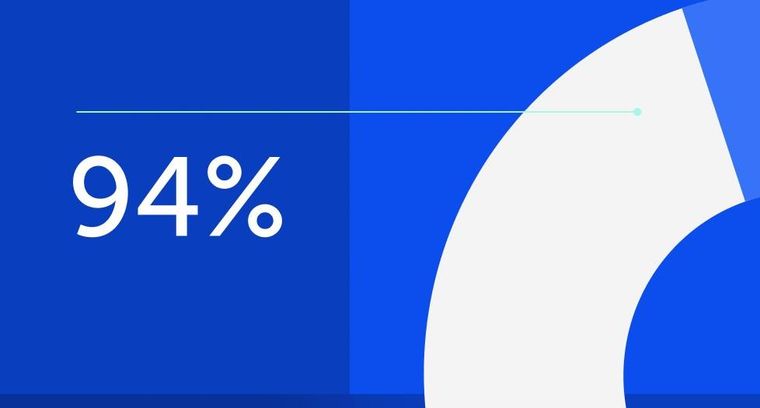
94% of researchers rate our articles as excellent or good
Learn more about the work of our research integrity team to safeguard the quality of each article we publish.
Find out more
ORIGINAL RESEARCH article
Front. Mar. Sci., 08 April 2025
Sec. Marine Ecosystem Ecology
Volume 12 - 2025 | https://doi.org/10.3389/fmars.2025.1563252
Introduction: This study focuses on the benthic macroalgae of the Nanji Islands, China, aiming to assess species diversity and biomass through a combination of qualitative and quantitative approaches using historical data.
Methods: We conducted a comprehensive survey of the benthic macroalgae, employing species identification and biomass estimation techniques across different seasons and tidal zones. Co-occurrence network analysis was used to explore species interactions and ecosystem stability. Historical data were compared to recent monitoring results to evaluate the impacts of marine reserves.
Results: A total of 127 species were identified, with Rhodophyta being the dominant group (57.48%), exhibiting remarkable ecological adaptability. Seasonal variation in biomass showed peaks in spring (1344.31 g·m⁻²) and the lowest in autumn (428.78 g·m⁻²). The mid/low-tide zones supported 2.6 times higher biomass than the high-tide zones. The southern communities demonstrated increased stability, with a co-occurrence network of 129 nodes and 999 edges. Biomass recovery of 61.14% was observed at key monitoring sites since the establishment of protected areas in 1990.
Discussion: Our findings emphasize the crucial role of marine reserves in reversing biodiversity loss and maintaining ecosystem health. The observed biomass recovery and species diversity restoration in protected areas underscore the effectiveness of these conservation strategies. The study also provides valuable insights into the distribution patterns of benthic macroalgae, highlighting the significance of seasonal and tidal zone influences on biodiversity and biomass.
The island ecosystem is one of the most important on Earth (Chi et al., 2017), and macrobenthic algae are a crucial component. These algae typically grow on rocks and coral reefs in the intertidal or subtidal zones (Ford et al., 1999), but they also occur on aquaculture equipment such as raft systems, cages, and ropes (He et al., 2021). The distribution of benthic macroalgal communities on islands varies with the seasons, with differences in both the horizontal and vertical distributions. Algae species typically show variation in dominance among ecological environments (Yang et al., 2017).
The Nanji Islands, situated at the southern end of the East China Sea, consist of 52 islands, each with an area greater than 500 m2 (Li et al., 2017; Cai, 2021). This region serves as a convergence zone for the Zhejiang Coastal Current and the Taiwan Warm Current. The complex topography, characterized by numerous islands and capes, induces a strong mixing of surface and deep waters, facilitating upwellings that contribute to exceptional water quality. This creates a nutrient-rich environment, providing ideal conditions for the growth and reproduction of marine life and supporting a high level of marine biodiversity (Li et al., 2017). The unique and diverse ecological features of the Nanji Islands have earned them the title “Kingdom of Shells and Algae,” and they are recognized as a globally important wetland area with typical and representative characteristics of island ecosystems (Cai, 2021); however, over-exploitation of local resources has led to ecological degradation. In response, the Nanji Islands National Nature Reserve was established in December 1990 as one of the first five national reserves approved by the State Council of China. This reserve is dedicated to the protection of marine biodiversity. In December 1998, it was designated by United Nations Educational, Scientific and Cultural Organization (UNESCO) as a member of the International Man and Biosphere Reserve Network (Cai, 2021). Human activities are strictly restricted in the core area. In the buffer zone, limited scientific research and ecological tourism are permitted.
The seaweed resources of the Nanji Islands attracted the attention of researchers as early as 1936 (Yang et al., 2017). Monitoring the trends in algal resources and changes in the natural environment has long been a focus of reserve management (Chen et al., 1994). To date, a total of 186 algal species have been recorded in the region, accounting for 18% of the total algal species in China (Sun et al., 2010). Sun et al. (2010) conducted a study in 2010 at Mazuao on Nanji Island, reviewing changes in the diversity of benthic macroalgae from 1960 to 2007. Over this 40-year period, only 87 species were recorded, marking a 41.7% decrease in benthic macroalgal species, along with rapid declines in both biodiversity and biomass. The community structure had changed significantly. The study also observed that the proportions of subtropical and warm-temperate species had increased, while the proportion of cold-temperate species had decreased. Additionally, several surveys were conducted on Nanji Island between 2008 and 2009, 2012 and 2013, and 2016 and 2017. These studies observed a significant decline in benthic macroalgal diversity compared to the findings of Zhang et al (Wu, 2019; Fu et al., 2011). The causes of this decline in biodiversity and the shift in community structure have been widely debated, with a consensus that climate change—in particular rising water temperatures and increased CO2 concentrations—is the primary driving factor, contributing to a trend of desertification in the intertidal zones of the Nanji Islands (Peng et al., 2009).
The distribution of seaweeds is strongly influenced by seasonal variation and tidal zones, as factors such as temperature, salinity, light, and drought tolerance are key determinants of biological activity (Li et al., 2019). Bi et al. (2013) investigated the distribution and variation of Sargassum on Gouqi Island, revealing differences in both the density and depth distribution of Sargassum across seasons. Similarly, Sun et al. (2010) conducted a long-term survey from 2000 to 2007, demonstrating that algae in the intertidal zone exhibit seasonal alternation patterns and complex, distinct zonal distributions, driven by fluctuations in temperature, tidal dynamics, and wind-induced waves.
Since being designated as a nature reserve in 1990, the Nanji Islands have benefited from various conservation efforts supported by the Chinese government (Peng et al., 2009). Despite these initiatives, previous studies have outlined the region’s algal diversity patterns; however, significant knowledge gaps remain, particularly regarding temporal dynamics. Notably, there is a lack of data on decadal-scale shifts in species composition, biomass distribution, and community structural stability. A comprehensive evaluation of changes in species diversity and biomass over time is thus needed. The research question of this study is to investigate the current status of benthic macroalgae in the Nanji Islands and compare it with historical data to understand the trends and factors influencing their distribution and abundance. To achieve this, an extensive survey was conducted across the islands, examining the community structure and diversity changes from multiple perspectives—including species composition, biomass distribution, and seasonal variations in dominant species, both horizontally and vertically. This research not only lays a scientific foundation for biodiversity recovery and sustainable management of the reserve through strategies such as core-area tourism restrictions, buffer zone optimization, and calcareous algae-monitored restoration, but also provides critical insights into how the regional marine ecosystem responds to the combined pressures of global change and intensified human activity.
