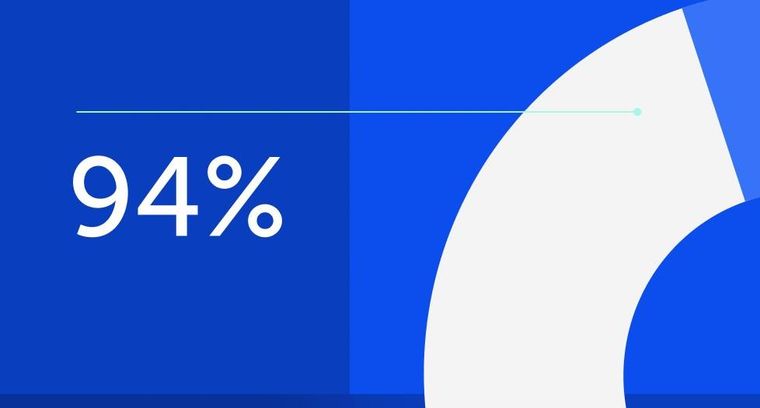
94% of researchers rate our articles as excellent or good
Learn more about the work of our research integrity team to safeguard the quality of each article we publish.
Find out more
ORIGINAL RESEARCH article
Front. Mar. Sci., 03 February 2025
Sec. Marine Fisheries, Aquaculture and Living Resources
Volume 12 - 2025 | https://doi.org/10.3389/fmars.2025.1490894
This article is part of the Research TopicFishery and aquaculture interdisciplinary integration to improve sustainable seafood productionView all 4 articles
Ecological risk assessment (ERA) has been widely used to assess species’ vulnerability to the impacts of fishing and then to prioritize any additional management actions to reduce impacts. The Ecological Risk Assessment for the Effects of the Fishing framework is based on a hierarchy of qualitative and semi-quantitative tools that work well in data-deficient situations. This study first used the Scale Intensity and Consequence (SICA) and Productive and Susceptibility Analyses (PSA) tools to evaluate the impacts of the industrial bottom trawl of southern brown shrimp on the Amazon Continental shelf in Northern Brazil. A total of 540 species were identified as having direct or indirect interaction with the trawls. The SICA identified that the main risk was related to fishing capture activities, potentially impacting the species’ population size. Of the 47 species evaluated in the PSA, 12 displayed low vulnerability, 23 displayed moderate vulnerability, and 12 displayed high vulnerability to the impacts of fishing. Future fisheries management should focus on reducing species vulnerability by prioritizing data collection for the most at-risk species. Also, fishing gear modification, such as bycatch exclusion devices (BRDs), should be employed to decrease the species’ vulnerability.
Crustaceans are one of the major commodities in the world’s fish market, accounting for approximately 22.8% of the total international trade value (FAO, 2022). In general, shrimps and prawns are one of the most valuable crustaceans, contributing to at least 16% of value trades or USD 24.7 billion in 2020, with 3.4 million tons captured (FAO, 2022). The trawl shrimp fisheries, driven by the demand and high prices of shrimps in the international markets, has become one of the most profitable fishing sectors at a global level, which has attracted the attention of the industries and increased catches (Gillett, 2008; Teixeira et al., 2020). However, despite their economic significance, these fisheries attract considerable controversy regarding sustainability (Araújo et al., 2022).
One of the main concerns associated with trawl shrimp fisheries is the ecological impact. Trawl fishing is considered one of the most destructive methods of the seabed, leading to ratings of low ecological sustainability (Isaac et al., 2009; Foster and Vincent, 2010; De Silva et al., 2022). One of the most significant impacts of trawl shrimp fisheries is the unintentional capture of non-targeted organisms during fishing activities, commonly defined as bycatch (Pérez Roda et al., 2019). The bycatch can be further divided into two groups: species that are retained on board with some value for marketing (byproduct) and the low-value unwanted species that are discarded to the sea, dead or alive (discards) (Davies et al., 2009). Discards represent around 9.1 million tons yearly, with the bottom trawls accounting for at least 45% of this total (4.2 million tons). High levels of unmanaged bycatch have the potential to close or harm the sustainability of major fisheries, affecting international markets, such as the decline of purse sine tuna fisheries in the USA and the closure of certain coastal areas in New Zealand, both due to the dolphin bycatch (Hall, 1998; Currey et al., 2012; Kirby and Ward, 2014). Most shrimp trawl fisheries have significant disproportionality catching more bycatch than shrimp, although the proportions vary worldwide (Pérez Roda et al., 2019).
Additionally, trawling affects species like marine reptiles, birds and mammals through direct capture or indirect prey removal and habitat destruction (Tasker et al., 2000; Da Rocha et al., 2021; Jog et al., 2022). These threats to biological communities occur more frequently in underdeveloped and developing countries, jeopardizing the sustainability in the long term of the business, fisheries resources, and biodiversity conservation (Lewison et al., 2004; Harrington et al., 2005). This is a common concern in highly diverse tropical regions, where shrimp trawling is seen as posing long-term threats to biodiversity (Eayrs, 2007; Farriols et al., 2017).
Information on bycatch composition and abundance is scarce in developing countries and is often not considered in management plans (Pérez Roda et al., 2019). Additionally, there is a lack of information about fishing interactions with threatened, endangered, and protected (TEP) species (Pérez Roda et al., 2019). Even information about target species is scarce or not available as open data (i.e. logbook reports), making a comprehensive assessment of the activity challenging for fisheries biologists (FAO, 2017; Peixoto et al., 2021; 2022a).
Most fishery management and sustainability efforts are underpinned by traditional single-species assessments based only on target species by measuring stock status and defining the maximum sustainable yield (MSY) limit (Rindorf et al., 2017). While this approach has become a global standard for managing fisheries and fishery stocks, it has some limitations, particularly in addressing wide impacts on ecosystems (Rindorf et al., 2017). Quantitative ecological indicators based only on a target species have been suggested as one of the causes of failures in ecosystem management (Pikitch et al., 2004). Although single-species approaches may be suitable to control the fishing effort on target species, such assessments neglect the broader ecological context, including ignoring fishing interaction in the ecosystem with other species, such as bycatch, changes in ecosystem structure and environmental issues (Cury and Christensen, 2005). These issues may also challenge fisheries sustainability (Pikitch et al., 2004; Gillett, 2008).
To address these challenges, Ecosystem-Based Fisheries Management (EBFM) emerged as a paradigm shift in fisheries management at the end of the last century and has been increasing as the new direction and the best approach for fisheries management (Nguyen, 2012). EBFM incorporates a comprehensive approach to understanding the target-species assessment, the impact on bycatch species, and broader ecological and environmental factors surrounding a fishery rather than focusing only on individual fish stocks. This is essential to integrating management measures and adaptative assessment (Pikitch et al., 2004; Webb and Smith, 2008; Hobday et al., 2011; Nguyen, 2012).
A practical semiquantitative framework to support EBFM achievement is the Ecological Risk Assessment for the Effects of Fishing (ERAEF), a semi-quantitative hierarchical approach that prioritizes higher-risk interactions (Hobday et al., 2007, 2011). This framework assesses three levels of risk of fisheries to ecosystems using qualitative (Scale Intensity Consequence Analysis – SICA), semi-quantitative (Productivity and Susceptibility Analysis – PSA), and fully quantitative (model-based) methods (Hobday et al., 2011). This method is useful in fishery data-poor scenarios with low requirement data, estimating relative risk and identifying species vulnerable to fishing impacts that require additional management attention (Hobday et al., 2011).
The industrial trawl fishery of southern brown shrimp (Penaeus subtilis) on the Amazon Continental Shelf (ACS) is a significant social and economic activity for the region, with a yearly average catch of 3,800 tons of shrimp, generating an annual income of more than 41 million USD (Araújo et al., 2022). Catches increased rapidly after the fishery commenced in the late 1950s through to 1988. Since then, catches have decreased (Aragão et al., 2015a). Different factors may contribute to this, such as instabilities in catch related to ecological and environmental factors and overcapitalization (Isaac et al., 1992; Aragão et al., 2015a; Sanz et al., 2016; Peixoto et al., 2021). Some fishery policies sought to increase fishery sustainability, consisting mainly of seasonal fishing closures and restricting the number of fleet vessels (Brasil, 1980, 2017, 2018). Despite the implementation of these measures and the good condition of the stock, with catches below MSY (Peixoto et al., 2021), the trawl shrimp fishery is still considered one of the least sustainable fisheries in the ACS (Isaac et al., 2009; Araújo et al., 2022), mostly due to its significant impacts over bycatch species, which represent up to 83% of catches, having an average ratio of 5 kg of bycatch to 1 kg of shrimp landed at ACS (Isaac and Braga, 1999; Lima et al., 2013).
While there is a considerable body of recent scientific research on various aspects of the target species (e.g., P. subtilis), on ACS (Aragão et al., 2015a, 2015b; Martins et al., 2015; Carvalho et al., 2016; França et al., 2019; Peixoto et al., 2021; 2022a; Araújo et al., 2022; Pinaya et al., 2022), studies are scarce concerning incidental catches, some of which might be facing unrecognized population impacts. Most existing studies only present a list of species caught incidentally (Cintra et al., 2017; Marceniuk et al., 2019; Cintra et al., 2020; Guimarães-Costa et al., 2020; Nóbrega et al., 2021). The lack of ecological and population data on this bycatch fauna and its interaction with the target species is of significant concern, as it can jeopardize the management approaches employed in these fisheries.
Here we applied the ERAEF framework, specifically the SICA and PSA tools, to assess two questions: i) What is the significant impact of the industrial trawl fishery of southern brown shrimp on the ecosystem in the ERAEF context? ii) Which species are most vulnerable to the impacts of fishing activities? The results may help identify the potential risk of fishery activities on the ecosystem and the vulnerability of bycatch. Efforts to quantify the negative interaction of species with fishing allow the development of appropriate management strategies to reduce mortality and plan measures that support sustainable fishing practices.
The industrial trawl fishery of southern brown shrimp (P. subtilis) operates along the ACS, northern Brazil. The study area of this work comprises the area off Amapá and Pará states, where the industrial trawl shrimp fishing effort is most intense, in depths between 15 and 95m, covering a total fishing area of 112,700 km2 (Figure 1) (Peixoto et al., 2022a).
This region is dominated by a complex and dynamic environment influenced by the discharge of the Amazon and Pará rivers (Isaac and Ferrari, 2017). The climate is characterized by two distinct periods: the rainy period from February to June, associated with high average rainfall (rainy season), and the dry period, from July to January, associated with low average rainfall (dry season) (Barthem and Schwassmann, 1994). This rainfall dynamic alternates the freshwater discharge that is responsible for the Amazon freshwater plume in the Atlantic Ocean (Barthem and Schwassmann, 1994).
