- 1College of Life Science and Engineering, Southwest University of Science and Technology, Mianyang, China
- 2Biomass Center, School of Life Science and Engineering, Southwest University of Science and Technology, Mianyang, Sichuan, China
- 3Key Laboratory of Freshwater Fisheries and Germplasm Resources Utilization, Ministry of Agriculture and Rural Affairs, Freshwater Fisheries Research Center, Chinese Academy of Fishery Sciences, Wuxi, China
Introduction: In recent years, with the continuous expansion of aquaculture areas worldwide and the outbreak of diseases, the use of antibiotics and chemical drugs is limited. Plant polysaccharides have received widespread attention due to their multiple bioactivities. However, research on the combined use of plant polysaccharides and Bacillus coagulans is still insufficient. Therefore, this study focuses on the impact of B.coagulans-fermented polysaccharides on Macrobrachium nipponense.
Methods: An 8-week feeding trial was conducted with seven groups: the control group (CT) and the Bacillus coagulans group (N),Atractylodes macrocephala polysaccharides group (NB), Saposhnikovia divaricata polysaccharides group (NF), Mannose group (NG), Astragalus polysaccharides group (NH) and Yu ping feng polysaccharides group (NP).
Results and discussion: The research results indicate that compared to the CT, the levels of AST and ALT were reduced in the group of N, NF and NG. The NF showed a significant increase in total antioxidant capacity (T-AOC) and total superoxide dismutase (SASC) levels. The NP had a significant increase in T-AOC and superoxide anion scavenging ability. The levels of total protein (TP) and malondialdehyde (MDA) in the group of NG, NB, and NP were significantly higher than those in the CT and N. Compared to the CT, the expression of Toll in the NP group, Myd88 and Dorsal in the NH group, and IMD and Relish in the NF and NP group were all significantly increased. Conversely, the expression of IMD in the NB and NG group and Relish in the NG group was significantly decreased. Additionally, the survival rate in the NP group was significantly higher than in other groups, and the NB group enhanced the weight gain of M.nipponense compared to the N. In summary, B.coagulans fermented with Yupingfeng polysaccharides and Astragalus polysaccharides can significantly enhance the antioxidant and immune capabilities of M.nipponense.
1 Introduction
Macrobrachium nipponense is a freshwater shrimp with a large breeding area in China and is steadily expanding worldwide (Jiang et al., 2019). The aquaculture industry has suffered significant economic losses in recent years due to recurrent outbreaks of prawn diseases (Hou et al., 2018; Joshi et al., 2014). Traditionally, antibiotics and chemical drugs were used to prevent and treat prawn diseases. However, their use is restricted due to increasing bacteria drug resistance and food safety concerns. While plant extracts offer a promising alternative to antibiotics, their cost-effectiveness remains challenging. Therefore, the search for safe, effective, and economically feasible plant extracts for treating prawn diseases remains a priority (Bulfon et al., 2015). Plant polysaccharides from plant extracts are considered natural alternatives to chemical drugs and antibiotics and are widely used in aquaculture (Tzianabos, 2000).
Prebiotics have been demonstrated to improve host health, which in turn facilitates the growth and development of prawns (Kiernan et al., 2023). Related studies have shown that prebiotics have the characteristics of improving the structure of intestinal flora and stimulating the proliferation of probiotics (Li and Gatlin, 2005; Munir et al., 2016). The main physiological functions of prebiotics include enhancing the body’s immunity (Sredkova et al., 2020), promoting the absorption of minerals (Karakan et al., 2021) and reducing inflammatory response (McLoughlin et al., 2017) and other efficacy. Prebiotics are polysaccharides extracted from plants and can be digested by the bacteria to produce short-chain fatty acids, which have anti-inflammatory and immune-enhancing effects (Jenab et al., 2020). Currently, Atractylodes rhizoma (Sun et al., 2023), mannooligosaccharides (Forsatkar et al., 2018), Yupingfeng polysaccharide (Su et al., 2020), and other prebiotics have been added to aquafeed. Atrhizoma polysaccharide plays a vital role in folk medicine, treating a variety of diseases (Pan et al., 2018) and improving immunity (Sun et al., 2015), which may be due to its ability to alleviate immunosuppression induced by cyclophosphamide (Li et al., 2019a, b). At the same time, Atractylodes polysaccharides can induce TLR4 to activate NF-κB, which in turn activates macrophages to synthesize cytokines (Wei et al., 2016). Previous studies have shown that Atractylodes polysaccharide also plays an important role in heat stress and immune function improvement (Xu et al., 2017; Xu and Tian, 2015). Adding mannooligosaccharides during fish breeding can promote the growth and reproduction (Forsatkar et al., 2018). Yupingfeng polysaccharide is an Astragalus membranaceus based ancient Chinese herbal medicine that regulates humoral and cellular immunity and inhibits inflammation (Fabrizio et al., 2013). Related studies have shown that Yupingfeng polysaccharide can improve the foregut microbiota and intestinal barrier of weanling rabbits and enhance the immunity of rabbits (Sun et al., 2016). Moreover, Yupingfeng polysaccharide could effectively improve the immune response, disease resistance and growth performance of Litopenia vannamen (Su et al., 2020). Astragalus polysaccharide has a variety of biological activities, such as immunomodulatory (Liu et al., 2017), antioxidant (Li et al., 2010), and antibacterial effects (Ma et al., 2017). In aquaculture, Astragalus polysaccharide has been shown to promote growth performance, improve physiological and biochemical indicators and increase the expression of genes related to lipid metabolism (Huang et al., 2023).Parsnip polysaccharide can modulate immune activity, significantly increase immune cell density and macrophage number, and reduce the expression of NO, TNF-α, IL-1β and IL-6 (Fan et al., 2023).
Bacillus coagulans is a lactic-producing bacillus that can produce short-chain fatty acids such as acetic acid and propionic acid, promote gastrointestinal peristalsis, and regulate the structure of microenvironment flora (Zhu et al., 2019). B.coagulans utilize polysaccharides for growth and secrete digestive enzymes and bacteriocins that promote digestion and absorption (Mazkour et al., 2022). B.coagulans can significantly improve shrimp growth performance and serum antioxidant capacity in shrimp culture (Sadat Hoseini Madani et al., 2018). B.coagulans can also up-regulate the transcription level and related enzyme activities of SOD and CAT genes in zebrafish, and protect zebrafish larvae against oxidative stress induced by copper sulfate (Ai et al., 2023). In livestock and poultry breeding, B.coagulans can improve the growth performance of C. vermilmilium, enhance intestinal innate immunity and improve intestinal microbial community (Fu et al., 2019). This study addresses the disease issues faced in the cultivation of M. nipponense by exploring the potential of B.coagulans-fermented plant polysaccharides as a green additive. Plant polysaccharides have garnered attention in aquaculture due to their diverse bioactivities, while B.coagulans is favored for its ability to promote growth, enhance antioxidant capacity, boost immunity and improve gut microbiota. The study focuses on the combined effects of B.coagulans and plant polysaccharides on M.nipponense, aiming to provide a new green additive application scheme for shrimp farming, which can help improve the breeding environment and increase breeding efficiency.
2 Materials and methods
2.1 Test material
Parsnip polysaccharide (NF), Atractylodes rhizoma polysaccharide (NB), Astragalus polysaccharide (NH) and Yuingfeng polysaccharide (NP) were obtained from Baoding Jizhong Biological Technology Co., Ltd (Hebei, China).Mannose (NG)was obtained from Qingdao Hehai Biotechnology Co., Ltd (Shandong, China). B.coagulans (N) was obtained from Jiangsu Su Wei Biological Co., Ltd. and Freshwater Fisheries Research Center of Chinese Academy of Fishery Sciences. The crude polysaccharide content was determined according to the industry standard NY/T 1676-2008, and its composition contents are shown in Table 1. The experimental prawns were provided by the Dapu Freshwater prawns experiment station of Freshwater Fisheries Research Center, Chinese Academy of Fisheries Sciences.
2.2 Feed preparation
B.coagulans were inoculated into an LB slant medium and cultured at 37°C for 24 hours to obtain the activated strains. Then 1% Astragalus polysaccharide,1% Yupingfeng polysaccharide, 1%Mannose, 4%Parsnip polysaccharide 4%,Atractylodes rhizoma polysaccharide were added to B.coagulans in an incubator at 37°C and 180 rpm for 24 h. B.coagulans with the polysaccharide fermentation broth were adjusted to 108 CFU/mL and added to the feed. The experimental feed formula is shown in Table 2. The experimental feed was made in the Fresh Water Center of the Chinese Academy of Fisheries Sciences, weighed and mixed with 60 mesh sieve, and finally mixed with oil and cultivated fermentation broth and water. The feed with a diameter of 1.0 mm was made in a twin-screw extruder, and the feed was dried in the shade and put into a refrigerator at -20°C to feed the prawns.