The Nanji Island Nature Reserve is situated in the southeastern region of Zhejiang Province, China (centered at 27°27′N, 121°05′E). It features a subtropical monsoon climate and is affected by the confluence of the Taiwan Warm Current and the Zhejiang Coastal Current. The reserve is divided into core zones, buffer zones, and experimental zones. In this study, 33 sampling sites were established within the reserve areas (Supplementary Table S1; Figure 1) covering key areas of the main island (M), the northern (N) and southern (S) sides of the island, as well as three additional sites on aquaculture rafts (Rft). Site selection was based on preliminary field reconnaissance to ensure representative coverage of the Nanji Islands’ diverse habitats, including wave-exposed and sheltered shores, as well as natural and aquaculture-influenced areas. The number of sites in each zone varied due to logistical constraints, such as accessibility and the spatial heterogeneity of the islands. For example, the high-tide zone, occupying a relatively smaller spatial extent compared to the mid- and low-tide zones, inherently limited the number of feasible sampling points. Additionally, some small reefs could only accommodate a single sampling point and were therefore excluded. Constantly shifting aquaculture facilities were also not included to ensure consistency in sampling locations.
The surveys were conducted during four periods, namely, from May 6 to 22, 2022 (spring), from August 10 to 18, 2022 (summer), from November 18 to 23, 2022 (autumn), and from February 17 to 23, 2023 (winter). A regular intertidal zone survey was carried out at the Nanji Island Marine Nature Reserve. The intertidal zone was divided into high, middle, and low tidal zones. Multiple survey points (at least 7) were established within each tidal zone. At each point, samples were collected from two 25 cm × 25 cm quadrats. All of the seaweeds within the quadrats were scraped off, washed, and then dried with absorbent paper before being brought back to the laboratory for weighing and species identification. Taxonomic identification is based on AlgaeBase (https://www.algaebase.org). Density is indirectly reflected by the biomass per unit area (g/m²). The entire survey was conducted following the Marine Survey Specifications (First Institute of Oceanography et al., 2007a, b).
Alpha biodiversity was quantified using the Shannon-Wiener diversity index (H’) (Wang et al., 2015). The classification of community structure based on H’ was as follows: H’ > 3 indicated a well-structured community; 3 > H’ > 2 indicated a relatively well-structured community; 2 > H’ > 1 indicated an average community structure, and H’< 1 indicated a poorly structured community (Borja and Muxika, 2005). The formula for the diversity index H is
Relative diversity was assessed using the Simpson index (D) (Wei, 2015):
Species richness was analyzed using the Margalef index (H) (Shi, 2019):
Species evenness was assessed using the Pielou index (J’) (Sun, 2021):
Dominant species were analyzed using McNaughton’s dominance formula (Liao, 2021), with dominant species defined as those with Y ≥ 0.02 (An et al., 2008):
where S is the total number of species; Pi is the proportion of species i’s biomass relative to the total biomass at the sampling site; Ni is the total biomass of species i; N is the total biomass of all of the species; and is the frequency of species i, defined as the percentage of sites where the species appears compared to the total number of sites.
The Sorenson similarity index Cs was used to compare the similarity between different sampling sites’ communities (The two 25 cm × 25 cm quadrats at each site were combined into one community sample to avoid small-scale heterogeneity.):
where j is the number of species common to both communities or sample sites; a and b are the total numbers of species in each of the two sample sites.
Algal species were categorized into five morphogroups based on the description of macroalgae morphological traits in WORMS (www.marinespecies.org): canopy algae, filamentous algae, sheet-like algae, coarse-branched algae, and calcareous algae in order to analyze the species interactions and their impact on the stability of community structure. These groups were then used in co-occurrence network analysis for their interactions and contribution to the stability of the community.
A co-occurrence network analysis was performed based on Spearman’s correlation to investigate the relationships among multiple species within each region. focusing on robust associations (|r| > 0.6) with statistical significance (FDR-adjusted P< 0.05) to reduce false positives. P values were corrected using the Benjamini-Hochberg method (Benjamini and Hochberg, 1995). In the network, nodes represent species, edges signify significant correlations, and edge weights indicate the strength of these correlations. The network was analyzed for modularity (with values > 0.4 indicating strong subgroup clustering) and betweenness centrality (to identify keystone species bridging subgroups). Visualization was done using Gephi’s ForceAtlas2 algorithm (Barberán et al., 2014), where node size reflects connectivity (degree). Symbiotic relationships were defined as significant positive correlations between species, with valid co-occurrence events marked by an absolute Spearman’s correlation coefficient (|r|) greater than 0.6 and FDR-adjusted P values< 0.05. All correlation analyses were performed in R v. 4.0.3 with the vegan and igraph packages (Csardi and Nepusz, 2006).
All statistical tests using one-way ANOVA test were conducted by using SPSS version 19.0 (SPSS, Inc., Chicago, IL, USA), while graphs were generated using Origin 2021 (OriginLab Corporation, Northampton, MA, USA) and GraphPad Prism 8 (GraphPad Software, San Diego, CA, USA). The statistical significance was set at P< 0.05.
As of 2023, a total of 186 species of benthic macroalgae had been reported in the Nanji Islands (Cai, 2021). In this study, extensive surveys conducted in 2022–2023 across the Nanji Islands identified 127 species of large seaweeds belonging to 63 genera. The diversity of Rhodophyta was the highest, comprising 34 genera and 73 species, accounting for 57.48% of the total species identified. This dominance contrasts with findings from the Zhongjieshan Islands, where Chlorophyta predominated (Qiu et al., 2011), likely reflecting the unique hydrological conditions of the Nanji Islands. Chlorophyta followed with seven genera and 27 species, 21.26% of the total. Phaeophyceae included 12 genera and 25 species, representing 19.69% of the total, while cyanobacteria were the least diverse, with only two genera and two species, accounting for 1.57% of the total (Supplementary Table S2).
Among the regions surveyed, the northern side (N) of the Nanji Islands had the highest species richness, with 127 species of algae. The southern islands (S) followed with 91 species, while the main island (M) had only 62 species, and the aquaculture rafts (Rft) had the fewest, with 58 species. Among the sampling sites, N_dts1 had the highest total number of algae species, with 78 species, followed by S_jy2 with 75 species, while M_hl and Rft_zy had the fewest, with only 21 species.
Most of the islands on the northern and southern sides are located within the core area of the nature reserve, where human activity is minimal, leading to the highest species richness. The main island situated largely within the buffer zone has many areas open to tourism; this has impacted the growth of algae, resulting in a decrease in diversity. In contrast, the aquaculture rafts represent a non-natural environment influenced by the water quality and conditions of the aquaculture facilities. This area was noticeably different from other regions, with significantly fewer species. The rafts primarily cultivate mussels and scallops, and algae are often attached to the rafts. These algae do not require desiccation and tend to be species that grow rapidly such as Gracilaria and Sargassum.
