- 1College of Marine Science and Environment Engineering, Dalian Ocean University, Dalian, China
- 2Key Laboratory of Environment Controlled Aquaculture (Dalian Ocean University) Ministry of Education, Dalian, China
- 3College of Food Science and Engineering, Dalian Ocean University, Dalian, China
- 4College of Fisheries and Life Science, Dalian Ocean University, Dalian, China
- 5Dalian Jinpu New District Marine and Fisheries Comprehensive Administrative Law Enforcement Team, Dalian, China
- 6Changhai County Marine and Fisheries Comprehensive Administrative Law Enforcement Team, Dalian, China
The black rockfish (Sebastes schlegelii) is a commercially important marine species in the Northwest Pacific Ocean. Its population has significantly declined due to overfishing and environmental changes. Stock enhancement has been launched in response to wild populations decline. However, limited evidence is available to confirm the ecological effects after release. Empirically, if the hatchery-released individuals are well-adapted to the wild environment, they should show good or similar nutritional conditions as well as their wild counterparts. Therefore, nutritional analysis was essential and conducted in the present study, by using 146 S. schlegelii individuals in order to compare the differences among hatchery-reared (before-release), hatchery-released, and wild conspecifics (post-release) groups in consideration of different environments during a whole stock enhancement practice. Our results exhibited that hatchery-reared S. schlegelii exhibited significantly lower levels of crude protein and amino acid content than that of hatchery-released and wild ones (P < 0.05). Specifically, both the hatchery-released and wild S. schlegelii generally showed similar trends of nutrition profiling compared with their hatchery-reared counterparts, such as moisture, crude ash, crude lipid, crude protein, and amino acid profiles (P > 0.05), indicating homogeneity in their nutritional contents. Our research suggested that S. schlegelii exhibited extensive ecological plasticity, and the variations in nutrition of a population was mainly influenced by environmental factors rather than the origin. However, several differences in fatty acid composition between hatchery-released and wild S. schlegelii indicated that hatchery-released fish might not have fully adapt to the food supply in the wild. This study provided insights into promoting responsible stock enhancement of this species in the future.
1 Introduction
The global capture fisheries production, except algae, was 92.3 million tonnes, with an evaluated value of United States dollars (USD) 159 billion in 2022 (FAO, 2024). Although world fisheries face many challenges, such as overfishing, habitat destruction, and pollution (Worm and Branch, 2012), the development of sustainable fishery has become a consensus to deal with the declining status of global fishery resources. As a sustainable fishery management approaches, stock enhancement refers to the practice of releasing hatchery-reared juveniles into existing populations to increase fishing and rebuild diminished resources in marine and freshwater (Blaxter, 2000);. China is one of the significant aquaculture producers, accounting for 36% of the global aquaculture production now (FAO, 2024). The development of aquaculture contributes to the supply of abundant seedlings for stock enhancement in coastal waters to restore local population resources (Taylor et al., 2017). The stock enhancement is recognized as an effective short-term fisheries management strategy to increase fishery production or conservation in the wild (Richardson et al., 2023). This approach has been extensively implemented not only in China but also in other waters whenever salt water or freshwater throughout the world (Kitada, 2020). The negative impacts of large-scale stock enhancement programs are a global concern (Kitada, 2018). One primary concern is the ecological impacts, particularly the adaptation of hatchery-reared juveniles after release and their ecological interactions with wild conspecifics (Johnsson et al., 2014; Pinter et al., 2019). Stock enhancement activities generally lack investigation and monitoring, with insufficient informative data for evaluation. Regarding the estimation of economic benefits (Hunt et al., 2017) and genetic impacts (Liu et al., 2018), the ecological effects of stock enhancement are even unclear, primarily due to difficulties in tracking hatchery-released juveniles in natural habitats. In one case, hatchery-released juvenile Japanese Spanish mackerel (Scomberomorus niphonius) tended to be larger than wild fish and had higher growth performance than wild ones (Nakajima et al., 2013). Density-dependent growth caused by competition for food could decrease the growth rate of both of hatchery-released juveniles and wild ones, as well as other competitive species, or even replace the wild populations when stocking exceeded the carrying capacity of the environment in salmons (Kitada, 2018). In contrast, other researches have revealed that hatchery-reared juveniles can adapt to the natural water in post-release and seem not to have negative impacts on wild populations (Wang et al., 2019). For example, a feeding ecology analysis from hatchery-released Japanese flounder (Paralichthys olivaceus) demonstrated that the growth status and the stomach contents of the hatchery-released and wild fish were almost identical. This inferred that post-release of P. olivaceus had well adaptability with their wild counterparts (Wang et al., 2019). Similar results were also reported in honmasu salmon (Oncorhynchus rhodurus) (Munakata et al., 2000), European grayling (Thymallus thymallus) (Turek et al., 2010), meagre (Argyrosomus regius) (Gil et al., 2014). Hence, research and monitoring are necessary to investigate the possible adaptation differences in hatchery-released fish.
Variations in fish nutrient composition, including proximate composition, amino acid contents, and fatty acid contents, are the result of adaptation to available food and environment (Grigorakis, 2007; Oh et al., 2023). It reflects the nutritional status of the fish itself and its interactions with the environment (Rosburg et al., 2019). Therefore, demonstration of the nutrition profiling of both hatchery-released fish and their wild conspecifics during the same period can indirectly respond to feeding selectivity for further exploring whether hatchery-released fish can exhibit similar feeding ecology with their wild counterparts and potential impacts of stock enhancement on wild fish after release (Tomiyama et al., 2011). Different life history or behavior may lead to differences in the specific nutritional components. Sprague et al. (2016) analyzed the fatty acid composition of over 3,000 Atlantic salmon (Salmo salar) farmed between 2006 and 2015 and found that terrestrial fatty acids of farmed populations have significantly increased alongside a decline in EPA and DHA levels, in comparison to wild populations (Sprague et al., 2016). Furthermore, Chakma et al. (2022) reported that the protein and carbohydrate content of farmed pangasius catfish (Pangasius hypophthalmus) was significantly higher than that of wild populations (Chakma et al., 2022). This indicated that the protein quality of farmed fish was relatively consistent, whereas wild fish exhibited significant fluctuations in feeding selectivity due to the diverse range of food sources available throughout their life cycle. In addition, research on nutritional compositions in one species among its different geographical populations, such as hilsa (Tenualosa ilisha) (De et al., 2019) and southern stingray (Hypanus americana) (Hoopes et al., 2020) illustrated that the habitat environment conditions could affect fish nutritional quality. However, few have been reported regarding nutrition analyses to reveal the characteristics of hatchery-released fish so far.
The black rockfish (Sebastes schlegelii) is widely distributed along the bottom of coastal areas of northern China, Japan and Korea, with a wide temperature range of 5-28°C (Chin et al., 2013). It is also an economic fishery species due to its high value. However, its stocks are declining due to overfishing and environmental changes (McGreer and Frid, 2017). Large-scale stock enhancement of S. schlegelii in China has been initiated since 2016, with an average release of more than 30 million juveniles annually. From 2016 to 2019, more than 97% of the total released number in China was concentrated in the coastal waters of Shandong Province each year. There were no releases of S. schlegelii in Liaoning Province before 2019. Therefore, the whole process of S. schlegelii stock enhancement was first traced in Liaoning Province in 2019. In our previous study, the hatchery-released individuals from wild fish were distinguished by recapture investigation using DNA profiling (Liu et al., 2022). Based on nutritional components analysis, the present study aimed to evaluate (1) whether hatchery-released S. schlegelii exhibited similar nutritional quality with their wild counterparts, (2) whether hatchery-reared S. schlegelii could adapt to the native environment after release. This study offers crucial insights into understanding the ecological effects of stock enhancement and serves as a valuable reference for successful stock enhancement activities in the future.
2 Materials and methods
2.1 Ethical statement
Animal experiments were conducted at the Fisheries Resources Laboratory of Dalian Ocean University, in accordance with Chinese laws, regulations, and ethical principles.
2.2 Sample collection
From April to May 2019, around 800 inseminated female S. schlegelii were collected from the Northern Yellow Sea area of Liaoning Province (Dalian). The species typically engages in mating activities during November and December of 2018, utilizing a polyandrous mating pattern in the wild. Consequently, only female fish were selected as broodstock for subsequent stock enhancement. In conclusion, a total of 489 broodstock females spawned in May 2019, with approximately 30 broodstock females placed in each breeding pond (50 m³) and allowed to spawn in a shaded environment. The effective population size of the broodstock population was found to be sufficiently large (Ne = 4,593.5, using the linkage disequilibrium method, Pcrit = 0.02) to produce first-generation offspring with high genetic diversity, no significant genetic differentiation with local wild populations (Liu et al., 2022). Subsequently, the offspring seeds were fed pellets in a running water system prior to release (Supplementary Table S1). Hatchery-reared S. schlegelii [n = 36, total length (TL): 8.332 ± 0.162 cm, mean ± standard error (S.E.)] were randomly selected as the before-release group for nutrition analysis. In addition, a total of 1.05 million S. schlegelii juveniles were released in the Northern Yellow Sea area of Liaoning Province in July 2019.
Fishery recapture investigations were then conducted monthly, and 710 S. schlegelii were captured by fishing trap from August to December post-release in 2019 (Figure 1). In our previous study, we used nine highly polymorphic microsatellite markers with Cervus 3.07 to assign parents. During recapture surveys, we distinguished 279 hatchery-released individuals and 431 wild ones based on likelihood calculations. The combined power of exclusion (CPE) of all the loci in this parentage assignment reached to 99.8% (Liu et al., 2022). From the 279 hatchery-released fish, 66 individuals (TL: 7.856 ± 0.356 cm, mean ± S.E.) were selected as the hatchery-released group based on their higher logarithm of the odds (LOD, ranging from 3 to 12) within the confidence interval for further analysis (Supplementary Table S2). In contrast, 44 of the 431 wild counterparts (TL: 8.705 ± 0.246 cm, mean ± S.E.) were also selected as the wild group due to their lower LOD scores (ranging from -5 to 2) outside the confidence interval (Supplementary Table S2). Therefore, 146 samples were divided into three groups (before-release, hatchery-released, and wild group) in order to analyze their nutritional profiles in the present study. In each group, due to different specimen sizes, 5 to 18 fish per month were used, respectively (Supplementary Table S3).
