- 1Doerr School of Sustainability, Stanford University, Stanford, CA, United States
- 2University of California, Santa Cruz, Institute of Marine Sciences, Santa Cruz, CA, United States
- 3Golden Honu Services of Oceania, Honolulu, HI, United States
- 4Southwest Fisheries Science Center, National Oceanic and Atmospheric Administration (NOAA), La Jolla, CA, United States
- 5Laboratorio de Genética, Unidad Académica Mazatlán, Instituto de Ciencias del Mar y Limnología, Universidad Nacional, Autónoma de México, Mazatlán, Sinaloa, Mexico
- 6Port of Nagoya Public Aquarium, Nagoya, Japan
- 7Golden Honu Services of Oceania, Newport, OR, United States
- 8Hawaii Preparatory Academy, Kamuela, HI, United States
- 9Usa Marine Biological Institute, Kochi University, Tosa, Kochi, Japan
- 10Marine Biology Program, University of Hawaii at Manoa, Honolulu, HI, United States
The North Pacific Transition Zone (NPTZ) is known as a global marine hotspot for many endangered and commercially significant highly mobile marine species. In the last few decades, the region has undergone unprecedented physical and biological transformations in response to climate variability and change. Although it is anticipated that many highly mobile species will need to adapt and shift their distributions, current predictions have relied on short-term data sets or modeled simulations. This has left a critical gap in our understanding of long-term (decadal or longer) change and species’ responses within the NPTZ. Here, we integrate nearly 3 decades of satellite tracking data from a climate sentinel, the juvenile North Pacific loggerhead sea turtle (Caretta caretta), with concurrent observations of sea surface temperature (SST) and chlorophyll-a concentrations to examine higher trophic level response to climate-induced changes within the eastern bounds of the NPTZ. Between 1997–2024, the NPTZ has warmed by 1.6°C and experienced an approximately 19% decline in mean surface chlorophyll-a concentration, a proxy for reduced productivity, resulting in a 28% (1.65 million km2) increase in total oligotrophic habitat in the eastern NPTZ. Over the same period, the average latitude of loggerhead sea turtle foraging habitat in the NPTZ has shifted northwards by 450–600 km. This represents a distributional shift rate of 116–200km/decade. In most years both the southern and northern range limits for the loggerhead turtle have shifted northward in tandem, indicating a habitat range shift rather than a range expansion. Our findings reveal significant physical and biological change to the NPTZ over the last quarter century and the first empirical evidence illustrating the substantial spatial response of a highly mobile megafaunal species. As the NPTZ continues to become more oligotrophic, these insights can provide vital information for dynamic conservation and management strategies within this critically important ecosystem.
Introduction
The North Pacific Ocean is undergoing an unprecedented transformation. Multi-decadal changes in the atmosphere and ocean have resulted in a northward expansion of subtropical gyre waters, a northward shift of the Kuroshio Current Extension current, and a decline in phytoplankton in the North Pacific Transition Zone (NPTZ) (Le et al., 2019; Yang et al., 2020; Kawakami et al., 2023). Against the backdrop of these climate-driven changes, discrete periods of anomalously warm sea surface temperatures known as marine heatwaves (MHWs) have produced some of the most intense warming episodes on record (Hobday et al., 2018; Oliver et al., 2018). The accelerated pace of these perturbations has led to profound biological shifts, with cascading effects propagating across the entire marine ecosystem (Smith et al., 2023; Welch et al., 2023; Farchadi et al., 2024; Gomes et al., 2024).
As a consequence of environmental change, a first response for many pelagic species is a geographic shift in search of favorable thermal and/or foraging habitats (Poloczanska et al., 2013; Pecl et al., 2017). The eastern North Pacific is known as a biodiversity hotspot for many highly migratory marine species (Block et al., 2011), such as tuna and sea turtles (Polovina et al., 2001), pinnipeds and sea birds (Block et al., 2011; Clay and Brooke, 2024), flying squid (Ichii et al., 2009; Polovina et al., 2017), sharks, and a variety of other fishes (Brodeur et al., 1999; Whitney, 2015). The rapid rate of change within the region has generated great interest as to how many of these species will be forced to adapt or adjust. Given that many of these higher trophic level species serve as “climate sentinels”, signaling otherwise unobserved shifts to the broader marine ecosystem (Hazen et al., 2019; Early-Capistrán et al., 2024), there is now a growing understanding of how North Pacific environmental variability will trigger species redistributions and thermal displacements (Jacox et al., 2020; Welch et al., 2023), altered predator-prey relationships (Thorne et al., 2016; Gomes et al., 2024) and increase reproductive failures (Hipfner et al., 2020). By the end of the century, projections have signaled significant, irreversible shifts to core habitats for many ecologically and commercially valuable mobile marine species (Hazen et al., 2013; Cheung et al., 2015; Lezama-Ochoa et al., 2024). As a result, there is substantial concern that ocean warming across the North Pacific will present new challenges and risks to species conservation and fisheries management strategies (Cheung et al., 2013; Cavole et al., 2016; Farchadi et al., 2024), as may be the case in the eastern North Pacific.
To date, most of our knowledge in this context comes from the well-studied California Current System (CCS), owing to the exceptional observational and tracking studies that have originated from this productive eastern boundary system (e.g (Block et al., 2011; Hazen et al., 2018; Welch et al., 2023; Lezama-Ochoa et al., 2024). These studies have examined the potential impacts to highly migratory species habitats in relation to both short- and long-term ocean warming within the CCS. Farther offshore, the expansion of the low-chlorophyll-a subtropical biome is expected to have significant ecological and economic consequences to many of these same vulnerable species within the region (Kobayashi et al., 2008; Cheung et al., 2009; Hazen et al., 2013). Due to the North Pacific’s expansiveness and the lack of observational data at scale, our ability to grasp the adaptive capacity of highly mobile species to recent change within the pelagic realm is less well understood (Holser et al., 2022).
Spanning the North Pacific, the NPTZ is the key oceanographic boundary between the subarctic and subtropical gyres. It serves as an important juncture for ocean currents, nutrient dynamics, and marine biodiversity. Roughly located between 30–32°N and 42–45°N latitudes, it contains the eastward flowing Kuroshio Extension Current west of the dateline which becomes the North Pacific Current east of the dateline. The region is also characterized by many temperatures, chlorophyll-a, salinity fronts, and mesoscale eddies which create areas of high biological productivity that attract highly migratory species previously described. As such, within these productive international waters, the region’s fisheries resources are targeted by fleets from many nations.
Previous investigations of species-environment shifts within the NPTZ have relied on short-term animal tracking data to examine seasonal or interannual relationships (e.g Polovina et al., 2001; Holser et al., 2022; Clay and Brooke, 2024). Simulations using climate-scenario models have added to our long-term (i.e., decadal or longer) expectations of how species may acclimate (Hazen et al., 2013). But forecasting distributional shifts can be challenging, as models may depart from anticipated patterns of range shift or movement, especially if climate-driven change occurs faster than expected (Chen et al., 2011; García Molinos et al., 2016). Given the multiple lines of evidence that signal biophysical change within the region (Polovina et al., 2008; Le et al., 2019; Yang et al., 2020), our inability to accurately capture long-term ecological response on the order of decades or longer leaves a critical gap in understanding how these species will adapt.
Addressing this gap, juvenile North Pacific loggerhead sea turtles (Caretta caretta) offer an excellent study species to monitor ecosystem change within the region. Loggerhead sea turtle populations in the Pacific Ocean are genetically distinct and Japan represents the sole nesting ground for individuals from the North Pacific population (Bowen et al., 1995). Their long-term dataset of satellite tracking within the North Pacific, spanning several decades (1997–2024), represents the most comprehensive population-level datasets of any marine species. Additionally, their affinity to the well-defined oceanographic properties of the North Pacific has been described in detail (Polovina et al., 2000; Polovina et al., 2001; Polovina et al., 2004; Polovina et al., 2006; Howell et al., 2008; Kobayashi et al., 2008; Abecassis et al., 2013). Decades of research on the species including electronic tagging studies shows that North Pacific loggerheads exhibit a well-established 1,000-km north-south seasonal movement that closely tracks the movement of the Transition Zone Chlorophyll Front (TZCF), a dynamic feature that marks the sharp surface chlorophyll-a gradient between the oligotrophic subtropical waters with surface chlorophyll-a less than 0.2mg/m3 to the south and the temperate waters with surface chlorophyll-a exceeding 0.2mg/m3 to the north (Polovina et al., 2001; Polovina et al., 2017). Such tracking data has shown that these turtles travel east and west across the NPTZ as they undertake seasonal north-south movements, occupying developmental habitats that span the open ocean and the mid latitudes of the North American coast (Polovina et al., 2001; Kobayashi et al., 2008; Briscoe et al., 2016).
As ectotherms, loggerheads are particularly sensitive to thermal cues, as temperature is one driver of habitat and distribution (Epperly et al., 1995) while prey abundance is the other (Plotkin et al., 1993; Parker et al., 2005; Mariani et al., 2023). North Pacific loggerhead sea turtles are known to be opportunistic foragers within their oceanic habitats, with a diet consisting of neustonic animals including Janthina spp., Carinara cithara, Vella vella, Lepas spp., Planes spp., and pyrosomas (see Parker et al., 2005). Given their sensitivity to ecosystem perturbations, conspicuity as an upper trophic level species, and vulnerable status as an endangered species (NOAA, 2021), they emerge as potent climate sentinels for gauging the on-going change to the overall structure and function of the NPTZ ecosystem (Sydeman et al., 2015; Hazen et al., 2019; Early-Capistrán et al., 2024). Through the application of our long-term data set, they may also serve as proxies for other highly mobile and protected species that utilize the region but are difficult to track.
This study combines almost 3 decades of loggerhead sea turtle data with concurrent ocean observations to investigate higher trophic level response to environmental change within the NPTZ. We first identify temporal trends in SST and chlorophyll-a within the eastern NPTZ. We then characterize the animals’ response to these changing ocean conditions. Our results identify a significant change to the NPTZ and thus latitudinal location of turtle habitat in the last quarter century and provide the first empirical evidence of important multi-decadal trends within the NPTZ.
Methods
Environmental data collection
The NPTZ domain was defined as the region between 30°N-45°N, 180°W-140°W, as shown in Figure 1. This area represents 5,532,704 km2 of the North Pacific Ocean. To examine changes to SST and chlorophyll-a within this region, monthly data from September 1997 to September 2024 were obtained for SST at a 5-km spatial resolution from NOAA Coral Reef Watch Program (NOAA, 2024) and chlorophyll-a data at a 4-km spatial resolution from Copernicus Marine Environmental Monitoring Service’s Global Ocean-Colour blended product (CMEMS, 2024). Turtle locations were matched to the SST and chlorophyll-a grids of these products and extracted underneath each location.