The dominant species of large benthic macroalgae in the Nanji Islands included Gloiopeltis furcata, Sargassum fusiforme, Sargassum thunbergii, Corallina officinalis, Scytosiphon lomentaria, Corallina pilulifera, Ishige okamurae, and Pyropia haitanensis, each with a dominance value (Y) greater than 0.02 at all of the sampling sites. Notably, S. thunbergii forms dense canopies that mitigate desiccation stress for understory species, while C. officinalis stabilizes substrates through calcification, enhancing habitat complexity (Nelson, 2009; Hofmann et al., 2012). These ecosystem engineers likely underpin the community’s resilience to environmental fluctuations. Similar to other long-term studies, these algae are typically widespread species found across most rocky shore habitats, forming the main components of the community structure.
In contrast, species with limited distribution such as Undaria pinnatifida, Gloiopeltis tenax, and Leathesia difformes were only found on the northern side of the islands. Wave impact is a primary ecological factor influencing the horizontal distribution of algae (Cai, 2021). Areas with strong waves tend to have a richer distribution of algae. For example, the M_mza site, located in a bay with mild wave action, had relatively fewer species, but the Chlorophyta grew particularly well; several species of Ulva were observed in sheltered bays. The M_lcj site, with steep shores and heavy waves, exhibited a diverse range of species, especially Rhodophyta.
In terms of overall biomass (Figure 2; Supplementary Table S3), the highest algal biomass in the Nanji Islands occurred in the aquaculture facilities, reaching 6191.20 g·m-2. This was primarily due to the abundant nutrients produced by aquaculture activities that promoted the rapid growth of several dominant species, leading to an accumulation of biomass. For natural areas, the average annual biomass at the northern sampling sites was 2806.83 g·m-2, while the main island had an average biomass of 2972.77 g·m-2. In contrast, the southern sites had an average biomass of 4984.22 g·m-2, significantly higher. This spatial disparity likely stems from the Taiwan Warm Current’s dual role in elevating water temperatures and delivering nutrient-rich upwelled waters (Pan et al., 1991; Li et al., 2024).
A comparison of the biomass of benthic macroalgae between the Nanji Islands and the Beiji Islands (Zhang et al., 2000), Zhongjieshan Islands (Qiu et al., 2011), and Yushan Islands (Ruan, 1994) revealed that the average annual biomass of benthic macroalgae in the Nanji Islands was 789.37 g·m-2, while in the Beiji Islands, this was 178.32 g·m-2; the Zhongjieshan Islands had an average annual biomass of 342.04 g·m-2, and in the Yushan Islands, the average was 315.50 g·m-2. Therefore, the Nanji Islands had the highest annual biomass compared to the other nearby islands. Zhu et al. (2011) investigated the species composition similarity of benthic macroalgae among these neighboring islands and found that the Nanji Islands algal community differed significantly from those on the other islands. This discrepancy may reflect the effectiveness of conservation measures in the Nanji Islands since 1990.
To explore the co-occurrence patterns of algae in different regions, co-occurrence networks of algal communities in each area were constructed (Figure 3). As Yang et al. (2024) pointed out, the edges and nodes within the network mirror the complexity of algal interactions. The co-occurrence network on the southern side of the Nanji Islands exhibited the greatest complexity, boasting 129 nodes and 999 edges. It also had a higher clustering coefficient, greater graph density, a shorter average path length, and a smaller network diameter compared to the other two regions. This clearly indicates that algal species in this area are intricately and closely connected. Through in-depth analysis, six key nodes acting as module hubs were identified. These nodes are associated with filamentous algae, sheet-like algae, and a small number of calcareous algae, suggesting strong positive correlations among these different algal morphogroups. For example, some filamentous algae might serve as an attachment substrate for sheet-like algae or cooperate in resource utilization, thus exemplifying a symbiotic relationship. In contrast, the network on the northern side of the Nanji Islands consisted of 86 nodes and 371 edges. Ten key nodes within the modular hubs were all part of the filamentous algal morphogroup. This finding highlights the crucial role of filamentous algae in the northern ecosystem and their close symbiotic associations with other species. It is likely that filamentous algae create a favorable microenvironment for other species or engage in collaborative interactions, such as in nutrient cycling. The algal network on the main island was the least complex, with 45 nodes and 91 edges. Fifteen key nodes were mainly distributed within the filamentous and sheet-like algal morphogroups. Despite having a lower network complexity compared to the southern side, a certain positive-correlation symbiotic relationship between filamentous and sheet-like algal functional groups was still discernible. Presumably, they possess some ecological niche complementarity, enabling them to adapt jointly to the main island’s ecological environment.
Figure 3. Co-occurrence networks of macrobenthic algal communities in the Nanji Islands. (A) The south side of the Nanji Islands. (B) The main island of the Nanji Islands. (C) The north side of the Nanji Islands. Each connection signifies a robust (Spearman’s |r| > 0.6) and statistically significant (FDR-adjusted P< 0.05) correlation. The size of each node is proportional to the number of species in each morphogroup. Shorter node path lengths and higher connection densities indicate stronger species associations within the network.
Overall, the results of the co-occurrence networks analysis suggest that symbiotic relationships prevail in the algal communities of the Nanji Islands. This is evidenced by the significant positive correlations (|r| > 0.6 and P< 0.05) observed in the network analyses of different regions. For example, on the southern side, multiple algal morphogroups form a complex network; on the main island, filamentous and sheet-like algae interact; and on the northern side, the network structure centered around filamentous algae as key nodes all point to the interdependence of different algal morphogroups. At the species level, although the relationships among filamentous algae and between filamentous algae and species of other morphogroups vary, an overall positive-correlation symbiotic trend is evident. For instance, in various regions, filamentous algae and other functional group algae together form key nodes, demonstrating their interaction and coexistence within the ecosystem. Notably, no clear evidence of competitive relationships was found in this analysis. Given the ecological environment and survey conditions of this study, it is plausible that competitive relationships are relatively unimportant, or no obvious signs of such relationships were detected.
Figueira et al. (2021) proposed that biodiversity enhances ecosystem stability mainly by promoting asynchronous species behavior, with species asynchrony being influenced by canopy algae and calcareous algae. For instance, calcareous algae play a significant role in the ecosystem. They form the substrate through the deposition of calcium carbonate and have an impact on seawater chemistry. Since seawater chemistry is vital for the survival of numerous organisms, calcareous algae thus contribute to the stabilization of the ecosystem (Nelson, 2009). The more complex occurrence network of algae on the southern side of the island, characterized by shorter paths, indicates a more stable community. This is likely related to the ecosystem’s ability to respond rapidly to disturbances (Watts and Strogatz, 1998).