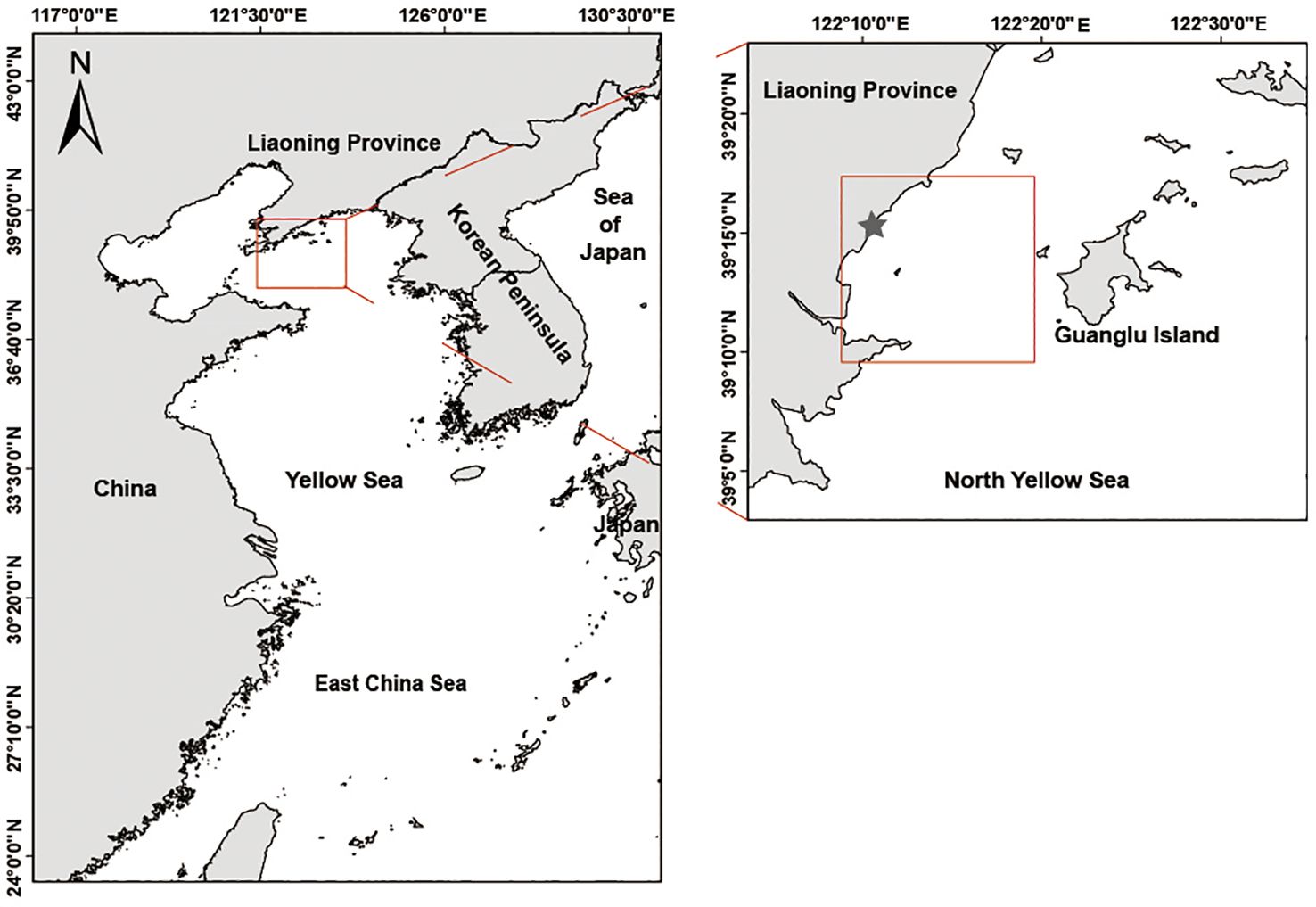
Figure 1. The Fishery recapture investigations of the S. schlegelii in North Yellow Sea. Hatchery-released and wild S. schlegelii were collected in the red dotted lines area after stock enhancement, grey star indicates the North Yellow Sea area of releasing S. schlegelii.
2.3 Proximate composition analysis
Prior to proximate composition analysis, in each month, the fish samples per group were randomly divided into three independent treatments as replicates for nutrient determinations. Each fish per group was prepared by removing the scales, skin and bones. The muscle tissues from each month were then chopped and mixed. Moisture was evaluated by using a hot air oven (DGG-9123A, Shanghai, China) for drying the sample at 95-105°C for 4 hours (Henken et al., 1986), and crude ash by high-temperature combustion at 550 ± 25°C (AOAC, 2023). Crude lipid content was determined using the Soxhlet extraction method, with Methyl tert-Butyl Ether (MTBE, boiling point: 40-60°C, Sigma, USA) as a solvent (Katikou and Robb, 2001). Crude protein content was assessed using the Kjeldahl method, and crude protein was estimated by multiplying the nitrogen percentage of 6.25 (Anantakrishnan and Srinivasa Pai, 1952).
2.4 Amino acid analysis
Amino acids were determined using high-performance liquid chromatography (HPLC, Agilent 1260, Germany) (Alaiz et al., 1992). The tissue samples were stored in the laboratory at -25°C for 24 hours. Subsequently, they were lyophilized for 48 hours at -53°C under a vacuum condition of less than 20 Pa using a freeze dryer (SCIENTZ-18N/A, Ningbo, China). Weigh the appropriate amount of freeze-dried sample into a 50 mL hydrolysis tube, and then samples (≥ 0.05 g) were hydrolyzed with 6 mol/L HCl at 110°C for 22 hours. Accurately, 100 μL of the sample was taken into a 15 mL centrifuge tube, placed in a vacuum drying oven, and dried at 60°C for 2 hours (the solvent was dried). After drying, the sample was diluted to 0.5 mL with water, mixed well and passed through a 0.45 μm organic membrane. After filtration and drying with nitrogen gas, the samples were neutralized and analyzed using a Hitachi amino acid analyzer (Hitachi L-8900, Tokyo, Japan) via EZChromElite software to detect amino acid contents. The tryptophan (Try) content was not measured in this study since Try was destroyed by acid hydrolysis.
2.5 Fatty acid analysis
The determination of fatty acids in S. schlegelii was performed using a gas chromatography-mass spectrometry (GC-MS) system (Trace 1310 ISQ, Waltham, USA). Briefly, a precisely measured homogenized sample was dispensed into a 100 mL tube and mixed with 2 mL of 95% ethanol, 4 mL of water, and 10 mL of 8.3 mol/L HCl. The mixture was hydrolyzed in an 80°C water bath for 40 minutes with periodic shaking, then cooled to room temperature. The hydrolyzed sample was mixed with 10 mL of 95% ethanol and extracted three times with 100 mL of an ether-petroleum ether mixture for fat extraction. The extract was evaporated to obtain the fat, which was then saponified and methylated by adding 4 mL of 2% sodium hydroxide in methanol and heating at 45°C for 30 minutes. Next, 4 mL of 14% boron trifluoride methanol solution (CNW) was added, and the mixture was heated at 45°C for another 30 minutes. After cooling, 3 mL of hexane was added, and the mixture was shaken for 2 minutes to extract fatty acid methyl esters (FAME). The upper layer of the supernatant was removed and filtered through a 0.45 μm membrane filter before analysis.
The GC-MS column used was a 50 m × 0.25 mm diameter column with a 0.25 μm film thickness. The gas chromatography conditions were as follows: initial temperature of 80°C held for 1 minute, increased to 160°C at a rate of 20°C/min and held for 1.5 minutes, then increased to 230°C at a rate of 5°C/min and held for 6 minutes. The injector temperature was set to 260°C, and the split ratio was 100:1. Fatty acid distribution was determined using FAME standards (Sigma, USA).
2.6 Data analysis
Statistical analysis was conducted using IBM SPSS 25.0. Prior to further analysis, all data were subjected to a test for normality based on the Shapiro-Wilk test and a test for homoscedasticity via the Levene test. The differences in moisture, crude ash, crude lipid, crude protein, amino acids, and fatty acids among the three groups were compared by one-way analysis of variance (ANOVA) with post hoc comparisons based on Tukey’s test or Nonparametric tests (Kruskal-Wallis Test). All analyses were performed at the significance level of P < 0.05 and P < 0.01, respectively. The results are presented as the mean ± S.E. of the mean unless otherwise stated. A paired sample t-test, otherwise, Wilcoxon signed-rank test was used to determine the significant differences in the nutritional value between hatchery-released fish and wild fish during the same month. The significance values have been adjusted by the Bonferroni correction for multiple tests.
3 Result
3.1 Proximate composition analysis
The proximate composition of the hatchery-reared, hatchery-released and wild S. schlegelii was presented in Table 1 and Figure 2. All groups were characterized by a high moisture content, consistently exceeding 77.225 ± 0.398 g/100 g wet weight (ww). Furthermore, the crude protein content was consistently higher than 16.936 ± 0.194 g/100 g (ww), while the lipid content was consistently higher than 0.848 ± 0.089 g/100 g (ww). In contrast, the crude ash content was always very low, not surpassing 1.482 ± 0.191 g/100 g (ww). Overall, in the comparison of all samples, there was no significant difference in crude ash content among hatchery-reared, hatchery-released and wild S. schlegelii, while the hatchery-reared S. schlegelii had relatively higher values (P > 0.05). The hatchery-reared S. schlegelii exhibited significantly higher moisture and crude lipid contents, but significantly lower crude protein content, compared to wild S. schlegelii (P < 0.05). The results showed a similar trend of mean value for proximate composition content in the hatchery-released S. schlegelii compared to wild S. schlegelii (Table 1).