2.3 Experimental design and feeding management
The experiment was divided into control group (CT) without adding polysaccharide, B.coagulans group (N), B.coagulans fermentation with Atractylodes polysaccharide group (NB), B.coagulans fermentation with Paratractylodes polysaccharide group (NF), B. coagulans fermentation with mannose group (NG), B.coagulans fermentation with Astragalus polysaccharide group (NH) and B.coagulans fermentation with Yulingfeng polysaccharide group (NP). A single factor completely randomized group design was used. A total of 1260 prawns were selected with an initial weight of 0.1-0.2 g and randomly divided into seven groups with three replicates per group (60 prawns per replicate) in a total of 21 circular fiberglass tanks (φ1.5 m, 800 L water per tank). After two weeks of temporary rearing, the prawn seedling was fed with the experimental diet thrice daily. The feeding situation was observed and feces and residual feed were sucked out. Ammonia nitrogen, dissolved oxygen, pH and nitrite content in water were measured regularly and cultured for 8 weeks. All procedures used in this experiment have been approved by the Institutional Animal Care and Use Committee of Southwest University of Science and Technology to ensure the legality and ethics of the experiment.
2.4 Sample collection
At the end of the culture experiment, the total weight of the prawns in each tank was weighed and the total number was counted. Then the hemolymph was extracted from the cardiac chamber and centrifuged (4°C, 4000 r/min, 10 min), and the supernatant was aspirated and placed in a -20°C refrigerator. Alsever’s solution was used as the anticoagulant (Taking 13.2 g of trisodium citrate solution, 4.8 g of citric acid, 14.7 g of glucose and fixing the volume to 1 L of double-distilled water) in a ratio of 1:1 with the hemolymph. At the same time, 12 prawns were collected from each group and loaded into 4 frozen storage tubes. Then, all the intestines from each group were loaded into two frozen storage tubes for subsequent indices measurement.
2.5 Challenge test
At the end of the culture experiment, 30 prawns were randomly selected from each group. Aeromonas hydrophila NJ-35 was activated with LB medium (glucose 15.80g/L, peptone 15.90 g/L, yeast powder 11 g/L, MgSO4 4.60 g/L), and the concentration of bacteria solution was adjusted to 1×107 CFU/mL for intramuscular injection in the experimental group. The mortality of M.nipponense was observed within 12h, 24h, 36h, 48h, 72h and 96h, and the survival rate was calculated.
2.6 Determination of growth indicators
Growth indicators were calculated using the following methods (Zhou et al., 2022):
2.7 Determination of serum biochemical indicators
Serum total cholesterol (TC), aspartate aminotransferase (AST), alanine aminotransferase (ALT), albumin (ALB) and triglyceride (TG) were measured by Mindrai automatic analyzer (BS-400). Serum total protein TP was measured by the Coomassie brilliant blue method.
2.8 Determination of antioxidant indexes
Hepatopancreas total protein (TP), malondialdehyde (MDA), superoxide dismutase (SOD), acid phosphatase (ACP), alkaline phosphatase, total antioxidant capacity (T-AOC) and superoxide anion scavenging capacity (SASC) were measured using kits from Nanjing Jiancheng Bioengineering Institute. The hepatopancreas α-amylase and lipase activities were measured using kits from Beijing Solaibao Biotechnology Co., Ltd.
2.9 Gene expression assays
The expression levels of Toll, Dorsal, Relish, IMD, Myd88 and β-actin were determined by fluorescence quantitative PCR method. All primers were synthesized by Shanghai Jierei Bioengineering Co., Ltd. The primer sequences in Table 3 are from the reference (Liu et al., 2022). Sample RNA was extracted using Trizol method, total DNA concentration and OD value were determined by NanoDrop 2000, and the concentration was adjusted to 500 ng/μL. The Novizan HiScript II Q RT SuperMix for qPCR(+gDNA wiper) kit was used, and the experimental procedures were reverse transcribed into cDNA according to the instructions for use, and the gene expression level was detected by real-time fluorescence quantitative analyzer from the reference (Liu et al., 2022).
2.10 Data analysis
Data were expressed as “mean ± standard error” and analyzed by one-way analysis of variance (ANOVA) and Duncan’s multiple comparisons using SPSS 26.0 statistical software. The significance level was P < 0.05 and indicated by different lowercase letters superscript.
3 Results
3.1 Effect of A.hydrophila on the survival rate of M.nipponense with polysaccharide fermentation with B.coagulans
The experimental design is shown in Figure 1A. Overall, M.nipponense mortality increased with infection time and the survival rate of the treatment group was higher than that of the CT (Figure 1B).The survival rates of M. nipponense at 96 h were 13.33-66.67% in the different groups (Figure 1B). In addition, the survival rate of NF group was 66.67% at 96 h, which was significantly higher than that of CT (P < 0.05, Figure 1C). These results indicated that NF group could effectively inhibit the toxic effect of A.hydrophila on M.nipponense.
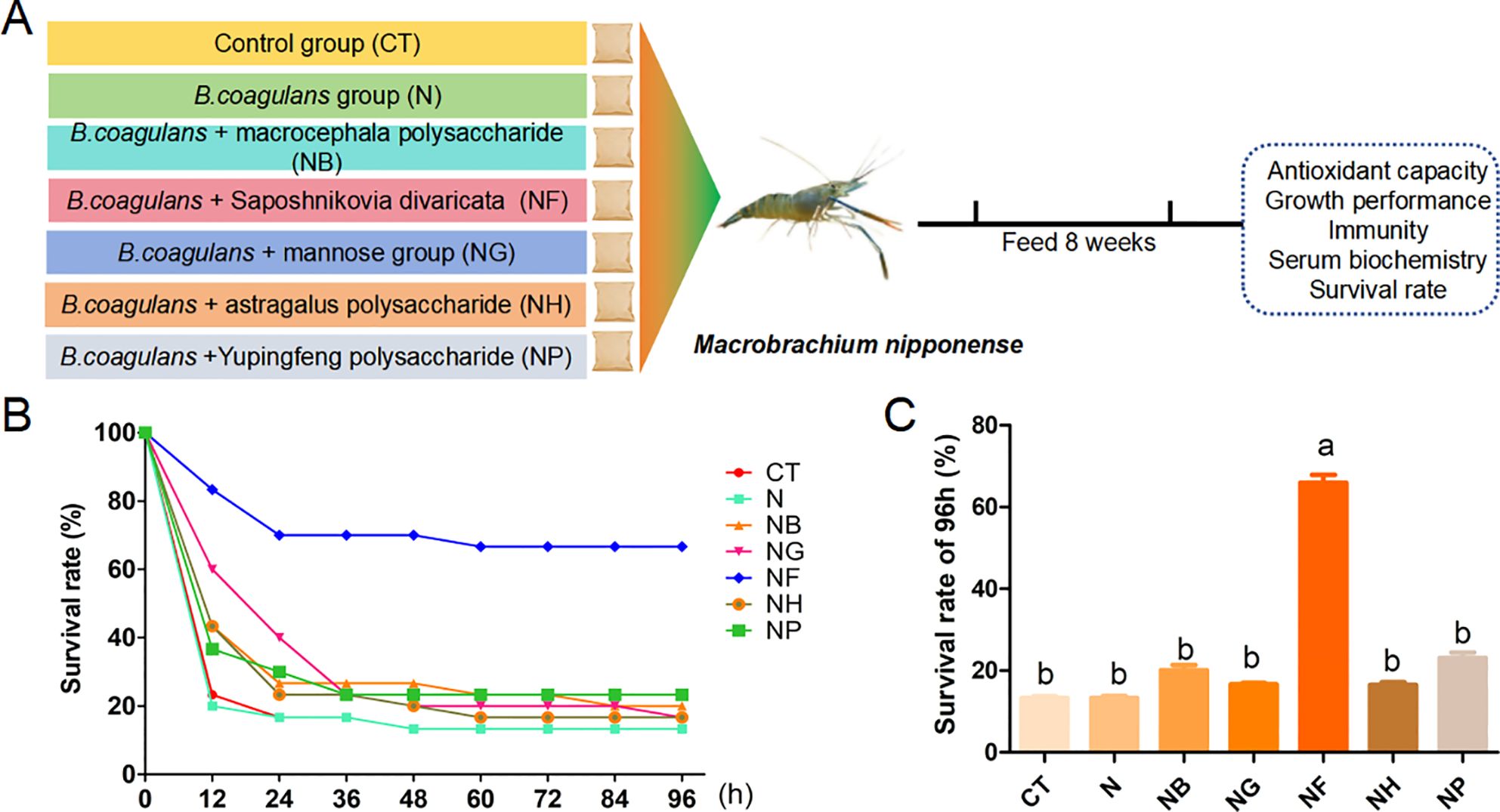
Figure 1. Changes in survival rate of A.hydrophila to M.nipponense. (A) Experimental design; (B) Change in survival rate of M.nipponense within 96 h; (C) Survival rate of M.nipponense. Means with different lowercase are significantly different (P < 0.05, Duncan's multiple comparisons).
3.2 Effects of polysaccharides fermentation with B.coagulans on growth performance of M.nipponense
Table 4 shows the effect of polysaccharide fermentation with B.coagulans on the growth performance of M.nipponense. Compared with the CT, the survival rate of the polysaccharide groups except mannose showed an upward trend, but the difference between the groups was not significant (P > 0.05).The weight gain rate of NB group was higher than that of the N (P < 0.05), but for the other groups there was no significant difference compared to the control (P > 0.05).
3.3 Effect of polysaccharides fermentation with B.coagulans on serum biochemistry of M.nipponense
Figure 2 shows the effects of polysaccharides and B.coagulans added to the diet on serum biochemistry of M.nipponense. The experimental design is shown in Figure 2A. Compared with the control CT and N, ALB, TC and TG in NH and NP group were significantly increased (P < 0.05) (Figures 2B, E, F).
