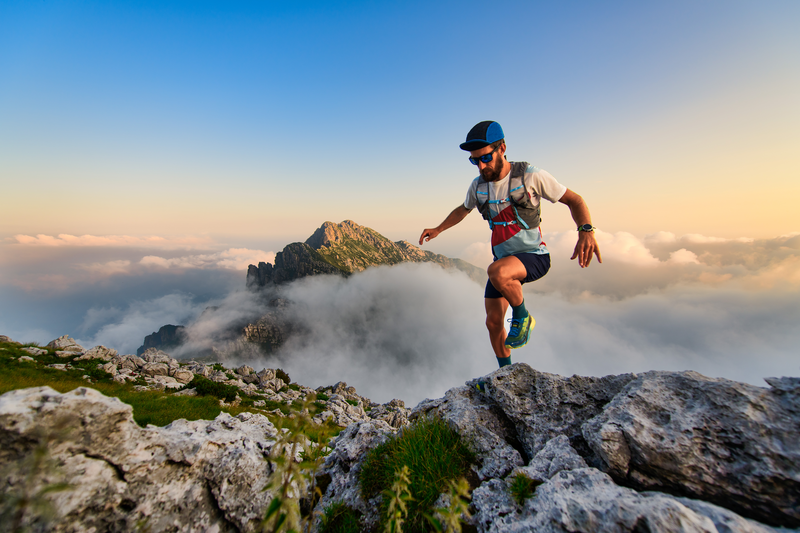
95% of researchers rate our articles as excellent or good
Learn more about the work of our research integrity team to safeguard the quality of each article we publish.
Find out more
ORIGINAL RESEARCH article
Front. Mar. Sci. , 10 February 2025
Sec. Deep-Sea Environments and Ecology
Volume 12 - 2025 | https://doi.org/10.3389/fmars.2025.1508160
In the oceans, ecological analyses of pelagic amphipods have mainly focused on the epipelagic zone with few studies in the deep waters. In this study, a coarse-scale vertical analysis, between 0 and 1000 m depth, was performed in the southern Gulf of Mexico during summer and winter. We hypothesize greater differences between the epi-and mesopelagic zones during the summer, because of a stronger vertical gradient in environmental conditions, especially temperature. As well, we think that the zooplankton biomass (as a measure of food availability) will play a significant role in regulating the amphipod distribution and abundance. Zooplankton samples were obtained at five levels (0-200, 200-400, 400-600, 600-800, 800-1000 m) of the water column using a stratified net system during the winter of 2013 and summer of 2014 in the southern Gulf of Mexico. To probe vertical differences, the amphipod community was analyzed considering two assemblages defined a priori, the ‘epipelagic’ and the ‘mesopelagic’; and considering each of the five sampling levels as separate groups. Results indicated that assemblages were significantly different in both seasons (ANOSIM test, p < 0.05), but differences were stronger in winter, which contradicts the first hypothesis. The vertical hydrological structure during the summer was characterized by a deepening of 15-18°C temperature values towards the upper mesopelagic zone, resulting in less heterogeneity between the epi- and the mesopelagic zones. A BEST-BIOENV test was used to evaluate the degree of association between the environmental (temperature, salinity, zooplankton biomass) and biological (amphipod composition and density) matrices. As expected, the zooplankton biomass was the most important factor affecting the distribution of the amphipods, especially during the summer (rho = 0.319, p = 0.001). The dominant species was Lestrigonus bengalensis in winter and the juveniles of the genus Primno in summer. The SIMPER analysis also showed these taxa as responsible for the discrimination of the epi- and mesopelagic assemblages. In a finer analysis taking the sampling levels as a factor, results indicated that, during the summer, the 200-400 m level showed a differentiation from the other deep levels; again, the effect of the deepening of temperature values between 15 and 18°C, could be the responsible. Comparisons of day/night sampling time in the average amphipod abundance indicated that only the members of the infraorder Physosomata showed significant differences during the summer (ANOSIM test, p < 0.05), which could be indicative of a migratory process. The diversity of the assemblages in both seasons was analyzed using alpha diversity species accumulation curves and a completeness analysis, using the sampling coverage. Seasonally, the summer was more diverse, while in the vertical plane, the mesopelagic zone was more diverse than the epipelagic one. We suggest further studies in the poorly sampled mesopelagic zone of the ocean to better understand the ecology of the deep-sea pelagic amphipods.
The order Amphipoda is a group of crustaceans present throughout the world, as they are found in all types of freshwater, estuarine, and marine environments (Vinogradov, 1999; LeCroy et al., 2009). In the marine environment, some species of amphipods are found suspended their entire life in the water column as plankton or in association with gelatinous zooplankters (Vinogradov et al., 1996). In the pelagic system, amphipods occur in specific depth ranges, or even as eurybathic species inhabiting a wide range of depths from the surface waters of the epipelagic layer to the deep-sea in the meso-, bathy-, and abyssopelagic zones (Thurston, 1976a, 1976b; Vinogradov et al., 1996; Lowry and Stoddart, 1997; Hughes and Lowry, 2015). Pelagic amphipods are mostly carnivores and eat a variety of zooplankton organisms such as copepods, other small crustaceans, or tissues of their hosts, the gelatinous organisms (Mazda et al., 2019; Espinosa-Leal et al., 2020) so, as part of the zooplankton, amphipods play an important role at the base of the pelagic food webs. Field observations at several spatial scales showed that the amphipods tend to inhabit highly productive areas (Gasca, 2004; Hereu et al., 2020; Domínguez-Nava et al., 2021). Indeed, it has been suggested that food availability positively impacts the abundance of amphipods (Sampaio de Souza et al., 2016; Violante-Huerta, 2019).
Pelagic amphipods comprise three suborders: Amphilochidea, Hyperiidea, and Senticaudata. The Hyperiidea, in particular those of the infraorder Physocephalata, have received much attention from researchers due to their high diversity and abundance in the epipelagic zone of the oceans (Vinogradov et al., 1996; Vinogradov, 1999; Lavaniegos, 2020). In contrast, the hyperiid amphipods of the infraorder Physosomata and non-hyperiids mostly inhabit the deep-sea (Vinogradov et al., 1996; Lowry and Stoddart, 1997; Hughes and Lowry, 2015; Violante-Huerta et al., 2020), which makes its ecological study difficult.
Most species of pelagic amphipods have a cosmopolitan or circumtropical distribution, except for those with a restricted distribution in the polar regions (Vinogradov et al., 1996; Vinogradov, 1999; Zeidler and De Broyer, 2009; Minutoli et al., 2023). Generally, their ecological studies have been focused mainly on intermediate spatial scales in the horizontal plane. The largest oceanic area studied to date is between 39° N and 45° S, in the Atlantic Ocean. In this area, the examination of species diversity and distribution allowed the identification of amphipod assemblages whose limits coincided with the biogeographic regions of the Atlantic Ocean (Burridge et al., 2016). At smaller spatial scales, some authors have observed that the horizontal distribution of pelagic amphipods was defined by mesoscale phenomena, such as ocean gyres and currents, temperature gradients, variations in productivity, upwellings, and the presence of gelatinous plankton (Gasca et al., 2009; Lavaniegos and Hereu, 2009; Valencia et al., 2013; Lavaniegos, 2014, 2020; Zhang et al., 2014; Sampaio de Souza et al., 2016; Espinosa-Leal et al., 2021). The degree to which these processes affect the amphipods depends largely on temporality, evidenced by seasonal or interannual changes in the community structure and distribution (Gorbatenko et al., 2017; Lavaniegos, 2020; Wang et al., 2020). In the horizontal plane, pelagic amphipods display a lower abundance and higher diversity in the oceanic zone in comparison to the neritic waters (Vinogradov, 1999; Gasca, 2004). Regarding the vertical plane, the studies show that the amphipods are more abundant in the epipelagic zone than the waters below 200 m (Thurston, 1976a, 1976b; Roe et al., 1984). However, the daytime collection schedule is also an important variable to detect the differences in abundance in the epipelagic zone (Shulenberger, 1977, 1978; Cornet and Gili, 1993; Pai et al., 2010), due to the migratory behavior of some amphipod species.
