- 1School of Life Science and Food Engineering, Faculty of Agriculture, Forestry and Food Engineering, Yibin University, Yibin, China
- 2Solid-State Fermentation Resource Utilization Key Laboratory of Sichuan Province, Faculty of Agriculture, Forestry and Food Engineering, Yibin University, Yibin, China
- 3Department of Agricultural Engineering, Faculty of Agriculture, Foods and Environment, Sana’a University, Sana’a, Yemen
- 4Program of Natural Product Medicinal Chemistry, Key Laboratory of Plant Resources Conservation and Sustainable Utilization, Plant Research Center, South China Botanical Garden, Chinese Academy of Sciences, Guangzhou, China
- 5South China National Botanical Garden, Guangzhou, China
- 6Food and Dairy Science and Technology Department, Faculty of Environmental Agricultural Science, Arish University, North Sinai, Egypt
Heavy metals (HMs) contamination significantly threatens plants, animals, aquatic ecosystems, and public health. HMs’ accumulation in aquatic environments has become a serious environmental issue due to their high stability, bioaccumulation, and biomagnification properties. These metals enter ecosystems through both natural sources and/or human activities. The toxicity of HMs poses a threat to the aquaculture sector’s sustainability by negatively affecting fish growth, reproduction, and overall physiology. Exposure to HMs in various forms can cause environmental damage, which may affect human health through direct poisoning symptoms or other adverse effects. One such effect is the increased production of reactive oxygen species (ROS), crucial metabolites that modulate the functions of pathogenic organisms and lead to oxidative stress. This, in turn, can contribute to the onset and exacerbation of numerous diseases that threaten human health. This review article examines the potential sources of HMs contamination in aquatic organisms, the impact of this contamination on the generation of ROS, and the implications for human health resulting from the consumption of contaminated aquatic products.
1 Introduction
Heavy metals (HMs) are a group of elements characterized by their high density, generally exceeding 5,000 kg/m3 (El-Sappah et al., 2024), which is at least five times greater than the density of water (Bharti and Sharma, 2022). This group primarily consists of transition metals, along with some metalloids, actinides, and lanthanides (Shahid et al., 2014). HMs are classified as trace elements due to their presence in trace concentrations (< 10 ppm) in various environmental matrices (Tchounwou et al., 2012). Some HMs, such as copper (Cu), iron (Fe), cobalt (Co), zinc (Zn), molybdenum (Mo), nickel (Ni), manganese (Mn), vanadium (V) and selenium (Se), play crucial roles in biological functions. However, elevated concentrations of these metals can become toxic, causing damage to living organisms’ cells (Munir et al., 2021; Ahmed et al., 2022). Conversely, several other HMs, including cadmium (Cd), arsenic (As), lead (Pb), mercury (Hg), and chromium (Cr), are not essential for biological systems and exhibit toxicity even at low concentrations, primarily due to their non-biodegradable nature (Mitra et al., 2022). The degree of HMs toxicity generally varies depending on the metal concentration, as shown in Table 1. Additionally, Co metal is a component of vitamin B12, and increasing its concentrations to >700 µg/L can cause toxic health effects (Leyssens et al., 2017). On the other hand, the toxicity of Se varies depending on its chemical form, with inorganic forms being more poisonous than organic ones (Genchi et al., 2023). However, increasing the daily dose of Se beyond 400 µg can lead to toxic effects (Misra et al., 2015). Although Mo toxicity is relatively low in humans, prolonged exposure to daily doses over 2 mg can lead to serious health consequences (Novotny and Peterson, 2018).
Although HMs are naturally present in the environment, their overuse in many industries for various purposes has drastically changed the ecological system by releasing excessive HMs into the soil and aquatic systems (Jamil Emon et al., 2023). In aquatic ecosystems, both water and sediments can contain HMs that can contaminate aquatic organisms. Therefore, this contamination poses a serious threat to associated aquatic fauna, particularly fish (Taslima et al., 2022). After entering aquatic systems, HMs dissolve in the water and readily accumulate in the various organs of organisms before moving to the bodies of human consumers (Authman et al., 2015). HMs that are bioaccumulative, non-biodegradable, and have a lengthy persistence period can concentrate within the food chain and have toxic effects at locations distant from the original source of pollution (Wahiduzzaman et al., 2022; Wang et al., 2022). Toxicity triggered by HMs in fish involves several aspects; however, each metal has different effects, leading to distinct toxicological symptoms. Toxic HMs in dissolved form can accumulate through circulation in aquatic organisms (Tchounwou et al., 2012), causing several complications to their health and physiological function. As a result, toxic HMs are transmitted into the food chain and eventually reach high concentrations in the human body, posing a serious threat to public health (Jamil Emon et al., 2023). These metals cause the oxidative degradation of biomolecules (DNA, proteins, and lipids) by generating free radicals (Abbas et al., 2022; Kumar et al., 2023).
One of the mechanisms by which HMs damage tissues is through the generation of reactive oxygen species (ROS) and the induction of oxidative stress in organisms exposed to this type of pollution (Kim et al., 2015; Rady et al., 2023). Furthermore, ROS are important mediators of metal-induced cellular responses and cancer development (Wang and Shi, 2001; Wang et al., 2021c). Disruptions in ROS equilibrium have also been observed in various diseases, and recent studies have suggested a dual role for ROS in carcinogenesis and the immune response in tumors (Yu et al., 2024). Considering this, there is a growing interest in investigating the effects of HMs in aquatic ecosystems since small quantities can be toxic to both aquatic organisms and humans (Kumar et al., 2023). Nevertheless, there are insufficient studies that demonstrate how consuming fish contaminated with HMs directly affects human organs. Specifically, more research is needed to accurately track the types and concentrations of HMs in fish, as well as their absorption rates in the human body, to better understand the implications of this pollution on public health. This review article examines the contamination of fish with HMs and the effect of this contamination on the generation of ROS in aquatic organisms, as well as the repercussions on human health.
2 HMs pollution sources
HMs are naturally occurring elements that can be transported through both natural phenomena and/or human activities, causing pollution in various habitats (Kumar et al., 2023). The Earth’s crust is the primary source of HMs in the environment (Haidar et al., 2023). Subsequently, natural phenomena and conditions such as weathering, rocks erosion, ore deposits (Muhammad et al., 2011), dust storms (Soleimani-Sardo et al., 2023), volcanic activities, sediment resuspension, spring waters (Di Paola et al., 2021; Haidar et al., 2023), forest fires, and biogenic processes (Zaynab et al., 2022) play a crucial role in extracting and transporting HMs to different ecosystems (Islam et al., 2015; Hossain et al., 2021). Human interventions are the primary cause of HMs contamination and its transmission (Kumar et al., 2023). Key sources include industrial activities such as battery production, mining, pigment manufacturing (yellow Cd), and plastic production (Muhammad et al., 2011; Alghasham et al., 2013). Agricultural practices, including the use of sewage water for irrigation, pesticides, and fertilizers, also contribute significantly to this issue (Srivastava et al., 2018; Kumar et al., 2023). Other sources include fuel combustion (e.g., tetraethyl lead), automobile exhaust, tanneries, electroplating, uranium mining, petrochemical spills, and sewage sludge (Al Mazed et al., 2022).
HMs enter aquatic environments through various pathways, including flooding, erosion, leaching, and direct human activities such as industrial effluents and municipal waste disposal. Pollution from crude oil exploration also leads to increased HM levels in water bodies (Figure 1). A substantial amount of industrial activity occurs in coastal areas, leading to the release of various chemical effluents into these environments (Dhaneesh et al., 2012). Furthermore, metals released from industrial facilities can be transported over long distances via wet or dry deposition (Shahid et al., 2013).
Once in the environment, HMs are transported to natural streams and rivers via household, industrial, or agricultural waste, making aquatic ecosystems the final destination for HMs pollution (Kumar et al., 2023). Intentional discharges of industrial waste and other pollutants into rivers, seas, and oceans further exacerbate the contamination of aquatic habitats, leading to ecological degradation and endangering aquatic life (El-Sappah et al., 2022; Shahjahan et al., 2022). Thus, one of the most serious environmental concerns affecting humans, animals, plants, and ecosystem safety is HMs contamination of water (Wang et al., 2021a; Zaynab et al., 2022). The indiscriminate release of these pollutants into the environment without appropriate treatment poses a severe threat to the existence of living organisms. Figure 1 depicts the main sources of HMs pollution originating from natural phenomena and human activities. Anthropogenic, such as those mentioned previously, can redistribute HMs pollutants across different environmental matrices including the aquatic environment. For example, crude oil exploration can be a potential source of HMs contamination in some aquatic environments, as HMs are components of crude oil, excavated earth layers, and the drilling fluids used (Antia et al., 2022; Umeoguaju et al., 2023). Some studies have indicated that the areas surrounding crude oil exploration locations are polluted with high levels of HMs (Johnston et al., 2019). Another major source of environmental HM contamination is waste containing HMs, such as electronic waste. Natural conditions, like erosion, can transport this waste into the soil and then into rivers and seas due to rain and the flow of groundwater (Kim et al., 2015).
3 Fish contamination with HMs
Fish are among the most extensively spread species in aquatic environments, making them more susceptible to HMs. HMs contamination of the aquatic ecosystem is one of the greatest environmental concerns due to its negative impact on both ecosystems and human health (Ali et al., 2020; Wahiduzzaman et al., 2022). Therefore, this type of pollution is one of the most prominent problems threatening water quality worldwide. The extent of HM’s toxicity to aquatic organisms varies according to their properties and the characteristics of the water source in which they live (Ahmed et al., 2022). Since HMs are non-biodegradable, they can cause toxicity in fish and other aquatic faunal communities, which can continue to be harmful indefinitely (Taslima et al., 2022). Due to these properties, HMs are deposited, assimilated, or incorporated into water and sediments of the aquatic ecosystems (Abdel-Baki et al., 2011).