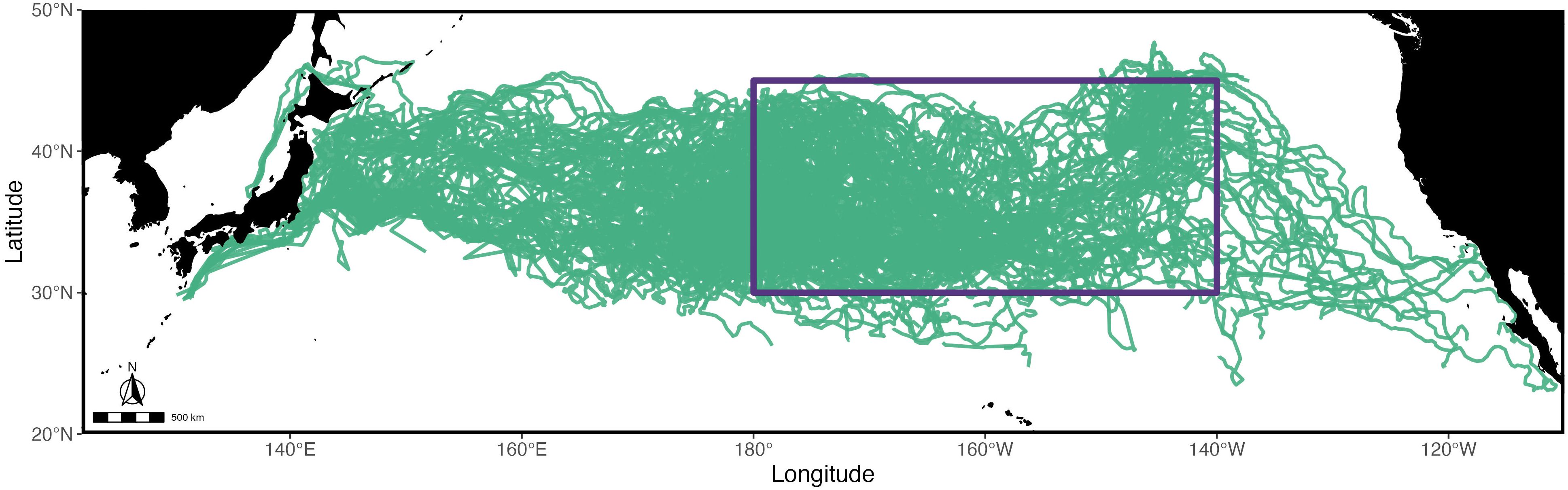
Figure 1. Study area map of historic (1997–2013) and STRETCH (2023–2024) juvenile loggerhead sea turtles in the North Pacific Ocean. Individual satellite tracks (n=220) are shown as green lines. The North Pacific Transition Zone (NPTZ) study area (30°N-45°N, 180°W-140°W) is represented with the purple box.
Loggerhead satellite tracking
Our study involves the synthesis of 307 juvenile North Pacific loggerhead sea turtle tracks between January 1997 - September 2024. Of these, 220 individuals utilized the waters within the eastern North Pacific Transition Zone study area (Figure 1; Table 1). For the remainder of the study, these turtles will be the study focus.

Table 1. Tracking summary of 220 juvenile North Pacific loggerhead sea turtles monitored from 1997–2024.
Of the 220 individuals, 193 were raised at the Port of Nagoya Public Aquarium in Nagoya, Japan. The remaining 27 individuals were wild turtles caught and released after interaction with the Hawaii-based longline fishery. Polovina et al. (2006) discussed the movements and activity between captive-reared and wild-caught North Pacific juvenile loggerhead sea turtles, demonstrating no observable difference in transmissions times, distance traveled, and seasonal movement between the two groups. Detailed methods on animal care, tagging, and release locations can be found in Abecassis et al. (2013) and Balazs et al. (2016). Briefly, Argos-linked satellite transmitters were attached to the carapace of all juveniles, following the procedures recommended in (Balazs et al., 1996). Argos-derived surface locations were collected by the NOAA PIFSC, Marine Turtle Research Program, Hawaii (1997–2013), and by Stanford University, California (2023–2024). All raw Argos turtle positions were fitted to a random walk State Space Model (SSM) using the ‘aniMotum’ package (Jonsen et al., 2023). This approach provides time-regularized estimates (24-h intervals) of animal location while accounting for observation error and irregularity.
Previous publications have examined the seasonal and interannual movements of individuals from this data set between 1997–2013 (Polovina et al., 2000; Polovina et al., 2001; Polovina et al., 2004; Polovina et al., 2006; Howell et al., 2008; Kobayashi et al., 2008; Howell et al., 2010; Abecassis et al., 2013; Briscoe et al., 2016; Briscoe et al., 2021). The inclusion of 53 turtles covering the period July 2023-September 2024 were part of the Sea Turtle Research Experiment on the Thermal Corridor Hypothesis (STRETCH) initiative (www.loggerheadstretch.org). This is the first publication of these new tracks and of the entire dataset that spans a 27-year study period. Collectively, these data represent the most extensive satellite tracking datasets of juvenile sea turtles from a single population.
Data analyses
Trend estimation within the NPTZ
We used generalized additive mixed models (GAMMs) to characterize seasonal and interannual trends in temperature, productivity, and turtle habitat across the NPTZ in the last 27 years. GAMMs are an extension of generalized additive models, commonly used to model complex ecological response shapes as they fit non-linear functions using data defined smoothers (Elith et al., 2006; Vanselow et al., 2021). Given the autocorrelative nature of the time series data sets, GAMMs allow for the incorporation of random effects to deal with the non-independence of observations.
We fit a suite of GAMMs with separate response variables to a base equation containing two independent variables: a smoothed monthly term to model seasonal (i.e., cyclical) patterns and a fixed linear term for year to model the long-term trend. Model response variables are described in detail below. Briefly, they included the average SST and chlorophyll-a concentrations across the NPTZ study area, the average area of low chlorophyll-a waters (total surface area < 0.2 mg/m3) within the study area, and the average loggerhead sea turtle distribution within the NPTZ by latitude. All data processing and analyses were performed using R (version 4.2.2) and Python (version 3.9.6). Statistical models were fit using the ‘mgcv’ package in R (Wood and Wood, 2015) using a gaussian distribution and identity link function.
Average SST and chlorophyll-a concentrations within the NPTZ
To examine concurrent changes in sea surface temperature and chlorophyll-a concentrations within the NPTZ in the past 27 years, we first computed mean monthly SST and chlorophyll-a concentrations spatial averages within the NPTZ study region between September 1997 - September 2024. Quarterly and annual means were also calculated to compare winter (Q1, January-March) and summer (Q3, July-September) trends in the NPTZ.
The time series of spatially averaged SST and chlorophyll-a concentrations were each used as response variables and fitted to the base equation. Chlorophyll-a concentrations were log-transformed for model fitting. Given the autocorrelative properties of the environmental time series (Simpson, 2018), a low order autoregressive (AR) structure was added to both models.
Area of oligotrophy
Satellite-derived surface chlorophyll-a imagery of the central North Pacific shows the large oligotrophic subtropical gyre as characterized by surface chlorophyll-a waters less than 0.2mg/m3. Thus, those waters in the NPTZ that have surface chlorophyll-a less than 0.2mg/m3 were used to characterize less productive, oligotrophic waters (Polovina et al., 2001). To quantify the seasonal and interannual variation of these oligotrophic waters in the NPTZ across the study period, we modelled the spatially averaged monthly and annual area of surface chlorophyll-a (km2) less than 0.2 mg/m3. Following a similar approach to Polovina et al. (2008), we first calculated the monthly mean, quarterly, and annual areas less than 0.2 mg/m3 surface chlorophyll-a concentrations between 30°N-45°N, 180°W-140°W. To determine the area in km2, longitudes were transformed as a function of 1-degree latitude increment using the equation: longitude * cos(latitude) (see Polovina et al., 2008). The average area (km2) < 0.2 mg/m3 chlorophyll-a concentration was then fitted to the base equation. Similar to the average SST and chlorophyll-a time series models, a low order AR structure was applied.
Loggerhead latitude
To characterize changes in observed loggerhead latitudinal distribution over time, we first filtered the tracking data set to only include tracks within the NPTZ region (Figure 1). The monthly mean turtle-latitude, turtle-SST, and turtle-chlorophyll-a concentrations were then calculated for each individual across the zonal band of longitude (180°W -140°W). Several additional candidate models were explored (see Supplementary Material), however the final GAMM was fitted to the base equation using latitude as the response variable and animal ID as a random effect.
Yearly grouped approach
The tracking data from 1997 to 2024 were not uniformly distributed across this entire period but fell into four discrete groupings: 1997–2000, 2004–2008, 2010–2013, and 2023–2024. As a simpler and perhaps more robust approach to the GAMM, we computed the annual and quarterly mean turtle latitudes, SSTs, and chlorophyll-a concentrations experienced by each year group and plotted the group means.
Results
Oceanographic trends
Results identified statistically significant seasonal and long-term trends across all GAMM models (p < 0.05). Given that the seasonal trends for chlorophyll-a, temperature, and turtles have been well-established within this region (Polovina et al., 2001; Bograd et al., 2004), our results focus on the long-term trends. Specifically, during the winter and spring vertical mixing and southward Ekman transport elevate nutrient and chlorophyll-a levels in the NPTZ while during summer and fall increased vertical stratification and relaxed westerly winds result in more oligotrophic conditions. For more details on seasonal dynamics please refer to the Supplementary Material.
From September 1997-September 2024, the annual trends in SST within the NPTZ study region from the GAMM showed a statistically significant linear increase in average surface temperatures from 15.82°C – 17.41°C (Figure 2; Table 2A). This represented an average increase of 1.59°C. Quarterly values showed this warming trend occurred during both the winter and summer months (Figure 2A). Seasonally, the NPTZ followed well-established trends (see Bograd et al., 2004) of cooler SSTs in the winter (< 15°C) and warmer SSTs in the summer (> 20°C) (Supplementary Figure S1A).
