- 1Guangdong Research Center on Reproductive Control and Breeding Technology of Indigenous Valuable Fish Species, Guangdong Provincial Key Laboratory of Aquatic Animal Disease Control and Healthy culture, Fisheries College, Guangdong Ocean University, Zhanjiang, China
- 2Development and Research Center for Biological Marine Resources, Southern Marine Science and Engineering Guangdong Laboratory (Zhanjiang), Zhanjiang, China
Spotted scat (Scatophagus argus) is an economically important marine species in China, with high ornamental and edible value. The effects of dietary supplementation of Lianjiang red orange peels, a natural carotenoid source, at 0, 3, 6, 9 and 12%, hereafter referred to as G0, G3, G6, G9 and G12, were measured on color values, carotenoid content, expression of genes such as cyp2j6, cyp1a1, hsp70, slc2a11, bco2, cyp26c1 after 4 weeks. Subsequently, the different color values (L*, a*, and b*), total carotenoids content, and gene expression pattern were analyzed. In the groups fed diets containing more than 9% Lianjiang red orange peels (9% and 12%), the L* value (lightness) decreased, while the a* value (redness) increased in red skin, and b* value (yellowness) increased in caudal fin (P < 0.05). Additionally, the total carotenoids content in red skin of the G9 group and caudal fin of the G12 group was substantially higher than in the other groups (P < 0.05). By comparative transcriptome analysis of the red skin in G0 and G9 groups, 419 differentially expressed genes (DEGs) were identified, of which 237 were upregulated and 182 were downregulated. Genes involved in carotenoid deposition (cyp2j6, cyp1a1) and pigment cell development (hsp70, slc2a11) were significantly upregulated, whereas genes related to carotenoid decomposition (bco2) and retinoic acid metabolism (cyp26c1) were significantly downregulated in the G9 group. These findings suggest that Lianjiang red orange peels, as a natural carotenoid source, enhance body coloration by influencing the deposition of carotenoid, pteridine pigments, erythrophore development, and inhibiting the oxidative cleavage of carotenoid and retinoic acid metabolism. This study enhances body coloration by influencing carotenoid and pteridine pigment deposition, and provides theoretical guidance for aquaculture practices involving spotted scat.
1 Introduction
Animal body coloration is a key characteristic closely linked to functions such as camouflage, photoprotection, mimicry, and warning predators (Luo et al., 2021). The orange, red, and yellow body colors in some vertebrates, like fishes, are determined by the deposition of carotenoid pigments in feathers and skin, respectively (Toomey et al., 2017). Carotenoids, which are lipid-soluble pigments, serve as the main pigment group in aquatic organisms (Nakano and Wiegertjes, 2020). The chemical structure, dietary concentration of carotenoids, and the species itself could influence pigmentation (Pereira Da Costa and Campos Miranda-Filho, 2020). Nevertheless, aquatic animals are unable to synthesize carotenoids endogenously and must obtain these essential pigments through their diet (Torrissen, 1985). In aquaculture, supplementing fish diets with synthetic carotenoids, such as astaxanthin, lutein, and β-carotene, is a prevalent practice to enhance the body coloration of farmed fish (Besen et al., 2019; Micah et al., 2022; Yi et al., 2016). Furthermore, the high cost of synthetic carotenoids increases feed expenses, reducing the economic benefits of aquaculture. Therefore, finding low-cost alternatives to synthetic carotenoids and reducing reliance on these supplements is crucial. In nature, carotenoids are commonly found in the orange-red, yellow, or red pigments of some archaea, fungi, algae, plants, and animals (Huang et al., 2021a; Svensson and Wong, 2011). In recent, marigold (Tagetes erecta), bee pollen extract, and various algae, such as Haematococcus pluvialis, Chlorella vulgaris, and Chlorella zofingiensis, have been added to aquatic animal feeds as the natural carotenoid sources to sucessfully improve the body color of aquatic animals (Jorjani et al., 2019; Torres Sánchez et al., 2020; Elbahnaswy and Elshopakey, 2024). Therefore, using natural carotenoid sources as dietary supplements to enhance the coloration of farmed fish offers both economical and practical advantages.
Annually, the global food industry generates substantial amounts of waste and by-products from various sources. These by-products are rich in nutritional, functional, and bioactive compounds, which can be incorporated into the diet of farmed animals to reduce waste and enhance the overall sustainability of the food system (Ababor et al., 2023; Rațu et al., 2023). Several studies have demonstrated that adding agricultural by-products into feed or replacing some feedstuffs could improve the body coloration in fish. For instance, the addition of pineapple (Ananas comosus (L.) Merr.) waste in the diet of Nile tilapia (Oreochromis niloticus) has been found to increase the redness of the flesh (Anis Mohamad Sukri et al., 2022). In carp (Cyprinus carpio), the addition of 5% carrot (Daucus carota var. sativa Hoffm.) powder to feed has been shown to act as a color enhancer (Jain et al., 2019). Similarly, in jewel cichlid (Hemichromis guttatus), supplementing the diet with 3% red pepper (Capsicum annuum) peel can also enhances body color (Yigit et al., 2021). Citrus is one of the most important economic crops and is widely distributed around the world. Citrus peel is rich in nutrients and bioactive compounds, such as vitamin C, minerals, and is an important natural carotenoids source (Kim et al., 2021; Rafiq et al., 2016). Studies have shown that adding 600 g/kg of citrus (Citrus reticulate) peels to the diet can significantly increase the total carotenoid content of gold crucian carp (Carassius auratus) skin and improve body color (Abbas et al., 2020). In gilthead sea bream (Sparus aurata) larvae, sweet orange (Citrus sinensis (L.) Osbeck) peel can improve the antioxidant activity (Salem et al., 2019). Lianjiang red orange, a renowned variety of orange, is an important economic agricultural product cultivated in Zhanjiang City, Guangdong Province, China. In this study, Lianjiang red orange peels were selected as a natural pigment source and added to the diets to enhancing the body coloration of farmed economic ornamental fish.
Transcriptome technology has been widely used to study gene expression patterns and mechanisms of fish body coloration. In red tilapia (Oreochromis spp.), transcriptome sequencing analyses have been performed to identify key genes involved in the four typical body color phenotypes, including those associated with melanin synthesis, transport and deposition of carotenoids, lipid metabolism, and xanthophore synthesis (Fang et al., 2022). Similarly, in Taiwan loach (Paramisgurnus dabryanus ssp. Taiwan), transcriptome sequencing analysis was conducted to explore the difference in skin phenotypes between wild-type and mutant individuals (golden red albinism), revealing traits such as golden pink eyes and golden red body (Huang et al., 2021c). Dietary supplementation with synthetic astaxanthin significantly enhanced the body coloration of blood parrot (Vieja melanurus♀×Amphilophus citrinellus♂). Transcriptome analysis further identified genes related to astaxanthin absorption and transport, such as lipoprotein lipase (lpl), phospholipid transfer protein (pltp), and StAR related lipid transfer domain containing 7 (stard7). Additionally, genes involved in retinoid metabolism, including alcohol dehydrogenase 1 (adh1), cytochrome P450 family 26 subfamily B member 1 (cyp26b1), cytochrome P450 family 27 subfamily C member 1 (cyp27c1), and retinol dehydrogenase 12, like (rdh12l), as well as genes linked to lipid metabolism and carotenoid deposition, such as apolipoprotein A1 (apoa1b) and apolipoprotein B (apodb), were also identified by transcriptome sequencing analysis (Micah et al., 2022). In spotted scat (Scatophagus argus), erythrophores, which are responsible for red coloration, appear in embryos before hatching. Transcriptome sequencing analysis has identified the expression profile of several key genes involved in carotenoid metabolism, including scavenger receptor class B member 1 (scarb1), perilipin 6 (plin6), perilipin 2 (plin2), apolipoprotein A (apoda), beta-carotene oxygenase 1 (bco1), and retinoid isomerohydrolase family 65 (rep65a). Additionally, genes associated with chromatophore differentiation, such as solute carrier family 2 member 15b (slc2a15b) and colony stimulating factor 1 receptor, a (csf1ra), were also identified (Liao et al., 2023). However, the detailed mechanisms of body red coloration require further elucidation.
Spotted scat is widely distributed across the Indo-Pacific waters, including the coasts of Guangxi, Taiwan, and Guangdong in China. It is recognized as an economically important aquaculture species in the southeastern coastal regions of China, valued for its high nutritional content, palatable flavor, strong adaptability, notable resistance to diseases and environmental stressors, vibrant coloration, low feeding costs, and high market value (Zhang et al., 2018; Huang et al., 2021b; Liao et al., 2023). However, under artificial farming conditions, black spots are not concentrated in spotted scat skins, and the red spots become pale, significantly diminishing the ornamental value. This study investigated the effect of different dosages of Lianjiang red orange peels on enhancing red coloration in spotted scat. The colorimetric values and total carotenoids in different skin regions and fins were measured, while also analyzing the expression of key genes regulating the body coloration using transcriptome sequencing and real-time fluorescence quantitative PCR (qRT-PCR). The findings in this study could help to understand the molecular mechanism of body red coloration in fish and offer guidance for improving and optimizing feed formulation in spotted scat artificial farming.
2 Materials and methods
2.1 Preparation of Lianjiang red orange peel
Lianjiang red oranges were purchased from Haida Basket (Zhanjiang, China), and washed with running water. The orange peels were collected, vacuum freeze-dried, and then crushed into powder. The powder was filled into the plastic sealing bags and stored at -20°C for further use. The primary nutrient composition of the Lianjiang red orange peels (% of dry matter) and carotenoid levels (mg kg-1) were analyzed, with the results presented in Table 1.
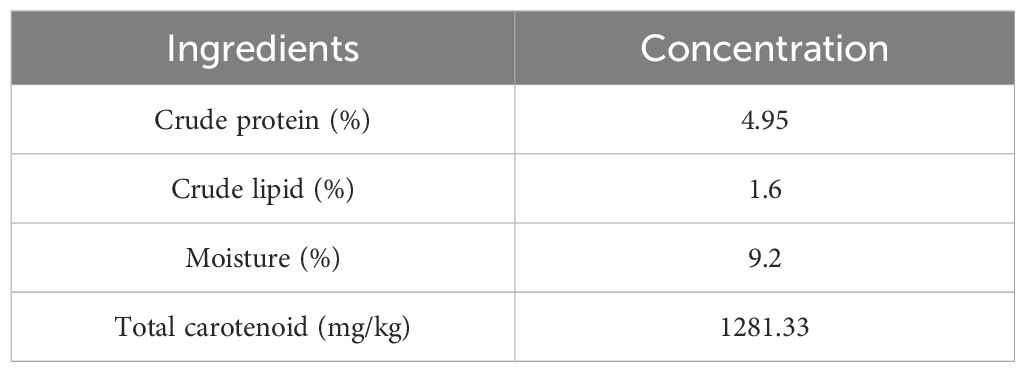
Table 1. Primary nutrient composition of the Lianjiang red orange peels (% of dry matter) and carotenoid levels (mg kg-1).
2.2 Preparation of the experimental diets containing different dosages of Lianjiang red orange peels and primary nutrition composition measurement
In this study, fishmeal, wheatmeal, and soya bean meal were employed as the primary protein sources in the experimental diets, with fish oil as the lipid source, and wheat meal as the carbohydrate source. Lianjiang red orange peels were then added to the diets, with the different dosages of 0, 3, 6, 9, and 12%. The ingredients and compositions of these experimental diets are detailed in Table 2. Each raw material was crushed through a 60-mesh sieve by a grinder and mixed using a step-by-step expansion method. Soybean lecithin and fish oil were then added sequentially. After screening, an appropriate amount of water was added and the mixture was thoroughly blended again. Subsequently, the diets were processed into pellets with a diameter of 1.50 mm by a screw extruder, air-dried, and stored in a refrigerator at -20°C.
Then, 200 g experimental diets were oven-dried at 105°C until a constant weight was reached, after which the moisture content was measured. Crude protein content was analyzed by kjeldahl method (Haineng K1100), crude fat content was determined by soxhlet extraction method (Fiber SZC101), and ash content in feed was measured by muffle furnace at 550°C (KSW-12-12) (Wang et al., 2020).