In the aquatic ecosystem, fish are hypersensitive to environmental conditions, which make them useful bioindicators for environmental monitoring, as they can easily metabolize, detoxify, and accumulate HMs in their bodies (Shahjahan et al., 2022). Aquatic organisms are adversely affected by heavy metal-mediated toxicity, which negatively impacts their interaction with their surroundings (Jamil Emon et al., 2023). Aquatic organisms can acquire metals by ingesting particulate matter suspended in water, ingesting contaminated food, exchanging ions across lipophilic membranes such as gills, and adsorbing them on tissues and membrane surfaces (Kawser Ahmed et al., 2016). The aquatic environment’s acidity may also facilitate the absorption of free divalent ions of different HMs through fish gills (Naz et al., 2023). In some fish species, toxic chemicals are rapidly absorbed through the gills, where HMs can accumulate in the gills due to their large surface area and less effective detoxification system compared to the liver (Dhaneesh et al., 2012). Aquatic organisms can ingest HMs at low concentrations through water uptake, and these concentrations can increase during biomagnification process (Abbas, 2024). Both bioaccumulation (the accumulation of pollutants in an organism’s tissues over its lifetime) and biomagnification (the increasing concentration of pollutants at higher trophic levels) of HMs can have severe biological effects. Consequently, HMs concentrations in large aquatic organisms usually increase with the organism’s size/age (i.e. bioaccumulation) and in higher trophic levels (i.e. biomagnification), meaning that top predators, large and long-living organisms are most affected by HMs (Danovaro et al., 2023).
Aquatic organisms can absorb these metals through the skin, gills, or the digestive system (contaminated food), allowing HMs to move through the bloodstream to the various organs (Ahmed et al., 2022), such as the liver, where biotransformation occurs (Hermenean et al., 2015). HMs can also be absorbed through the osmoregulation process, which is an essential physiological function, leading to similar toxic effects (Bera et al., 2022). Large amounts of HMs may accumulate in fish tissues such as muscles, gills, intestines, liver, and kidneys depending on metal concentrations, exposure duration, and other environmental factors like temperature, salinity, pH, and metal hardness (Dhaneesh et al., 2012). The uptake of HMs in fish gills indicates their presence in aquatic systems, while accumulation in the liver reflects the deposition of these metals, which is influenced by the fish’s size, sex, age, feeding behaviors, and living environment (Kumar et al., 2023). Additionally, the uptake process of HMs in the liver is associated with its metabolic functions (Zhao et al., 2012).
The presence of HMs in aquatic environments exposes fish and other organisms to oxidative stress due to their ability to undergo redox cycles. These HMs can interact with biological particles that contain sulfur, nitrogen, oxygen, and other elements, which affect or alter the structures and functions of enzymes, proteins, hormones, and other substances, thus ultimately leading to damage in fish tissues and organs (Shahjahan et al., 2022).
HMs toxicity in fish can cause various physiological and biochemical changes, including reduced growth and reproductive activity, as indicated by lower gonad somatic index (GSI), fecundity, fertilization, and hatching rates (Jamil Emon et al., 2023). Some HMs can also exhibit carcinogenic (Kortei et al., 2020) and mutagenic (Jamil Emon et al., 2023) properties in aquatic organisms, including fish. They can cause structural damage, functional and biochemical disorders, oxidative stress, genotoxicity, as well as fatal pathology in the liver, kidneys, and reproductive, respiratory, and nervous systems (Haseeb et al., 2022). HMs can also affect the blood’s ability to carry oxygen by altering the hemoglobin (Hb), hematocrit (Hct), and red blood cells (RBCs) values in various ways, depending on the fish species, metal type, and exposure duration (Fazio, 2019). Numerous studies have assessed and analyzed the risk of HMs contamination in various rivers, seas, and lakes, with high concentrations reported in the Gulf of Guinea (Nyarko et al., 2023), Buriganga river (Kawser Ahmed et al., 2016), Buriganga and Turag rivers (Baki et al., 2020), Thamirabarani river (Arisekar et al., 2020), Dhaleswari river (Wahiduzzaman et al., 2022), Ganga river (Siddiqui et al., 2019), Turag-Tongi-Balu river channel (Hossain et al., 2024), Amazon river estuary (Viana et al., 2023), Brazilian amazon (Basta et al., 2023), the Niger Delta region of Nigeria (Umeoguaju et al., 2022), Bardawil lake (Abbas, 2024), and Caspian sea (Bakhshalizadeh et al., 2022). Additionally, the concentrations of many HMs were found to be significantly higher in polluted sites in the Red Sea compared to unpolluted reference sites (Al-Hasawi and Hassanine, 2022).
4 Reactive oxygen species
Molecular oxygen has two unpaired electrons with parallel spins in its outer valence shell, which makes it unable to react with most organic molecules directly. Instead, the most prevalent mechanism of oxygen reduction involves stepwise single-electron transfers that produce free radicals (Magnani and Mattevi, 2019). These free radicals are unstable molecules resulting from oxidative stress (Kumar et al., 2023). ROS are bioactive molecules that are natural byproducts of a series of reactions originating from the metabolism of cellular oxygen (Corcoran and Cotter, 2013; Nakamura and Takada, 2021). These ROS are naturally produced due to the transfer of electrons to oxygen on the inner mitochondrial membrane (Parvez et al., 2018; Rauf et al., 2024). This term refers to all highly reactive oxygen derivatives (O2) that participate in cellular electrons (Corcoran and Cotter, 2013; Bekhet and Eid, 2021).
Aerobic organisms continuously generate ROS, such as superoxide radicals (O2•−), hydroxyl radical (•OH), and hydrogen peroxide (H2O2) (Klaunig and Wang, 2018). ROS are produced during oxidative metabolism and function as cell signaling molecules (Wang et al., 2021c). The generation of ROS, which is essential for many signaling pathways, is mediated by mitochondrial respiration (Juan et al., 2021), and numerous physiological and biochemical activities in cells (Mansoor et al., 2023). Therefore, mitochondria are regarded as the most significant cellular ROS generators due to their continuous activity throughout metabolism (Castro et al., 2016). The adenosine triphosphate (ATP) generation from oxygen and simple carbohydrates is facilitated by this process, which is integral to oxidative phosphorylation (Li et al., 2013). Five pivotal protein complexes are involved in this complex process: complex I is nicotinamide adenine dinucleotide (NAD) + hydrogen (H) (NADH); complex II is succinate; complex III is ubiquinol; complex IV is cytochrome c oxidase; and complex V is F1F0-ATP synthase (Yu et al., 2024). These complexes produce superoxide (O2•–) due to electron leakage. Afterward, superoxide is converted into H2O2 in intermembrane space by the enzyme superoxide dismutase 1 (SOD1) (Li et al., 2013). Additionally, a subgroup of ROS is generated by nicotinamide adenine dinucleotide phosphate (NADPH) oxidases (NOX) as a transmembrane protein family. These enzymes transport electrons across biological membranes and catalyze the conversion of oxygen into superoxide. The catalytic subunit NOX2 plays a significant role in this process (Cipriano et al., 2023). During the metabolic processes, the peroxisome catabolizes biomolecules using some enzymes to remove hydrogen in an oxidative reaction, creating ROS such as hydrogen peroxide as a metabolic byproduct (Brieger et al., 2012).
Low to medium levels of these species are essential for maintaining the oxidation-reduction equilibrium and numerous necessary physiological functions, including regulating blood pressure and cognitive and immune functions (Bardaweel et al., 2018). However, the overproduction of ROS can disrupt oxidation-reduction equilibrium, leading to oxidative stress. This damage can irreparably harm cell structures, resulting in apoptosis and necrosis due to the oxidation of proteins, lipids, carbohydrates, DNA, and RNA (Checa and Aran, 2020; Juan et al., 2021; Guo et al., 2023). The influence of ROS at a cellular level depends on the species, concentration, location, and duration of exposure (Castro et al., 2016). Consequently, the overproduction of ROS can aggravate the development of inflammatory symptoms and contribute to the onset of many associated diseases (Liu et al., 2023).
ROS includes free radical such as O2•–, •OH, peroxyl (RO2•–), alkoxyl radicals (RO•) and hydroperoxyl (HO•2); and non-free radical oxygen intermediates (i.e. peroxides), which contain unpaired valence shell electrons (Corcoran and Cotter, 2013; Shields et al., 2021). Non-radical ROS include H2O2, singlet oxygen (1O2), hypochlorous acid (HOCl) (Pizzino et al., 2017), the hydroxide ion (OH–) and organic peroxides (ROOH) (Shields et al., 2021). These non-radical species have volatile O-O bonds (Corcoran and Cotter, 2013). The non-free radical ROS can undergo redox reactions to produce free radicals as shown in chemical Equations 1-5 (Shields et al., 2021).
Other common ROS include nitrogen dioxide radical (NO2•), peroxynitrite (the physiological combination of peroxynitrite, ONOO− and its more reactive protonated version, peroxynitrous acid, ONOOH), carbonate radical anion (CO3•−), hypohalous acids (HOCl), and hypobromous acids (HOBr) (Murphy et al., 2022). Furthermore, nitric oxide radical (NO•), which has important physiological functions, is generated during the oxidation of L-arginine by nitric oxide synthase (NOS) (Kumar and Pandey, 2015).
