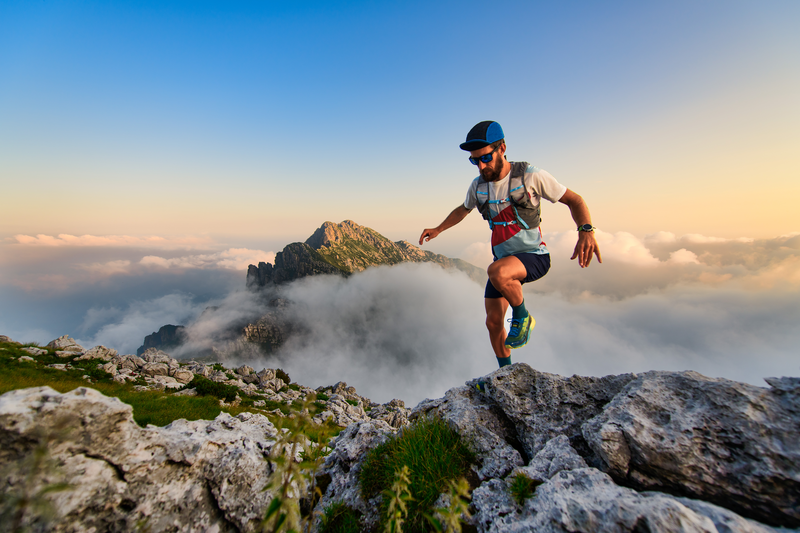
94% of researchers rate our articles as excellent or good
Learn more about the work of our research integrity team to safeguard the quality of each article we publish.
Find out more
ORIGINAL RESEARCH article
Front. Mar. Sci. , 03 February 2025
Sec. Marine Pollution
Volume 12 - 2025 | https://doi.org/10.3389/fmars.2025.1546186
This article is part of the Research Topic New Pollutants in the Marine Ecosystem: Environmental and Human Health from a One Health Perspective View all articles
Introduction: Steroid hormones are widely present in the environment and pose potential risks to organisms. Previous studies of steroid hormones have predominantly focused on terrestrial environments, with few studies conducted in marine environments.
Methods: In this study, we analyzed the occurrence of 44 steroid hormones in seawater, sediment, and marine organisms collected from the coast of Guangdong, China.
Results: Total concentration of steroid hormones ranged from 0.11 to 30.15 ng/L in seawater, ND to 8.58 ng/g (dw) in sediments, and ND to 80.52 ng/g (ww) in organisms. The highest average concentrations of steroid hormones detected in seawater, sediments, and marine organisms were progestins, estrogens, and glucocorticoids, respectively. Steroid hormone concentrations in seawater were significantly higher during the dry season than the rainy season. The concentrations of steroid hormones in Guanghai Bay, the Pearl River Estuary, Daya Bay, and Zhelin Bay were significantly higher than those in other bays.
Discussion: Negative correlations were observed between steroid hormones and salinity, indicating a potential continental input. Risk assessment results indicated that 17α-ethinylestradiol in Zhelin Bay posed high risks. Nevertheless, the consumption of seafood does not pose a significant health risk to humans. To the best of our knowledge, this is the first study to concurrently analyze androgens, glucocorticoids, progestins, and estrogens in water, sediment, and organisms from diverse marine environments.
Steroid hormones, including estrogens, androgens, progestogens, and glucocorticoids, are ubiquitous environmental contaminants with potential adverse effects on humans, animals, and plants (Fent, 2015). They are extensively used in medicine to treat or prevent diseases in both humans and animals and as growth promoters in animal husbandry (Streck, 2009). Although the majority of steroid hormones undergo degradation in wastewater treatment plants (WWTPs), their complete removal may not be achieved. Consequently, effluents from livestock breeding, aquaculture, industry, and domestic sewage sources, which potentially contain substantial quantities of hormones and their metabolites, may be discharged into the natural environment (Zhong et al., 2021). Besides, minor amounts of steroid hormones are introduced into natural aquatic environments via direct discharge (Yazdan et al., 2022).
Many studies have reported that steroid hormones are widely detected in various environmental media worldwide. Steroid hormones have been detected in surface water, groundwater, seawater, animal farm effluent, and WWTPs effluent, with concentrations up to 1440, 390, 831, 1720, and 4650 ng/L, respectively (Zhang et al., 2018; Lu et al., 2020; Ojoghoro et al., 2021; Zhong et al., 2021). Steroid hormones have also been detected in soil, sediment, and feces, with concentrations up to 135, 4800, and 7000 ng/g, respectively (Aris et al., 2014; Adeel et al., 2017; Huanyu et al., 2022). Several studies have also detected steroid hormones in aquatic organisms from both wild and marine aquaculture farms (Ojoghoro et al., 2021).
Environmental steroid hormones may pose a risk to wildlife because they are known for their potent endocrine-disrupting effects, which can have negative ecological and human health impacts (Ismanto et al., 2022). Several studies have demonstrated that steroid hormone exposure can feminize male fish (DeQuattro et al., 2015) alter anal fin development (Frankel et al., 2016), damage reproductive organs and reproductive behavior (Dong et al., 2022), and reduce larval survival and growth in aquatic animals (Islam et al., 2020).
Previous studies on steroid hormones have predominantly focused on terrestrial environments, with comparatively few studies in marine environments, with the exception of the Yellow, Yangtze, and Pearl River Estuaries. Therefore, it is necessary to study the occurrence, source, and risk of steroid hormones in various marine environments.
The coast of Guangdong is a highly developed region with approximately 130 million people residing in Guangdong Province, China. Guangdong's coastline features various functional regions, including areas for marine farming, fishing harbors, tourist destinations, farmlands, and multipurpose zones. The concentration of steroids may vary across these distinct functional zones, making the coastal area of Guangdong an ideal case study for obtaining comprehensive insights into steroid contamination in diverse marine environments.
This study analyzed the levels of steroid hormones in seawater, sediment, and marine organisms from the Guangdong coastline. The ultimate objective was to provide novel and comprehensive insights into the occurrence, risk, and sources of steroid hormones in diverse marine environments. This information is essential for effective management of steroid hormone pollution in coastal regions.