2.3 Experimental animals and design
Spotted scat, used in this study, was reared at Donghai Island Cultivation Base, the Marine Biological Base of Guangdong Ocean University (Zhanjiang, Guangdong, China). To prevent stress, all fish underwent a seven-day acclimatization period, during which they were fed commercial pellets (Guangdong Yuequn Ocean Biotechnology Co. Ltd., Jieyang, China), containing at least 48% crude protein and 5% fat. The experiment was approved by the Institutional Animal Care and Use Committee (IA-CUS) of Guangdong Ocean University and conducted in accordance with the laws of China and the regulations on biological research. Prior to sampling, all fish were anesthetized with eugenol (1:10,000) (Shanghai Reagent Corp., China).
A total of 450 spotted scat (average body weight 6.12 ± 0.26 g) were randomly divided into five groups. The fish were reared in 60 L oblong tank, with three tanks per group, serving as the biological replicates, and 30 fish per tank. The groups, labeled Con (control), G3, G6, G9, and G12, were fed diets containing 0%, 3%, 6%, 9%, and 12% Lianjiang red orange peels for 4 weeks, respectively. All fish were fed with the experimental diets twice daily at 09:00 and 17:00. One hour after feeding, the residual feed and feces were siphoned out. The temperature of the seawater was maintained at 32 ± 1°C, with a pH range of 7.5−8.0, the ammonia nitrogen levels were kept between 0.4 and 0.6 mg/L, and the dissolved oxygen levels were maintained at 6.4 ± 0.2 mg/L. The water was changed twice daily.
Nine fish were randomly selected for sampling the red skin, dorsal fin, and caudal fin of spotted scat from each group. The body weight and colorimetric values of six different body regions (Figure 1A) were measured for each fish. The sampled tissues of spotted scat were immediately frozen in liquid nitrogen and subsequently transferred to -80℃ for RNA extraction, sequencing, and measurement of total carotenoids.
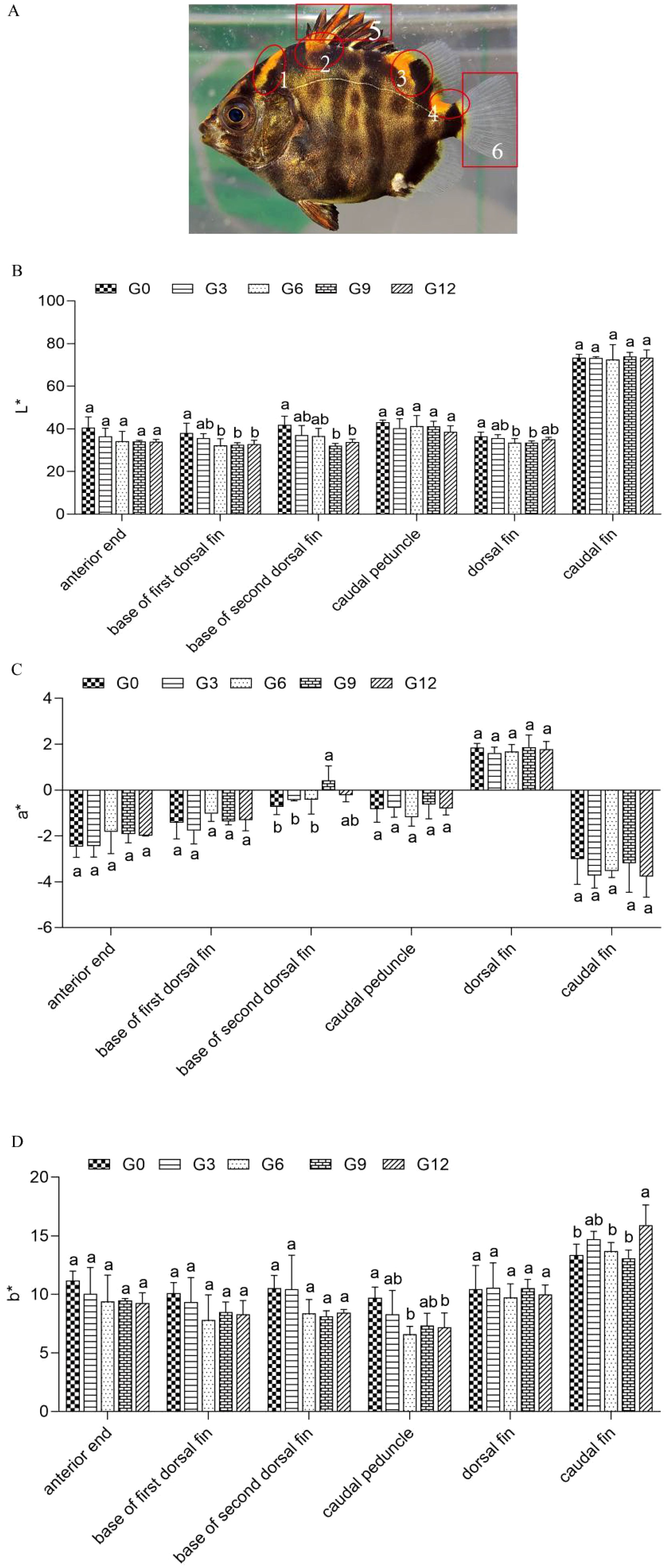
Figure 1. L*, a* and b* colorimetric values in spotted scat fed with diets containing different dosages of Lianjiang red orange peels. (A) Sample of skin region for measuring L*, a* and b* colorimetric values in spotted scat. Numbers 1, 2, 3, 4, 5, and 6 represent anterior end, base of the first dorsal fin, the joint of the first and second dorsal fin, the joint of second dorsal and caudal fin, dorsal fin and caudal fin, respectively. The comparative analysis (B) L*, (C) a* and (D) b* value in spotted scat. Date were expressed as mean ± SE (n=3), the different superscript letters above error bar represent the significant difference between different groups (P < 0.05).
2.4 Colorimetric value measurements
The colorimetric values of four different red skin regions, dorsal and caudal fins were measured using a Konica Minolta CR-400 (Minolta Camera Co. Ltd., Asaka, Japan). Before measurement, each fish was swabbed dry and the white board of the colorimeter was calibrated. For each region, measurements were taken twice. The probe was rotated 180° between the first and second measurements to ensure accuracy, and the colorimetric value was recorded as follows: a: red, -a: green; b: yellow, -b: blue; L: lightness, 100 L: white, 0 L: black.
2.5 Measurement of total carotenoids in different skin regions, fins and experimental diets
The dried Lianjiang red orange peels (purchased from Haida Basket and vacuum freeze-dried into dried orange peels) and experimental diets were crushed into powder using a grinder, while the skin tissues of spotted scat were crushed in liquid nitrogen. A total of 0.1g of peel, skin tissues (with 0.01g of anhydrous sodium sulfate), and experimental diets were placed in three different 2 mL centrifuge tubes. Three biological replicates were prepared for peels, skin tissues (with three fish tissue samples per replicate), and experiment diets. Total carotenoids of Lianjiang red orange peels and experiment diets were measured before the feeding experiment in this study. Then, 2 mL of pigment extract solution (n-hexane: acetone: ethanol absolute = 2: 1: 1, containing 0.01% 2,6-Di-tert-butyl-4-methylphenol) was added into each centrifuge tube. The mixture was homogenized for 10 min and then transferred to a new 15 mL centrifuge tube. The solution was adjusted to 10 mL and placed in a refrigerator at 4 ℃ for 24 h. The next day, the solution was centrifuged at 4 ℃, 6000 r/min for 10 min. The upper layer of pigment-containing solution was collected and transferred to a new 15 mL centrifuge tube. The supernatant was washed three times with saturated NaCl solution until neutral. Finally, the absorbance value of the pigment extracts was measured using a UV spectrophotometer at the maximum absorption peak to calculate the total content of carotenoids. The calculation formula was depicted as follows:
where C is the carotenoid content (mg/kg), A is the absorbance value, K is a constant (104), W is the volume of extract (mL), E is the molar extinction coefficient (2500), and M is the weight of the sample (g).
2.6 Total RNA extraction, library construction, and sequencing
Total RNA was extracted from the red skin of spotted scat using Trizol reagent (Life Technologies, Carlsbad, CA, USA) according to the manufacturer’s instructions. Six individuals were sampled in each group, and all samples of four red skin regions (Figure 1A) from two spotted scat were pooled per tube, with three tubes per group, encompassing both the G9 feeding and control groups. The quality of the RNA samples was evaluated using a NanoDrop 2000 Spectrophotometer (Thermo Fisher Scientific, Santa Clara, CA, USA) and 1% agarose gel electrophoresis. Only total RNA samples with an RNA integrity number (RIN) score of seven or above were chosen for sequencing.
Six sequencing libraries were constructed using the NEBNext Ultra RNA Library Prep Kit for Illumina® (NEB, Ipswich, MA, USA) in accordance with the manufacturer’s instructions. The Ribo-Zero™ magnetic kit (Epicentre, Madison, WI, USA) was employed to remove ribosomal RNA from the total RNA, while the Oligo (dT) magnetic bead (Illumina, San Diego, CA, USA) enrichment method was utilized to enrich eukaryotic mRNA. The fragmentation buffer was then used to fragment the enriched mRNA into short fragments, and the mRNA was reverse transcribed into cDNA using the NEBNext Ultra RNA Library Prep Kit for Illumina sequencing (NEB, New England Biolabs, Ipswich, MA, USA). After performing end-repair and A base insertion, the purified double-stranded cDNA fragments were ligated to Illumina sequencing adapters. With agarose gel electrophoresis, the 200 bp ligation products were chosen. The fragments were amplified, purified, and then used for sequencing by Gene Denovo Biotechnology Co. (Guangzhou, China) using the Illumina NovaSeq 6000 platform to generate 150-bp paired-end reads.
2.7 Transcriptome assembly, gene function annotation and differential expression analysis
To obtain high-quality clean reads, raw reads were filtered and stored using Fastp (version 0.18.0) (Fan et al., 2023). The following parameters were applied: first, reads containing adapters were removed; second, reads with more than 10% unknown nucleotides (N) were discarded; and third, low-quality reads with over 50% low-quality (Q-value ≤ 20) bases were eliminated. We examined the clean data’s Q20, Q30, GC content, and sequence duplication levels. All downstream analyses were performed with clean, high-quality data. Next, using HISAT2 tools soft (Version 2.2.4), clean reads from each library were mapped to the spotted scat reference genome sequence (https://bigd.big.ac.cn/gwh; accession number GWHAOSK00000000.1). All sequencing data from clean libraries were submitted to the National Center for Biotechnology Information (NCBI) Sequence Read Archive (SRA) with a Bioproject number: PRJNA1121255.
Gene functions were annotated using the NCBI non-redundant protein database (NR; ftp://ftp.ncbi.nih.gov/blast/db/, accessed on 25 April 2024) through the BLASTx program (version 2.2.26) (https://blast.ncbi.nlm.nih.gov/) with an E-value threshold of 1 × 10−5, Swiss-Prot (http://www.uniprot.org/, accessed on 25 April 2024), Kyoto Encyclopedia of Genes and Genomes (KEGG; http://www.genome.jp/kegg/, accessed on 25 April 2024), and Gene Ontology (GO; http://www.geneontology.org/, accessed on 25 April 2024) databases using BLASTx (v. 2.2.26; https://blast.ncbi.nlm.nih.gov/, accessed on 25 April 2024) with an E-value threshold of 1 × 10−5.
The String Tie software (version 2.2.0) was used to calculate the FPKM value. The R program DESeq2 (1.16.1) was utilized to determine the difference in genes between the G9 and control groups. DESeq2 uses a model based on the negative binomial distribution to provide statistical methods for identifying differential expression in digital gene expression data. The DEG threshold for significance was set to P < 0.05 and |log2FoldChange| ≥ 1. After performing GO and KEGG pathway enrichment analysis on DEGs, a statistically significant result was defined as P < 0.05.