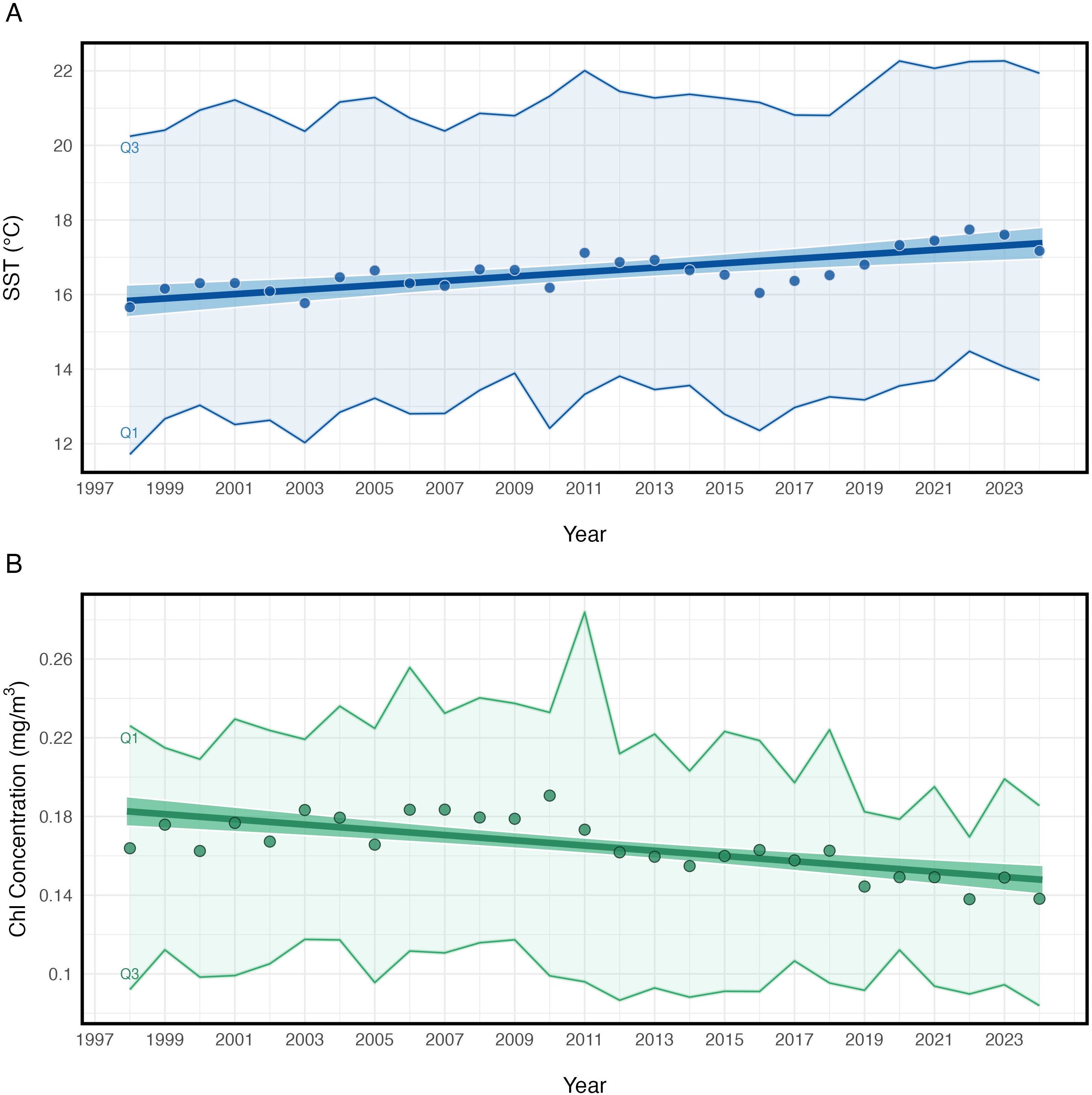
Figure 2. Fitted trend lines of mean SST (A) and mean chlorophyll-a concentration (B) by year, between 30°N-45°N, 180°W-140°W. Annual means are shown as circles. The shaded regions depict the extent of the winter (Q1) and summer (Q3) quarterly means.
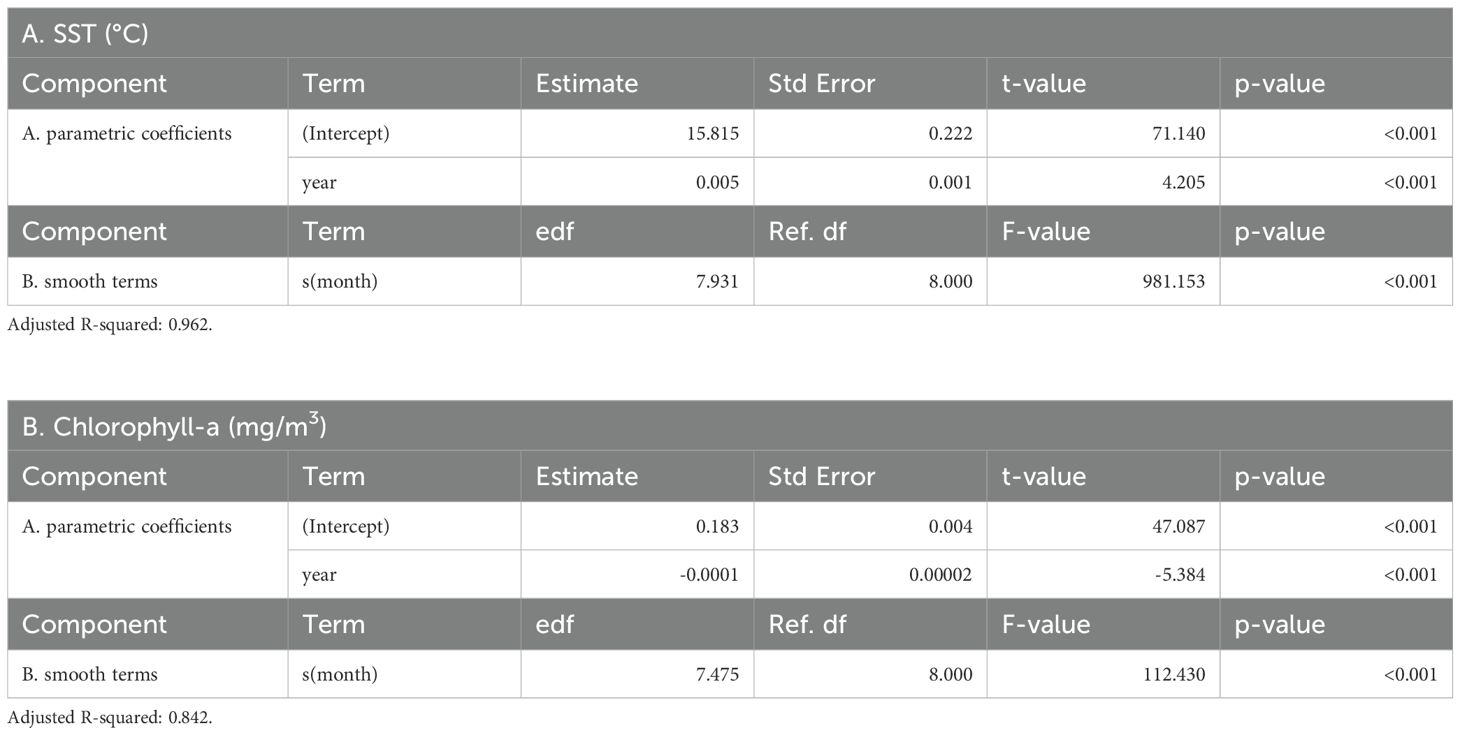
Table 2. Generalized additive mixed model results of (A) average SST and (B) chlorophyll-a concentration within the NPTZ.
The long-term trend in average chlorophyll-a concentrations showed a statistically significant decline over the study period from approximately 0.17 mg/m3 in the late 1990’s to 0.14 mg/m3 in recent years (Figure 2B; Table 2B). This represented an average decrease of 19.58% in chlorophyll-a concentrations within the study period. While annual chlorophyll-a concentrations increased between 1998–2011, average concentrations began to decrease with a substantial shift in the winter of 2012 and have continued to decline since then, most notably in the winter months (Figure 2B). Seasonally, chlorophyll-a concentrations were greatest in the winter (> 0.20 mg/m3) and lowest in the summer (< 0.10 mg/m3) (Supplementary Figure S1B).
Figure 3 shows the strong seasonal expansion and contraction of the oligotrophic waters in the NPTZ. The total area of oligotrophic water is reduced to less than 3 million km2 in the winter when chlorophyll-a concentrations are at a maximum (Supplementary Figures S1B, S2). During the summer as the climatological position of the TZCF migrates north, regional SSTs and vertical stratification increase account for over 5 million km2 (Supplementary Figure S2). Since 1997, annual trends in oligotrophic waters have exhibited a statistically significant linear increase (Table 3). The average total area of oligotrophic waters has increased by approximately 1.65 million km2 (Figure 3). This correlates to a 28.07% increase in oligotrophic waters in just a quarter century. The most notable changes occurred in the winter months especially since 2011. Between 2011 and 2023/2024 the proportion of oligotrophic waters in the winter in the NPTZ increased from 25.17% to greater than 60% of the NPTZ area (Figure 3).