Forty-four steroid hormones were selected as target compounds, comprising 14 androgens, 5 glucocorticoids, 21 progestins, and 4 estrogens. Basic chemical information for the target compounds is provided in Supplementary Table S1 of the Supporting Information (SI). The internal standards (ISTDs) used included testosterone-16,16,17-d3, stanozolol-d3, cortisol-d2, mifepristone-d2, melengestrol acetate-d3, 19-nortestosterone-d6, progesterone-d9, estrone-d4, and 17β-estradiol-d4.
HPLC-grade organic solvents were purchased from Merck (Germany). Ultrapure water was obtained using a Milli-Q ultrapure system (Millipore, USA). All stock solutions were prepared in methanol, stored at -18 °C, and kept away from light to prevent degradation until use.
Samples were collected from ten bays along the coast of Guangdong, including Zhenhai Bay (ZHW), Guanghai Bay (GHW), the Pearl River Estuary (ZJK), Dapeng Bay (DPW), Daya Bay (DYW), Honghai Bay (HHW), Shenquan Bay (SQW), Guangao Bay (GAW), Shantou Port (STG), and Zhelin Bay (ZLW). Detailed information regarding the sampling sites is provided in Figure 1 and Supplementary Table S2. A total of 68 water samples (two replicates each), 68 sediment samples, and 29 marine organism samples were collected in August 2021, during the rainy season. To investigate seasonal variations, additional 68 water samples (two replicates each) were collected in March 2022 during the dry season.
Figure 1. Locations of the sampling sites. ZHW, Zhenhai Bay; GHW, Guanghai Bay; ZJK, Pearl River Estuary; DPW, Dapeng Bay; DYW, Daya Bay; HHW, Honghai Bay; SQW, Shenquan Bay; GAW, Guangao Bay; STG, Shantou Port; ZLW, Zhelin Bay.
Two liters of water were collected in stainless steel buckets and then filled into two separate 1 L brown glass bottles that had been rinsed with tap water, ultrapure water, and methanol prior to use. Immediately after collection, the pH of the surface water samples was adjusted to 3.0 using 4 M H2SO4, and 50 mL of methanol was added to prevent microbial activity. Samples were stored at 4°C and transported to the laboratory.
Surface sediment sample (0-4 cm in depth) was collected using a grab sampler and placed in a 100 mL brown wide-mouth glass bottle.
Marine organism samples were collected by bottom trawl and stored at -18°C after collection. Detailed information regarding the marine organism samples is provided in Supplementary Table S3.
All water samples were filtered through 0.7 μm GF/C Whatman filters prior to solid-phase extraction (SPE). The samples were spiked with 50 μL 1 mg/L of isotope-labele ISTDs. Each water sample was then loaded onto a HLB cartridge (500 mg, 6 mL, Waters) at a flow rate of 5-10 mL/min. The sample washing step was performed using 5 mL methanol, 4 mL ethyl acetate, and 3 mL dichloromethane. Subsequently, the samples were evaporated to dryness under a stream of nitrogen.
The freeze-dried sediment samples were ground, passed through a 0.3 mm sieve, and fully homogenized. A 2.00 g aliquot of the pretreated sediment was weighed into a 50 mL centrifuge tube. The sample was spiked with 50 μL 1 mg/L of ISTDs and allowed to stand overnight at 4°C in the dark. Before extraction, 5 mL of 0.1 M EDTA–McIlvaine buffer solution was added to the samples, followed by vortexing. Subsequently, 10 mL formic acid/acetonitrile (1/50, v/v) and a ceramic homogenizer were added to the mixture and vortexed thoroughly for 30 s. Subsequently, the QuEChERS extraction salts (4 g Na2SO4 and 1 g NaCl) were added to the tube, shaken immediately, and centrifuged at 6000 rpm for 5 min. The supernatant was then transferred to a QuEChERS dSPE tube, vortexed for 1 min, and centrifuged at 5000 rpm for 5 min. The supernatant was then transferred to a 10 mL glass test tube and dried under a stream of nitrogen.
Before pretreatment, the weight and length of each marine organism were recorded. A 2 g (wet weight) portion of homogenized muscle tissue was accurately weighed into a 50 mL centrifuge tube and mixed with 50 μL of 1 mg/L ISTDs. Prior to extraction, 5 mL of 0.1 M EDTA–McIlvaine buffer solution and two ceramic homogenizers were added to the samples and vortexed for 3 min. Then, 100 μL digestive enzyme was added to the samples, which were subsequently kept at 37°C in the dark overnight. Then, 8 mL of formic acid/acetonitrile (1/50, v/v) was added to the centrifuge tubes, shaken immediately, and centrifuged at 6000 rpm for 5 min. The supernatant was then transferred to Captiva EMR-Lipid cartridges for defatting and purification. The filtrate was then collected in a 10 mL glass test tube and dried under a stream of nitrogen.
Finally, each sample was redissolved in 500 μL of methanol, passed through a 0.22 μm filter, and transferred to a brown glass sample vial for analysis.
High-performance liquid chromatography coupled with tandem mass spectrometry (HPLC-MS/MS, Agilent 1260 Infinity- AB SCIEX 4000 Qtrap) was used to analyze androgens, progestogens, and glucocorticoids. The chromatographic column was an Agilent Poroshell 120 EC-C18 column (2.1 mm × 100 mm, 2.7 µm). The column oven temperature was maintained at 25°C in the positive ion mode (ESI +), and the injection volume was 5 μL. The mobile phase consisted of (A) methanol and (B) ultrapure aqueous solution containing 0.05% formic acid (v/v) at a flow rate of 0.25 mL/min. The gradient program involved a stepwise increase in the concentration of phase A over 14 min as follows: 60% A at 0 min, held for 5 min, 100% A from 6 to 8 min, and 60% A from 8.5 to 14 min. Nitrogen was used as collision gas.
Ultra-performance liquid chromatography coupled with a Xevo TQ-S triple quadrupole mass spectrometer (Waters Co., Milford, MA, USA) was used to analyze estrogens. In negative ion mode (ESI-), chromatographic separation was performed on an ACQUITY UPLC BEH C18 column (2.1 × 50 mm, 1.7 µm). The column temperature was maintained at 40°C and the injection volume was 5 μL. The mobile phase consisted of 0.05% (v/v) ammonium acetate in Milli-Q water (A) and methanol (B), at a flow rate of 0.4 mL/min. The total analysis time for each sample was 5.2 mins. The gradient elution program was as follows: 40% B at 0 min, increased to 98% B at 3 min, held at 98% B from 3.5 to 3.8 min, returned to 40% B from 3.8 to 4.0 min, and holding for 0.7 min.