2.8 Validation of gene expression by real-time PCR analysis (qRT-PCR)
For qRT-PCR analysis, eight DEGs (P < 0.05 and |log2FC| ≥ 1) involved in carotenoid metabolism and deposition, including cytochrome P450, family 2, subfamily j, polypeptide 6 (cyp2j6), cytochrome P450 family 1 subfamily A member 1 (cyp1a1), pigment cells development (heat shock protein 70 (hsp70), solute carrier family 2 member 11 (slc2a11)), carotenoids decomposition (9’,10’-β-carotene oxygenase (bco2)), retinoic acid metabolism (cytochrome P450 family 26 subfamily C member 1 (cyp26c1), dehydrogenase/reductase 13 (dhrs13)), and randomly selected gene (myelocytomatosis oncogene (myc)), were chosen to confirm the accuracy of the transcriptome data. In addition, the expression of four key genes, cyp1a1, slc2a11, bco2, and cyp26c1, was detected by qRT-PCR to further evaluate the effect of feeding Lianjiang red orange peels at different dosages on improving coloration. Primer 6.0 software was used to design primers. The primers used in this study are shown in Table 3. Reverse transcription was carried out to synthesize cDNA using TransScript® Uni All-in-One First-Strand cDNA Synthesis Super Mix (TransGen, Beijing, China). qRT-PCR was performed using PerfectStart® Green qRT-PCR SuperMix (TransGenTrans, Beijing, China) on the Roche LightCycler® 96 System (Roche Diagnostics, Basel, Switzerland). The qRT-PCR program consists of an initial denaturation at 94°C for 30 s, succeeded by 40 cycles of 94°C for 5 s, 59°C for 15 s, and 72°C for 10 s. Additionally, β-actin was used as a reference gene to normalize the expression values. Each group consisted of three biological replicates, and each sample in the qRT-PCR reaction was analyzed with three technical replicates. The relative gene expression levels were determined using the 2−ΔΔCt method. The mean values (m-values) of 2−ΔΔCt for each sample were calculated by averaging the three technical replicates. Subsequently, the mean for the m-values for gene expression levels of the three samples in G0 group was calculated to normalize the fold changes (FC). The FC values or each sample in the G9 group were then computed using the formula: FC = m-values of 2−ΔΔCt (for the G9 group)/Means of m-values (for the G0 groups), and then the Log2(FC) values were calculated. The data expressed as means ± standard error (SE) (n = 3). The graphical representation of the data was generated using GraphPad Prism 9.0 software (GraphPad Software Inc., San Diego, CA, USA).
2.9 Data calculation and analysis
The total carotenoids of fish skin are expressed as means ± standard error (SE) (n = 3). The data were analyzed by one-way ANOVA and Duncan’s Multiple Range test. Statistical analysis was performed using SPSS Version 25 at a significance level of 0.05.
3 Results
3.1 Colorimetric value evaluation
After being fed diets containing Lianjiang red orange peels for four weeks, the body color of spotted scat was photographed (Figure 2). Measurements indicated that the L* value of skins at the base of the first dorsal fin and at the joint of the first and second dorsal fins gradually decreased with increasing dosages of orange peel in the diets, showing significant differences among control and G6, G9, G12 group (P < 0.05) (Figure 1B). The a* value of the erythema at the joint of the first and second dorsal fin in the G9 group was significantly higher than that of the control group (P < 0.05), while no any statistical differences were observed in other groups or in other body regions among different groups (P > 0.05) (Figure 1C). In the G12 group, the b* value of the caudal fin was significantly higher (P < 0.05), while the b* value at the joint of second dorsal and caudal fin was significantly lower (P < 0.05), compared to the control group (Figure 1D).
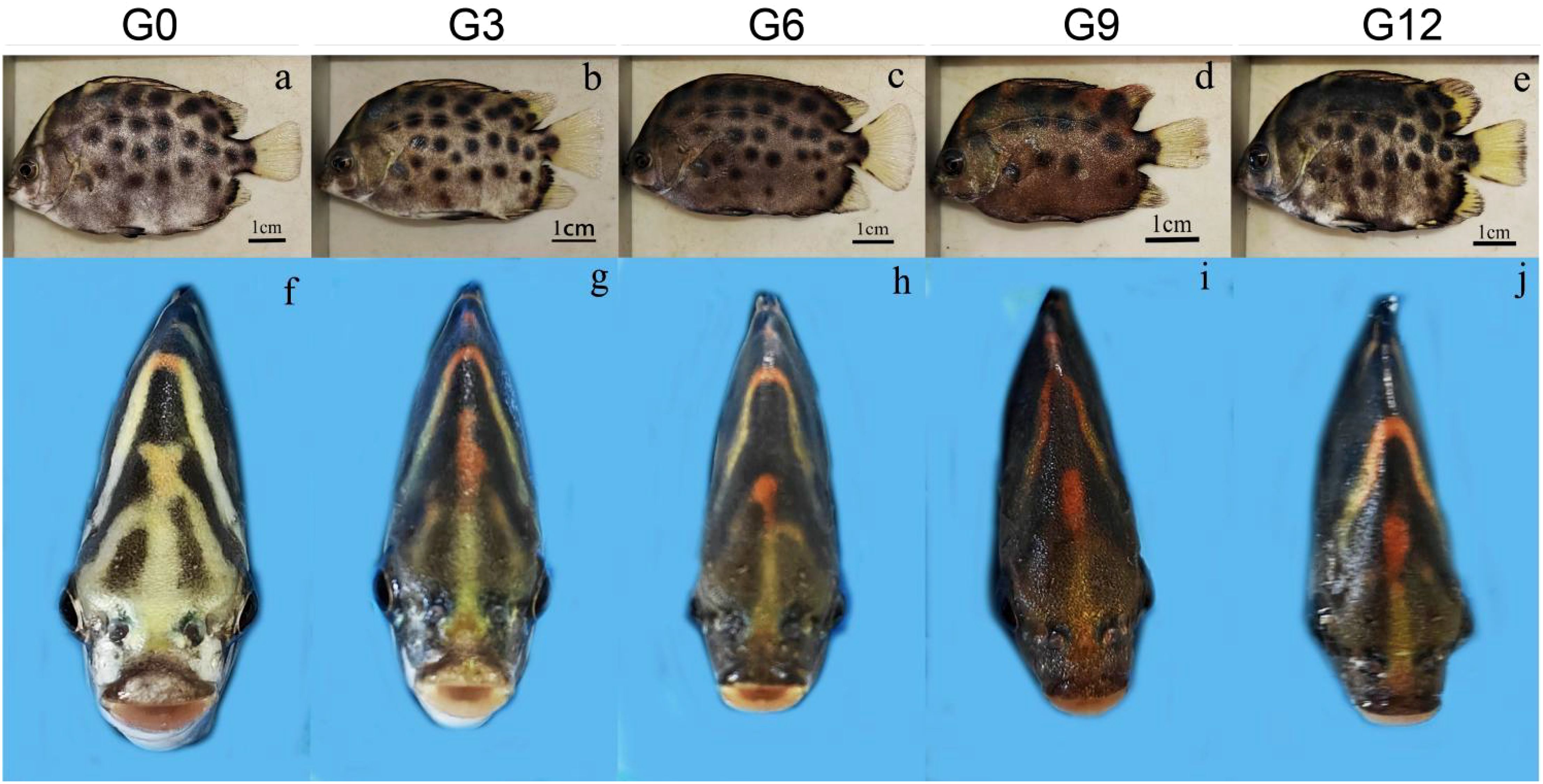
Figure 2. Body color of spotted scat fed with diets containing different dosages of Lianjiang red orange peels. The side (A–E) and frontal (F–J) views of the red body regions in spotted scat. The background of the (F–J) diagram of the front view is filled with blue using Photoshop CC 2019 (version 20.x).
3.2 Total carotenoid content
No significant differences were observed in the total carotenoid content of the dorsal fin among all groups (P > 0.05). However, the total carotenoid content in the red spot skin (RS) of spotted scat significantly increased when the dosage of Lianjiang red orange peels reached 9%, compared to other groups, except for the G12 group. In the caudal fin, the total carotenoid content in both the G9 and G12 group were significantly higher than that in control group (P < 0.05) (Figure 3). Compared to the control and other dosage Lianjiang red orange peels fedding groups, the significant changes of colorimetric values in the red skin region and total carotenoid content of spotted scat from G9 group were observed, indicating a more pronounced enhancement of skin coloration in the G9 group.
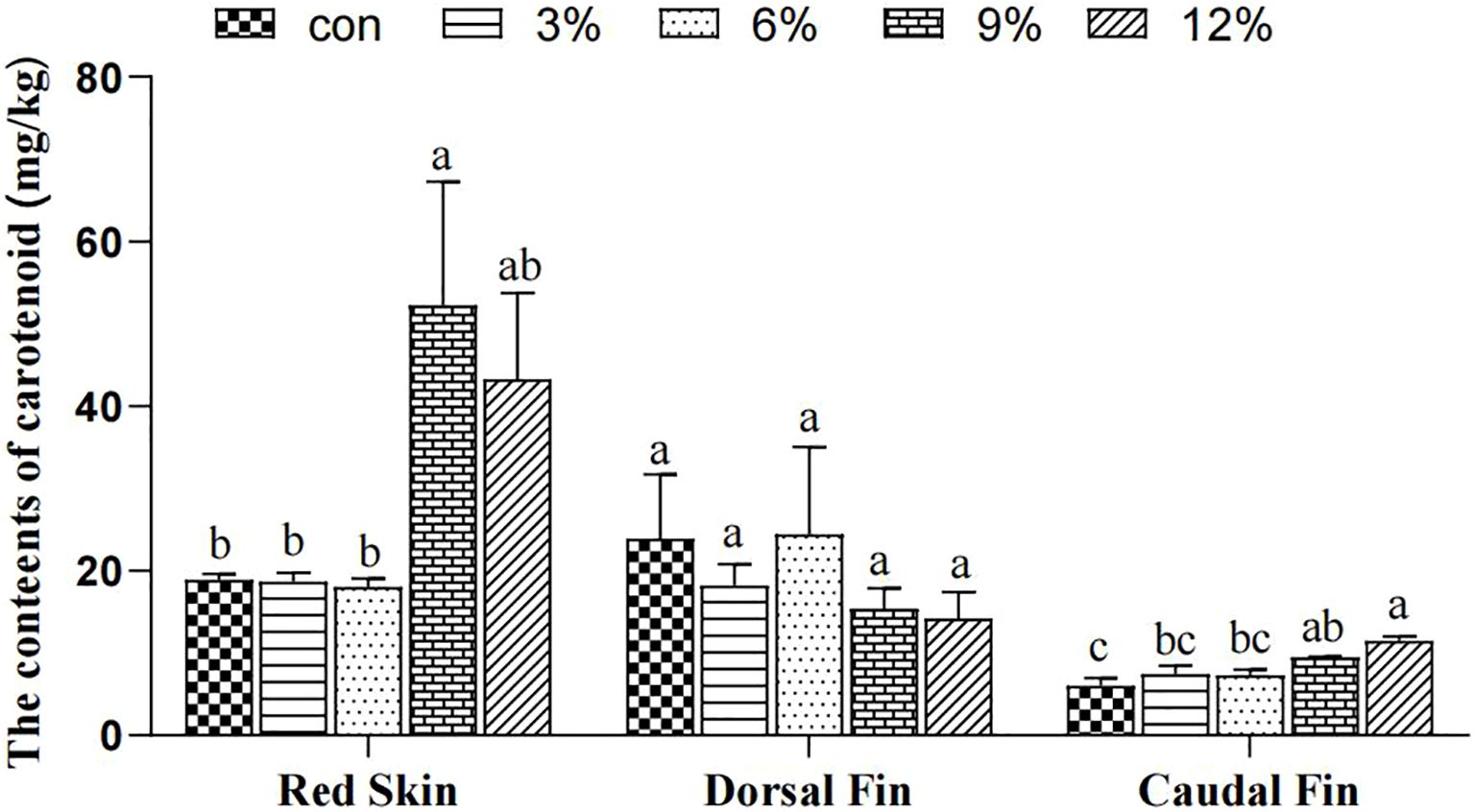
Figure 3. Total carotenoids content in different skin regions and fins of spotted scat. Date were expressed as mean ± SE (n=3), the different superscript letters above error bar represent the significant difference between different groups (P < 0.05).
3.3 Illumina sequencing and gene annotation, functional enrichment analyses of DEGs
Based on the body coloration enhancement in G9 group, transcriptome sequencing of the red skin from the G9 and control groups was carried out to assess the molecular mechanism of dietary Lianjiang red orange peels on coloration enhancement. After quality control of sequencing data for six cDNA libraries, constructed using red skin samples from spotted scat in both the control and G9 group, a total of 34,185,768,758 clean reads were obtained. The Q20 and Q30 values exceeded 98% and 94%, respectively. The GC content was above 48% (Table 4), indicating high-quality sequencing data suitable for further analysis.