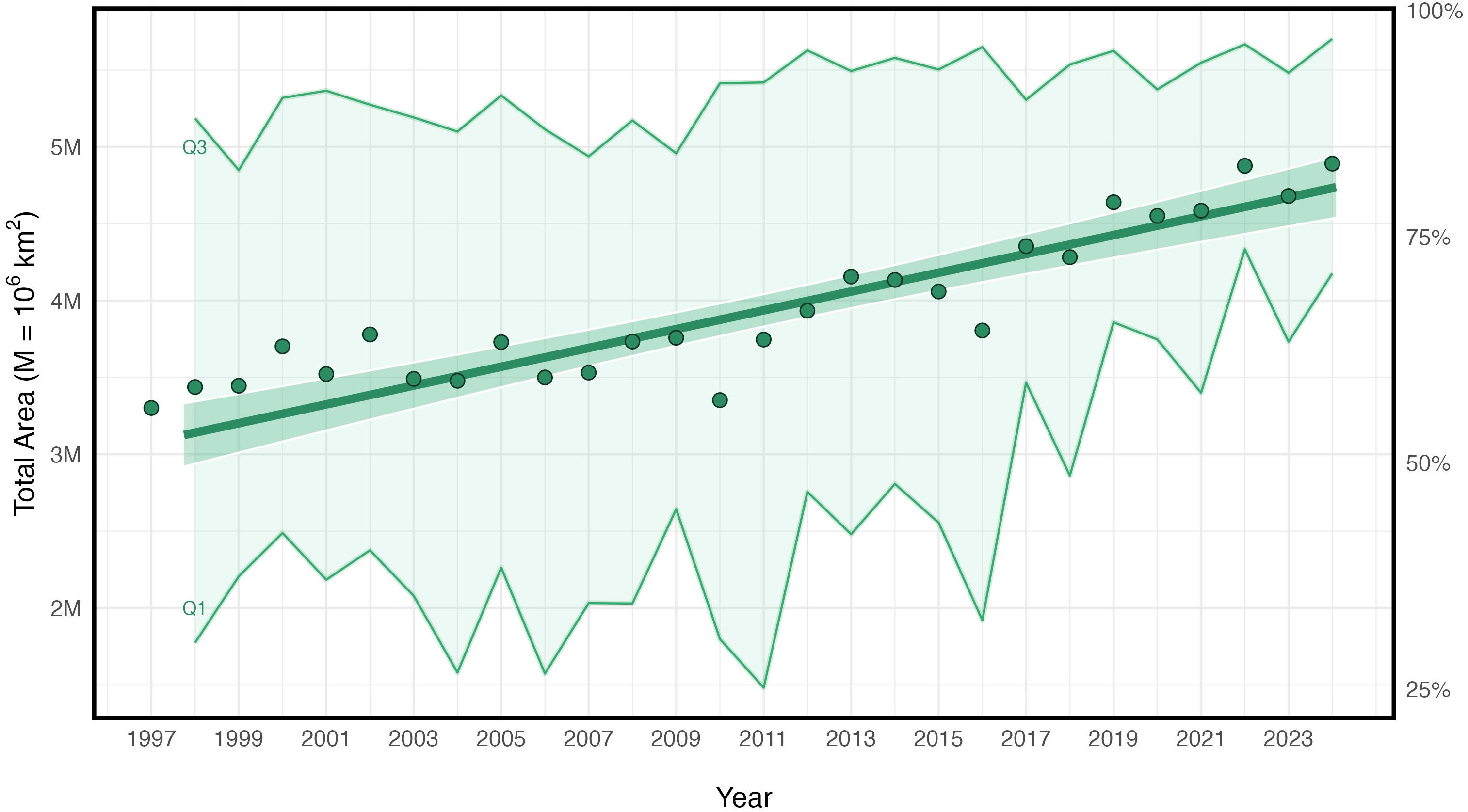
Figure 3. Fitted trend lines showing the change in total area (km2) of oligotrophic waters (surface chlorophyll-a < 0.2 mg/m3 by year, between 30°N-45°N, 180°W-140°W). Annual means are shown as green circles. The y-axes represent the total change in millions of km2 (shown on the left) and percent of total area (shown on the right). The shaded regions depict the extent of the winter (Q1) and summer (Q3) seasonal means.
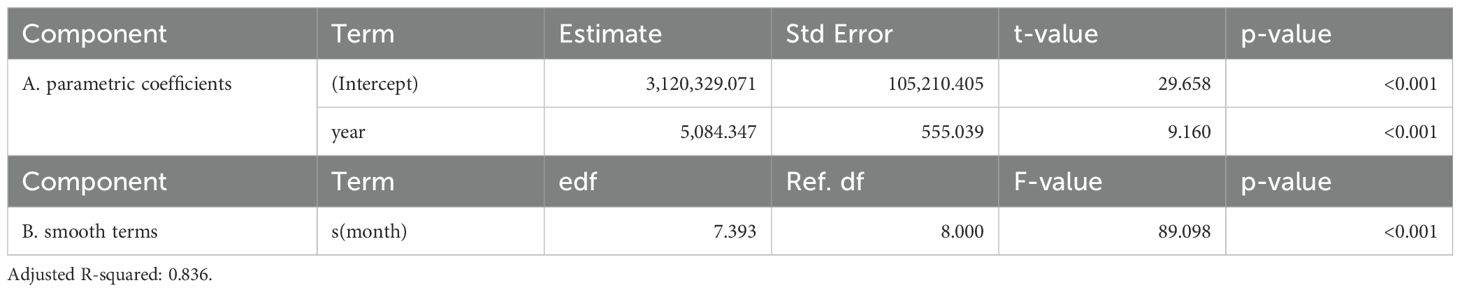
Table 3. Generalized additive mixed model results of total area (km2) below 0.2 mg/m3 chlorophyll-a concentrations within the NPTZ.
Turtle habitat trends
Of the 220 North Pacific loggerheads in the data set, 167 satellite tracks from 1997–2013 and 53 from 2023–2024 utilized the NPTZ domain (30°N-45°N, 180°W-140°W). From 1997–2013, average individual total transmission length was 420 days (range: 41–1,434 days) and 8,337 km (range: 737–25,900 km). From 2023–2024, average individual total transmission length was 153 days (range: 30–262 days) and 3,447 km (range: 551–8,439 km) (Table 1).
Average turtle latitude followed an expected N-S trend of about 10° in latitude (Supplementary Figure S3), as turtles tracked the productive TZCF from its southernmost position in the winter months (Feb-Mar) to its northernmost position in the summer months (Aug-Sept).
Turtles occupied habitat with a mean of 17.63°C SST (+/- 1.80°C SD) and 0.16 mg/m3 chlorophyll-a concentrations (+/- 0.07 mg/m3 SD) (Supplementary Figures S4, S5). Results from the fitted GAMM estimated average loggerhead habitat has gradually shifted north between 1997–2024 by about 450 km, from 34.6°N to 39.0°N latitude (Figure 4A; Table 4). The years with the greatest tagging data (i.e. 2005-2007) were apparent in the GAMM fit. When the turtle tracks were aggregated by discrete year groups, the number of individuals within each year grouping were more evenly distributed. Results of this comparative analysis were similar to the GAMM outputs, but indicated a potentially greater northward shift as the average annual mean latitude showed a 600 km increase, from 32.4°N to 41.7°N latitude (Figure 4B). Further, in most years, both the southern (winter) and northern (summer) habitat latitudes showed similar northward shifts, indicating that the extent of the seasonal migration of about 1,000 km remains unchanged (Figure 4B).
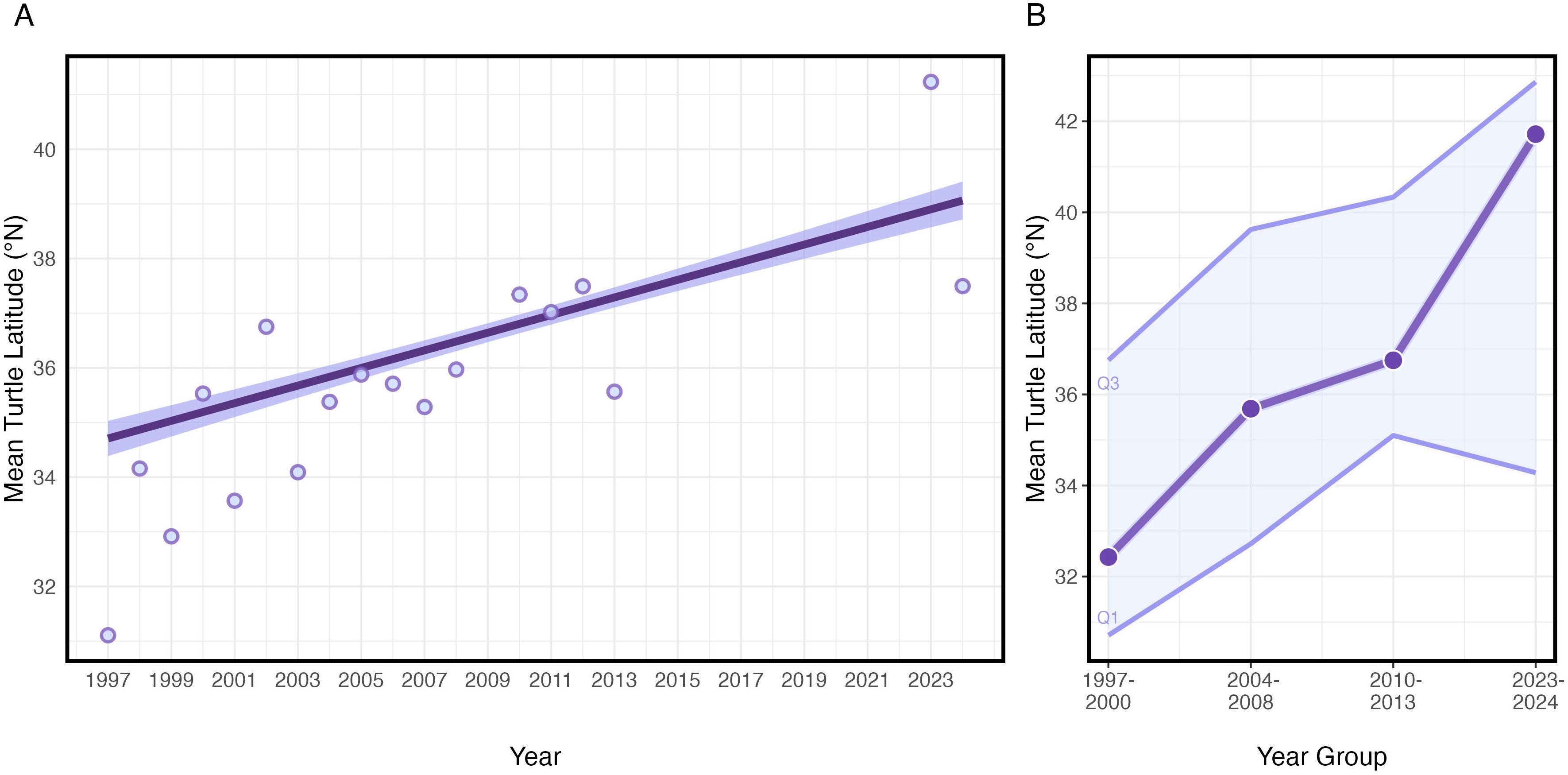
Figure 4. (A) Fitted trend lines showing the mean turtle latitude by year (30°N-45°N, 180°W-140°W) between September 1997 – September 2024. The shaded region depicts the 95% confidence interval. Observed annual mean turtle positions are shown as purples circles. (B) Mean turtle latitude grouped by year (1997–2000, 2004–2008, 2010–2013, and 2023–2024) and averaged across the study area from 180°W-140°W longitude. Spatial means are shown as circles. Quarterly means (Q1, Q3) are shown as thin boundary lines.