The bycatch species data was collected on board industrial trawlers during commercial fishing operations. A total of 42 trawls of approximately four to six hours each were monitored during six commercial fishing trips in 2018 during the highest shrimp abundance (June) and lowest abundance (July to November). These trawls were carried out using either jib or flat trawl net type. During the fishing trips, only one trawl was monitored daily due to logistical constraints. One quarter of the total bycatch was collected from each of the 42 trawls. All the bycatch collected individuals were packaged in duly identified plastic bags and frozen on the vessel until later transported to the laboratory for identification.
Additionally, we conducted a thorough literature review to create a comprehensive and accurate list of the bycatch species of the southern brown shrimp industrial trawl fishery in this region. We also engaged in informal discussions and interviews with various stakeholders, including fishers, researchers, experts, and other individuals involved in this fishery. This collaborative effort allowed us to gather valuable insights and feedback on the fishery’s non-target species composition and features.
The ecological risk assessment for the effects of the fishing (ERAEF) framework employs a structured three-level hierarchical approach (Hobday et al., 2011). The first level, Scale Intensity and Consequence Analysis (SICA), is a comprehensive qualitative evaluation based on a literature review, expert input, and feedback from fishers to screen out potential risks. This analysis evaluates the species components’ risk from fishing activities and screens out the low-consequence activities using a conservative approach. The components identified as high risk proceed to the second level, which involves a Productivity and Susceptibility Analysis (PSA). This semiquantitative analysis assesses the species and categories them as low, moderate, and high-risk categories by their vulnerability to the effects of fishing. It estimates species’ vulnerability to overfishing, decreasing uncertainty and identifying key risk species (Hobday et al., 2007, 2011). For this study, we have completed levels 1 and 2 (Figure 2), and we will explain these aspects in greater detail in the following sections.
Figure 2. Overview of ERAEF framework showing focus on level 1 and level 2. Modified from Hobday et al. (2011).
The Scale Intensity Consequence Analysis (SICA) seeks to identify and evaluate the hazards (fishing activities) that could significantly impact the species components of the fisheries. Based on the SICA approach, hazards here are the activities undertaken in the fishing process and any external activities that can potentially lead to harm. The species components were categorized into three groups: i) target species, ii) bycatch and byproduct species, and iii) Threatened, Endangered, and Protected (TEP) species. The unit of analysis within each group is the individual species. The analysis evaluates these components based on scale (temporal and spatial) and intensity and, based on this, assigns a consequence score to measure the risk impact. To ensure that low risk components are genuinely low, SICA employs a conservative “worst-case” scenario (Hobday et al., 2007, 2011).
The analysis includes a set of 32 hazards used to identify what hazard occurs in the fishery under analysis and should, therefore, undergo evaluation in the SICA (Supplementary Material 1; Figure 3). The SICA process uses attribute tables and is only applied to the “worse-case” case unit of each species component analyzed for each hazard identified, allowing focus on the most plausible high risk scenario (Supplementary Material 2). When there is limited or uncertain information about the potential risk, the logical approach was employed to choose the plausible highest risk score (Hobday et al., 2011). The scale, intensity and consequence were assigned for each hazard identified in this fishery that might adversely affect the species’ components. The consequence score scale ranges from 1 to 6, with 1: negligible, 2: minor, 3: moderate, 4: major, 5: severe, and 6: intolerable. The confidence of the consequence score is rated either low (1) or high (2), according to the level of scientific knowledge (peer-reviewed papers, books and reports). Both scores were obtained following the reference guide from Hobday et al. (2007) to score the risk’s scale, intensity, and consequence. The findings of the SICA assessment are presented in the report summary Table (Level 1, SICA), which includes the consequence scores for all combinations of activities and components (Supplementary Material 3). The species components were assessed at Level 2 (PSA) with a consequence score of 3 or higher.
Figure 3. Generic overview of the risk of activities undertaken by the fishing process (Direct impact of fishing) subdivided into fishing activity following the ERAEF method.
The Productivity and Susceptibility Analysis (PSA) was used to evaluate the vulnerability (v) of the stocks. In this context, vulnerability is defined as a function between productivity (P) and susceptibility (S) attributes. It refers to a level of impact from fishing in which fishing mortality can exceed the capacity of a species to renew itself, thereby identifying which unit of analysis are most vulnerable to overfishing (Stobutzki et al., 2002; Patrick et al., 2010; Hobday et al., 2011). This analysis considers two distinct sets of attributes: P, which encompasses the species’ life history characteristics, and S, which reflects the potential reduction or removal of a population (Patrick et al., 2010; Hobday et al., 2011). The underlying premise of this approach is that evaluating the relationship between P and S can generate a single score that may be used to quantify the risk of stock to overexploitation (Hobday et al., 2011; Stobutzki et al., 2001a; 2001b; 2002). The three-level system risk categories were defined for each attribute P and S by using a quantile method (Lira et al., 2022). The three categories range from 1 (low risk - high productivity and/or low susceptibility) to 3 (high risk - low productivity and/or high susceptibility) (Patrick et al., 2010; Hobday et al., 2011). These scores were assigned for each attribute (Tables 1, 2).
Table 1. Productivity attributes and rankings used to estimate the vulnerability of stock caught in the industrial bottom trawl shrimp fishing along the Amazon continental shelf.
Table 2. Susceptibility attributes and thresholds score used to estimate the vulnerability of stock caught in the industrial bottom trawl shrimp fishing along the Amazon Continental Shelf.
The overall v score was calculated for each stock. This score was obtained by the calculation of the Euclidean distance of the weighted P and S scores according to the following equation:
where P is the weighted productivity score, S is the total susceptibility score, v is the vulnerability score, and X0 and Y0 are the biplot’s origin coordinates.
According to the ERAEF framework, all species identified at the SICA level should ideally be assessed at the PSA level. However, the SICA assessment identified an exceptionally high number of species, making it nearly impossible to assess each one individually due to resource constraints. To manage this, we developed additional criteria to narrow down and prioritize species for the PSA assessment. For PSA, 47 species were classified from an initial list of 107 species based on one or more criteria: (i) most vulnerable components following the SICA, (ii) elasmobranchs, due to their life history characteristics, elasmobranchs are naturally vulnerable to fishing impacts. These species typically have slow growth rates, late maturity, and low reproductive output, making them more vulnerable to overfishing. Additionally, there is a lack of stock assessments and sufficient data for these species, which limits our ability to evaluate their population status effectively. This inherent vulnerability and data deficiency justified their inclusion in the selection criteria; (iii) commercial species, the term “commercial species”, refers to species that are not the primary target of the fishery but have market value and are, therefore, retained on the boat for sale. These species are typically kept and sold as by-products, even though they are not the main focus of the fishery; (iv) frequent and abundant species, according to Garcia and Vieira-Sobrinho (2001), the species “frequent and abundant” observed in the trawls; and (v) species classified as critically endangered (CR), endangered (EN), vulnerable (VU), near threatened (NT), data deficient (DD) or not evaluated (NE) according to The International Union for Conservation of Nature’s Red List (IUCN) and through the regional evaluation performed by the Instituto Chico Mendes de Conservação da Biodiversidade (Instituto Chico Mendes de Conservação da Biodiversidade, 2018).
Eight life-history traits-related attributes were chosen to be employed in the productivity analyses of PSA. The levels of the high, medium and low risk threshold scores for the seven attributes are classified below (Table 1). Regional literature was prioritized for obtaining species parameters whenever possible; when unavailable, studies from other regions were consulted. The Supplementary Material (Supplementary Material 4) provides empirical calculations of the attribute values.
1. von Bertalanffy somatic growth coefficient (k, year-1): The mean rate at which a species reaches its maximal size. It correlates positively with production; the higher is k value, more productive a stock is. Short-lived species’ productivity and k value are higher than those of long-lived species (Patrick et al., 2010; von Bertalanffy, 1957). When the growth coefficient (k) was not available in the literature, it was estimated using the empirical equation of Le Quesne and Jennings (2012), details of the equations used are given in Supplementary Material 4.
2. Maximum size (Lmax, cm): A species’ maximum size and productivity are inversely correlated; large species have low productivity due to their long life span (Roberts and Hawkins, 1999; Patrick et al., 2010). The values were obtained from the literature. Regional literature was prioritized for obtaining species parameters whenever possible; when unavailable, studies from other regions were consulted.
3. Size at first-maturity length (L50, cm): The mean total length at which 50% of individuals can produce offspring. Since long-lived species with slow growth take longer to achieve L50, this metric negatively correlates with productivity (Beverton and Holt, 1957). The values were obtained from the literature; when unavailable, the value was estimated according to Binohlan and Froese (2009) based on the maximum total length (Lmax), details of the equations used are given in Supplementary Material 4.
4. Intrinsic rate of population growth (r, year-1): This is the highest population growth possible without fishing. The attribute has a positive correlation with productivity. High values of r indicate high productivity (von Bertalanffy, 1938). The values were obtained from the literature. When not available, the value was estimated using the equation proposed by Mertz (1970) using a life table. The life table was calculated based on the natural mortality (M) estimates developed by Gislason et al. (2010), details of the equations used are given in Supplementary Material 4.
5. Mean trophic level (TL): Defines the level of each species in the food chain. The TL is negatively associated with productivity because higher trophic levels are less productive than lower ones (Lindeman, 1942; Pauly et al., 1998; Patrick et al., 2010). To standardize the technique used to determine trophic level values, all data were obtained from FishBase, which incorporates information on trophic ecology, habitat, diet, food consumption, diet composition, and predators of various fish species (Froese and Pauly, 2000).
6. Maximum age (Amax, year): Maximum age reported. Negatively linked with productivity. High values may indicate low productivity. The values were obtained from literature. Taylor’s (1960) empirical equation (Amax=k+(2.996/t0) was used to calculate the value of Amax, when the information were not available in the literature, as detailed in Supplementary Material 4.
7. Natural mortality (M): The rate (year−1) of deaths from all causes except fishing (e.g., disease, predation, and starvation). Highly productive species spend more energy on the quantity than the quality of their spawn, resulting in high mortality (positive correlation). We used the application developed by Jason Cope to calculate mortality (Cope and Hamel, 2022). Details of the equations used are given in Supplementary Material 4.
8. Breeding strategy (BS): The qualitative attribute, represents the level of mortality expected in the early stages of life (Patrick et al., 2010), based on characteristics of parental health investment, described by Winemiller (1989) and modified by King and McFarlane (2003): i) placement of zygotes or larvae (from 0 to 2): e.g. no placement or maintenance in a nest; ii) parental protection of zygotes or larvae (from 0 to 4) and iii) nutritional contribution (from 0 to 8). Relation to the attribute: 0 = high - species with no placement of zygotes or larvae, parental protection and nutritional contribution; 1 to 3 = moderate - species with few of these characteristics; 4 to 14 = low = species with some of these characteristics.
