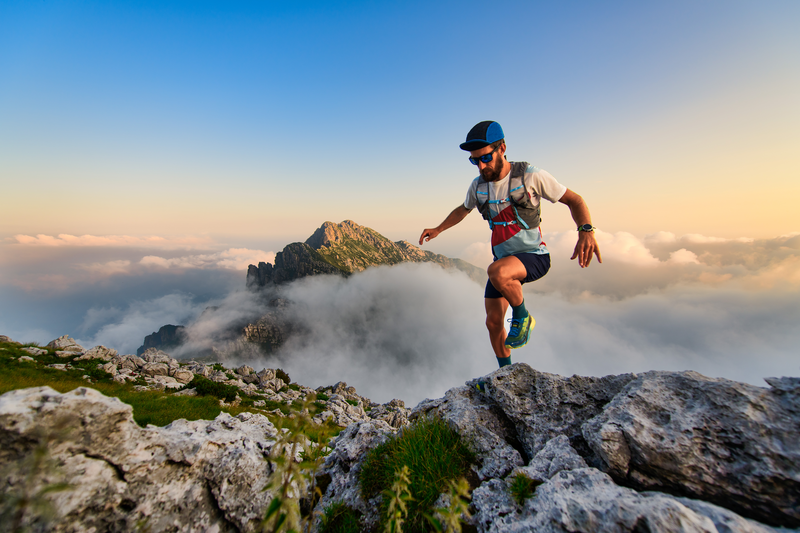
94% of researchers rate our articles as excellent or good
Learn more about the work of our research integrity team to safeguard the quality of each article we publish.
Find out more
ORIGINAL RESEARCH article
Front. Mar. Sci. , 13 January 2025
Sec. Marine Fisheries, Aquaculture and Living Resources
Volume 11 - 2024 | https://doi.org/10.3389/fmars.2024.1511121
Understanding seasonal variations in fish communities is important for fishery resources conservation and management in fishery economic zones. However, there are still knowledge gaps regarding to the differential responses of fish functional traits to various disturbances. Here, we sampled fish communities of 20 sites during autumn (November 2023) and spring (April 2024) in Zhoushan Fishing Ground. Taxonomic diversity (TD), functional diversity (FD), and functional redundancy (FR) were calculated to investigate the state of the fish communities and their seasonal changes. Beta diversity was calculated based on the pairwise communities of spring and autumn and decomposed into turnover and nestedness proportions, to investigate the dissimilarities of the fish communities between the two seasons and their drive mechanisms. We also applied R-mode linked to Q-mode (RLQ) and fourth-corner methods to test the relationships between fish functional traits and environmental variables (trait-environment relationships). These results showed that the mean values of taxonomic and functional beta diversities were 0.852 and 0.769, respectively, which indicate that taxonomic and functional structures of the fish communities have high dissimilarities between spring and autumn. However, the taxonomic and functional structures showed different ecological processes of seasonal changes with the former were mainly driven by species turnover (occupied 89.9% of the total dissimilarity) and the latter were driven by both functional turnover and nestedness (occupied 57.9% and 42.1% of the total dissimilarity, respectively). Species occurrence, environmental variables, and functional traits matrices were closely correlated, which mainly result from the significant trait-environmental relationships. Combining with TD, FD, and FR, we concluded that the fish communities in autumn had higher resistance to environmental changes than in spring. Our findings are accepted to serve as a basis for fishery management.
Understanding the temporal dynamics of biocommunities is a foundational topic in community ecology (Rull, 2012). On short-term scales, studying the seasonal variations in fish community structures and their ecological processes and drivers can provide guidelines for biological conservation and fishery resource management (Galib et al., 2016). Several methods for describing biological community structures have been recorded in the literatures and have been extended from early taxonomic structures to functional structures (Lai et al., 2022; Yuan et al., 2024). Numerous taxonomic structure indicators of biocommunities can also be applied to measure their functional structure. For example, functional alpha diversity is derived from the calculation principle of taxonomic alpha diversity, which is used to describe the heterogeneity of interspecific functional traits within a community (Mammola et al., 2020). Moreover, beta diversity can also be computed with the basis of species occurrence and biological functional traits to describe the dissimilarity of pairwise communities in their taxonomic and functional structures (Villéger et al., 2013). However, both alpha and beta diversities were studied based on taxonomic level of organisms in several previous studies because of shortage in data of functional traits and tools of algorithms (Li et al., 2018; Cunha et al., 2019). Recently, considering that the functional traits of organisms largely control their responses to environmental disturbances (He et al., 2022), a growing number of researchers have investigated community dynamics simultaneously used species- and trait-based approaches to capture comprehensive information about community dynamics (Li et al., 2023; Yuan et al., 2024).
Against the background of the sustained decline in biodiversity due to natural and anthropogenic disturbances, quantifying the ecological processes that drive changes in biocommunities has become a key issue in conservation biology (Chase, 2003). Numerous existing studies have focused on species gains and/or losses, which result in community reorganization and increases or declines in species number (Liu et al., 2023; Coulon et al., 2024). Baselga (2010) decomposed beta diversity into turnover and nestedness proportions to describe the above processes of changes in biocommunities (i.e., species gains and/or losses). However, different species occupy different ecological niches in ecosystems because of variations in their functional traits (Miller et al., 2023). Therefore, species gains and/or losses, which are the processes of changes in the taxonomic structure of biocommunities, inevitably cause changes in their functional structure (Halpern and Floeter, 2020). Accordingly, beta diversity calculated based on functional traits can also be decomposed into the above two components to describe the ecological processes of changes in functional structures of biocommunities (Villéger et al., 2013). For instance, Castro et al. (2023) revealed that both taxonomic and functional beta diversities of the fish communities in continental Chile declined throughout their history (pre-European) to their current composition. The decline in taxonomic beta diversity included species gains and losses. The species with gains were mainly six exotic Salmonidae with similar functional traits, and those with losses were endangered and vulnerable native species with unique functional traits, which caused the functional nestedness proportion was higher than the functional turnover proportion.