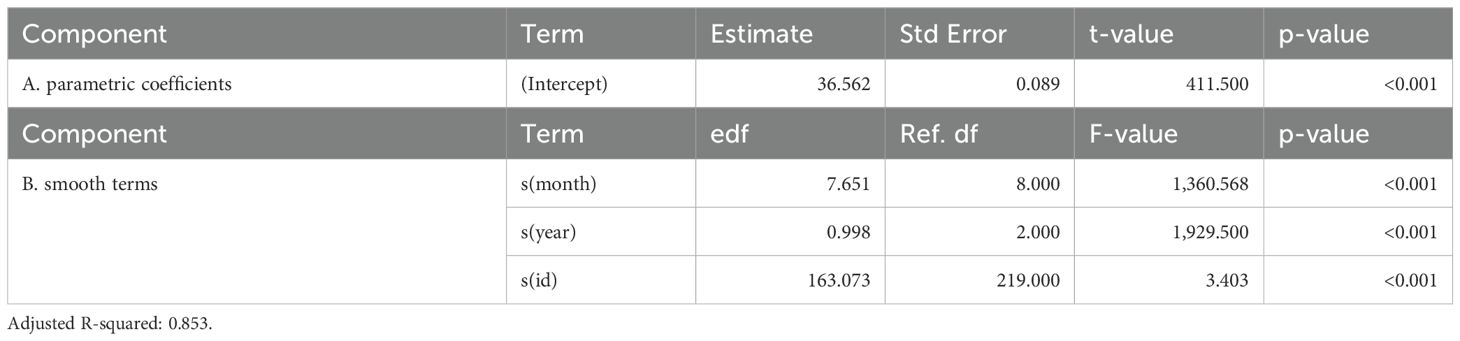
Table 4. Generalized additive mixed model results of average loggerhead latitudinal movements within the NPTZ.
Across the same discrete year groups, average turtle SST and chlorophyll-a associations within the NPTZ showed some year to year variability but were within preferred habitat ranges (Supplementary Figures S4, S5). When grouped into multiple years, average turtle associated SSTs varied from 16.5°C (2010-2013), 17.6°C (2024-2007, 2023–2024), to 18.9°C (1997-2000) (Figure 5A). Average turtle associated chlorophyll-a concentrations varied from 0.12 mg/m3 (1997-2000, 2023-2024), 0.18 mg/m3 (2004-2008), to 0.20 mg/m3 (2010-2013) (Figure 5B).
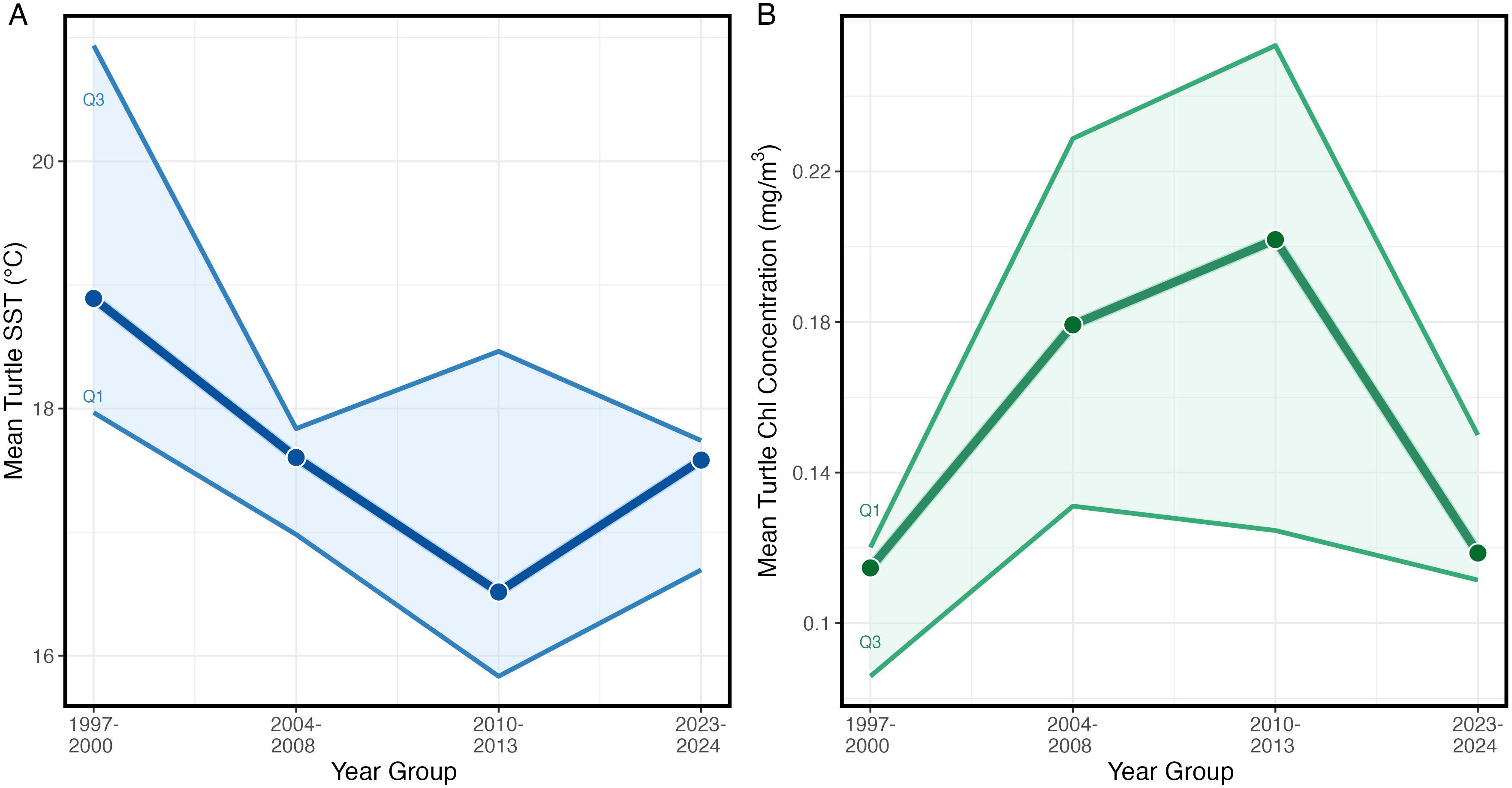
Figure 5. Annual variation in turtle associated (A) sea surface temperatures, and (B) chlorophyll-a concentrations (mg/m3) grouped by year (1997–2000, 2004–2008, 2010–2013, and 2023–2024) and averaged across the study area from 180°W-140°W longitude. Spatial means are shown as circles. Quarterly means (Q1, Q3) are shown as thin boundary lines.
Discussion
Over the past 27 years, various subsets of this loggerhead sea turtle electronic tracking data set have played pivotal roles in defining the ecological significance of the NPTZ and how these animals use this region (Polovina et al., 2000; Polovina et al., 2001; Polovina et al., 2004; Polovina et al., 2006; Kobayashi et al., 2008). Since then, our understanding of this dynamic but persistent basin-scale ocean feature has grown into a well-studied marine productivity hotspot for migratory marine animals and commercial fisheries (Polovina et al., 2001; Kappes et al., 2010; Block et al., 2011; Hazen et al., 2013; Xu et al., 2017). Across this same period, the eastern North Pacific has experienced an unprecedented rate of change. The combined impacts of MHWs and long-term climate change threaten key ecological structure and functions that have defined the NPTZ. While evidence of physical forcing and lower trophic level alterations has been mounting (Polovina et al., 2008; Polovina et al., 2011), our ability to map these changes onto higher trophic levels has been limited. To date, our knowledge has been informed by short-term studies and end-of-century simulations, but there has been scarce long-term information (decadal-scale or longer) that integrates across these climatic extremes.
Our results show that over the past 27 years the NPTZ has become more oligotrophic with the rate of greatest increase during the winter and especially since 2011 (Figure 3). Specifically, the eastern NPTZ has gradually warmed by an average of 1.59°C but experienced a much more substantial biological change with mean chlorophyll-a density declining by almost 19.58% (Figures 2A, B), with the greatest signals of change occurring in the winter months. Across the study period, the 5-year average winter SSTs in the NPTZ have increased from approximately 12.4°C from the beginning of the study period to 13.8°C at the end of the study period while average chlorophyll-a concentrations have decreased from approximately 0.22 mg/m3 to 0.185 mg/m3 during this same time. This represents a significant increase in oligotrophic waters, from ~3 million km2 to 4.5 million km2, with a notable increase since ~2011 (Figure 3).
Results from our study align with previous research signaling significant biophysical change within the North Pacific Ocean. Polovina et al. (2008); Polovina et al. (2011) and Yang et al. (2020) identified climate-induced poleward expansion of the subtropical gyre and reduced productivity due to ocean warming. Le et al. (2019) and Kawakami et al. (2023) reported a northward shift of the Kuroshio Current Extension current and a decline in phytoplankton abundance in the NPTZ. The impacts of the increasing trend in recent MHW events (Smith et al., 2023) are evident in the further reduction of chlorophyll-a since 2012 (Figure 2), however our results highlight a longer-term change within the region over the past few decades.
In response to these changes, loggerhead sea turtles have shifted their foraging grounds northwards by 450 km (from the GAMM) to 600 km (from the year group), or about 116–200 km per decade (Figures 4A, B). Our results indicate that this population-level shift is not just a northern limit range extension of habitat, but rather indicative of a pervasive northward shift across both the lower and upper bounds of their habitat range (Figure 4B). The 200 km/decade northward shift is considerably more than the global mean rate for marine organisms of less than 100 km per decade (Poloczanska et al., 2013; Pinsky et al., 2020), but is closer to the upper-end rate of leading edge expansions for other higher trophic level species (i.e., bony fish, 277.5 km per decade) (Poloczanska et al., 2013). These rates suggest that the ecology of the eastern NPTZ may be changing more rapidly than other regions and our ability to measure the habitat of a higher trophic level species moving northwards in response to these changes is unique. Even though the NPTZ has warmed and experienced an almost 20% decline in chlorophyll-a, the loggerhead sea turtles despite their northward movement, continued to occupy waters within preferred habitat (Figures 5A, B). Broadly, these results suggest that turtles are adapting to a changing ocean by undertaking significant geographic shifts to maintain suitable habitat.