Seven attributes related to distribution, abundance, and fishery aspects were selected to employ in the PSA. The details of the scores are shown in Table 2, and the attributes are described in depth below.
1. Frequency of occurrence and abundance (FOA): The classification was adapted from Garcia and Vieira-Sobrinho (2001). Species with a catch percentage (PN%) greater than the ratio 100/S, where S is the number of species present in the trawls set, were considered abundant. Species with a frequency of occurrence (FO%) greater than 50% were considered frequent.
2. Overlap area (OA): This vulnerability attribute relates the overlap between the species’ distribution and preferred habitat with fishing areas. For example, pelagic species or those with a preferred habitat in reef and coral areas will have lower vulnerability to trawling. In contrast, demersal species that occur primarily on muddy bottoms will have a higher probability of capture.
3. Value of the fishery: Greater effort is expected on populations with higher economic value, which may make them more susceptible to overfishing. The value of the fishery was determined using the price of initial commercialization per kilogram. The information for classifying the species was obtained from the literature, knowledge of the price, and use of the species for livelihoods in the studied area (Hilborn et al., 2003; Anderson et al., 2008; Pauly and Zeller, 2016).
4. Spawning Potential Ratio (SPR): This model calculates the proportion of unfished spawning potential under any level of fishing pressure (Walters and Martell, 2004; Patrick et al., 2010; Hordyk et al., 2015). The SPR was calculated using the LB-SPR method, which uses estimates of the natural mortality rate (M) in relation to the parameter K of the von Bertalanffy growth equation (M/K) to estimate the spawning potential (SPR) from data on the size composition of an exploited stock (Hordyk et al., 2016). Details of the equations used are given in Supplementary Material 4.
5. Mixed Trophic Impact (MTI): The MTI was calculated using a trophic model developed using Ecopath with Ecosim model (EwE) (Christensen et al., 2005). The index represents the impacts of one species/group of species on the biomass of another species or group of species (Christensen et al., 2008), considering natural mortality (M) and mortality caused by fishing (F) (Ulanowicz and Puccia, 1990). The MTI was calculated using a trophic model developed using Ecopath with Ecosim model (EwE) (Christensen et al., 2005). The trophic model EwE developed by Isaac and Frédou (2022) simulates the effect of fishing on the trophic chain, assuming the impact of the increase or decrease in the target species’ population on other species or groups of species. Negative values of MTI from a fleet in a species indicate a negative impact due to fishing and, consequently, greater susceptibility (Christensen et al., 2005).
6. Percentage of individuals >L50: Total average length that 50% of the individuals can reproduce. Considering that species that are long-lived and slow growing spend more time to reach L50, this attribute is inversely proportional. The excessive capture of immature individuals leads to a decline in the reproductive population, defining overfishing by recruitment. This reduction undermines the species’ potential for regeneration and can ultimately result in the collapse of fish stocks. When L50 values were unavailable in the literature, the size at first maturity was estimated using the relationship proposed by Froese and Binohlan (2000).
7. Aggregation behavior: The attributes include observing species behaviors that can affect catchabilities, such as schooling and aggregation (Patrick et al., 2010; Freitas et al., 2023). These behavioral responses are considered in the catch and literature.
The correlation between the productivity and susceptibility attributes and the species’ vulnerability will be different between them and variable according to the environment. Therefore, to consider the influence of each attribute on vulnerability, a simulation was carried out giving different weights to the attributes (weights 1, 2 and 3) to infer the variability of vulnerability according to the weight given to each attribute. The attributes were weighted according to Lucena-Frédou et al. (2017) to calculate the vulnerability. Productivity and susceptibility attributes were given a weight of 2, except for the productivity attributes (Lmax, k, and r), which received a weight of 3 since they are key population parameters in the species’ life history strategy.
Redundancy among attributes was not assessed since no relevant changes among assigned risks and vulnerability ranking were observed in previous studies (Lucena-Frédou et al., 2017; Lira et al., 2022). The species is given a vulnerability value from 1 to 3. This value is ranked and can be classified as high, medium or low risk. The weight could be subjective if applied equally to each attribute since each attribute can impact the species’ vulnerability differently. Applying simulations with different weights and calculating the range of productivity and susceptibility values, and consequently, the species’ risk, reduces the sensitivity of the approach applied. Therefore, to reduce the model uncertainties caused by the weight subjectivity, we performed 10,000 random simulations, assigning weights from 1 to 3 for all attributes. The standard deviation of the vulnerability values was calculated through the simulations. Thus, it was possible to evaluate whether there was a change in the risk classification (low, medium, high) according to the weights attributed, decreasing model uncertainties. All analyses were performed using the R environment (R Core Team v4.2.2, 2020).
The trawl fishery on ACS targets the southern brown shrimp P. subtilis. In this study, 203 species were recorded across all monitored trawls during the sample collection. As a result of the extensive scientific literature review, stakeholder interviews and observations, additionally, 337 species were identified as bycatch or somehow associated with this fishery (Supplementary Material 5), which have either direct or indirect interaction with industrial trawl fisheries on the ACS. Direct interaction refers to species physically collected in the trawls during monitoring. Indirect interaction relates to species that are associated with the trawl fisheries but were not collected in the trawls themselves. All these species compose 10 taxa distributed in seven phyla, 20 classes, 53 orders, and 170 families (Figure 4). The fish species dominate this composition, accounting for approximately 63% of the species composition, 93.1% belonging to the class Actinopterygii and 6.9% to Elasmobranchii. The IUCN red list has assessed 333 species out of the 540, 277 are categorized as least concern (LC), and 56 are in some risk category; most are Elasmobranchii species (Figure 5). Furthermore, the ICMBio threatened Brazilian species list has evaluated 40 of the 540 species. Among these, 19 are in the LC category, while 21 are in some risk category (Figure 5). About 7.66% of the species caught are byproducts, and the vast majority (92.15%) have no commercial value and are discarded back to sea.
Figure 4. Frequency of occurrence of taxonomic groups present in the industrial shrimp trawling on the Amazon Continental Shelf.
Figure 5. Relative frequency of species occurrence in the industrial shrimp trawling fishery on the Amazon Continental Shelf on the red list of threatened animals of IUCN and Brazilian threatened species list. LC, Least Concern; DD, Data Deficient; VU, Vulnerable; NT, Near Threatened; CR, Critically Endangered; EN, Endangered.
Out of the total 32 fishing activities assessed, the SICA analysis identified 22 activities to occur in the industrial trawl shrimp fishing operations on the ACS (Supplementary Material 1). Following the SICA analysis across three species components, the main risk identified was related to the fishing capture activities, potentially impacting the species’ population size (Supplementary Material 2). The most important impact falls on species in some IUCN or ICMBio risk categories (TEP species). The most vulnerable species belong to the class Elasmobranchii. There are also cumulative impacts from other fisheries that share the same fishing ground, like the red snapper fishery and multispecies industrial trawl fisheries. Sharks and grouper species were highlighted as high-risk due to being caught as a byproduct in different fisheries, like red snapper fishery, an industrial trawl of laulau catfish, and multispecies industrial trawl fishery in the ACS, which can affect the population size. There was high confidence in the risks assigned to the target species. In contrast, confidence levels were low in bycatch, byproduct, and TEP species due to a lack of information and high uncertainty associated with the confidence score (Figure 6).
Figure 6. Frequency of consequence scores and confidence levels (high and low) across fishery and external activities for (A) target, (B) Bycatch and Byproduct, and (C) TEP species present in industrial trawl shrimp fishery on the Amazon Continental Shelf.
From the extensive list of 540 species associated with the trawl shrimp fishery, 47 species were classified from an initial list, to be evaluated with the PSA (Figure 7; Table 3). The range of productivity values varied from 1 to 3, while susceptibility values varied from 1.29 to 2.71. The vulnerability score encompassed a broad spectrum, ranging from the lowest value of 0.30 (Haemulopsis corvinaeformis) to the highest of 2.29 (Mustelus canis). The target species, P. subtilis, exhibited moderate vulnerability, with an average score of 1.71. Among the species, 25.53% (12 species) displayed low vulnerability, 48.93% (23 species) displayed moderate vulnerability, and 25.53% (12 species) displayed high vulnerability. Most of the bycatch showed moderate (15 species) and low (12 species) vulnerability. A total of 15 byproduct species evaluated, showed moderate (7 species) to high (8 species) vulnerability. All the species categorized under the conservation status of CR, EN and VU exhibited high vulnerability levels, emphasizing their susceptibility to fishing impact.
Figure 7. Overall distribution of the vulnerability (risk) scores of 47 stocks in the industrial shrimp trawl- fishery on the Amazon Continental Shelf. The plot is divided into three groups by the lines and the color scale reflecting the gradient of vulnerability values, with blue being low, yellow moderate and red being high. The black arrow is the vulnerability categories from low to high. The range lines at each point show the standard deviation for each productivity and susceptibility axis with 10,000 runs. Abbreviations of each species are in Table 3.
Table 3. The summary of the 47 species assessed in PSA according to the role in the fishery (TA, Target; BP, Byproduct; BC, Bycatch), category of IUCN list and risk category, X: when the species were not evaluated by IUCN.
Only ten species of the 47 are still in the same risk category after simulation with different weights assignment (Figure 8). Among the 12 species classified as high risk, 90% of them still have the probability of remaining into this category. Conversely, the low risk species exhibited more uncertainty, with six out of 12 species having an 80% or higher probability of being categorized as low risk. Most species fell into the moderate vulnerability category, displaying minimal uncertainty in their classification (Figure 8).
Figure 8. The overall probability of risk from the uncertainty simulations of 47 stocks explored by industrial shrimp trawling along the Amazon continental shelf. Species are distributed from left to right, according to the vulnerability rank: low (blue), moderate (yellow), and high (red). Abbreviations of species are in Table 3.
Overfishing, and in particular, ecosystem overfishing, has been reported as one of the most frequent human impacts on the oceans, causing irreparable harm and population loss (Jackson et al., 2001; Coll et al., 2008; McCauley et al., 2015; Pacoureau et al., 2021). This issue is especially critical for bycatch species, as the scientific information on population parameters and the conservation status of species caught incidentally is limited, being a challenge for evaluating sustainability (Zhou et al., 2009). The high diversity of species in the industrial trawls in the tropics and insufficient data make traditional single-stock assessments impractical (Beddington et al., 2007; Nguyen, 2012).