The principal component analysis showed that the control and G9 groups clustered together, while different treatments were clearly separated. The first and second principal components explained 80% and 11% of the total variance, respectively (Figure 4A). Transcriptome analysis revealed a total of 419 DEGs between the G9-RS and the control group, using the criterion |log2FC| ≥ 1 and P < 0.05. Of these, 237 DEGs were upregulated genes, and 182 DEGs were downregulated genes (Figure 4B).
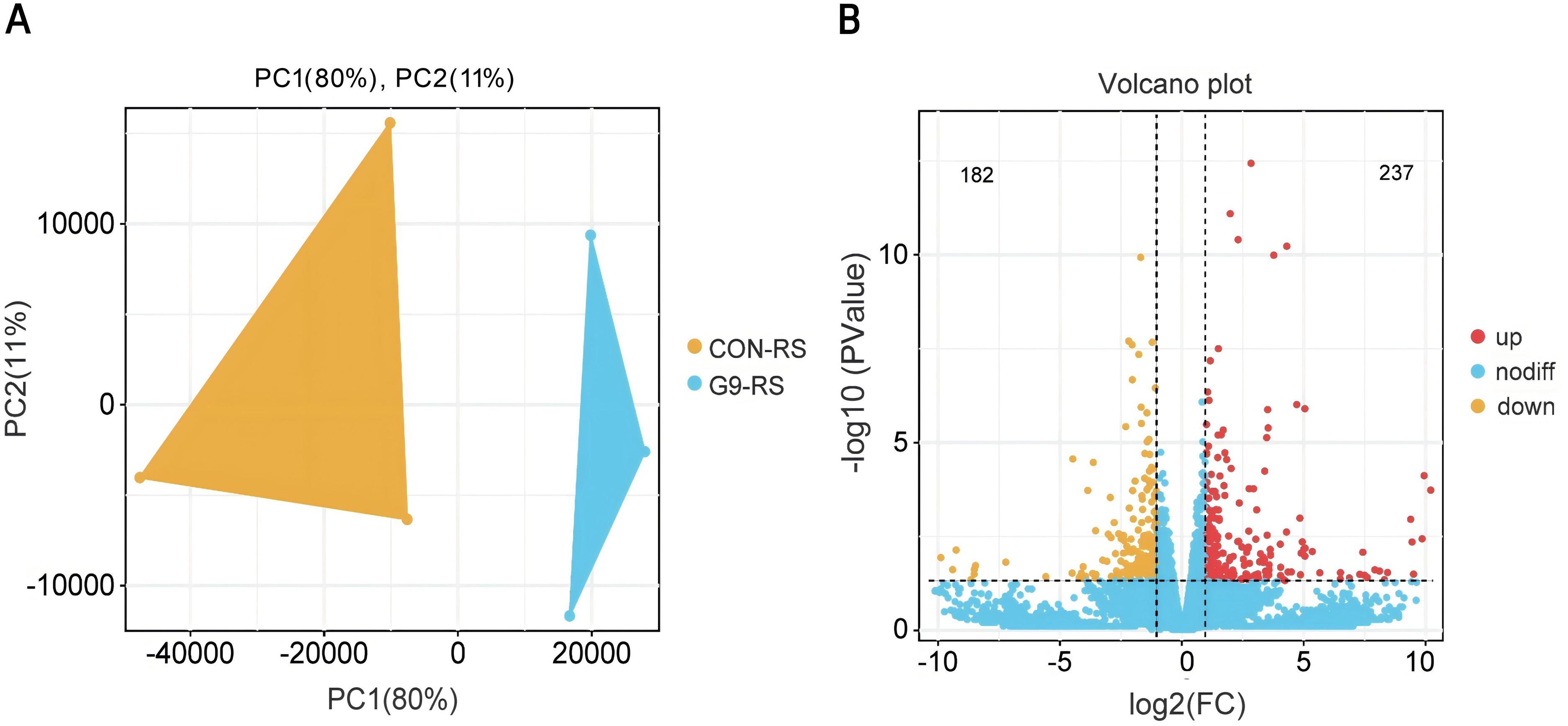
Figure 4. Principal component analysis and volcano plot of DEGs in red spots skin of spotted scat. (A) PCA, (B) Volcano plot. The up- and down-regulated DEGs are represent by red and orange dots, respectively. non-differentially expressed genes.
GO annotation analysis showed that DEGs were enriched in 51 GO classifications, including 24 related to Biological Process (BP), 9 to Molecular Function (MF), and 18 to Cellular Component (CC). In BP, DEGs were associated with processes such as single-organism process (GO: 0044699), cellular process (GO: 0009987), biological regulation (GO: 0065007), metabolic process (GO: 0008152), and other processes. Notably, the retinoid metabolic process (GO: 0001523), carotene metabolic process (GO: 0016119), and response to low-density lipoprotein particle (GO: 0055098) were significantly enriched and correlated with carotenoid metabolism and absorption. In MF, DEGs were enriched in binding (GO: 0005488), catalytic activity (GO: 0003824), molecular transducer activity (GO: 0060089), transporter activity (GO: 0005215), and nucleic acid binding transcription factor activity (GO: 0001071). In CC, DEGs were enriched in cell part (GO: 0044464), cell (GO: 0005623), membrane (GO: 0016020), organelle (GO: 0043226), and membrane part (GO: 0044425) (Figure 5).
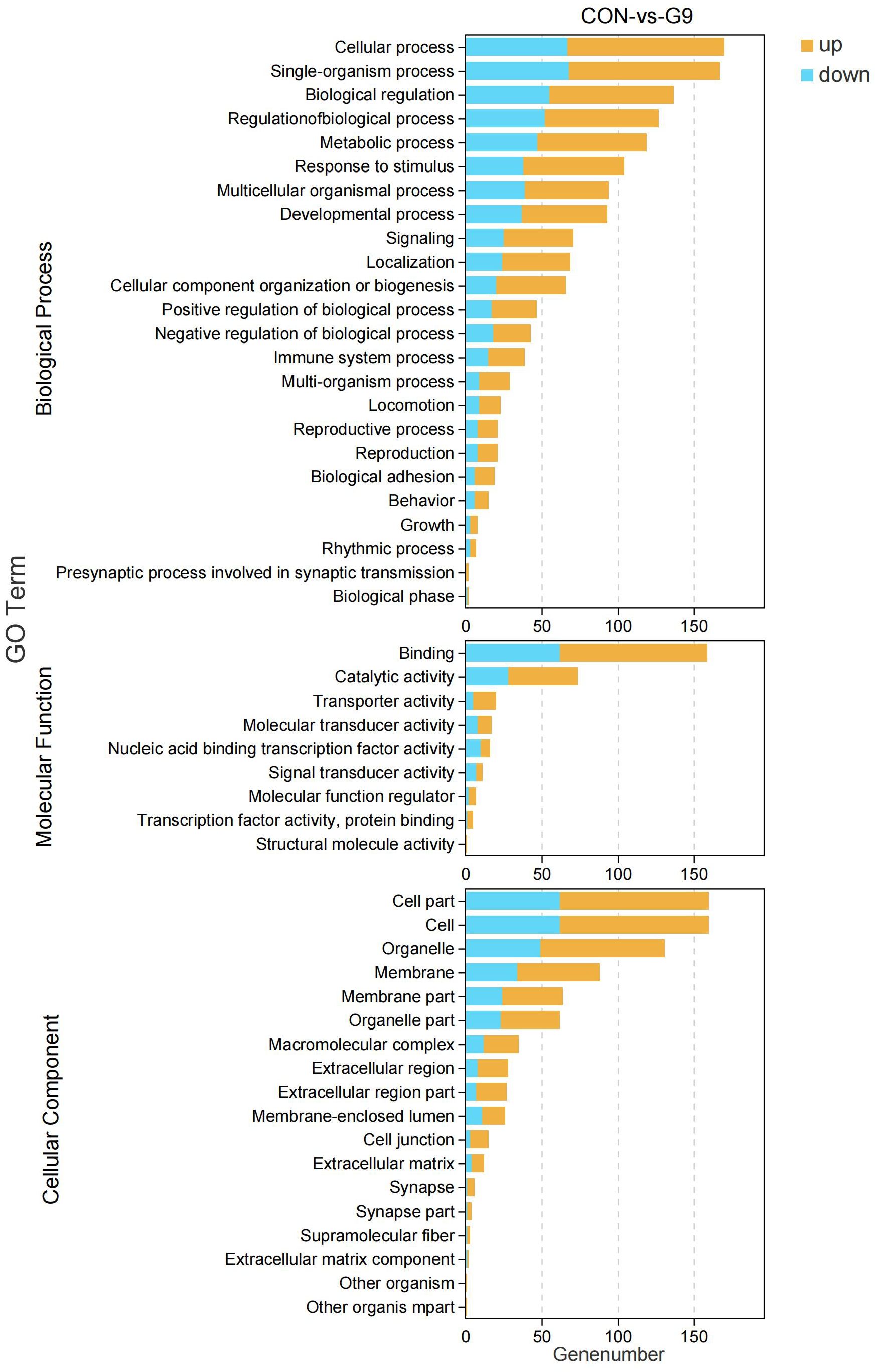
Figure 5. GO enrichment analysis of DEGs in red skins of G9 vs. Con group. Orange and blue column represent the up-regulated and down-regulated DEGs enriched in GO terms, respectively.
KEGG enrichment analysis revealed that DEGs were mainly enriched in pathways related to antigen processing and presentation, estrogen signaling, IL-17 signaling, viral protein interaction with cytokine and cytokine receptor, and bladder cancer, etc. Notably, pathways associated with fat digestion and absorption, MAPK signaling, and ether lipid metabolism were found to be related to carotenoid metabolism (Figure 6).
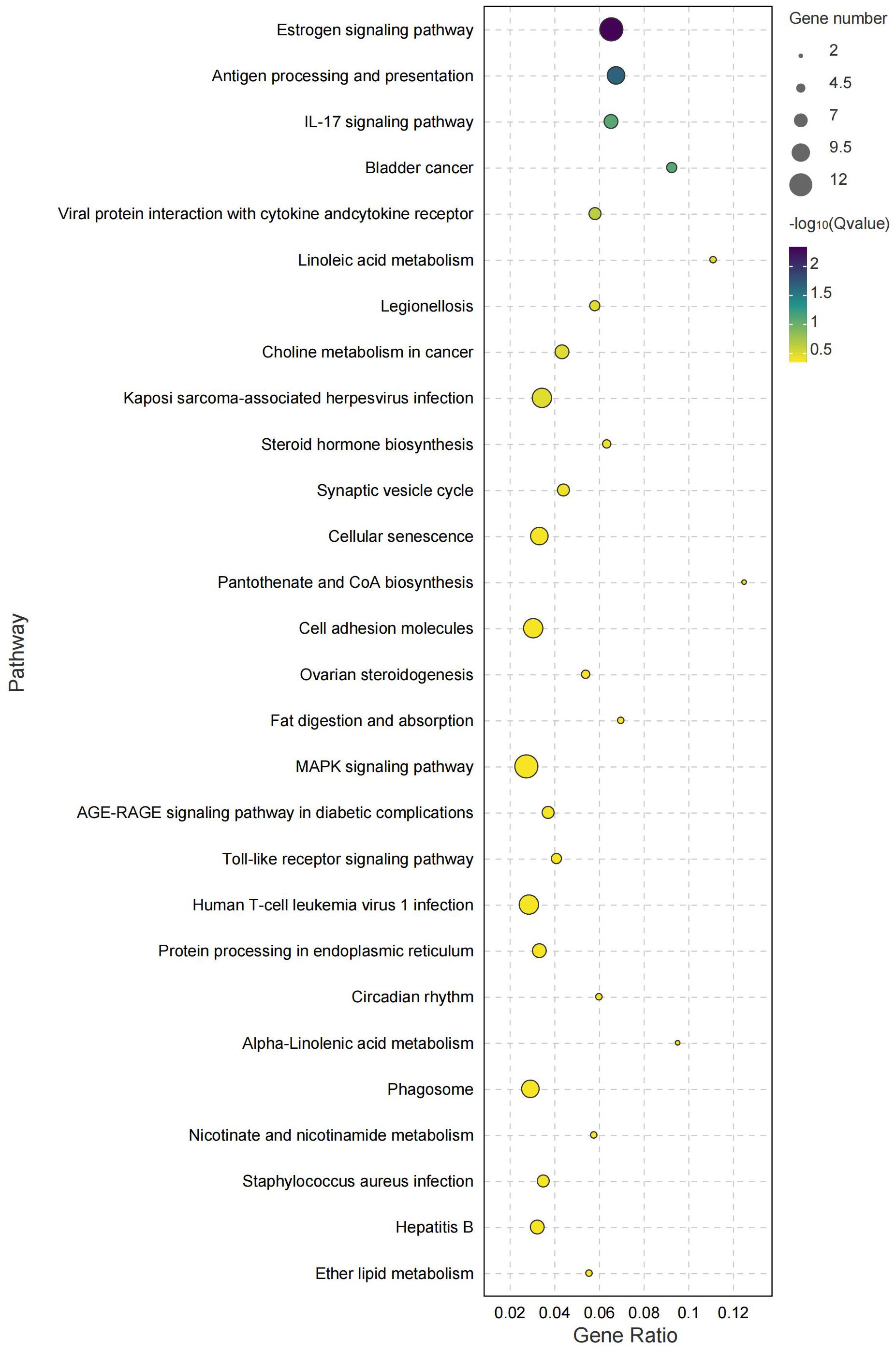
Figure 6. KEGG pathway enrichment analysis of DEGs in orange-red skins of G9 vs. Con group. The circles represent numbers of DEGs enriched in each pathway. Different colors represent the value of log10 (Q-value).