In Mexico, mesoscale studies have analyzed the relationship between physical factors and amphipod assemblages in epipelagic waters of the Pacific (Lavaniegos and Hereu, 2009; Lavaniegos, 2014, 2017, 2020) and Atlantic Oceans (Gasca, 2003a, 2009; Gasca et al., 2009; Domínguez-Nava et al., 2021; Sanvicente-Añorve et al., 2023). However, those studies considered only the horizontal plane, except for Domínguez-Nava et al. (2021), who analyzed fine-scale vertical distribution (0-100 m depth) of hyperiid amphipods in the Mexican Caribbean. Therefore, there is a need to analyze the assemblages of pelagic amphipods in the vertical plane at a larger spatial scale, such as a coarse-scale (100 m to 100 km), to explore the distribution and diversity of this important zooplankton group in the deep sea, particularly in the mesopelagic zone. Here, we document a coarse-scale vertical analysis of the structural changes of the pelagic amphipod assemblages in the southern Gulf of Mexico, considering the main differences between epipelagic and mesopelagic zones during two contrasting seasons, winter and summer. We hypothesize greater differences between the epi-and mesopelagic zones during the summer, because of a strong gradient in environmental conditions in the water column, especially temperature. As well, we think that the zooplankton biomass (as a measure of food availability) will be a key factor in controlling the amphipod distribution and abundance.
Zooplankton sampling was carried out in the oceanic province of the southern Gulf of Mexico aboard the R/V “Justo Sierra” in two contrasting seasons, winter 2013 (24 oceanographic stations from January 25 to February 3) and summer 2014 (31stations from June 4 to 14) (Figure 1; Supplementary Table S1). At each station, temperature and salinity were recorded before the zooplankton sampling with a CTD sonde (Sea-Bird SBE 9) from the surface to 1000 m depth. Zooplankton samples were obtained using a stratified cylinder-conical zooplankton multinet system (nylon, 75 cm mouth, 2 m length, and 500 µm mesh size); this system consisted of a Double -Trip Mechanism (General Oceanics 1000-DT), whose purpose is the opening and closure of plankton nets. At each oceanographic station, samples were obtained at five depth levels of the water column: 0–200 m; 200–400 m; 400–600 m; 600–800 m; and 800–1000 m, depending on bottom depth. Sampling was performed for approximately 25 minutes following double oblique tows at a speed of approximately 3 knots; each net was equipped with a standard mechanical flowmeter (General Oceanics 2030R) to calculate the filtered water. The oceanographic stations were arranged in five longitudinal transects for each season (transects Bw to Fw in winter, and As to Es in summer), but their latitudinal positions slightly differed (Figure 1). The time of day (day/night) at which each sampling was carried out was recorded. A total of 255 samples were obtained from which 104 were taken in winter and 151 in summer. Zooplankton samples were fixed in a 4% formalin-seawater solution and, posteriorly, preserved in 70% ethanol because of the objectives of the project, concerning one, the study of fish larvae growth throughout the analysis of otoliths, which are best preserved in alcohol (Campana, 1989).
Figure 1. Sampling stations located along five transects during winter 2013 (Bw-Fw) and summer 2014 (As-Es) in the southern Gulf of Mexico.
In the laboratory, the zooplankton biomass of each sample was estimated by the displacement volume method and data were expressed as mL/1000 m3 (Postel et al., 2000). Besides, all the amphipods were sorted from samples identified by performing microdissections of the structures of taxonomical interest under a stereoscopic microscope (Leica M80), following specialized literature (Vinogradov et al., 1996; Vinogradov, 1999; Zeidler, 2003a, 2003b, 2004a, 2004b, 2006, 2009, 2016; Hughes and Lowry, 2015; Violante-Huerta et al., 2020). The abundance data of each sample were standardized to 1000 m3 of filtered water (ind/1000 m3).
The hydrological conditions in each sampling season were characterized by the use of θ-SA diagrams. To create these diagrams, conservative temperature (θ) and absolute salinity (SA) values were calculated following the TEOS-10 equation (McDougall and Barker, 2011). The water masses were identified in the θ-SA diagrams according to the latest classification carried out for the Gulf of Mexico (Cervantes-Díaz et al., 2022). Water masses were located in the vertical plane by plotting longitudinal transects of the density (Sigma) to make comparisons with the temperature variations. Because greater variability was found at the first two levels, only values from 0 to 400 m depth were plotted. This analysis was done using Ocean Data View v5.6.5 software. We also calculated the mean integrated value of each parameter at each sampling level to explore their association with the biological data.
For each sampling season, the species/stations matrix was transformed by applying a square root to smooth the bias of dominant species, and after that, the Bray-Curtis similarity index was applied. Then, to probe the differences in the vertical plane, the amphipod community was analyzed from two points of view: first, considering two assemblages defined a priori, the ‘epipelagic’ and the ‘mesopelagic’; and second, considering each of the five sampling levels as separate groups.
The ‘epipelagic’ assemblage was represented by the first sampling level of 0-200 m depth, and the ‘mesopelagic’ assemblage was composed by the four sampling levels between 200-1000 m depth. For each season, a nMDS analysis was applied to the similarity matrix, and the environmental variables (zooplankton biomass, temperature, salinity) were also plotted as bubbles to represent their influence on the structure of the assemblages. The correlation between the biological and environmental matrices was evaluated using a BEST-BIOENV test based on Spearman’s rank correlation coefficient (Clarke and Ainsworth, 1993). Besides, to test the difference between the two assemblages, an ANOSIM hypothesis test (9999 permutations) was performed. The R ANOSIM test is a non-parametric method based on ranks used to determine if two or more groups are statistically different. The R values near 1 indicate differences among groups, whereas values near zero suggest more similarity among them. Furthermore, a SIMPER analysis was also used to determine the taxa with the greatest contribution to the separation of assemblages. These analyses were carried out with PRIMER v7 software (Clarke et al., 2014). The amphipod abundance data were plotted on vertical transects of temperature using the SURFER v15 software.
The two assemblages were also compared in terms of diversity. In both seasons, we used species accumulation curves of alpha diversity with interpolation-extrapolation of Hill Numbers and the incident-based estimator Chao2 (Chao and Jost, 2012). In addition, we estimated the sample completeness to infer the representativeness of the sampling effort of each assemblage (Chao and Jost, 2012), considering a common sampling coverage value of 0.95 to compare the alpha diversity. This integrated methodology yielded less biased comparisons between a set of communities by the use of an equal sample coverage (completeness) value (Chao and Jost, 2012). Diversity analysis was performed with the statistical software R using the iNEXT package (Hsieh et al., 2016).