Innovative approaches like the ERAEF framework have emerged to address these challenges. The ERAEF framework is designed for low-data scenarios, considering both target species and other elements of the ecosystem’s biological dimension (Pikitch et al., 2004; Smith et al., 2007; Patrick et al., 2010; Hobday et al., 2011). The tools within this framework, such as SICA and PSA, integrate qualitative and quantitative data from various sources, enabling the assessment and ranking of species most affected by fishing activities (Patrick et al., 2010; Hobday et al., 2011; Ormseth and Spencer, 2011; Osio et al., 2015). These methods are particularly effective in data-poor fisheries with high species diversity. Although they are not intended to replace traditional stock assessments or provide reference points, they can be an alternative to assess the species (Stobutzki et al., 2001a; Zhou et al., 2016; Lucena-Frédou et al., 2017).
Understanding the compositions of catches is crucial for assessing how fishing practices affect aquatic organisms (Link, 2002). By identifying and measuring species vulnerabilities, these tools provide crucial information for policymakers and stakeholders (Hobday et al., 2011; Zhou et al., 2016). These tools help identify species requiring immediate conservation attention, guiding efforts to protect the most impacted marine populations and improve their conservation status (Lucena-Frédou et al., 2017; Peixoto et al., 2022b; Griffiths et al., 2024).
Our research reveals that industrial shrimp trawl catches in the ACS region consists of approximately 540 species. Studies from various regions highlight the diversity of the bycatch species globally. For instance, recent assessments in the northeastern Brazil reported by Freitas et al. (2023) and Lira et al. (2022) documented 119 and 90 fish bycatch species, respectively. In other regions, Rábago-Quiroz et al. (2012) documented 243 species in the Gulf of California, Mexico, Mendo et al. (2022) identified 277 species in Northern Peru, Abdulqader et al. (2020) recorded 104 species in the Arabian Gulf, Saudi Arabia, and Rodrigues-Filho et al. (2020) found 149 species in southern Brazil. The number of species in the ACS region is richer and more diverse than previous estimates from other trawl shrimp fisheries in tropical and subtropical regions worldwide.
This higher diversity can be attributed to several factors. The industrial scale and greater fishing power of the ACS fleet contrast with the predominantly artisanal fisheries in other regions of Brazil. Additionally, the high productivity of ACS waters is mostly influenced by the massive discharge from the Amazon basin, which creates a dynamic freshwater plume in the Atlantic Ocean that function as a dispersal system, influencing salinity, nutrient distribution, and sediments levels, creating highly dynamic and productivity environment in this area (Nobre and Molion, 1986; Gallo and Vinzon, 2005; Isaac and Ferrari, 2017; Nittrouer et al., 2021). The nutrients from the Amazon supports this biodiversity, enhances the ecological richness and sustains multiple fisheries at the mouth of the Amazon River (Weber et al., 2017).
Additionally, in this study, we included species that could potentially have negative interactions with the fishing activities, such as birds that alter their behavior by following the fleet and the possibility of collisions with mammals (Tasker et al., 2000; Da Rocha et al., 2021; Jog et al., 2022). Although not directly caught in the trawling net, they may have potentially harmful interactions and be indirectly affected (Hobday et al., 2007). This approach, often overlooked, emphasize the need for a broader view of the species affected by trawl fishing activities, tailored conservation and management strategies.
The SICA analysis has highlighted the major impacts within this fishery, particularly the high rate of incidental catch, including probably a significant proportion of juveniles (Isaac and Frédou, 2022), affecting the population size of those populations, and the TEP species being the most affected. This is primarily due to the low selectivity of trawl gear, a common problem in shrimp trawling that leads to an unintentional capture of a wide range of non-target species, increasing bycatch levels and impacting marine populations (Davies et al., 2009; Marceniuk et al., 2019; Pérez Roda et al., 2019). Our assessment also identified direct and indirect adverse effects of trawl capture, such as seabed disruption, negatively impacting demersal and benthic species, and cumulative impacts from different fishing fleets sharing the same grounds (Thrush and Dyton, 2002; Clark et al., 2016). Trawl fisheries are widely recognized as highly destructive (Foden et al., 2011; Paradinas et al., 2016). Bottom trawls, in particular, contribute to sediment resuspension and seabed scraping, increasing mortality rates among benthic organisms, such as polychaetes, sponges and rays (Guimarães-Costa et al., 2020; Nóbrega et al., 2021). These impacts can alter community diversity and biomass patterns, influencing nutrient flows in trophic webs (Dellapenna et al., 2006; Kaiser et al., 2006; Sciberras et al., 2018; Hiddink et al., 2019; McConnaughey et al., 2020). Moreover, the pressure on stocks intensifies as different fishery fleets share fishing areas or boundaries, catching the same bycatch species. For instance, industrial and artisanal fleets operate at various levels along the ACS, making management difficult. This overlapping of fishing fleets with different fishing gear, such as trawls, long lines, traps and nets, can intensify cumulative bycatch mortality, habitat degradation and reduce species resilience (Hall et al., 2000; Zhou et al., 2019). Future assessments should encompass all industrial, semi-industrial, and artisanal fisheries that operate along the ACS region. This would allow a comprehensive evaluation of cumulative impacts on shared species across multiple fisheries and facilitate exploring marine spatial planning as a potential management approach (Halpern et al., 2008; Zhou et al., 2019).
Another issue within the shrimp trawl fleet is the illegal practice of shark fining, where the crew uses hooks and lines to catch sharks while anchored at night. The fins, a highly economic commodity in the Asian market, are collected, while the shark’s carcass is discarded (Clarke, 2004; Clarke et al., 2007). This practice of fin fishing, which is illegal in Brazil (Brasil, 2012), persists and increases fishing pressure on sharks. This subproduct market is a common practice in the ACS, species like Cynoscion acoupa are explored for high-value subproducts, such as the gas bladder (fish maw), a which surpasses the value of the meat (Moura et al., 2023). These practices are secretive and part of the illegal, unreported and unregulated (IUU) fishing market in the ACS, driven mainly by the high demand of the Asian market. The ACS is home to about 34 species of sharks and 36 batoid, some of them endemic to this area and in some risk categories by the IUCN and ICMBio red list (Dulvy et al., 2014; Marceniuk et al., 2019). The lack of studies quantifying IUU and subproduct value chains, combined with insufficient enforcement in this region, threatens species conservation. These issues must be addressed to ensure sustainable management practices.
The conservative approach of SICA is mainly due to the variance in data reliability across different species groups. Information on target species, such as the ecological and population parameters, is generally more reliable and well-documented due to more intensive studies (Isaac et al., 1992; Ehrhardt et al., 1999; Cintra et al., 2004; Aragão, 2012; Aragão et al., 2015a; 2015b; Peixoto et al., 2021; Peixoto et al., 2022a). In contrast, information on bycatch, byproduct and TEP species is often based on expert and fisher knowledge, which, while valuable, might introduce uncertainty due to limited empirical validation. Most studies on bycatch focus on species composition (Marceniuk et al., 2019; Lucena-Frédou et al., 2017; Guimarães-Costa et al., 2020; Nóbrega et al., 2021). The results should be interpreted with caution due to using a conservative approach in the absence of data to avoid potential underestimation.
In our PSA, 47 species from industrial shrimp trawling were assessed. All species in the low-risk category belong to the bycatch component and are classified as LC at the IUCN or ICMBio red list. This species tends to be pelagic, benthopelagic, or small-sized species, typically exhibits high productivity, with high fecundity rates, fast growth, and faster turnover of generations, and broad geographic distributions, all these traits contribute to high resilience and reducing their susceptibility (Mohamed et al., 2021). The target species, southern brown shrimp, showed moderate risk, consistent with previous traditional stock assessments. This assessment indicates that although fishing mortality is high, the exploitation levels are still managed below the MSY (Peixoto et al., 2021). This might reflect the single-species management approach focused on the target species, neglecting the non-target species that receive the most significant impacts on the fishery (Freitas et al., 2023).
Conversely, species like sharks, rays, and TEP species tend to exhibit moderate and high-risk. This is mainly due to the combination of low productivity traits due to slow growth, long lifespans, low fecundity late maturation, and high susceptibility due to high abundance in the bottom trawl, the overlap of ground fishing and high captures of young individuals, making them highly vulnerable (Rigby and Simpfendorfer, 2015; Lira et al., 2022). These results align with other PSA assessments around the globe (Cortés et al., 2010; Osio et al., 2015; Lin et al., 2020; Georgeson et al., 2020; Peixoto et al., 2022b).
As in most fisheries assessments in developing countries, the lack of data on many species caught by trawl shrimp fisheries in the ACS presents a major challenge. One of the main difficulties in this work was the uncertainty, limitation, or lack of data for non-target species. Basic ecology and biology information, such as population parameters, fecundity, larval stages, life span, and reproduction, is often missing for most of the bycatch. This information is usually available only to targets or species with economic value (Lucena-Frédou et al., 2016, 2017). In such cases, data from closely related taxa or other regions, or precautionary high scores, are used, though this can introduce bias (Cope et al., 2011; Hobday et al., 2011). Despite their limitations, these tools used in this work are effective in data-limited fisheries to highlight high risk species for management measures. They provide valuable information on identifying priority stocks for managing and making decisions when quantitative analyses, as stock assessments are unavailable due to the lack of data (Murua et al., 2009; Patrick et al., 2010; Cortés et al., 2010; Zhou et al., 2011; Lucena-Frédou et al., 2017; Griffiths et al., 2019; Previero and Gasalla, 2019; Lin et al., 2020; Georgeson et al., 2020; Peixoto et al., 2022b; Fatema et al., 2022; Freitas et al., 2023).
Future fisheries management should focus on reducing species vulnerability by prioritizing data collection on species life-history traits for the most at-risk species. Comprehensive research on non-target species and mandatory data collection for high risk species are essential for performing more quantitative approaches as eSAFE, and EASI-Fish, for cumulative fishing mortality impacts, or stock assessments, for reference points (Hordyk and Carruthers, 2018; Griffiths et al., 2019; Zhou et al., 2019). These further analyses and discussions could help address specific gear modifications, such as bycatch reduction devices to avoid high-risk species (Lin et al., 2020). Public policies must make it easier for companies to adopt these technological modifications that allow for more ecologically sustainable fisheries. Policies that provide economic incentives, such as tax breaks, grants for equipment upgrades, or certifications for eco-friendly fishing practices, can encourage companies to adopt gear modifications. Bycatch reduction devices (BRD) have been known in the literature for decades (Brewer et al., 1998; Watson et al., 1999; Eayrs, 2007). However, its practical implementation has been a major problem in Brazil due to the difficulty in accepting boat owners in its use, either for fear of reducing catches of the target species or due to the costs of modifying the gears (Guanais et al., 2015). Integrating research with outreach and training initiatives to help understand both the environmental benefits and the long-term economic gains from reducing bycatch may be a way for encourage boat owners to engage in the voluntary use of these technologies (Mesquita and Medeiros, 2023).