All experimental data was subjected to strict quality assurance and quality control processes. Two field blanks and two laboratory blanks were prepared for each batch to assess potential contamination during the experimental procedure. None of the target compounds were detected in the blank samples. Seven standard solution concentrations (0.5, 2, 8, 30, 100, 200, and 500 μg/L) were used to calculate calibration curves (R2 > 0.995). The limit of detection (LOD) and limit of quantitation (LOQ) were defined as the sample concentrations at signal-to-noise ratios (S/N) of three and ten times, respectively. The recoveries of the target steroid hormones ranged from 63% to 117% in water samples, 56% to 122% in sediment samples, and 64% to 111% in organism samples (Supplementary Table S5). The relative standard deviations (RSDs) of field parallel samples were all below 15%.
The bioconcentration factor (BCF, L/kg) was used to describe the uptake and enrichment of substances from the surrounding water by aquatic organisms.
where Cb (ng/g ww) is the concentration of steroid hormones in the marine organism samples and CW (ng/L) is the concentration of steroid hormones in the seawater samples.
The ecological risks associated with the levels of steroid hormones in aquatic environments were assessed using risk quotients (RQ):
where MEC is the measured environmental concentration (ng/L) and PNEC is the predicted no-effect concentration (ng/L). The latter was calculated by dividing the quotient of the median effective concentration (EC50) or lethal concentration (LC50) by an assessment factor (AF) for acute toxicity associated with water. Estimated daily intake (EDI) and hazard quotient (HQ) were calculated using the following formulae:
where Cs (ng/g ww) represents the concentration of steroid hormones in the organism samples; Ms (g/(d·person)) is the consumption rate of seafood per person per day; Wp (kg bw/person) represents the average body weight of each age group. RfD is the reference dose for daily intake. ADI is the average daily intake. EDI is the ratio of the estimated daily intake.
The Mann–Whitney test and Pearson's correlation coefficients were conducted using SPSS (IBM SPSS Statistics) 22.0. Redundancy analysis was performed using Canoco5.0. CorelDRAW 2024, ArcGIS 10.8, and Origin 2021 were used to visualize the results.
Among the 44 steroid hormones analyzed, 16 were detected in seawater, with concentrations ranging from 0.01 ng/L (medroxyprogesterone) to 28.32 ng/L (5α-dihydroprogesterone) (Figure 2). The average concentrations of androgens, glucocorticoids, progestins and estrogens in seawater were 0.03, 0.01, 0.09, and 0.04 ng/L, respectively (Table 1). The concentrations of androgens, glucocorticoids, and progestins in seawater in this study are higher than those in the Pearl River Estuary (Chen et al., 2022; Xu et al., 2024), Beihai Bay of China (Ren et al., 2022), and the coast of Spain (Méndez-Catalán et al., 2024), but lower than those in the South China Sea (Liu et al., 2015). The concentrations of estrogens in seawater in this study are comparable to those observed in Halifax Harbour in Canada (Robinson et al., 2009), the coast of South Florida (Singh et al., 2009), and Chesapeake Bay in the USA (He et al., 2019), but significantly lower than those recorded in the central Venice Lagoon in Italy (Pojana et al., 2007) and Marmara Sea in Turkey (Korkmaz et al., 2022). Detailed comparisons of contaminant levels in various media from this study with those from previous studies are shown in Supplementary Table S4–S6.
Figure 2. Spatial distribution of steroid hormones in seawater (A), sediment (B), and marine organisms (C) along the coast of Guangdong. The mean concentration of each steroid hormone during the dry and rainy seasons was used to determine its spatial distribution in seawater. Different columns at the same sampling site were used to represent the concentrations of different types of marine organisms. ZHW, Zhenhai Bay; GHW, Guanghai Bay; ZJK, Pearl River Estuary; DPW, Dapeng Bay; DYW, Daya Bay; HHW, Honghai Bay; SQW, Shenquan Bay; GAW, Guangao Bay; STG, Shantou Port; ZLW, Zhelin Bay.
During the rainy and dry seasons, 11 and 14 steroid hormones were detected, respectively, with average concentrations of 0.02 and 0.09 ng/L, respectively. The detection ranges were 0.01 to 8.34 ng/L and 0.02 to 56.6 ng/L, respectively. 5α-dihydroprogesterone showed the highest concentrations in both seasons, although the concentration in the dry season was 6.8 times than that in the rainy season (Figure 3).
Figure 3. Seasonal distribution of steroid hormones in seawater. (A) Mean concentration of each steroid hormone in each bay; the left side of each bar group represents the rainy season, and the right side represents the dry season. (B) Mean concentration of each steroid hormone at all sampling sites during the dry and rainy seasons.
The concentrations of some other steroid hormones in seawater during the dry season were also significantly higher than those during the rainy season, including 4-androstene-3,17-dione, androsterone, 5α-dihydroprogesterone, and 17α-hydroxyprogesterone acetate. The detection rate of 4-androstene-3,17-dione was 95.0% in the dry season, but only 19.1% in the rainy season. This discrepancy is likely due to the higher precipitation and the resulting dilution effects during the rainy season. The average precipitation in Guangdong is 264 mm during the rainy season and 128 mm during the dry season (http://slt.gd.gov.cn/).
Among the 44 steroid hormones analyzed, 12 were detected in the sediment, among which progesterone and 4-androstene-3,17-dione showed the highest detection rates of approximately 73.5% and 51.5%, respectively. Concentration of each steroid hormone ranged from 0.03 ng/g (4-androstene-3,17-dione) to 4.56 ng/g (5α-dihydroprogesterone) (dw) (Figure 2). The average concentrations of androgens, progestins and estrogens in sediment were 0.01, 0.04, and 0.05 ng/g, respectively. Glucocorticoids were not detected in the present study. The mean concentrations followed the order of estrogens > progestins > androgens > glucocorticoids. These results are similar to those of a previous study that explored the fishing port environment along the southeastern coast of China (Liu et al., 2022). This pattern may be attributed to the fact that progestogens are expected to absorb more readily onto sediments than estrogens, androgens, and glucocorticoids, due to their significantly higher log Kow values ranging from 3.1 to 5.4 (Fent, 2015).The androgen levels are comparable to those reported in the Pearl River Estuary (Chen et al., 2022; Liu et al., 2023), Beihai Bay in China (Ren et al., 2022), and Hailing Bay in the South China Sea (Liu et al., 2015), but lower than those in the Marmara Sea in Turkey (Aysel and Yurdun, 2023). The levels of progestogens are higher than those reported in Pulau Kukup Johor, Malaysia (Ismail et al., 2020) but are two orders of magnitude lower than the levels in the Marmara Sea in Turkey (Aysel and Yurdun, 2023).The concentrations of estrogens are comparable to those in the Halifax harbor in Canada (Robinson et al., 2009) and Xiamen Bay in China (Zhang et al., 2009), but are lower than those in the Santos and São Vicente estuaries in Brazil (Pusceddu et al., 2019), Kuwait's coastal areas (Saeed et al., 2017), and the Venice lagoon in Italy (Pojana et al., 2007). Overall, steroid hormone concentrations in sediments from the coast of Guangdong are relatively low.