In the second approach, considering the five sampling levels as a factor, the similarity matrix of each season was subjected to a metric MDS using Bootstrap to reduce the stress in the two-dimensional plane. This analysis generates average values and regions of each factor (levels) to identify trends in data sets and improve their graphic representation in the ordination plane, through randomization of the data with replacements (Jacoby and Armstrong, 2014). Furthermore, the average abundance of the entire community, as well as the three main groups of species (infraorder Physocephalata, infraorder Phyososomata, and non-hyperiid species) was plotted by sampling level to visualize the differences between day- and nighttime. An ANOSIM hypothesis test (9999 permutations) was used to determine whether the five sampling levels and the day- and nighttime average abundance had statistically significant differences. Particularly, for this finer analysis, we compared the diversity (by completeness) between levels using the common sampling coverage value of 0.85 to interpolate the alpha diversity.
The θ-SA diagrams allowed us to recognize the five main water masses in the Gulf: the Caribbean Surface Water (CSW), the Subtropical Underwater (SUW), the Gulf Common Water (GCW), the Tropical Atlantic Central Water (TACW), and the Antarctic Intermediate Water (AAIW) (Figure 2). The greatest variations of temperature and salinity occurred above 200 m depth and the main difference between the seasons was due to temperature (θ): values around 29°C were recorded in most surface waters during summer, while in winter they did not exceed 25°C. Salinity (SA) had a variation of 2 units from the surface to the depth (Figure 2).
Figure 2. Conservative temperature (θ) – absolute salinity (SA) diagrams of data from the oceanic province sampled during winter and summer in the southern Gulf of Mexico. Water masses: Caribbean Surface Water (CSW), North Atlantic Subtropical Underwater (SUW), Gulf Common Water (GCW), Tropical Atlantic Central Water (TACW), and Antarctic Intermediate Water (AAIW).
The vertical transects showed that the characteristic water masses of the epipelagic zone (CSW and GCW) occurred above 200 m depth, with some depth variations along each transect during the winter and the summer (Figures 3, 4). Regarding the temperature, the values between 15 and 18°C, associated with the TACW water mass, occurred deeper in some areas, especially during the summer in the northernmost transect, where these values were observed up to 300 m depth (Figure 4, Transect As).
Figure 3. Vertical distribution of the water density (kg/m3) and potential temperature (°C) in the southern Gulf of Mexico during winter 2013. Water masses abbreviations are in Figure 2 caption.
Figure 4. Vertical distribution of the water density (kg/m3) and potential temperature (°C) in the southern Gulf of Mexico during summer 2014. Water masses abbreviations are in Figure 2 caption.
In total, 9574 individuals were separated, and 120 species were identified: 87 in winter (‘epipelagic’: 69 species; ‘mesopelagic’: 76) and 115 in summer (‘epipelagic’: 88; ‘mesopelagic’: 108) (Table 1).
Table 1. Density (ind/1000 m3) of amphipod species during winter (2013) and summer (2014) in the southern Gulf of Mexico. n, number of samples.
From the organismal point of view, some differences were observed between assemblages. Most species here recorded were found in both assemblages, but the ‘mesopelagic’ contains a large number of exclusive species in the two seasons (18 in winter and 27 in summer; Table 1). Generally, exclusive taxa in the mesopelagic assemblage were from the Physosomata infraorder (Scina, Lanceola, Mimonectes, Ctenoscina, Archaeoscina) and some others from the non-hyperiid amphipods (Cyphocaris, Metacyphocaris, Eusirella).
The assemblages defined a priori ‘epipelagic’ and ‘mesopelagic’ were differentiated by the nMDS analysis in both seasons: winter (Figure 5) and summer (Figure 6). Differences between assemblages were confirmed by the ANOSIM test (winter: global R = 0.557, p = 0.001; summer: global R = 0.41, p = 0.001).
Figure 5. Amphipod community represented by the (A) assemblages defined a priori (‘epipelagic’ and ‘mesopelagic’) through the nMDS with the (B) zooplankton biomass, (C) salinity and (D) temperature overlayed as bubbles, during winter in the southern Gulf of Mexico.
Figure 6. Amphipod community represented by the (A) assemblages defined a priori (‘epipelagic’ and ‘mesopelagic’) through the nMDS with the (B) zooplankton biomass, (C) salinity and (D) temperature overlayed as bubbles, during summer in the southern Gulf of Mexico.
The main difference between assemblages was the density of amphipod species (Table 1). These differences were more evident when plotting the density in the vertical plane where the highest values (more than 60 ind/1000 m3) were mainly observed above 200 m depth (Figure 7). In the ‘mesopelagic’ assemblage, density was low (< 60 ind/1000 m3), especially during the winter, when values around 10 ind/1000 m3 were more common (Figure 7). Among the seasons, the highest abundance was found during the summer, at least twice as much as the winter in epi- and mesopelagic assemblages (Table 1).
Figure 7. Density of amphipods in the ‘epipelagic’ and ‘mesopelagic’ assemblages represented over vertical temperature planes in the southern Gulf of Mexico during winter and summer. The location of each transect is shown in Figure 1.
Visualizing the effect of environmental variables on the biological data, it seems that the zooplankton biomass had the major influence during the summer (Figure 6); however, during the winter, it seems that no one variable stands out over the other (Figure 5). In accordance, the BEST-BIOENV test showed the zooplankton biomass to have the best correlation with the amphipod matrix during the summer (rho = 0.319, p = 0.001) (Table 2). During winter, all the variables and the combination of them (salinity, biomass, temperature) had nearly the same (rho ~ 0.44, p = 0.001) influence on the structure of the community (Table 2). Besides, the SIMPER analysis indicated that Lestrigonus bengalensis was the species with the greatest contribution to the separation of the assemblages during winter, and the juveniles of the Primno genus during summer (Table 3).
Table 2. Weighted Spearman’s rank correlation between biotic and environmental variables using the BEST-BIOENV test.
Table 3. Species that contributed the most to the differentiation of the assemblages defined a priori in the southern Gulf of Mexico during winter 2013 and summer 2014 according to the SIMPER analysis.
Regarding diversity, the ‘epipelagic’ assemblage recorded the lowest number of observed species (69 in winter; 88 in summer) compared to the ‘mesopelagic’ (76 in winter; 108 in summer) (Figures 8A, B). The reaching of an asymptote in the ‘epipelagic’ curve during winter indicates the reduction of uncertainty in the detection of the incidence of other species. The above was a consequence of the sample coverage values of assemblages which were greater than 0.95 (Figures 8C, D) indicating a good representativeness of the sampling effort in both seasons. Finally, the interpolation of the species richness to a completeness value of 0.95 confirmed the previously observed pattern with the highest diversity associated with the ‘mesopelagic’ assemblage (80 in winter; 85 in summer), contrasting with the low values in the ‘epipelagic’ one (70 in winter and summer) (Figures 8E, F).
Figure 8. Interpolation and extrapolation of richness values and sample completeness analyses of pelagic amphipod assemblages from the southern Gulf of Mexico. (A) Species accumulation curve based on the incidence of species for each assemblage during the winter, and (B) summer; (C) Sample-coverage accumulation curve based on incidence for each assemblage during the winter, and (D) summer; (E) Sample completeness curves during winter, and (F) summer.
The metric MDS analysis with bootstrap performed taking the sampling levels as factors, separated the 0-200 m level from those located below 200 m depth for both seasons (Figure 9). During the winter, levels of the mesopelagic zone were highly homogeneous (ANOSIM test, p > 0.05) (Figure 9). In contrast, during the summer, the pair-R values of the 200-400 m level with the other levels of the mesopelagic zone are close to zero indicating certain affinity; however, the p-value showed significant differences of the 200-400 m level with two mesopelagic levels (Table 4). Thus, the mesopelagic zone is more heterogeneous in summer than in winter. SIMPER analysis indicated that Stenopleura atlantica was the species with the greatest contribution to the separation between the 200-400 m level and the other deep levels of the ‘mesopelagic’ assemblage during this season (Table 5).