Seasonal changes have a significant impact on the species composition and biomass of benthic macroalgae, with water temperature being the main ecological factor influencing this variation (Chefaoui et al., 2024). Spring is the season for the germination and growth of seaweeds. During this time, most temperate species have not yet disappeared, while warm-water species have already emerged, resulting in the highest level of species richness (Zhang et al., 2024). A total of 117 species were identified, and the biomass reached its peak at 1344.31 g·m-2, with high algal density. Rhodophyta dominated, with 69 species (58.97%) and the highest biomass of 696.70 g·m-2. Phaeophyceae accounted for 27.0% of the biomass, while Chlorophyta contributed 21.2% of the biomass. In autumn, the number of species was the least, with only 66 species identified, and biomass decreased to 428.78 g·m-2, the lowest density observed. In winter, many algae began to germinate, increasing the species count to 78. Rhodophyta accounted for 49 species (62.82%) (Table 1; Figure 4A).
Figure 4. Seasonal distribution of benthic macroalgae in the Nanji Islands. (A) Biomass and density distribution. (B) α-diversity of macroalgae in different seasons. (C) α-diversity of macroalgae in different regions. *P< 0.05, **P< 0.01, ***P< 0.001.
Throughout the seasons, Rhodophyta consistently had the highest species count and demonstrated strong competitiveness in terms of biomass and density, reflecting their superior adaptability and survival ability in the ecosystem. This resilience of Rhodophyta aligns with studies in other temperate marine ecosystems, such as the rocky shores of the Northeast Pacific, where they also dominate under variable environmental conditions (Nelson, 2009). Studies have revealed that Rhodophyta frequently thrive under adverse abiotic environmental stressors, such as the desiccation and hyperosmotic conditions in the intertidal zone (Chen et al., 2022). These conditions often limit the growth of other algae. Phaeophyceae, with an average proportion of 19.69%, showed a certain level of stability; however, the biomass and density of Phaeophyceae decreased in autumn, a pattern that may be related to seasonal changes in environmental factors such as temperature, light, and nutrients. Similar trends in Phaeophyceae biomass reduction in autumn have been observed in the Sargasso Sea, where seasonal variations in light availability and water temperature play crucial roles (Ford et al., 1999). Chlorophyta, with an average proportion of 22.23%, had the fewest species in winter (only 15 species), while biomass and density were lowest in autumn. Cyanobacteria were represented by only two species, present in all of the seasons except winter; however, due to their low abundance and minimal proportion, they occupied a disadvantaged position in this ecosystem (Figure 4A; Supplementary Table S4).
The α-diversity analysis across different seasons indicated that for the Nanji Islands, there were no significant differences in the Shannon-Wiener or Simpson diversity indices, nor in the Pielou evenness index. Nevertheless, the Margalef species richness index demonstrated more distinct seasonal variations. In spring, both the biodiversity and richness indices were higher compared to the other three seasons (P< 0.05), whereas in autumn, these indices reached their lowest levels (Figure 4B).
Within each region, the α-diversity of benthic macroalgae across different seasons showed significant differences (Figure 4C). Specifically, the diversity indices of the northern side and the main island displayed distinct seasonal variations, with spring having the highest values and autumn the lowest (P< 0.05). The consistently high Pielou index across regions (excluding rafts) implies an even distribution of species. This is likely because of niche partitioning and a decrease in the dominance of stress-tolerant species. Conversely, the low Shannon (H’) and Simpson (D) indices suggest limited species richness and weak dominance hierarchies, especially in autumn. The seasonal patterns, with high indices in spring and low in autumn, are in line with the temperature-driven phenology of algae. Spring’s optimal conditions, including moderate temperature, sufficient light, and abundant nutrients, promote the recruitment of new species and enhance overall diversity. In contrast, autumn’s cooling temperatures and reduced light favor a smaller number of generalist species, such as C. officinalis, thus decreasing richness (as measured by the Margalef index) and diversity (as measured by the Shannon and Simpson indices).
Although the southern side also followed a similar trend, the Shannon-Wiener and Simpson diversity indices showed minimal change between summer and autumn. This can be ascribed to the strong influence of the Taiwan Warm Current, which results in higher water temperatures and less seasonal variability in this area (Shu et al, 2020) (Figure 4C). This influence of the Taiwan Warm Current on reducing seasonal diversity fluctuations in the southern Nanji Islands is comparable to the role of the Kuroshio Current in maintaining relatively stable algal communities in some Japanese coastal regions (Chen, 1996).
The subdued seasonal fluctuations in raft communities probably stem from the artificially stabilized conditions, such as the consistent nutrient runoff from aquaculture and the reduced tidal exposure. These conditions are favorable for opportunistic, fast-growing species like Sargassum, which can thrive throughout the year. As a result, seasonal succession is suppressed, and low diversity indices are maintained despite high evenness. This pattern of suppressed seasonal succession in raft communities is also observed in other aquaculture-intensive areas, such as the coastal waters of Southeast Asia, where continuous nutrient inputs support the growth of fast-growing algal species (He et al., 2021).
The algal flora of the Nanji Islands belongs to the East China Sea subregion within the Indo-West Pacific plant region. The area represents a transitional zone from warm temperate to subtropical characteristics (Li et al., 2024). The community structure exhibits clear temperate features, with warm-water algae dominating in both abundance and species counts across all of the seasons.
The survey results revealed that warm-temperate algae were the most abundant, with 71 species (55.91%) identified. Notable species included S. thunbergii, Gloiopeltis furcata, and S. fusiforme, the dominant species in the intertidal zone of the Nanji Islands. Cold-temperate algae accounted for 20 species (15.75%), while no cold-water algae were found. Only one tropical species, Scinaia tsinglanensis, was identified, and it was considered a rare species.
Subtropical algae exhibited a certain degree of dominance, with 36 species (28.34%) identified, including those in the genus Corallina. Sun et al. (2008) noted that subtropical coralline algae were the absolute dominant species at their survey sites (Mazuao Bay), and calcareous Corallina species tended to calcify surrounding algae. Similar findings have been reported by researchers who observed the growth and rapid population expansion of Corallina at Mazuao Bay (Tang et al., 2014) and intertidal zones of Nanji Islands (Fu et al., 2011). In our survey, coralline algae were abundant across all of the seasons and at almost all of the sampling sites. The increasing atmospheric CO2 concentration affects the seawater pH and carbon concentrations in different forms; this in turn influences the calcification processes in the ocean (Gao et al., 1993a, Gao et al., 1993b, Gao, 1999; Xu et al., 2006). Coralline algae are highly calcified Rhodophyta that can withstand direct wave impacts and current strikes. Their overgrowth in the Nanji Islands suggests ecological disturbance in the region (Hofmann et al., 2012; Cannell, 1993).
In addition to coralline algae, other subtropical species such as Gracilaria chouae, Ulva fasciata, and Dictyopteris latiuscula demonstrated relatively high biomass. These species were once considered to be at the northernmost distribution limit in China around the Nanji Islands (Fu et al., 2011; Cai, 2021). Similar patterns have been reported in previous surveys, such as those conducted by Sun et al. (2000–2007) and Wu et al. (2016–2017) in which warm-temperate algae were most abundant; subtropical algae followed in abundance, while no cold-water algae were found in the Nanji Islands (Sun et al., 2008; Wu, 2019).