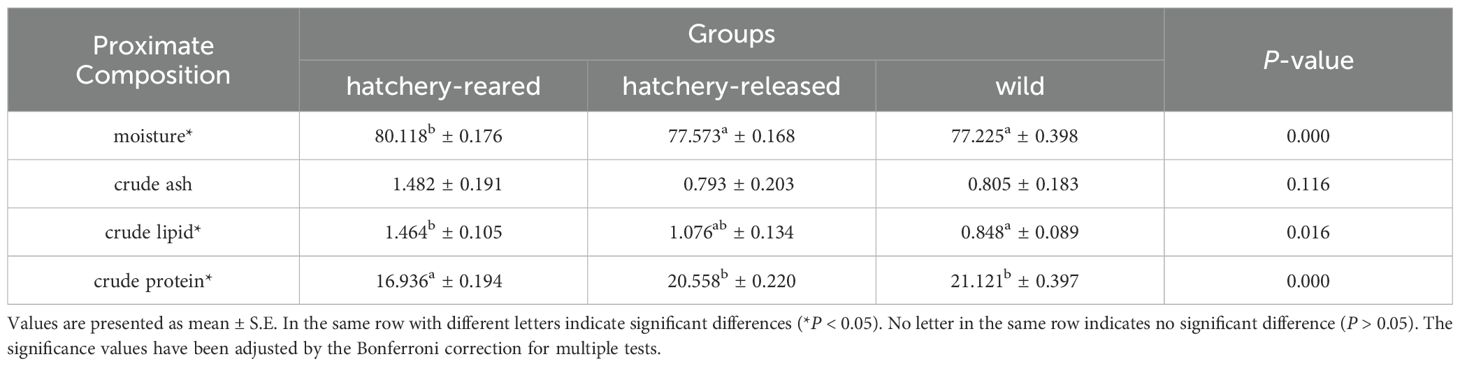
Table 1. Proximate composition of hatchery-reared, hatchery-released and wild S. schlegelii (g/100 g, wet weight).
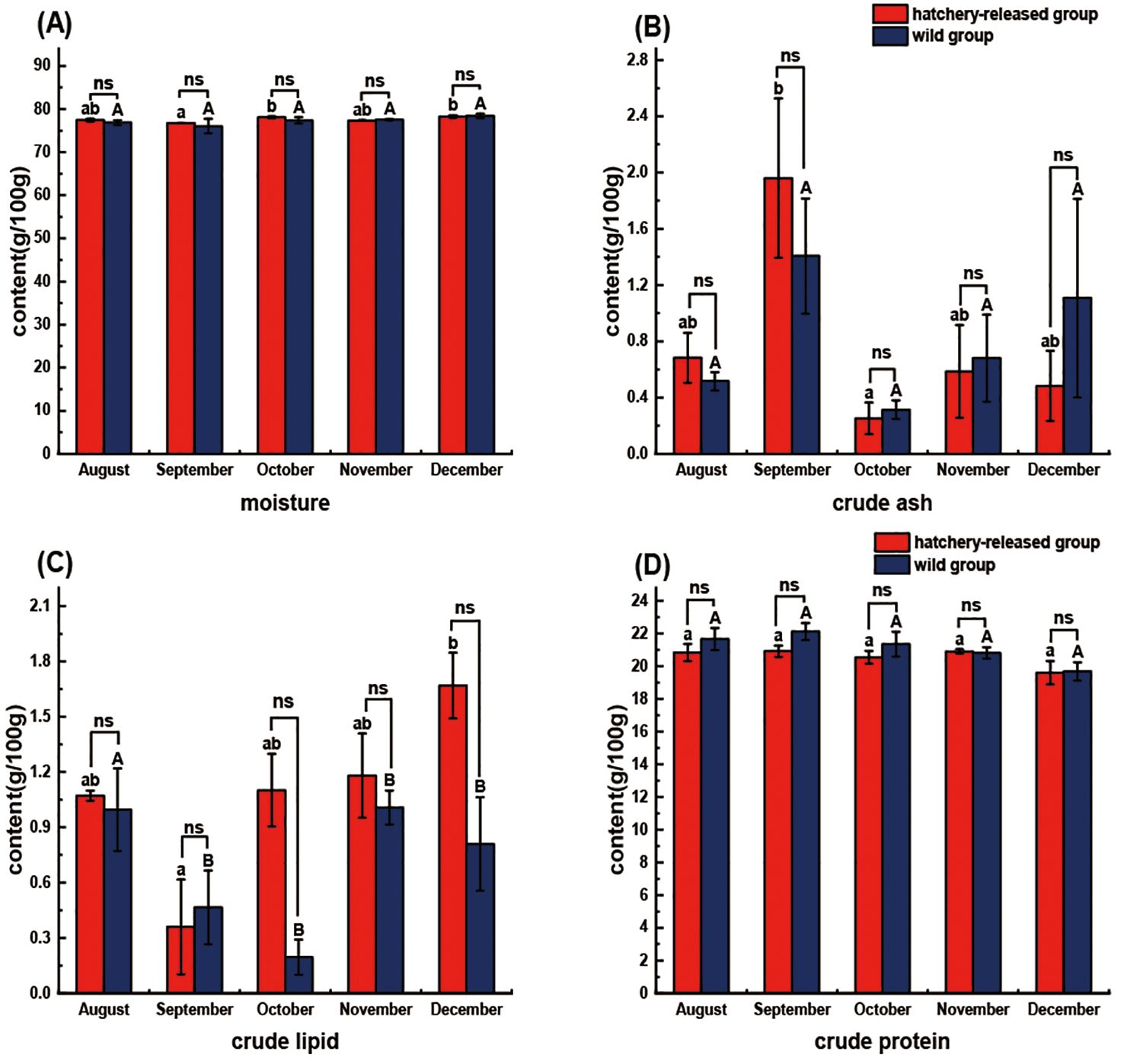
Figure 2. Comparison of proximate composition between hatchery-released and wild S. schlegelii. (A) moisture; (B) crude ash; (C) crude lipid; (D) crude protein. Red bar, hatchery-released S. schlegelii; blue bar, wild S. schlegelii. Different letters indicate significant differences (P < 0.05), and significant differences are compared within capital letters or lowercase letters. And ns, no significant differences; *, P < 0.05. Values are presented as mean ± S.E.
Significant differences were observed in the study of seasonal variations in proximate composition among hatchery-released S. schlegelii across different months. Specifically, S. schlegelii exhibited significantly higher levels of moisture in October and December compared to those in September (P < 0.05), whereas the crude ash content was notably lower in October relative to September (P < 0.05). Additionally, the crude lipid content in December was significantly higher than that in September (P < 0.05). The proximate composition of wild S. schlegelii was relatively steady from August to December. No significant differences were observed in moisture, crude ash, crude lipid, and crude protein content (P > 0.05). In contrast to the wild fish, the hatchery-released S. schlegelii exhibited an opposing trend in moisture and crude ash content variation (Figure 2). Comparison of hatchery-released and wild S. schlegelii in the same months revealed no significant difference in all the proximate composition content (P > 0.05) (Figure 2). It is worth noting that hatchery-released and wild S. schlegelii almost share the similar proximate profile trends during the post-release period in the wild, which can be inferred that hatchery-reared S. schlegelii can adjust quickly from “hatchery” to their wild counterparts in order to adapt to the natural environment.
3.2 Amino acids analysis
In this study, 17 amino acids were detected in S. schlegelii, including eight non-essential amino acids (NEAA: Asp, Glu, Cys, Ser, Ala, Pro, Tyr, His) and nine essential amino acids (EAA: His, Arg, Thr, Val, Met, Ile, Leu, Phe, Lys). The amino acid composition of hatchery-reared, hatchery-released, and wild S. schlegelii is displayed in Table 2. There was a significant difference in the NEAA content among these three groups, whereas intermediate value was also observed in hatchery-released S. schlegelii (P < 0.05), 7.985 ± 0.203 g/100 g (ww) (hatchery-reared) vs 9.327 ± 0.105 g/100 g (ww) (hatchery-released) vs 9.762 ± 0.083 g/100 g (ww) (wild), which is in agreement with the results of proximate composition. Except for the Pro, there was no significant difference in the levels of the other 16 types of amino acids between hatchery-released and wild S. schlegelii (P > 0.05). These 16 types of amino acid contents were significantly higher in both hatchery-released and wild S. schlegelii than in hatchery-reared S. schlegelii (P < 0.05). In fact, the hatchery-released and wild S. schlegelii were significantly richer in NEAA, EAA and total amino acid (TAA) than that of the hatchery-reared ones (P < 0.05) (Table 2). The results showed that the living environment obviously affected the amino acid contents of S. schlegelii.