The O2•– and H2O2 are the main agents in ROS signaling generated by the mitochondrial electron transport chain (Sinenko et al., 2021). As well as ROS compounds are generated by lipid metabolism in peroxisomes (Checa and Aran, 2020), the activity of different cytosolic enzymes including cyclooxygenases (Martínez-Revelles et al., 2013), or proteins in plasma membranes that use NADPH as an electron donor (García et al., 2023). Furthermore, ROS are generated by many enzymes including NADPH oxidases (NOXs), nitric oxide synthases, xanthine oxidases, peroxisomal components (Magnani and Mattevi, 2019; Juan et al., 2021), thymidine phosphorylase, lipoxygenases, and cyclooxygenase (Yu et al., 2024). Moreover, they are generated by ionizing, ultraviolet (UV) radiation, and the metabolism of many drugs and xenobiotics (Juan et al., 2021), with cytochromes P450 enzymes playing a crucial role in reducing molecular oxygen to generate O2•– and H2O2 (Collin, 2019). Additionally, oxidants are released during protein folding and disulfide bond formation in the endoplasmic reticulum, leading to the production of highly reactive species, including O2•–, H2O2, •OH, and 1O2 (Juan et al., 2021). Both enzymatic and non-enzymatic processes result in the constant production of ROS (Rauf et al., 2024). ROS can be produced constitutively by cells and serve as intracellular signaling molecules, activating redox-sensitive signaling pathways that alter the levels of cytoprotective regulators (Zhang et al., 2016; Checa and Aran, 2020).
5 Effect of HMs on ROS generation
In biological systems, ROS are generated as a natural byproduct of cellular aerobic metabolism (Juan et al., 2021). The vast majority of oxygen (> 90%) consumed by eukaryote organisms is converted to energy in the form of ATP using four-electron mechanisms by cytochrome oxidase in the electron transport chain (ETC) without releasing ROS. In contrast, the remaining oxygen is reduced through one-electron successive pathways that begin with converting molecular oxygen to the O2•–, which can then be reduced by a one-electron mechanism to generate H2O2, •OH, and water (Lushchak, 2016). Molecular oxygen is necessary for all aerobic organisms and plays a crucial role in biological processes. Despite its beneficial function, oxygen is a potent oxidant that causes oxidative stress when exposed to environmental stressors such as HMs (Kumar et al., 2023). Intracellular ROS levels rise in response to various stimuli, causing the oxidation of cysteine residues in cytoplasmic proteins, including phosphatases and kinases, affecting signal transduction pathways (Corcoran and Cotter, 2013).
The primary endogenous sources of ROS generation typically include oxidative phosphorylation, P450 cytochrome, peroxisomes, and the activation of inflammatory cells (Klaunig and Wang, 2018). Moreover, there are supporting factors such as immune cell activation, ischemia, pathogens, cancer, strenuous exercise, psychological stress, and aging (Pizzino et al., 2017). On the other hand, ROS can be generated by exogenous causes such as exposure to air pollutants, tobacco, asbestos, toxins, and radiation (Gharagozloo and Aitken, 2011; Wang et al., 2021c). Exogenous sources of ROS generation also include exposure to HMs, such as Hg, As, Fe, Pb, and Cd. Once these substances enter the body, they undergo degradation or metabolism, and free radicals are produced as byproducts (Pizzino et al., 2017). Long-term exposure to HMs induces fish to produce large amounts of ROS and accumulate them, which in turn leads to the oxidation of major biomolecules in fish tissues (Fatima et al., 2015). Furthermore, toxic substances such as HMs can increase ROS production due to their ability to alter mitochondrial membrane permeability and inhibit ROS clearance enzymes in the cellular antioxidant tract (Sun et al., 2022; Mansoor et al., 2023). HMs contamination enhances the generation of ROS through various mechanisms. It has been established that exposure to HMs can disrupt the cellular redox state and mitochondrial electron transport, causing increased production of ROS (Eskander and Saleh, 2020). In addition, HMs such as Cd, As, Fe, Cu, Ni, Pb, Cr, and Hg can deplete enzyme activities through lipid peroxidation and react with nuclear proteins and DNA, generating reactive radicals that can damage cells (Madkour, 2020). HMs also have the ability to alter various physiological and biochemical processes, including enzymatic functions and cell respiratory rates. This is because HMs can interact with different elements of the ETC, changing their activity and mostly causing an increase in ROS production (Mansoor et al., 2023). As shown in Figure 2. some of these HMs (Cd, Pb, and Hg) can interfere with mitochondrial functions by binding to the ETC’s components in organism cells, causing electron disruption and partial reduction of oxygen, resulting in O2•− formation, which is considered the beginning of the generation of other ROS such as H2O2 and OH• (Reddam et al., 2022). In the same context, Cd prevents ATPase, glutathione peroxidase (GPx), lactate dehydrogenase (LDH), and superoxide dismutase (SOD) activities, promoting lipid peroxidation levels and ROS generation (Genchi et al., 2020). Moreover, HMs such as As, Cd, and Hg can deplete thiol groups (−OH) in glutathione, a key antioxidant that neutralizes ROS, leading to decreased availability for ROS scavenging (Lushchak, 2016).
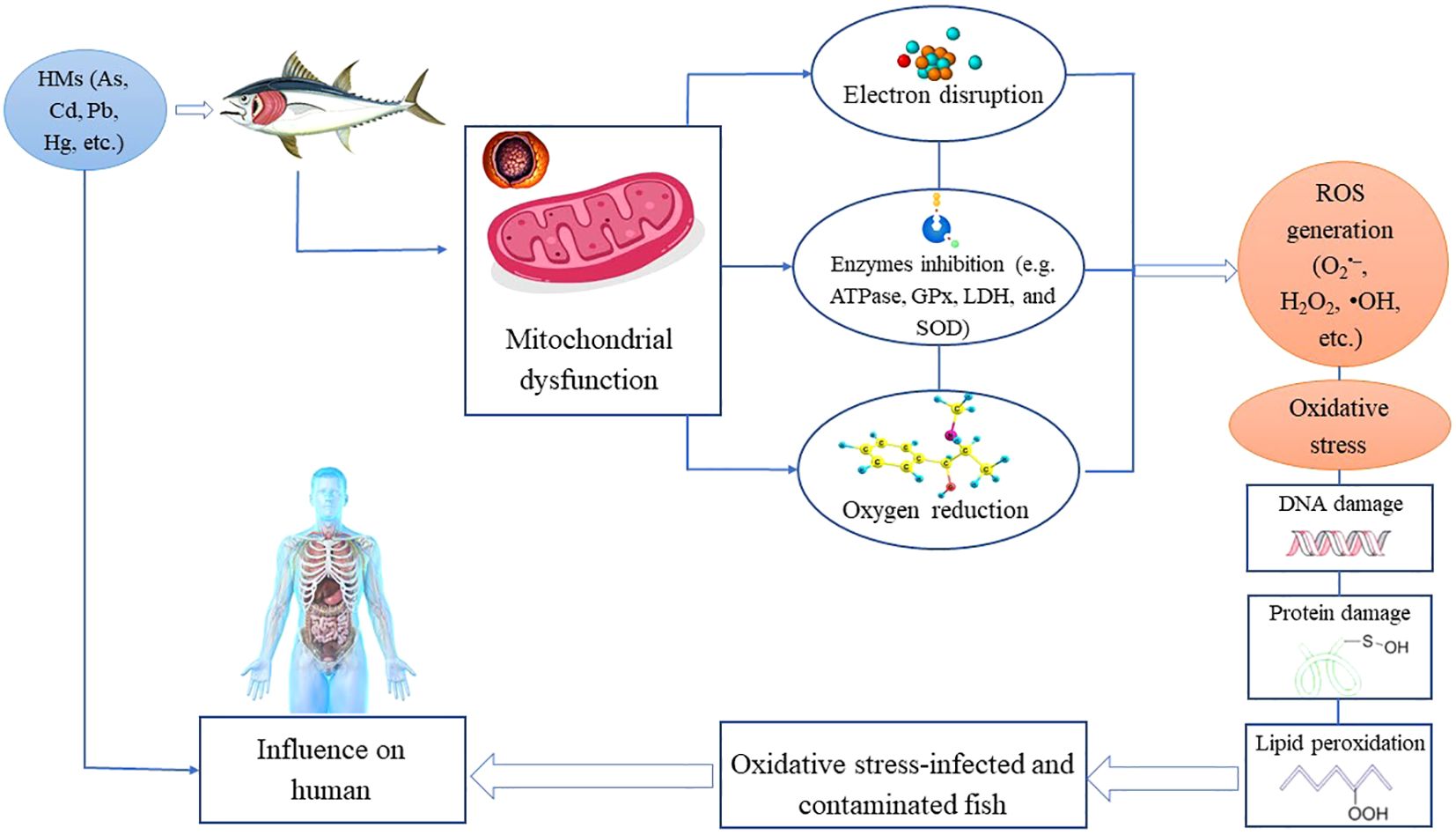
Figure 2. HMs-induced oxidative stress pathways by interfering with mitochondrial functions through binding to the ETC’s components in cells, generating ROS that cause damage to DNA, proteins, and lipids.
Other metals, particularly iron, can produce ROS through the Fenton reaction (Equation 5) without the aid of enzymes, where, Fe2+ reacts with H2O2 to produce a highly reactive hydroxy radical (•OH), resulting in damage to DNA and other related biomolecules (Bystrom et al., 2014). On the other hand, ions with variable valence are among the most common inducers of oxidative stress. Their capacity to accept/donate electrons make them susceptible to free radical processes. Among these ions, Fe, Cu, Mn, and Cr are the most commonly studied due to their important biological properties and ability to stimulate ROS generation by involvement in the Haber–Weiss reaction (Lushchak, 2016), as shown in Equation 6. Additionally, in the Haber–Weiss reaction, O2•– reacts with H2O2 (Equation 7) to generate HO• radicals as well (Collin, 2019).
Where Me is a metal ion with variable valence
The toxic effects of many HMs are caused by redox cycling, which generates ROS. Lipid peroxidation (LPO) is the most common effect of ROS and is thus commonly used as an indicator of fish health (Javed et al., 2016). Additionally, redox inactive metals such as Cd, Pb, and Zn inhibit key antioxidant constituents in cells, notably non-enzymatic and enzymatic antioxidants containing thiols (Hermenean et al., 2015). HMs activate NADPH oxidase and protein kinase C (PKC), leading to the intensified generation of intracellular ROS (Sun et al., 2022). Cells treated with Cr generate ROS by inducing NADPH oxidase (NOX) (Wang et al., 2011).