3.4 Validation of RNA-Seq data using qRT-PCR
To verify the accuracy of the transcriptome data, eight DEGs, including dhrs13, hsp70, cyp1a1, slc2a11, cyp2j6, bco2, cyp26c1, and myc, were selected for validation through qRT-PCR. These genes are involved in carotenoid metabolism, deposition, pigment cell development, retinoic acid metabolism, and red coloration. The expression patterns of the above DEGs were consistent with the RNA-Seq results, indicating the reliability and accuracy of transcriptome data (Figure 7).
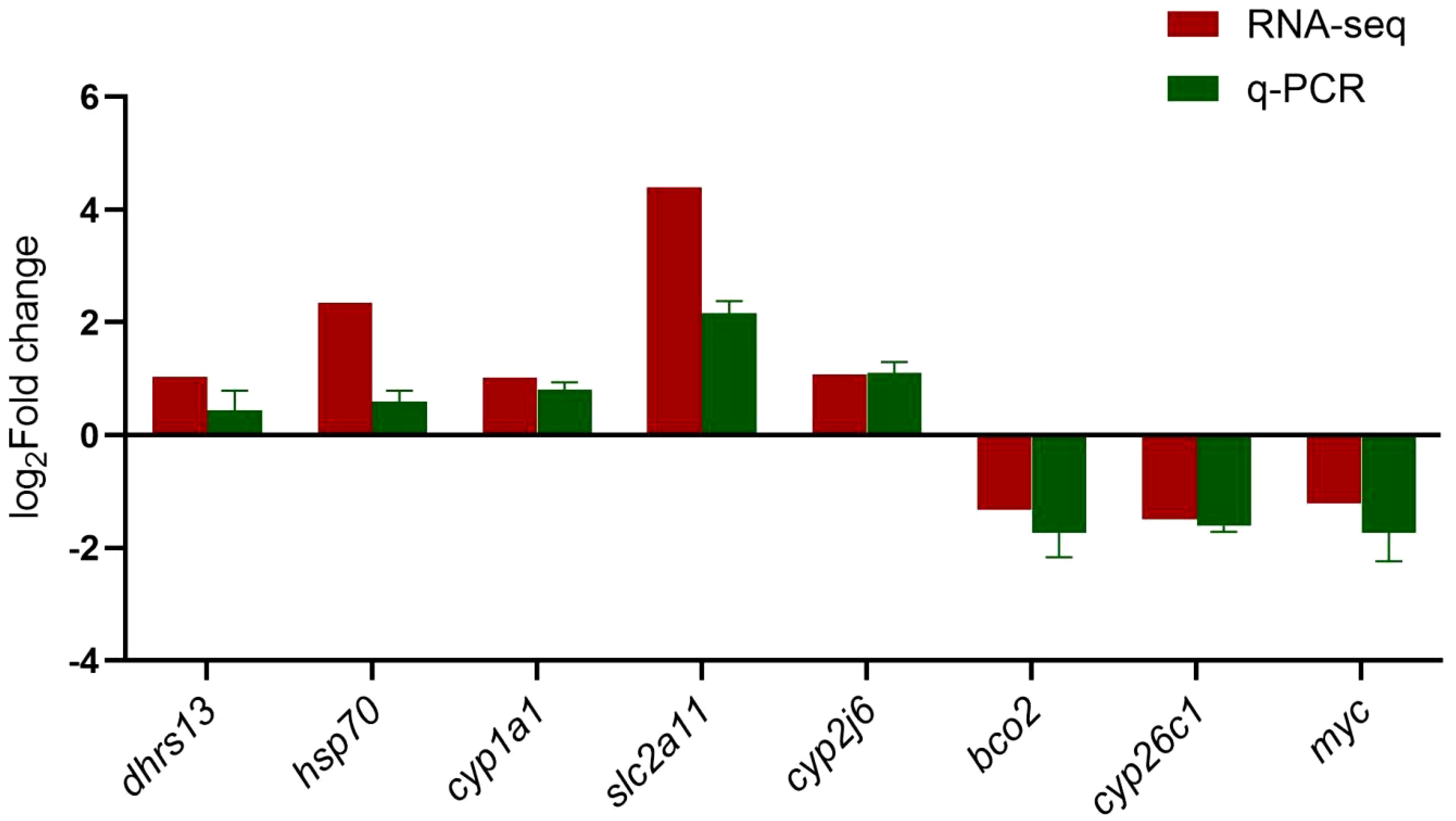
Figure 7. Validation of genes expression pattern of DEGs by qRT-PCR. The relative expression level of messenger RNA (mRNA) transcripts was detected using qRT-PCR, and calculated using the 2−ΔΔCt method. Data are expressed as means ± SE (n = 3). β-actin was used as the reference gene to normalized genes expression. The red and green columns represent the Log2FC (Fold changes) values of DEGs expression in G9 vs. Con group, analyzed using RNA-seq and qRT-PCR, respectively.
The expression of genes involved in carotenoid and retinoic acid metabolisms was analyzed using qRT-PCR. The mRNA expression of bco2 was significantly lower in all groups treated with different dosages of Lianjiang red orange peels compared to the control group (P < 0.05), with no significant difference observed among the above treatment groups. Similarly, the mRNA expression of cyp26c1 was also significantly lower in the treatment groups than in the control group, showing a dosage-dependent manner. In contrast, the mRNA expression of slc2a11 in the G9 group was significantly higher than that in the other groups. Additionally, the mRNA expression of cyp1a1 was significantly higher in the G3 and G9 groups than in control, G6, and G12 groups (P < 0.05) (Figure 8).
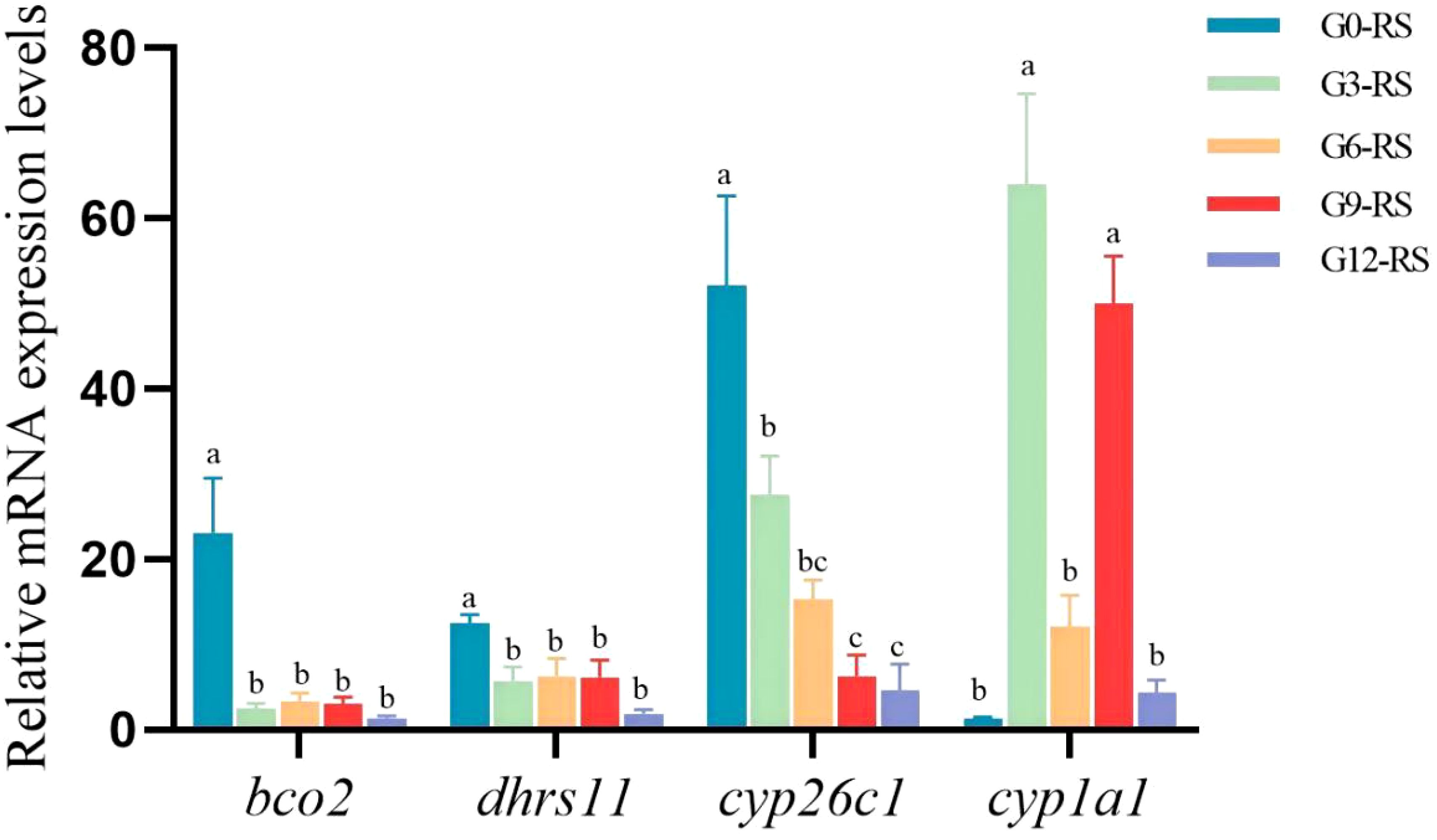
Figure 8. Expression of genes involved in carotenoid and retinoic acid metabolism in spotted scat at different feeding groups. The relative expression level of mRNA transcripts was detected by qRT-PCR and evaluated using the formula, R = 2−ΔΔCt. Data are presented as mean ± SE (n = 3), β-actin was used as the reference gene to normalized genes expression. The different superscript letters above error bar represent the significant difference between different groups (P < 0.05). The different color columns represent the gene expression in different groups. The RS means red skin.
4 Discussion
4.1 Improvement of fish body color through diets supplemented with natural carotenoid additives
Previous studies have demonstrated that incorporating natural carotenoid sources, such as red pepper (Capsicum annuum) peels, rose (Rosa indica) petals (with a carotenoid content of 50 mg/kg), carrot powder (carotenoid content of 164.5 mg/kg), marigold (Calendula officinalis) powder (carotenoid content of 40.47 mg/kg), marigold petals and sweet orange peels, crude palm oil, into diets can significantly increase the a* and b* values, as well as the total carotenoid content in the skin or muscle of jewel cichlid, koi carp (Cyprinus carpio L.), blue gourami (Trichogaster trichopterus), queen loach (Botia dario), gold crucian carp and giant fresh water prawn (Macrobrachium rosenbergii) (Yigit et al., 2021; Jain and Kaur, 2016; Jain et al., 2019; Jorjani et al., 2019; Tripathy et al., 2019; Abbas et al., 2020; Kim et al., 2012). As a result, when the carotenoid content in the feed reached 40 mg/kg, these fish species exhibited more vivid and vibrant body coloration. In this study, the total carotenoid content in Lianjiang red orange peels was reached to 1281.33 mg/kg, which is significantly higher than that of other natural plant sources. After a 4-week feeding period with diets containing 9% Lianjiang red orange peels (carotenoid content of 926.67 mg/kg), the a* value (redness) at the joint of the first and second dorsal fins of spotted scat increased significantly. Moreover, the addition of 12% Lianjiang red orange peels (carotenoid content of 1193.33 mg/kg) led to a significant increase in the b* value (yellowness) of the caudal fin, and at the joint of the second dorsal and caudal fins of spotted scat. Consistent with the observed deeper coloration in the red spots on the skin and caudal fin, the total carotenoid content significantly increased after feeding diets containing 9% and 12% Lianjiang red orange peels. However, no significant differences were observed in the b* value or total carotenoid content in the dorsal fin of spotted scat, regardless of the dosage of Lianjiang red orange peels. These findings indicate that adding an appropriate amount of Lianjiang red orange peels in the diet can enhance carotenoid content and improve body coloration, specifically in the red skin and caudal fin, demonstrating tissue-specific enhancement.