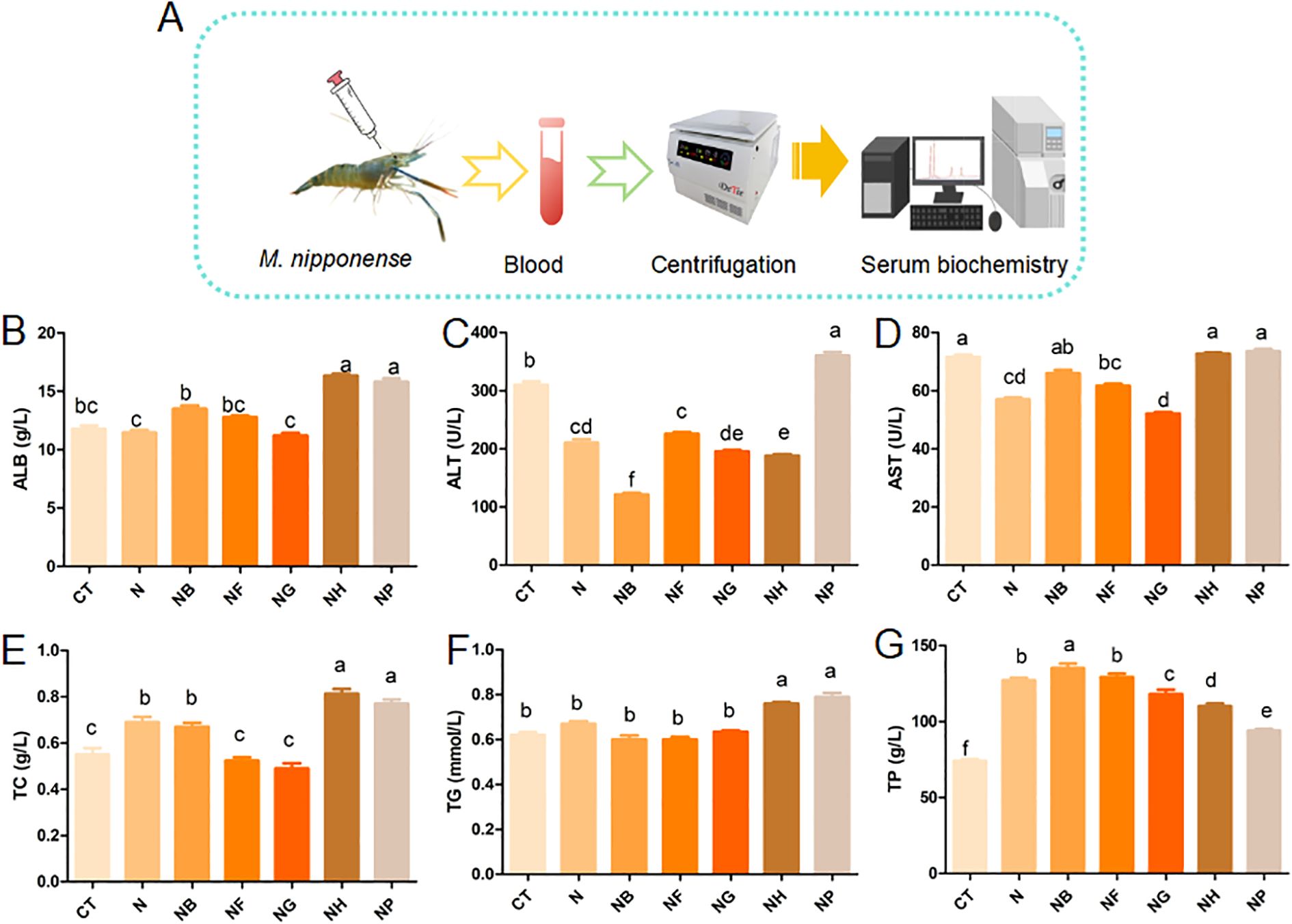
Figure 2. Effect of polysaccharide fermentation on serum biochemistry of M.nipponense (A) Experimental design; (B) ALB content; (C) ALT content; (D) AST content; (E) TC content; (F) TG content; (G) TP content. Means with different lowercase are significantly different (P < 0.05, Duncan's multiple comparisons).
ALT in NB, NG and NH were significantly lower than those of the CT and N (P < 0.05) (Figure 2C). TP in NB was significantly higher than that of the CT (P < 0.05) (Figure 2G). AST in the NG group was significantly decreased compared with the control (P < 0.05) (Figure 2D). Figure 2 showed that the group of N, NF and NG significantly reduced the content of AST and ALT compared to the CT (P < 0.05).
3.4 Effect of polysaccharide fermentation with B.coagulans on digestive enzymes of M.nipponense
Hepatopancreas lipase levels were significantly different between the group of N and NG or NB compared with the CT (P < 0.05) (Figure 3A). Hepatopancreatic α-amylase was significantly higher in the group of N, NG, NB, NF and NH than in the CT (P < 0.05) except NP group (Figure 3B).
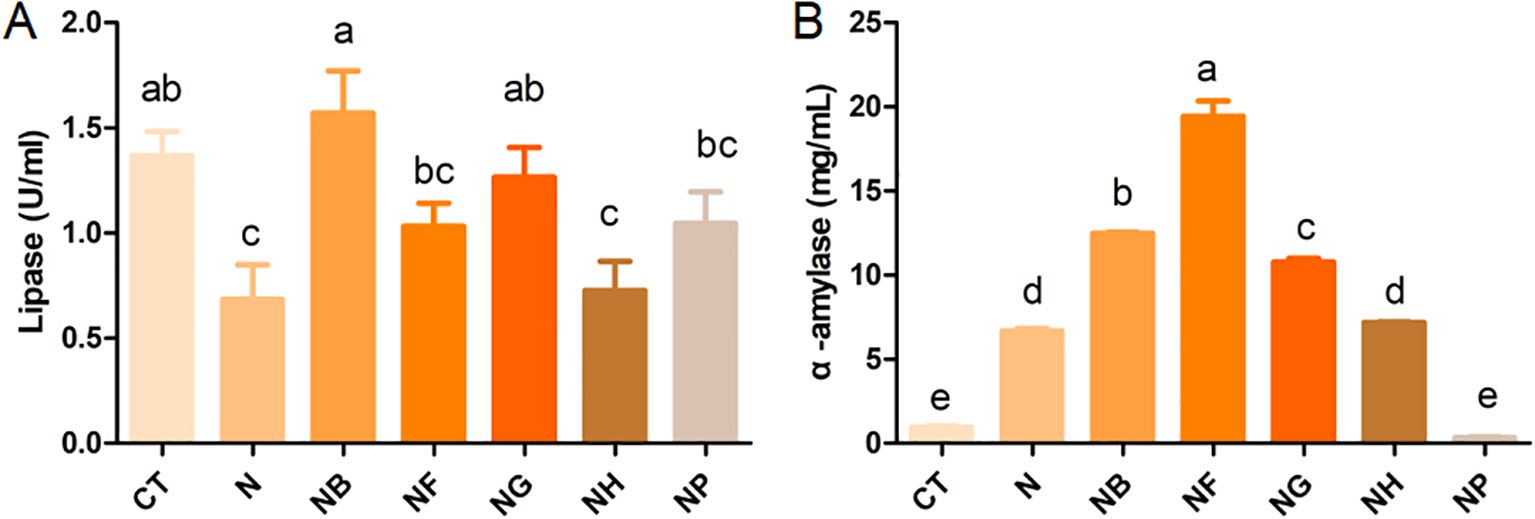
Figure 3. Effect of polysaccharide fermentation on digestive enzymes of M.nipponense (A) Lipase content; (B) α-amylase content. Means with different lowercase are significantly different (P < 0.05, Duncan's multiple comparisons).
3.5 Effect of polysaccharides fermentation with B.coagulans on antioxidant capacity of M.nipponense
When polysaccharides and B.coagulans were added to the feed, the hepatopancreatic enzyme activities of M.nipponense are shown in Table 5. Compared with the group of CT and N, the TP in NG, NB and NP groups significantly increased (P < 0.05) and the SOD in NP group significantly decreased (P < 0.05). NF group reduced the MDA compared to the N group (P < 0.05). ACP in the group of NB and NP, T-AOC and SASC in NP group were significantly increased compared with the CT and N group (P < 0.05), but T-AOC and SASC were significantly decreased in NB and NF group (P < 0.05).
3.6 Effect of polysaccharides fermentation with B.coagulans on immune gene expression of M.nipponense
The expression levels of Toll, Myd88, Dorsal, IMD and Relish genes are shown in Figure 4. The expression level of Toll, Myd88, Relish and Dorsal in NH group was significantly increased compared with the control group (P < 0.05) (Figures 4A–C, E). Compared with the control group, the expression level of Toll in NP was significantly increased and the expression level of Toll in NG was reduced considerably (P < 0.05) (Figure 4B). The expression of Myd88 and Dorsal in NB, NG and NF group were significantly decreased compared with the CT (P < 0.05) (Figures 4A, C).