Although the ecological processes of turnover and nestedness jointly regulate biocommunity structure, their relative importance largely depends on species composition and species-environment relationships (He et al., 2022). How organisms respond to environmental changes have been demonstrated to be determined by their functional traits because traits characterize the ecological roles played by organisms and interactions between species and environmental variables (Violle et al., 2009). Therefore, trait–based approaches can reveal community assembling mechanisms and provide better understanding of community dynamics and their ecological processes than taxonomic methods (White et al., 2018). Recently, more and more studies profoundly revealed the spatiotemporal dynamics of fish communities and their driving mechanisms in various ecosystems by using trait-based approaches; these works have largely contributed to biological conservation and fishery resource management in their localities (Barnett et al., 2019; Sarremejane et al., 2020).
Zhoushan Fishing Ground (ZFG) is an important fishery economic zone in China and has rich species (Zhou et al., 2013). In recent few decades, the area has undergone multiple pressures, such as aquaculture, climate change, and other natural and anthropogenic disturbances, resulting in a serious decline in its fishery resources (Ni and Lu, 2003; Wang et al., 2023). In order to maintain and restore the fishery resources in the ZFG, a series of fishery management policies have been sequentially proposed, including the most famous “summer moratorium of marine fishing”. The seasonal patterns of fish communities are an important basis for fishery resource evaluation and ecosystem-based fishery management (Luo et al., 2024). However, although several studies have revealed the seasonal variations in the fish communities by using taxonomic methods, how their functional structure change with seasons is still unclear. In addition, to the best of our knowledge, there were no studies on the processes of seasonal changes in the fish communities. The knowledge gap seriously hinder the ecosystem-based fishery management in the study area. Therefore, a thorough knowledge of seasonal variations in fish communities in the ZFG is urgently required.
Here, we calculated taxonomic fiversity (TD), functional diversity (FD), and functional redundancy (FR) of the fish communities in the ZFG and tested their changes between autumn 2023 and spring 2024. We investigated the ecological processes of the seasonal changes in the fish communities by beta diversity and its turnover and nestedness proportions for the pairwise communities between spring and autumn. In addition, we explored the trait-environment relationships by using RLQ and fourth-corner methods. Given that the seasonal dynamics of the species and functional trait composition in the ZFG which demonstrated by Li et al. (2023), we hypothesized that TD and FD would be significantly different between spring and autumn, while FR would be determined by the functional traits of gain and loss species between the two seasons. Given that there are interspecific differences of functional traits of fishes (Miller et al., 2023), we hypothesized that the taxonomic and functional structures would be change with seasons via different ecological processes. The results are expected to reveal seasonal variations in the fish communities and their drivers in the ZFG to provide information for fishery management planning.
The ZFG is located in the northwest of the East China Sea from 29.5°N to 31° N and 120.5°E to 125° E with a sea area of approximately 10 800 km2. It provides feeding and spawning grounds for numerous economically important fishes, shrimps and crabs, serving as the largest fishing ground in China. During autumn (November 2023) and spring (April 2024), 20 sites were set and surveyed in the ZFG (Figure 1). Sampling in the two surveys was conducted through traditional trawl fishing (width, 50 m; mesh size, 160 mm) on the fishing vessel Zhe Pu Yu No. 30097 with the same fishing effort (3 knots for 1 h at each site). The analysis and processing of biological samples were conducted according to “the Marine Survey Specification—Part 6: Marine Biological Survey”.
Nine functional traits of fishes, including habitat use, reproductive trait, and life history, were collected (Table 1). The functional traits of habitat use included mean temperature preference (MTP) and max depth (MD), which are associated with habitat preference and capture the responses of fishes to temperature changes (Freitas et al., 2021). Reproductive traits, including length at maturity (LM), age at maturity (AM), and generation time (GT), are associated with population dynamics and affect the resilience of fishes to biotic and abiotic stressors (Lowerre-Barbieri et al., 2011). Life history traits, including growth coefficient (K), Q/B, life span (LS), and trophic level (TL), are associated with energy conversion, interspecific interactions, and environmental adaptability (Petrik, 2019). The data of the fish functional traits were extracted from FishBase (https://figshare.com/s/72c681c513d4435eed0f).
Five environmental variables, including water temperature (℃), chlorophyll-a concentration (Chl-a, mg/m3), dissolved oxygen (DO, mg/L), salinity (psu), and conductivity (ms/cm), were selected to study the impacts of abiotic factors on the fish communities. Water temperature, DO, salinity, and conductivity were measured using Conductivity-Temperature-Depth (CTD) during in situ surveys. Chl-a data were derived from Copernicus-Marine-Service (https://resources.marine.copernicus.eu/), and were extracted by using ArcGIS 10.7 (Esri, the USA).
Functional space was established with the basis of species occurrence and functional traits data. It was measured as the maximum volume of a convex hull based on species distribution (Villéger et al., 2008). In order to ensure the accuracy of functional space, Principal coordinate analysis (PCA) was firstly conducted on all functional traits of fishes (Maire et al., 2015). Subsequently, the first three PCA axes were selected to establish the functional space (a three-dimensional space) because they explained the majority of the variance in functional traits (78.4%). Given that species number must exceed functional traits number to establish a functional space successfully, only communities with species number > 3 were retained.