Among the 44 hormones analyzed, 27 were detected in marine organisms, with concentrations ranging from 0.01 ng/g (4-pregnene-17α,20β-diol-3-one) to 79.33 ng/g (cortisol) (ww) (Figure 2). The average concentrations of androgens, glucocorticoids, progestins and estrogens in marine organisms were 0.06, 0.71, 0.01, and 0.19 ng/g, respectively. The levels of androgens are slightly higher than those reported in the Pearl River Delta in China (Chen et al., 2022) and the Gulf of Gdańsk in Poland (Zabrzańska et al., 2015). The levels of glucocorticoids are significantly higher than those in the Klang River Estuary in Malaysia (Omar et al., 2019), primarily because of the high concentrations of cortisol. A previous study showed that cortisol levels in fish increase when they are exposed to environmental contaminants (Rohonczy et al., 2021), which may partly explain the high level of cortisol in the organisms in this study. The concentrations of progestogens are relatively high compared with those in the Po Delta in Italy and the Ebro Delta in Spain (Álvarez-Muñoz et al., 2015), but are lower than the concentrations measured in the Pearl River Delta, China (Chen et al., 2022). The concentrations of estrogens are higher than those reported in Malaysia's Klang River estuary (Omar et al., 2019) and Portugal's Tagus Estuary (Álvarez-Muñoz et al., 2015), but lower than those observed in Chesapeake Bay, USA (Álvarez-Muñoz et al., 2015), mariculture areas in the Pearl River Delta in China (Chen et al., 2022), and the Venice lagoon in Italy (Pojana et al., 2007).
The bioconcentration factors (BCFs) for 5α-dihydroprogesterone, 17α-hydroxyprogesterone acetate, and estrone were lower than 2000 L/kg (Figure 4), suggesting their low bioaccumulation potential in marine organisms. Additionally, desthystilbestrol is potentially bioaccumulative in marine organisms. The BCFs for 5α-dihydrotestosterone, cortisol, drospirenone, medroxyprogesterone, medroxyprogesterone acetate, and 4-pregnen-17α,20β-diol-3-one were higher than 5000 L/kg in most marine organisms, indicating that these compounds exhibit strong bioaccumulative potential. Among all species, Caranx formosanus, Sardinella melanura and Clupanodon thrissa exhibited stronger accumulation of steroid hormones. The bioaccumulation of steroid hormones in marine organisms can be attributed to various factors, including living habit, nutrient level, lipid content, metabolic capacity, sex, and life stage of the species, and lipophilicity and environmental concentration of steroid hormones (Ho et al., 2023; Liao et al., 2024).
Figure 4. Bioconcentration factor of steroid hormones in marine organisms along the coast of Guangdong. BCFs above 5000 mean significant bioaccumulation of the compound within the organism. BCFs between 2000 and 5000 suggest potential bioaccumulation. BCFs below 2000 indicate negligible bioaccumulation. The BCFs were calculated only for substances detected in both seawater and organisms.
The ecological risks posed by steroid hormones in the seawater along the coast of Guangdong are shown in Figure 5. 4-Androstene-3,17-dione, 5α-dihydrotestosterone, 4-hydroxyandrost-4-ene-17-dione, 17α-trenbolone, 17β-trenbolone, testosterone, dexamethasone, prednisolone, melengestrol acetate, 19-norethindrone, and 17β-estradiol posed no risk to aquatic life (RQ < 0.01). Progesterone posed low or insignificant risks (0.01 < RQ < 0.1). Cortisol presented medium risks (0.1 < RQ < 1). However, 17α-ethinylestradiol in Zhelin Bay indicated high risk (RQ > 1). Considering the detected concentrations, detection rates, and risk assessment results, progestins in the marine environment deserves greater attention in the future.
Figure 5. Ecological risks of steroid hormones in seawater along the coast of Guangdong. Darker colors indicate higher risk. The ecological risk assessment was conducted only for substances with available toxicological data. The PNECs used are presented in Supplementary Table S7.
The estimated daily intakes (EDIs) of steroid hormones in different populations are summarized in Supplementary Table S8. For male in the age groups of children, youths, and adults, the EDIs through fish consumption are 0.04 to 61.08, 0.06 to 79.51, and 0.02 to 30.93 ng/ (kg bw·d), respectively. For females in the same age groups, the EDIs are 0.03 to 38.37, 0.04 to 52.41, and 0.02 to 33.62 ng/ (kg bw·d), respectively. The acceptable daily intake (ADI) or reference dose (RfD) of only nine contaminants are available and used to calculate the hazard quotient (HQ) values (Supplementary Table S9). All HQ values are less than 1, indicating that there is no risk to human health from consuming these aquatic products from the coast of Guangdong.
As shown in Figure 2, the concentrations of steroid hormones in the seawater of Guanghai Bay were far greater than those in the other bays, followed by those in the Pearl River Estuary. In the sediment, the highest concentration of steroid hormones was detected in Zhelin Bay, followed by Guanghai Bay and the Pearl River Estuary. Among the marine organisms, the highest concentration of steroid hormones was detected in the Pearl River Estuary, followed by Daya Bay. In addition, an ecological risk assessment showed that 17α-ethinylestradiol in Zhelin Bay indicated high risk (RQ > 1). In summary, the concentrations of steroid hormones in Guanghai Bay, the Pearl River Estuary, Daya Bay, and Zhelin Bay were significantly higher than those in other bays.