Ocean currents are a major factor influencing the distribution and growth of algae. The Nanji Islands are located at the confluence of the Taiwan Warm Current and the Zhejiang-Fujian Coastal Current. When these two currents meet, upwelling and eddies are formed, bringing nutrient-rich species to the surface and thereby creating favorable conditions for the growth and development of benthic macroalgae (Li et al., 2024). This also introduces species with different temperature tolerances to the Nanji Islands. The southern side of the islands is strongly influenced by the Taiwan Warm Current, a branch of the Kuroshio Current characterized by high temperatures, salinity, and nutrient levels (Chen, 1996). The northern side is more influenced by the Zhejiang-Fujian Coastal Current characterized by low temperatures, low salinity, and high nutrient levels. We observed that the northern side harbored more cold-temperate species (20 species), while the southern side had only 12 such species. However, due to the higher overall species richness on the northern side, the total number of subtropical and warm-temperate species on the southern side did not exceed that on the northern side. Seasonal differences were evident, with subtropical and tropical species accounting for 89.01% of the species on the southern side during summer, greater than the 85.71% on the northern side. In contrast, during winter, cold-temperate species account for 25.97% of the species on the northern side, higher than the 22.44% on the southern side (Table 2). In summer, the Taiwan Warm Current is stronger than the Zhejiang-Fujian Coastal Current (Li et al., 2024), causing subtropical species to migrate northward to the southern islands. For example, Sargassum and Dictyopteris were widely distributed in areas such as S_poy, S_jy, and S_xma. In winter, the Zhejiang-Fujian Coastal Current becomes stronger than the warm current, bringing cold-water species such as Pyropia/Neopyropia and Polysiphonia senticulosa to the northern islands in areas such as N_dls, N_hjs, and N_zy. This seasonal interaction of ocean currents not only influences the spatial distribution of species but may also have profound effects on ecosystem stability and biodiversity.
Table 2. Thermal distribution of benthic macroalgae across different regions and seasons in the Nanji islands.
Tidal fluctuations cause regular vertical zonation of seaweeds, with algae forming distinct vertical distributions in response to tidal changes (Umanzor et al., 2019). In this study, we analyzed the changes in biomass across the high, mid, and low tidal zones. The results showed that the mid-tidal zone and lower low-tidal zone were the primary areas for algal growth, with the mid-tidal zone exhibiting the highest biomass, reaching 1947.29 g·m-2, with an average biomass of 486.82 g·m-2 across the stations. The low-tidal zone followed with a biomass of 964.03 g·m-2, while the high-tidal zone showed the lowest biomass at 727.97 g·m-2, with an average biomass of only 181.99 g·m-2. There was clear vertical zonation of seaweeds. The mid-tidal zone had an optimal environment for algal growth, as it received both sunlight and abundant nutrients from seawater agitation, leading to the highest biomass. The low-tidal zone, lacking sufficient sunlight, had limited algal growth. The high-tidal zone frequently faces desiccation that hinders algal growth, leading to the lowest biomass in this zone (Fu et al., 2011). This vertical distribution pattern highlights the influence of environmental factors such as light availability and tidal exposure on the growth and distribution of benthic macroalgae (Roberts et al., 2018).
Eighteen species of benthic macroalgae were collected in the high tidal zone of the Nanji Islands, with Rhodophyta being the dominant group. The species included Bangia fuscopurpurea, Gelidium amansii, and Gloiopeltis furcata, along with lesser numbers of Cyanophyceae such as Lyngbya semiplena and Chlothrix crustacea. S. thunbergii was the dominant brown alga in the high tidal zone. Biomass in the high tidal zone was highest in spring, with the maximum recorded at site S_poy, reaching 744.07 g·m-2. In contrast, biomass was lowest in winter, with the site M_my recording only 4.02 g·m-2.
Forty-one species of benthic macroalgae were collected in the middle tidal zone of the Nanji Islands, with Rhodophyta and Chlorophyta being the dominant groups, along with a small number of Phaeophyceae. The Rhodophyta included species in the genera Amphiroa, Corallina, and Hypnea, with G. furcata and Pyropia forming common communities. The Chlorophyta included Chaetomorpha, Bryopsis, and Ulva. Phaeophyceae included Ectocarpus siliculosus, I. okamurae, S. fusiforme, and S. thunbergii.
The lower part of the middle tidal zone showed abundant algal growth, with the highest species richness. The peak biomass in the middle tidal zone occurred in winter, with site M_hyt reaching 1796.79 g·m-2, while the lowest biomass was recorded in autumn at site N_xls, with only 67.32 g·m-2.
A total of 64 species of benthic macroalgae were found exclusively in the low-tidal and subtidal zones, including Rhodophyta such as Grateloupia and Hypnea, as well as Phaeophyceae such as Sargassum and Dictyota. The upper part of the subtidal zone was nearly completely dominated by Sargassum. Biomass in the low-tidal zone was highest in spring, with site M_zdw reaching 844.93 g·m-2, while the lowest biomass was observed in autumn at site M_hl, with only 4.55 g·m-2 (Figure 5A).
Figure 5. Vertical distribution of benthic macroalgae in the Nanji Islands. (A) Biomass at different sampling sites. (B) α-diversity of macroalgae at different tidal zones. *P< 0.05, **P< 0.01.
Although each tidal zone had its dominant species, the same type of seaweed could occur in other zones (Fu et al., 2011). Chlorophyta gradually decreased from the high tide zone to the low tide zone; Phaeophyceae were typically distributed in the mid-tidal zone, and Rhodophyta increased from the high tide zone to the low tide zone. Moreover, the vertical distribution of seaweed biomass showed significant seasonal variation. In spring, the biomass was concentrated in the lower mid-tidal zone; during summer and autumn, the biomass of algae was higher in the lower mid-tidal and low tide zones, and in winter, the biomass was higher in the lower mid-tidal and middle zones.
The α-diversity analysis of seaweeds in different tidal zones (Figure 5B) showed similar trends in community structure across the tidal zones and raft-based macroalgae among the different seasons. Specifically, the Simpson diversity index and Pielou evenness index showed no significant differences; however, the Margalef species richness index indicated significant differences across the tidal zones. Both biodiversity and species richness were higher in the lower mid and low tide zones compared to the high tide zone and raft areas, with the high tide zone showing the lowest biodiversity and species richness.
We selected the M_mza site for comparison with historical data. Sun et al. (2010) analyzed changes in the species composition of benthic macroalgae at the Mazuao site (the M_mza site in this study) from 1960 to 2007. There was a decline in algal species biodiversity over the 40-year period they examined. Specifically, the number of algae species during 1960–1965, 1980–1985, and 2000–2007 were 84, 73, and 49, respectively. The rates of decline between these 20-year intervals were 13.1% and 32.9%, with the most rapid decrease occurring between 1985 and 2000 (Sun et al., 2010). Tang et al. (2014) found only 24 species of macroalgae in four seasonal surveys at the same site from 2012 to 2013, concluding that algal species had sharply decreased. In comparison to other long-term monitoring sites in similar marine ecosystems, such as the rocky shores of the Californian coast where algal biodiversity also faced challenges due to climate change and human activities (Benedetti-Cecchi et al., 2001), the decline at the M_mza site is part of a broader global trend.