FR can be measured using the formula FR = SD - FD (de Bello et al., 2007). SD represents the number and distribution regularity of species within a community, and is known as Simpson index. The FD index represents the dissimilarities of functional traits among species, and computed by the formula from de Bello et al. (2011). Functional space and FD were calculated by using the “FD” package in R 4.2.0.
Beta diversity often used to measure the dissimilarity between two communities, and can be further decomposed into two opposite components (that is turnover and nestedness) to reflect the ecological processes of changes in biocommunities (Novillo and Ojeda, 2020). In addition, beta diversity can be computed with the basis of species occurrence and functional traits data, named taxonomic beta diversity (T.jac) and functional beta diversity (F.jac), respectively. T.jac and its turnover (T.jtu) and nestedness (T.jne) proportions were computed by using the method from Baselga (2010). A high T.jac value indicates that pairwise communities have low similarity in terms of taxonomic structure. By contrast, a low T.jac value indicates that pairwise communities share numerous species. However, T.jac cannot reflects the drivers of the variations in pairwise communities. Such drivers can be captured by T.jtu and T.jne. A high T.jtu value indicates that the difference in pairwise communities is mainly caused by species replacement. A high T.jne value indicates that the difference in pairwise communities is mainly caused by difference in the number of species.
F.jac and its turnover (F.jtu) and nestedness (F.jne) proportions were calculated by using the method from Villéger et al. (2013). In terms of functional structure of biocommunities, the ecological meanings of F.jtu and F.jne are corresponding to the T.jtu and T.jne, respectively. All indices in this section were computed by using the “betapart” package in R 4.2.0.
Paired t-test was applied to analyze the significant differences of environmental variables, TD (measured as species richness), FD, and FR between spring and autumn. The statistical result of p < 0.05 was accepted as a statistical meaning. Before the t-test was conducted, all data of the environmental variables and diversity indices were subjected to log10(X + 1) transformation to minimize the effect of outliers as well as satisfy the multivariate normality assumptions. This section was conducted by using the SPSS 21.0 software.
RLQ and fourth-corner methods were applied to investigate the trait-environment relationships. These methods were conducted on the basis of three matrices, including the species occurrence matrix (L), environmental variable matrix (R), and functional trait matrix (Q). RLQ was firstly used to test the associations among R, L, and Q. In running process of RLQ, random permutation models 2 and 4 were repeated 9999 times to test the null hypothesis (i.e., no association existed between Q and R). Statistical significance was accepted only when the results of models 2 and 4 were p < 0.05. Secondly, conducting fourth-corner method to analyze whether the first two axes of RLQ have significant correlations with specific environmental variables and functional traits. The section was conducted by using “ade4” package in R (Dray and Dufour, 2007). All data were standardized by method of “min-max normalization” to eliminate the influence of dimensions.
All five environmental variables showed significant differences between spring and autumn. In specific, water temperature and Chl-a were significant higher in spring than that values in autumn, while DO, salinity, and conductivity were significant lower in spring than that values in autumn (Table 2).
In the ZFG, a total of 106 species were captured, of which 70 and 87 species were captured in spring 2024 and autumn 2023, respectively (Supplementary Table S1). There were 51 species shared between the two seasons, 19 and 36 species were only appeared in spring and autumn, respectively. The volume of the functional space established by all fishes in spring was higher than that in autumn, with values of 51.28 and 33.13, respectively (Figure 2). In the functional space of the ZFG established by the first three axis of PCA analysis based on functional traits of all fishes in both two surveys, the regularity of species distribution were similar in spring and autumn (Figure 2).
Figure 2. Species distribution in the three-dimensional functional space established with the basis of the first three PCA axes of fish functional traits. The green and orange balls in (A, B) indicate species occurred in spring and autumn, respectively. The grey balls in (A, B) indicate species occurred in autumn and spring, respectively.
TD and FR showed significant difference between spring and autumn (Figures 3A, C). Compared to spring, TD and FR have significantly increased in autumn (TD: spring, 13 ± 8.4; autumn, 19 ± 8.3; FR: spring, 0.51 ± 0.13; autumn, 0.64 ± 0.09). FD showed no significant difference between the two seasons, but the mean value in autumn is slightly higher than that in spring (Figure 3B).
Figure 3. TD (A), FD (B), and FR (C) of the fish communities in the ZFG in autumn and spring. ns: p > 0.05, *: p < 0.05, **: p < 0.01.
The mean values of T.jac, T.jtu, and T.jne are 0.852, 0.766, and 0.086, respectively (Figure 4A). The results indicated that taxonomic structures in spring and autumn have high dissimilarity. Change in taxonomic structures between spring and autumn was mainly driven by species turnover which occupied 89.9% of the total dissimilarity, while species nestedness only occupied 10.1% of the total dissimilarity.
Figure 4. Triangle plots describe T.jac (A) and F.jac (B) of all pairs of communities between spring and autumn and their turnover (T.jtu, F.jtu) and nestedness (T.jne, F.jne) proportions.
In terms of seasonal changes in functional structure, the mean values of F.jac, F.jtu, and F.jne are 0.769, 0.445, and 0.324, respectively (Figure 4B). According to the formula of the indices, the functional spaces of fish communities in spring and autumn have high dissimilarity. The functional space turnover and nestedness have similar contributions to the changes of functional space between spring and autumn, with 57.9% and 42.1% of the total dissimilarity, respectively.