Figure 9. Amphipod community represented by sampling level through the MDS with bootstrap analysis, southern Gulf of Mexico.
Table 4. Results of the R ANOSIM test and associated significance values (in brackets) among the five sampling levels during the summer of 2014.
Table 5. Species that contributed the most to the differentiation of sampling levels of the ‘mesopelagic’ assemblage of the southern Gulf of Mexico during the summer of 2014, according to the SIMPER analysis.
Records of zooplankton biomass and mean amphipod density were higher in summer, showing a decreasing pattern with depth (Table 6). Considering day and night sampling time, no differences were observed in the whole amphipod density between the stations sampled in the upper 200 m layer in both seasons (Figure 10A), and this was confirmed by the ANOSIM test (winter: global R = -0.08, p = 0.927; summer: global R = 0.068, p = 0.072). However, looking the amphipod community as separate groups (Physocephalata, Phyososomata, and non-hyperiids) we found that, during the summer, the infraorder Physosomata showed significant differences (R = 0.598, p = 0.001) in the 0-200 level between the night- (~25 ind/1000 m3) and daytime (~3 ind/1000 m3) (Figure 10C). In contrast, the infraorder Physocephalata and the non-hyperiids had no significant differences (ANOSIM test, p > 0.05) (Figures 10B, D) in any of the seasons.
Table 6. Mean (± SD) of zooplankton biomass (mL/1000 m3) and amphipod density (ind/1000 m3) at each sampling level and two seasons in the southern Gulf of Mexico.
Figure 10. Average abundance of pelagic amphipods by sampling level during day- and nighttime in two contrasting seasons in the southern Gulf of Mexico. (A) Overall abundance of amphipods, (B) Physocephalata, (C) Physosomata, and (D) non-hyperiid species.
The species accumulation curves of the alpha diversity among sampling levels indicated that species richness was highest in the 0-200 m level (69 in winter; 88 in summer) and gradually decreased with depth during the two seasons (Figures 11A, B). In general, sampling coverage values of the levels were ≥ 0.85 indicating a good representativeness of their sampling effort (Figures 11C, D). Interpolation of the species richness to a completeness value of 0.85 indicated that the highest diversity was in the 400-600 m level during winter with 50 species (Figure 11E), and in the 200-400 m level during summer with 60 species (Figure 11F).
Figure 11. Interpolation and extrapolation of species richness values and sample completeness analyses of pelagic amphipods by sampling level from the southern Gulf of Mexico. (A) Species accumulation curve based on the incidence of species for each sampling level during the winter, and (B) summer; (C) Sample-coverage accumulation curve based on incidence for each sampling level during the winter, and (D) summer; (E) Sample completeness curves during winter, and (F) summer.
The mesopelagic zone of the oceans has been hardly explored. Ecological and diversity information of zooplankton in this zone is very scarce. In this study, the coarse-scale vertical analysis demonstrated that the assemblages defined a priori ‘epipelagic’ and ‘mesopelagic’ showed significant differences (ANOSIM test, p < 0.05), being stronger in winter. This result contradicts the hypothesis that the summer would have the greatest difference due to stronger gradients in environmental conditions, especially temperature. Paradoxically, the deepening of 15-18°C temperature values in summer, related to the TACW water mass, makes the upper mesopelagic zone (up to 300 m depth) more similar to the epipelagic zone (Figure 4, transect As) than in winter. In turn, this could induce a deepening of the epipelagic assemblage during the summer, resulting in less heterogeneity between the epi- and the mesopelagic zones.
As expected, the BEST-BIOENV test signaled the zooplankton biomass (as a measure of food availability) as an important factor affecting the distribution of the amphipods in the water column, especially during the summer (Table 2). In winter, the temperature and salinity conditions gained importance, but the best three models involved the zooplankton biomass (Table 2). Despite being surrounded by land masses, the Gulf of Mexico exhibits similar features to oligotrophic regions of the great oceans in terms of zooplankton biomass (Landry and Swalethorp, 2021). While seasonal changes in secondary productivity over the shelf are marked and strongly dependent on continental water discharges, in the oceanic area of the southern Gulf, productivity is lower and seasonal changes less pronounced than over the shelf; even though, in both zones, the summer exhibits the highest zooplankton biomass (Zavala-García et al., 2016; Färber-Lorda et al., 2019). As stated, pelagic amphipods tend to inhabit areas of high productivity (Gasca, 2004; Hereu et al., 2020), where they can easily find their food. In this study, amphipod density in the epipelagic zone was at least ten times higher than the mesopelagic zone in summer, and four times in winter (Table 6). The zooplankton biomass showed a similar pattern among the seasons. Following our results, other studies found the greatest abundance of pelagic amphipods during the summer. Besides the food availability, during this warm season occurs a strong reproductive activity of some amphipods. For instance, species of Lestrigonus and Primno have recorded a high abundance of juveniles and adults in this warm period in several world oceans (Gasca, 2004; Gasca and Suárez-Morales, 2004; Zhang et al., 2014; Hereu et al., 2020).
According to the SIMPER analysis, the species with the greatest contribution to the separation of the assemblages ‘epipelagic’ and ‘mesopelagic’ were: L. bengalensis during winter, and juveniles of the genus Primno during summer (Table 3). Lestrigonus bengalensis is widely recognized as the dominant species in the Gulf of Mexico (Gasca, 2003a, 2003b, 2004; Gasca et al., 2009; Hereu et al., 2020; Sanvicente-Añorve et al., 2023) and this is probably the reason why it had the greatest contribution to the separation of both assemblages during winter. This species has a wide vertical range (>1000 m depth) (García-Madrigal, 2007), which allows the individuals to inhabit the mesopelagic zone; however, its dominance is restricted to the epipelagic zone as observed in the current survey (Table 1) and other regions of the world (Thurston, 1976b; Shulenberger, 1978; Siegel-Causey, 1982; Vinogradov et al., 1996; Vinogradov, 1999). During summer, L. bengalensis was not the dominant species, nevertheless, its abundance was more than double that recorded in winter (Table 1), confirming the affinity of the species with the warm season, as previously observed in the Gulf (Gasca, 2004; Hereu et al., 2020).
The species of the genus Primno are commonly found in the Gulf (LeCroy et al., 2009), and their vertical distribution range reaches 1000 m depth (Vinogradov et al., 1996; Vinogradov, 1999). In the Gulf, juveniles of the genus Primno have been observed as a dominant taxon during the summer, suggesting greater reproductive activity during the warm season (Gasca, 2004; Hereu et al., 2020). This could be the reason why the taxon dominated in the summer season (Tables 1, 3).