6 Implications of fish-HMs contamination on human health
Fishes are classified as one of the foods that occupy the top of the food pyramid. Therefore, they are susceptible to the biomagnification of HMs and are most likely to serve as carriers of HMs to humans (Kumar et al., 2023). The accumulation of HMs in seafood exposes human consumers to the harmful consequences of these metals (Umeoguaju et al., 2023). Because of this, fish are frequently employed as crucial biological indicators to examine metal concentrations in their habitats and to evaluate the ecological and health hazards related to the discharge of waste resulting from human activities (Naz et al., 2023). HMs can directly interact with mitochondria, altering the physiological conditions of cells such as intracellular calcium homeostasis. This affects the dynamics and function of mitochondria and cells, ultimately impacting the body’s physiological state, which can contribute to the onset and progression of certain illnesses (Sun et al., 2022). Risk assessment is a valuable tool for evaluating the potential effects of HMs pollutants, as it considers the total exposure of individuals to these pollutants in a specific location (Zaghloul et al., 2024).
Aquatic organisms, especially fish, are an essential source of polyunsaturated omega-3 fatty acids, vitamins, minerals, and high-value proteins. As HMs can accumulate in fish bodies, they can readily enter the human body mainly through the consumption of seafood products, causing acute and long-term negative health complications (Bakhshalizadeh et al., 2022). Humans can absorb HMs through the digestive system (contaminated food and water), skin, or inhalation (Witkowska et al., 2021). Thus, the absorption rate of HMs in the human body depends on the HM’s type and how it enters the body. For example, HMs accumulate in the human body when contaminated fish are consumed (Alam et al., 2023), causing severe dysfunction, malnourishment, and immune system destruction (Mehnaz et al., 2023). Furthermore, HMs affect the kidneys, brain, nerves, liver, skin, and heart, among other organs in the human body (Mitra et al., 2022). HMs interfere with the functioning of native proteins by attaching to free thiols or other functional groups, causing side chain oxidation in amino acids, obstructing protein folding, and/or displacing necessary metal ions from enzymes (Witkowska et al., 2021). Exposure to HMs, either permanently or at concentrations above safe levels, can lead to serious short-term or long-term health problems, such as disorders of the gastrointestinal, respiratory, neurological, reproductive, cardiovascular, renal, and hematopoietic systems (Garza-Lombó et al., 2018; Witkowska et al., 2021).
The peroxidative activity of some HMs generates significant levels of ROS, aiding in protumorigenic signaling, and the growth of cancerous cells (Mitra et al., 2022). The liver and kidneys have a strong propensity to accumulate HMs at higher concentrations than other tissues in the human body (Al-Hasawi and Hassanine, 2022). Increased exposure to these metals can have serious negative effects on these organs and the rest of the body (Table 2). HMs effects depend on several factors, including the dose, exposure manner, chemical species, exposed individuals’ ages, genders, genetics, and nutritional status of the exposed individuals (Tchounwou et al., 2012). Moreover, different cooking procedures can affect the HMs content in fish (Abbas, 2024).
Although the natural production of ROS by cells is regulated, an increase in ROS can be pathological and result in oxidative stress and several diseases (Juan et al., 2021). As previously explained, oxidative stress occurs when there is an imbalance between the generation of ROS and the antioxidant defense mechanisms of the body (Wang et al., 2020a; Nakamura and Takada, 2021). This disruption in the oxidation-reduction (redox) balance causes molecular damage (Checa and Aran, 2020). Consequently, oxidative stress has been implicated in the development of several chronic diseases, such as cancer, aging, diabetes, and hypertension (Pizzino et al., 2017; Sharifi-Rad et al., 2020). The free radicals and reactive molecules in biological systems, produced during electron transfer reactions by either losing or accepting an electron, are derived from ROS or reactive nitrogen species (RNS) (Jomova et al., 2023). Checa and Aran (2020) reported that alongside genetic mutations, excessive ROS leads to irreversible oxidative change in lipids, proteins (including oxidation and peroxidation), and glycans (resulting in advanced lipoxidation and glycation end products), which impair their function and can stimulate illness or cell death. The lipid peroxidation process converts unsaturated fatty acids into small hydrocarbon molecules, forming lipid-free radicals classified as ROS. These radicals are highly damaging to proteins, lipids, and carbohydrates due to oxidative damage resulting from the inhibition of antioxidant mechanisms (Haseeb et al., 2022). In addition, the release of ROS damages cellular proteins, lipids, and DNA, causing cell damage and potentially leading to carcinogenesis (Reczek and Chandel, 2017). Certain HMs impair the sensitivity of cancer to treatment and accelerate its growth through various mechanisms, such as breaching DNA structure, decreasing antioxidant protection, and inhibiting the activity of enzymes involved in repairing damage (Romaniuk et al., 2017; Pietrzak et al., 2021; Witkowska et al., 2021). HMs can also damage organelles and cell components such as membranes, mitochondria, lysosomes, nuclei, and endoplasmic reticulum (Wang and Shi, 2001). Finally, increased ROS concentrations in semen plasma can lead to male infertility (Gharagozloo and Aitken, 2011; Bardaweel et al., 2018).
7 Conclusions and recommendations
HMs contamination is a significant contemporary environmental challenge, driven by an increasing number of pollution sources and the widespread dispersion of these toxic elements. The contamination of water and soil with HMs adversely impacts human health, as well as terrestrial and aquatic ecosystems. The bioaccumulation of HMs can severely impact the physiology of aquatic organisms, hindering their growth and reproduction. Aside from their toxic effects, the presence of HMs enhances the generation of ROS in both aquatic life and humans, leading to oxidative stress. This condition is a major contributor to various health issues, including cancer, kidney damage, liver disease, and skin problems. To effectively address the issue of HMs contamination, more preventive measures must be implemented. This includes enacting stricter laws regarding the disposal of heavy metal-containing waste and strengthening environmental regulations. Furthermore, targeted remediation efforts, such as soil and water decontamination techniques, may be required in areas with high levels of HMs contamination. Collaboration among policymakers, environmental agencies, and the general public is required to develop and implement effective strategies to address this pressing environmental concern.
Author contributions
YW: Writing – original draft, Writing – review & editing. AN: Writing – original draft, Writing – review & editing. CZ: Writing – original draft, Writing – review & editing. WA: Writing – original draft, Writing – review & editing. SA: Writing – original draft, Writing – review & editing.
Funding
The author(s) declare that financial support was received for the research, authorship, and/or publication of this article. This study was supported by Demonstration course of curriculum ideology “Food Safety”, and Promotion and practice of food innovative experiment based on “two abilities and one degree”.
Conflict of interest
The authors declare that the research was conducted in the absence of any commercial or financial relationships that could be construed as a potential conflict of interest.
Publisher’s note
All claims expressed in this article are solely those of the authors and do not necessarily represent those of their affiliated organizations, or those of the publisher, the editors and the reviewers. Any product that may be evaluated in this article, or claim that may be made by its manufacturer, is not guaranteed or endorsed by the publisher.