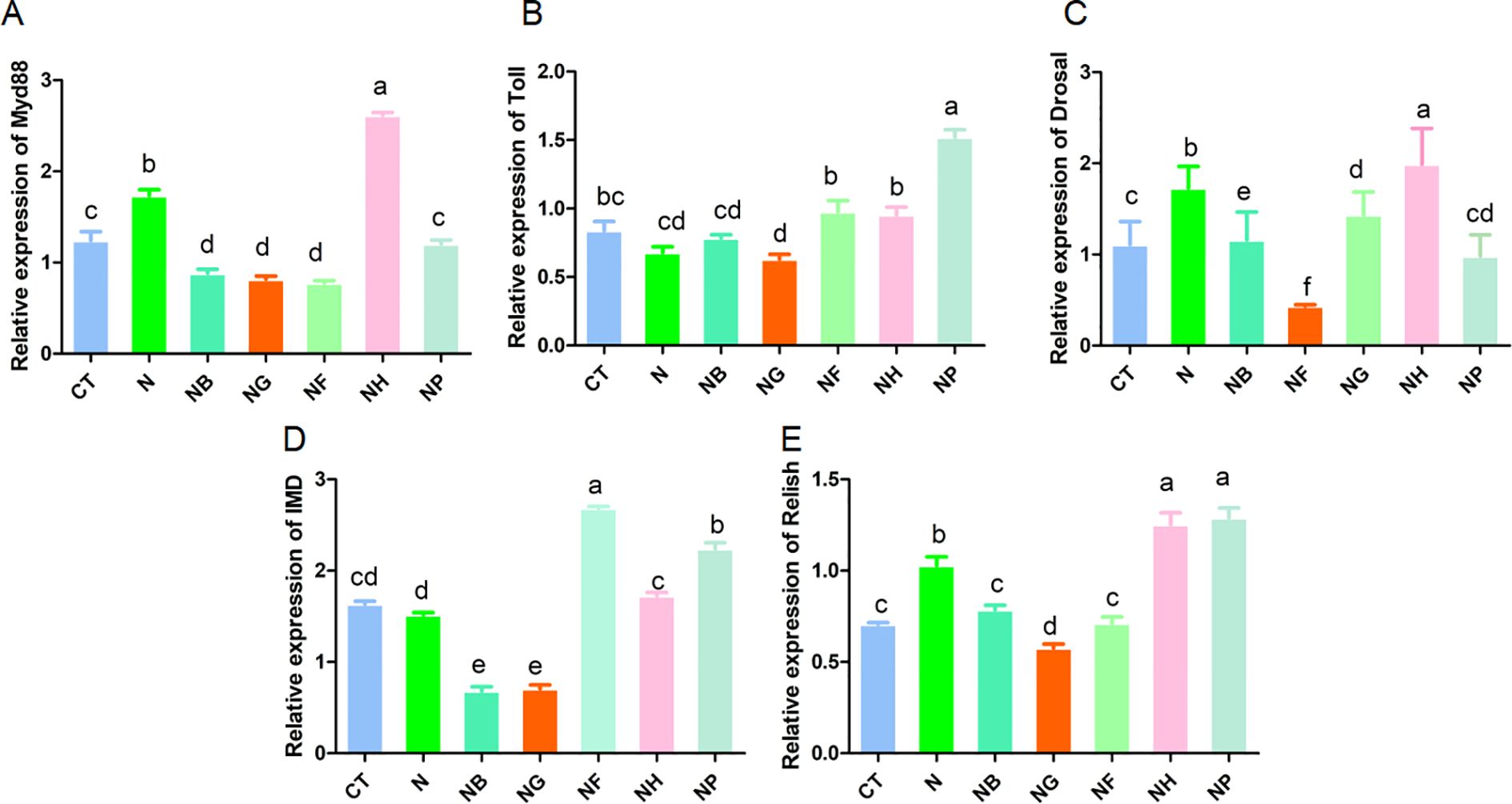
Figure 4. Effect of polysaccharide fermentation on gene expression in M.nipponense (A) Myd88 expression; (B) Toll expression; (C) Dorsal expression; (D) IMD expression; (E) Relish expression. Means with different lowercase are significantly different (P < 0.05, Duncan's multiple comparisons).
The expression of IMD and Relish in NF and NP group increased significantly (P < 0.05) (Figures 4D, E), the expression of IMD in NB and NG group decreased significantly (P < 0.05) (Figure 4D) and the expression of Relish in NG group decreased significantly (P < 0.05) (Figure 4E).
4 Discussion
Polysaccharides in aquaculture have attracted considerable attention and commercial interest over the past few decades. A continual search for novel, eco-friendly additives remains essential. Therefore, prebiotic polysaccharides have become a hotspot in research. Prebiotics, which contain polysaccharides and other substances, can promote animal health Plant polysaccharides have been widely studied for their various biological activities. These include antiviral activity, antioxidant activity, anti-fatigue and liver-protective effects (Liu et al., 2015). Five plant polysaccharides (Atractylodes polysaccharide, Paraspinatus polysaccharide, Mannose, Astragalus polysaccharide and Yulingfeng polysaccharide) can improve growth performance, antioxidant capacity and immune function in animals (Wu, 2020; Su et al., 2020; Cui et al., 2023; Erdenebileg et al., 2023). In addition, B.coagulans can promote animal growth, improve antioxidant capacity, enhance immunity and improve intestinal microbial flora (Zhang et al., 2021). Meanwhile, B.coagulans can break down plant polysaccharides and promote their uptake in animals (Shinde et al., 2020). However, there are few studies on the use of plant polysaccharides in combination with B.coagulans. Therefore, the effect of polysaccharide fermentation with B.coagulans for M.nipponense was investigated in this experiment.
It was found that the survival rate of the group fed with the polysaccharide B.coagulans was the highest. This may be due to the anti-inflammatory, antibacterial and immune-enhancing effects of paraspinals (Javadi and Sahebkar, 2017). Meanwhile, Paraspinatus polysaccharides also possessed antioxidant and anti-allergic properties (Guang et al., 2023). In addition, B.coagulans was found to fully restore the immunosuppression induced by cyclophosphamide, thereby improving its immunity (Bomko et al., 2017), which may also be an essential reason for the improved survival rate. Therefore, it was shown that feeding M.nipponense with Paraspinatus polysaccharide fermented by B.coagulans could improve the survival rate of A.hydrophila infection.
B.coagulans can produce enzymes such as protease, lipase and amylase (Gupta et al., 2016), which promote the absorption of nutrients in the intestine of animals. Studies have found that feeding compound bacterial agents such as Bacillus subtilis for Pengze crucian carassius improved the activities of protease, amylase and lipase (Luo et al., 2021). In addition, studies on Clostridium butyricum and B.coagulans have found that they can improve the gut microbiota structure and promote the growth of steelhead trout (Fan et al., 2019). The results of this study showed that the hepatopancreatic digestive enzyme activities of M.nipponense were increased after Atractylodes polysaccharide, mannose and Paratractylodes polysaccharide, which was consistent with the results of previous studies. Therefore, adding polysaccharides of Atractylodes japonicum, Paraspinatus, Astragalus and Yupingfeng to in the diet can improve the activities of non-digestible enzymes of M.nipponense. This result is mainly caused by B.coagulans, but there are few studies on the effect of the polysaccharides (Atractylodes rhizoma polysaccharide, Paraspinatus polysaccharide, Astragalus polysaccharide, Yupingfeng polysaccharide) on digestive enzymes. The ability was limited to the study of the effects of polysaccharides of Atractylodes rhizoma, Paratractylodes and Astragalus on digestive enzymes of M.nipponense. Therefore, the molecular mechanism underlying the effect of polysaccharides on the digestive enzymes of M.nipponense could not be determined.
Serum biochemistry reflects homeostatic changes in the body’s internal environment. ALT is mainly distributed in hepatocyte plasma and AST is primarily present in hepatocyte mitochondria and plasma. Hepatocyte necrosis causes ALT and AST to enter the blood circulation, thereby increasing the levels of ALT and AST in the body (Iweala et al., 2019). Oxidative stress in the liver can cause lipid peroxidation, thereby changing the permeability of the liver cell membrane and increasing ALP levels in vivo (Albasher et al., 2019). At the same time, liver injury can cause the rapid diffusion of fatty acids in the liver, resulting in the increase of TG and TC content in the liver. TG and TC also directly reflect the degree of lipid peroxidation in the liver (Wang et al., 2019b). Reduced liver function can further impair renal function, affecting the synthesis, transport, and release of ALB in the body, thereby reducing the level of ALB in the blood (Dongzhe et al., 2023). In the present study, the N, NF, NG, NH and NB significantly reduced the content of ALT. However, the N, NF and NG significantly reduced AST content. It has been previously found that dietary supplementation of Bacillus coagulans significantly reduced serum ALT and AST levels induced by high cholesterol in rats (Aminlari et al., 2018). The elevation of ALT and AST induced by cadmium poisoning was alleviated in rats fed with symbiotics B.coagulans with Lactobacillus plantarum and inulin (Jafarpour et al., 2017). In other words, B.coagulans mixed with polysaccharide can reduce ALT and AST content and protect the liver. Mannose and Atractylodes polysaccharide can effectively protect the liver injury in mice by significantly decreasing the activities of AST and ALT in serum (Han et al., 2016). Dietary Astragalus polysaccharide supplementation can improve the growth performance, alleviate liver dysfunction, and reduce ALT and AST content in the liver of piglets (Wang et al., 2019a). In addition, relevant studies have shown that dietary Astragalus polysaccharide can significantly reduce the content of glucose, triglyceride, cholesterol and nitric oxide in fish serum (Wu et al., 2019). In addition, it was found that replacing fish meal with Atractylodes Macrocephala Polysaccharide decreased the albumin and globulin concentrations and increased the feed coefficient and total bile acid activity of catfish (Zhuo et al., 2022). In summary, this is consistent with the present study and confirms the authenticity of the data in this study.