The most polluted sites in the Pearl River Estuary were ZJK01 and ZJK02, which may be attributed to riverine input from the Pearl River. Data suggest that the Pearl River drains approximately 320 billion m3 per year of surface runoff into the Pearl River Estuary (Zhao et al., 2019). Steroid hormones have been detected in surface waters, sediment, and suspended particulate matter from the Pearl River system up to 78.7 ng/L, 38.0 ng/g, and 33.1 ng/g, respectively (Zhao et al., 2011; Gong et al., 2023). Furthermore, higher concentrations of steroid hormones were observed at ZJK13, ZJK15, ZJK17, and ZJK18, which are located near WWTPs and densely populated areas of Zhuhai. This observation is in agreement with the results of previous study (Xu et al., 2024).
Guanghai Bay experiences substantial discharge pressure from the Pearl River (Gu et al., 2024). Seawater samples collected in Guanghai Bay at sites GHW05 and GHW06 exhibited higher steroid hormone concentrations, which may be due to their location at the mouth of the bay where the Pearl River Estuary tidal current enters Guanghai Bay. The high concentration detected in seawater is due to wave action and increased water flow, which causes pollutants adsorbed in sediments to be released back into seawater (Wang et al., 2022; Gu et al., 2024).
Sampling site DYW01 is located near aquaculture and agricultural areas. Studies have shown that the feces of livestock and poultry are commonly used for agricultural fertilization (Lin et al., 2024), and artificial addition of steroid hormones also occurs in aquaculture (Liu et al., 2015, 2024). Thus, agricultural runoff and aquaculture breeding wastes are important contributors to the elevated concentrations of steroid hormones in Daya Bay (Lin et al., 2024).
Zhelin Bay is the largest marine cage culture base in China, and has become one of the most densely populated areas for marine aquaculture (Xia et al., 2017), which may account for the high concentrations of steroid hormones (Li et al., 2021). The occurrence of steroid hormones in sediments is associated with direct dosing of steroid hormones and feeding with medicated feed (Xie et al., 2019). Studies have shown that the concentration of steroid hormones detected in sediments of marine aquaculture areas is significantly higher than other areas (Lu et al., 2020; Chen et al., 2022).
The influence of human and environmental variables on steroid distribution was analyzed using redundancy analysis (RDA) (Supplementary Table S10). The results indicated that domestic water consumption of urban residents was the most significant anthropogenic factor affecting steroid levels.
In order to investigate the potential sources of steroid hormones identified in the coastal areas of Guangdong, the correlations between steroid hormone concentrations and environmental parameters were analyzed (Figure 6, Supplementary Table S11-S12). Pearson correlation analysis demonstrated that many steroid hormones were significantly correlated with aqueous physicochemical parameters, such as salinity, chemical oxygen demand (COD), dissolved oxygen (DO), total nitrogen (TN), total phosphorus (TP), inorganic nitrogen (IN), suspended solid (SS), and chlorophyll a (Chl a) (Figure 6A). A negative correlation was observed between 4-androstene-3,17-dione, cortisol, prednisone, norethisterone acetate, diethylstilbestrol, and medroxyprogesterone concentrations and salinity (p < 0.05), indicating a potential continental input. Estrone was positively correlated with Chl a, which is consistent with the findings of a previous study on the Pearl River Delta (Deich et al., 2021). These correlations suggest common sources of steroid hormones and nitrogen and phosphorus, such as wastewater discharge, agriculture, and aquaculture industry (Yi et al., 2019; Li et al., 2021). Dydrogesterone exhibited a positive and statistically significant correlation with 5α-dihydroprogesterone and progesterone, indicating a potential common origin for these compounds. Dydrogesterone, 5α-dihydroprogesterone, and progesterone have been detected in swine wastewater, swine feces, and effluents from WWTPs (Liu et al., 2020). 5α-dihydroprogesterone and progesterone have been detected in feed and sediment samples from freshwater aquaculture ponds (Liu et al., 2024). Therefore, steroid hormones in seawater may originate from aquaculture waste, wastewater discharge, livestock production, and agricultural surface runoff. These results suggest a diversity of steroid hormone sources along the coast of Guangdong.
Figure 6. Pearson correlation coefficients between steroid hormone concentrations and environmental parameters in seawater (A) and sediment (B). Blue indicates a negative correlation, whereas red indicates a positive correlation. Larger circles and darker colors indicate stronger correlations. The number represents the correlation coefficient, with larger values signifying a higher correlation. COD, chemical oxygen demand; DO, dissolved oxygen; TN, total nitrogen; TP, total phosphorus; IN, inorganic nitrogen; SS, suspended solid; Chl a, chlorophyll a; TOC, total organic carbon.
Pearson correlation analysis showed significant correlations between many steroid hormones and particle size, salinity, oil pollutants, heavy metals, and total organic carbon (TOC) (Figure 6B). Salinity was negatively correlated with testosterone in the sediment in the present study, suggesting that terrestrial input may be an important source. TOC was positively correlated with testosterone in the sediment, as a higher organic matter content has been reported to have a higher sorption affinity (Qi and Zhang, 2016). Particle size exhibited significant positive relationships with 5α-dihydroprogesterone, progesterone, and 17β-estradiol, which agrees with a previous study (Sangster et al., 2015), and may be due to the combined effects of cation exchange capacity, percentage of organic carbon, and surface area. Oil pollutants positively correlated with 19-nortestosterone, testosterone, and progesteron. Progesterone positively correlated with 4-androstene-3,17-dione, 19-nortestosterone, 5α-dihydroprogesterone, estrone, and 17β-estradiol, suggesting common sources. In addition, Pb was positively correlated with testosterone in the sediment.
This study measured the levels of 44 steroid hormones in seawater, sediments, and organisms from the coast of Guangdong. Total hormone concentrations ranged from 0.11 to 30.15 ng/L in seawater, ND to 8.58 ng/g (dw) in sediments, and ND to 80.52 ng/g (ww) in organisms. Compared to other coastal areas, the coastal region of Guangdong showed lower concentrations of steroid hormones in seawater and sediment, while higher levels in marine organisms. The concentrations of steroid hormones in Guanghai Bay, the Pearl River Estuary, Daya Bay, and Zhelin Bay were significantly higher than those in other bays. Steroid hormone concentrations in seawater were higher during the dry season than the rainy season. Ecological risk assessment results indicated that 17α-ethinylestradiol in Zhelin Bay posed high risks. However, no health risks were associated with the consumption of seafood from the coast of the Guangdong. Overall, our study revealed the occurrence of steroid hormones in the marine environment, which is essential for management of steroid hormone pollution.