References
Abbas M. M. M. (2024). Heavy metal levels and cancer risk assessments of the commercial Denis, Sparus aurata collected from Bardawil Lake and private fish farm waters as a cultured source, Egypt. Biol. Trace Elem. Res. 202, 2864–2877. doi: 10.1007/s12011-023-03880-0
Abbas M., Li Y., Elbaiomy R. G., Yan K., Ragauskas A. J., Yadav V., et al. (2022). Genome-wide analysis and expression profiling of SlHsp70 gene family in Solanum lycopersicum revealed higher expression of SlHsp70-11 in roots under Cd2+ stress. Front. Biosci. (Landmark Ed) 27, 186. doi: 10.31083/j.fbl2706186
Abdel-Baki A., Dkhil M., Al-Quraishy S. (2011). Bioaccumulation of some heavy metals in tilapia fish relevant to their concentration in water and sediment of Wadi Hanifah, Saudi Arabia. Afr. J. Biotechnol. 10, 2541–2547. doi: 10.5897/AJB10.1772
Ahmed I., Zakiya A., Fazio F. (2022). Effects of aquatic heavy metal intoxication on the level of hematocrit and hemoglobin in fishes: a review. Front. Environ. Sci. 10. doi: 10.3389/fenvs.2022.919204
Alam M., Rohani M. F., Hossain M. S. (2023). Heavy metals accumulation in some important fish species cultured in commercial fish farm of Natore, Bangladesh and possible health risk evaluation. Emerg. Contam. 9, 100254. doi: 10.1016/j.emcon.2023.100254
Alghasham A., Salem T. A., Meki A.-R. M. (2013). Effect of cadmium-polluted water on plasma levels of tumor necrosis factor-α, interleukin-6 and oxidative status biomarkers in rats: Protective effect of curcumin. Food Chem. Toxicol. 1, 160–164. doi: 10.1016/j.fct.2013.05.059
Al-Hasawi Z., Hassanine R. (2022). Effect of heavy metal pollution on the blood biochemical parameters and liver histology of the Lethrinid fish, Lethrinus harak from the Red Sea. Pak. J. Zool. 55, 1771. doi: 10.17582/journal.pjz/20220223170218
Al Hossain M. A., Yajima I., Tazaki A., Xu H., Saheduzzaman M., Ohgami N., et al. (2019). Chromium-mediated hyperpigmentation of skin in male tannery workers in Bangladesh. Chemosphere 1, 611–617. doi: 10.1016/j.chemosphere.2019.04.112
Ali M. M., Ali M. L., Proshad R., Islam S., Rahman Z., Kormoker T. (2020). Assessment of trace elements in the demersal fishes of a coastal river in Bangladesh: a public health concern. Thalassas: Int. J. Mar. Sci. 36, 641–655. doi: 10.1007/s41208-020-00227-7
Al Mazed M., Rahman M., Ahmed S. (2022). A review on effects of heavy metals on aquatic animals and public health significance. Vet. Sci. Res. Rev. 8, 96–104. doi: 10.17582/journal.vsrr/2022/8.2.96.104
Amadou A., Praud D., Coudon T., Danjou A. M., Faure E., Deygas F., et al. (2021). Exposure to airborne cadmium and breast cancer stage, grade and histology at diagnosis: findings from the E3N cohort study. Sci. Rep. 11, 23088. doi: 10.1038/s41598-021-01243-0
Antia M., Ezejiofor A. N., Obasi C. N., Orisakwe O. E. (2022). Environmental and public health effects of spent drilling fluid: An updated systematic review. J. Hazard. Mater. Adv. 7, 100120. doi: 10.1016/j.hazadv.2022.100120
Arisekar U., Shakila R. J., Shalini R., Jeyasekaran G. (2020). Human health risk assessment of heavy metals in aquatic sediments and freshwater fish caught from Thamirabarani River, the Western Ghats of South Tamil Nadu. Mar. pollut. Bull. 159, 111496. doi: 10.1016/j.marpolbul.2020.111496
Authman M. M., Zaki M. S., Khallaf E. A., Abbas H. H. (2015). Use of fish as bio-indicator of the effects of heavy metals pollution. J. Aquac. Res. Dev. 6, 328. doi: 10.4172/2155-9546.1000328
Bakhshalizadeh S., Mora-Medina R., Fazio F., Parrino V., Ayala-Soldado N. (2022). Determination of the heavy metal bioaccumulation patterns in muscles of two species of mullets from the Southern Caspian Sea. Animals 12, 2819. doi: 10.3390/ani12202819
Baki M. A., Shojib M. F. H., Sehrin S., Chakraborty S., Choudhury T. R., Bristy M. S., et al. (2020). Health risk assessment of heavy metal accumulation in the Buriganga and Turag River systems for Puntius ticto, Heteropneustes fossilis, and Channa punctatus. Environ. Geochem. Health 42, 531–543. doi: 10.1007/s10653-019-00386-4
Bardaweel S. K., Gul M., Alzweiri M., Ishaqat A., Alsalamat H. A., Bashatwah R. M. (2018). Reactive oxygen species: The dual role in physiological and pathological conditions of the human body. Eurasian J. Med. 50, 193–201. doi: 10.5152/eurasianjmed.2018.17397
Basta P. C., De Vasconcellos A. C. S., Hallwass G., Yokota D., Pinto D.D.O.D.E.R., De Aguiar D. S., et al. (2023). Risk assessment of mercury-contaminated fish consumption in the Brazilian Amazon: an ecological study. Toxics 11, 800. doi: 10.3390/toxics11090800
Baszuk P., Janasik B., Pietrzak S., Marciniak W., Reszka E., Białkowska K., et al. (2021). Lung cancer occurrence—correlation with serum chromium levels and genotypes. Biol. Trace Elem. Res. 199, 1228–1236. doi: 10.1007/s12011-020-02240-6
Bekhet O. H., Eid M. E. (2021). The interplay between reactive oxygen species and antioxidants in cancer progression and therapy: a narrative review. Transl. Cancer Res. 10, 4196. doi: 10.21037/tcr-21-629
Bera T., Kumar S., Devi M., Kumar V., Behera B., Das B. (2022). Effect of heavy metals in fish reproduction: A review. J. Environ. Biol. 43, 631–642. doi: 10.22438/jeb/43/5/MRN-4042
Bharti R., Sharma R. (2022). Effect of heavy metals: An overview. Mater. Today: Proc. 51, 880–885. doi: 10.1016/j.matpr.2021.06.278
Brieger K., Schiavone S., Miller F. J. Jr., Krause K.-H. (2012). Reactive oxygen species: from health to disease. Swiss Med. Wkly. 142, w13659. doi: 10.4414/smw.2012.13659
Büyüköztürk S., Gelincik A., Ünal D., Demirtürk M., Çelik D. D., Erden S., et al. (2015). Oral nickel exposure may induce Type I hypersensitivity reaction in nickel-sensitized subjects. Int. Immunopharmacol. 26, 92–96. doi: 10.1016/j.intimp.2015.03.012
Bystrom L. M., Guzman M. L., Rivella S. (2014). Iron and reactive oxygen species: friends or foes of cancer cells? Antioxid. Redox Sign. 20, 1917–1924. doi: 10.1089/ars.2012.5014
Castro J. P., Grune T., Speckmann B. (2016). The two faces of reactive oxygen species (ROS) in adipocyte function and dysfunction. Biol. Chem. 397, 709–724. doi: 10.1515/hsz-2015-0305
Checa J., Aran J. M. (2020). Reactive oxygen species: drivers of physiological and pathological processes. J. Inflamm. Res. 13, 1057–1073. doi: 10.2147/JIR.S275595
Chen X., Li Y., Zhang B., Zhou A., Zheng T., Huang Z., et al. (2018). Maternal exposure to nickel in relation to preterm delivery. Chemosphere 193, 1157–1163. doi: 10.1016/j.chemosphere.2017.11.121
Cipriano A., Viviano M., Feoli A., Milite C., Sarno G., Castellano S., et al. (2023). NADPH oxidases: from molecular mechanisms to current inhibitors. J. Med. Chem. 66, 11632–11655. doi: 10.1021/acs.jmedchem.3c00770
Collin F. (2019). Chemical basis of reactive oxygen species reactivity and involvement in neurodegenerative diseases. Int. J. Mol. Sci. 20, 2407. doi: 10.3390/ijms20102407
Control, C.F.D., Prevention (2019). Fourth national report on human exposure to environmental chemicals, updated tables, January 2019 Vol. 1 (Atlanta, GA: US Department of Health and Human Services, Centers for Disease Control and Prevention), 75822.
Corcoran A., Cotter T. G. (2013). Redox regulation of protein kinases. FEBS J. 280, 1944–1965. doi: 10.1111/febs.12224
Danovaro R., Cocozza Di Montanara A., Corinaldesi C., Dell’anno A., Illuminati S., Willis T. J., et al. (2023). Bioaccumulation and biomagnification of heavy metals in marine micro-predators. Commun. Biol. 6, 1206. doi: 10.1038/s42003-023-05539-x
Dehghan S. F., Mehrifar Y., Ardalan A. (2019). The relationship between exposure to lead-containing welding fumes and the levels of reproductive hormones. Ann. Glob. Health 85, 125. doi: 10.5334/aogh.2617
Dhaneesh K. V., Gopi M., Ganeshamurthy R., Kumar T. T. A., Balasubramanian T. (2012). Bio-accumulation of metals on reef associated organisms of Lakshadweep Archipelago. Food Chem. 131, 985–991. doi: 10.1016/j.foodchem.2011.09.097
Di Paola D., Capparucci F., Lanteri G., Cordaro M., Crupi R., Siracusa R., et al. (2021). Combined toxicity of xenobiotics Bisphenol A and heavy metals on zebrafish embryos (Danio rerio). Toxics 9, 344. doi: 10.3390/toxics9120344
El-Sappah A. H., Seif M. M., Abdel-Kader H. H., Soaud S. A., Abdelghaffar A. M., El-Sappah H. H., et al. (2022). Genotoxicity and trace elements contents analysis in Nile Tilapia (Oreochromis niloticus) indicated the levels of aquatic contamination at three Egyptian areas. Front. Vet. Sci. 9. doi: 10.3389/fvets.2022.818866
El-Sappah A. H., Zhu Y., Huang Q., Chen B., Soaud S. A., Abd Elhamid M. A., et al. (2024). Plants’ molecular behavior to heavy metals: from criticality to toxicity. Front. Plant Sci. 15. doi: 10.3389/fpls.2024.1423625
Eskander S. B., Saleh H. M. (2020). “Heavy metal-induced oxidative stress and related cellular process,” in Cellular and Molecular Phytotoxicity of Heavy Metals (Springer Nature, Cham), 99–123. doi: 10.1007/978-3-030-45975-8_7