Oxidative stress is a primary biochemical reaction of organisms to resist external environmental stimulation and adapt to the living environment. The oxidative defense system is the basis of resistance to external stimuli, which is a balanced system composed of antioxidant enzymes and oxidative stress products. SOD is an important antioxidant enzyme in the oxidative defense system. SASC and T-AOC reflect the body’s overall antioxidant capacity. MDA is critical for oxidative stress and demonstrates the degree of oxidative damage in the body. Oxidative stress can also lead to decreased immunity and damage the immune system. ACP and AKP, as immune enzyme indicators, reflect the body’s immune ability. In this study, it was found that diet made from the fermentation broth of B.coagulans with Yupingfeng polysaccharide significantly increased the levels of ACP, AKP, SASC and T-AOC in M.nipponense. These results suggested that the fermentation broth of B.coagulans with Yupingfeng polysaccharide could increase the antioxidant capacity and immune capacity of M.nipponense. This may be due to the improvement of the antioxidant capacity of M.nipponense by regulating serum immunity through the fermentation broth of B.coagulans of Yupingping polysaccharide. Previous studies have shown that Yupingfeng polysaccharide could down-regulate the mRNA expression levels of pro-inflammatory cytokines, NF-kB, TLR-4 and iNOs, enhancing the antioxidant capacity (Sun et al., 2017). At the same time, Yupingfeng polysaccharide could significantly increase the density of immune cells and the number of macrophages and reduce the levels of NO, TNF-α, IL-1β and IL-6. Adding 1.6 g/kg Yupingfeng polysaccharide to the diet of grass carp can improve the immunity of grass carp (Wang et al., 2016). The above results indicated that Paratricans polysaccharide had some immunomodulatory activity (Fan et al., 2023). In addition, it was found that the fermentation broth of Atractylodes rhizoma polysaccharide increased the contents of ACP and AKP in M.nipponense. Atractylodes polysaccharide and Atractylodes lactone have been found to have anti-inflammatory and immunomodulatory effects in previous studies (Gu et al., 2019; Tang et al., 2017). However, the defect of this study is that the cause of the high MDA in the fermentation broth of B.coagulans with polysaccharide was not explored.
Invertebrates rely on innate immunity to defend against disease invading their bodies (Reboul and Ewbank, 2016) and shrimp are crustaceans and rely mainly on innate immunity (Patnaik et al., 2023). These include physical defense, cellular immunity, and humoral immunity (Riera Romo et al., 2016; Thaiss et al., 2016). In the model organism Drosophila antimicrobial peptide gene pathway, Toll and IMD play an important role in mediating G- and G+ infections, respectively (Huang et al., 2009) and shrimp immunity (Su et al., 2020). Toll receptor, Myd88 and Dorsal are key signaling molecules in the Toll pathway (Dong et al., 2022) and Myd88 is a receptor protein that connects Toll (Arts et al., 2007). Relish, a downstream transcription factor of the IMD pathway, belongs to the NF-kB family and participates in the body’s humoral immune response (Luo et al., 2022). IMD immunodeficiency is involved in antimicrobial responses in vivo. Previous studies found that Yupingfeng polysaccharide could enhance serum immunity and Toll gene expression in Litopenia vannamen (Su et al., 2020). At the same time, researchers showed that Yupingfeng could regulate the release of cytokines in mouse macrophages, mainly due to the enhanced degradation of IkBα, which in turn activated NF-kB, causing pro-inflammatory cytokine-related protein and mRNA expression (Fabrizio et al., 2013). In addition, Yupingfeng also inhibited the expression of pro-inflammatory cytokines (Fabrizio et al., 2013). The expression levels of Toll, IMD and Relish in the hepatopancreas of Litopenaeus vannaeus can be significantly increased by adding Marine Rhodotorula to the diet (Jin et al., 2022). Increased expression of Relish was observed in the hepatopancreas after artificial infection with A. hydrophila (Liang, 2022). In addition, Yupingfeng polysaccharide was found to increase the expression of Toll receptors in the serum of Litopenia vannamen and promote the body health (Su et al., 2020). Astragaloside has also been found to exert anti-inflammatory effects through the Myd88/NF-kB pathway (Shi et al., 2021). Astragalus polysaccharides can improve juvenile crucian carp’s growth performance and innate immunity (Wu, 2020). This is consistent with the results of the present study, which showed that the addition of Astragalus and Yupingfeng polysaccharides to the diet of M. nipponense increased the expression of Myd88 and Toll, respectively, and thus increased the expression of related immune genes in M.nipponense.
In general, these fermented polysaccharides’ primary mechanisms of action involve absorption in the intestines, entry into the bloodstream, and effects on various tissues and organs throughout the body. Specifically, Yupingfeng polysaccharides enhance liver function and the liver’s antioxidant capacity by increasing the serum levels of TP, ALB, AKP and T-AOC Saposhnikovia divaricata polysaccharides can increase the content of TP in the serum, improve liver function, and regulate the liver’s antioxidant function by adjusting the content of AKP and TP. Additionally, Saposhnikovia divaricata polysaccharides can increase the content of alpha-amylase content, enhancing metabolic function. Astragalus polysaccharides can reduce the content of ALT in the serum and increase the content of TP, thereby enhancing liver function. Moreover, Astragalus polysaccharides enhance the body’s antioxidant function by increasing the content of T-AOC, TP, and AKP, thereby enhancing the body’s immune capacity (Figure 5). In summary, among the five types of fermented polysaccharides screened, Yupingfeng polysaccharides, Saposhnikovia divaricata polysaccharides and Astragalus polysaccharides have a significant effect on improving the antioxidant capacity and immunity of M.nipponense.
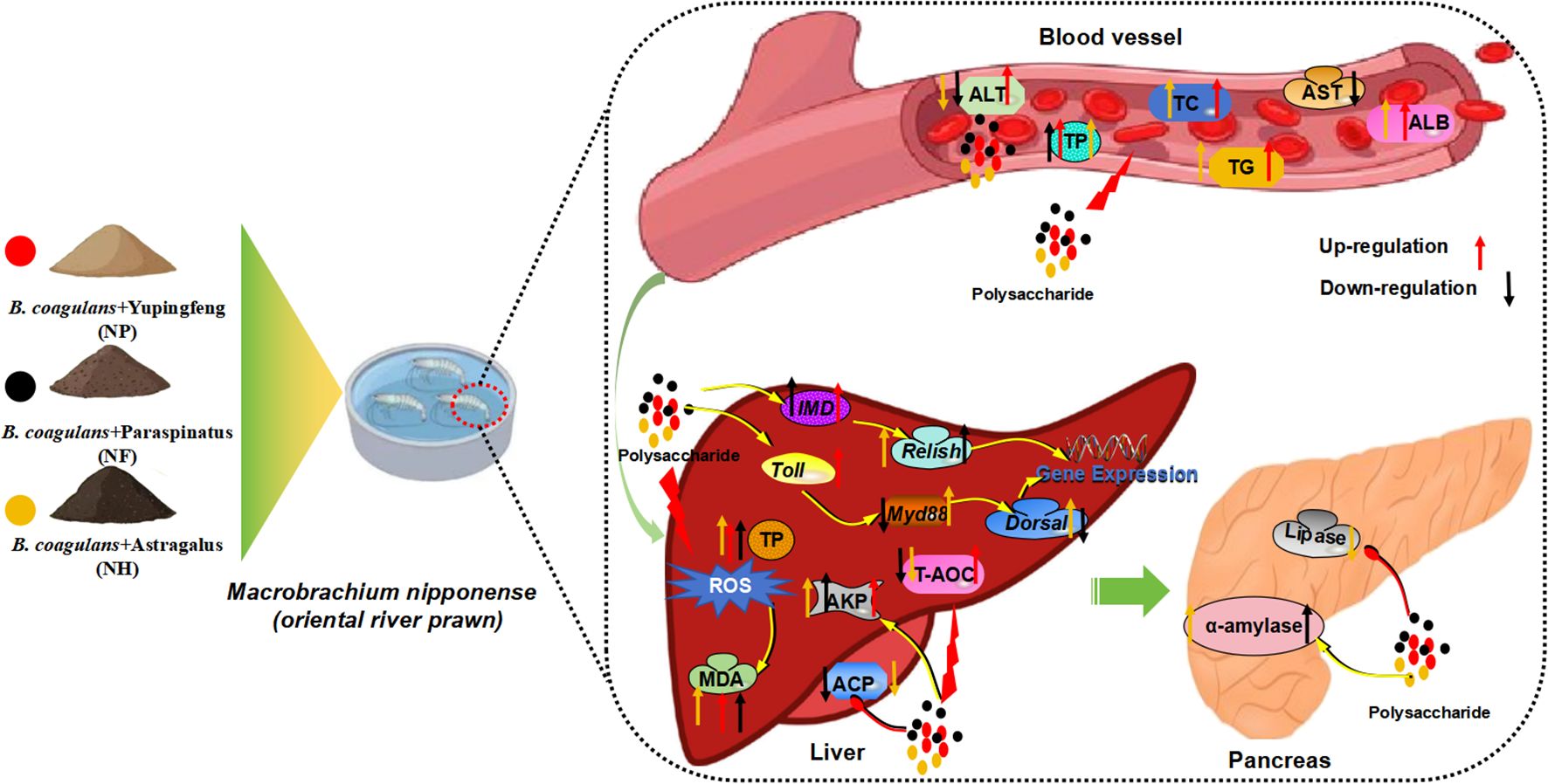
Figure 5. The regulatory mechanisms of Yupingfeng polysaccharide, Saposhnikovia divaricata polysaccharides and Astragalus polysaccharide for M. nipponense. Toll, Toll-like receptor; Myd88, Myeloid differentiation primary response gene 88; Dorsal, Dorsal-related immunity factor; IMD, Immunodeficiency; Relish, Relish protein; TP, Total protein; MDA, Malondialdehyde; ACP, Acid phosphatase; AKP, Alkaline phosphatase; T-AOC, Total antioxidant capacity.