4.2 Molecular mechanism of Lianjiang red orange peels in improving body color in spotted scat
In aquatic animals, dietary carotenoids are typically absorbed in the intestines and subsequently transported to target tissues, such as the skin and muscle, where they are deposited to contribute to coloration (Chatzifotis et al., 2005; Fang et al., 2022). Since carotenoids are fat-soluble and highly hydrophobic, their transport is widely recognized as being closely linked to the circulation associated with lipoproteins (Rodríguez-Bernaldo De Quirós and Costa, 2006; Sakudoh et al., 2013). Specifically, the binding of carotenoids to certain lipoproteins or apolipoproteins is crucial for their effective transport to designated tissues for deposition (Li et al., 2024). In blood parrotfish and leopard coral grouper (Plectropomus leopardus), after being fed diets supplemented with synthetic astaxanthin (Carophyll® pink powder) and natural astaxanthin from Haematococcus pluvialis, transcriptome analysis revealed that DEGs were enriched in pathways related to fatty acid degradation, α-linolenic acid metabolism, and the MAPK and PPAR signaling pathways (Zhang et al., 2023; Micah et al., 2022). Similarly, in spotted scat, DEGs in red skin were enriched in pathways associated with fat digestion and absorption, ether lipid metabolism, α-linolenic acid metabolism, and the MAPK pathway.
Additionally, several key genes have been identified and shown to be involved in carotenoid transport, deposition, metabolism, and red coloration, including fatty acid binding protein 2 (fabp2), stard7, apoa1b, apodb, bco1, beta-carotene 15, 15-dioxygenase 2-like (bco2l), solute carrier family 7 member 11 (slc7a11), paired box 7 (pax7), hsp70, and stard5. The bco2 gene encodes a carotene lyase enzyme, which catalyzes the oxidative cleavage of colored carotenoids derived from the diet into colorless apocarotenoids, a crucial step in carotenoid degradation. A previous study have shown that mutations in bco2 lead to the accumulation of carotenoids in the adipose tissue of Norwegian sheep (Ovis aries) (Våge and Boman, 2010), similar to findings in mice and chickens (Li et al., 2014; Fallahshahroudi et al., 2019). These studies suggest that disruption of bco2 can result in carotenoid accumulation. In ridgetail white prawn (Exopalaemon carinicauda), bco2 mutants displayed a more pronounced yellow body color than the wild-type (Sun et al., 2020). Similarly, in East African cichlid fish (Tropheus duboisi “Maswa”), bco2 was highly expressed in the white skin areas, compared to the yellow skin regions, which aligns with the lack of colored carotenoids (Ahi et al., 2020). In malabar snapper, the expression of bco2-l in the skin and caudal fin was downregulated after dietary astaxanthin supplementation (Poon et al., 2023). In line with these findings, the supplementation of more than 9% Lianjiang red orange peels in the diets of spotted scat significantly downregulated bco2 mRNA expression, leading to an increase in carotenoid content in the red skin of the fish. Therefore, the downregulation of bco2 may be responsible for the enhanced carotenoid deposition and improved coloration in the skin of spotted scat.
Hsp70, a member of the heat shock protein (HSP) family, plays a crucial role in various cellular processes, including cell proliferation, differentiation, apoptosis, signal transduction, protein homeostasis, and protein folding (Belenichev et al., 2023; Shrestha and Young, 2016). Recently, few recent studies found that Hsp70 seems to involve in body coloration. Under heat stress and bacterial challenge, it was found that the expression of CnHSP70 was up-regulated in the same tissue of golden and brown noble scallops (Chlamys nobilis), but the expression levels were different, and this difference may be related to their different carotenoid content (Cheng et al., 2019). It has been reported that Hsp70 family member binding immunoglobulin protein facilitates post-translational modification of tyrosinase (TYR), thus promoting melanin synthesis (Wang et al., 2005). Human heat shock protein 70 member 1A (HSP70-1A) may play a role in regulating the rate of melanin synthesis by chaperoning melanogenic enzymes such as TYR in melanocytes, as well as regulating autophagic activity that degrades pigment-containing melanosomes in keratinocytes and autophagic melanosome degradation in keratinocytes (Murase et al., 2016). During the body color transition from gray to red in adult red crucian carp (Carassius auratus red var.), the expression of hsp70 mRNA was significantly upregulated (Zhang et al., 2017). Similarly, in Malabar snapper, hsp70 mRNA expression, one of the key genes involved in red coloration enhancement, was highly expressed in the skin after feeding on astaxanthin (Poon et al., 2023). In line with these findings, the expression of hsp70 mRNA was induced in the skin of spotted scat in the group fed with 9% Lianjiang red orange peels, further confirming its potential role in enhancing red coloration.
The solute carrier (SLC) transporter family, the second-largest group of membrane proteins, mediates a wide range of solute transport across biological membranes (Zhang et al., 2019). Some SLC members have been identified as candidate genes influencing tissue pigmentation (Gan et al., 2021; Jiang et al., 2014), including slc2a11, solute carrier family 2 member 15 (slc2a15), and solute carrier family 24 member 5 (slc24a5). In pigeons (Columba livia), solute carrier family 2 member 11b (slc2a11b) is essential for pterin-based pigmentation in the iris; a point mutation leads to decreased slc2a11b expression and the absence of xanthophores (Andrade et al., 2021). In fish, the slc2a11 gene is known to be critical for skin pigmentation and chromatophore differentiation. For example, in medaka (Oryzias latipes), mutations in the slc2a11 gene lead to defects in xanthophore differentiation, while overexpression of the slc2a11 gene promotes xanthophore deposition (Kimura et al., 2014). In rainbow trout (Oncorhynchus mykiss), slc2a11 expression was significantly upregulated in the skin of yellow mutants compared to wild-type individuals (Wu et al., 2021, 2022). In the present study, the expression of slc2a11 in spotted scat fed a diet containing 9% Lianjiang red orange peels was markedly enhanced, potentially facilitating xanthophore deposition and improving skin coloration.
Numerous cytochrome P450 (CYP) enzymes typically catalyze mono-oxygenase processes using molecular oxygen and an equivalent amount of electrons, mediating the metabolism of endogenous substrates such as steroids, fatty acids, vitamins, and prostanoids (Bishop-Bailey et al., 2014; Uno et al., 2012; Wang et al., 2007). Previous studies have demonstrated that CYP genes also play a role in erythrophore formation, as well as in the metabolism and deposition of carotenoids (Lopes et al., 2016; Mundy et al., 2016). For instance, in birds, cytochrome P450, family 2, subfamily J, polypeptide 19 (cyp2j19) has been identified as a candidate gene for carotenoid ketolase, which could convert yellow dietary carotenoids into red ketocarotenoids (Emerling, 2017; Kirschel et al., 2020), contributing to yellow and red coloration in beaks and feathers (Lopes et al., 2016; Toomey et al., 2022). In zebrafish (Danio albolineatus), the homolog of avian cyp2j19 is identified as cytochrome P450, family 2, subfamily AE, polypeptide 2 (cyp2ae2), which works in conjunction with 3-hydroxybutyrate dehydrogenase type 1a (bdh1a) to convert dietary carotenoids into ketocarotenoids, thereby mediating red coloration and maintaining ketocarotenoids. Mutations in cyp2ae2 and bdh1a result in the loss of erythrophores in the fin region and a significant decrease in astaxanthin content (Huang et al., 2021a). However, in this study, the expression of cyp2ae2 in spotted scat showed no significant difference between the control group and the group fed with 9% Lianjiang red orange peels. Instead, three other cytochrome P450 family members - cyp2j6, cyp1a1, and cyp26c1-were identified. In yesso scallop (Patinopecten yessoensis), the expression of cyp2j6 was positively correlated with the concentrations of pectenolone and pectenoxanthin (Wang et al., 2022). In crimson snapper (Lutjanus erythropterus), the expression of the carotene oxidation gene cyp2j6 was upregulated in juveniles at 3 days post-hatching (dph), a key developmental stage for pigmentation during which xanthophores gradually appear, indicating the involvement of cyp2j6 in xanthophore deposition (Xu et al., 2023). In the present study, cyp2j6 mRNA expression was upregulated in spotted scat fed a diet containing 9% Lianjiang red orange peels, coinciding with the improvement in body coloration and increased carotenoid content. These results suggest that cyp2j6 may be involved in carotenoid metabolism, xanthophore deposition, and skin coloration.
Another member of the CYP gene family, cyp1a1, encodes a monooxygenase that metabolizes both exogenous and endogenous substrates (Mescher and Haarmann-Stemmann, 2018). In the liver of ungulates (deer, cattle, and horses) and mice, the content of β-carotene has been positively correlated with cyp1a1 protein expression (Darwish et al., 2010). In rats (Rattus norvegicus), astaxanthin extracted from Haematococcus pluvialis could induce high cyp1a1 mRNA expression in liver cancer cells (Ohno et al., 2012). In this study, cyp1a1 mRNA expression was significantly upregulated in spotted scat fed with 9% Lianjiang red orange peels. These results suggest that natural carotenoids can induce high cyp1a1 mRNA expression, which is correlated with the content of endogenous carotenoids.
In addition, dietary carotenoids are commonly found in fruits and vegetables. Among them, β-carotene, α-carotene, and β-cryptoxanthin are referred to as pro-vitamin A carotenoids, as they can be converted into retinol (vitamin A) (Zhong et al., 2018). It is well known that retinol can be further converted into retinoic acid. Therefore, diets rich in carotenoids may influence retinoic acid metabolism. For instance, cytochrome P450 family 26 enzymes, including Cyp26a1 (cytochrome P450 family 26 subfamily A member 1), Cyp26b1 (subfamily B member 1), and Cyp26c1 (subfamily C member 1), are responsible for retinoic acid metabolism (Isoherranen and Zhong, 2019). In blood parrots, the addition of astaxanthin to the diet significantly reduced the expression of the cyp26b1 gene in the skin (Micah et al., 2022). Similarly, in the present study, cyp26c1 expression was significantly decreased in the group fed with Lianjiang red orange peels. Moreover, a previous study showed that β-apocarotenoids significantly inhibited retinoic acid-induced Cyp26a1 expression in HEPG2 cells (Harrison, 2022). Thus, feeding Lianjiang red orange peels may affect the conversion of carotenoids to retinoic acid in spotted scat. Collectively, these findings suggest that diets incorporating Lianjiang red orange peels influence the expression of genes associated with carotenoid absorption, metabolism, and deposition, leading to increased carotenoid content and enhanced red coloration in spotted scat.
5 Conclusion
The results of the study demonstrate that supplementing feed with Lianjiang red orange peels as a carotenoid source significantly increases the a* and b* values and content of carotenoids in the red skin and fins of spotted scat, likely due to a higher carotenoid content in these areas. Comparative transcriptome analysis of the red skin revealed that DEGs were enriched in α-linoleic acid metabolism and MAPK signaling pathway, both of which are involved in the deposition of red pigments. The upregulation of Cytochrome P450 gene family members (cyp2j6 and cyp1a1) and pigment-related genes (hsp70 and slc2a11) could promote the deposition of carotenoids and pteridine pigments after feeding diets with 9% orange peels. The downregulation of bco2 and cyp26c1 could enhance the body red color in spotted scat by inhibiting the oxidative cleavage of carotenoids and retinoic acid metabolism. These findings suggest that Lianjiang red orange peels have significant potential as a natural carotenoid source for enhancing fish coloration. Future studies should explore the effects of incorporating Lianjiang red orange peel into the diets to feed various sizes of fish and the prolonged feeding time on coloration enhancement. Additionally, the mechanism of Lianjiang red orange peels on the enhancement of red pigmentation in fish should be further explored.