However, for loggerheads the ability to maintain an adaptive response to a changing environment may become more difficult over time. Globally, the rate of climate-driven change continues to accelerate the poleward expansion of many tropical and subtropical species, a phenomenon known as tropicalization (Osland et al., 2021; Zarzyczny et al., 2023). For such an important biogeographic transition zone such as the NPTZ, the expansion of warmer, more oligotrophic waters may create latitudinal alterations in prey availability and composition. These changes can appear subtle but can push many upper trophic level predators into new habitats and forage communities, with potentially significant top-down impacts to the structure and function of the rest of the marine ecosystem (Zarzyczny et al., 2023; Gomes et al., 2024). The composition and energetic density of prey at new latitudes can impact metabolic rates and reproductive outputs, given well-established energetic costs for long-distance migrators (Alerstam et al., 2003; Hays, 2008). As a sentinel species, the northern shift of juvenile loggerheads to access forage habitat may serve as an early warning sign of tropicalization within the NPTZ. This is especially true as species such as sea turtles have a narrower thermal tolerance compared to other highly mobile species within the region (Hazen et al., 2013). If prey assemblages continue to shift polewards at a rate faster than warming temperatures, sea turtles may be one of the first mobile species to lose the ability to synchronize the availability of forage habitat with thermal displacements. For example, Brodeur et al. (2018) and Gomes et al. (2024) reported an observed northward expansion of several gelatinous loggerhead prey species, such as pyrosomes, within the CCS. The increased abundance of these prey species coincided with the occurrence of juvenile loggerhead sea turtles in the Pacific Northwest further north than usual (Arden, 2023; Early-Capistrán et al., 2024; Ramirez, 2024). Within the past 5 years, a total of 20 stranded loggerheads have been recorded off of the US and Canada West Coast (NOAA Sea Turtle Stranding Network, 2024). Scat analysis of one particular individual found as far north as Vancouver, Canada, revealed the presence of pyrosomes (Parker et al., 2024) suggesting loggerheads may be following these typically tropical prey sources further north. If forage habitat continues to change at a faster rate than thermal tolerances, winners and losers will emerge, with many species struggling to keep pace. For North Pacific loggerhead sea turtles, a northward expansion becomes highly relevant in terms of energetic costs to forage at new latitudes while maintaining connectivity to known coastal habitats, such as the well-established developmental grounds of Baja California, Mexico (Nichols et al., 2000; Peckham et al., 2011; Wingfield et al., 2011; Seminoff et al., 2014; Tomaszewicz et al., 2015). Individuals will be faced with the need to travel farther south or establish new coastal habitats along the California coast (Allen et al., 2013; Eguchi et al., 2018; Welch et al., 2019).
Our study provides critical information as to how animals are responding to significant long-term change in one of the oceans most productive regions. To date, most studies have emphasized the role of temperature in reshaping habitat. Indeed, temperature plays a significant role in marine biodiversity patterns (Tittensor et al., 2010) and geographic redistributions (Poloczanska et al., 2013; McHenry et al., 2019). But recent insights have broadened our understanding of other environmental covariates (i.e., salinity, currents, productivity, pH) in species’ responses to climate change (see Poloczanska et al., 2016; McHenry et al., 2019). For sea turtles, a conventional approach might be to overemphasize temperature-based models when projecting species’ responses to change, especially given extrinsic thermal vulnerabilities (Goudarzi et al., 2024). Here we also underscore the importance of including other environmental variables in anticipating species’ shifts in a changing ocean.
Conclusions
Within the last few decades, the North Pacific has exhibited unprecedented change. The changes that we are seeing in the NPTZ oceanography and the northward shift in latitude of loggerhead sea turtles appear to be part of a broader northward expansion of the subtropical gyre and northward shift of the NPTZ as has been projected in climate models and observed with SST and altimetry data trends (Polovina et al., 2011; Yang et al., 2020). The documented northward shift in loggerhead presence is likely indicative of broader ecosystem impacts within the NPTZ including impacts to the foraging habitat of commercially valuable pelagic fishes, and other protected species. Understanding how sentinel species will respond and adapt to such an accelerated transformation is imperative to effectively maintaining and managing healthy ecological connections across their entire North Pacific habitat. To date, our ability to track changes to the environment and animal response has been limited. Future research is needed to fully understand these important changes within the context of a warming ocean. The outcomes of this study provide first-order documentation of a significant change to environmental conditions that have defined this ecologically important region and a population-level response by a high trophic level predator species that is highly sensitive to ecosystem change. In a warmer, less productive NPTZ, range shifts and expansions will result in habitat gains and losses and will pose new management challenges within international jurisdictions (Santos et al., 2024). For example, as loggerheads move further north, those that head eastward may enter the California Current at higher latitudes and face new mortality risks from different fisheries and cold stunning. Continued observation of decadal-scale animal response can be assimilated with short-term (i.e., seasonal, interannual) and longer-term information (i.e. climate modeled predictions). Such information will be critical for conservation assessments and management strategies for this endangered species. Sentinel species like loggerhead sea turtles, highly migratory and exceptionally sensitive to environmental changes, offer invaluable insights into the health and stability of the North Pacific ecosystem, and may provide early warning signals to improve forecasted species’ responses in a warming ocean. The exceptional dataset used in this study offers the first longitudinal perspective of its kind, giving scientists the ability to track subtle yet significant shifts in oceanic conditions and animal distributions, facilitating more accurate predictions, and enhancing dynamic management strategies (Howell et al., 2015; Maxwell et al., 2015).
Data availability statement
This study has been conducted using publicly available environmental data from NOAA Coral Reef Watch Version 3.1 (https://coralreefwatch.noaa.gov) and E.U. Copernicus Marine Service Information Global Ocean Colour (https://doi.org/10.48670/moi-00283). Please contact Denise Parker (ZGVuaXNlLm0ucGFya2VyQG91dGxvb2suY29t) and Larry Crowder (bGJjcm93ZGVyQHN0YW5mb3JkLmVkdQ==) to discuss data availability of the juvenile loggerhead tracking data set used here.
Ethics statement
The animal study was approved by Stanford University Protocol APLAC-34400. The study was conducted in accordance with the local legislation and institutional requirements.
Author contributions
DB: Conceptualization, Data curation, Formal analysis, Methodology, Visualization, Writing – original draft, Writing – review & editing. LC: Funding acquisition, Writing – review & editing. GB: Writing – review & editing, Data curation, Resources. JS: Writing – review & editing. AA: Writing – review & editing. CL: Resources, Writing – review & editing. MK: Data curation, Resources, Writing – review & editing. MM: Data curation, Writing – review & editing, Resources. DP: Writing – review & editing, Data curation. MR: Data curation, Writing – review & editing. TS: Writing – review & editing, Data curation, Resources. BS: Writing – review & editing. CT: Writing – review & editing. NY: Writing – review & editing, Resources. JP: Writing – review & editing, Conceptualization, Methodology, Writing – original draft.
Funding
The author(s) declare financial support was received for the research, authorship, and/or publication of this article. Funding was provided by the Gordon and Betty Moore Foundation and the National Geographic Society.
Acknowledgments
The authors would like to gratefully acknowledge the personnel at Port of Nagoya Public Aquarium and the students and staff of Usa Marine Biological Institute, Kochi University, for their generous role in securing hatchling sea turtles for this study. We would like to thank Laura Jim and the students of HPA for their field assistance. We thank Nick Wegner and the two reviewers for their constructive feedback, and we thank Erin LaCasella (NOAA) for providing sea turtle stranding information.
Conflict of interest
The authors declare that the research was conducted in the absence of any commercial or financial relationships that could be construed as a potential conflict of interest.
Generative AI statement
The author(s) declare that no Generative AI was used in the creation of this manuscript.
Publisher’s note
All claims expressed in this article are solely those of the authors and do not necessarily represent those of their affiliated organizations, or those of the publisher, the editors and the reviewers. Any product that may be evaluated in this article, or claim that may be made by its manufacturer, is not guaranteed or endorsed by the publisher.
Supplementary material
The Supplementary Material for this article can be found online at: https://www.frontiersin.org/articles/10.3389/fmars.2024.1513162/full#supplementary-material
References
Abecassis M., Senina I., Lehodey P., Gaspar P., Parker D., Balazs G., et al. (2013). A model of loggerhead sea turtle (Caretta caretta) habitat and movement in the oceanic North Pacific. PloS One 8, e73274. doi: 10.1371/journal.pone.0073274
Alerstam T., Hedenström A., Åkesson S. (2003). Long-distance migration: evolution and determinants. Oikos 103, 247–260. doi: 10.1034/j.1600-0706.2003.12559.x
Allen C. D., Lemons G. E., Eguchi T., LeRoux R. A., Fahy C. C., Dutton P. H., et al. (2013). Stable isotope analysis reveals migratory origin of loggerhead turtles in the Southern California Bight. Mar. Ecol. Prog. Ser. 472, 275–285. doi: 10.3354/meps10023
Arden A. (2023). Dead loggerhead sea turtle washes ashore on Oregon coast (Nexstar Media Inc). Available online at: https://www.koin.com/local/oregon-coast/dead-loggerhead-sea-turtle-washes-ashore-on-oregon-coast/
Balazs G., Miya R., Beavers S. (1996). Procedures to attach a satellite transmitter to the carapace of an adult green turtle, Chelonia mydas. NOAA Tech. Memorandum NMFS-SEFSC 387, 21–26.
Balazs G. H., Rice M., Parker D., Polovina J. (2016). High-seas releases of Pacific loggerheads from ships of opportunity. Indian Ocean SouthEast Asian (ISOSEA) Marine Turtle Memorandum of Understanding (MoU), c/o UNEP Regional Office for Asia and the Pacific. Available online at: https://georgehbalazs.com/ (Accessed February 13, 2024).
Block B. A., Jonsen I. D., Jorgensen S. J., Winship A. J., Shaffer S. A., Bograd S. J., et al. (2011). Tracking apex marine predator movements in a dynamic ocean. Nature 475, 86–90. doi: 10.1038/nature10082
Bograd S. J., Foley D. G., Schwing F. B., Wilson C., Laurs R. M., Polovina J. J., et al. (2004). On the seasonal and interannual migrations of the transition zone chlorophyll front. Geophysical Res. Lett. 31, 1–5. doi: 10.1029/2004GL020637
Bowen B., Abreu-Grobois F., Balazs G., Kamezaki N., Limpus C. J., Ferl R. (1995). Trans-Pacific migrations of the loggerhead turtle (Caretta caretta) demonstrated with mitochondrial DNA markers. Proc. Natl. Acad. Sci. 92, 3731–3734. doi: 10.1073/pnas.92.9.3731
Briscoe D., Parker D., Bograd S., Hazen E., Scales K., Balazs G., et al. (2016). Multi-year tracking reveals extensive pelagic phase of juvenile loggerhead sea turtles in the North Pacific. Movement Ecol. 4, 1–12. doi: 10.1186/s40462-016-0087-4
Briscoe D. K., Turner Tomaszewicz C. N., Seminoff J. A., Parker D. M., Balazs G. H., Polovina J. J., et al. (2021). Dynamic thermal corridor may connect endangered loggerhead sea turtles across the Pacific Ocean. Front. Mar. Sci. 8, 630590. doi: 10.3389/fmars.2021.630590
Brodeur R., McKinnell S., Nagasawa K., Pearcy W., Radchenko V., Takagi S. (1999). Epipelagic nekton of the North Pacific subarctic and transition zones. Prog. Oceanography 43, 365–397. doi: 10.1016/S0079-6611(99)00013-0
Brodeur R., Perry I., Boldt J., Flostrand L., Galbraith M., King J., et al. (2018). An unusual gelatinous plankton event in the NE Pacific: the great pyrosome bloom of 2017. PICES Press 26, 22–27.