The original contributions presented in the study are included in the article/Supplementary Material. Further inquiries can be directed to the corresponding author.
Ethical approval was not required for the study involving animals in accordance with the local legislation and institutional requirements because Since the data is derived from industrial fishing of southern brown shrimp on the Amazon continental shelf with bycatch species, which are unintentionally caught by commercial shrimp fishing and discarded back into the sea, frequently already dead, the permission of the ethics committee was unnecessary.
UP: Conceptualization, Data curation, Formal analysis, Investigation, Methodology, Writing – original draft. MS: Formal analysis, Methodology, Supervision, Writing – review & editing. AH: Formal analysis, Methodology, Supervision, Writing – review & editing. BB: Methodology, Project administration, Supervision, Writing – review & editing. RP: Formal analysis, Writing – review & editing. FF: Funding acquisition, Project administration, Writing – review & editing. VI: Funding acquisition, Project administration, Resources, Supervision, Writing – review & editing.
The author(s) declare that financial support was received for the research, authorship, and/or publication of this article. This research was funded by the Project “Shrimp_NEN: Rede cooperativa multidisciplinar de apoio à gestão de estoques de camarão no Norte e Nordeste do Brasil com foco ecossistêmico” Process 445766/2015-8 MCTI/MPA/CNPq and “Camarões do Norte: Economia e valoração ambiental do uso de dispositivos de exclusão de fauna acompanhante na pesca industrial do camarão rosa na Plataforma Norte do Brasil” chamada 8/2022, 26/2023. First author funded by PosDoc Fellowship (ref.: 168398/2022-2). The Article Processing Charges were granted by the Programade Apoio à Publicação Qualificada (PAPQ) of the UniversidadeFederal do Pará (UFPA).
The authors declare that the research was conducted in the absence of any commercial or financial relationships that could be construed as a potential conflict of interest.
All claims expressed in this article are solely those of the authors and do not necessarily represent those of their affiliated organizations, or those of the publisher, the editors and the reviewers. Any product that may be evaluated in this article, or claim that may be made by its manufacturer, is not guaranteed or endorsed by the publisher.
The Supplementary Material for this article can be found online at: https://www.frontiersin.org/articles/10.3389/fmars.2025.1490894/full#supplementary-material
Abdulqader E. A., Abdurahiman P., Mansour L., Harrath A. H., Qurban M. A., Rabaoui L. (2020). Bycatch and discards of shrimp trawling in the Saudi waters of the Arabian Gulf: Ecosystem impact assessment and implications for a sustainable fishery management. Fisheries Res. 229, 105596. doi: 10.1016/j.fishres.2020.105596
Anderson C. N. K., Hsieh C. H., Sandin S. A., Hewitt R., Hollowed A., Beddington J., et al. (2008). Why fishing magnifies fluctuations in fish abundance. Nature 452, 835–839. doi: 10.1038/nature06851
Aragão J. A. N. (2012). Dinâmica populacional e avaliação do estoque do camarão rosa (Farfantepenaeus subtilis Pérez Farfante 1967) na plataforma continental amazônica brasileira. [Tese de Doutorado]. Escola de Engenharia de São Carlos da Universidade de São Paulo, São Carlos (SP.
Aragão J. A. N., Cintra I. H. A., Silva K. C. A. (2015a). Situação da pesca de camarões na plataforma continental amazônica. Acta Fisheries Aquat. Resources 3, 61–76. doi: 10.2312/Actafish.2015.3.2.61-76
Aragão J. A. N., Silva K. C. A., Cintra I. H. A. (2015b). Pesca Industrial do camarão-rosa na Plataforma Continental Amazônica: aspectos da dinâmica da população, avaliação do estoque e influência dos parâmetros ambientais. Acta Fisheries Aquat. Resources 3, 77–90. doi: 10.2312/Actafish.2015.3.1.77-90
Araújo J. G., Mello Filho A., Peixoto U. I., Bentes B., Santos M., Dutka-Gianelli J., et al. (2022). Multidimensional evaluation of brown shrimp trawling fisheries on the amazon continental shelf. Front. Mar. Sci. 9. doi: 10.3389/fmars.2022.801758
Barthem R. B., Schwassmann H. O. (1994). The Amazon river influence over the seasonal displacement of the salty wedges in Tocantins estuary, Brazil 1983-1985. Boletim do Museu Paraense Emílio Goeldi. 10, 119–130. Available at: http://repositorio.museu-goeldi.br/handle/mgoeldi/496.
Beddington J. R., Agnew D. J., Clark C. W. (2007). Current problems in the management of marine fisheries. Science 316, 1713–1716. doi: 10.1126/science.1137362
Beverton R. J. H., Holt S. J. (1957). On the Dynamics of Exploited Fish Populations (Londres: Chapman & Hall).
Binohlan C., Froese R. (2009). Empirical equations for estimating maximum length from length at first maturity J. Appl. Ichthyol. 25, 611–613. doi: 10.1111/j.1439-0426.2009.01317.x
Brasil (1980). Portaria SUDEPE n° N-007 de 25 de fevereiro de 1980. Available online at: https://www.icmbio.gov.br/cepsul/images/stories/legislacao/Portaria/1980/p_sudepe_07_n_1980_limitabarcosarrastoparapescacamaraorosa_regiaonorte.pdf (Accessed May 29th, 2020).
Brasil (2012). Instrução Normativa Interministerial MPA/MMA N° 14 de 26 de novembro de 2012. Available online at: https://www.icmbio.gov.br/cepsul/images/stories/legislacao/Instrucao_normativa/2012/in_inter_mpa_mma_14_2012_normasprocedimentoscapturatubaroes_raias.pdf (Accessed November 24th, 2020).
Brasil (2017). Portaria Interministerial n° 75, de 20 de dezembro de 2017. Available online at: https://www.gov.br/agricultura/pt-br/assuntos/aquicultura-e-pesca/legislacao/defesos/portaria-interministerial-mdic-mma-no-75_12_2017.pdf/view (Accessed July 25, 2020).
Brasil (2018). Portaria Interministerial MDIC/MMA n° 15, de 10 de janeiro de 2018. Available online at: https://www.gov.br/agricultura/pt-br/assuntos/aquicultura-e-pesca/legislacao/arrasto/portaria-mdic-mma-no-15-de-10-01-2018.pdf/view (Accessed July 25th, 2020).
Brewer D., Rawlinson N., Eayrs S., Burridge C. (1998). An assessment of bycatch reduction devices in a tropical Australian prawn trawl fishery. Fisheries Res. 36, 195–215. doi: 10.1016/S0165-7836(98)00096-4
Carvalho A. S. S., Martinelli-Lemos J. M., Nevis A. B., Isaac V. (2016). Spatio-temporal variation of thedensity of shrimps Farfantepenaeus subtilis, Litopenaeus schmitti and Xiphopenaeus kroyeri (Crustacea;Decapoda) in the Curuçá estuary, north of Brazil. Boletim do Instituto Pesca 42, 598–610. doi: 10.20950/1678-2305.2016v42n3p598
Christensen V., Walters C. J., Pauly D. (2005). Ecopath with Ecosim: a user’s guide. (Vancouver: Fisheries Centre, University of British Columbia), 31. Available online at: http://citeseerx.ist.psu.edu/viewdoc/download?doi=10.1.1.122.6467&rep=rep1&type=pdf.
Cintra I. H. A., Aragão J. A. N., Silva K. C. A. (2004). Maturação gonadal do camarão-rosa, Farfantepenaeus subtilis (Pérez-Farfante 1967), na região norte do Brasil. Boletim Técnico Cientifico do CEPNOR. Belém 4, 21–29.
Cintra I. H. A., Paiva K. S., Herrmann M., Martins J. C., Klautau A. G. C. M., Silva K. C. A. (2017). Carcinofauna acompanhante do camarão-rosa em pescarias industriais na plataforma continental amazônica. ACTAPESCA 5, 83–91.
Cintra I. H. A., Paiva K. d. S., Silva K. C. d. A., Martins D. E. G., Klautau A. G. C. d., dos Santos W. C. R., et al. (2020). On the carcino-group bycatch in the industrial fishery for southern brown shrimp on the Amazon continental shelf. Res. Soc. Dev. 9, e110985508. doi: 10.33448/rsd-v9i8.5508
Clark M. R., Althaus F., Schlacher T. A., Williams A., Bowden D. A., Rowden A. A. (2016). The impacts of deep-sea fisheries on benthic communities: a review. ICES J. Mar. Sci. 73 (suppl_1), i51–i69. doi: 10.1093/icesjms/fsv123
Clarke S. (2004). Shark Product Trade in Hong Kong and Mainland China and Implementation of the CITES Shark Listings (Hong Kong, China: TRAFFIC East Asia). Available online at: https://portals.iucn.org/library/sites/library/files/documents/Traf-084.pdf (Accessed July 8, 2020).
Clarke S., Milner-Gulland E. J., Bjorndal T. (2007). Social, economic, and regulatory drivers of the shark fin trade. Mar. Resource Econ. 22, 305–327. doi: 10.1086/mre.22.3.42629561
Coll M., Libralato S., Tudela S., Palomera I., Pranovi F. (2008). Ecosystem overfishing in the ocean. PloS One 3, e3881. doi: 10.1371/journal.pone.0003881
Cope J. M., DeVore J., Dick E. J., Ames K., Budrick J., Erickson D. L., et al. (2011). An approach to defining stock complexes for U.S. West coast groundfishes using vulnerabilities and ecological distributions. North Am. J. Fisheries Manage. 31, 589–604. doi: 10.1080/02755947.2011.591264
Cope J. M., Hamel O. S. (2022). Upgrading from M version 0.2: An application-based method for practical estimation, evaluation and uncertainty characterization of natural mortality. Fisheries Res. 256. doi: 10.1016/j.fishres.2022.106493
Cortés E., Arocha F., Beerkircher L., Carvalho F., Domingo A., Heupel M., et al. (2010). Ecological risk assessment of pelagic sharks caught in Atlantic pelagic longline fisheries. Aquat. Living Resour. 23, 25–34. doi: 10.1051/alr/2009044
Currey R. J. C., Boren L. J., Sharp B. R., Peterson D. (2012). A risk assessment of threats to Maui’s dolphins (Wellington: Ministry for Primary Industries and Department of Conservation), 51 p.