Data availability statement
The datasets presented in this study can be found in online repositories. The names of the repository/repositories and accession number(s) can be found in the article/supplementary material.
Ethics statement
The animal studies were approved by The Guangdong Ocean University Animal Research and Ethics Committee authorized each and every experimental procedure used in this work (NIH Pub. No.85-23, amended 1996). There are no protected or endangered species included in this study. Every attempt was made to reduce the animals’ suffering. Every experiment was carried out in compliance with all applicable laws and rules. The studies were conducted in accordance with the local legislation and institutional requirements. Written informed consent was obtained from the owners for the participation of their animals in this study.
Author contributions
TH: Data curation, Investigation, Methodology, Validation, Visualization, Writing – original draft. YL: Investigation, Methodology, Validation, Writing – original draft. LY: Validation, Writing – original draft. XL: Validation, Writing – original draft. GS: Resources, Writing – original draft. CZ: Resources, Writing – original draft. DJ: Funding acquisition, Resources, Writing – original draft. GL: Funding acquisition, Resources, Supervision, Writing – original draft. HS: Funding acquisition, Supervision, Writing – review & editing.
Funding
The author(s) declare financial support was received for the research, authorship, and/or publication of this article. This research was supported by grants from the National Natural Science Foundation of China (grant numbers: 32473155, 32201420 and 32002367), the Guangdong Provincial Science and Technology Program (grant number: 2023B0202010005), Guangdong Basic and Applied Basic Research Foundation (grant number: 2023A1515012880 and 2024A1515010328), the Zhanjiang Science and Technology Plan Project (grant number: 2022A01046).
Acknowledgments
The authors would like to thank Yu-Cong Hong of the Guangdong Provincial Key Laboratory of Aquatic Larvae Feed, Jieyang 522000, China for his help with the preparation of experiment in this paper. The authors express their gratitude to the funding bodies for their support and acknowledge the contributions of the laboratory members, whose rigorous experimentation and valuable discussions have been instrumental to the achievement of the project goals.
Conflict of interest
The authors declare that the research was conducted in the absence of any commercial or financial relationships that could be construed as a potential conflict of interest.
Generative AI statement
The author(s) declare that no Generative AI was used in the creation of this manuscript.
Publisher’s note
All claims expressed in this article are solely those of the authors and do not necessarily represent those of their affiliated organizations, or those of the publisher, the editors and the reviewers. Any product that may be evaluated in this article, or claim that may be made by its manufacturer, is not guaranteed or endorsed by the publisher.
References
Ababor S., Tamiru M., Alkhtib A., Wamatu J., Kuyu C. G., Teka T. A., et al. (2023). The use of biologically converted agricultural byproducts in chicken nutrition. Sustainability 15, 14562. doi: 10.3390/su151914562
Abbas S., Haider M. S., Kafayet F., Ashraf S., Batool M. (2020). Effect of citrus peels mingled diets on carassius auratus coloration. Pakistan J. Zoology 52, 425–824. doi: 10.17582/journal.pjz/20161107041109
Ahi E. P., Lecaudey L. A., Ziegelbecker A., Steiner O., Glabonjat R., Goessler W., et al. (2020). Comparative transcriptomics reveals candidate carotenoid color genes in an East African cichlid fish. BMC Genomics 21, 54. doi: 10.1186/s12864-020-6473-8
Andrade P., Gazda M. A., Araújo P. M., Afonso S., Rasmussen J. A., Marques C. I., et al. (2021). Molecular parallelisms between pigmentation in the avian iris and the integument of ectothermic vertebrates. PloS Genet. 17, e1009404. doi: 10.1371/journal.pgen.1009404
Anis Mohamad Sukri S., Andu Y., Tuan Harith Z., Sarijan S., Naim Firdaus Pauzi M., Seong Wei L., et al. (2022). Effect of feeding pineapple waste on growth performance, texture quality and flesh colour of nile tilapia (Oreochromis niloticus) fingerlings. Saudi Journal of Biological Sciences 29, 2514–2519. doi: 10.1016/j.sjbs.2021.12.027
Belenichev I. F., Aliyeva O. G., Popazova O. O., Bukhtiyarova N. V. (2023). Involvement of heat shock proteins HSP70 in the mechanisms of endogenous neuroprotection: the prospect of using HSP70 modulators. Front. Cell Neurosci. 17. doi: 10.3389/fncel.2023.1131683
Besen K. P., Melim E. W. H., Cunha L. D., Favaretto E. D., Moreira M., Fabregat T. E. H. P. (2019). Lutein as a natural carotenoid source: Effect on growth, survival and skin pigmentation of goldfish juveniles (Carassius auratus). Aquaculture Res. 50, 2200–2206. doi: 10.1111/are.14101
Bishop-Bailey D., Thomson S., Askari A., Faulkner A., Wheeler-Jones C. (2014). Lipid-metabolizing CYPs in the regulation and dysregulation of metabolism. Annu. Rev. Nutr. 34, 261–279. doi: 10.1146/annurev-nutr-071813-105747
Chatzifotis S., Pavlidis M., Jimeno C. D., Vardanis G., Sterioti A., Divanach P. (2005). The effect of different carotenoid sources on skin coloration of cultured red porgy (Pagrus pagrus). Aquac Res. 36, 1517–1525. doi: 10.1111/j.1365-2109.2005.01374.x
Cheng D. W., Liu H. X., Zhang H. K., Soon T. K., Ye T., Li S. K., et al. (2019). Differential expressions of HSP70 gene between golden and brown noble scallops Chlamys nobilis under heat stress and bacterial challenge. Fish Shellfish Immunol. 94, 924–933. doi: 10.1016/j.fsi.2019.10.018
Darwish W. S., Ikenaka Y., Ohno M., Eldaly E. A., Ishizuka M. (2010). Carotenoids as regulators for inter-species difference in cytochrome P450 1A expression and activity in ungulates and rats. Food Chem. Toxicol. 48, 3201–3208. doi: 10.1016/j.fct.2010.08.022
Elbahnaswy S., Elshopakey G. E. (2024). Recent progress in practical applications of a potential carotenoid astaxanthin in aquaculture industry: a review. Fish Physiol. Biochem. 50, 97–126. doi: 10.1007/s10695-022-01167-0
Emerling C. A. (2017). Independent pseudogenization of CYP2J19 in penguins, owls and kiwis implicates gene in red carotenoid synthesis. Mol. Phylogenet. Evol. 118, 47–53. doi: 10.1101/130468
Fallahshahroudi A., Sorato E., Altimiras J., Jensen P. (2019). The domestic BCO2 allele buffers low-carotenoid diets in chickens: possible fitness increase through species hybridization. Genetics 212, 1445–1452. doi: 10.1534/genetics.119.302258
Fan S., Shi H. J., Peng Y. X., Huang Y. Q., Jiang M. Y., Li G. L., et al. (2023). Dietary aromatase inhibitor treatment converts XY gsdf homozygous mutants to sub-fertile male in Nile tilapia (Oreochromis niloticus). Aquaculture 569, 739381. doi: 10.1016/j.aquaculture.2023.739381
Fang W., Huang J., Li S. Z., Lu J. G. (2022). Identification of pigment genes (melanin, carotenoid and pteridine) associated with skin color variant in red tilapia using transcriptome analysis. Aquaculture 547, 737429. doi: 10.1016/j.aquaculture.2021.737429
Gan W., Chung-Davidson Y. W., Chen Z., Song S. Y., Cui W. Y., He W., et al. (2021). Global tissue transcriptomic analysis to improve genome annotation and unravel skin pigmentation in goldfish. Sci. Rep. 11, 1815. doi: 10.1038/s41598-020-80168-6
Harrison E. H. (2022). Carotenoids, β-apocarotenoids, and retinoids: the long and the short of it. Nutrients 14, 1411. doi: 10.3390/nu14071411
Huang D., Lewis V. M., Foster T. N., Toomey M. B., Corbo J. C., Parichy D. M. (2021a). Development and genetics of red coloration in the zebrafish relative Danio albolineatus. eLife 10, e70253. doi: 10.7554/eLife.70253
Huang Y. Q., Mustapha U. F., Huang Y., Tian C. X., Yang W., Chen H. P., et al. (2021b). A chromosome—Level genome assembly of the spotted scat (Scatophagus argus). Genome Biol. Evol. 13, evab092. doi: 10.1093/gbe/evab092
Huang Z. H., Ma B. H., Guo X. L., Wang H. H., Ma A., Sun Z. B., et al. (2021c). Comparative transcriptome analysis of the molecular mechanism underlying the golden red colour in mutant Taiwanese loach. Aquaculture 543, 736979. doi: 10.1016/j.aquaculture.2021.736979
Isoherranen N., Zhong G. (2019). Biochemical and physiological importance of the CYP26 retinoic acid hydroxylases. Pharmacol. Ther. 204, 107400. doi: 10.1016/j.pharmthera.2019.107400
Jain A., Kaur V. I. (2016). Rose petal meal as a potential natural carotenoid source for pigmentation and growth of freshwater ornamental fish, koi carp, Cyprinus carpio (Linnaeus). Indian J. Ecol. 43, 239–244.