RLQ analysis results revealed significant relationships among L, R, and Q, with p < 0.05 for both models 2 and 4. The first two RLQ axes explained the major section of the trait-environment relationships with 96.82% of the total co-inertia, in especial Axis 1 which explained 89.33% (Table 3). In the first RLQ axis, the negative part indicates that LM, TL, MD, GT, LS, and AM (Figure 5D) associated with DO, salinity and conductivity (Figure 5C) corresponded to the species Lophius litulon, Conger myriaster, Bregmaceros mcclellandi, Hoplobrotula armata, Pagrus major, Pseudorhombus arsius, Pseudechidna brummeri, Cynoglossus joyneri, and Cynoglossus abbreviatus (Figure 5B), which preferentially inhabit spring (Figure 5A). The positive part indicates that K, MTP, and Q/B (Figure 5D) associated with water temperature and Chl-a (Figure 5C) corresponded to the species Saurida elongata, Harpadon nehereus, Hippocampus kelloggi, Johnius grypotus, Repomucenus olidus, Parapercis sexfasciata, Stolephorus chinensis, and Apogonichthys ocellatus, which preferentially inhabit autumn (Figure 5A).
Figure 5. Summary of the first two axes of RLQ analysis: (A), scores of sites, (B), scores of species, (C), coefficients of environmental variables, and (D), functional traits of fishes. The d value in the upper right corner in each small graph indicates its grid size. Ai and Bi in (A) are the sampling sites in spring and autumn, respectively. DO: dissolved oxygen, Chl-a: chlorophyll-a concentration. The meaning of the letters in (D) are listed in Table 1.
Further, fourth-corner analysis was applied to exam the correlations between single functional trait and the two RLQ axes of environmental variables, and single environmental variable and the two RLQ axes of fish functional traits, respectively. Results indicate that the first RLQ functional axis has significant positive correlations with water temperature, and has significant negative correlations with salinity and DO (p < 0.05) (Supplementary Figure S1). AM, LS and GT have significant negative correlations with the first RLQ environmental axis (p < 0.05) (Supplementary Figure S1). In terms of Axis 2, there were no significant associations were found for both environmental variables and fish functional traits. Significantly associated functional traits with higher water temperature were lower relative AM, LS and GT (Figure 6). Functional traits associated with higher salinity was relative higher AM. Functional traits associated with higher DO were higher AM, LS and GT.
Figure 6. The fourth-corner analysis results. Blue and red cells represent the significant negative and positive trait-environment relationships, respectively. DO: dissolved oxygen, Chl-a: chlorophyll-a concentration. Upper letters represent the functional traits of fishes and can be seen in Table 1.
In this work, we found that in the ZFG, TD and FR significantly differed between spring 2024 and autumn 2023, but FD did not significantly differ between the two seasons. The seasonal changes in diversity indices result from the seasonal changes in fish community structures which are a universal phenomena in various ecosystems (Galib et al., 2016; Li et al., 2023). Numerous biotic and abiotic drivers, including variations in physio-ecological characteristics of living things and environmental gradients, cause seasonal dynamics of fish communities (Loher and Seitz, 2006; Lai et al., 2022). In some fishery economic zones, seasonal fishing is another important driver of the seasonal patterns of the fish communities (Xing et al., 2020). Notably, TD and FD changed differently with seasons in this study. Consistent with our result, some studies also found that TD and FD respond differently to natural and anthropogenic disturbances and often exhibit different spatiotemporal dynamics (Li et al., 2023; Yuan et al., 2024). Their results may be attributed to the inconsistent processes of TD and FD in fish assembly (Cilleros et al., 2016). Given that the species-environmental relationships are dependent on the functional traits of species, FD is always highly sensitive to environmental changes (Kendzierska and Janas, 2024). Considering that whether a species can successfully settle in an ecosystem is rely on the species-environmental relationships, TD is closely related to environmental characteristics (Agiadi et al., 2024). Therefore, TD and FD can be considered as the integrated effects of environmental filtering. Importantly, the seasonal pattern of FR was consistent with TD, and both of them were significantly higher in autumn than that in spring. This result suggested that the species that occurred only in autumn had similar functional traits in contrast to the species shared by the two seasons. This finding can also demonstrated by the absence of significant changes in FD. Additionally, although TD in spring was lower than that in autumn, functional space showed opposite seasonal patterns. This result also indicates that species in autumn shared more similar functional traits than those in spring. These results demonstrated that FR and FD lacked necessary connections because of the various functional traits of different fishes (Zeng et al., 2022). In accordance with the ecological meaning of FR, fish communities with high FR values are expected to be influenced negligibly by species loss (Aune et al., 2018). In addition, it is widely believed that FR is closely positively related to ecosystem stability (Biggs et al., 2020). Given the high FR in autumn 2023, we propose that in the ZFG, the fish community in autumn has higher resistance to environmental changes than that in spring.
Studing the various aspects of beta diversity can deeply promotes our insights into the intricate processes of the changes in fish communities (Novillo and Ojeda, 2020). The mean values of T.jac and F.jac in the ZFG were high, indicating that the taxonomic and functional structures substantially differed between spring and autumn. Figure 4 illustrates that the dissimilarity of the taxonomic structure between any pairwise communities of spring and autumn concentrated in the part of larger T.jtu, whereas functional beta diversity showed a disperse distribution. These results suggested that the dissimilarity of the taxonomic structures between spring and autumn was mainly driven by species turnover (the process contains both species gain and loss with similar number), whereas that of functional structures was driven by both functional space turnover and expansion or contraction between the two seasons (Villéger et al., 2013). In the ZFG, we identified 21 and 36 species occurred only in spring and autumn, respectively. Some seasonally reproductive species cyclically migrate into or out the area in specific seasons (Goertler et al., 2021). Other species only appear in one season, possibly for foraging or overwintering needs (Chen, 2022). However, species replacement did not result in an equal degree of functional space replacement, with T.jtu accounting for 89.9% of T.jac and F.jtu only accounting for 57.9% of F.jac. These results indicated that approximately half of the species involved in turnover had similar functional trait values. Beta diversity indices are effective for deeply understanding the mechanisms of biocommunity construction and have implications for predicting the future processes of biotic homogenization (Castro et al., 2023). By combining beta diversity and its turnover and nestedness proportions, TD, FD, and FR indices, we found complex relationships between fish communities in spring 2024 and autumn 2023, which can provide important guidelines for biodiversity sustainable management in the ZFG.
