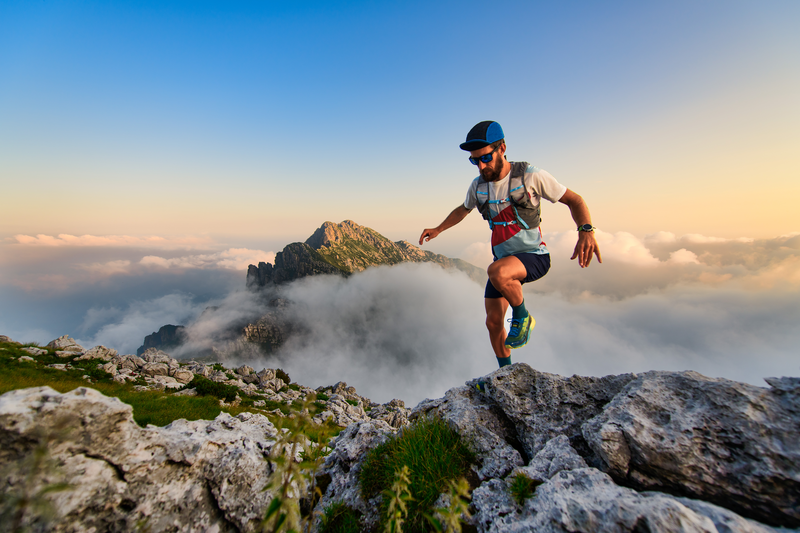
94% of researchers rate our articles as excellent or good
Learn more about the work of our research integrity team to safeguard the quality of each article we publish.
Find out more
EDITORIAL article
Front. Mar. Sci. , 17 December 2024
Sec. Marine Biogeochemistry
Volume 11 - 2024 | https://doi.org/10.3389/fmars.2024.1531887
This article is part of the Research Topic Role of the Southern Ocean in Atmospheric pCO2 Change: Observations, Simulations and Paleorecords View all 13 articles
Editorial on the Research Topic
Role of the Southern Ocean in atmospheric pCO2 change: observations, simulations and paleorecords
The Southern Ocean plays a key role in atmospheric CO2 sequestration, accounting for ~40-50% of the anthropogenic CO2 absorbed by the modern ocean (Landschützer et al., 2015; Gruber et al., 2019). The Southern Ocean also played a critical role in modulating variation in the atmospheric partial pressure of carbon dioxide (pCO2) in the geologic past on both orbital and millennial timescales (Anderson et al., 2009; Sigman et al., 2010; Gottschalk et al., 2016). Moreover, the Southern Ocean influences atmospheric and oceanic circulation in the tropics remotely, including low-latitude atmospheric CO2 exchange (Sarmiento et al., 2004; Hendry and Brzezinski, 2014; Sigman et al., 2021). Thus, the Southern Ocean is a key component of the global climate system through its influence on atmospheric CO2 variations at a range of timescales (Fischer et al., 2010; Rae et al., 2018; Dong et al., 2024). However, the processes and mechanisms of Southern Ocean influence on atmospheric pCO2 and global climate changes are still not well understood. To fill this gap, this Research Topic integrates the results of modern observations, paleoclimate data, and model simulations to promote a comprehensive understanding of the significance of the Southern Ocean in global climate change from the perspective of the carbon cycle.
This Research Topic collected 12 articles, including 11 original research articles and 1 perspective article. The articles focus on in-situ analyses of carbon and other nutrients and watermass factors, recent advances in simulation of the effects of overturning circulation on atmospheric pCO2, and palaeoceanographic reconstructions of carbon cycle (-related) processes. These articles can be classified into the three themes explored below.
Li et al. analyzed sea-surface temperature (SST) data from the Southern Ocean since 1870 to resolve why SSTs have been cooling since 1980 despite a global warming trend (Yang et al., 2023). They found that three main modes [i.e., the Global Temperature Anomaly, Atlantic Multidecadal Oscillation (AMO), and El Niño-Southern Oscillation (ENSO)] can explain over 70% of SST variability. The negative phase of the AMO and the positive phase of the ENSO counteract the effects of global warming, resulting in a cooling trend in Southern Ocean SSTs since 1980.