Cury P. M., Christensen V. (2005). Quantitative ecosystem indicators for fisheries management. ICES J. Mar. Sci. 62, 307–310. doi: 10.1016/j.icesjms.2005.02.003
Da Rocha N., Oppel S., Prince S., Matjila S., Shaanika T. M., Naomab C., et al. (2021). Reduction in seabird mortality in Namibian fisheries following the introduction of bycatch regulation. Biol. Conserv. 253, 108915. doi: 10.1016/j.biocon.2020.108915
Davies R. W. D., Cripps S. J., Nickson A., Porter G. (2009). Defining and estimating global marine fisheries bycatch. Mar. Policy 33, 661–672. doi: 10.1016/j.marpol.2009.01.003
Dellapenna T. M., Allison M. A., Gill G. A., Lehman R. D., Warnken K. W. (2006). The impact of shrimp trawling and associated sediment resuspension in mud dominated, shallow estuaries. Estuar. Coast. Shelf Sci. 69, 519–530. doi: 10.1016/j.ecss.2006.04.024
De Silva A. G., Samarasekara M. A., Thusyanthini R., De Silva A. K. (2022). Prevention of destructive fishing practices: prospects and challenges in law reform. FGS - CJMR J. 07, 36–61.
Dulvy N. K., Sarah L. F., John A. M., Rachel D. C., Peter M. K., Lucy R. H., et al. (2014). Extinction risk and conservation of the world’s sharks and rays. eLife 3. doi: 10.7554/eLife.00590.001
Eayrs S. (2007). A Guide to Bycatch Reduction in Tropical Shrimp-trawl Fisheries. Revised Edition (Rome: FAO), 108p.
Ehrhardt N. M., Aragão J. A. N., Silva K. C. A. (1999). Stock assessment of the industrial pink shrimp (Penaeus subtilis) fishery in Northern Brazil. In: CFRAMP/FAO/DANIDA Stock Assessment Workshop on the Shrimp and Groundfish on the Guyana-Brasil Shelf, Port of Spain, Trinidad and Tobago: CFRAMP/FAO/DANIDA, pp. 99–111.
Farriols M. T., Ordines F., Somerfield P. J., Pasqual C., Hidalgo M., Guijarro B., et al. (2017). Bottom trawl impacts on Mediterranean demersal fish diversity: Not so obvious or are we too late? Continental Shelf Res. 137, 84–102. doi: 10.1016/j.csr.2016.11.011
Fatema U. K., Faruque H., Salam M. A., Matsuda H. (2022). Vulnerability assessment of target shrimps and bycatch species from industrial shrimp trawl fishery in the bay of Bengal, Bangladesh. Sustainability 14, 1691. doi: 10.3390/su14031691
Foden J., Rogers S. I., Jones A. P. (2011). Human pressures on UK seabed habitats: A cumulative impact assessment. Mar. Ecol. Prog. Ser. 428, 33–47. doi: 10.3354/meps09064
Food and Agriculture Organization of the United Nations (2017). Background documents for the Workshop on investing in ecosystem-based shrimp and groundfish fisheries management of the Guianas – Brazil shelf, Barbados, 7–8 September 2015. FAO Fisheries and Aquaculture Circular No. 1120 (Rome, Italy: FAO). Available at: http://www.fao.org/3/a-i5648e.pdf.
Food and Agriculture Organization of the United Nations (2022). The State of World Fisheries and Aquaculture 2022. Towards Blue Transformation (Rome: FAO). doi: 10.4060/cc0461en
Foster S. J., Vincent A. C. J. (2010). Tropical shrimp trawl fisheries: fishers’ knowledge of and attitudes about a doomed fishery. Mar. Policy 34, 437–446. doi: 10.1016/j.marpol.2009.09.010
França N. F. C., Moraes A. B., Carvalho-Batista A., Melo M. C. B., López-Greco L., Mantelatto F. L., et al. (2019). Farfantepenaeus subtilis (Pérez-Farfante 1967) and F. brasiliensis (Latreille 1817) (Decapoda, Penaeidae): Ontogenetic comparison using the combined analysis of secondary sexual characters and molecular markers. Fish. Res. 216, 89–95. doi: 10.1016/j.fishres.2019.03.024
Freitas A. J. R., Passarone R., Lira A. S., Pelage L., Lucena-Frédou F. (2023). Vulnerability assessment of species caught by the shrimp trawl fishery in northeastern Brazil. Regional Stud. Mar. Sci. 61, 102949. doi: 10.1016/j.rsma.2023.102949
Froese R., Binohlan C. (2000). Empirical relationships to estimate asymptotic length, length at first maturity and length at maximum yield per recruit in fishes, with a simple method to evaluate length frequency data. J. Fish Biol. 56, 758–773. doi: 10.1006/jfbi.1999.1194
Froese R., Pauly D. (Eds.) (2000). FishBase 2000: concepts, design and data sources (Los Baños, Laguna, Philippines: ICLARM), 344 p.
Gallo M. N., Vinzon S. B. (2005). Generation of overtides and compound tides in Amazon estuary. Ocean Dynamics 55, 441–448. doi: 10.1007/s10236-005-0003-8
Garcia A. M., Vieira-Sobrinho J. P. (2001). O aumento da diversidade de peixes no estuário da Lagoa dos Patos durante o episódio El Niño 1997-1998. Atlántica, Río Grande 23, 85–96.
Georgeson L., Rigby C. L., Emery T. J., Fuller M., Hartog J., Williams A. J., et al. (2020). Ecological risks of demersal fishing on deepwater chondrichthyan populations in the Southern Indian and South Pacific Oceans. ICES J. Mar. Sci. 77, 1711–1727. doi: 10.1093/icesjms/fsaa019
Gillett R. (2008). Global study of shrimp fisheries. FAO Fish. Techn. Pap. No 475 (Rome: FAO), 331p. Available at: http://www.fao.org/3/a-i0300e.pdf.
Gislason H., Daan N., Rice J. C., Pope J. G. (2010). Size, growth, temperature and the natural mortality of marine fish. Fish Fisheries 11, 149–158. doi: 10.1111/j.1467-2979.2009.00350.x
Griffiths S., Kesner-Reyes K., Garilao C., Duffy L. M., Román M. H. (2019). Ecological Assessment of the Sustainable Impacts by Fisheries (EASI-Fish): a flexible vulnerability assessment approach to quantify the cumulative impacts of fishing in data-limited settings. Mar. Ecol. Prog. Ser. 625, 89–113. doi: 10.3354/meps13032
Griffiths S. P., Wallace B. P., Cáceres V., Rodríguez L. H., Lopez J., Abregfo M. (2024). Vulnerability of the Critically Endangered leatherback turtle to fisheries bycatch in the eastern Pacific Ocean. II. Assessment of mitigation measures. Endang. Species Res. 53, 295–326. doi: 10.3354/esr01305
Guanais J. H. G., Rodrigo P. M., Patrick A. M. (2015). Designing a framework for addressing bycatch problems in Brazilian small-scale trawl fisheries. Mar. Policy 51, 111–118. doi: 10.1016/j.marpol.2014.07.004
Guimarães-Costa A., Machado F. S., Reis-Filho J. A., Andrade M., Araújo G. R., Corrêa E. M. R., et al. (2020). DNA barcoding for the assessment of the taxonomy and conservation status of the fish bycatch of the northern brazilian shrimp trawl fishery. Front. Mar. Sci. 7, 566021. doi: 10.3389/fmars.2020.566021
Hall M. A. (1998). An ecological view of the tuna-dolphin problem: impacts and trade-offs. Rev. Fish Biol. Fisheries 8, 1–34. doi: 10.1023/A:1008854816580
Hall M. A., Alverson D. L., Metuzals K. I. (2000). By-catch: problems and solutions. Mar. pollut. Bull. 41, 204–219. doi: 10.1016/S0025-326X(00)00111-9
Halpern B. S., McLeod K. L., Rosenberg A. A., Crowder L. B. (2008). Managing for cumulative impacts in ecosystem-based management through ocean zoning. Ocean Coast. Manage. 51, 203–211. doi: 10.1016/j.ocecoaman.2007.08.002
Harrington J. M., Myers R. A., Rosenberg A. A. (2005). Wasted fishery resources: discarded by-catch in the USA. Fish Fisheries 6, 350–361. doi: 10.1111/j.1467-2979.2005.00201.x
Hiddink J. G., Jennings S., Sciberras M., Bolam S. G., Cambiè G., McConnaughey R. A., et al. (2019). Assessing bottom trawling impacts based on the longevity of benthic invertebrates. J. Appl. Ecol. 56, 1075–1084. doi: 10.1111/1365-2664.13278
Hilborn R., Branch T. A., Ernst B., Magnusson A., Minte-Vera C. V., Scheuerell M. D., et al. (2003). State of the world’s fisheries. Annu. Rev. Environ. Resour. 28, 359–399. doi: 10.1146/annurev.energy.28.050302.105509
Hobday A. J., Smith A. D. M., Stobutzki I. C., Bulman C., Daley R., Dambacher J. M., et al. (2011). Ecological risk assessment for the effects of fishing. Fisheries Res. 108, 372–384. doi: 10.1016/j.fishres.2011.01.013
Hobday A. J., Smith A. D. M., Webb H., Daley R., Wayte S., Bulman C., et al. (2007). Ecological Risk Assessment for the Effects of Fishing: Methodology. Report R04/1072 for the Australian Fisheries Management Authority, canberra. July 2007. Available online at: https://www.sprfmo.int/assets/Meetings/Meetings-before-2013/Scientific-Working-Group/SWG-07-2009/SP-07-SWG-INF-03-CSIRO-AFMA-2007-Ecological-Risk-Assessment-Methodology-2.pdf.
Hordyk A. R., Carruthers T. R. (2018). A quantitative evaluation of a qualitative risk assessment framework: Examining the assumptions and predictions of the Productivity Susceptibility Analysis (PSA). PloS One 13, e0198298. doi: 10.1371/journal.pone.0198298
Hordyk A., Ono K., Valencia S., Loneragan N., Prince J. (2015). A novel length-based empirical estimation method of spawning potential ratio (SPR), and tests of its performance, for small-scale, data-poor fisheries. ICES J. Mar. Sci. 72, 217–231. doi: 10.1093/icesjms/fsu004
Hordyk A. R., Plummer M., Walters C. (2016). A simple length-structured model based on life history ratios and incorporating size-dependent selectivity: application to spawning potential ratios for data-poor stocks. Can. J. Fisheries Aquat. Sci. 73, 1787–1799. doi: 10.1139/cjfas-2015-0422
Instituto Chico Mendes de Conservação da Biodiversidade (2016). Livro Vermelho da Fauna Brasileira Ameaçada de Extinção: Volume VI - Peixes. In: Instituto Chico Mendes de Conservação da Biodiversidade (Org.). Livro Vermelho da Fauna Brasileira Ameaçada de Extinção. Brasília: ICMBio. 1232p.