RLQ and fourth-corner analyses results revealed that close correlations among L, R, and Q. Species gain or loss is associated with their functional traits and environmental changes (He et al., 2022). In our study, all five environmental variables exhibited significant changes between spring 2024 and autumn 2023. If environmental changes exceed the tolerance ranges of a species, the species will no longer survive in the area. In the meanwhile, other suitable species may migrate into and resettle in the area because of environmental filtering (Kraft et al., 2015). Therefore, fish community assembly and their responses to natural and anthropogenic pressures are mainly determined by the trait-environmental relationships (Baptista et al., 2021).
Additionally, the ordination of biotic and abiotic variables in the two-dimensional coordinate system based on the first two RLQ analyses indicated that water temperature, salinity and DO are important variables influencing functional structure of the fish communities. According to previous studies, functional structure of biocommunities is tightly related to the trait-environment relationships (Araújo et al., 2024). In this study, high LM was significantly correlated with low water temperature and high DO and salinity, as well as the presence of species in spring. LM is a common index of sexual maturity characteristics and is often used as an important basis for determining the minimum allowable length for fishing (Stark, 2012). A high LM value indicates that a species has a large individual size and is often categorized as a cold-water species (Fontoura et al., 2009). This finding may account for the significant negative relationship between LM and water temperature. A higher Q/B value was significantly correlated with low water temperature and high DO, as well as the presence of species in autumn. Q/B is an index of trophic interaction and can be used to describe food consumption by a species (Wiff et al., 2017). The natural phenomenon where species in low water temperature areas often require more food intake to maintain their functions may explain the negative relationship between Q/B and water temperature. Within a certain range, high DO can promote the metabolism of organisms, thus accounting for the positive relationship between DO and Q/B (Tran-Duy et al., 2011). High TL was significantly correlated with low water temperature and high DO, as well as the presence of species in spring. TL indicates the position of species in food chain, providing information on predator–prey interactions (Pasquaud et al., 2010). Previous studies have demonstrated that increasing water temperature is more favorable to species at low TLs than to those with large body sizes (Daufresne et al., 2009; Lefort et al., 2015). Thus, continued shortening in the food chain and cascading of ecological effects caused by global warming should be emphasized (Guiet et al., 2016). The relationship between TL and DO may be mainly impacted by water temperature, with high water temperatures always accompanied with low DO. In fact, the impacts of environmental changes on organisms are very complex as there are close relationships between different environmental variables, which thereby expanding or compressing the tolerance of some functional traits to environmental changes (Elsdon and Gillanders, 2004). Therefore, to capture more comprehensive the trait-environment relationships in natural ecosystems, the interactive effects of various environmental variables and their collaborative impacts on biological functional traits are needed to further studied.
The study revealed the different variations in the taxonomic and functional structures of the fish communities in the ZFG with seasons, as well as the resulting different seasonal variations in the TD, FD, and FR. Overall, both the taxonomic and functional structures exhibit high dissimilarities between spring and autumn. The variations in the taxonomic structures were mainly caused by species turnover, whereas that in the functional structures were caused by functional turnover and nestedness. Despite the important discoveries that taxonomic and functional structures underwent different changes, further studies on long temporal scales are needed because our data were limited to 2023–2024. The seasonal changes in the fish communities were closely related to the trait-environment relationships. In specific, LM, Q/B, and TL were significantly positively related with DO, and significantly negatively related with water temperature. Given that the FR showed significantly higher in autumn than in spring, we propose that the fish communities in autumn had higher resistance to environmental changes. This study provides substantial insight into the short-term variations in the fish communities in the ZFG, which provide guidelines for fishery management in the study area. In future, we will conduct further research on the long-term dynamics of the fish communities and their ecological processes to reveal the mechanisms of the fish communities assembly in the ZFG.
Data of fishery and environmental variables will be available on reasonable request. Data of fish functional traits are available at http://www.fishbase.org. Requests to access these datasets should be directed to YW,eWluZ2JpbndhbmdAMTI2LmNvbQ==.
YL: Conceptualization, Formal analysis, Funding acquisition, Methodology, Software, Writing – original draft, Writing – review & editing. CY: Data curation, Formal Analysis, Writing – original draft. PJ: Data curation, Resources, Writing – original draft. BL: Data curation, Resources, Writing – original draft. JW: Data curation, Funding acquisition, Writing – review & editing. CZ: Data curation, Resources, Writing – original draft. WZ: Software, Writing – original draft. YW: Funding acquisition, Resources, Writing – review & editing.
The author(s) declare financial support was received for the research, authorship, and/or publication of this article. This work was supported by the Natural Science Foundation of Zhejiang Province, China (ZCLQ24D0601), the General Research Projects of the Zhejiang Provincial Department of Education (Y202353756), the Science and Technology Project of Zhoushan (2022C41003), the Public Welfare Technology Application Research Project of Zhejiang (LGN21C190009), and the National Natural Science Foundation of China (42206203).
The authors declare that the research was conducted in the absence of any commercial or financial relationships that could be construed as a potential conflict of interest.
The author(s) declare that no Generative AI was used in the creation of this manuscript.