Farzan S. F., Howe C. G., Chen Y., Gilbert-Diamond D., Korrick S., Jackson B. P., et al. (2021). Prenatal and postnatal mercury exposure and blood pressure in childhood. Environ. Int. 146, 106201. doi: 10.1016/j.envint.2020.106201
Fatima M., Usmani N., Firdaus F., Zafeer M. F., Ahmad S., Akhtar K., et al. (2015). In vivo induction of antioxidant response and oxidative stress associated with genotoxicity and histopathological alteration in two commercial fish species due to heavy metals exposure in northern India (Kali) river. Comp. Biochem. Physiol. Part C: Toxicol. Pharmacol. 176-177, 17–30. doi: 10.1016/j.cbpc.2015.07.004
Fazio F. (2019). Fish hematology analysis as an important tool of aquaculture: a review. Aquaculture 500, 237–242. doi: 10.1016/j.aquaculture.2018.10.030
García J. G., Ansorena E., Izal I., Zalba G., De Miguel C., Milagro F. I. (2023). Structure, regulation, and physiological functions of NADPH oxidase 5 (NOX5). J. Physiol. Biochem. 79, 383–395. doi: 10.1007/s13105-023-00955-3
Garza-Lombó C., Posadas Y., Quintanar L., Gonsebatt M. E., Franco R. (2018). Neurotoxicity linked to dysfunctional metal ion homeostasis and xenobiotic metal exposure: redox signaling and oxidative stress. Antioxid. Redox Sign. 28, 1669–1703. doi: 10.1089/ars.2017.7272
Genchi G., Lauria G., Catalano A., Sinicropi M. S., Carocci A. (2023). Biological activity of selenium and its impact on human health. Int. J. Mol. Sci. 24, 2633. doi: 10.3390/ijms24032633
Genchi G., Sinicropi M. S., Lauria G., Carocci A., Catalano A. (2020). The effects of cadmium toxicity. Int. J. Environ. Res. Public Health 17, 3782. doi: 10.3390/ijerph17113782
Gharagozloo P., Aitken R. J. (2011). The role of sperm oxidative stress in male infertility and the significance of oral antioxidant therapy. Hum. Reprod. 26, 1628–1640. doi: 10.1093/humrep/der132
Goullé J.-P., Le Roux P., Castanet M., Mahieu L., Guyet-Job S., Guerbet M. (2015). Metallic profile of whole blood and plasma in a series of 99 healthy children. J. Anal. Toxicol. 39, 707–713. doi: 10.1093/jat/bkv088
Guo W., Xing Y., Luo X., Li F., Ren M., Liang Y. (2023). Reactive oxygen species: A crosslink between plant and human eukaryotic cell systems. Int. J. Mol. Sci. 24, 13052. doi: 10.3390/ijms241713052
Haidar Z., Fatema K., Shoily S. S., Sajib A. A. (2023). Disease-associated metabolic pathways affected by heavy metals and metalloid. Toxicol. Rep. 10, 554–570. doi: 10.1016/j.toxrep.2023.04.010
Haseeb A., Fozia, Ahmad I., Ullah H., Iqbal A., Ullah R., et al. (2022). Retracted] ecotoxicological assessment of heavy metal and its biochemical effect in fishes. BioMed. Res. Int. 2022, 3787838. doi: 10.1155/2022/3787838
Hermenean A., Damache G., Albu P., Ardelean A., Ardelean G., Ardelean D. P., et al. (2015). Histopatological alterations and oxidative stress in liver and kidney of Leuciscus cephalus following exposure to heavy metals in the Tur River, North Western Romania. Ecotoxicol. Environ. Saf. 119, 198–205. doi: 10.1016/j.ecoenv.2015.05.029
Hossain M. M., Jahan I., Al Nahian A., Zhuang Z., Maxwell S. J., Ali M. Y., et al. (2024). Immediate health risk: Concentration of heavy metals in contaminated freshwater fishes from the river channel of Turag-Tongi-Balu. Environ. Toxicol. 39, 120–134. doi: 10.1002/tox.23959
Hossain M., Rahaman A., Hasan M. J., Uddin M. M., Khatun N., Shamsuddin S. M. (2021). Comparative seasonal assessment of pollution and health risks associated with heavy metals in water, sediment and Fish of Buriganga and Turag River in Dhaka City, Bangladesh. SN Appl. Sci. 3, 509. doi: 10.1007/s42452-021-04464-0
Hussain M. I., Naeem M., Khan Z. I., Akhtar S., Nadeem M., Alnuwaiser M. A., et al. (2022). Cadmium (Cd) and copper (Cu) exposure and bioaccumulation arrays in farm ruminants: impact of forage ecotypes, ecological sites and body organs. Sustainability 14, 12595. doi: 10.3390/su141912595
Islam M. S., Ahmed M. K., Raknuzzaman M., Habibullah-Al-Mamun M., Islam M. K. (2015). Heavy metal pollution in surface water and sediment: a preliminary assessment of an urban river in a developing country. Ecol. Indic. 48, 282–291. doi: 10.1016/j.ecolind.2014.08.016
Jamil Emon F., Rohani M. F., Sumaiya N., Tuj Jannat M. F., Akter Y., Shahjahan M., et al. (2023). Bioaccumulation and bioremediation of heavy metals in fishes—A review. Toxics 11, 510. doi: 10.3390/toxics11060510
Javed M., Ahmad I., Usmani N., Ahmad M. (2016). Studies on biomarkers of oxidative stress and associated genotoxicity and histopathology in Channa punctatus from heavy metal polluted canal. Chemosphere 151, 210–219. doi: 10.1016/j.chemosphere.2016.02.080
Johnston J. E., Lim E., Roh H. (2019). Impact of upstream oil extraction and environmental public health: A review of the evidence. Sci. Total Environ. 657, 187–199. doi: 10.1016/j.scitotenv.2018.11.483
Jomova K., Raptova R., Alomar S. Y., Alwasel S. H., Nepovimova E., Kuca K., et al. (2023). Reactive oxygen species, toxicity, oxidative stress, and antioxidants: Chronic diseases and aging. Arch. Toxicol. 97, 2499–2574. doi: 10.1007/s00204-023-03562-9
Juan C. A., Pérez de la Lastra J. M., Plou F. J., Pérez-Lebeña E. (2021). The chemistry of reactive oxygen species (ROS) revisited: outlining their role in biological macromolecules (DNA, lipids and proteins) and induced pathologies. Int. J. Mol. Sci. 22, 4642. doi: 10.3390/ijms22094642
Kahlson M. A., Dixon S. J. (2022). Copper-induced cell death. Science 375, 1231–1232. doi: 10.1126/science.abo3959
Kang P., Shin H. Y., Kim K. Y. (2021). Association between dyslipidemia and mercury exposure in adults. Int. J. Environ. Res. Public Health 18, 775. doi: 10.3390/ijerph18020775
Kawser Ahmed M., Baki M. A., Kundu G. K., Saiful Islam M., Monirul Islam M., Muzammel Hossain M. (2016). Human health risks from heavy metals in fish of Buriganga river, Bangladesh. SpringerPlus 5, 1–12. doi: 10.1186/s40064-016-3357-0
Kim H. S., Kim Y. J., Seo Y. R. (2015). An overview of carcinogenic heavy metal: molecular toxicity mechanism and prevention. J. Cancer Prev. 20, 232–240. doi: 10.15430/JCP.2015.20.4.23
Klaunig J. E., Wang Z. (2018). Oxidative stress in carcinogenesis. Curr. Opin. Toxicol. 7, 116–121. doi: 10.1016/j.cotox.2017.11.014
Kontoghiorghes G. J. (2023). Iron load toxicity in medicine: from molecular and cellular aspects to clinical implications. Int. J. Mol. Sci. 24, 12928. doi: 10.3390/ijms241612928
Kortei N. K., Heymann M. E., Essuman E. K., Kpodo F. M., Akonor P. T., Lokpo S. Y., et al. (2020). Health risk assessment and levels of toxic metals in fishes (Oreochromis noliticus and Clarias Anguillaris) from Ankobrah and Pra basins: Impact of illegal mining activities on food safety. Toxicol. Rep. 7, 360–369. doi: 10.1016/j.toxrep.2020.02.011
Kulshreshtha D., Ganguly J., Jog M. (2021). Manganese and movement disorders: a review. J. Mov. Disord. 14, 93–102. doi: 10.14802/jmd.20123
Kumar S., Pandey A. (2015). Free radicals: health implications and their mitigation by herbals. Br. J. Med. Med. Res. 7, 438–457. doi: 10.9734/BJMMR/2015/16284
Kumar M., Singh S., Jain A., Yadav S., Dubey A., Trivedi S. P. (2023). A review on heavy metal-induced toxicity in fishes: Bioaccumulation, antioxidant defense system, histopathological manifestations, and transcriptional profiling of genes. J. Trace Elem. Med. Biol. 83, 127377. doi: 10.1016/j.jtemb.2023.127377
Lee S., Cho S.-R., Jeong I., Park J. B., Shin M.-Y., Kim S., et al. (2020). Mercury exposure and associations with hyperlipidemia and elevated liver enzymes: A nationwide cross-sectional survey. Toxics 8, 47. doi: 10.3390/toxics8030047
Lee S.-H., Choi B., Park S.-J., Kim Y.-S., Joo N.-S. (2017). The cut-off value of blood mercury concentration in relation to insulin resistance. J. Obes. Metab. Syndr. 26, 197–203. doi: 10.7570/jomes.2017.26.3.197
Leyssens L., Vinck B., van der Straeten C., Wuyts F., Maes L. (2017). Cobalt toxicity in humans—A review of the potential sources and systemic health effects. Toxicology 387, 43–56. doi: 10.1016/j.tox.2017.05.015
Li X., Fang P., Mai J., Choi E. T., Wang H., Yang X.-F. (2013). Targeting mitochondrial reactive oxygen species as novel therapy for inflammatory diseases and cancers. J. Hematol. Oncol. 6, 1–19. doi: 10.1186/1756-8722-6-19
Liu J., Han X., Zhang T., Tian K., Li Z., Luo F. (2023). Reactive oxygen species (ROS) scavenging biomaterials for anti-inflammatory diseases: From mechanism to therapy. J. Hematol. Oncol. 16, 116. doi: 10.1186/s13045-023-01512-7
Lushchak V. I. (2016). Contaminant-induced oxidative stress in fish: a mechanistic approach. Fish Physiol. Biochem. 42, 711–747. doi: 10.1007/s10695-015-0171-5
Madkour L. (2020). “Heavy metals and free radical-induced cell death mechanisms,” in Reactive Oxygen Species (ROS), Nanoparticles, and Endoplasmic Reticulum (ER) Stress-Induced Cell Death Mechanisms (Elsevier Science, Amsterdam, The Netherlands).