However, the situation at M_mza has its unique aspects. In the current survey, we identified 43 species at the M_mza site. Although the number of algal species was still lower than that in 2007, the percentage of species loss appeared to have slowed significantly (Table 3). From 2007 to 2022, the decline was only 12.2%, and there was even a marked increase compared to that in 2013, suggesting that the establishment of protected areas has played a significant role in conserving the region. After years of restoration, the population is gradually recovering; however, certain species that were once dominant such as Sargassum nigrifolioides and Hincksia zhejiangensis were not found in this survey (Tang et al., 2014). The current reduction in algal diversity has likely been influenced by global climate change and human activities, as the M_mza site is located on the main island where there is significant anthropogenic interference due to tourism and residential development. Numerous studies have shown that human activities significantly influence the structure and composition of algal communities. For example, in many coastal areas around the world, increased tourism has led to habitat destruction and pollution, both of which can negatively impact algal communities. In the case of the M_mza site, the influx of tourists may have led to physical damage to the algal habitats and increased nutrient inputs from sewage, disrupting the natural balance of the ecosystem (Benedetti-Cecchi et al., 2001). Overfishing eliminates crucial herbivores, which in turn causes an increase in the abundance of fleshy macroalgae and cyanobacteria, while simultaneously leading to a decrease in crustose coralline algae. Additionally, eutrophication and sedimentation resulting from coastal activities foster the growth of fleshy macroalgae (Brown et al., 2017; Benedetti-Cecchi et al., 2001).
Table 3. The temporal variation in the species number of benthic macroalgae adapted to different temperatures at the M_mza site of the Nanji Islands.
The proportions of subtropical and warm-temperate species increased between 1980 and 2007, while cold-temperate algae decreased; this is thought to be related to rising water temperatures and increased human activity (Fu et al., 2011). Similar trends have been observed in other temperate-subtropical transition zones, such as the waters off the coast of South Africa, where shifts in species composition due to climate change have been documented (Hofmann et al., 2012). Interestingly, in our 2022 survey, the proportion of cold-temperate algae species was significantly increased at 27.9% compared to 4.1% in 2000. In contrast, the proportion of warm-temperate and subtropical species had decreased (Table 3).
Regarding the species richness at this site, surveys conducted between 1960 and 2007 recorded 50 species of Rhodophyta, 22 species of Phaeophyceae, 13 species of Chlorophyta, and two species of Cyanophyceae. In contrast, the current survey identified only 25 species of Rhodophyta, seven species of Phaeophyceae, and 11 species of Chlorophyta, with no Cyanophyceae detected. Over the past 60 years, both Rhodophyta and Phaeophyceae have experienced significant declines, while the decrease in Chlorophyta (Chlorophyta) has been relatively modest.
From the perspective of biomass changes, between 1985 and 2006, the annual benthic macroalgae biomass at this site decreased by 52.5%, with the most significant reductions occurring in spring and winter, by 54.1% and 87.2%, respectively. Biomass in summer and autumn showed slight increases (Sun et al., 2010). By 2022, the total biomass had recovered to 3643.14 g·m-2, marking an increase of 61.14% compared to that in 2006 (Table 4). The decline in algal biomass from 1985 to 2006 was directly associated with a sharp decrease in the abundance of macroalgae (e.g., Sargassum and Grateloupia) (Sun et al., 2010). There were also significant decreases and disappearance of warm-temperate, filamentous, and sheet-like species (e.g., Ulothrix, Pyropia, Enteromorpha, and Ulva pertusa). In this study, the dominant species at this site included Ulva intestinalis, B. fuscopurpurea, G. furcata, E. siliculosus, and S. thunbergii, and their increase in biomass may have contributed positively to the overall increase in biomass.
Table 4. Comparison of benthic macroalgae biomass at the M_mza site in four seasons from 1985 to 2022 (g/m2).
This study provides a comprehensive qualitative and quantitative assessment of benthic macroalgae in the intertidal zone and aquaculture facilities of the Nanji Islands, China, incorporating a systematic evaluation of species diversity using historical data. The findings highlight the high diversity of benthic macroalgae in this region, with historical records documenting 186 species, of which 127 were identified in this survey. Seasonal analysis revealed that biomass peaked in spring and reached its lowest levels in autumn. Biomass distribution varied significantly across intertidal zones, with the highest abundance in the mid- and low-tide zones and the lowest in the high-tide zone. In terms of seasonal species composition, Rhodophyta dominated throughout the year, exhibiting particularly high species richness and abundance in spring and winter. The algal community structure on the southern side of the Nanji Islands demonstrated greater stability and higher species diversity. Notably, the establishment of marine protected areas has played a crucial role in conserving large macroalgal species, leading to increased species diversity and biomass.
These findings underscore the importance of marine protected areas in mitigating biodiversity loss and fostering ecosystem recovery, serving as a model for coastal conservation. Future research should focus on long-term monitoring to assess ecological resilience under climate change, particularly examining temperature-induced species shifts and the impacts of ocean acidification on calcified algae. Expanding such studies to other biogeographic regions would further enhance our understanding of macroalgal responses to anthropogenic and climatic stressors, informing adaptive management strategies for sustainable marine biodiversity conservation.
The original contributions presented in the study are included in the article/Supplementary Material. Further inquiries can be directed to the corresponding authors.
XW: Data curation, Writing – original draft. SX: Resources, Writing – original draft. WC: Resources, Writing – original draft. XN: Resources, Writing – original draft. WX: Data curation, Methodology, Writing – original draft. QL: Funding acquisition, Project administration, Supervision, Writing – original draft. HC: Funding acquisition, Writing – review & editing.
The author(s) declare that financial support was received for the research and/or publication of this article. This research was supported by China Agriculture Research System of MOF and MARA (CARS-50), NSFC (32373099), Major Scientific and Technological Project of Ningbo (2021Z004, 2021Z103), Key Scientific and Technological Grant of Zhejiang for Breeding New Agricultural (Aquaculture) Varieties (2021C02069-9). We thank LetPub (www.letpub.com.cn) for its linguistic assistance during the preparation of this manuscript.
The authors declare that the research was conducted in the absence of any commercial or financial relationships that could be construed as a potential conflict of interest.
The author(s) declare that no Generative AI was used in the creation of this manuscript.
All claims expressed in this article are solely those of the authors and do not necessarily represent those of their affiliated organizations, or those of the publisher, the editors and the reviewers. Any product that may be evaluated in this article, or claim that may be made by its manufacturer, is not guaranteed or endorsed by the publisher.