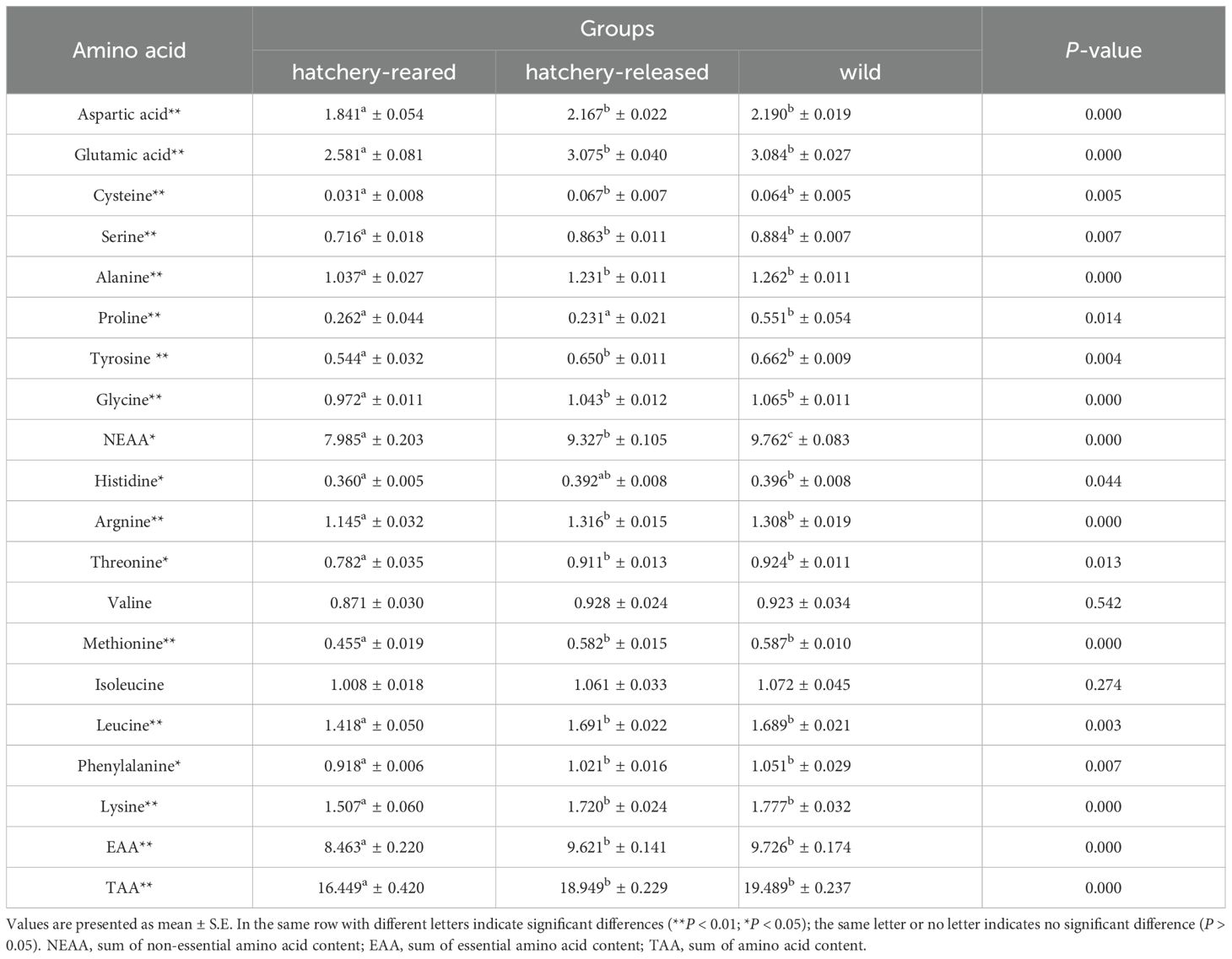
Table 2. Amino acid composition of hatchery-reared, hatchery-released and wild S. schlegelii (g/100 g, wet weight).
When comparing amino acid composition between months within the hatchery-released or wild group, separately, we also only found limited significant contrasts, such as Phe and Lys in wild fish and Ser in hatchery-released fish, respectively (Table 2 and Figure 3). Concerning the whole investigation period from August to December, we actually found relatively slight differences in the amino acid profile between hatchery- released and wild S. schlegelii (P < 0.05), only for Ser in October, 0.819 ± 0.009 g/100 g (ww) (hatchery-released) vs 0.909 ± 0.023 g/100 g (ww) (wild); Pro in October, 0.206 ± 0.055 g/100 g (ww) (hatchery-released) vs 0.401 ± 0.059 g/100 g (ww) (wild), November 0.263 ± 0.043 g/100 g (ww) (hatchery-released) vs 0.655 ± 0.025 g/100 g (ww) (wild), and December 0.257 ± 0.087 g/100 g (ww) (hatchery-released) vs 0.624 ± 0.055 g/100 g (ww) (wild); Val in August 0.903 ± 0.044 g/100 g (ww) (hatchery-released) vs 1.020 ± 0.019 g/100 g (ww) (wild), as well as Lys in August 1.681 ± 0.038 g/100 g (ww) (hatchery-released) vs 1.932 ± 0.016 g/100 g (ww) (wild).
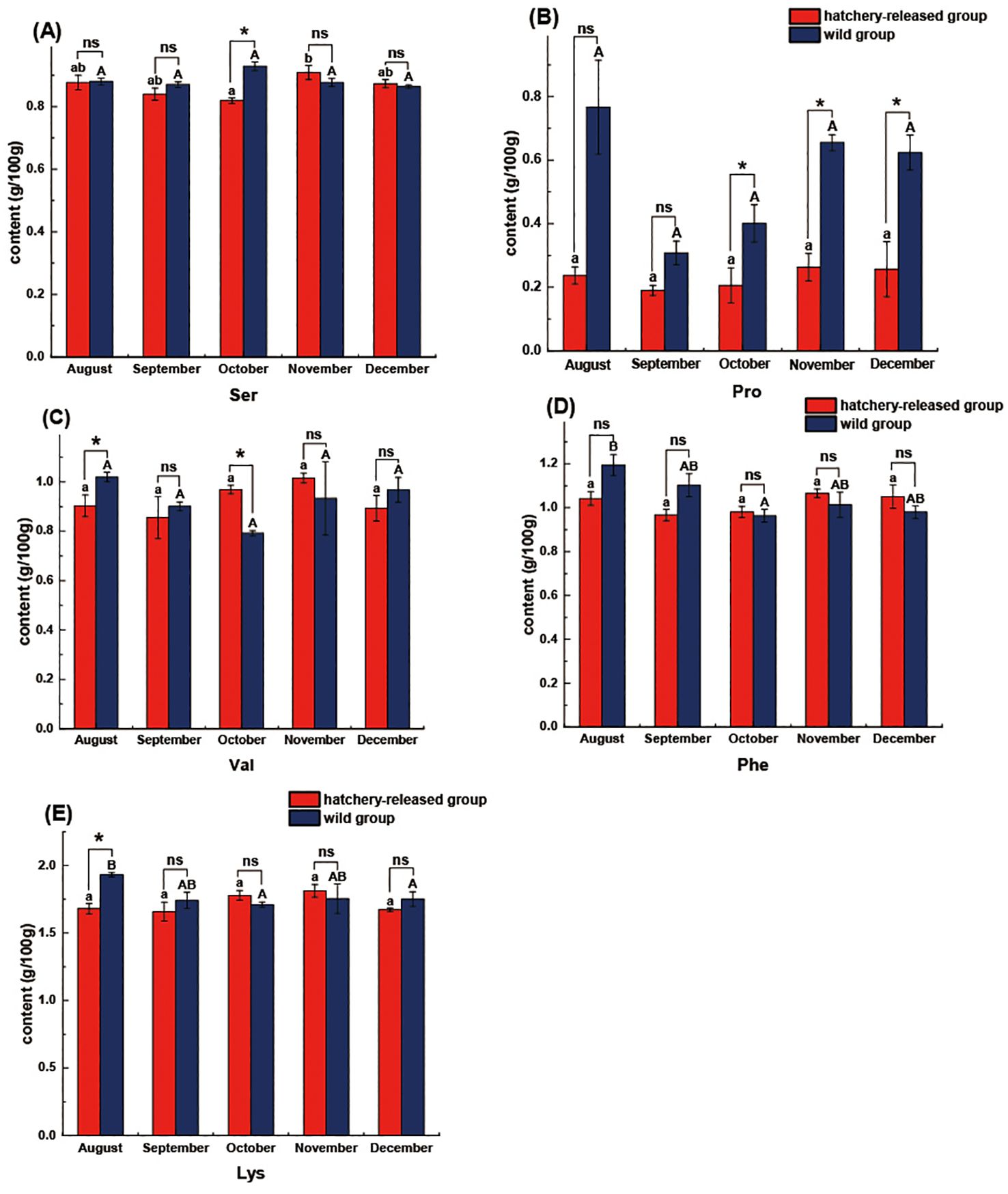
Figure 3. Comparison of amino acid content between hatchery-released and wild S. schlegelii. (A) Ser; (B) Pro; (C) Val; (D) Phe; (E) Lys. Red bar, hatchery-released S. schlegelii; blue bar, wild S. schlegelii. Different letters indicate significant differences (P < 0.05), and significant differences are compared within capital letters or lowercase letters. And ns, no significant differences; *, P < 0.05. Values are presented as mean ± S.E.
The amino acid profile indicated that hatchery-released S. schlegelii had obviously similar amino acid compositions with the wild S. schlegelii, but sharply in contrast to the hatchery-reared ones, and this finding is in accord with the results of proximate composition.