However, the ecological risk assessment results in this study cannot fully reflect the risk posed by steroid hormones on Guangdong's coastline, as toxicological data for some compounds are absent. Further research is warranted to evaluate the risks associated with steroid hormones in nearshore marine environments.
The original contributions presented in the study are included in the article/Supplementary Material. Further inquiries can be directed to the corresponding author.
XZ: Data curation, Investigation, Visualization, Writing – original draft. YLJ: Investigation, Writing – original draft, Methodology. HD: Investigation, Writing – original draft. YL: Investigation, Writing – original draft. SL: Supervision, Writing – review & editing. YXJ: Supervision, Writing – review & editing, Funding acquisition, Project administration. KS: Supervision, Writing – review & editing.
The author(s) declare financial support was received for the research, authorship, and/or publication of this article. This research was supported by the Guangzhou Municipal Science and Technology Bureau (No. 2023A04J0947), the National Natural Science of China (42107309), and the Fundamental Research Funds for the Central Public Welfare Research Institutes (PM-zx703-202104-068).
The authors declare that the research was conducted in the absence of any commercial or financial relationships that could be construed as a potential conflict of interest.
The author(s) declare that no Generative AI was used in the creation of this manuscript.
All claims expressed in this article are solely those of the authors and do not necessarily represent those of their affiliated organizations, or those of the publisher, the editors and the reviewers. Any product that may be evaluated in this article, or claim that may be made by its manufacturer, is not guaranteed or endorsed by the publisher.
The Supplementary Material for this article can be found online at: https://www.frontiersin.org/articles/10.3389/fmars.2025.1546186/full#supplementary-material
Adeel M., Song X., Wang Y., Francis D., Yang Y. (2017). Environmental impact of estrogens on human, animal and plant life: A critical review. Environ. Int. 99, 107–119. doi: 10.1016/j.envint.2016.12.010
Álvarez-Muñoz D., Rodríguez-Mozaz S., Maulvault A. L., Tediosi A., Fernández-Tejedor M., Van den Heuvel F., et al. (2015). Occurrence of pharmaceuticals and endocrine disrupting compounds in macroalgaes, bivalves, and fish from coastal areas in Europe. Environ. Res. 143, 56–64. doi: 10.1016/j.envres.2015.09.018
Aris A. Z., Shamsuddin A. S., Praveena S. M. (2014). Occurrence of 17α-ethynylestradiol (EE2) in the environment and effect on exposed biota: a review. Environ. Int. 69, 104–119. doi: 10.1016/j.envint.2014.04.011
Aysel E., Yurdun T. (2023). Analysis of selected steroid hormones in sea of Marmara sediment samples by LC-ESI/MS-MS. Istanbul J. Pharm 53, 329+. doi: 10.26650/IstanbulJPharm.2023.1277041
Chen Y., Xie H., Junaid M., Xu N., Zhu Y., Tao H., et al. (2022). Spatiotemporal distribution, source apportionment and risk assessment of typical hormones and phenolic endocrine disrupting chemicals in environmental and biological samples from the mariculture areas in the Pearl River Delta, China. Sci. Total Environ. 807, 150752. doi: 10.1016/j.scitotenv.2021.150752
Deich C., Frazão H. C., Appelt J. S., Li W., Pohlmann T., Waniek J. J. (2021). Occurrence and distribution of estrogenic substances in the northern South China Sea. Sci. Total Environ. 770, 145239. doi: 10.1016/j.scitotenv.2021.145239
DeQuattro Z. A., Hemming J. D. C., Barry T. P. (2015). Effects of androstenedione exposure on fathead minnow (Pimephales promelas) reproduction and embryonic development. Environ. Toxicol. Chem. 34, 2549–2554. doi: 10.1002/etc.3092
Dong Z., Chen Y., Li X., Zhang N., Guo Y., Liang Y., et al. (2022). Norethindrone alters growth, sex differentiation and gene expression in marine medaka (Oryzias melastigma). Environ. Toxicol. 37, 1211–1221. doi: 10.1002/tox.23477
Fent K. (2015). Progestins as endocrine disrupters in aquatic ecosystems: Concentrations, effects and risk assessment. Environ. Int. 84, 115–130. doi: 10.1016/j.envint.2015.06.012
Frankel T. E., Meyer M. T., Orlando E. F. (2016). Aqueous exposure to the progestin, levonorgestrel, alters anal fin development and reproductive behavior in the eastern mosquitofish (Gambusia holbrooki). Gen. Comp. Endocrinol. 234, 161–169. doi: 10.1016/j.ygcen.2016.01.007
Gong J., Zhou Y., Lin C., Li Q., Han C., Yang K., et al. (2023). Suspended particulate matter-associated environmental corticosteroids in the Pearl River, China: Occurrence, distribution, and partitioning. Sci. Total Environ. 884, 163701. doi: 10.1016/j.scitotenv.2023.163701
Gu H., Wu M., Zhao F., Wang Z., Ni Z., Li D., et al. (2024). Using multiple radionuclides to trace water and particle dynamics in Guanghai Bay, Western Guangdong. Atomic Energy Sci. Technol. 58, 2032–2044. doi: 10.7538/yzk.2024.youxian.0184
He K., Hain E., Timm A., Tarnowski M., Blaney L. (2019). Occurrence of antibiotics, estrogenic hormones, and UV-filters in water, sediment, and oyster tissue from the Chesapeake Bay. Sci. Total Environ. 650, 3101–3109. doi: 10.1016/j.scitotenv.2018.10.021
Ho Q. T., Frantzen S., Nilsen B. M., Nøstbakken O. J., Azad A. M., Duinker A., et al. (2023). Congener-specific accumulation of persistent organic pollutants in marine fish from the Northeast Atlantic Ocean. J. Hazardous Materials 457, 131758. doi: 10.1016/j.jhazmat.2023.131758
Huanyu T., Jianghong S., Wei G., Jiawei Z., Hui G., Yunhe W. (2022). Environmental fate and toxicity of androgens: A critical review. Environ. Res. 214, 113849. doi: 10.1016/j.envres.2022.113849