Cavole L. M., Demko A. M., Diner R. E., Giddings A., Koester I., Pagniello C. M., et al. (2016). Biological impacts of the 2013–2015 warm-water anomaly in the Northeast Pacific: winners, losers, and the future. Oceanography 29, 273–285. doi: 10.5670/oceanog.2016.32
Chen I.-C., Hill J. K., Ohlemüller R., Roy D. B., Thomas C. D. (2011). Rapid range shifts of species associated with high levels of climate warming. Science 333, 1024–1026. doi: 10.1126/science.1206432
Cheung W. W., Brodeur R. D., Okey T. A., Pauly D. (2015). Projecting future changes in distributions of pelagic fish species of Northeast Pacific shelf seas. Prog. Oceanography 130, 19–31. doi: 10.1016/j.pocean.2014.09.003
Cheung W. W., Lam V. W., Sarmiento J. L., Kearney K., Watson R., Pauly D. (2009). Projecting global marine biodiversity impacts under climate change scenarios. Fish fisheries 10, 235–251. doi: 10.1111/j.1467-2979.2008.00315.x
Cheung W. W., Watson R., Pauly D. (2013). Signature of ocean warming in global fisheries catch. Nature 497, 365–368. doi: 10.1038/nature12156
Clay T. A., Brooke M. (2024). Trans-equatorial migration links oceanic frontal habitats across the Pacific Ocean: year-round movements and foraging activity of a small gadfly petrel. Mar. Biol. 171, 60. doi: 10.1007/s00227-023-04373-3
CMEMS EU Copernicus Marine Service, I. (2024). Global Ocean-Colour Blended Product. Available online at: https://marine.copernicus.eu/
Early-Capistrán M. M., Crane N. L., Crowder L. B., Garibay-Melo G., Seminoff J. A., Johnston D. (2024). Ethnobiological methods enhance our capacity to document potential climate sentinels: a loggerhead sea turtle (Caretta caretta) case study. Front. Mar. Sci. 11, 1407575. doi: 10.3389/fmars.2024.1407575
Eguchi T., McClatchie S., Wilson C., Benson S. R., LeRoux R. A., Seminoff J. A. (2018). Loggerhead turtles (Caretta caretta) in the California Current: abundance, distribution, and anomalous warming of the North Pacific. Front. Mar. Sci. 5, 452. doi: 10.3389/fmars.2018.00452
Elith J., Graham C., Anderson P. R., Dudík M., Ferrier S., Guisan A., et al. (2006). Novel methods improve prediction of species’ distributions from occurrence data. Ecography 29, 129–151. doi: 10.1111/j.2006.0906-7590.04596.x
Epperly S. P., Braun J., Chester A. J., Cross F. A., Merriner J. V., Tester P. A. (1995). Winter distribution of sea turtles in the vicinity of Cape Hatteras and their interactions with the summer flounder trawl fishery. Bull. Mar. Sci. 56, 547–568.
Farchadi N., Welch H., Braun C. D., Allyn A. J., Bograd S. J., Brodie S., et al. (2024). Marine heatwaves redistribute pelagic fishing fleets. Fish Fisheries. 25, 602–618. doi: 10.1111/faf.12828
García Molinos J., Halpern B. S., Schoeman D. S., Brown C. J., Kiessling W., Moore P. J., et al. (2016). Climate velocity and the future global redistribution of marine biodiversity. Nat. Climate Change 6, 83–88. doi: 10.1038/nclimate2769
Gomes D. G., Ruzicka J. J., Crozier L. G., Huff D. D., Brodeur R. D., Stewart J. D. (2024). Marine heatwaves disrupt ecosystem structure and function via altered food webs and energy flux. Nat. Commun. 15, 1988. doi: 10.1038/s41467-024-46263-2
Goudarzi F., Doxa A., Hemami M.-R., Mazaris A. D. (2024). Thermal vulnerability of sea turtle foraging grounds around the globe. Commun. Biol. 7, 347. doi: 10.1038/s42003-024-06013-y
Hays G. C. (2008). Sea turtles: a review of some key recent discoveries and remaining questions. J. Exp. Mar. Biol. Ecol. 356, 1–7. doi: 10.1016/j.jembe.2007.12.016
Hazen E. L., Abrahms B., Brodie S., Carroll G., Jacox M. G., Savoca M. S., et al. (2019). Marine top predators as climate and ecosystem sentinels. Front. Ecol. Environ. 17, 565–574. doi: 10.1002/fee.v17.10
Hazen E. L., Jorgensen S., Rykaczewski R. R., Bograd S. J., Foley D. G., Jonsen I. D., et al. (2013). Predicted habitat shifts of Pacific top predators in a changing climate. Nat. Climate Change 3, 234–238. doi: 10.1038/nclimate1686
Hazen E. L., Scales K. L., Maxwell S. M., Briscoe D. K., Welch H., Bograd S. J., et al. (2018). A dynamic ocean management tool to reduce bycatch and support sustainable fisheries. Sci. Adv. 4, eaar3001. doi: 10.1126/sciadv.aar3001
Hipfner J. M., Galbraith M., Bertram D. F., Green D. J. (2020). Basin-scale oceanographic processes, zooplankton community structure, and diet and reproduction of a sentinel North Pacific seabird over a 22-year period. Prog. Oceanography 182, 102290. doi: 10.1016/j.pocean.2020.102290
Hobday A. J., Oliver E. C., Gupta A. S., Benthuysen J. A., Burrows M. T., Donat M. G., et al. (2018). Categorizing and naming marine heatwaves. Oceanography 31, 162–173. doi: 10.5670/oceanog.2018.205
Holser R. R., Keates T. R., Costa D. P., Edwards C. A. (2022). Extent and magnitude of subsurface anomalies during the Northeast Pacific Blob as measured by animal-borne sensors. J. Geophysical Research: Oceans 127, e2021JC018356. doi: 10.1029/2021JC018356
Howell E. A., Dutton P. H., Polovina J. J., Bailey H., Parker D. M., Balazs G. H. (2010). Oceanographic influences on the dive behavior of juvenile loggerhead turtles (Caretta caretta) in the North Pacific Ocean. Mar. Biol. 157, 1011–1026. doi: 10.1007/s00227-009-1381-0
Howell E. A., Kobayashi D. R., Parker D. M., Balazs G. H., Polovina J. J. (2008). TurtleWatch: a tool to aid in the bycatch reduction of loggerhead turtles Caretta caretta in the Hawaii-based pelagic longline fishery. Endangered Species Res. 5, 267–278. doi: 10.3354/esr00096
Howell E. A., Hoover A., Benson S. R., Bailey H., Polovina J. J., Seminoff J. A., et al. (2015). Enhancing the TurtleWatch product for leatherback sea turtles, a dynamic habitat model for ecosystem-based management. Fisheries Oceanography 24, 57–68. doi: 10.1111/fog.2015.24.issue-1
Ichii T., Mahapatra K., Sakai M., Okada Y. (2009). Life history of the neon flying squid: effect of the oceanographic regime in the North Pacific Ocean. Mar. Ecol. Prog. Ser. 378, 1–11. doi: 10.3354/meps07873
Jacox M. G., Alexander M. A., Bograd S. J., Scott J. D. (2020). Thermal displacement by marine heatwaves. Nature 584, 82–86. doi: 10.1038/s41586-020-2534-z
Jonsen I. D., Grecian W. J., Phillips L., Carroll G., McMahon C., Harcourt R. G., et al. (2023). aniMotum, an R package for animal movement data: Rapid quality control, behavioural estimation and simulation. Methods Ecol. Evol. 14, 806–816. doi: 10.1111/2041-210X.14060
Kappes M. A., Shaffer S. A., Tremblay Y., Foley D. G., Palacios D. M., Robinson P. W., et al. (2010). Hawaiian albatrosses track interannual variability of marine habitats in the North Pacific. Prog. Oceanography 86, 246–260. doi: 10.1016/j.pocean.2010.04.012
Kawakami Y., Nakano H., Urakawa L. S., Toyoda T., Aoki K., Usui N. (2023). Northward shift of the Kuroshio Extension during 1993–2021. Sci. Rep. 13, 16223. doi: 10.1038/s41598-023-43009-w
Kobayashi D. R., Polovina J. J., Parker D. M., Kamezaki N., Cheng I.-J., Uchida I., et al. (2008). Pelagic habitat characterization of loggerhead sea turtles, Caretta caretta, in the North Pacific Ocean (1997–2006): Insights from satellite tag tracking and remotely sensed data. J. Exp. Mar. Biol. Ecol. 356, 96–114. doi: 10.1016/j.jembe.2007.12.019
Le C., Wu S., Hu C., Beck M. W., Yang X. (2019). Phytoplankton decline in the eastern North Pacific transition zone associated with atmospheric blocking. Global Change Biol. 25, 3485–3493. doi: 10.1111/gcb.v25.10
Lezama-Ochoa N., Brodie S., Welch H., Jacox M. G., Pozo Buil M., Fiechter J., et al. (2024). Divergent responses of highly migratory species to climate change in the California Current. Diversity Distributions 30, e13800. doi: 10.1111/ddi.13800
Mariani G., Bellucci F., Cocumelli C., Raso C., Hochscheid S., Roncari C., et al. (2023). Dietary preferences of Loggerhead Sea Turtles (Caretta caretta) in two Mediterranean Feeding grounds: does Prey Selection Change with Habitat Use throughout their life cycle? Animals 13, 654. doi: 10.3390/ani13040654
Maxwell S. M., Hazen E. L., Lewison R. L., Dunn D. C., Bailey H., Bograd S. J., et al. (2015). Dynamic ocean management: Defining and conceptualizing real-time management of the ocean. Mar. Policy 58, 42–50. doi: 10.1016/j.marpol.2015.03.014
McHenry J., Welch H., Lester S. E., Saba V. (2019). Projecting marine species range shifts from only temperature can mask climate vulnerability. Global Change Biol. 25, 4208–4221. doi: 10.1111/gcb.v25.12
Nichols W. J., Resendiz A., Seminoff J. A., Resendiz B. (2000). Transpacific migration of a loggerhead turtle monitored by satellite telemetry. Bull. Mar. Sci. 67, 937–947.
NOAA F. (2021). Protected Resources Regulations and Actions: Listing Loggerhead Sea Turtle Under the ESA. Available online at: https://www.fisheries.noaa.gov/action/listing-loggerhead-sea-turtle-under-esa (Accessed July 24, 2024).
NOAA. (2024). NOAA Coral Reef Watch Version 3.1, NOAA Coral Reef Watch. Available online at: https://coralreefwatch.noaa.gov/.
NOAA Sea Turtle Stranding Network. (2024). Available online at: https://www.fisheries.noaa.gov/national/marine-life-distress/sea-turtle-stranding-and-salvage-network.