Magnani F., Mattevi A. (2019). Structure and mechanisms of ROS generation by NADPH oxidases. Curr. Opin. Struct. Biol. 59, 91–97. doi: 10.1016/j.sbi.2019.03.001
Mansoor S., Ali A., Kour N., Bornhorst J., Alharbi K., Rinklebe J., et al. (2023). Heavy metal induced oxidative stress mitigation and ROS scavenging in plants. Plants 12, 3003. doi: 10.3390/plants12163003
Martínez-Revelles S., Avendaño M. S., García-Redondo A. B., Alvarez Y., Aguado A., Pérez-Girón J. V., et al. (2013). Reciprocal relationship between reactive oxygen species and cyclooxygenase-2 and vascular dysfunction in hypertension. Antioxid. Redox Sign. 18, 51–65. doi: 10.1089/ars.2011.4335
Mehnaz M., Jolly Y. N., Alam A. R., Kabir J., Akter S., Mamun K. M., et al. (2023). Prediction of hazardous effect of heavy metals of point-source wastewater on fish (Anabas cobojius) and human health. Biol. Trace Elem. Res. 201, 3031–3049. doi: 10.1007/s12011-022-03378-1
Misra S., Boylan M., Selvam A., Spallholz J. E., Björnstedt M. (2015). Redox-active selenium compounds—From toxicity and cell death to cancer treatment. Nutrients 7, 3536–3556. doi: 10.3390/nu7053536
Mitra S., Chakraborty A. J., Tareq A. M., Emran T. B., Nainu F., Khusro A., et al. (2022). Impact of heavy metals on the environment and human health: Novel therapeutic insights to counter the toxicity. J. King Saud Univ. Sci. 34, 101865. doi: 10.1016/j.jksus.2022.101865
Muhammad S., Shah M. T., Khan S. (2011). Health risk assessment of heavy metals and their source apportionment in drinking water of Kohistan region, northern Pakistan. Microchem. J. 98, 334–343. doi: 10.1016/j.microc.2011.03.003
Munir N., Jahangeer M., Bouyahya A., El Omari N., Ghchime R., Balahbib A., et al. (2021). Heavy metal contamination of natural foods is a serious health issue: A review. Sustainability 14, 161. doi: 10.3390/su14010161
Murphy M. P., Bayir H., Belousov V., Chang C. J., Davies K. J., Davies M. J., et al. (2022). Guidelines for measuring reactive oxygen species and oxidative damage in cells and in vivo. Nat. Metab. 4, 651–662. doi: 10.1038/s42255-022-00591-z
Nakamura H., Takada K. (2021). Reactive oxygen species in cancer: Current findings and future directions. Cancer Sci. 112, 3945–3952. doi: 10.1111/cas.15068
Nakhaee S., Amirabadizadeh A., Brent J., Mehrpour O. (2019). Impact of chronic lead exposure on liver and kidney function and haematologic parameters. Basic Clin. Pharmacol. Toxicol. 124, 621–628. doi: 10.1111/bcpt.13179
Naz S., Chatha A. M. M., Danabas D., Khan M. F., Xu Y., Zhu P., et al. (2023). Bioaccumulation pattern and health risk assessment of heavy metals in Cirrhinus mrigala at Panjnad headworks, Bahawalpur, Pakistan. Toxics 11, 596. doi: 10.3390/toxics11070596
Neal A. P., Guilarte T. R. (2012). Mechanisms of heavy metal neurotoxicity: lead and manganese. J. Drug Metabo. Toxicol. 5, 002. doi: 10.1039/C2TX20064C
Niu Y.-Y., Zhang Y.-Y., Zhu Z., Zhang X.-Q., Liu X., Zhu S.-Y., et al. (2020). Elevated intracellular copper contributes a unique role to kidney fibrosis by lysyl oxidase mediated matrix crosslinking. Cell Death Dis. 11, 211. doi: 10.1038/s41419-020-2404-5
Novotny J. A., Peterson C. A. (2018). Molybdenum. Adv. Nutr. 9, 272–273. doi: 10.1093/advances/nmx001
Nyarko E., Boateng C. M., ASamoah O., Edusei M. O., Mahu E. (2023). Potential human health risks associated with ingestion of heavy metals through fish consumption in the Gulf of Guinea. Toxicol. Rep. 10, 117–123. doi: 10.1016/j.toxrep.2023.01.005
Obeng-Gyasi E., Ferguson A. C., Stamatakis K. A., Province M. A. (2021). Combined effect of lead exposure and allostatic load on cardiovascular disease mortality—a preliminary study. Int. J. Environ. Res. Public Health 18, 6879. doi: 10.3390/ijerph18136879
Papadopoulou E., Botton J., Caspersen I. H., Alexander J., Eggesbø M., Haugen M., et al. (2021). Maternal seafood intake during pregnancy, prenatal mercury exposure and child body mass index trajectories up to 8 years. Int. J. Epidemiol. 50, 1134–1146. doi: 10.1093/ije/dyab035
Parvez S., Long M. J., Poganik J. R., Aye Y. (2018). Redox signaling by reactive electrophiles and oxidants. Chem. Rev. 118, 8798–8888. doi: 10.1021/acs.chemrev.9b00066
Peng Y., Hu J., Li Y., Zhang B., Liu W., Li H., et al. (2018). Exposure to chromium during pregnancy and longitudinally assessed fetal growth: findings from a prospective cohort. Environ. Int. 121, 375–382. doi: 10.1016/j.envint.2018.09.003
Pietrzak S., Wójcik J., Baszuk P., Marciniak W., Wojtyś M., Dębniak T., et al. (2021). Influence of the levels of arsenic, cadmium, mercury and lead on overall survival in lung cancer. Biomolecules 11, 1160. doi: 10.3390/biom11081160
Pizzino G., Irrera N., Cucinotta M., Pallio G., Mannino F., Arcoraci V., et al. (2017). Oxidative stress: harms and benefits for human health. Oxid. Med. Cell. Longev. 2017, 8416763. doi: 10.1155/2017/8416763
Quezada-Pinedo H. G., Cassel F., Duijts L., Muckenthaler M. U., Gassmann M., Jaddoe V. W., et al. (2021). Maternal iron status in pregnancy and child health outcomes after birth: a systematic review and meta-analysis. Nutrients 13, 2221. doi: 10.3390/nu13072221
Rady M. M., Elrys A. S., Selem E., Mohsen A. A., Arnaout S. M., El-Sappah A. H., et al. (2023). Spirulina platensis extract improves the production and defenses of the common bean grown in a heavy metals-contaminated saline soil. J. Environ. Sci. 129, 240–257. doi: 10.1016/j.jes.2022.09.011
Rahaman M. S., Rahman M. M., Mise N., Sikder M. T., Ichihara G., Uddin M. K., et al. (2021). Environmental arsenic exposure and its contribution to human diseases, toxicity mechanism and management. Environ. pollut. 289, 117940. doi: 10.1016/j.envpol.2021.117940
Rauf A., Khalil A. A., Awadallah S., Khan S. A., Abu-Izneid T., Kamran M., et al. (2024). Reactive oxygen species in biological systems: Pathways, associated diseases, and potential inhibitors—A review. Food Sci. Nutr. 12, 675–693. doi: 10.1002/fsn3.3784
Reczek C. R., Chandel N. S. (2017). The two faces of reactive oxygen species in cancer. Annu. Rev. Cancer Biol. 1, 79–98. doi: 10.1146/annurev-cancerbio-041916-065808
Reddam A., Mclarnan S., Kupsco A. (2022). Environmental chemical exposures and mitochondrial dysfunction: a review of recent literature. Curr. Environ. Health Rep. 9, 631–649. doi: 10.1007/s40572-022-00371-7
Romaniuk А., Lyndin M., Sikora V., Lyndina Y., Romaniuk S., Sikora K. (2017). Heavy metals effect on breast cancer progression. J. Occup. Med. Toxicol. 12, 1–9. doi: 10.1186/s12995-017-0178-1
Sears C. G., Poulsen A. H., Eliot M., Howe C. J., James K. A., Harrington J. M., et al. (2021). Urine cadmium and acute myocardial infarction among never smokers in the Danish Diet, Cancer and Health cohort. Environ. Int. 150, 106428. doi: 10.1016/j.envint.2021.106428
Shahid M., Pourrut B., Dumat C., Nadeem M., Aslam M., Pinelli E. (2014). Heavy-metal-induced reactive oxygen species: phytotoxicity and physicochemical changes in plants. Rev. Environ. Contam. Toxicol. 232, 1–44. doi: 10.1007/978-3-319-06746-9_1
Shahid M., Xiong T., Castrec-Rouelle M., Leveque T., Dumat C. (2013). Water extraction kinetics of metals, arsenic and dissolved organic carbon from industrial contaminated poplar leaves. J. Environ. Sci. 25, 2451–2459. doi: 10.1016/S1001-0742(12)60197-1
Shahjahan M., Taslima K., Rahman M. S., Al-Emran M., Alam S. I., Faggio C. (2022). Effects of heavy metals on fish physiology–a review. Chemosphere 300, 134519. doi: 10.1016/j.chemosphere.2022.134519
Shaji E., Santosh M., Sarath K., Prakash P., Deepchand V., Divya B. (2021). Arsenic contamination of groundwater: A global synopsis with focus on the Indian Peninsula. Geosci. Front. 12, 101079. doi: 10.1016/j.gsf.2020.08.015
Sharifi-Rad M., Anil Kumar N. V., Zucca P., Varoni E. M., Dini L., Panzarini E., et al. (2020). Lifestyle, oxidative stress, and antioxidants: back and forth in the pathophysiology of chronic diseases. Front. Physiol. 11. doi: 10.3389/fphys.2020.0
Shields H. J., Traa A., Van Raamsdonk J. M. (2021). Beneficial and detrimental effects of reactive oxygen species on lifespan: a comprehensive review of comparative and experimental studies. Front. Cell Dev. Biol. 9. doi: 10.3389/fcell.2021.628157
Siddiqui E., Verma K., Pandey U., Pandey J. (2019). Metal contamination in seven tributaries of the Ganga River and assessment of human health risk from fish consumption. Arch. Environ. Contam. Toxicol. 77, 263–278. doi: 10.1007/s00244-019-00638-5