The analysis performed taking the sampling levels as a factor showed differentiation of the 200-400 m level in the mesopelagic zone during the summer (Figure 9; Table 4). This could be explained by the deepening of the TACW water mass (Figure 4, transect As), which separates the second level (200-400 m) from the other deep ones (Figure 9; Table 4). In a recent study, Cervantes-Díaz et al. (2022) indicated that the deepening of water masses in the southern Gulf could be related to the noticeable presence of the CSW water mass during this warm season. However, the effect of this hydrological feature on the vertical structure of zooplankton communities had not been previously explored. According to the SIMPER analysis, a non-hyperiid species (Stenopleura atlantica) had the greatest contribution to the separation of the levels of the mesopelagic zone during the summer (Table 5), due to its dominance in the upper mesopelagic zone (200-400 m). In the vertical plane, S. atlantica is found from 0 to 1000 m depth, however, the highest abundance of adults, juveniles, and ovigerous females occurs above 200 m depth, which confirms the affinity of the species to inhabit the warm waters of the epipelagic zone (Thurston, 1976a; Vinogradov, 1999; Violante-Huerta et al., 2020). In ecological terms, pelagic amphipods of the suborder Hyperiidea are the most studied due to their high abundance and diversity; however, our results suggest that a better understanding of the vertical distribution patterns of amphipods could be attained by including non-hyperiid species, such as S. atlantica.
Daytime differences in total amphipod abundance were not observed in any season (Figure 10), and this is consistent with the last survey in the Gulf (Hereu et al., 2020). The diurnal vertical migration range of the pelagic amphipods is generally less than 100 m (Thurston, 1976b; Shulenberger, 1977), so it can only be observed at finer sampling scales (Cornet and Gili, 1993; Pai et al., 2010; Domínguez-Nava et al., 2021; Schaafsma et al., 2024). Vertical migration has been related to some preferences for occupy a specific depth range during the day- or nighttime or even as a response to physiological requirements (Elder and Seibel, 2015a, 2015b; Taniguchi et al., 2023). In this study, the broader interval of sampled levels likely makes it difficult to observe this general migratory pattern. However, in the finer analysis made by groups, we found that during the summer, the abundance of members of the infraorder Physosomata showed significant differences (ANOSIM test, p < 0.05) in the 0-200 level between the night- and daytime samplings (Figure 10C). These organisms are mainly inhabitants of the meso- and bathypelagic zones (Vinogradov et al., 1996; Vinogradov, 1999), so their greater presence in the upper layer (0-200 m) during the night could be indicative of a migratory process, as other field observations found (Thurston, 1976b; Granata et al., 2020; Domínguez-Nava et al., 2021; Véliz et al., 2021). The daily migration range of the Physosomata species is not well known, but most probably our findings correspond to species that inhabit the upper mesopelagic zone (e.g. Scina, Acanthoscina) (Thurston, 1976b), which can reach the adjacent epipelagic zone during their daily vertical movements.
This study recorded the greatest number of species in the Gulf of Mexico to date (Table 1). Previous studies in the Gulf have only examined the epipelagic community of amphipods, recording an average of 61 species, with a maximum value of 79 species (Gasca, 2003a, 2003b, 2004; Gasca et al., 2009; Hereu et al., 2020; Sanvicente-Añorve et al., 2023). The large number of species recorded here was due to the examination of the previously unexplored mesopelagic zone; indeed, this region recorded the greatest interpolated values in species (Figures 11E, F). The high diversity in the mesopelagic zone was related to the occurrence of some exclusive species from the deep waters, especially those belonging to the infraorder Physosomata (Table 1). Species of the infraorder Physosomata (Hyperiidea) inhabit mainly the mesopelagic waters (Thurston, 1976b; Vinogradov et al., 1996; Vinogradov, 1999), which explains the observed pattern in this study.
Seasonally, interpolations to a common sampling coverage value showed that the highest species richness was found during summer (Figure 8F). Our findings partially agree with a previous study conducted in the oceanic zone of the southern Gulf in winter and summer (Gasca et al., 2009), which found the highest species richness in summer, but associated with the lower amphipod density. This implies that more research is required because the seasonal cycles of abundance and diversity in the study area are not well understood.
When exploring the diversity by sampling level, the interpolation indicated that the greatest diversity was found in the ‘mesopelagic’ assemblage: in the 400-600 m level in winter, and the 200-400 m level in summer (Figures 11E, F). A deep-sea diversity peak was previously observed in other zooplankton groups and has been related to the diurnal vertical migration of species (Angel, 1989; Andersen et al., 2001; Steinberg et al., 2008). A recent study revealed that copepods increased their diversity in the deeper mesopelagic zone, probably due to less competition in that environment with limited food resources (Stefanoudis et al., 2019). Other environmental features related to the greater diversity in the deep sea could be a lower risk of predation, more stable hydrological conditions, or even the occurrence of different water masses (Kosobokova and Hopcroft, 2010; Kosobokova et al., 2011). However, these statements are difficult to probe due to the complexity and methodological replication to obtain the sampling representativeness in this extreme environment (McClain and Schlacher, 2015; Paulus, 2021).
In the Gulf of Mexico, the mesopelagic zone is considered an “ecotone” of tropical, subtropical, and temperate species (Sutton et al., 2017), as evidenced by the presence of water masses of different origins (Figure 2). Our results demonstrated that the greatest diversity lies in this scarcely explored area, suggesting the need of further studies to better understand the ecology of the deep-sea pelagic amphipods of the Gulf.
This is the first study in Mexico that explores the mesopelagic amphipod community and compares it with that of the epipelagic zone, during two contrasting seasons, winter and summer. We hypothesize stronger differences between the epi- and the mesopelagic zones in summer because of stronger vertical environmental conditions and, we also propose that the zooplankton biomass, as a measure of food availability, would have an important role in the distribution of amphipods. Zooplankton samples were obtained using stratified plankton nets in the oceanic province of the Gulf of Mexico in five levels of the water column, from surface to 1000 m depth; nevertheless, sampling effort was uneven among the levels. To ensure the reliability of the results, biological data were treated through robust non-parametric multivariate analyses employing bootstrap procedures and permutation tests, and diversity analyses were based on sample completeness models to avoid bias in the sampling effort among sampling levels. Our results indicated that the differences between ‘epipelagic’ and ‘mesopelagic’ assemblages were stronger during the winter, which contradicts the first part of the hypothesis. During the summer, the analysis of the vertical structure of environmental variables showed a deepening of the TACW water mass to the upper mesopelagic (up to 300 m depth) zone, resulting in less heterogeneity of the amphipod community between the epi- and mesopelagic zones. As well, the finer analysis made by sampling levels, showed the 200-400 m level to be different from the remainder mesopelagic levels during the summer. The association between the amphipod composition/abundance and environmental (temperature, salinity, zooplankton biomass) matrices signaled the zooplankton biomass as the main factor determining the structure of the amphipod community, especially during the summer. The diversity analyses performed with innovative methods taking into account the sampling coverage indicated that, seasonally, the summer had the highest diversity and, vertically, the mesopelagic zone. We encourage the exploration of the deep-sea amphipod community to improve the ecological knowledge of this group in the oceans.
The original contributions presented in the study are included in the article/Supplementary Material. Further inquiries can be directed to the corresponding authors.
The manuscript presents research on animals that do not require ethical approval for their study.
MV-H: Conceptualization, Data curation, Formal analysis, Investigation, Methodology, Supervision, Validation, Writing – original draft, Writing – review & editing. LS-A: Conceptualization, Formal analysis, Funding acquisition, Investigation, Methodology, Supervision, Validation, Writing – original draft, Writing – review & editing, Data curation. MA-M: Methodology, Software, Supervision, Writing – review & editing, Formal analysis, Validation. EG-C: Formal analysis, Methodology, Software, Supervision, Writing – review & editing, Validation.