5 Conclusion
M.nipponense was co-influenced by polysaccharides and B.coagulans and the adding of fermented polysaccharides to the diet increased the weight gain of the prawns. The combined use of polysaccharide fermentation with B.coagulans can improve the survival rate of M.nipponense infected with A.hydrophila; the survival rate of the group with B.coagulans and Saposhnikovia divaricata polysaccharides (NF) was 66.67% at 96 hours. Adding polysaccharides fermented with Bacillus coagulans to the diet significantly impacted the prawns’ immune capacity and digestive enzyme activity. The addition of fermented Astragalus and Yupingfeng polysaccharides with B. coagulans to the diet increased the expression of Myd88 and Toll, respectively. The addition of fermented Atractylodes macrocephala polysaccharides with Saposhnikovia divaricata polysaccharides and Mannose, as well as Yupingfeng polysaccharides with B.coagulans, significantly reduced the expression of Relish and promoted the growth of the M.nipponense.
Data availability statement
The raw data supporting the conclusions of this article will be made available by the authors, without undue reservation.
Author contributions
YW: Conceptualization, Investigation, Writing – original draft. YL: Methodology, Project administration, Writing – original draft, Writing – review & editing. JY: Data curation, Methodology, Writing – original draft. ZL: Funding acquisition, Methodology, Writing – review & editing. WW: Conceptualization, Writing – original draft. LJ: Methodology, Supervision, Writing – review & editing. BL: Formal analysis, Funding acquisition, Writing – original draft.
Funding
The author(s) declare financial support was received for the research, authorship, and/or publication of this article. This work was supported by Jiangsu Province Agricultural Science and Technology Independent Innovation Fund (CX(23)2008), the earmarked fund for Jiangsu Agricultural Industry Technology System (JATS (2023)470); the Central Public-Interest Scientific Institution Basal Research Fund, CAFS (2022XT04, 2023TD63); China Agriculture Research System of MOF and MARA (CARS-48) and the “333 High Level Talent Project in Key Industry” of Jiangsu Province. The authors would like to thank the staff for their assistance during experiments at the Key Laboratory of Aquatic Animal Nutrition and Health, Freshwater Fisheries Research Center, Chinese Academy of Fishery Science.
Conflict of interest
The authors declare that the research was conducted in the absence of any commercial or financial relationships that could be construed as a potential conflict of interest.
Generative AI statement
The author(s) declare that no Generative AI was used in the creation of this manuscript.
Publisher’s note
All claims expressed in this article are solely those of the authors and do not necessarily represent those of their affiliated organizations, or those of the publisher, the editors and the reviewers. Any product that may be evaluated in this article, or claim that may be made by its manufacturer, is not guaranteed or endorsed by the publisher.
References
Ai F., Huang X., Wu Y., Ji C., Gao Y., Yu T., et al. (2023). Alleviative effects of a novel strain Bacillus coagulans XY2 on copper-induced toxicity in zebrafish larvae. J. Environ. Sci. 125, 750–760. doi: 10.1016/j.jes.2022.02.010
Albasher G., Almeer R., Al-Otibi F. O., Al-Kubaisi N., Mahmoud A. M. (2019). Ameliorative Effect of Beta vulgaris Root Extract on Chlorpyrifos-Induced oxidative stress, Inflammation and Liver Injury in Rats. Biomolecules 9, 261–278. doi: 10.3390/biom9070261
Aminlari L., Shekarforoush S. S., Hosseinzadeh S., Nazifi S., Sajedianfard J., Eskandari M. H. (2018). Effect of Probiotics Bacillus coagulans and Lactobacillus plantarum on Lipid Profile and Feces Bacteria of Rats Fed Cholesterol-Enriched Diet. Probiotics Antimicrob. Proteins. 11, 1163–1171. doi: 10.1007/s12602-018-9480-1
Arts J. A. J., Cornelissen F. H. J., Cijsouw T., Hermsen T., Savelkoul H. F. J., Stet R. J. M. (2007). Molecular cloning and expression of a Toll receptor in the giant tiger shrimp, Penaeus monodon. Fish Shellfish Immun. 23, 504–513. doi: 10.1016/j.fsi.2006.08.018
Bomko T. V., Nosalskaya T. N., Kabluchko T. V., Lisnyak Y. V., Martynov A. V. (2017). Immunotropic aspect of the Bacillus coagulans probiotic action. J. Pharm. Pharmacol. 69, 1033–1040. doi: 10.1111/jphp.12726
Bulfon C., Volpatti D., Galeotti M. (2015). Current research on the use of plant-derived products in farmed fish. Aquac. Res. 46, 513–551. doi: 10.1111/are.12238
Cui Y., Zhang X., Jiao L., Tao X., Li M., Lu J., et al. (2023). The regulation effect of Atractylodis macrocephalae polysaccharides on the growth performance, antioxidant capacity and immune function in Litopenaeus vannamei. Aquac. Rep. 31, 101641. doi: 10.1016/j.aqrep.2023.101641
Dong B., Wu L., Chen Q., Xu W., Li D., Han D., et al. (2022). Tolerance Assessment of Atractylodes macrocephala Polysaccharide in the Diet of Largemouth Bass (Micropterus salmoides). Antioxidants. 11, 1581–1592. doi: 10.3390/antiox11081581
Dongzhe C., Xianjie G., Liang Z., Guangjun H. (2023). Retracted: serum TGF-β1 and VEGF levels reflect the liver hardness and function in children with biliary atresia. Comput. Math Method M. 2023, 1–1. doi: 10.1155/2023/9797525
Erdenebileg S., Son Y.-J., Kim M., Oidovsambuu S., Cha K. H., Kwon J., et al. (2023). Saposhnikovia divaricata root and its major components ameliorate inflammation and altered gut microbial diversity and compositions in dextran sodium sulfate-induced colitis. Integr. Med. Res. 12, 100998. doi: 10.1016/j.imr.2023.100998
Fabrizio M., Du C. Y. Q., Choi R. C. Y., Zheng K. Y. Z., Dong T. T. X., Lau D. T. W., et al. (2013). Yu ping feng san, an ancient Chinese herbal decoction containing astragali radix, atractylodis macrocephalae rhizoma and saposhnikoviae radix, regulates the release of cytokines in murine macrophages. PloS One 8, e78622. doi: 10.1371/journal.pone.0078622
Fan H., Sun M., Li J., Zhang S., Tu G., Liu K., et al. (2023). Structure characterization and immunomodulatory activity of a polysaccharide from Saposhnikoviae Radix. Int. J. Biol. Macromol. 233, 123502. doi: 10.1016/j.ijbiomac.2023.123502
Fan Y., Ye H., Wang X., Li L., Wang Y., Diao J. (2019). Effects of Clostridium butyricum and Bacillus coagulans on growth performance, hepatic function and intestinal microbiota in rainbow trout Oncorhynchus mykiss. J. Dalian Ocean Univ. 34, 198–203. doi: 10.16535/j.cnki.dlhyxb.2019.02.007
Forsatkar M. N., Nematollahi M. A., Rafiee G., Farahmand H., Lawrence C. (2018). Effects of the prebiotic mannan-oligosaccharide on feed deprived zebrafish: Growth and reproduction. Aquac. Res. 49, 2822–2832. doi: 10.1111/are.13745
Fu R., Chen D., Tian G., Zheng P., Mao X., Yu J., et al. (2019). Effect of dietary supplementation of Bacillus coagulans or yeast hydrolysates on growth performance, antioxidant activity, cytokines and intestinal microflora of growing-finishing pigs. Anim. Nutr. 5, 366–372. doi: 10.1016/j.aninu.2019.06.003
Gu S., Li L., Huang H., Wang B., Zhang T. (2019). Antitumor, antiviral, and anti-inflammatory efficacy of essential oils from atractylodes macrocephala koidz. Produced with different processing methods. Molecules. 24, 2956–2970. doi: 10.3390/molecules24162956
Guang X., Ming Q., Mengqi Y., Tian L., Yuying G., Kaihe W., et al. (2023). Structural characterization of a polysaccharide derived from Saposhnikovia divaricatee (Turcz.) Schischk with anti-allergic and antioxidant activities. J. Ethnopharmacol. 19, 117–125. doi: 10.1016/j.jep.2023.117425