3.3 Fatty acids analysis
A total of 35 common fatty acids were detected in this study, of which 15 fatty acids were successfully identified. These include four saturated fatty acids (SFA: C14:0, C16:0, C18:0, C24:0), five monounsaturated fatty acids (MUFA: C16:1, C20:1, C24:1, C18:1n9c, C22:1n9) and six polyunsaturated fatty acids (PUFA: C18:2n6c, C18:3n3, C20:3n3, C20:4n6 (ARA), C20:5n3 (EPA), C22:6n3 (DHA)). The results are displayed in Table 3. The fatty acids profile demonstrated obvious differences among groups, being the general distribution between SFA, MUFA, and PUFA variables. Our analysis suggested that the hatchery-reared S. schlegelii had significantly higher SFA and MUFA content than that of hatchery-released and wild ones, 0.188 ± 0.016 g/100 g (ww) (hatchery-reared) vs 0.132 ± 0.012 g/100 g (ww) (hatchery-released) vs 0.116 ± 0.008 g/100 g (ww) (wild) for SFA; 0.23 ± 0.021 g/100 g (ww) (hatchery-reared) vs 0.125 ± 0.013 g/100 g (ww) (hatchery-released) vs 0.096 ± 0.006 g/100 g (ww) (wild) for MUFA, respectively (P < 0.05). In addition, hatchery-released and wild S. schlegelii also had less PUFA and total fatty acid contents than the hatchery-reared ones. In the first case (SFA), there was also a significant difference that hatchery-released and wild S. schlegelii was lack of C16:0 than hatchery-reared fish, 0.125 ± 0.011 g/100 g (ww) (hatchery-reared) vs 0.085 ± 0.008 g/100 g (ww) (hatchery-released) vs 0.075 ± 0.005 g/100 g (ww) (wild) (P < 0.05). In MUFA, whereas the hatchery-released and wild S. schlegelii exhibited significantly less contents of C16:1 and C18:1n9c, in contrast of hatchery-reared ones, 0.038 ± 0.005 g/100 g (ww) (hatchery-reared) vs 0.014 ± 0.002 g/100 g (ww) (hatchery-released) vs 0.011 ± 0.001 g/100 g (ww) (wild) in C16:1; 0.145 ± 0.014 g/100 g (ww) (hatchery-reared) vs 0.060 ± 0.007 g/100 g (ww) (hatchery-released) vs 0.052 ± 0.004 g/100 g (ww) (wild) in C18:1n9c, respectively (P < 0.01). For PUFA, hatchery-reared fish also showed the highest values, whether in C18:2n6c, EPA, DHA, n-3 PUFA, and n-6 PUFA contents (P < 0.05). On the contrary, hatchery-reared fish has less ARA than that of hatchery-released and wild ones, 0.012 ± 0.001 g/100 g (ww) (hatchery-reared) vs 0.026 ± 0002 g/100 g (ww) (hatchery-released) vs 0.019 ± 0.003 g/100 g (ww) (wild) in PUFA (P < 0.05).
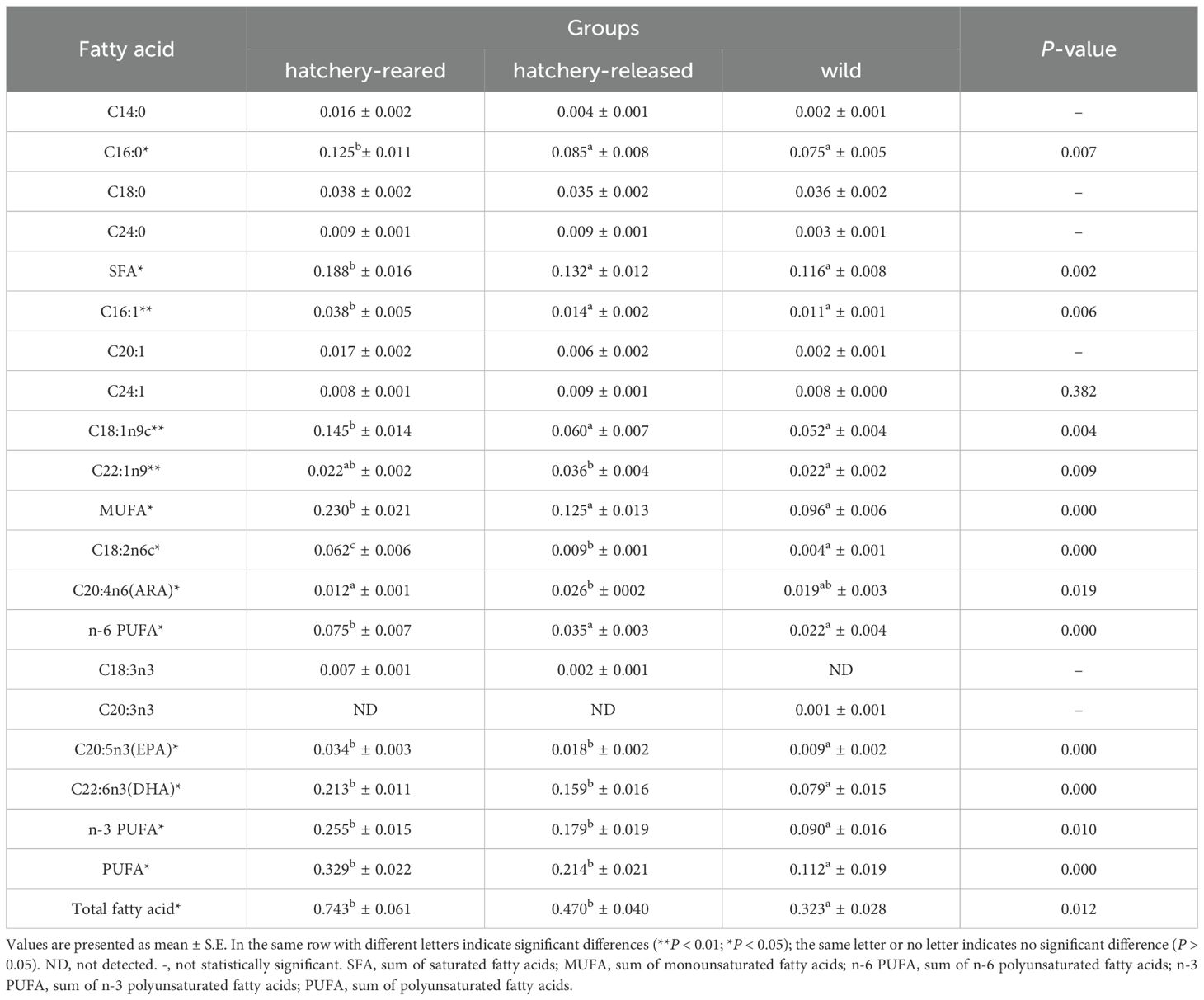
Table 3. Fatty acid composition of hatchery-reared, hatchery-released and wild S. schlegelii (g/100g, wet weight).
In the study of hatchery-released S. schlegelii during the whole investigation period, the fish is significantly richer C24:0, DHA, n-3 PUFA, PUFA, and total fatty acid contents in September than that of December (P < 0.05), while C24:1 content in August was significantly higher than that of November and December, respectively (P < 0.05). Furthermore, the hatchery-released S. schlegelii in August were also significantly richer C22:1n9 than in October (P < 0.05) (Figure 4). Additionally, the S. schlegelii was significantly richer n-6 PUFA content in September than in August, October, and December (P < 0.05). Concerning the wild S. schlegelii, there was no significant difference in fatty acid profile during the whole investigation period, except for C18:0 in SFA (Figure 4).
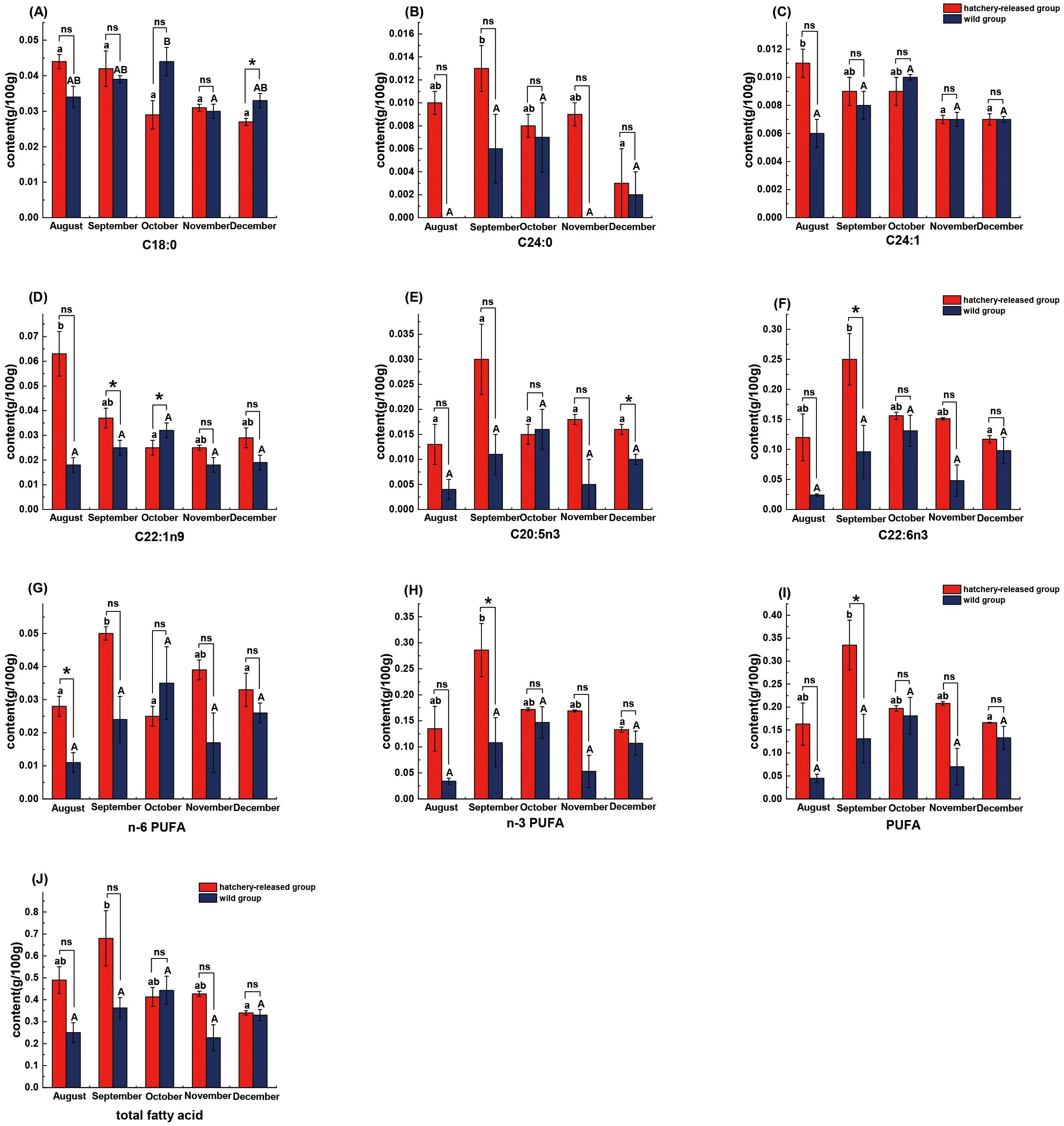
Figure 4. Comparison of fatty acid content between hatchery-released and wild S. schlegelii. (A) C18:0; (B) C24:0; (C) C24:1; (D) C22:1n9; (E) C20:5n3; (F) C22:6n3; (G) n-6 PUFA; (H) n-3 PUFA; (I) PUFA; (J) total fatty acid. Red bar, hatchery-released S. schlegelii; blue bar, wild S. schlegelii. Different letters indicate significant differences (P < 0.05), and significant differences are compared within capital letters or lowercase letters. And ns, no significant differences; *, P < 0.05. Values are presented as mean ± S.E.