All claims expressed in this article are solely those of the authors and do not necessarily represent those of their affiliated organizations, or those of the publisher, the editors and the reviewers. Any product that may be evaluated in this article, or claim that may be made by its manufacturer, is not guaranteed or endorsed by the publisher.
The Supplementary Material for this article can be found online at: https://www.frontiersin.org/articles/10.3389/fmars.2024.1511121/full#supplementary-material
Agiadi K., Hohmann N., Gliozzi E., Thivaiou D., Bosellini F. R., Taviani M., et al. (2024). The marine biodiversity impact of the Late Miocene Mediterranean salinity crisis. Science 385, 986–991. doi: 10.1126/science.adp3703
Araújo F. G., de Azevedo M. C. C., Guedes G. H. S., Pinto B. C. T. (2024). Trait–environment relationship of riverine fish assemblages across a human footprint mosaic. Hydrobiologia 851, 1135–1151. doi: 10.1007/s10750-023-05370-9
Aune M., Aschan M. M., Greenacre M., Dolgov A. V., Fossheim M., Primicerio R. (2018). Functional roles and redundancy of demersal Barents Sea fish: Ecological implications of environmental change. PloS One 13, e0207451. doi: 10.1371/journal.pone.0207451
Baptista J., van der Linden P., Martinho F., Martins R., Carneiro M., Bento E. G., et al. (2021). The functional composition of nearshore fish communities demonstrated by trait analysis: Response to environmental gradients. Mar. pollut. Bull. 169, 112562. doi: 10.1016/j.marpolbul.2021.112562
Barnett L. A. K., Jacobsen N. S., Thorson J. T., Cope J. M. (2019). Realizing the potential of trait-based approaches to advance fisheries science. Fish Fish. 20, 1034–1050. doi: 10.1111/faf.12395
Baselga A. (2010). Partitioning the turnover and nestedness components of beta diversity. Global. Ecol. Biogeogr. 19, 134–143. doi: 10.1111/J.1466-8238.2009.00490.X
Beukhof E., Dencker T. S., Pecuchet L., Lindegren M. (2019). Spatio-temporal variation in marine fsh traits reveals community-wide responses to environmental change. Mar. Ecol. Prog. Ser. 610, 205–222. doi: 10.3354/meps12826
Biggs C. R., Yeager L. A., Bolser D. G., Bonsell C., Dichiera A. M., Hou Z., et al. (2020). Does functional redundancy affect ecological stability and resilience? A review and meta-analysis. Ecosphere 11, e03184. doi: 10.1002/ecs2.3184
Blažek R., Polačik M., Reichard M. (2013). Rapid growth, early maturation and short generation time in African annual fishes. EvoDevo 4, 24. doi: 10.1186/2041-9139-4-24
Castro S. A., Rojas P., Vila I., Jaksic F. M. (2023). Covariation of taxonomic and functional facets of β-diversity in Chilean freshwater fish assemblages: Implications for current and future processes of biotic homogenization. PloS One 18, e0281483. doi: 10.1371/journal.pone.0281483
Chase J. M. (2003). Community assembly: when should history matter? Oecologia 136, 489–498. doi: 10.1007/s00442-003-1311-7
Chen X. (2022). “Shoaling and migration of fish and their relationships with environment,” in Theory and Method of Fisheries Forecasting. Ed. Chen X. (Springer, Singapore), 39–85. doi: 10.1007/978-981-19-2956-4_3
Cilleros K., Allard L., Grenouillet G., Brosse S. (2016). Taxonomic and functional diversity patterns reveal diferent processes shaping European and Amazonian stream fsh assemblages. J. Biogeogr. 43, 1832–1843. doi: 10.1111/jbi.12839
Coulon N., Elliott S., Teichert N., Auber A., McLean M., Barreau T., et al. (2024). Northeast Atlantic elasmobranch community on the move: Functional reorganization in response to climate change. Global Change Biol. 30, e17157. doi: 10.1111/gcb.17157
Cunha E. R., Winemiller K. O., da Silva J. C. B., Lopes T. M., Gomes L. C., Thomaz S. M., et al. (2019). [amp]]alpha; and β diversity of fishes in relation to a gradient of habitat structural complexity supports the role of environmental filtering in community assembly. Aquat. Sci. 81, 38. doi: 10.1007/s00027-019-0634-3
Daufresne M., Lengfellner K., Sommer U. (2009). Global warming benefits the small in aquatic ecosystems. PNAS 106, 12788–12793. doi: 10.1073/pnas.0902080106
de Bello F., Lavorel S., Albert C. H., Thuiller W., Grigulis K., Dolezal J., et al. (2011). Quantifying the relevance of intraspecific trait variability for functional diversity. Methods Ecol. Evol. 2, 163–174. doi: 10.1111/j.2041-210X.2010.00071.x
de Bello F., Leps J., Lavorel S., Moretti M. (2007). Importance of species abundance for assessment of trait composition: an example based on pollinator communities. Community Ecol. 8, 163–170. doi: 10.1556/ComEc.8.2007.2.3
Dray S., Dufour A.-B. (2007). The ade4 package: implementing the duality diagram for ecologists. J. Stat. Software 22, 1–20. doi: 10.18637/jss.v022.i04
Elsdon T. S., Gillanders B. M. (2004). Fish otolith chemistry influenced by exposure to multiple environmental variables. J. Exp. Mar. Biol. Ecol. 313, 269–284. doi: 10.1016/j.jembe.2004.08.010
Fontoura N. F., Braun A. S., Milani P. C. C. (2009). Estimating size at first maturity (L50) from gonadosomatic index (GSI) data. Neotrop. Ichthyol. 7, 217–222. doi: 10.1590/S1679-62252009000200013
Freitas C., Villegas-Ríos D., Moland E., Olsen E. M. (2021). Sea temperature effects on depth use and habitat selection in a marine fish community. J. Anim. Ecol. 90, 1787–1800. doi: 10.1111/1365-2656.13497
Froese R., Pauly D. Eds. (2014). FishBase. World Wide Web electronic publication. Available online at: www.fshbase.org.version (Accessed February, 2024).