Gupta A., Verma G., Gupta P. (2016). Growth performance, feed utilization, digestive enzyme activity, innate immunity and protection against Vibrio harveyi of freshwater prawn, Macrobrachium rosenbergii fed diets supplemented with Bacillus coagulans. Aquacult Int. 24, 1379–1392. doi: 10.1007/s10499-016-9996-x
Han B., Gao Y., Wang Y., Wang L., Shang Z., Wang S., et al. (2016). Protective effect of a polysaccharide from Rhizoma Atractylodis Macrocephalae on acute liver injury in mice. Int. J. Biol. Macromol. 87, 85–91. doi: 10.1016/j.ijbiomac.2016.01.086
Hou D., Huang Z., Zeng S., Liu J., Wei D., Deng X., et al. (2018). Intestinal bacterial signatures of white feces syndrome in shrimp. Appl. Microbiol. Biot. 102, 3701–3709. doi: 10.1007/s00253-018-8855-2
Huang X.-D., Yin Z.-X., Liao J.-X., Wang P.-H., Yang L.-S., Ai H.-S., et al. (2009). Identification and functional study of a shrimp Relish homologue. Fish Shellfish Immunol. 27, 230–238. doi: 10.1016/j.fsi.2009.05.003
Huang Z., Ye Y., Xu A., Li Z., Du Z.-Y. (2023). Effects of Astragalus membranaceus Polysaccharides on Growth Performance, Physiological and Biochemical Parameters, and Expression of Genes Related to Lipid Metabolism of Spotted Sea Bass, Lateolabrax maculatus. Aquacult Nutr. 2023, 1–18. doi: 10.1155/2023/6191330
Iweala E. E. J., Evbakhavbokun W. O., Maduagwu E. N. (2019). Antioxidant and hepatoprotective effect of cajanus cajan in N-nitrosodiethylamine-induced liver damage. Sci. Pharm. 87, 24–33. doi: 10.3390/scipharm87030024
Jafarpour D., Shekarforoush S. S., Ghaisari H. R., Nazifi S., Sajedianfard J., Eskandari M. H. (2017). Protective effects of synbiotic diets of Bacillus coagulans, Lactobacillus plantarum and inulin against acute cadmium toxicity in rats. BMC Complem Altern. M. 17, 291. doi: 10.1186/s12906-017-1803-3
Javadi B., Sahebkar A. (2017). Natural products with anti-inflammatory and immunomodulatory activities against autoimmune myocarditis. Pharmacol. Res. 124, 34–42. doi: 10.1016/j.phrs.2017.07.022
Jenab A., Roghanian R., Emtiazi G. (2020). Bacterial natural compounds with anti-inflammatory and immunomodulatory properties (Mini review). Drug Des. Dev. Ther. 14, 3787–3801. doi: 10.2147/dddt.S261283
Jiang L., Feng J., Ying R., Yin F., Pei S., Lu J., et al. (2019). Individual and combined effects of ammonia-N and sulfide on the immune function and intestinal microbiota of Pacific white shrimp Litopenaeus vannamei. Fish Shellfish Immun. 92, 230–240. doi: 10.1016/j.fsi.2019.06.020
Jin P., Li H., Tian X., Wang B., Liulong T., He Y., et al. (2022). Effects of different addition forms of Rhodotorula mucilaginosa on growth, digestive enzyme activity and immune indexes of Litopenaeus vannamei. Trans. Oceanol. Limnol. 44, 18–26. doi: 10.13984/j.cnki.cn37-1141.2022.05.003
Joshi J., Srisala J., Truong V. H., Chen I. T., Nuangsaeng B., Suthienkul O., et al. (2014). Variation in Vibrio parahaemolyticus isolates from a single Thai shrimp farm experiencing an outbreak of acute hepatopancreatic necrosis disease (AHPND). Aquaculture. 428-429, 297–302. doi: 10.1016/j.aquaculture.2014.03.030
Karakan T., Tuohy K. M., Janssen-van Solingen G. (2021). Low-dose lactulose as a prebiotic for improved gut health and enhanced mineral absorption. Front. Nutr. 8. doi: 10.3389/fnut.2021.672925
Kiernan D. P., O’Doherty J. V., Sweeney T. (2023). The effect of prebiotic supplements on the gastrointestinal microbiota and associated health parameters in pigs. Animals. 13, 1–15. doi: 10.3390/ani13193012
Li R., Chen W.-c., Wang W.-p., Tian W.-y., Zhang X.-g. (2010). Antioxidant activity of Astragalus polysaccharides and antitumour activity of the polysaccharides and siRNA. Carbohydr. Polym. 82, 240–244. doi: 10.1016/j.carbpol.2010.02.048
Li P., Gatlin D. M. (2005). Evaluation of the prebiotic GroBiotic®-A and brewers yeast as dietary supplements for sub-adult hybrid striped bass (Morone chrysops×M. saxatilis) challenged in situ with Mycobacterium marinum. Aquaculture. 248, 197–205. doi: 10.1016/j.aquaculture.2005.03.005
Li B.-X., Li W.-Y., Tian Y.-B., Guo S.-X., Huang Y.-M., Xu D.-N., et al. (2019a). Polysaccharide of Atractylodes macrocephalaKoidz Enhances Cytokine Secretion by Stimulating theTLR4–MyD88–NF-κBSignaling Pathway in the Mouse Spleen. J. Med. Food. 22, 937–943. doi: 10.1089/jmf.2018.4393
Li K., Li S., Wang D., Li X., Wu X., Liu X., et al. (2019b). Extraction, characterization, antitumor and immunological activities of hemicellulose polysaccharide from astragalus radix herb residue. Molecules 24, 3644–3657. doi: 10.3390/molecules24203644
Liang F. (2022). Cloning, expression and transcriptome analysis of Keygenes in Toll and IMD Pathways of Fenneropenaeuspenicillatus under bacterial stress. J. BeiBu Gulf Univ. 41 (3), 1–10. doi: 10.44243/d.cnki.gbbgu.2022.000010
Liu X., Jiang S., Liu B., Zhou Q., Sun C., Zheng X., et al. (2022). Dietary effect of ferulic acid on growth performance, physiological response, non-specific immunity and disease resistance of oriental river prawn (Macrobrachium nipponense). Aquacult. Rep. 24, 1–8. doi: 10.1016/j.aqrep.2022.101162
Liu J., Willför S., Xu C. (2015). A review of bioactive plant polysaccharides: Biological activities, functionalization, and biomedical applications. Bioact. Carbohydr. Diet. Fibre. 5, 31–61. doi: 10.1016/j.bcdf.2014.12.001
Liu A.-j., Yu J., Ji H.-y., Zhang H.-c., Zhang Y., Liu H.-p. (2017). Extraction of a novel cold-water-soluble polysaccharide from astragalus membranaceus and its antitumor and immunological activities. Molecules 23, 62. doi: 10.3390/molecules23010062
Luo S., Jiang Y., Zhao L., Zhang H., Zhang X. (2021). Effects of different Bacillus sp.on growth performance, muscle nutrient composition, intestinal structure and digestive enzyme activities of Pengze. Feed China. 13, 73–79. doi: 10.15906/j.cnki.cn11-2975/s.20211315
Luo W., Lin K., Hua J., Han J., Zhang Q., Chen L., et al. (2022). Schisandrin B attenuates diabetic cardiomyopathy by targeting myD88 and inhibiting myD88-dependent inflammation. Adv. Sci. 9, e2202590. doi: 10.1002/advs.202202590
Ma Y., Liu C., Qu D., Chen Y., Huang M., Liu Y. (2017). Antibacterial evaluation of sliver nanoparticles synthesized by polysaccharides from Astragalus membranaceus roots. Biomed. Pharmacother. 89, 351–357. doi: 10.1016/j.biopha.2017.02.009
Mazkour S., Shekarforoush S. S., Basiri S., Namazi F., Zarei-Kordshouli F. (2022). Protective effects of oral administration of mixed probiotic spores of Bacillus subtilis and Bacillus coagulans on gut microbiota changes and intestinal and liver damage of rats infected with Salmonella Typhimurium. J. Food Saf. 42, 1–11. doi: 10.1111/jfs.12981
McLoughlin R. F., Berthon B. S., Jensen M. E., Baines K. J., Wood L. G. (2017). Short-chain fatty acids, prebiotics, synbiotics, and systemic inflammation: a systematic review and meta-analysis. Am. J. Clin. Nutr. 106, 930–945. doi: 10.3945/ajcn.117.156265
Munir M. B., Hashim R., Abdul Manaf M. S., Nor S. A. M. (2016). Dietary prebiotics and probiotics influence the growth performance, feed utilisation, and body indices of snakehead (Channa striata) fingerlings. Trop. Life Sci. Res. 27, 111–125. doi: 10.21315/tlsr2016.27.2.9
Pan S., Jiang L., Wu S. (2018). Stimulating effects of polysaccharide from Angelica sinensis on the nonspecific immunity of white shrimps (Litopenaeus vannamei). Fish Shellfish Immunol. 74, 170–174. doi: 10.1016/j.fsi.2017.12.067
Patnaik B. B., Baliarsingh S., Sarkar A., Hameed A. S. S., Lee Y. S., Jo Y. H., et al. (2023). The role of pattern recognition receptors in crustacean innate immunity. Rev. Aquacult. 16, 190–233. doi: 10.1111/raq.12829