The Supplementary Material for this article can be found online at: https://www.frontiersin.org/articles/10.3389/fmars.2025.1563252/full#supplementary-material
An C. G., Zhao Y. L., Lin L., Li J. Y., Cui L. L., Ma C. Y. (2008). The biodiversity of marobenthos of intertidal zone on Chongming Island in summer. Acta Ecol. Sin. 28 (2), 577–586. doi: 10.3321/j.issn:1000-0933.2008.02.016
Barberán A., Bates S. T., Casamayor E. O., Fierer N. (2014). Using network analysis to explore co-occurrence patterns in soil microbial communities. ISME J. 8, 952. doi: 10.1038/ismej.2013.236
Benedetti-Cecchi L., Pannacciulli F., Bulleri F., Moschella P. S., Airoldi L., Relini G., et al. (2001). Predicting the consequences of anthropogenic disturbance: large-scale effects of loss of canopy algae on rocky shores. Mar. Ecol. Prog. Ser. 214, 137–150. doi: 10.3354/meps214137
Benjamini Y., Hochberg Y. (1995). Controlling the false discovery rate: a practical and powerful approach to multiple testing. J. R Stat. Soc. Ser. B Stat. Methodol. 57, 289–300. doi: 10.1111/j.2517-6161.1995.tb02031.x
Bi Y. X., Zhang S. Y., Wu Z. L. (2013). Seasonal variations of Sargassum horneri distribution around Gougi lsland of East China. Chin. J. Ecol. 32 (5), 1255–1259. doi: 10.13292/j.1000-4890.2013.0217
Borja A., Muxika I. (2005). Guidelines for the use of AMBI (AZTI’s Marine Biotic Index) in the assessment of the benthic ecological quality. Mar. Pollut. Bull. 50 (7), 787–789. doi: 10.1016/j.marpolbul.2005.04.040
Brown K. T., Bender-Champ D., Bryant D. E., Dove S., Hoegh-Guldberg O. (2017). Human activities influence benthic community structure and the composition of the coral-algal interactions in the central Maldives. J. Exp. Mar. Biol. Ecol. 497, 33–40. doi: 10.1016/j.jembe.2017.09.006
Cai H. C. (2021). Brief history of nanji islands national marine nature reserve (Beijing: Ocean Press).
Cannell J. P. R. (1993). Algae as a source of biologically activeproducts. J. Pestic. Sci. 39, 147–153. doi: 10.1002/ps.2780390208
Chefaoui R. M., Martínez B. D. C., Viejo R. M. (2024). Temporal variability of sea surface temperature affects marine macrophytes range retractions as well as gradual warming. Sci. Rep. 14 (1), 14206. doi: 10.1038/s41598-024-64745-7
Chen C. (1996). The Kuroshio intermediate water is the major source of nutrients on the East China Sea continental shelf. Oceanol. Acta 19, 523–527.
Chen H., Chu J. S. C., Chen J., Luo Q., Wang H., Lu R., et al. (2022). Insights into the ancient adaptation to intertidal environments by red algae based on a genomic and multiomics investigation of Neoporphyra haitanensis. Mol. Biol. Evol. 39, msab315. doi: 10.1093/molbev/msab315
Chen G. T., Yang X. L., Yang J. Y., Gao A. G. (1994). Ecological and environment qualitative study in the intertidal zone and land area of Nanji Archipelago. Donghai Mar. Sci. 12 (2), 1–15.
Chi Y., Shi H. H., Wang Y. Y., Guo Z., Ma D. M. (2017). Evaluation on spatial heterogeneity of island ecosystem carrying capacity-A case study of southern Miaodao Archipelago. China Environ. Sci. 37, 1188–1200. doi: 10.3969/j.issn.1000-6923
Figueira T., Martins N., Ayres-Ostrock L., Plastino E., Enrich-Prast A., Oliveira V. (2021). Erratum to: The effects of phosphate on physiological responses and carbohydrate production in Ulva fasciata (Chlorophyta) from upwelling and non-upwelling site. Bot. Mar. 64, 161–161. doi: 10.1515/bot-2021-2002
First Institute of Oceanography, State Oceanic Administration, Third Institute of Oceanography, State Oceanic Administration (2007a). Specifications for oceanographic survey—Part 6: Marine biological survey (GB/T 12763.6-2007) (Beijing: Standards Press of China).
First Institute of Oceanography, State Oceanic Administration, Third Institute of Oceanography, State Oceanic Administration (2007b). Specifications for oceanographic survey—Part 9: Guidelines for marine ecological survey (GB/T 12763.9-2007) (Beijing: Standards Press of China).
Ford R. B., Thrush S. F., Probert P. K. (1999). Macrobenthic colonisation of disturbances on an intertidal sandflat: the influence of season and buried algae. Mar. Ecol. Prog. Ser. 191, 163–174. doi: 10.3354/meps191163
Fu C. H., Jiang X. M., Mao X. X., Xu C. B. (2011). Distribution characteristics of benthic Algae in intertidal zone of Dachai Island of Nanji Archipelago in Zhejiang Province. J. Ningbo Univ. Nat. Sci. Eng. Ed. 24, 25–30. doi: 10.3969/j.issn.1001-5132.2011.02.005
Gao K., Aruga Y., Asada K., Ishihara T., Akano T., Kiyohara M. (1993a). Calcification in the articulated coralline alga Carollina pululifera, with special reference to the effect of elevated atmospheric CO2. Mar. Biol. 117, 129–132. doi: 10.1007/BF00346434
Gao K., Aruga Y., Asada K., Kiyohara M. (1993b). Influence of enhanced CO2 on growth and photosynthesis of the red algae Gracilaria sp. and G. Chilensis. J. Appl. Phycol. 5, 563–571. doi: 10.1007/BF02184635
He P. M., Duan Y. L., Liu Q., Liu J. L., Liu W., Zhang J. H., et al. (2021). Strategy of macroalgae eco-remediation with cases in nearshore China. J. Appl. Oceanogr. 40, 557–563. doi: 10.3969/J.ISSN.2095-4972
Hofmann L. C., Yildiz G., Hanelt D., Bischof K. (2012). Physiological responses of the calcifying rhodophyte, Corallina officinalis (L.), to future CO2 levels. Mar. Biol. 159 (4), 783–792. doi: 10.1007/s00227-011-1854-9
Li Y. H., Chen W. D., Cai H. C., Sun Z. M., Xu K. D. (2017). Spatio-temporal variation of benthic diatom diversity and community structure in a sandy intertidal zone of the Nanji Islands, China. Biodiversity Sci. 25, 981–989. doi: 10.17520/biods.2017052
Li J. Y., Liu Y. C., Liu Y., Wang Q. H., Gao X., Gong Q. L. (2019). Effects of temperature and salinity on the growth and biochemical composition of the brown alga Sargassum fusiforme (Fucales, Phaeophyceae). J. Appl. Phycol. 31, 3061–3068. doi: 10.1007/s10811-019-01795-9
Li H., Xu A. N., Yang Z., Lei Y., Chen J., Zhan Z., et al. (2024). Assessing the changes in marine microalgae diversity in the Nanji Islands Nature Reserve over the past decades using sediment eDNA. Front. Mar. Sci. 11, 1466434. doi: 10.3389/fmars.2024.1466434
Liao C. H. (2021). Spatial distribution of coral community and benthic algae and their ecological impacts across the South China Sea. [Ph.D. dissertation] (Guangxi Province, China: Guangxi University).