Galib S. M., Rashid M. A., Chaki N., Mohsin A. B. M., Joadder M. A. R. (2016). Seasonal variation and community structure of fishes in the Mahananda River with special reference to conservation issues. J. Fish. 4, 325–334. doi: 10.17017/j.fish.110
Goertler P., Mahardja B., Sommer T. (2021). Striped bass (Morone saxatilis) migration timing driven by estuary outflow and sea surface temperature in the San Francisco Bay-Delta, California. Sci. Rep-UK. 11, 1510. doi: 10.1038/s41598-020-80517-5
Guiet J., Aumont O., Poggiale J.-C., Maury O. (2016). Effects of lower trophic level biomass and water temperature on fish communities: A modelling study. Prog. Oceanogr. 146, 22–37. doi: 10.1016/J.POCEAN.2016.04.003
Halpern B. S., Floeter S. R. (2020). Functional diversity responses to changing species richness in reef fish communities. Mar. Ecol. Prog. Ser. 364, 147–156. doi: 10.3354/meps07553
He S., Wang B., Chen K., Soininen J. (2022). Patterns in aquatic metacommunities are associated with environmental and trait heterogeneity. Freshw. Biol. 68, 91–102. doi: 10.1111/fwb.14011
Kendzierska H., Janas U. (2024). Functional diversity of macrozoobenthos under adverse oxygen conditions in the southern Baltic Sea. Sci. Rep-UK. 14, 8946. doi: 10.1038/s41598-024-59354-3
Kraft N. J. B., Adler P. B., Godoy ó., James E. C., Fuller S., Levine J. M., et al. (2015). Community assembly, coexistence and the environmental filtering metaphor. Funct. Ecol. 29, 592–599. doi: 10.1111/1365-2435.12345
Lai H., Bi S., Yi H., Guo D., Li H., Wang G., et al. (2022). Seasonal variation in the functional structure of demersal fish communities and response to the environmental changes in the Pearl River Estuary, China. Ecol. Indic. 144, 109525. doi: 10.1016/j.ecolind.2022.109525
Lefort S., Aumont O., Bopp L., Arsouze T., Gehlen M., Maury O. (2015). Spatial and body-size dependent response of marine pelagic communities to projected global climate change. Global Change Biol. 21, 154–164. doi: 10.1111/gcb.12679
Li Y., Tao J., Chu L., Yan Y. (2018). Effects of anthropogenic disturbances on α and β diversity of fish assemblages and their longitudinal patterns in subtropical streams, China. Ecol. Freshw. Fish 27, 433–441. doi: 10.1111/eff.12358
Li Y., Wang J., Ju P., Zhang C., Liu B., Wang Y. (2023). Different responses of taxonomic and functional diversity to environmental changes: case study of fish communities in the Zhoushan fishing ground, China. Aquat. Sci. 85, 117. doi: 10.1007/s00027-023-01012-3
Liu O. R., Ward E. J., Anderson S. C., Andrews K. S., Barnett L. A., Brodie S., et al. (2023). Species redistribution creates unequal outcomes for multispecies fisheries under projected climate change. Sci. Adv. 9, eadg5468. doi: 10.1126/sciadv.adg5468
Loher T., Seitz A. (2006). Seasonal migration and environmental conditions of Pacific halibut Hippoglossus stenolepis, elucidated from pop-up archival transmitting (PAT) tags. Mar. Ecol. Prog. Ser. 317, 259–271. doi: 10.3354/meps317259
Lowerre-Barbieri S. K., Ganias K., Saborido-Rey F., Murua H., Hunter J. R. (2011). Reproductive timing in marine fishes: Variability, temporal scales, and methods. Mar. Coast. Fish. 3, 71–91. doi: 10.1080/19425120.2011.556932
Luo Y., Yao Z., Hong L., Wang Z., Xie S., Song Y. (2024). Seasonal dynamics and habitat preferences of juvenile fish assemblages in the Wanquan Estuary, South China: Implications for conservation and management. Reg. Stud. Mar. Sci. 77, 103624. doi: 10.1016/j.rsma.2024.103624
Maire E., Grenouillet G., Brosse S., Villéger S. (2015). How many dimensions are needed to accurately assess functional diversity? A pragmatic approach for assessing the quality of functional spaces. Global Ecol. Biogeogr. 24, 728–740. doi: 10.1111/geb.12299
Mammola S., Carmona C. P., Guillerma T., Cardoso P. (2020). Concepts and applications in functional diversity. Funct. Ecol. 35, 1869–1885. doi: 10.1111/1365-2435.13882
Miller M. G. R., Reimer J. D., Sommer B., Cook K. M., Pandolfi J. M., Obuchi M., et al. (2023). Temperate functional niche availability not resident-invader competition shapes tropicalisation in reef fishes. Nat. Commun. 14, 2181. doi: 10.1038/s41467-023-37550-5
Nay T. J., Johansen J. L., Habary A., Steffensen J. F., Rummer J. (2015). Behavioural thermoregulation in a temperature-sensitive coral reef fish, the five-lined cardinalfish (Cheilodipterus quinquelineatus). Coral Reefs 34, 1261–1265. doi: 10.1007/s00338-015-1353-4
Ni H., Lu J. (2003). Present utilization situation of main fishery resources in Zhoushan Fishing Ground. Chine. J. Appl. Ecol. 14, 569–572. doi: 10.13287/j.1001-9332.2003.0145
Novillo A., Ojeda R. (2020). Turnover, richness difference and nestedness of rodent assemblages along the southern Andes elevation gradient. Austral Ecol. 46, 239–248. doi: 10.1111/aec.12974