Zhang et al. identified strong seasonal variations of siliceous microplankton fluxes and radiolarian assemblages from a sediment trap, which are dominated by seasonality in sea ice cover and plankton community composition in Prydz Bay polynya, Antarctica. The results of this study provide new insights concerning the fluxes and assemblages of siliceous microplankton as carbon-cycle proxies in the geological past (Ragueneau et al., 2000; Tréguer et al., 2018).
Huang et al. analyzed iron (Fe) speciation and examined the relationship of highly reactive Fe (FeHR) and organic carbon in surface sediments from Prydz Bay and the adjacent Southern Ocean. They discovered that the FeHR to total Fe (FeT) ratios are lowest for the surface sediments in the global ocean due to weak bedrock weathering and slow glacier melting. They proposed that the influence of FeHR on sedimentary organic matter significantly varies across different sedimentary environments and sediment categories.
Wu et al. analyzed grain size, coarse fraction lithology, and clay mineral composition of surface sediments to constrain their provenance and delivery mode in the Ross Sea, Antarctica. They identified four types of bulk sediments, classified three sorts of coarse fractions, and quantified the distribution of clay minerals. Their findings reveal that surface sediments were mainly transported by icebergs and bottom currents. This study demonstrates the dynamic character of glaciers draining into the Ross Sea, underscoring their potential contribution to future sea-level rise under global warming conditions.
Yang D. et al. reconstructed spatio-temporal variations of organic matter sources and environmental changes from surface and core sediments in the Ross Sea, Antarctica. They revealed distinct spatial patterns of organic matter sources related to production of marine phytoplankton and bacteria and terrestrial bryophytes, and inputs of mid-latitude dust and low-latitude higher plant leaf waxes. They also indicated obvious temporal variations of organic matter sources consistent with ice shelf dynamics since the Last Glacial Maximum (LGM).
Yang W. et al. used observational data of physical properties and carbon chemistry in the ocean-surface watermass to explore the influence of sea ice melting on surface pCO2 in the western Arctic Ocean. They discovered distinct controls on surface pCO2 variability of the Chukchi Sea continental shelf and the Canada Basin, with biological production being the dominant influence in the former area and various factors including biological production, ice meltwater dilution, air-sea CO2 exchange, and surface temperature being the dominant influences in the latter area. Hopefully, similar studies will be undertaken in the future in the Southern Ocean, where sea ice frequently grows and melts.
Menviel and Spence discussed the impact of the Southern Ocean circulation on atmospheric pCO2 based on the merging of published and newly performed simulations. Both strengthening and poleward shift of the Southern Hemispheric Westerlies can lead to natural CO2 outgassing. Enhanced Antarctic Bottom Water formation/transport can decrease deep-water natural dissolved inorganic carbon (DIC) concentrations and increase surface-ocean natural DIC concentrations. This study provides a new perspective on past, present and future relationships between changes in atmospheric CO2 and Southern Ocean circulation resulting from both dynamic and buoyancy forcing.
Hu et al. used surface productivity and deep redox proxies to constrain variations in the deep respired carbon pool of the Cosmonaut Sea, Antarctica, and its impact on the glacial atmospheric pCO2 budget. They found that waxing and waning of the respired carbon pool contributed to drawdown of atmospheric pCO2 during the LGM and rising atmospheric pCO2 during the Last Deglaciation, respectively.
Chen et al. suggested that weathered material from the Prydz Bay and Enderby Land coastlines is the main source of sediment to the Cosmonaut Sea, Antarctica. They also discussed the synchronization of East Antarctic Icesheet dynamics with changes in Antarctic climate since the LGM. The results of this study and Hu et al. are a critical supplement for palaeoceanographic research in the Cosmonaut Sea, which is rarely studied.
Lei et al. examined the historical impacts of Circumpolar Deep Water (CDW) on organic matter and ice-rafted debris (IRD) with a palaeoceanographic record from the Amundsen Sea, Antarctica. They identified six environmental events characterized by covariation among CDW intensity, organic carbon concentrations and isotopes, and IRD concentrations since ~6 ka. This study highlights the importance of the CDW in the regional environmental evolution of the Southern Ocean.
Kim et al. investigated glacial-interglacial changes in oceanic environments and depositional processes, linking them to icesheet/ice shelf variations using two sediment cores from the Bellingshausen Sea continental rise. They demonstrated that sediment composition, surface productivity, deep-ocean redox conditions, and bottom-water formation manifest a pronounced glacial cycle, which was closely linked to icesheet dynamics.