Reboul J., Ewbank J. J. (2016). GPCRs in invertebrate innate immunity. Biochem. Pharmacol. 114, 82–87. doi: 10.1016/j.bcp.2016.05.015
Riera Romo M., Pérez-Martínez D., Castillo Ferrer C. (2016). Innate immunity in vertebrates: an overview. Immunology. 148, 125–139. doi: 10.1111/imm.12597
Sadat Hoseini Madani N., Adorian T. J., Ghafari Farsani H., Hoseinifar S. H. (2018). The effects of dietary probiotic Bacilli (Bacillus subtilis and Bacillus licheniformis) on growth performance, feed efficiency, body composition and immune parameters of whiteleg shrimp (Litopenaeus vannamei) postlarvae. Aquacult. Res. 49, 1926–1933. doi: 10.1111/are.13648
Shi H., Zhou P., Gao G., Liu P. p., Wang S. s., Song R., et al. (2021). Astragaloside IV prevents acute myocardial infarction by inhibiting the TLR4/MyD88/NF-κB signaling pathway. J. Food Biochem. 45, e13757–1–e13757–12. doi: 10.1111/jfbc.13757
Shinde T., Vemuri R., Shastri S., Perera A. P., Gondalia S. V., Beale D. J., et al. (2020). Modulating the microbiome and immune responses using whole plant fibre in synbiotic combination with fibre-digesting probiotic attenuates chronic colonic inflammation in spontaneous colitic mice model of IBD. Nutrients. 12, 2380–2395. doi: 10.3390/nu12082380
Sredkova P., Batsalova T., Moten D., Dzhambazov B. (2020). Prebiotics can change immunomodulatory properties of probiotics. Cent. Eur. J. Immunol. 45, 248–255. doi: 10.5114/ceji.2020.101237
Su C., Fan D., Pan L., Lu Y., Wang Y., Zhang M. (2020). Effects of Yu-Ping-Feng polysaccharides (YPS) on the immune response, intestinal microbiota, disease resistance and growth performance of Litopenaeus vannamei. Fish Shellfish Immunol. 105, 104–116. doi: 10.1016/j.fsi.2020.07.003
Sun Z., Li D., Wang H., Ding Y., Zhang C., Wang S., et al. (2023). Polysaccharide of Atractylodes macrocephala Koidz(PAMK) protects against DEHP-induced apoptosis in grass carp hepatocytes. Fish Shellfish Immunol. 134, 1–11. doi: 10.1016/j.fsi.2023.108587
Sun W., Meng K., Qi C., Yang X., Wang Y., Fan W., et al. (2015). Immune-enhancing activity of polysaccharides isolated from Atractylodis macrocephalae Koidz. Carbohydr. Polym. 126, 91–96. doi: 10.1016/j.carbpol.2015.03.034
Sun H., Ni X., Song X., Wen B., Zhou Y., Zou F., et al. (2016). Fermented Yupingfeng polysaccharides enhance immunity by improving the foregut microflora and intestinal barrier in weaning rex rabbits. Appl. Microbiol. Biotechnol. 100, 8105–8120. doi: 10.1007/s00253-016-7619-0
Sun H., Ni X., Zeng D., Zou F., Yang M., Peng Z., et al. (2017). Bidirectional immunomodulating activity of fermented polysaccharides from Yupingfeng. Res. Vet. Sci. 110, 22–28. doi: 10.1016/j.rvsc.2016.10.015
Tang X.-M., Liao Z.-K., Huang Y.-W., Lin X., Wu L.-C. (2017). Atractylenolide I protects against lipopolysaccharide-induced disseminated intravascular coagulation by anti-inflammatory and anticoagulation effect. Asian Pac. J. Trop. Med. 10, 582–587. doi: 10.1016/j.apjtm.2017.06.007
Thaiss C. A., Zmora N., Levy M., Elinav E. (2016). The microbiome and innate immunity. Nature. 535, 65–74. doi: 10.1038/nature18847
Tzianabos A. O. (2000). Polysaccharide immunomodulators as therapeutic agents: structural aspects and biologic function. Clin. Microbiol. Rev. 13, 523–533. doi: 10.1128/cmr.13.4.523
Wang Z., Liu M., Yu H., Yin F., Du Y., Tang H., et al. (2016). Effects of yupingfeng polysaccharides on growth performance, immune organ indexes and hepatopancreas non-specific immune indexes of grass carp (Ctenopharyngodon idellus). Chin. J. Anim. Nutr. 28, 3679–3686. doi: 10.3969/j.issn.1006-267x.2016.11.038
Wang R., Yang Z., Zhang J., Mu J., Zhou X., Zhao X. (2019b). Liver injury induced by carbon tetrachloride in mice is prevented by the antioxidant capacity of anji white tea polyphenols. Antioxidants 8, 64–76. doi: 10.3390/antiox8030064
Wang K., Zhang H., Han Q., Lan J., Chen G., Cao G., et al. (2019a). Effects of astragalus and ginseng polysaccharides on growth performance, immune function and intestinal barrier in weaned piglets challenged with lipopolysaccharide. J. Anim. Physiol. Anim. Nutr. 104, 1096–1105. doi: 10.1111/jpn.13244
Wei W., Xiao H.-T., Bao W.-R., Ma D.-L., Leung C.-H., Han X.-Q., et al. (2016). TLR-4 may mediate signaling pathways of Astragalus polysaccharide RAP induced cytokine expression of RAW264.7 cells. J. Ethnopharmacol. 179, 243–252. doi: 10.1016/j.jep.2015.12.060
Wu S. (2020). Dietary Astragalus membranaceus polysaccharide ameliorates the growth performance and innate immunity of juvenile crucian carp (Carassius auratus). Int. J. Biol. Macromol. 149, 877–881. doi: 10.1016/j.ijbiomac.2020.02.005
Wu F., Yang C., Wen H., Zhang C., Jiang M., Liu W., et al. (2019). Improving low-temperature stress tolerance of tilapia, Oreochromis niloticus: A functional analysis of Astragalus membranaceus. J. World Aquacult. Soc 50, 749–762. doi: 10.1111/jwas.12586
Xu D., Li W., Li B., Tian Y., Huang Y. (2017). The effect of selenium and polysaccharide of Atractylodes macrocephala Koidz. (PAMK) on endoplasmic reticulum stress and apoptosis in chicken spleen induced by heat stress. RSC Adv. 7, 7519–7525. doi: 10.1039/c6ra27730f
Xu D., Tian Y. (2015). Selenium and polysaccharides of atractylodes macrocephala koidz play different roles in improving the immune response induced by heat stress in chickens. Biol. Trace Elem. Res. 168, 235–241. doi: 10.1007/s12011-015-0351-2
Zhang B., Zhang H., Yu Y., Zhang R., Wu Y., Yue M., et al. (2021). Effects of Bacillus Coagulans on growth performance, antioxidant capacity, immunity function, and gut health in broilers. Poult. Sci. 100, 371–380. doi: 10.1016/j.psj.2021.101168
Zhou X., Wang Y., Yu J., Li J., Wu Q., Bao S., et al. (2022). Effects of dietary fermented Chinese herbal medicines on growth performance, digestive enzyme activity, liver antioxidant capacity, and intestinal inflammatory gene expression of juvenile largemouth bass (Micropterus salmoides). Aquacult. Rep. 25, 1–9. doi: 10.1016/j.aqrep.2022.101269
Zhu X.-D., Shi X., Wang S.-W., Chu J., Zhu W.-H., Ye B.-C., et al. (2019). High-throughput screening of high lactic acid-producing Bacillus coagulans by droplet microfluidic based flow cytometry with fluorescence activated cell sorting. RSC Adv. 9, 4507–4513. doi: 10.1039/c8ra09684h
Keywords: antioxidation, Bacillus coagulans, Macrobrachium nipponense, polysaccharide, immunity
Citation: Wang Y, Liang Y, Yu J, Li Z, Wang W, Jiang L and Liu B (2024) Effects of polysaccharide fermentation with Bacillus coagulans on growth, antioxidant and immunity of Macrobrachium nipponense (riental river prawn). Front. Mar. Sci. 11:1514651. doi: 10.3389/fmars.2024.1514651
Received: 21 October 2024; Accepted: 25 November 2024;
Published: 11 December 2024.
Edited by:
Luodong Huang, Guangxi University, ChinaReviewed by:
Abdallah Ghonimy, Yellow Sea Fisheries Research Institute (CAFS), ChinaAmit Ranjan, Tamil Nadu Fisheries University, India
Bulu Mohanta, Seemanta Institute of Pharmaceutical Sciences (SIPS), India
Copyright © 2024 Wang, Liang, Yu, Li, Wang, Jiang and Liu. This is an open-access article distributed under the terms of the Creative Commons Attribution License (CC BY). The use, distribution or reproduction in other forums is permitted, provided the original author(s) and the copyright owner(s) are credited and that the original publication in this journal is cited, in accordance with accepted academic practice. No use, distribution or reproduction is permitted which does not comply with these terms.
*Correspondence: Li Jiang, MTMwNTQ2MjM4MEBxcS5jb20=; Bo Liu, bGl1YkBmZnJjLmNu
†These authors have contributed equally to this work