Islam R., Kit Yu R. M., O'Connor W. A., Anh Tran T. K., Andrew-Priestley M., Leusch F. D. L., et al. (2020). Parental exposure to the synthetic estrogen 17α-ethinylestradiol (EE2) affects offspring development in the Sydney rock oyster, Saccostrea glomerata. Environ. pollut. (Barking Essex 1987) 266, 114994. doi: 10.1016/j.envpol.2020.114994
Ismail N. A. H., Wee S. Y., Haron D. E. M., Kamarulzaman N. H., Aris A. Z. (2020). Occurrence of endocrine disrupting compounds in mariculture sediment of Pulau Kukup, Johor, Malaysia. Mar. pollut. Bull. 150, 110735. doi: 10.1016/j.marpolbul.2019.110735
Ismanto A., Hadibarata T., Kristanti R. A., Maslukah L., Safinatunnajah N., Kusumastuti W. (2022). Endocrine disrupting chemicals (EDCs) in environmental matrices: Occurrence, fate, health impact, physio-chemical and bioremediation technology. Environ. pollut. (Barking Essex 1987) 302, 119061. doi: 10.1016/j.envpol.2022.119061
Korkmaz N. E., Savun-Hekimoğlu B., Aksu A., Burak S., Caglar N. B. (2022). Occurrence, sources and environmental risk assessment of pharmaceuticals in the Sea of Marmara, Turkey. Sci. Total Environ. 819, 152996. doi: 10.1016/j.scitotenv.2022.152996
Li W., Zhang Z., Zhang R., Sun A., Lu Y., Chen J., et al. (2021). Spatiotemporal occurrence, sources and risk assessment of polycyclic aromatic hydrocarbons in a typical mariculture ecosystem. Water Res. 204, 117632. doi: 10.1016/j.watres.2021.117632
Liao Z., Jian Y., Lu J., Liu Y., Li Q., Deng X., et al. (2024). Distribution, migration patterns, and food chain human health risks of endocrine-disrupting chemicals in water, sediments, and fish in the Xiangjiang River. Sci. Total Environ. 930, 172484. doi: 10.1016/j.scitotenv.2024.172484
Lin H., Zhou L., Lu S., Yang H., Li Y., Yang X. (2024). Occurrence and spatiotemporal distribution of natural and synthetic steroid hormones in soil, water, and sediment systems in suburban agricultural area of Guangzhou City, China. J. Hazardous Materials 470, 134288. doi: 10.1016/j.jhazmat.2024.134288
Liu S., Chen J., Zhang J., Liu Y., Hu L., Chen X., et al. (2020). Microbial transformation of progesterone and dydrogesterone by bacteria from swine wastewater: Degradation kinetics and products identification. Sci. Total Environ. 701, 134930. doi: 10.1016/j.scitotenv.2019.134930
Liu S., Chen H., Zhou G., Liu S., Yue W., Yu S., et al. (2015). Occurrence, source analysis and risk assessment of androgens, glucocorticoids and progestagens in the Hailing Bay region, South China Sea. Sci. Total Environ. 536, 99–107. doi: 10.1016/j.scitotenv.2015.07.028
Liu S., Li Y., Ning J., Xu L., Wang L., Huang D., et al. (2024). Occurrence, bioaccumulation, and potential risks of steroid hormones in freshwater aquaculture ponds in South China. Water 16, 2872. doi: 10.3390/w16202872
Liu S., Tian F., Pan Y., Li H., Lin L., Hou R., et al. (2022). Contamination and ecological risks of steroid metabolites require more attention in the environment: Evidence from the fishing ports. Sci. Total Environ. 807, 150814. doi: 10.1016/j.scitotenv.2021.150814
Liu S., Xu R., Pan Y., Huang Q., Wu N., Li H., et al. (2023). Free and conjugated forms of metabolites are indispensable components of steroids: The first evidence from an estuarine food web. Water Res. 235, 119913. doi: 10.1016/j.watres.2023.119913
Lu J., Zhang C., Wu J., Zhang Y., Lin Y. (2020). Seasonal distribution, risks, and sources of endocrine disrupting chemicals in coastal waters: Will these emerging contaminants pose potential risks in marine environment at continental-scale? Chemosphere 247, 125907. doi: 10.1016/j.chemosphere.2020.125907
Méndez-Catalán J., Socas-Hernández C., Jiménez-Skrzypek G., Hernández-Borges J., González-Sálamo J. (2024). Evaluation of the presence of emerging contaminants in wastewater and seawater using automated solid-phase extraction and ultra-high-performance liquid chromatography coupled to tandem mass spectrometry. J. Chromatogr. Open 6, 100178. doi: 10.1016/j.jcoa.2024.100178
Ojoghoro J. O., Scrimshaw M. D., Sumpter J. P. (2021). Steroid hormones in the aquatic environment. Sci. Total Environ. 792, 148306. doi: 10.1016/j.scitotenv.2021.148306
Omar T. F. T., Aris A. Z., Yusoff F. M., Mustafa S. (2019). Occurrence and level of emerging organic contaminant in fish and mollusk from Klang River estuary, Malaysia and assessment on human health risk. Environ. pollut. 248, 763–773. doi: 10.1016/j.envpol.2019.02.060
Pojana G., Gomiero A., Jonkers N., Marcomini A. (2007). Natural and synthetic endocrine disrupting compounds (EDCs) in water, sediment and biota of a coastal lagoon. Environ. Int. 33, 929–936. doi: 10.1016/j.envint.2007.05.003
Pusceddu F. H., Sugauara L. E., de Marchi M. R., Choueri R. B., Castro Í.B. (2019). Estrogen levels in surface sediments from a multi-impacted Brazilian estuarine system. Mar. pollut. Bull. 142, 576–580. doi: 10.1016/j.marpolbul.2019.03.052
Qi Y., Zhang T. C. (2016). Sorption of testosterone on partially-dispersed soil particles of different size fractions: Methodology and implications. Water Res. 92, 1–10. doi: 10.1016/j.watres.2016.01.036
Ren C., Tan X., Huang C., Zhao H., Lan W. (2022). Sources, pollution characteristics, and ecological risk assessment of steroids in Beihai Bay, Guangxi. Water 14, 1399. doi: 10.3390/w14091399
Robinson B. J., Hui J. P. M., Soo E. C., Hellou J. (2009). Estrogenic compounds in seawater and sediment from Halifax Harbour, Nova Scotia, Canada. Environ. Toxicol. Chem. 28, 18–25. doi: 10.1897/08-203.1