Isaac V. J., Braga T. M. P. (1999). Rejeição de pescado nas pescarias da Região Norte do Brasil. Arquivos de Ciências do Mar Vol. 32 (Fortaleza: Arquivos de ciencia do Mar), 39–54. Available at: http://www.repositorio.ufc.br/handle/riufc/1100.
Isaac V. J., Dias Neto J., Damasceno F. G. (1992). Camarão-rosa da costa norte. Biologia, dinâmica e administração pesqueira. IBAMA, Série Estudos de Pesca Vol. 1 (Brasília: IBAMA), 1–187.
Isaac V. J., Ferrari S. F. (2017). Assessment and management of the north Brazil shelf large marine ecosystem. Environ. Dev. 22, 97–110. doi: 10.1016/j.envdev.2016.11.004
Isaac V. J., Frédou F. L. (2022), 139p. SHRIMP_NEN. Rede cooperativa multidisciplinar para subsidiar o manejo da pesca dos estoques de camarões da região Norte e Nordeste do Brasil com foco ecossistêmico. Chamada MCTI/MPS/CNPQ N° 22/2015: A CONTRIBUIÇÃO DA CIÊNCIA PESQUEIRA PARA UMA PESCA SUSTENTÁVEL 77 Ordenamento da Pesca Marinha Brasileira – Linha Temática III: Camarões da Costa Norte/Nordete (N/NE). Relatório Final. Novembro de 2022.
Isaac V. J., Santo R. V. E., Bentes B., Frédou F. L., Mourão K. R. M., Frédou T. (2009). An interdisciplinary evaluation of fishery production systems off the state of Pará in North Brazil. J. Appl. Ichthyol. 25, 244–255. doi: 10.1111/j.1439-0426.2009.01274.x
Jackson J. B., Kirby M. X., Berger W. H., Bjorndal K. A., Botsford L. W., Bourque B. J., et al. (2001). Historical overfishing and the recent collapse of coastal ecosystems. Science 293, 629–637. doi: 10.1126/science.1059199
Jog K., Sutaria D., Diedrich A., Grech A., Marsh H. (2022). Marine mammal interactions with fisheries: review of research and management trends across commercial and small-scale fisheries. Front. Mar. Sci. 9. doi: 10.3389/fmars.2022.758013
Kaiser M. J., Clarke K. R., Hinz H., Austen M. C. V., Somerfield P. J., Karakassis I. (2006). Global analysis of response and recovery of benthic biota to fishing. Mar. Ecol. Prog. Ser. 311, 1–14. doi: 10.3354/meps311001
King J. R., McFarlane G. A. (2003). Marine fish life history strategies: applications to fishery management. Fish. Manag. Ecol. 10, 249–264. doi: 10.1046/j.1365-2400.2003.00359.x
Kirby D. S., Ward P. (2014). Standards for the effective management of fisheries bycatch. Mar. Policy 44, 419–426. doi: 10.1016/j.marpol.2013.10.008
Le Quesne W. J. F., Jennings S. (2012). Predicting species vulnerability with minimal data to support rapid risk assessment of fishing impacts on biodiversity. J. Appl. Ecol. 49, 20–28.
Lewison R. L., Crowder L. B., Read A. J., Freeman S. A. (2004). Understanding impacts of fisheries bycatch on marine megafauna. Trends Ecol. Evol. 19, 598–604. doi: 10.1016/j.tree.2004.09.004
Lima J. D. F., Silva T. C. D., Silva L. M. A. D., Lin Garcia J. D. S. (2013). Brachyuran crustaceans from the bycatch of prawn fisheries at the mouth of the Amazon river. Acta Amazonica 43, 91–98. doi: 10.1590/S0044-59672013000100011
Lin C.-Y., Wang S.-P., Chiang W.-C., Griffiths S., Yeh H.-M. (2020). Ecological risk assessment of species impacted by fisheries in waters off eastern Taiwan. Fish. Manag. Ecol. 27, 345–356. doi: 10.1111/fme.12417
Lindeman R. L. (1942). The trophic-dynamic aspect of ecology. Ecology 23, 399–417. doi: 10.2307/1930126
Link J. S. (2002). Ecological considerations in fisheries management: when does it matter? Fisheries 27, 10–17. doi: 10.1577/1548-8446(2002)027<0010:ECIFM>2.0.CO;2
Lira A. S., Loc’h F. L., Andrade H. A., Lucena-Frédou F. (2022). Vulnerability of marine resources affected by a small-scale tropical shrimp fishery in Northeast Brazil. ICES J. Mar. Sci. 79, 633–647. doi: 10.1093/icesjms/fsac004
Lucena-Frédou F., Kell L., Frédou T., Gaertner D., Potier M., Bach P., et al. (2016). Life history traits and fishery patterns of teleosts caught by the tuna longline fishery in the South Atlantic and Indian Oceans. Fisheries Res. 179, 308–321. doi: 10.1016/j.fishres.2016.03.013
Lucena-Frédou F., Kell L., Frédou T., Gaertner D., Potier M., Bach P., et al. (2017). Vulnerability of teleosts caught by the pelagic tuna longline fleets in South Atlantic and Western Indian Oceans. Deep Sea Res. Part II: Topical Stud. Oceanogr. 140, 230–241. doi: 10.1016/j.dsr2.2016.10.008
Marceniuk A. P., Rotundo M. M., Caires R. A., Cordeiro A. P. B., Wosiacki W. B., Oliveira C., et al. (2019). The bony fishes (Teleostei) caught by industrial trawlers off the Brazilian North coast, with insights into its conservation. Neotropical Ichthyol. 17, e180038. doi: 10.1590/1982-0224-20180038
Martins D. E. G., Camargo-Zorro M. S. F., Walfir P. M., Cintra I. H. A., Silva K. C. A. (2015). Spatial distribution of southern brown shrimp (Farfantepenaeus subtilis) on the Amazon continental shelf: a fishery, marine geology and GIS integrated approach. Braz. J. Oceanogr. 63, 397–406. doi: 10.1590/S1679-87592015090106304
McCauley D. J., Pinsky M. L., Palumbi S. R., Estes J. A., Joyce F. H., Warner R. R. (2015). Marine defaunation: Animal loss in the global ocean. Science 347, 1255641. doi: 10.1126/science.1255641
McConnaughey R. A., Hiddink J. G., Jennings S., Pitcher C. R., Kaiser M. J., Suuronen P., et al. (2020). Choosing best practices for managing impacts of trawl fishing on seabed habitats and biota. Fish Fisheries 21, 319–337. doi: 10.1111/faf.12431
Mendo J., Mendo T., Gil-Kodaka P., Martina J., Gómez I., Delgado R., et al. (2022). Bycatch and discards in the artisanal shrimp trawl fishery in Northern Peru. PloS One 17, e0268128. doi: 10.1371/journal.pone.0268128
Mertz D. B. (1970). “Notes on methods used in life-history studies,” in Readings in Ecology and Ecological Genetics. Eds. Connell J. H., Mertz D. B., Murdoch W. W. (Harper & Row, New York), 4–17.
Mesquita I. C. G., Medeiros R. P. (2023). Integrating research and fishing extension approaches to engage small-scale fishers in the participatory evaluation and voluntary use of bycatch reduction devices. Mar. Policy 152, 105599. doi: 10.1016/j.marpol.2023.105599
Mohamed K. S., Sathianandan T. V., Vivekanandan E., Kuriakose S., Ganga U., Pillai S. L., et al. (2021). Application of biological and fisheriesattributes to assess the vulnerability and resilienceof tropical marine fish species. PloS One 16, e0255879. doi: 10.1371/journal.pone.0255879
Moura H. T. G. d. S., Mescouto N. L. d. C., de Souza M. C. P., Nunes Z. M. P., da Silva B. B. (2023). Performance indicators for the large-scale Acoupa weakfish fishery of the Amazon continental shelf. Fish. Manag. Ecol. 30, 545–554. doi: 10.1111/fme.12642
Murua H., Arrizabalaga H., Huang J., Romanov E., Bach P., de Bruyn P., et al. (2009). Ecological risk assessment (ERA) for species caught in fisheries managed by the Indian Ocean Tuna Commission (IOTC): a first attempt (Mahé, Seychelles: Indian Ocean Tuna Commission).
Nguyen T. V. (2012). Ecosystem-based fishery management: A review of concepts and ecological economic models. J. Ecosyst. Manage. 13, 1–14. Available at: https://jem-online.org/index.php/jem/article/view/142/460.
Nittrouer C. A., DeMaster D. J., Kuehl S. A., Figueiredo A. G. Jr., Sternberg R. W., Faria L. E. C., et al. (2021). Amazon sediment transport and accumulation along the continuum of mixed fluvial and marine processes. Ann Rev Mar Sci. 13, 501–536. doi: 10.1146/annurev-marine-010816-060457
Nobre C. A., Molion L. C. B. (1986). Boletim de Monitoramento e Análise Climática – Climanálise – Número Especial, Edição Comemorativa de 10 anos (São José dos Campos, SP: INPE), 125 pp.
Nóbrega P., Meira Dos Santos C., Cordeiro A., Martinelli-Lemos J. (2021). Invertebrates assemblage captured by a pink shrimp’s fishery on Amazon continental shelf. Lat. Am. J. Aquat. Res. 49, 227–241. doi: 10.3856/vol49-issue2-fulltext-2600
Ormseth O. A., Spencer P. D. (2011). An assessment of vulnerability in Alaska groundfish. Fisheries Res. 112, 127–133. doi: 10.1016/j.fishres.2011.02.010
Osio G. C., Orio A., Millar C. P. (2015). Assessing the vulnerability of Mediterranean demersal stocks and predicting exploitation status of unassessed stocks. Fish. Res. 171, 110–121. doi: 10.1016/j.fishres.2015.02.005
Pacoureau N., Rigby C. L., Kyne P. M., Sherley R. B., Winker H., Carlson J. K., et al. (2021). Half a century of global decline in oceanic sharks and rays. Nature 589, 567–571. doi: 10.1038/s41586-020-03173-9
Paradinas I., Marın M., Pennino M. G., Lopez-Quılez A., Conesa D., Barreda D., et al. (2016). Identifying the best fishing-suitable areas under the new European discard ban. ICES J. Mar. Sci. 73, 2479–2487. doi: 10.1093/icesjms/fsw114
Patrick W. S., Spencer P., Link J., Cope J., Field J., Kobayashi D., et al. (2010). Using productivity and susceptibility indices to assess the vulnerability of United States fish stocks to overfishing. Fishery Bulletin. 108, 305–322. Available at: http://fishbull.noaa.gov/1083/patrick.pdf.