The author(s) declare financial support was received for the research, authorship, and/or publication of this article. The first author is grateful for the support of the Posgrado en Ciencias del Mar y Limnología, Universidad Nacional Autónoma de México, and the scholarship (862851) granted by the CONAHCYT in Mexico. The Instituto de Ciencias del Mar y Limnología, Universidad Nacional Autónoma de México provided financial resources for this study and supported the oceanographic cruises ZOOMEP, coordinated by Dr. César Flores-Coto.
The authors also appreciate the technical assistance of F. Zavala-García and M. Martínez-Mayén during the development of this research. We are grateful to the editor and the reviewers for their comments that allowed us to improve the manuscript.
The authors declare that the research was conducted in the absence of any commercial or financial relationships that could be construed as a potential conflict of interest.
The author(s) declare that no Generative AI was used in the creation of this manuscript.
All claims expressed in this article are solely those of the authors and do not necessarily represent those of their affiliated organizations, or those of the publisher, the editors and the reviewers. Any product that may be evaluated in this article, or claim that may be made by its manufacturer, is not guaranteed or endorsed by the publisher.
The Supplementary Material for this article can be found online at: https://www.frontiersin.org/articles/10.3389/fmars.2025.1508160/full#supplementary-material
Andersen V., Gubanova A., Nival P., Ruellet T. (2001). Zooplankton community during the transition from spring bloom to oligotrophy in the open NW Mediterranean and effects of wind events. 2. Vertical distributions and migrations. J. Plankton Res. 23, 243–261. doi: 10.1093/plankt/23.3.243
Angel M. V. (1989). Vertical profiles of pelagic communities in the vicinity of the Azores Front and their implications to deep ocean ecology. Prog. Oceanogr. 22, 1–46. doi: 10.1016/0079-6611(89)90009-8
Burridge A. K., Tump M., Vonk R., Goetze E., Peijnenburg K. (2016). Diversity and distribution of hyperiid amphipods along a latitudinal transect in the Atlantic Ocean. Prog. Oceanogr. 158, 224–235. doi: 10.1016/j.pocean.2016.08.003
Campana S. E. (1989). Otolith microstructure of three larval gadids in the Gulf of Maine, with inferences on early life history. Can. J. Zool. 67, 1401–1410. doi: 10.1139/z89-199
Cervantes-Díaz G. Y., Hernández-Ayón J. M., Zirino A., Herzka S. Z., Camacho-Ibar V., Norzagaray O., et al. (2022). Understanding upper water mass dynamics in the Gulf of Mexico by linking physical and biogeochemical features. J. Mar. Syst. 225, 103647. doi: 10.1016/j.jmarsys.2021.103647
Chao A., Jost L. (2012). Coverage-based rarefaction and extrapolation: standardizing samples by completeness rather than size. Ecology 93, 2533–2547. doi: 10.1890/11-1952.1
Clarke K. R., Ainsworth M. (1993). A method of linking multivariate community structure to environmental variables. Mar. Ecol. Prog. Ser. 92, 205–219. doi: 10.3354/meps092205
Clarke K. R., Gorley R. N., Somerfield P. J., Warwick R. M. (2014). Change in marine communities: An approach to statistical analysis and interpretation (Plymouth: PRIMER-E Ltd).
Cornet C., Gili J. M. (1993). Vertical distribution and daily migrations of amphipods in the northern Benguela in relation to water column stratification. Deep Sea Res. I 40, 2295–2306. doi: 10.1016/0967-0637(93)90105-C
Domínguez-Nava A., Gasca R., Carrillo L., Vásquez-Yeomans L., Suárez-Morales E. (2021). Hyperiid amphipod vertical distribution and community structure in the upper 100 m of the northwestern Caribbean Sea. Bull. Mar. Sci. 97, 401–426. doi: 10.5343/bms.2020.0030
Elder L. E., Seibel B. A. (2015a). Ecophysiological implications of vertical migration into oxygen minimum zones for the hyperiid amphipod. Phronima sedentaria. J. Plankton Res. 37, 897–911. doi: 10.1093/plankt/fbv066
Elder L. E., Seibel B. A. (2015b). The thermal stress response to diel vertical migration in the hyperiid amphipod Phronima sedentaria. Comp. Biochem. Physiol. Part A 187, 20–26. doi: 10.1016/j.cbpa.2015.04.008
Espinosa-Leal L., Bode A., Escribano R. (2020). Zonal and depth patterns in the trophic and community structure of hyperiid amphipods in the Southeast Pacific. Deep Sea Res. I 165, 103402. doi: 10.1016/j.dsr.2020.103402
Espinosa-Leal L., Escribano R., Riquelme-Bugueño R., Corredor-Acosta A. (2021). Distribution and biodiversity patterns of hyperiid amphipods across the coastal-offshore gradient of the sub-tropical Southeast Pacific. Mar. Biodivers. 51, 13. doi: 10.1007/s12526-020-01152-x
Färber-Lorda J., Athié G., Camacho Ibar V., Daessle L. W., Molina O. (2019). The relationship between zooplankton distribution and hydrography in oceanic waters of the Southern Gulf of Mexico. J. Mar. Syst. 192, 28–41. doi: 10.1016/j.jmarsys.2018.12.009
García-Madrigal M. S. (2007). Annotated checklist of the amphipods (Peracarida: Amphipoda) from the Tropical Eastern Pacific. Contributions to Study East Pacific Crustaceans 4, 63–195.
Gasca R. (2003a). Hyperiid amphipods (Crustacea: Peracarida) in relation to a cold-core ring in the Gulf of Mexico. Hydrobiologia 510, 115–124. doi: 10.1023/B:HYDR.0000008637.16933.6f
Gasca R. (2003b). Hyperiid amphipods (Crustacea: Peracarida) and spring mesoscale features in the Gulf of Mexico. Mar. Ecol. 24, 303–317. doi: 10.1046/j.1439-0485.2003.00834.x
Gasca R. (2004). Distribution and abundance of hyperiid amphipods in relation to summer mesoscale features in the southern Gulf of Mexico. J. Plankton Res. 26, 993–1003. doi: 10.1093/plankt/fbh091
Gasca R. (2009). Diversity of hyperiid amphipods (Crustacea: Peracarida) in the western Caribbean Sea: news from the deep. Zool. Stud. 48, 63–70.
Gasca R., Manzanilla H., Suárez-Morales E. (2009). Distribution of hyperiid amphipods (Crustacea) of the southern Gulf of Mexico, summer and winte. J. Plankton Res. 31, 1493–1504. doi: 10.1093/plankt/fbp096
Gasca R., Suárez-Morales E. (2004). Distribution and abundance of hyperiid amphipods (Crustacea: Peracarida) of the Mexican Caribbean Sea, (August 1986). Caribbean J. Sci. 40, 23–30.