Oliver E. C., Perkins-Kirkpatrick S. E., Holbrook N., Bindoff N. (2018). Anthropogenic and natural influences on record 2016 marine heat waves. Bulletin of the American Meteorological Society 99, S44–S48. doi: 10.1175/BAMS-D-17-0093.1
Osland M. J., Stevens P. W., Lamont M. M., Brusca R. C., Hart K. M., Waddle J. H., et al. (2021). Tropicalization of temperate ecosystems in North America: The northward range expansion of tropical organisms in response to warming winter temperatures. Global Change Biol. 27, 3009–3034. doi: 10.1111/gcb.v27.13
Parker D., Girdlestone C., Akhurst L., Haulena M., Balazs G. (2024). Summary of Prey Items from Fecal Sample Collected from a Stranded Loggerhead turtle, Vancouver Island, Canada. 1–7. Unpublished.
Parker D. M., Cooke W. J., Balazs G. H. (2005). Diet of oceanic loggerhead sea turtles (Caretta caretta) in the central North Pacific. 2005. Fishery Bull. 103, 142–152.
Peckham S. H., Maldonado-Diaz D., Tremblay Y., Ochoa R., Polovina J., Balazs G., et al. (2011). Demographic implications of alternative foraging strategies in juvenile loggerhead turtles Caretta caretta of the North Pacific Ocean. Mar. Ecol. Prog. Ser. 425, 269–280. doi: 10.3354/meps08995
Pecl G. T., Araújo M. B., Bell J. D., Blanchard J., Bonebrake T. C., Chen I.-C., et al. (2017). Biodiversity redistribution under climate change: Impacts on ecosystems and human well-being. Science 355, eaai9214. doi: 10.1126/science.aai9214
Pinsky M. L., Selden R. L., Kitchel Z. J. (2020). Climate-driven shifts in marine species ranges: scaling from organisms to communities. Annu. Rev. Mar. Sci. 12, 153–179. doi: 10.1146/annurev-marine-010419-010916
Plotkin P., Wicksten M. K., Amos A. E. (1993). Feeding ecology of the loggerhead sea turtle Caretta in the Northwestern Gulf of Mexico. Mar. Biol. 115, 1–15. doi: 10.1007/BF00349379
Poloczanska E. S., Brown C. J., Sydeman W. J., Kiessling W., Schoeman D. S., Moore P. J., et al. (2013). Global imprint of climate change on marine life. Nat. Climate Change 3, 919–925. doi: 10.1038/nclimate1958
Poloczanska E. S., Burrows M. T., Brown C. J., García Molinos J., Halpern B. S., Hoegh-Guldberg O., et al. (2016). Responses of marine organisms to climate change across oceans. Front. Mar. Sci. 3, 62. doi: 10.3389/fmars.2016.00062
Polovina J., Uchida I., Balazs G., Howell E. A., Parker D., Dutton P. (2006). The Kuroshio Extension Bifurcation Region: a pelagic hotspot for juvenile loggerhead sea turtles. Deep Sea Res. Part II: Topical Stud. Oceanography 53, 326–339. doi: 10.1016/j.dsr2.2006.01.006
Polovina J. J., Balazs G. H., Howell E. A., Parker D. M., Seki M. P., Dutton P. H. (2004). Forage and migration habitat of loggerhead (Caretta caretta) and olive ridley (Lepidochelys olivacea) sea turtles in the central North Pacific Ocean. Fisheries Oceanography 13, 36–51. doi: 10.1046/j.1365-2419.2003.00270.x
Polovina J. J., Dunne J. P., Woodworth P. A., Howell E. A. (2011). Projected expansion of the subtropical biome and contraction of the temperate and equatorial upwelling biomes in the North Pacific under global warming. ICES J. Mar. Sci. 68, 986–995. doi: 10.1093/icesjms/fsq198
Polovina J. J., Howell E. A., Abecassis M. (2008). Ocean's least productive waters are expanding. Geophysical Res. Lett. 35. doi: 10.1029/2007GL031745
Polovina J. J., Howell E., Kobayashi D. R., Seki M. P. (2001). The transition zone chlorophyll front, a dynamic global feature defining migration and forage habitat for marine resources. Prog. oceanography 49, 469–483. doi: 10.1016/S0079-6611(01)00036-2
Polovina J. J., Howell E. A., Kobayashi D. R., Seki M. P. (2017). The transition zone chlorophyll front updated: advances from a decade of research. Prog. Oceanography 150, 79–85. doi: 10.1016/j.pocean.2015.01.006
Polovina J. J., Kobayashi D. R., Parker D. M., Seki M. P., Balazs G. H. (2000). Turtles on the edge: movement of loggerhead turtles (Caretta caretta) along oceanic fronts, spanning longline fishing grounds in the central North Pacific, 1997–1998. Fisheries Oceanography 9, 71–82. doi: 10.1046/j.1365-2419.2000.00123.x
Ramirez Q. (2024). Cold-stunned loggerhead turtle found in Oregon now recovering in Washington. Available online at: https://www.king5.com/.
Santos B. S., Hazen E. L., Welch H., Lezama-Ochoa N., Block B. A., Costa D. P., et al. (2024). Beyond boundaries: governance considerations for climate-driven habitat shifts of highly migratory marine species across jurisdictions. NPJ Ocean Sustainability 3, 22. doi: 10.1038/s44183-024-00059-5
Seminoff J. A., Eguchi T., Carretta J., Allen C. D., Prosperi D., Rangel R., et al. (2014). Loggerhead sea turtle abundance at a foraging hotspot in the eastern Pacific Ocean: implications for at-sea conservation. Endangered Species Res. 24, 207–220. doi: 10.3354/esr00601
Simpson G. L. (2018). Modelling palaeoecological time series using generalised additive models. Front. Ecol. Evol. 6, 149. doi: 10.3389/fevo.2018.00149
Smith K. E., Burrows M. T., Hobday A. J., King N. G., Moore P. J., Sen Gupta A., et al. (2023). Biological impacts of marine heatwaves. Annu. Rev. Mar. Sci. 15, 119–145. doi: 10.1146/annurev-marine-032122-121437
Sydeman W. J., Thompson S. A., Santora J. A., Koslow J. A., Goericke R., Ohman M. D. (2015). Climate–ecosystem change off southern California: time-dependent seabird predator–prey numerical responses. Deep Sea Res. Part II: Topical Stud. Oceanography 112, 158–170. doi: 10.1016/j.dsr2.2014.03.008
Thorne L., Conners M., Hazen E., Bograd S., Antolos M., Costa D., et al. (2016). Effects of El Niño-driven changes in wind patterns on North Pacific albatrosses. J. R. Soc. Interface 13, 20160196. doi: 10.1098/rsif.2016.0196
Tittensor D. P., Mora C., Jetz W., Lotze H. K., Ricard D., Berghe E. V., et al. (2010). Global patterns and predictors of marine biodiversity across taxa. Nature 466, 1098–1101. doi: 10.1038/nature09329
Tomaszewicz C. N. T., Seminoff J. A., Avens L., Goshe L. R., Peckham S. H., Rguez-Baron J. M., et al. (2015). Age and residency duration of loggerhead turtles at a North Pacific bycatch hotspot using skeletochronology. Biol. Conserv. 186, 134–142. doi: 10.1016/j.biocon.2015.03.015
Vanselow K. A., Zandler H., Samimi C. (2021). Time series analysis of land cover change in dry mountains: insights from the Tajik Pamirs. Remote Sens. 13, 3951. doi: 10.3390/rs13193951
Welch H., Hazen E. L., Briscoe D. K., Bograd S. J., Jacox M. G., Eguchi T., et al. (2019). Environmental indicators to reduce loggerhead turtle bycatch offshore of Southern California. Ecol. Indic. 98, 657–664. doi: 10.1016/j.ecolind.2018.11.001
Welch H., Savoca M. S., Brodie S., Jacox M. G., Muhling B. A., Clay T. A., et al. (2023). Impacts of marine heatwaves on top predator distributions are variable but predictable. Nat. Commun. 14, 5188. doi: 10.1038/s41467-023-40849-y
Whitney F. A. (2015). Anomalous winter winds decrease 2014 transition zone productivity in the NE Pacific. Geophysical Res. Lett. 42, 428–431. doi: 10.1002/2014GL062634
Wingfield D. K., Peckham S. H., Foley D. G., Palacios D. M., Lavaniegos B. E., Durazo R., et al. (2011). The making of a productivity hotspot in the coastal ocean. PloS One 6, e27874. doi: 10.1371/journal.pone.0027874
Xu Y., Nieto K., Teo S. L., McClatchie S., Holmes J. (2017). Influence of fronts on the spatial distribution of albacore tuna (Thunnus alalunga) in the Northeast Pacific over the past 30 years (1982–2011). Prog. Oceanography 150, 72–78. doi: 10.1016/j.pocean.2015.04.013
Yang H., Lohmann G., Krebs‐Kanzow U., Ionita M., Shi X., Sidorenko D., et al. (2020). Poleward shift of the major ocean gyres detected in a warming climate. Geophysical Res. Lett. 47, e2019GL085868. doi: 10.1029/2019GL085868
Keywords: North Pacific Ocean, loggerhead sea turtle, sea surface temperature, habitat, climate change, Transition Zone
Citation: Briscoe DK, Crowder LB, Balazs GH, Seminoff JA, Abreu A, Lee Hing CA, Kurita M, Mori M, Parker DM, Rice MR, Saito T, Santos BS, Turner Tomaszewicz CN, Yamaguchi N and Polovina JJ (2025) Multi-decade northward shift of loggerhead sea turtle pelagic habitat as the eastern North Pacific Transition Zone becomes more oligotrophic. Front. Mar. Sci. 11:1513162. doi: 10.3389/fmars.2024.1513162
Received: 18 October 2024; Accepted: 06 December 2024;
Published: 22 January 2025.
Edited by:
Mariana M. P. B. Fuentes, Florida State University, United StatesReviewed by:
Dmitry Lajus, Independent Researcher, Saint Petersburg, RussiaKristen Marie Hart, United States Geological Survey (USGS), United States
Copyright © 2025 Briscoe, Crowder, Balazs, Seminoff, Abreu, Lee Hing, Kurita, Mori, Parker, Rice, Saito, Santos, Turner Tomaszewicz, Yamaguchi and Polovina. This is an open-access article distributed under the terms of the Creative Commons Attribution License (CC BY). The use, distribution or reproduction in other forums is permitted, provided the original author(s) and the copyright owner(s) are credited and that the original publication in this journal is cited, in accordance with accepted academic practice. No use, distribution or reproduction is permitted which does not comply with these terms.
*Correspondence: Dana K. Briscoe, ZGJyaXNjb2VAc3RhbmZvcmQuZWR1