Pauly D., Christensen V., Dalsgaard J., Froese R., Torres F. (1998). Fishing down marine food webs. Science 279, 860–863. doi: 10.1126/science.279.5352.860
Pauly D., Zeller D. (2016). Catch reconstructions reveal that global marine fisheries catches are higher than reported and declining. Nat. Commun. 7, 10244. doi: 10.33448/rsd-v10i8.17394
Peixoto U. I., Bentes B., Andrade H. A., Isaac V. J. (2021). Length-based assessment of southern brown shrimp stock from trawl fisheries on the Amazon Continental Shelf. Res. Soc. Dev.10, e44410817394. doi: 10.33448/rsd-v10i8.17394
Peixoto U. I., Casal-Ribeiro M., Medeiros-Leal W. M., Novoa-Pabon A., Pinho M., Santos R. (2022b). Scientific and fisher’s knowledge-based ecological risk assessment: combining approaches to determine the vulnerability of fisheries stocks. Sustainability 14, 14870. doi: 10.3390/su142214870
Peixoto U. I., Mello-Filho A. S., Bentes B., Isaac V. J. (2022a). Trawl fishing fleet operations used to illustrate the life cycle of the southern brown shrimp: insights to management and sustainable fisheries. Fishes 7, 141. doi: 10.3390/fishes7030141
Pérez Roda M. A., Gilman E., Huntington T., Kennelly S. J., Suuronen P., Chaloupka M., et al. (2019). “A third assessment of global marine fisheries discards,” in FAO Fisheries and Aquaculture Technical Paper No. 633 (FAO, Rome), 78 pp. Available at: http://www.fao.org/3/CA2905EN/ca2905en.pdf.
Pinaya W. H. D., Campos R., Maia B. P. S., Nunes Z. M. P., Bentes B. (2022). Impacts of climate variability on the southern brown shrimp (Penaeussubtilis) Fishery on the Amazon Shelf. Poult Fish Wildl Sci. 10, 198.
Pikitch E. K., Santora C., Babcock E. A., Bakun A., Bonfil R., Conover D. O., et al. (2004). Ecosystem-based fishery management. Science 305, 346–347. doi: 10.1126/science.1098222
Previero M., Gasalla M. A. (2019). Risk assessment of small-scale reef fisheries off the Abrolhos Bank: Snappers and groupers under a multidimensional evaluation. Fish. Manag. Ecol. 27, 231–247. doi: 10.1111/fme.12406
Rábago-Quiroz C. H., López-Martínez J., Valdez-Holguín J. E., Nevárez-Martínez M. O., Acevedo-Cervantes A. (2012). Fish assemblages in the bycatch of bottom shrimp trawls on the west side of the Gulf of California, Mexico. Mar. Biol. Res. 8, 865–876. doi: 10.1080/17451000.2012.692161
R Core Team (2020). R: A language and environment for statistical computing (Vienna, Austria: R Foundation for Statistical Computing). Available at: https://www.r-project.org. RStudio 2023.03.0 + 386 “Cherry Blossom” Release (3c53477afb13ab959aeb5b34df1f10c237b256c3, 2023-03-09) for macOS.
Rigby C., Simpfendorfer C. A. (2015). Patterns in life history traits of deep-water chondrichthyans. Deep Sea Res. Part II: Topical Stud. Oceanogr. 115, 30–40. doi: 10.1016/j.dsr2.2013.09.004
Rindorf A., Mumford J., Baranowski P., Clausen L. W., García D., Hintzen N. T., et al. (2017). Moving beyond the MSY concept to reflect multidimensional fisheries management objectives. Mar. Policy 85, 33–41. doi: 10.1016/j.marpol.2017.08.012
Roberts C. M., Hawkins J. P. (1999). Extinction risk in the sea. Trends Ecol. Evol. 14, 241–248. doi: 10.1016/S0169-5347(98)01584-5
Rodrigues-Filho J. L., Dolbeth M., Bernardes J. J. Jr., Ogashawara I., Branco J. O. (2020). Using an integrative approach to evaluate shrimp bycatch from subtropical data-poor fisheries. Fisheries Res. 230, 105587. doi: 10.1016/j.fishres.2020.105587
Sanz N., Diop B., Blanchard F., Lampert L. (2016). On the influence of environmental factors on harvest: the French Guiana shrimp fishery paradox. Environ. Econ. Policy Stud. 19, 233–247. doi: 10.1007/s10018-016-0153-6
Sciberras M., Hiddink J. G., Jennings S., Szostek C. L., Hughes K. M., Kneafsey B., et al. (2018). Response of benthic fauna to experimental bottom fishing: A global meta-analysis. Fish Fisheries 19, 698–715. doi: 10.1111/faf.12283
Smith A. D. M., Fulton E. J., Hobday A. J., Smith D. C., Shoulder P. (2007). Scientific tools to support the practical implementation of ecosystem-based fisheries management. ICES J. Mar. Sci. 64, 633–639. doi: 10.1093/icesjms/fsm041
Stobutzki I., Miller M., Brewer D. (2001a). Sustainability of fishery bycatch: A process for assessing highly diverse and numerous bycatch. Environ. Conserv. 28, 167–181. doi: 10.1017/S0376892901000170
Stobutzki I. C., Miller M. J., Heales D. S., Brewer D. T. (2002). Sustainability of elasmobranchs caught as bycatch in a tropical prawn (shrimp) trawl fishery. Fishery Bull. 100, 800–821. Available at: https://spo.nmfs.noaa.gov/sites/default/files/pdf-content/2002/1004/stobut.pdf.
Stobutzki I. C., Miller M. J., Jones P., Salini J. P. (2001b). Bycatch diversity and variation in penaeid fisheries; the implications for monitoring. Fisheries Res. 53, 283–301. doi: 10.1016/S0165-7836(00)00273-3
Tasker M. L., Camphuysen C. J., Cooper J., Garthe S., Montevecchi W. A., Blaber S. J. M. (2000). The impacts of fishing on marine birds. J. Mar. Sci. 57, 531–547. doi: 10.1006/jmsc.2000.0714
Taylor C. C. (1960). Temperature, growth, and mortality – the pacific cockle. ICES J. Mar. Sci. 26, 117–124. doi: 10.1093/icesjms/26.1.117
Teixeira E. C., da Silva V. E. L., Fabré N. N., Batista V. S. (2020). Marine shrimp fisheries research—a mismatch on spatial and thematic needs. Scientometrics 122, 591–606. doi: 10.1007/s11192-019-03276-9
Thrush S. F., Dyton O. K. (2002). Disturbance to marine benthic habitats by trawling and dredging: implications for marine biodiversity. Annu. Rev. Ecol. Evol. Sys. 33. doi: 10.1146/annurev.ecolsys.33.010802.150515
von Bertalanffy L. (1938). A quantitative theory of organic growth (inquiries on growth laws II). Hum. Biol. 10, 181–213.
von Bertalanffy L. (1957). Quantitative laws in metabolism and growth. Q. Rev. Biol. 32, 217–231. doi: 10.1086/401873
Walters C. J., Martell S. J. (2004). Fisheries Ecology and Management (Princeton University Press). Available at: http://www.jstor.org/stable/j.ctv10vm1f6.
Watson J., Foster D., Nichols S., Shah S., Scoll-oenlon E., Nanc J. (1999). The development of bycatch reduction technology in the southeastern United States shrimp fishery. Mar. Technol. Soc. J. 33, 51–56. doi: 10.4031/MTSJ.33.2.8
Webb H., Smith T. (2008). Review of the scope, assessment methods and management responses for fisheries ESD and EBFM in Australia. Fisheries Research and Development Corporation, Report 2004/101 (Canberra, Australia: Fisheries Research and Development Corporation), 387 pp.
Weber S. C., Carpenter E. J., Coles V. J., Yager P. L., Goes J., Montoya J. P. (2017). Amazon River influence on nitrogen fixation and export production in the western tropical North Atlantic. Limnol. Oceanogr. 62, 618–631. doi: 10.1002/lno.10448
Winemiller K. O. (1989). Patterns of variation in life history among South American fishes in seasonal environments. Oecologia 81, 225–241. doi: 10.1007/BF00379810
Zhou S., Griffiths S. ,. P., Miller M. (2009). Sustainability assessment for fishing effects (SAFE) on highly diverse and data-limited fish bycatch in a tropical prawn trawl fishery. Mar. Freshw. Res. 60, 563–570. doi: 10.1071/MF08207
Zhou S., Hobday A. J., Bulman C. M., Fuller M., Daley R. M. (2019). A data-limited method for assessing cumulative fishing risk on bycatch. ICES J. Mar. Sci. 76, 837–847. doi: 10.1093/icesjms/fsy206
Zhou S., Hobday A. J., Dichmont C. M., Smith A. D. M. (2016). Ecological risk assessments for the effects of fishing: a comparison and validation of PSA and SAFE. Fisheries Res. 183, 518–529. doi: 10.1016/j.fishres.2010.09.028
Keywords: industrial fisheries, productivity and suscetibility, amazon coast, fishery management, incidental catch
Citation: Peixoto UI, Sporcic M, Hobday AJ, Bentes B, Passarone R, Fredou FL and Isaac VJ (2025) Ecological risk assessment of marine resources caught as bycatch in industrial bottom trawl shrimp fishery in the Amazon Continental Shelf. Front. Mar. Sci. 12:1490894. doi: 10.3389/fmars.2025.1490894
Received: 03 September 2024; Accepted: 08 January 2025;
Published: 03 February 2025.
Edited by:
Oscar Sosa-Nishizaki, Center for Scientific Research and Higher Education in Ensenada (CICESE), MexicoReviewed by:
Javier Tovar-Ávila, Instituto Nacional de Pesca y Acuacultura (INAPESCA), MexicoCopyright © 2025 Peixoto, Sporcic, Hobday, Bentes, Passarone, Fredou and Isaac. This is an open-access article distributed under the terms of the Creative Commons Attribution License (CC BY). The use, distribution or reproduction in other forums is permitted, provided the original author(s) and the copyright owner(s) are credited and that the original publication in this journal is cited, in accordance with accepted academic practice. No use, distribution or reproduction is permitted which does not comply with these terms.
*Correspondence: Ualerson I. Peixoto, dWFsZXJzb25wZWl4b3RvQGdtYWlsLmNvbQ==
Disclaimer: All claims expressed in this article are solely those of the authors and do not necessarily represent those of their affiliated organizations, or those of the publisher, the editors and the reviewers. Any product that may be evaluated in this article or claim that may be made by its manufacturer is not guaranteed or endorsed by the publisher.
Research integrity at Frontiers
Learn more about the work of our research integrity team to safeguard the quality of each article we publish.