Kenlee et al. performed Fe speciation analyses in cores from the subtropical (18-33°N) Atlantic Ocean core that are dominated by eolian dust from the Sahara Desert. They suggested that dust fluxes decrease but bioavailability of eolian Fe increases with the distance of atmospheric transport. Proximal and distal sediments show inverse Fe speciation patterns (i.e., high versus low FeHR/FeT, respectively), which are linked to lower Fe solubility and primary production in the water column and to greater carbonate dilution/dissolution at proximal versus distal sites. This study underscores the significance of Fe dynamics in regulating glacial-interglacial atmospheric pCO2 variation in multiple oceanic regions, particularly in the Southern Ocean, where variation in dust delivery on glacial-interglacial timescales is pronounced (Lamy et al., 2014; Martínez-García et al., 2014).
The influence of the Southern Ocean on atmospheric pCO2 and global climate evolution is complicated, being related not only to the carbon cycle but also to the nitrogen and silicon cycles, sulfur and oxygen cycles, iron cycle, hydrological cycle, and icesheet/ice shelf dynamics. The articles collected on this Research Topic represent state-of-the-art investigations into the above-mentioned biogeochemical and dynamic processes at a range of timescales based on modern observations, paleoclimate data, and model simulations. These collective efforts are of great scientific significance in providing new insights concerning the role of the Southern Ocean in modulating variations in atmospheric pCO2 and global climate in the past, present and future. Despite the progress made by the studies in this Research Topic, key scientific issues remain incompletely understood and will require further investigation in the future in order to achieve a better understanding of the role of the Southern Ocean in global climate change from the perspective of the carbon cycle.
ZX: Conceptualization, Funding acquisition, Writing – original draft. XZ: Writing – review & editing. DQ: Writing – review & editing. JZ: Writing – review & editing. TA: Conceptualization, Writing – review & editing.
The author(s) declare that financial support was received for the research, authorship, and/or publication of this article. This study was supported by the National Natural Science Foundation of China (grant no. 42076232), the Impact and Response of Antarctic Seas to Climate Change (grant no. IRASCC2020-2022-01-03-02), and the Taishan Scholars Project Funding (grant nos. TS20190963 and TSQN202211265). We thank all the authors, reviewers, editors, staff who have participated in this Research Topic.
The authors declare that the research was conducted in the absence of any commercial or financial relationships that could be construed as a potential conflict of interest.
All claims expressed in this article are solely those of the authors and do not necessarily represent those of their affiliated organizations, or those of the publisher, the editors and the reviewers. Any product that may be evaluated in this article, or claim that may be made by its manufacturer, is not guaranteed or endorsed by the publisher.
Anderson R. F., Ali S., Bradtmiller L. I., Nielsen S. H. H., Fleisher M. Q., Anderson B. E., et al. (2009). Wind-driven upwelling in the Southern Ocean and the deglacial rise in atmospheric CO2. Science 323, 1443–1448. doi: 10.1126/science.1167441
Dong Y., Bakker D. C. E., Bell T. G., Yang M., Landschützer P., Hauck J., et al. (2024). Direct observational evidence of strong CO2 uptake in the Southern Ocean. Sci. Adv. 10, eadn5781. doi: 10.1126/sciadv.adn5781
Fischer H., Schmitt J., Lüthi D., Stocker T. F., Tschumi T., Parekh P., et al. (2010). The role of Southern Ocean processes in orbital and millennial CO2 variations - A synthesis. Quaternary Sci. Rev. 29, 193–205. doi: 10.1016/j.quascirev.2009.06.007
Gottschalk J., Skinner L. C., Lippold J., Vogel H., Frank N., Jaccard S. L., et al. (2016). Biological and physical controls in the Southern Ocean on past millennial-scale atmospheric CO2 changes. Nat. Commun. 7, 11539. doi: 10.1038/ncomms11539