In contrast to the fatty acid profile between hatchery-released and wild S. schlegelii from August to December, it was possible to suggest that hatchery-released and wild fish almost own the same SFA and MUFA, except for C22:1n9. Nevertheless, in fact, hatchery-released S. schlegelii was significantly richer in PUFA than the wild ones, 0.214 ± 0.021 g/100 g (ww) (hatchery-released) vs 0.112 ± 0.019 g/100 g (ww) (wild), This led to the finally that hatchery-released S. schlegelii had significantly higher total fatty acid content than wild S. schlegelii. Furthermore, the PUFA of hatchery-released S. schlegelii exhibited a certain degree of similarity, particularly in EPA and DHA, to that of hatchery-reared fish. In conclusion, the present findings indicate that hatchery-released S. schlegelii has not yet attained the same fatty acid profile as the wild S. schlegelii. Therefore, considering of the proximate compositions and amino acids, the observed changes in fatty acid composition appear to be a delay in response to environmental and dietary alterations.
4 Discussion
The S. schlegelii is a critical species for aquaculture and stock enhancement in the Northwest Pacific Ocean region. The nutritional value of fish is influenced by several factors, including the environment, the intrinsic characteristics of the fish, and the bait organisms (Alberdi et al., 2019). Fishes generally exhibited diverse plastic throughout their life history and gene-environment interactions, such as epigenetics could influence their phenotypes. Hence, fish physiology and biological phenotypes will develop differently depending on their living environment (Johnsson et al., 2014). These fish phenotypes could be regarded as ecologically adaptation indicators for hatchery-released fish (Johnsson et al., 2014). The success of a stock enhancement program requires the maintenance of economic profit while minimizing negative genetic and ecological effects (Taylor et al., 2017). On the other hand, a successful stock enhancement depends on the capacity of fish phenotypes that domesticated population readapts to the natural environment (Gil et al., 2014). Changes in phenotypes, such as nutritional compositions post-release, could be monitored to determine of whether hatchery-released fish adapt well to the wild environment or fail to adapt to wild conditions (Grigorakis, 2007). Considering limited study cases of ecological effects during stock enhancement at present, therefore, exploring the differences in nutritional profiles before-release (hatchery-reared) and post-release (hatchery-released vs wild) will contribute to clarifying the ecological effects and ultimately be beneficial for stock enhancement.
4.1 Proximate composition analysis
The present results showed that the crude protein content of hatchery-reared S. schlegelii was significantly lower than that of wild S. schlegelii (P < 0.05) (Table 1). This finding between hatchery-reared and wild S. schlegelii was in accordance with previous results of other species, such as silver pomfret (Pampus argenteus) (Zhao et al., 2010), large yellow croaker (Larimichthys crocea) (Guo et al., 2019) and S. schlegelii (Gao et al., 2023). Additionally, hatchery-reared S. schlegelii had significantly higher moisture and crude lipid content compared to wild ones (P < 0.05). Previous research showed that environmental factors such as temperature, salinity, and food availability could significantly contribute to the biochemical composition of an organism (Kim et al., 2015), which led to an environment-dependent biochemical composition within the species.
Interestingly, all of the moisture, crude lipid, and crude protein contents of the hatchery-released S. schlegelii exhibited intermediate values between hatchery-reared and wild ones in this research. It is possible to indicate that hatchery-released S. schlegelii seems to be a swift transition from “hatchery” to the native environment. Moreover, there was no significant difference in proximate profile from August to December between hatchery-released and wild fish, which also confirmed that hatchery-released S. schlegelii could adapt to the natural environment post-release.
4.2 Amino acid analysis
Amino acids are the fundamental components of proteins and play crucial roles in cell construction, antibody protein production, and tissue repair (Hou et al., 2024). There was no significant difference in most of the amino acid contents between hatchery-released and wild groups (P > 0.05), except that the hatchery-released fish exhibited a significantly lower Pro and then resulted in decreased NEAA content than that of wild counterparts (P < 0.05) (Table 2). Pro involves collagen biosynthesis and cellular metabolism (De et al., 2019). The cultural practice before release, including almost feeding a single pellet source, may ultimately cause differences in Pro levels between the hatchery-released and wild S. schlegelii in post-release (Oztekin et al., 2020).
In addition, the habitat and feeding behaviors were also markedly different between the natural environment and hatchery, which can quickly influence the nutrition profile of fish (Cardoso et al., 2023). The previous research found that the above factors had a profound effect on the amino acid profile in fish (De et al., 2019). The present results indicated that the hatchery-reared S. schlegelii generally exhibited significantly lower levels of amino acids than that of wild counterparts. This similar phenomenon was also observed in other fish species, such as P. hypophthalmus (Chakma et al., 2022), Axillary seabream (Pagellus acarne) (Oztekin et al., 2020), and Ussuri catfish (Pseudobagrus ussuriensis) (Wang et al., 2013). Although no significant difference was found in Val and Iso (P > 0.05) between before-release and post-release groups (Table 2), the most surprising finding is that hatchery-released S. schlegelii after release showed almost similar amino acid profiles with their wild counterparts (P > 0.05), but significantly different with hatchery-reared ones (P < 0.05). Previous research reported changes in amino acid levels within species could contribute to apparent seasonal variations in wild sea bass (Dicentrarchus labrax) (Özyurt and Polat, 2006). Nevertheless, no significant differences in NEAA, EAA and TAA in present research were observed regardless of hatchery-released or wild S. schlegelii from August to December. The observed discrepancies are likely to be attributable to differences in species.
On the other hand, amino acid comparative analyses of the hatchery-released and wild S. schlegelii in the same months revealed only a few specific amino acids (4 out of 17 amino acids), including Ser, Pro, Val, Lys exhibited significant differences (P < 0.05) (Figure 3), no significant differences were found in most of amino acid composition as well as the levels of NEAA, EAA, or TAA across months.
Adaptation to natural habitats and food sources is critical for the survival of hatchery-released fish (Tomiyama et al., 2011). Post-release predation in hatchery-released fish can be expected to occur in the wild in order to survive and adapt to the environment and ultimately exhibit similar nutrition profiles to wild fish. The similarity amino acid composition profile in this research between hatchery-released and wild S. schlegelii was consistent with the above proximate composition finding, which indicated that the hatchery-released S. schlegelii, after release, could transfer from “hatchery” to the wild in order to adapt to their natural environment.
4.3 Fatty acid analysis
The fatty acid results of the present study were in accordance with previous research about S. schlegeli (Han et al., 2019). The primary fatty acids for SFA, MUFA, and PUFA in S. schlegeli are C16:0, C18:1n9c, and DHA, respectively (Han et al., 2019). In our study, the levels of SFA and MUFA were significantly higher in the hatchery-reared group compared to the hatchery-released and wild groups (P < 0.05). This similar tendency has also been shown in other fish species, such as rainbow trout (Oncorhynchus mykiss) (Fallah et al., 2011) and yellow tail (Seriola lalandi) (O'Neill et al., 2015). The observed variations in the results can likely be attributed to a complex interplay of several key environmental variables, including fluctuations in temperature, variations in salinity levels, and the availability and diversity of food sources (Silva et al., 2021). In our results, the n-6 PUFA content of the hatchery-released S. schlegelii was close to the wild S. schlegelii, but significantly lower than the hatchery-reared fish (P < 0.05). In O. mykiss (Fallah et al., 2011), S. lalandi (O'Neill et al., 2015), and P. hypophthalmus (Chakma et al., 2022) the hatchery-reared group has obviously more n-6 PUFA than that of the wild group. In contrast, we found both the hatchery-released and hatchery-reared S. schlegelii displayed similar n-3 PUFA (for instance, EPA and DHA), as well as the PUFA content, but significantly higher than that of the wild fish (P < 0.05). It may be attributed to the fact that the food resource of hatchery-reared S. schlegelii was rich in PUFA, regardless of n-6 PUFA or n-3 PUFA before release. However, the PUFA availability in the wild seemed to be limited, resulting in a decline in the PUFA content of the hatchery-released fish subsequently (Cardoso et al., 2023). In general, the DHA and EPA are essential for biological membranes, nerves, and vision, and are not easily facilitated for organism energy supply (Zavorka et al., 2023). It is worth noting that hatchery-released S. schlegelii consumed a relatively greater proportion of n-6 PUFA and a lower proportion of n-3 PUFA in the wild. Consequently, the n-6 PUFA content of the hatchery-released S. schlegelii is close to the wild counterparts, while the n-3 PUFA is close to the hatchery-reared counterparts. Furthermore, the total fatty acid content in the hatchery-released group was also similar to that of the wild group. These findings indicate that the hatchery-released S. schlegelii is adapting to fatty acid changes from “hatchery” to the “wild” in consideration of the district food supply and living environment after release (Lee et al., 2021).