Pasquaud S., Pillrt M., David V., Sautour B., Elie P. (2010). Determination of fish trophic levels in an estuarine system. Estuar. Coast. Shelf S. 86, 237–246. doi: 10.1016/j.ecss.2009.11.019
Petrik C. M. (2019). “Chapter 16 – Life history of marine fishes and their implications for the future oceans,” in Predicting Future Oceans, 165–172. doi: 10.1016/B978-0-12-817945-1.00016-2
Rull V. (2012). Community ecology: diversity and dynamics over time. Community Ecol. 13, 102–116. doi: 10.1556/comec.13.2012.1.13
Sarremejane R., Cid N., Stubington R., Datry T., Alp M., Cañedo-Argüelles M., et al. (2020). Disperse, a trait database to assess the dispersal potential of European aquatic macroinvertebrates. Nature 7, 386. doi: 10.1038/s41597-020-00732-7
Stark J. W. (2012). Contrasting maturation and growth of northern rock sole in the eastern Bering Sea and gulf of Alaska for the purpose of stock management. N. Am. J. Fish. Manage. 32, 93–99. doi: 10.1080/02755947.2012.655845
Tran-Duy A., Dam A. A. V., Schrama J. W. (2011). Feed intake, growth and metabolism of Nile tilapia (Oreochromis niloticus) in relation to dissolved oxygen concentration. Aquac. Res. 43, 730–744. doi: 10.1111/J.1365-2109.2011.02882.X
Villéger S., Grenouillet G., Brosse S. (2013). Decomposing functional β-diversity reveals that low functional β-diversity is driven by low functional turnover in European fish assemblages. Global Ecol. Biogeogr. 22, 671–681. doi: 10.1111/geb.12021
Villéger S., Mason N. W. H., Mouillot D. (2008). New multidimensional functional diversity indices for a multifaceted framework in functional ecology. Ecology 89, 2290–2301. doi: 10.1890/07-1206.1
Violle C., Castro H., Richarte J., Navas M. L. (2009). Intraspecific seed trait variations and competition: passive or adaptive response? Funct. Ecol. 23, 612–620. doi: 10.1111/j.1365-2435.2009.01539.x
Wang J., Zheng Y., Li Y., Wang Y. (2023). Potential risks, source apportionment, and health risk assessment of dissolved heavy metals in Zhoushan fishing ground, China. Mar. pollut. Bull. 189, 114751. doi: 10.1016/j.marpolbul.2023.114751
White H. J., Montgomery W. I., Storchová L., Hořák J., Lennon J. J. (2018). Does functional homogenization accompany taxonomic homogenization of British birds and how do biotic factors and climate affect these processes? Ecol. Evol. 8, 7365–7377. doi: 10.1002/ece3.4267
Wiff R., Barrientos M. A., Segura A. M., Milessi A. C. (2017). The invariance of production per unit of food consumed in fish populations. Theor. Biosci. 136, 179–185. doi: 10.1007/s12064-017-0241-6
Xing L., Chen Y., Zhang C., Li B., Shin Y., Ren Y. (2020). Evaluating impacts of pulse fishing on the effectiveness of seasonal closure. Acta Oceanol. Sin. 39, 89–99. doi: 10.1007/s13131-020-1536-x
Yuan S., Sun X., Tu Z., Sun J., Wang X., Zhang P. (2024). Assessment of the characteristics of demersal fish communities using species-and trait-based approaches in the coastal habitats along Rongcheng Bay, Shandong Peninsula, China. J. Ocean U. China 23, 786–796. doi: 10.1007/s11802-024-5763-1
Zeng Z., Cheung W. W. L., Lai H., Yi H., Bi S., Li H., et al. (2022). Species and functional dynamics of demersal fish community and responses to disturbances in the Pearl River Estuary. Front. Mar. Sci. 9. doi: 10.3389/fmars.2022.921595
Keywords: marine fish, functional traits, beta diversity, trait-based approach, RLQ and fourth-corner methods
Citation: Li Y, Yan C, Ju P, Liu B, Wang J, Zhang C, Zhang W and Wang Y (2025) How the fish community structure change with seasons in Zhoushan Fishing Ground, China? Front. Mar. Sci. 11:1511121. doi: 10.3389/fmars.2024.1511121
Received: 14 October 2024; Accepted: 23 December 2024;
Published: 13 January 2025.
Edited by:
Çetin Keskin, Istanbul University, TürkiyeCopyright © 2025 Li, Yan, Ju, Liu, Wang, Zhang, Zhang and Wang. This is an open-access article distributed under the terms of the Creative Commons Attribution License (CC BY). The use, distribution or reproduction in other forums is permitted, provided the original author(s) and the copyright owner(s) are credited and that the original publication in this journal is cited, in accordance with accepted academic practice. No use, distribution or reproduction is permitted which does not comply with these terms.
*Correspondence: Yingbin Wang, eWluZ2JpbndhbmdAMTI2LmNvbQ==
Disclaimer: All claims expressed in this article are solely those of the authors and do not necessarily represent those of their affiliated organizations, or those of the publisher, the editors and the reviewers. Any product that may be evaluated in this article or claim that may be made by its manufacturer is not guaranteed or endorsed by the publisher.
Research integrity at Frontiers
Learn more about the work of our research integrity team to safeguard the quality of each article we publish.