Gorbatenko K. M., Grishan R. P., Dudkov S. P. (2017). Biology and distribution of hyperiids in the Sea of Okhotsk. Oceanology 57, 278–288. doi: 10.1134/S0001437016060023
Granata A., Bergamasco A., Battaglia P., Milisenda G., Pansera M., Bonanzinga V., et al. (2020). Vertical distribution and diel migration of zooplankton and micronekton in Polcevera submarine canyon of the Ligurian mesopelagic zone (NW Mediterranean Sea). Progr. Oceanol. 183, 102298. doi: 10.1016/j.pocean.2020.102298
Hereu C. M., Arteaga M. C., Galindo-Sánchez C. E., Herzka S. Z., Batta-Lona P. G., Jiménez-Rosenberg S. P. A. (2020). Zooplankton summer composition in the southern Gulf of Mexico with emphasis on salp and hyperiid amphipod assemblages. J. Mar. Biol. Assoc. U. K. 100, 665–680. doi: 10.1017/S0025315420000715
Hsieh T. C., Ma K. H., Chao A. (2016). iNEXT: an R package for rarefaction and extrapolation of species diversity (Hill numbers). Methods Ecol. Evol. 7, 1451–1456. doi: 10.1111/2041-210X.12613
Hughes L. E., Lowry J. K. (2015). A review of the world Cyphocarididae with description of three new species (Crustacea, Amphipoda, Lysianassoidea). Zootaxa 4058, 1–40. doi: 10.11646/zootaxa.4058.1.1
Jacoby W. G., Armstrong D. A. (2014). Bootstrap confidence regions for multidimensional scaling solutions. Am. J. Polit. Sci. 58, 264–278. doi: 10.1111/ajps.12056
Kosobokova K. N., Hopcroft R. R. (2010). Diversity and vertical distribution of mesozooplankton in the Arctic's Canada Basin. Deep Sea Res. Part II: Top. Stud. Oceanogr. 57, 96–110. doi: 10.1016/j.dsr2.2009.08.009
Kosobokova K. N., Hopcroft R. R., Hirche H. J. (2011). Patterns of zooplankton diversity through the depths of the Arctic’s central basins. Mar. Biodiver. 41, 29–50. doi: 10.1007/s12526-010-0057-9
Landry M. R., Swalethorp R. (2021). Mesozooplankton biomass, grazing and trophic structure in the bluefin tuna spawning area of the oceanic Gulf of Mexico. J. Plank. Res. 44, 677–691. doi: 10.1093/plankt/fbab008
Lavaniegos B. E. (2014). Pelagic amphipod assemblage associated with subarctic water off the west coast of the Baja California peninsula. J. Mar. Syst. 132, 1–12. doi: 10.1016/j.jmarsys.2013.12.012
Lavaniegos B. E. (2017). Changes in composition of summer hyperiid amphipods from a subtropical region of the California current during 2002–2008. J. Mar. Syst. 165, 13–26. doi: 10.1016/j.jmarsys.2016.09.001
Lavaniegos B. E. (2020). Hyperiid amphipods from the Gulf of Ulloa and offshore region, Baja California: The possible role of the gelatinous zooplankton as a transport vector into the coastal shelf waters. PloS One 15, e0233071. doi: 10.1371/journal.pone.0233071
Lavaniegos B. E., Hereu C. M. (2009). Seasonal variation in hyperiid amphipod abundance and diversity and influence mesoscale structures off Baja California. Mar. Ecol. Prog. Ser. 394, 137–152. doi: 10.3354/meps08285
LeCroy S., Gasca R., Winfield I., Ortiz M., Escobar-Briones E. (2009). “Amphipoda (Crustacea) of the Gulf of Mexico,” in Gulf of Mexico, Origins, Waters and Biota, Volume 1, Biodiversity. Eds. Felder D., Camp D. (Texas A&M University Press. Corpus Christi), 941–972.
Lowry J. K., Stoddart H. E. (1997). Amphipoda crustacea IV. Families aristiidae, cyphocarididae, endevoursidae, lysianassidae, scopelocheiridae, uristidae. Memoirs Hourglass Cruises 10, 1–148.
Mazda Y., Sasagawa E., Linuma Y., Wakabayashi K. (2019). Maternal care and juvenile feeding in a hyperiid amphipod (Oxycephalus clausi Bovallius (1887) in association with gelatinous zooplankton. Mar. Biol. Ress. 15, 541–547. doi: 10.1080/17451000.2019.1694692
McClain C. R., Schlacher T. A. (2015). On some hypotheses of diversity of animal life at great depths on the sea floor. Mar. Ecol. 36, 849–872. doi: 10.1111/maec.12288
McDougall T. J., Barker P. M. (2011). Getting Started with TEOS-10 and the Gibbs Seawater (GSW) Oceanographic Toolbox (Sydney: SCOR/IAPSO), WG127.
Minutoli R., Bergamasco A., Guglielmo L., Swadling K. M., Bergamasco A., Veneziano F., et al. (2023). Species diversity and spatial distribution of pelagic amphipods in Terra Nova Bay (Ross Sea, Southern Ocean). Polar Biol. 46, 821–835. doi: 10.1007/s00300-023-03166-0
Pai I. K., Tendulkar S., Pereir M. L. (2010). “Zooplankton diel vertical migration at Andaman Sea,” in Recent trends in biodiversity of Andaman and Nicobar islands. Kolkata: Zoological Survey of India. Eds. Ramakrishna R., Raghunathan C., Sivaperuman C. (Zoological survey of India, Calcutta), 113–129.
Paulus E. (2021). Shedding light on deep-sea biodiversity—A highly vulnerable habitat in the face of anthropogenic change. Front. Mar. Sci. 8. doi: 10.3389/fmars.2021.667048
Postel L., Fock H., Hagen W. (2000). “Biomass and abundance,” in ICES zooplankton methodology manual. Eds. Harris R., Wiebe P., Lenz J., Skjoldal H. R., Huntley M. (Academic Press, London), 83–192.
Roe H. S. J., James P. T., Thurston M. H. (1984). The diel migrations and distributions within a mesopelagic community in the north east Atlantic. 6. Medusae, ctenophores, amphipods and euphausiids. Prog. Oceanogr. 13, 425–460. doi: 10.1016/0079-6611(84)90015-6
Sampaio de Souza C., Rodrigues da Conceiição L., de Oliveira Mafalda Junior P. (2016). Hyperiid amphipods around the seamounts and islands off northeastern Brazil. Braz. J. Oceanogr. 64, 339–352. doi: 10.1590/S1679-87592016123306404
Sanvicente-Añorve L., Ramírez-Velázquez B., Hermoso-Salazar M. (2023). Pelagic amphipods (Crustacea, Amphipoda, Hyperiidea) from the southern Gulf of Mexico with notes on the distribution of species. Biodivers. Data J. 11, e97347. doi: 10.3897/BDJ.11.e97347
Schaafsma F. L., Matsuno K., Driscoll R., Sasaki H., van Regteren M., Driscoll S., et al. (2024). Zooplankton communities at the sea surface of the eastern Indian sector of the Southern Ocean during the austral summer of 2018/2019. Prog. Oceanogr. 226, 103303. doi: 10.1016/j.pocean.2024.103303
Shulenberger E. (1977). Hyperiid amphipods from the zooplankton community of the North Pacific central gyre. Mar. Biol. 42, 375–385. doi: 10.1007/BF00402200
Shulenberger E. (1978). Vertical distribution, diurnal migrations, and sampling problems of hyperiid amphipods in the North Pacific central gyre. Deep-Sea Res. 25, 605–623. doi: 10.1016/0146-6291(78)90616-1
Siegel-Causey D. (1982). Factors determining the distribution of hyperiid Amphipoda in the Gulf of California. University of Arizona, Arizona.