A five-month investigation in the present study revealed that fatty acid profiles within hatchery-released and wild S. schlegelii exhibited seasonal variation. However, the trends of fatty acid contents between them were distinctly different. There were 4 out of 15 types of fatty acid (C24:0, C24:1, C22:1n9, and DHA) showed significant differences in the hatchery-released S. schlegelii. In contrast, the fatty acid level of wild S. schlegelii was relatively steady from August to December, except C18:0 in October and November, respectively (P < 0.05). Since the availability of food in the natural environment is subject to variations in temperature, salt content, influx of freshwater, and exposure to solar energy (Xu et al., 2022), changes in the above environmental conditions in different seasons may have an influence on the abundance of prey (Nakajima et al., 2013). And wild S. schlegelii seemed to have developed an effective adaptive mechanism in response to such seasonal fluctuations in fatty acid levels, but hatchery-released S. schlegelii were not yet. Several fatty acid compositions, such as C18:0, C22:1n9, and DHA, showed significant differences between hatchery-released and wild counterparts, which may also be due to the above reasons.
5 Conclusion
This study has provided nutritional profile information on the before-release and post-release of S. schlegelii, which can provide us with a deep insight into the ecological effects derived from stock enhancement. The hatchery-reared S. schlegelii exhibited contrasting nutritional characteristics to their wild counterparts, with significantly higher moisture, crude lipid, and fatty acids, but significantly lower protein and amino acids. However, above distinct variations in nutritional composition were not observed between hatchery-reared and hatchery-released fish. Hatchery-released fish exhibited intermediate nutritional composition values among these three groups. The nutritional trends observed in both hatchery-released and wild S. schlegelii were found to be almost similar at different times post-release. Our results suggest that hatchery-reared S. schlegelii can adjust from “hatchery” to their wild counterparts and become adaptable to the natural environment after release. However, several significant differences in fatty acid composition indicate that hatchery-released fish did not seem to adapt to the food supply post-release completely. Future studies should pay more attention to understanding the ecological effects of stock enhancement.
Data availability statement
The original contributions presented in the study are included in the article/Supplementary Material, further inquiries can be directed to the corresponding author.
Ethics statement
The animal study was approved by Animal experiments were conducted at the Fisheries Resources Laboratory of Dalian Ocean University, in accordance with Chinese laws, regulations, and ethical principles. The study was conducted in accordance with the local legislation and institutional requirements.
Author contributions
LW: Data curation, Investigation, Methodology, Writing – original draft. YH: Investigation, Methodology, Writing – review & editing. XW: Investigation, Methodology, Writing – review & editing. XL: Methodology, Writing – review & editing. RZ: Methodology, Writing – review & editing. QL: Conceptualization, Investigation, Methodology, Writing – review & editing. CW: Investigation, Writing – review & editing. JC: Investigation, Writing – review & editing. ML: Methodology, Writing – review & editing. ZM: Resources, Writing – review & editing. SQ: Resources, Writing – review & editing.
Funding
The author(s) declare financial support was received for the research, authorship, and/or publication of this article. This study was supported by the National Natural Science Foundation of China (42030408), Dalian Outstanding Young Talents in Science and Technology (2023RJ010), the Project of National Key Research and Development Program of China (2023YFD2401103), General Program of Liaoning Natural Science Foundation (2023-MSLH-001), the General Project of Education Department of Liaoning Province (LJ212410158023, 2024JBZDZ002).
Conflict of interest
The authors declare that the research was conducted in the absence of any commercial or financial relationships that could be construed as a potential conflict of interest.
Generative AI statement
The author(s) declare that no Generative AI was used in the creation of this manuscript.
Publisher’s note
All claims expressed in this article are solely those of the authors and do not necessarily represent those of their affiliated organizations, or those of the publisher, the editors and the reviewers. Any product that may be evaluated in this article, or claim that may be made by its manufacturer, is not guaranteed or endorsed by the publisher.
Supplementary material
The Supplementary Material for this article can be found online at: https://www.frontiersin.org/articles/10.3389/fmars.2024.1536508/full#supplementary-material
References
Alaiz M., Navarro J. L., Girón J., Vioque E. (1992). Amino acid analysis by high-performance liquid chromatography after derivatization with diethyl ethoxymethylenemalonate. J. Chormatogr A. 591, 181–186. doi: 10.1016/0021-9673(92)80236-N
Alberdi A., Aizpurua O., Bohmann K., Gopalakrishnan S., Lynggaard C., Nielsen M., et al. (2019). Promises and pitfalls of using high-throughput sequencing for diet analysis. Mol. Ecol. Res. 19, 327–348. doi: 10.1111/1755-0998.12960
Anantakrishnan S. V., Srinivasa Pai K. V. (1952). The kjeldahl method of nitrogen determination. Proc. Indian Acad. Sci. 36, 299–305. doi: 10.1007/BF03172239
AOAC (2023).Official method 938.08 ash of seafood. In: Official Methods of Analysis of AOAC INTERNATIONAL (New York: AOAC Publications) (Accessed 2626 November 2024).
Blaxter J. H. S. (2000). The enhancement of marine fish stocks. Adv. Mar. Biol. 38, 1–54. doi: 10.1016/S0065-2881(00)38002-6
Cardoso C., Almeida J., Coelho I., Delgado I., Gomes R., Quintã R., et al. (2023). Farming a wild seaweed and changes to its composition, bioactivity, and bioaccessibility: The Saccorhiza polyschides case study. Aquaculture 566, 739217. doi: 10.1016/j.aquaculture.2022.739217
Chakma S., Rahman M. A., Siddik M. A. B., Hoque M. S., Islam S. M. M., Vatsos I. N. N. (2022). Nutritional Profiling of Wild (Pangasius pangasius) and Farmed (Pangasius hypophthalmus) Pangasius Catfish with Implications to Human Health. FISHES-BASEL 7, 309. doi: 10.3390/fishes7060309
Chin B. S., Nakagawa M., Noda T., Wada T., Yamashita Y. (2013). Determining optimal release habitat for black rockfish, sebastes schlegelii: examining growth rate, feeding condition, and return rate. Rev. Fish Sci. Aquac. 21, 286–298. doi: 10.1080/10641262.2013.837364
De D., Mukherjee S., Anand P. S. S., Kumar P., Suresh V. R., Vijayan K. K. (2019). Nutritional profiling of hilsa (Tenualosa ilisha) of different size groups and sensory evaluation of their adults from different riverine systems. Sci. Rep. 9, 19306. doi: 10.1038/s41598-019-55845-w
Fallah A. A., Siavash Saei-Dehkordi S., Nematollahi A. (2011). Comparative assessment of proximate composition, physicochemical parameters, fatty acid profile and mineral content in farmed and wild rainbow trout (Oncorhynchus mykiss). Int. J. Food Sci. Tech 46, 767–773. doi: 10.1111/j.1365-2621.2011.02554.x
FAO (2024). The State of World Fisheries and Aquaculture 2024 – Towards Blue Transformation in action. (Rome: Food and Agriculture Organization). doi: 10.4060/cd0683en
Gao X., Zhai H., Peng Z., Yu J., Yan L., Wang W., et al. (2023). Comparison of nutritional quality, flesh quality, muscle cellularity, and expression of muscle growth-related genes between wild and recirculating aquaculture system (RAS)-farmed black rockfish (Sebastes schlegelii). Aquacult Int. 31, 2263–2280. doi: 10.1007/s10499-023-01087-7
Gil M. M., Palmer M., Grau A., Deudero S., Alconchel J. I., Catalan I. A. (2014). Adapting to the wild: the case of aquaculture-produced and released meagres Argyrosomus regius. J. Fish Biol. 84, 10–30. doi: 10.1111/jfb.12241
Grigorakis K. (2007). Compositional and organoleptic quality of farmed and wild gilthead sea bream (Sparus aurata) and sea bass (Dicentrarchus labrax) and factors affecting it: A review. Aquaculture 272, 55–75. doi: 10.1016/j.aquaculture.2007.04.062
Guo Q. Y., Xing X. L., Wang L., Song W., Jiang Z. J., Wang L. M. (2019). Study on the difference of nutrition and quality between deep-water cage culture and wild large yellow croaker. Fish Inform Strateg 34, 53–60. doi: 10.13233/j.cnki.fishis.2019.01.009
Han H. Z., Wang T. T., Zhang M. L., Wang F., Liu Y., Sun N., et al. (2019). Analysis on the composition of amino acid and fatty acid and their changes during early growth stage of Sebastes schlegelii. Acta Hydrobiol Sin. 43, 526–536. doi: 10.7541/2019.064
Henken A. M., Lucas H., Tijssen P. A. T., Machiels M. A. M. (1986). A comparison between methods used to determine the energy content of feed, fish and feces samples. Aquaculture. 58, 195–201. doi: 10.1016/0044-8486(86)90085-2