Rohonczy J., O'Dwyer K., Rochette A., Robinson S. A., Forbes M. R. (2021). Meta-analysis shows environmental contaminants elevate cortisol levels in teleost fish – Effect sizes depend on contaminant class and duration of experimental exposure. Sci. Total Environ. 800, 149402. doi: 10.1016/j.scitotenv.2021.149402
Saeed T., Al-Jandal N., Abusam A., Taqi H., Al-Khabbaz A., Zafar J. (2017). Sources and levels of endocrine disrupting compounds (EDCs) in Kuwait's coastal areas. Mar. pollut. Bull. 118, 407–412. doi: 10.1016/j.marpolbul.2017.03.010
Sangster J. L., Oke H., Zhang Y., Bartelt-Hunt S. L. (2015). The effect of particle size on sorption of estrogens, androgens and progestagens in aquatic sediment. J. Hazardous Materials 299, 112–121. doi: 10.1016/j.jhazmat.2015.05.046
Singh S. P., Azua A., Chaudhary A., Khan S., Willett K. L., Gardinali P. R. (2009). Occurrence and distribution of steroids, hormones and selected pharmaceuticals in South Florida coastal environments. Ecotoxicology 19, 338–350. doi: 10.1007/s10646-009-0416-0
Streck G. (2009). Chemical and biological analysis of estrogenic, progestagenic and androgenic steroids in the environment. TrAC Trends Analytical Chem. 28, 635–652. doi: 10.1016/j.trac.2009.03.006
Wang A., Wu X., Bi N., Ralston D. K., Wang C., Wang H. (2022). Combined effects of waves and tides on bottom sediment resuspension in the southern Yellow Sea. Mar. Geol. 452, 106892. doi: 10.1016/j.margeo.2022.106892
Xia L., Liu Z., Xu S. (2017). Estimation on pollution load and analysis on spatial characteristics of mariculture in Zhelin Bay. Guangdong Agric. Sci. 44, 151–157. doi: 10.16768/j.issn.1004-874X.2017.12.023
Xie H., Hao H., Xu N., Liang X., Gao D., Xu Y., et al. (2019). Pharmaceuticals and personal care products in water, sediments, aquatic organisms, and fish feeds in the Pearl River Delta: Occurrence, distribution, potential sources, and health risk assessment. Sci. total Environ. 659, 230–239. doi: 10.1016/j.scitotenv.2018.12.222
Xu R., Liu S., Pan Y., Wu N., Huang Q., Li H., et al. (2024). Steroid metabolites as overlooked emerging contaminants: Insights from multimedia partitioning and source–sink simulation in an estuarine environment. J. Hazardous Materials 461, 132673. doi: 10.1016/j.jhazmat.2023.132673
Yazdan M. M. S., Kumar R., Leung S. W. (2022). The environmental and health impacts of steroids and hormones in wastewater effluent, as well as existing removal technologies: A review. Ecologies 3, 206–224. doi: 10.3390/ecologies3020016
Yi X., Zhang C., Liu H., Wu R., Tian D., Ruan J., et al. (2019). Occurrence and distribution of neonicotinoid insecticides in surface water and sediment of the Guangzhou section of the Pearl River, South China. Environ. pollut. (Barking Essex 1987) 251, 892–900. doi: 10.1016/j.envpol.2019.05.062
Zabrzańska S., Smolarz K., Hallmann A., Konieczna L., Bączek T., Wołowicz M. (2015). Sex-related differences in steroid concentrations in the blue mussel (Mytilus edulis trossulus) from the southern Baltic Sea. Comp. Biochem. Physiol. Part A: Mol. Integr. Physiol. 183, 14–19. doi: 10.1016/j.cbpa.2014.12.029
Zhang X., Li Q., Li G., Wang Z., Yan C. (2009). Levels of estrogenic compounds in Xiamen Bay sediment, China. Mar. pollut. Bull. 58, 1210–1216. doi: 10.1016/j.marpolbul.2009.03.011
Zhang J., Ying G., Yang Y., Liu W., Liu S., Chen J., et al. (2018). Occurrence, fate and risk assessment of androgens in ten wastewater treatment plants and receiving rivers of South China. Chemosphere 201, 644–654. doi: 10.1016/j.chemosphere.2018.02.144
Zhao X., Qiu W., Zheng Y., Xiong J., Gao C., Hu S. (2019). Occurrence, distribution, bioaccumulation, and ecological risk of bisphenol analogues, parabens and their metabolites in the Pearl River Estuary, South China. Ecotoxicol. Environ. Saf. 180, 43–52. doi: 10.1016/j.ecoenv.2019.04.083
Zhao J., Ying G., Chen F., Liu Y., Wang L., Yang B., et al. (2011). Estrogenic activity profiles and risks in surface waters and sediments of the Pearl River system in South China assessed by chemical analysis and in vitro bioassay. J. Environ. Monit. 13, 813–821. doi: 10.1039/C0EM00473A
Keywords: steroid hormones, risk assessment, spatial distribution, bioaccumulation, coastal areas
Citation: Zhao X, Jiang Y, Deng H, Lu Y, Li S, Jiang Y and Sun K (2025) Spatiotemporal occurrence, distribution, and risk of steroid hormones along the coast of Guangdong, China. Front. Mar. Sci. 12:1546186. doi: 10.3389/fmars.2025.1546186
Received: 16 December 2024; Accepted: 15 January 2025;
Published: 03 February 2025.
Edited by:
Xiaowei Zheng, Shanghai Jiao Tong University, ChinaReviewed by:
Xian Sun, Sun Yat-sen University, ChinaCopyright © 2025 Zhao, Jiang, Deng, Lu, Li, Jiang and Sun. This is an open-access article distributed under the terms of the Creative Commons Attribution License (CC BY). The use, distribution or reproduction in other forums is permitted, provided the original author(s) and the copyright owner(s) are credited and that the original publication in this journal is cited, in accordance with accepted academic practice. No use, distribution or reproduction is permitted which does not comply with these terms.
*Correspondence: Yuxia Jiang, amlhbmd5dXhpYTNAZm94bWFpbC5jb20=
Disclaimer: All claims expressed in this article are solely those of the authors and do not necessarily represent those of their affiliated organizations, or those of the publisher, the editors and the reviewers. Any product that may be evaluated in this article or claim that may be made by its manufacturer is not guaranteed or endorsed by the publisher.
Research integrity at Frontiers
Learn more about the work of our research integrity team to safeguard the quality of each article we publish.