Jain A., Kaur V. I., Hollyappa S. A. (2019). Effect of dietary supplementation of carrot meal on survival, growth and pigmentation of freshwater ornamental fish, koi carp, cyprinus carpio (L.). Indian J. Anim. Nutr. 36, 405. doi: 10.5958/2231-6744.2019.00066.5
Jiang Y. L., Zhang S. H., Xu J., Feng J. X., Mahboob S., Al-Ghanim K. A., et al. (2014). Comparative transcriptome analysis reveals the genetic basis of skin color variation in common carp. PloS One 9, e108200. doi: 10.1371/journal.pone.0108200
Jorjani M., Sharif Rohani M. S., Rostami A. M., Ako H., Tan Shau Hwai A. (2019). Pigmentation and growth performance in the blue gourami, Trichogaster trichopterus, fed marigold, Calendula officinalis, powder, a natural carotenoid source. J. World Aquaculture Soc. 50, 789–799. doi: 10.1111/jwas.12562
Kim D. S., Lee S., Park S. M., Yun S. H., Gab H. S., Kim S. S., et al. (2021). Comparative metabolomics analysis of citrus varieties. Foods 10, 2826. doi: 10.3390/foods10112826
Kim Y. C., Romano N., Lee K. S., Teoh C. Y., Ng W. K. (2012). Effects of replacing dietary fish oil and squid liver oil with vegetable oils on the growth, tissue fatty acid profile and total carotenoids of the giant freshwater prawn, Macrobrachium rosenbergii. Aquaculture Res. 1–10. doi: 10.1111/j.1365-2109.2012.03179.x
Kimura T., Nagao Y., Hashimoto H., Yamamoto-Shiraishi Y., Yamamoto S., Yabe T., et al. (2014). Leucophores are similar to xanthophores in their specification and differentiation processes in medaka. Proc. Natl. Acad. Sci. United States America 111, 7343–7348. doi: 10.1073/pnas.1311254111
Kirschel A. N. G., Nwankwo E. C., Pierce D. K., Lukhele S. M., Moysi M., Ogolowa B. O., et al. (2020). CYP2J19 mediates carotenoid colour introgression across a natural avian hybrid zone. Mol. Ecol. 29, 4970–4984. doi: 10.1111/mec.15691
Li B. J., Chen L., Yan M. Z., Jiang Z., Xue Y. G., Xu P. (2024). Integrative transcriptomics and metabolomics analysis of body color formation in the common carp. Aquaculture 579, 740143. doi: 10.1016/j.aquaculture.2023.740143
Li B. X., Vachali P. P., Gorusupudi A., Shen Z. Q., Sharifzadeh H., Besch B. M., et al. (2014). Inactivity of human β,β-carotene-9′,10′-dioxygenase (BCO2) underlies retinal accumulation of the human macular carotenoid pigment. Proc. Natl. Acad. Sci. United States America 111, 10173–10178. doi: 10.1073/pnas.1402526111
Liao Y. G., Shi H. J., Han T., Jiang D. N., Lu B. Y., Shi G., et al. (2023). Pigment Identification and Gene Expression Analysis during Erythrophore Development in Spotted Scat (Scatophagus argus) Larvae. Int. J. Mol. Sci. 24, 15356. doi: 10.3390/ijms242015356
Lopes R. J., Johnson J. D., Toomey M. B., Ferreira M. S., Araujo P. M., Melo-Ferreira J., et al. (2016). Genetic basis for red coloration in birds. Curr. Biol. 26, 1427–1434. doi: 10.1016/j.cub.2016.03.076
Luo M. K., Lu G. Q., Yin H. R., Wang L. M., Atuganile M., Dong Z. J. (2021). Fish pigmentation and coloration: Molecular mechanisms and aquaculture perspectives. Rev. Aquaculture 13, 2395–2412. doi: 10.1111/raq.12583
Mescher M., Haarmann-Stemmann T. (2018). Modulation of CYP1A1 metabolism: From adverse health effects to chemoprevention and therapeutic options. Pharmacol. Ther. 187, 71–87. doi: 10.1016/j.pharmthera.2018.02.012
Micah A. D., Wen B., Wang Q., Zhang Y., Yusuf A., Thierry N. N. B., et al. (2022). Effect of dietary astaxanthin on growth, body color, biochemical parameters and transcriptome profiling of juvenile blood parrotfish (Vieja melanurus ♀ × Amphilophus citrinellus ♂). Aquaculture Rep. 24, 101142. doi: 10.1016/j.aqrep.2022.101142
Mundy N. I., Stapley J., Bennison C., Tucker R., Twyman H., Kim K. W., et al. (2016). Red carotenoid coloration in the zebra finch is controlled by a cytochrome P450 gene cluster. Curr. Biol. 26, 1435–1440. doi: 10.1016/j.cub.2016.04.047
Murase D., Hachiya A., Fullenkamp R., Beck A., Moriwaki S., Hase T., et al. (2016). Variation in Hsp70-1A expression contributes to skin color diversity. J. Invest. Dermatol. 136, 1681–1691. doi: 10.1016/j.jid.2016.03.038
Nakano T., Wiegertjes G. (2020). Properties of carotenoids in fish fitness: A review. Mar. Drugs 18, 568. doi: 10.3390/md18110568
Ohno M., Darwish W. S., Ikenaka Y., Miki W., Fujita S., Ishizuka M. (2012). Astaxanthin rich crude extract of Haematococcus pluvialis induces cytochrome P450 1A1 mRNA by activating aryl hydrocarbon receptor in rat hepatoma H4IIE cells. Food Chem. 130, 356–361. doi: 10.1016/j.foodchem.2011.07.050
Pereira Da Costa D., Campos Miranda-Filho K. (2020). The use of carotenoid pigments as food additives for aquatic organisms and their functional roles. Rev. Aquaculture 12, 1567–1578. doi: 10.1111/raq.12398
Poon Z. W. J., Shen X., Uichanco J. A., Terence C., Chua S. W. G., Domingos J. A. (2023). Comparative transcriptome analysis reveals factors involved in the influence of dietary astaxanthin on body colouration of Malabar Snapper (Lutjanus malabaricus). Aquaculture 562, 738874. doi: 10.1016/j.aquaculture.2022.738874
Rațu R. N., Veleșcu I. D., Stoica F., Usturoi A., Arsenoaia V. N., Crivei I. C., et al. (2023). Application of agri-food by-products in the food industry. Agriculture 13, 1559. doi: 10.3390/agriculture13081559
Rafiq S., Kaul R., Sofi S. A., Bashir N., Nayik G. A. (2016). Citrus peel as a source of functional ingredient: A Review. J. Saudi Soc. Agric. Sci. 17, 351–358. doi: 10.1016/j.jssas.2016.07.006
Rodríguez-Bernaldo De Quirós A., Costa H. S. (2006). Analysis of carotenoids in vegetable and plasma samples: A review. J. Food Composition Anal. 19, 97–111. doi: 10.1016/j.jfca.2005.04.004
Sakudoh T., Kuwazaki S., Iizuka T., Narukawa J., Yamamoto K., Uchino K., et al. (2013). CD36 homolog divergence is responsible for the selectivity of carotenoid species migration to the silk gland of the silkworm Bombyx mori. J. Lipid Res. 54, 482–495. doi: 10.1194/jlr.M032771
Salem M. E., Abdel-Ghany H. M., Sallam A. E., El-Feky M. M. M., Almisherfi H. M., Mohamed E.‐S. (2019). Effects of dietary orange peel on growth performance, antioxidant activity, intestinal microbiota and liver histology of Gilthead sea bream (Sparus aurata) larvae. Aquaculture Nutr. 25, 1087–1097. doi: 10.1111/anu.12925
Shrestha L., Young J. C. (2016). Function and chemotypes of human hsp70 chaperones. Curr. Topics Medicinal Chem. 16, 2812–2828. doi: 10.2174/1568026616666160413142028
Sun Y. Y., Liu M. F., Yan C. C., Yang H., Wu Z., Liu Y., et al. (2020). CRISPR/Cas9-mediated deletion of β, β-carotene 9′, 10′-oxygenase gene (EcBCO2) from Exopalaemon carinicauda. Int. J. Biol. Macromolecules 151, 168–177. doi: 10.1016/j.ijbiomac.2020.02.073
Svensson, Wong (2011). Carotenoid-based signals in behavioural ecology: a review. Behaviour 148, 131–189. doi: 10.1163/000579510X548673
Toomey M. B., Lopes R. J., Araújo P. M., Johnson J. D., Gazda M. A., Afonso S., et al. (2017). High-density lipoprotein receptor SCARB1 is required for carotenoid coloration in birds. Proc. Natl. Acad. Sci. United States America 114, 5219–5224. doi: 10.1073/pnas.1700751114
Toomey M. B., Marques C. I., Araújo P. M., Huang D., Zhong S., Liu Y., et al. (2022). A mechanism for red coloration in vertebrates. Curr. Biol. 32, 4201–4214.e12. doi: 10.1016/j.cub.2022.08.013
Torres Sánchez E. G., Fuenmayor C. A., Vásquez Mejía S. M., Díaz-Moreno C., Suárez Mahecha H. (2020). Effect of bee pollen extract as a source of natural carotenoids on the growth performance and pigmentation of rainbow trout (Oncorhynchus mykiss). Aquaculture 514, 734490. doi: 10.1016/j.aquaculture.2019.734490
Torrissen O. J. (1985). Pigmentation of salmonids: Factors affecting carotenoid deposition in rainbow trout (Salmo gairdneri). Aquaculture 46, 133–142. doi: 10.1016/0044-8486(85)90197-8
Tripathy P. S., Devi N. C., Parhi J., Priyadarshi H., Patel A. B., Pandey P. K., et al. (2019). Molecular mechanisms of natural carotenoid-based pigmentation of queen loach, botia dario (Hamilto) under captive condition. Sci. Rep. 9, 12585. doi: 10.1038/s41598-019-48982-9
Uno T., Ishizuka M., Itakura T. (2012). Cytochrome P450 (CYP) in fish. Environ. Toxicol. Pharmacol. 34, 1–13. doi: 10.1016/j.etap.2012.02.004
Våge D. I., Boman I. A. (2010). A nonsense mutation in the beta-carotene oxygenase 2 (BCO2) gene is tightly associated with accumulation of carotenoids in adipose tissue in sheep (Ovis aries). BMC Genet. 11, 10. doi: 10.1186/1471-2156-11-10
Wang N., Daniels R., Hebert D. N. (2005). The cotranslational maturation of the type I membrane glycoprotein tyrosinase: the heat shock protein 70 system hands off to the lectin-based chaperone system. Mol. Biol. Cell 16, 3740–3752. doi: 10.1091/mbc.e05-05-0381
Wang P., Li X., Wang X. Q., Peng N., Luo Z. J. (2020). Effects of dietary xanthophyll supplementation on growth performance, body color, carotenoids, and blood chemistry indices of chinese soft-shelled turtle pelodiscus sinensis. North Am. J. Aquaculture 82, 394–404. doi: 10.1002/naaq.10161
Wang S. Y., Wang H. Z., Zhao L., Zhang Y. H., Li T. T., Liu S. Q., et al. (2022). Identification of genes associated with carotenoids accumulation in scallop (Patinopecten yessoensis). Aquaculture 550, 737850. doi: 10.1016/j.aquaculture.2021.737850
Wang L., Yao J. H., Chen L., Chen J. Z., Xue J. G., Jia W. L. (2007). Expression and possible functional roles of cytochromes P450 2J1 (zfCyp2J1) in zebrafish. Biochem. Biophys. Res. Commun. 352, 850–855. doi: 10.1016/j.bbrc.2006.11.129
Wu S. J., Huang J. Q., Li Y. J., Zhao L., Liu Z. (2022). Analysis of yellow mutant rainbow trout transcriptomes at different developmental stages reveals dynamic regulation of skin pigmentation genes. Sci. Rep. 12, 256. doi: 10.1038/s41598-021-04255-y
Wu S. J., Huang J. Q., Li Y. J., Zhao L., Liu Z., Kang Y. J., et al. (2021). Integrative mRNA-miRNA interaction analysis reveals the molecular mechanism of skin color variation between wild-type and yellow mutant rainbow trout (Oncorhynchus mykiss). Comp. Biochem. Physiol. Part D: Genomics Proteomics 40, 100914. doi: 10.1016/j.cbd.2021.100914
Xu Z. M., Liang Q. L., Chen Z. Z., Dong Z. D., Guo Y. S., Wang Z. D. (2023). Weighted Gene Co-Expression Network Analysis of red body color formation of crimson snapper, Lutjanus erythropterus. Aquaculture Rep. 31, 101651. doi: 10.1016/j.aqrep.2023.101651
Yi X., Li J., Xu W., Zhang W., Mai K. (2016). Effects of dietary lutein/canthaxanthin ratio on the growth and pigmentation of large yellow croaker Larimichthys croceus. Aquaculture Nutr. 22, 683–690. doi: 10.1111/anu.12289
Yigit N. O., Ozdal A. M., Bahadir Koca S., Ozmen O. (2021). Effect on pigmentation, growth and liver histopathology of red pepper (Capsicum annuum) added in different ratios to jewel cichlid (Hemichromis guttatus) diet. Aquaculture Res. 52, 5579–5584. doi: 10.1111/are.15433
Zhang Y. Q., Liu J. H., Peng L. Y., Ren L., Zhang H. Q., Zou L. J., et al. (2017). Comparative transcriptome analysis of molecular mechanism underlying gray-to-red body color formation in red crucian carp (Carassius auratus, red var.). Fish Physiol. Biochem. 43, 1387–1398. doi: 10.1007/s10695-017-0379-7
Zhang J. P., Tian C. X., Zhu K. F., Liu Y., Zhao C., Jiang M. Y., et al. (2023). Effects of natural and synthetic astaxanthin on growth, body color, and transcriptome and metabolome profiles in the leopard coralgrouper (Plectropomus leopardus). Animals 13, 1252. doi: 10.3390/ani13071252
Zhang G., Wang W., Su M. L., Zhang J. B. (2018). Effects of recombinant gonadotropin hormones on the gonadal maturation in the spotted scat, Scatophagus argus. Aquaculture 483, 263–272. doi: 10.1016/j.aquaculture.2017.10.017
Zhang Y., Zhang Y. O., Sun K., Meng Z. Y., Chen L. G. (2019). The SLC transporter in nutrient and metabolic sensing, regulation, and drug development. J. Mol. Cell Biol. 11, 1–13. doi: 10.1093/jmcb/mjy052
Keywords: spotted scat, Lianjiang red orange, natural carotenoids source, body color enhancement, transcriptome analysis
Citation: Han T, Liao Y, Yang L, Li X, Shi G, Jiang D-N, Zhu C, Li G and Shi H (2024) Enhancement of body red coloration in juvenile spotted scat (Scatophagus argus) by dietary supplementation with Lianjiang red orange peels as a natural carotenoid source. Front. Mar. Sci. 11:1515751. doi: 10.3389/fmars.2024.1515751
Received: 23 October 2024; Accepted: 26 November 2024;
Published: 16 December 2024.
Edited by:
Shubo Jin, Chinese Academy of Fishery Sciences (CAFS), ChinaCopyright © 2024 Han, Liao, Yang, Li, Shi, Jiang, Zhu, Li and Shi. This is an open-access article distributed under the terms of the Creative Commons Attribution License (CC BY). The use, distribution or reproduction in other forums is permitted, provided the original author(s) and the copyright owner(s) are credited and that the original publication in this journal is cited, in accordance with accepted academic practice. No use, distribution or reproduction is permitted which does not comply with these terms.
*Correspondence: Hongjuan Shi, c2hpaGpAZ2RvdS5lZHUuY24=