Sinenko S. A., Starkova T. Y., Kuzmin A. A., Tomilin A. N. (2021). Physiological signaling functions of reactive oxygen species in stem cells: From flies to man. Front. Cell Dev. Biol. 9. doi: 10.3389/fcell.2021.714370
Soleimani-Sardo M., Shirani M., Strezov V. (2023). Heavy metal pollution levels and health risk assessment of dust storms in Jazmurian region, Iran. Sci. Rep. 13, 7337. doi: 10.1038/s41598-023-34318-1
Srivastava V., Gupta S. K., Singh P., Sharma B., Singh R. P. (2018). Biochemical, physiological, and yield responses of lady’s finger (Abelmoschus esculentus L.) grown on varying ratios of municipal solid waste vermicompost. Int. J. Recycl. Org. Waste Agricul. 7, 241–250. doi: 10.1007/s40093-018-0210-1
Sun Q., Li Y., Shi L., Hussain R., Mehmood K., Tang Z., et al. (2022). Heavy metals induced mitochondrial dysfunction in animals: Molecular mechanism of toxicity. Toxicology 469, 153136. doi: 10.1016/j.tox.2022.153136
Taslima K., Al-Emran M., Rahman M. S., Hasan J., Ferdous Z., Rohani M. F., et al. (2022). Impacts of heavy metals on early development, growth and reproduction of fish–A review. Toxicol. Rep. 9, 858–868. doi: 10.1016/j.toxrep.2022.04.013
Tchounwou P. B., Yedjou C. G., Patlolla A. K., Sutton D. J. (2012). Heavy metal toxicity and the environment. Mol. Clin. Environ. Toxicol. Environ. Toxicol. 3, 133–164. doi: 10.1007/978-3-7643-8340-4_6
Titcomb T. J., Liu B., Lehmler H. J., Snetselaar L. G., Bao W. (2021). Environmental nickel exposure and diabetes in a nationally representative sample of US adults. Expo. Health 13, 697–704. doi: 10.1007/s12403-021-00413-9
Tvrda E., Peer R., Sikka S. C., Agarwal A. (2015). Iron and copper in male reproduction: a double-edged sword. J. Assist. Reprod. Genet. 32, 3–16. doi: 10.1007/s10815-014-0344-7
Umeoguaju F. U., Akaninwor J. O., Essien E. B., Amadi B. A. (2022). Heavy metal profile of surface and ground water samples from the Niger Delta region of Nigeria: a systematic review and meta-analysis. Environ. Monit. Assess. 194, 1–37. doi: 10.1007/s10661-021-09688-6
Umeoguaju F. U., Akaninwor J. O., Essien E. B., Amadi B. A., Igboekwe C. O., Ononamadu C. J., et al. (2023). Heavy metals contamination of seafood from the crude oil-impacted Niger Delta Region of Nigeria: A systematic review and meta-analysis. Toxicol. Rep. 11, 58–82. doi: 10.1016/j.toxrep.2023.06.011
Vahter M. (2008). Health effects of early life exposure to arsenic. Basic Clin. Pharmacol. Toxicol. 102, 204–211. doi: 10.1111/j.1742-7843.2007.00168.x
Viana L. F., De Souza D. C. D., Da Silva E. B., Kummrow F., Cardoso C. A. L., Lima N. A., et al. (2023). Bioaccumulation of metals and genotoxic effects in females of Colomesus asellus collected in an Amazon River estuary, Amapá, Brazil. Limnetica 42, 203–214.
Wahiduzzaman M., Islam M. M., Sikder A. H. F., Parveen Z. (2022). Bioaccumulation and heavy metal contamination in fish species of the Dhaleswari River of Bangladesh and related human health implications. Biol. Trace Elem. Res. 200, 3854–3866. doi: 10.1007/s12011-021-02963-0
Wang Y., Qi H., Liu Y., Duan C., Liu X., Xia T., et al. (2021c). The double-edged roles of ROS in cancer prevention and therapy. Theranostics 11, 4839. doi: 10.7150/thno.56747
Wang N., Qiu Y., Hu K., Huang C., Xiang J., Li H., et al. (2021a). One-step synthesis of cake-like biosorbents from plant biomass for the effective removal and recovery heavy metals: Effect of plant species and roles of xanthation. Chemosphere 266, 129129. doi: 10.1016/j.chemosphere.2020.129129
Wang S., Shi X. (2001). Molecular mechanisms of metal toxicity and carcinogenesis. Mol. Cell. Biochem. 222, 3–9. doi: 10.1023/A:1017918013293
Wang X., Son Y.-O., Chang Q., Sun L., Hitron J. A., Budhraja A., et al. (2011). NADPH oxidase activation is required in reactive oxygen species generation and cell transformation induced by hexavalent chromium. Toxicol. Rep. 123, 399–410. doi: 10.1093/toxsci/kfr180
Wang J., Wu T., Fang L., Liu C., Liu X., Li H., et al. (2020a). Peptides from walnut (Juglans mandshurica Maxim.) protect hepatic HepG2 cells from high glucose-induced insulin resistance and oxidative stress. Food Funct. 11, 8112–8121. doi: 10.1039/d0fo01753a
Wang X., Wu Y., Sun X., Guo Q., Xia W., Wu Y., et al. (2021b). Arsenic exposure and metabolism in relation to blood pressure changes in pregnant women. Ecotoxicol. Environ. Saf. 222, 112527. doi: 10.1016/j.ecoenv.2021.112527
Wang T., Zhang J., Xu Y. (2020b). Epigenetic basis of lead-induced neurological disorders. Int. J. Environ. Res. Public Health 17, 4878. doi: 10.3390/ijerph17134878
Wang S., Zheng N., Sun S., An Q., Li P., Li X., et al. (2022). Trends and health risk of trace metals in fishes in Liaodong Bay, China, From 2015 to 2020. Front. Mar. Sci. 8. doi: 10.3389/fmars.2021.789572
Witkowska D., Słowik J., Chilicka K. (2021). Heavy metals and human health: Possible exposure pathways and the competition for protein binding sites. Molecules 26, 6060. doi: 10.3390/molecules26196060
Witt B., Stiboller M., Raschke S., Friese S., Ebert F., Schwerdtle T. (2021). Characterizing effects of excess copper levels in a human astrocytic cell line with focus on oxidative stress markers. J. Trace Elem. Med. Biol. 65, 126711. doi: 10.1016/j.jtemb.2021.126711
Xia B., Yuan J., Pang L., He K. (2022). Chromium [Cr (VI)] exposure causes cytotoxicity of human bronchial epithelial cells (16-HBE) and proteomic alterations. Int. J. Toxicol. 41, 225–233. doi: 10.1177/10915818221078277
Yan L.-J., Allen D. C. (2021). Cadmium-induced kidney injury: Oxidative damage as a unifying mechanism. Biomolecules 11, 1575. doi: 10.3390/biom11111575
Yang H., Liu C.-N., Wolf R. M., Ralle M., Dev S., Pierson H., et al. (2019). Obesity is associated with copper elevation in serum and tissues. Metallomics 11, 1363–1371. doi: 10.1039/c9mt00148d
Yu Y., Liu S., Yang L., Song P., Liu Z., Liu X., et al. (2024). Roles of reactive oxygen species in inflammation and cancer. MedComm 5, e519. doi: 10.1002/mco2.519
Yu M., Zhang J. (2017). Serum and hair nickel levels and breast cancer: systematic review and meta-analysis. Biol. Trace Elem. Res. 179, 32–37. doi: 10.1007/s12011-017-0949-7
Zaghloul G. Y., Eissa H. A., Zaghloul A. Y., Kelany M. S., Hamed M. A., Moselhy K. M. E. (2024). Impact of some heavy metal accumulation in different organs on fish quality from Bardawil Lake and human health risks assessment. Geochem. Trans. 25, 1. doi: 10.1186/s12932-023-00084-2
Zaynab M., Al-Yahyai R., Ameen A., Sharif Y., Ali L., Fatima M., et al. (2022). Health and environmental effects of heavy metals. J. King Saud Univ. Sci. 34, 101653. doi: 10.1016/j.jksus.2021.101653
Zhang J., Wang X., Vikash V., Ye Q., Wu D., Liu Y., et al. (2016). ROS and ROS-mediated cellular signaling. Oxid. Med. Cell. Longev. 2016, 4350965. doi: 10.1155/2016/4350965
Zhao S., Feng C., Quan W., Chen X., Niu J., Shen Z. (2012). Role of living environments in the accumulation characteristics of heavy metals in fishes and crabs in the Yangtze River Estuary, China. Mar. pollut. Bull. 64, 1163–1171. doi: 10.1016/j.marpolbul.2012.03.023
Zhou C.-C., He Y.-Q., Gao Z.-Y., Wu M.-Q., Yan C.-H. (2020). Sex differences in the effects of lead exposure on growth and development in young children. Chemosphere 250, 126294. doi: 10.1016/j.chemosphere.2020.126294
Keywords: heavy metals, fish contamination, reactive oxygen species, oxidative stress, human health
Citation: Wang Y, Noman A, Zhang C, AL-Bukhaiti WQ and Abed SM (2024) Effect of fish-heavy metals contamination on the generation of reactive oxygen species and its implications on human health: a review. Front. Mar. Sci. 11:1500870. doi: 10.3389/fmars.2024.1500870
Received: 24 September 2024; Accepted: 12 November 2024;
Published: 29 November 2024.
Edited by:
Heba H. Abdel-Kader, National Institute of Oceanography and Fisheries (NIOF), EgyptReviewed by:
Tariq Aziz, University of Ioannina, GreeceRania G. Elbaiomy, Sichuan University of Science and Engineering, China
Copyright © 2024 Wang, Noman, Zhang, AL-Bukhaiti and Abed. This is an open-access article distributed under the terms of the Creative Commons Attribution License (CC BY). The use, distribution or reproduction in other forums is permitted, provided the original author(s) and the copyright owner(s) are credited and that the original publication in this journal is cited, in accordance with accepted academic practice. No use, distribution or reproduction is permitted which does not comply with these terms.
*Correspondence: Anwar Noman, YW53YXJub21hbjE1QHlhaG9vLmNvbQ==