Stefanoudis P. V., Rivers M., Ford H., Yashayaev I. M., Rogers A. D., Woodall L. C. (2019). Changes in zooplankton communities from epipelagic to lower mesopelagic waters. Mar. Environ. Res. 146, 1–11. doi: 10.1016/j.marenvres.2019.02.014
Steinberg D. K., Cope J. S., Wilson S. E., Kobari T. (2008). A comparison of mesopelagic mesozooplankton community structure in the subtropical and subarctic North Pacific Ocean. Deep Sea Res. Part II: Top. Stud. Oceanogr. 55, 1615–1635. doi: 10.1016/j.dsr2.2008.04.025
Sutton T. T., Clark M. R., Dunn D. C., Halpin P. N., Rogers A. D., Guinotte J., et al. (2017). A global biogeographic classification of the mesopelagic zone. Deep Sea Res. I: Oceanogr. Res. Pap. 126, 85–102. doi: 10.1016/j.dsr.2017.05.006
Taniguchi R., Amei K., Tokuhiro K., Yamada Y., Kitamura M., Yamaguchi A. (2023). Diel, seasonal and vertical changes in the pelagic amphipod communities in the subarctic Pacific: insights from imaging analysis. J. Plankton Res. 45, 554–570. doi: 10.1093/plankt/fbad017
Thurston M. H. (1976a). The vertical distribution and diurnal migration of the Crustacea Amphipoda collected during the SOND Cruise 1965 I. J. Mar. Biol. Assoc. U. K. 56 (2), 359–382. doi: 10.1017/S002531540001897X
Thurston M. H. (1976b). The vertical distribution and diurnal migration of the Crustacea Amphipoda collected during the SOND Cruise 1965 II. J. Mar. Biol. Assoc. U. K. 56 (2), 383–470. doi: 10.1017/S0025315400018981
Valencia B., Lavaniegos B., Giraldo A., Rodríguez-Rubio E. (2013). Temporal and spatial variation of hyperiid amphipod assemblages in response to hydrographic processes in the Panama Bight, eastern tropical Pacific. Deep Sea Res. I: Oceanogr. Res. Pap. 73, 46–61. doi: 10.1016/j.dsr.2012.11.009
Véliz C., Mujica A., Nava M. L. (2021). Hyperiid amphipods distribution between the central coast and oceanic islands off Chile, southeastern Pacific. Lat. Am. J. Aquat. Res. 49, 169–181. doi: 10.3856/vol49-issue1-fulltext-2489
Vinogradov G. (1999). “Amphipoda,” in South Atlantic Zooplankton. Ed. Boltovskoy D. (Backhuys Publishers, Leiden), 1141–1240.
Vinogradov M. E., Volkov A., Semenova T. (1996). Hyperiid amphipods (Amphipoda, Hyperiidea) of the world oceans (Lebanon: Science Publishers Inc).
Violante-Huerta M. (2019). Estructura de la comunidad de anfípodos (Crustacea: Peracarida) planctónicos del sur del Golfo de México. Universidad Nacional Autónoma de México, Mexico city.
Violante-Huerta M., Sanvicente-Añorve L., Marrón-Becerra A., Lemus-Santana E., Flores-Coto C. (2020). First records of non-hyperiid planktonic amphipods (Amphilochidea and Senticaudata) from deep waters of southwestern Gulf of Mexico, with an identification key. Rev. Mex. Biodivers. 91, e912975. doi: 10.22201/ib.20078706e.2020.91.2975
Wang L. G., Ning J. J., Li Y. F., Du F. Y. (2020). Responses of hyperiid (Amphipoda) communities to monsoon reversal in the central South China Sea. Prog. Oceanogr. 189, 102440. doi: 10.1016/j.pocean.2020.102440
Zavala-García F., Flores-Coto C., de la Luz Espinosa-Fuentes M. (2016). Relationship between zooplankton biomass and continental water discharges in the southern Gulf of Mexico, (1984-2001). Rev. Biol. Mar. Oceanogr. 51, 21–31. doi: 10.4067/S0718-19572016000100003
Zeidler W. (2003a). A review of the hyperiidean amphipod family Cystisomatidae Willemöes-Suhm 1875 (Crustacea: Amphipoda: Hyperiidea). Zootaxa 141, 1–43. doi: 10.11646/zootaxa.141.1.1
Zeidler W. (2003b). A review of the hyperiidean amphipod superfamily Vibilioidea Bowman and Gruner 1973 (Crustacea: Amphipoda: Hyperiidea). Zootaxa 280, 1–104. doi: 10.11646/zootaxa.280.1.1
Zeidler W. (2004a). A review of the families and genera of the hyperiidean amphipod superfamily Pronimoidea Bowman & Gruner 1973 (Crustacea: Amphipoda: Hyperiidae). Zootaxa 567, 1–66. doi: 10.11646/zootaxa.567.1.1
Zeidler W. (2004b). A review of the hyperiidean amphipod superfamily Lycaeopsoidea Bowman & Gruner 1973 (Crustacea: Amphipoda: Hyperiidea). Zootaxa 520, 1–18. doi: 10.11646/zootaxa.520.1.1
Zeidler W. (2006). A review of the hyperiidean amphipod superfamily Archaeoscinoidea Vinogradov, Volkov & Semenova 1982 (Crustacea: Amphipoda: Hyperiidea). Zootaxa 1125, 1–37. doi: 10.11646/zootaxa.1125.1.1
Zeidler W. (2009). A review of the hyperiidean amphipod superfamily Lanceoloidea Bowman & Gruner 1973 (Crustacea: Amphipoda: Hyperiidea). Zootaxa 2000, 1–117. doi: 10.11646/zootaxa.2000.1.1
Zeidler W. (2016). A review of the families and genera of the superfamily Platysceloidea Bowman & Gruner 1973 (Crustacea: Amphipoda: Hyperiidea), together with keys to the families, genera and species. Zootaxa 4192, 1–136. doi: 10.11646/zootaxa.4192.1.1
Zeidler W., De Broyer C. (2009). Catalogue of the Hyperiidean Amphipoda (Crustacea) of the Southern Ocean with distribution and ecological data (Brussels: Institut Royal des Sciences Naturalles de Belgique).
Keywords: water masses, diversity, hyperiidea, mesopelagic layer, stratified sampling, zooplankton
Citation: Violante-Huerta M, Sanvicente-Añorve L, Alatorre-Mendieta M and Guerra-Castro E (2025) Coarse-scale vertical distribution of pelagic amphipods in two contrasting seasons of the southern Gulf of Mexico. Front. Mar. Sci. 12:1508160. doi: 10.3389/fmars.2025.1508160
Received: 08 October 2024; Accepted: 14 January 2025;
Published: 10 February 2025.
Edited by:
Alessandro Cau, University of Cagliari, ItalyReviewed by:
Carolina E. Gonzalez, University of Concepcion, ChileCopyright © 2025 Violante-Huerta, Sanvicente-Añorve, Alatorre-Mendieta and Guerra-Castro. This is an open-access article distributed under the terms of the Creative Commons Attribution License (CC BY). The use, distribution or reproduction in other forums is permitted, provided the original author(s) and the copyright owner(s) are credited and that the original publication in this journal is cited, in accordance with accepted academic practice. No use, distribution or reproduction is permitted which does not comply with these terms.
*Correspondence: Marco Violante-Huerta, bWFyY29fdmlvbGFudGVAaG90bWFpbC5jb20=; Laura Sanvicente-Añorve, bGVzYUB1bmFtLm14
†Present address: Miguel Alatorre-Mendieta, Retired, Mexico City, Mexico
Disclaimer: All claims expressed in this article are solely those of the authors and do not necessarily represent those of their affiliated organizations, or those of the publisher, the editors and the reviewers. Any product that may be evaluated in this article or claim that may be made by its manufacturer is not guaranteed or endorsed by the publisher.
Research integrity at Frontiers
Learn more about the work of our research integrity team to safeguard the quality of each article we publish.