Nelson W. A. (2009). Calcified macroalgae–critical to coastal ecosystems and vulnerable to change: a review. Mar. Freshw. Res. 60, 787–801. doi: 10.1071/MF08335
Pan Y. Q., Su J. L., Xu D. R. (1991). The Characteristics of hydrology structure and seasonal variations in continental shelf northern from Taiwan Warm. Res. Papers Kuroshio. 17, 172–181.
Peng X., Xie Q. L., Chen S. B., Huang X. L., Qiu J. B., Zhong W., et al. (2009). Distribution of intertidal benthos and the human impact in Nanji Islands, China. Oceanol. Limnol. Sin. 40 (5), 584–589. doi: 10.3321/j.issn:0029-814X
Qiu W. W., Gui F. K., Wu C. W. (2011). Macroalgae survey attached on offshore fish cages in Zhongjieshan Islands. J. Zhejiang Ocean Univ. Nat. Sci. (Beijing China). 30, 194–199. doi: 10.3969/j.issn.1008-830X.2011.03.016
Roberts E. M., Bowers D. G., Davies A. J. (2018). Tidal modulation of seabed light and its implications for benthic algae. Limnol. Oceanogr. 63, 91–106. doi: 10.1002/lno.10616
Ruan J. H. (1994). Preliminary study on the intertidal ecology of marine algae on Yushan Islands. Donghai. Mar. Sci. 12 (4), 48–57.
Shi W. S. (2019). Trend analysis of margalef′s diversity index (D) of algae in Zhangze Reservoir. Shanxi Hydrotechnics. 45 (1), 93–96. doi: 10.3969/j.issn.1006-8139.2019.01.028
Shu W., Wang P., Zhang H., Ding M., Wu B. (2020). Seasonal and spatial distribution and assembly processes of bacterioplankton communities in a subtropical urban river. FEMS Microbiol. Ecol. 96, fiaa154. doi: 10.1093/femsec/fiaa154
Sun S. P. (2021). Community assembly and biodiversity patterns of benthic diatom in Anzhaixin River Basin. [Master’s thesis] (Heilongjiang Province, China: Harbin Normal University).
Sun J. Z., Chen W. D., Zhuang D. G., Zheng H. Y., Lin L., Feng S. J. (2008). In situ ecological studies of the subtidal brown alga Sargasssum horneri at Nanji Island of China. South China Fish. Sci. 4, 58–63. doi: 10.3969/j.issn.2095-0780
Sun J. Z., Ning X. R., Le F. F., Chen W. D., Zhuang D. G. (2010). Long term changes of biodiversity of benthic macroalgae in the intertidal zone of the Nanji Islands. Acta Ecol. Sin. 30, 106–112. doi: 10.1016/j.chnaes.2010.03.010
Tang Y. B., Liao Y. B., Shou L., Zeng J. N., Gao A. G., Chen Q. Z., et al. (2014). Influence of coralline algae on biodiversity of macrobenthic community in intertidal zone of Nanji Islands. Biodiversity Sci. 22 (5), 640–648. doi: 10.3724/SP.J.1003.2014.13240
Umanzor S., Ladah L. B., Calderón-Aguilera L. E., Zertuche-González J. A. (2019). Testing the relative importance of intertidal seaweeds as ecosystem engineers across tidal heights. J. Exp. Mar. Biol. Ecol. 511, 100–107. doi: 10.1016/j.jembe.2018.11.008
Wang J., Jiao Y., Ren Y. P., Xue Y., Ji Y. P., Xu B. D. (2015). Comparative study on two computing methods for estimating Shannon-Wiener diversity index. J. Fish. China. 39 (8), 1257–1263. doi: 10.11964/jfc.20150509886
Watts D. J., Strogatz S. H. (1998). Collective dynamics of ‘small-world’ networks. Nature. 393, 440–442. doi: 10.1038/30918
Wei N. (2015). Preliminary study on the community structure of algal plants in Harbin section of Songhua River. [Master’s thesis] (Heilongjiang Province, China: Harbin Normal University).
Wu E. W. (2019). Effects of different conservation intensities on biodiversity of benthic macro-algae in the intertidal zone of Nanji Islands. [Master’s thesis] (Zhejiang Province, China: Zhejiang Ocean University).
Xu J. R., Sun S., Yin J. P., Wang Y. S. (2006). Adavances of studies on marine carbon cycle. Acta Ecol. Sin. 26, 566–575. doi: 10.3321/j.issn:1000-0933.2006.02.033
Yang C. H., Cai J. B., Zhang P., Chen W. D., Nan C. R. (2017). Determination of heavy metal contents in macroalgae from the Nanji Islands, China. Mar. Environ. Science. 36, 372–378. doi: 10.13634/j.cnki.mes20170309
Yang J., Wang C. X., Wang Z., Li Y. J., Yu H. Y., Feng J., et al. (2024). Distribution patterns and co-occurrence network of eukaryotic algae in different salinity waters of Yuncheng Salt Lake. China. Sci. Rep. 14 (1), 8340–8340. doi: 10.1038/s41598-024-58636-0
Zhang Z., Wang F. P., Lei L. Y., Zheng N., Shen Z. Y., Mu J. L. (2024). Spatio-temporal dynamics of the carbonate system during macroalgae farming season in a semi-closed bay in southeast China. Front. Mar. Sci. 11, 1375839. doi: 10.3389/fmars.2024.1375839
Zhang Y. P., Ying X. P., Wu H. L., Wang Y. N. (2000). The characteristics of the benthic community composition in the rocky intertidal zone of Beiji Islands. Trans. Oceanology Limnology. 4, 26–33.
Keywords: Nanji Islands, benthic macroalgae, survey, species diversity, biomass
Citation: Wang X, Xie S, Chen W, Ni X, Xu W, Luo Q and Chen H (2025) Distribution and biodiversity of benthic macroalgae in the Nanji Islands, China. Front. Mar. Sci. 12:1563252. doi: 10.3389/fmars.2025.1563252
Received: 19 January 2025; Accepted: 19 March 2025;
Published: 08 April 2025.
Edited by:
Martin F. Soto-Jimenez, National Autonomous University of Mexico, MexicoReviewed by:
Xinming Lei, hinese Academy of Sciences, ChinaCopyright © 2025 Wang, Xie, Chen, Ni, Xu, Luo and Chen. This is an open-access article distributed under the terms of the Creative Commons Attribution License (CC BY). The use, distribution or reproduction in other forums is permitted, provided the original author(s) and the copyright owner(s) are credited and that the original publication in this journal is cited, in accordance with accepted academic practice. No use, distribution or reproduction is permitted which does not comply with these terms.
*Correspondence: Qijun Luo, bHVvcWlqdW5AbmJ1LmVkdS5jbg==; Haimin Chen, Y2hlbmhhaW1pbkBuYnUuZWR1LmNu
Disclaimer: All claims expressed in this article are solely those of the authors and do not necessarily represent those of their affiliated organizations, or those of the publisher, the editors and the reviewers. Any product that may be evaluated in this article or claim that may be made by its manufacturer is not guaranteed or endorsed by the publisher.
Research integrity at Frontiers
Learn more about the work of our research integrity team to safeguard the quality of each article we publish.