Hoopes L. A., Clauss T. M., Browning N. E., Delaune A. J., Wetherbee B. M., Shivji M., et al. (2020). Seasonal patterns in stable isotope and fatty acid profiles of southern stingrays (Hypanus americana) at Stingray City Sandbar, Grand Cayman. Sci. Rep. 10, 19753. doi: 10.1038/s41598-020-76858-w
Hou M., Sun W., Ma Y., Ye H., Zhai X., Xue Y., et al. (2024). Comparative analysis for nutrients, flavor compounds, and lipidome revealed the edible value of pond-cultured male Pelodiscus sinensis with different ages. Food Chem. 454, 139795. doi: 10.1016/j.foodchem.2024.139795
Hunt T. L., Scarborough H., Giri K., Douglas J. W., Jones P. (2017). Assessing the cost-effectiveness of a fish stocking program in a culture-based recreational fishery. Fish Res. 186, 468–477. doi: 10.1016/j.fishres.2016.09.003
Johnsson J. I., Brockmark S., Naeslund J. (2014). Environmental effects on behavioural development consequences for fitness of captive-reared fishes in the wild. J. Fish Biol. 85, 1946–1971. doi: 10.1111/jfb.12547
Katikou P., Robb D. H. F. (2001). Evaluation and comparison of the CEM rapid extraction method with official standard methods for the determination of lipid content in fillets of farmed Atlantic salmon (Salmo salar). Aquaculture 194, 99–105. doi: 10.1016/s0044-8486(00)00500-7
Kim Y. S., Delgado D. I., Cano A., Sawada Y. (2015). Effect of temperature and salinity on hatching and larval survival of yellowfin tuna Thunnus albacares. Fish Sci. 81, 891–897. doi: 10.1007/s12562-015-0901-8
Kitada S. (2018). Economic, ecological and genetic impacts of marine stock enhancement and sea ranching: A systematic review. Fis Fish. 19, 511–532. doi: 10.1111/faf.12271
Kitada S. (2020). Lessons from Japan marine stock enhancement and sea ranching programmes over 100 years. Rev. Aquacult. 12, 1944–1969. doi: 10.1111/raq.12418
Lee D.-Y., Lee C.-H., Kim K.-D., Lim H. J., Kim H. S. (2021). Effects of diet supplementation with plant juice processing by-products on juvenile black rockfish (Sebastes schlegelii) growth performance, feed utilization, non-specific immunity, and disease resistance against Vibrio harveyi. Aquacult. Rep. 21, 100831. doi: 10.1016/j.aqrep.2021.100831
Liu Q., Cui F., Hu P. F., Yi G. T., Ge Y. W., Liu W. L., et al. (2018). Using of microsatellite DNA profiling to identify hatchery-reared seed and assess potential genetic risks associated with large-scale release of swimming crab Portunus trituberculatus in Panjin, China. Fish Rese. 207, 187–196. doi: 10.1016/j.fishres.2018.05.003
Liu Q., Wang Z., Li W., Zhang Q., Liu B., Li M., et al. (2022). Genetic Stock Identification and Adaptability of Hatchery-Reared Black Rockfish, Sebastes schlegelii, Released Into the North Yellow Sea waters. Front. Mar. Sci. 09. doi: 10.3389/fmars.2022.800607
McGreer M., Frid A. (2017). Declining size and age of rockfishes (Sebastes spp.) inherent to Indigenous cultures of Pacific Canada. Ocean Coast. Manage. 145, 14–20. doi: 10.1016/j.ocecoaman.2017.04.019
Munakata A., Björnsson B. T., Jönsson E., Amano M., Ikuta K., Kitamura S., et al. (2000). Post-release adaptation processes of hatchery-reared honmasu salmon parr. J. Fish Biol. 56, 163–172. doi: 10.1006/jfbi.1999.1147
Nakajima K., Kitada S., Yamazaki H., Takemori H., Obata Y., Iwamoto A., et al. (2013). Ecological interactions between hatchery and wild fish: a case study based on the highly piscivorous Japanese Spanish mackerel. Aquacult Env. Interac. 3, 231–243. doi: 10.3354/aei00065
O'Neill B., Le Roux A., Hoffman L. C. (2015). Comparative study of the nutritional composition of wild versus farmed yellowtail (Seriola lalandi). Aquaculture. 448, 169–175. doi: 10.1016/j.aquaculture.2015.05.034
Oh H. Y., Lee T. H., Lee C.-H., Lee D.-Y., Sohn M.-Y., Kwon R.-W., et al. (2023). Effects of by-products from producing yacon (Smallanthus sonchifolius) juice as feed additive on growth performance, digestive enzyme activity, antioxidant status, related gene expression, and disease resistance against Streptococcus iniae in juvenile black rockfish (Sebastes schlegelii). Aquaculture 569, 739383. doi: 10.1016/j.aquaculture.2023.739383
Oztekin A., Yigit M., Kizilkaya B., Ucyol N., Tan E., Yilmaz S., et al. (2020). Nutritional quality of amino acid in farmed, farm-aggregated and wild Axillary seabream (Pagellus acarne ) with implications to Human Health. Aquacult Res. 5), 1844–1853. doi: 10.1111/are.14534
Özyurt G., Polat A. (2006). Amino acid and fatty acid composition of wild sea bass (Dicentrarchus labra): a seasonal differentiation. Eur. Food Res. Technol. 222, 316–320. doi: 10.1007/s00217-005-0040-z
Pinter K., Epifanio J., Unfer G. (2019). Release of hatchery-reared brown trout (Salmo trutt) as a threat to wild populations? A case study from Austria. Fish Res. 219, 105296. doi: 10.1016/j.fishres.2019.05.013
Richardson L. E., Lenfant P., Clarke L. J., Fontcuberta A., Gudefin A., Lecaillon G., et al. (2023). Examining current best-practices for the use of wild post-larvae capture, culture, and release for fisheries enhancement. Front. Mar. Sci. 9. doi: 10.3389/fmars.2022.1058497
Rosburg A. J., Fletcher B. L., Barnes M. E., Treft C. E., Bursell B. R. (2019). Vertically-suspended environmental enrichment structures improve the growth of juvenile landlocked fall chinook salmon. Int. J. Innov. Stud. Aquat Biol. Fish 5, 17–24. doi: 10.20431/2454-7670.0501004
Silva D. C. C., Neto J. M., Nunes C., Gonçalves F. J. M., Coimbra M. A., Marques J. C., et al. (2021). Assessment of seasonal and spatial variations in the nutritional content of six edible marine bivalve species by the response of a set of integrated biomarkers. Ecol. Indic. 124, 107378. doi: 10.1016/j.ecolind.2021.107378
Sprague M., Dick J. R., Tocher D. R. (2016). Impact of sustainable feeds on omega-3 long-chain fatty acid levels in farmed Atlantic salmon 2006-2015. Sci. Rep. 6, 21892. doi: 10.1038/srep21892
Taylor M. D., Chick R. C., Lorenzen K., Agnalt A.-L., Leber K. M., Lee Blankenship H., et al. (2017). Fisheries enhancement and restoration in a changing world. Fish Res. 186, 407–412. doi: 10.1016/j.fishres.2016.10.004
Tomiyama T., Watanabe M., G.Ebe K. (2011). Post-release feeding and growth of hatchery-reared Japanese flounder Paralichthys olivaceus: relevance to stocking effectiveness. J. Fish Biol. 78, 1423–1436. doi: 10.1111/j.1095-8649.2011.02949.x
Turek J., Randak T., Horky P., Zlabek V., Velisek J., Slavik O., et al. (2010). Post-release growth and dispersal of pond and hatchery-reared European grayling Thymallus thymallus compared with their wild conspecifics in a small stream. J. Fish Biol. 76, 684–693. doi: 10.1111/j.1095-8649.2009.02526.x
Wang Q. L., Si F., Yu S. D., Yuan L. L., Yu Q. H., Jiang X. F., et al. (2019). Identification and adaptability of hatchery-origin Japanese flounder released into the Bohai Sea after the summer fishing moratorium. Aquaculture. 504, 164–171. doi: 10.1016/j.aquaculture.2018.11.039
Wang Y., Yu S., Ma G., Chen S., Shi Y., Yang Y. (2013). Comparative study of proximate composition and amino acid in farmed and wild Pseudobagrus ussuriensis muscles. Int. J. Food Sci. Tech. 49, 983–989. doi: 10.1111/ijfs.12391
Worm B., Branch T. A. (2012). The future of fish. Trends Ecol. Evol. 27, 594–599. doi: 10.1016/j.tree.2012.07.005
Xu H. G., Bi Q. Z., Meng X. X., Duan M., Wei Y. L., Liang M. Q. (2022). Response of lipid and fatty acid composition of turbot to starvation under different dietary lipid levels in the previous feeding period. Food Res. Int. 151, 110905. doi: 10.1016/j.foodres.2021.110905
Zavorka L., Blanco A., Chaguaceda F., Cucherousset J., Killen S. S., Lienart C., et al. (2023). The role of vital dietary biomolecules in eco-evo-devo dynamics. Trends Ecol. Evol. 38, 72–84. doi: 10.1016/j.tree.2022.08.010
Keywords: Sebastes schlegelii, stock enhancement, before-release, post-release, nutritional composition
Citation: Wang L, He Y, Wei X, Liang X, Zhang R, Liu Q, Wang C, Chen J, Li M, Ma Z and Qu S (2025) Seasonal variations and comparative nutritional composition of hatchery-reared, hatchery-released, and wild black rockfish (Sebastes schlegelii). Front. Mar. Sci. 11:1536508. doi: 10.3389/fmars.2024.1536508
Received: 29 November 2024; Accepted: 31 December 2024;
Published: 23 January 2025.
Edited by:
Lijuan Wang, Chinese Academy of Sciences (CAS), ChinaReviewed by:
Shengyong Xu, Zhejiang Ocean University, ChinaAmit Ranjan, Tamil Nadu Fisheries University, India
Na Song, Ocean University of China, China
Copyright © 2025 Wang, He, Wei, Liang, Zhang, Liu, Wang, Chen, Li, Ma and Qu. This is an open-access article distributed under the terms of the Creative Commons Attribution License (CC BY). The use, distribution or reproduction in other forums is permitted, provided the original author(s) and the copyright owner(s) are credited and that the original publication in this journal is cited, in accordance with accepted academic practice. No use, distribution or reproduction is permitted which does not comply with these terms.
*Correspondence: Qi Liu, bGl1cWlzdW5zb25AMTYzLmNvbQ==