Gruber N., Landschützer P., Lovenduski N. S. (2019). The variable Southern Ocean carbon sink. Annu. Rev. Mar. Sci. 11, 159–186. doi: 10.1146/annurev-marine-121916-063407
Hendry K. R., Brzezinski M. A. (2014). Using silicon isotopes to understand the role of the Southern Ocean in modern and ancient biogeochemistry and climate. Quaternary Sci. Rev. 89, 13–26. doi: 10.1016/j.quascirev.2014.01.019
Lamy F., Gersonde R., Winckler G., Esper O., Jaeschke A., Kuhn G., et al. (2014). Increased dust deposition in the Pacific Southern Ocean during glacial periods. Science 343, 403–407. doi: 10.1126/science.1245424
Landschützer P., Gruber N., Haumann F. A., Rödenbeck C., Bakker D. C. E., van Heuven S., et al. (2015). The reinvigoration of the Southern Ocean carbon sink. Science 349, 1221–1224. doi: 10.1126/science.aab2620
Martínez-García A., Sigman D. M., Ren H., Anderson R. F., Straub M., Hodell D. A., et al. (2014). Iron fertilization of the Subantarctic Ocean during the last ice age. Science 343, 1347–1350. doi: 10.1126/science.1246848
Rae J. W. B., Burke A., Robinson L. F., Adkins J. F., Chen T., Cole C., et al. (2018). CO2 storage and release in the deep Southern Ocean on millennial to centennial timescales. Nature 562, 569–573. doi: 10.1038/s41586-018-0614-0
Ragueneau O., Tréguer P., Leynaert A., Anderson R. F., Brzezinski M. A., DeMaster D. J., et al. (2000). A review of the Si cycle in the modern ocean: recent progress and missing gaps in the application of biogenic opal as a paleoproductivity proxy. Global Planetary Change 26, 317–365. doi: 10.1016/S0921-8181(00)00052-7
Sarmiento J. L., Gruber N., Brzezinski M. A., Dunne J. P. (2004). High-latitude controls of thermocline nutrients and low latitude biological productivity. Nature 427, 56–60. doi: 10.1038/nature02127
Sigman D. M., Fripiat F., Studer A. S., Kemeny P. C., Martínez-García A., Hain M. P., et al. (2021). The Southern Ocean during the ice ages: A review of the Antarctic surface isolation hypothesis, with comparison to the North Pacific. Quaternary Sci. Rev. 254, 106732. doi: 10.1016/j.quascirev.2020.106732
Sigman D. M., Hain M. P., Haug G. H. (2010). The polar ocean and glacial cycles in atmospheric CO2 concentration. Nature 466, 47–55. doi: 10.1038/nature09149
Tréguer P., Bowler C., Moriceau B., Dutkiewicz S., Gehlen M., Aumont O., et al. (2018). Influence of diatom diversity on the ocean biological carbon pump. Nat. Geosci. 11, 27–37. doi: 10.1038/s41561-017-0028-x
Keywords: ventilation, productivity, iron dynamics, overturning circulation, carbon cycle, sea ice, Antarctic icesheet
Citation: Xiong Z, Zhang X, Qi D, Zhao J and Algeo TJ (2024) Editorial: Role of the Southern Ocean in atmospheric pCO2 change: observations, simulations and paleorecords. Front. Mar. Sci. 11:1531887. doi: 10.3389/fmars.2024.1531887
Received: 21 November 2024; Accepted: 25 November 2024;
Published: 17 December 2024.
Edited and Reviewed by:
Eric ‘Pieter Achterberg, Helmholtz Association of German Research Centres (HZ), GermanyCopyright © 2024 Xiong, Zhang, Qi, Zhao and Algeo. This is an open-access article distributed under the terms of the Creative Commons Attribution License (CC BY). The use, distribution or reproduction in other forums is permitted, provided the original author(s) and the copyright owner(s) are credited and that the original publication in this journal is cited, in accordance with accepted academic practice. No use, distribution or reproduction is permitted which does not comply with these terms.
*Correspondence: Zhifang Xiong, emhmeGlvbmdAZmlvLm9yZy5jbg==
Disclaimer: All claims expressed in this article are solely those of the authors and do not necessarily represent those of their affiliated organizations, or those of the publisher, the editors and the reviewers. Any product that may be evaluated in this article or claim that may be made by its manufacturer is not guaranteed or endorsed by the publisher.
Research integrity at Frontiers
Learn more about the work of our research integrity team to safeguard the quality of each article we publish.