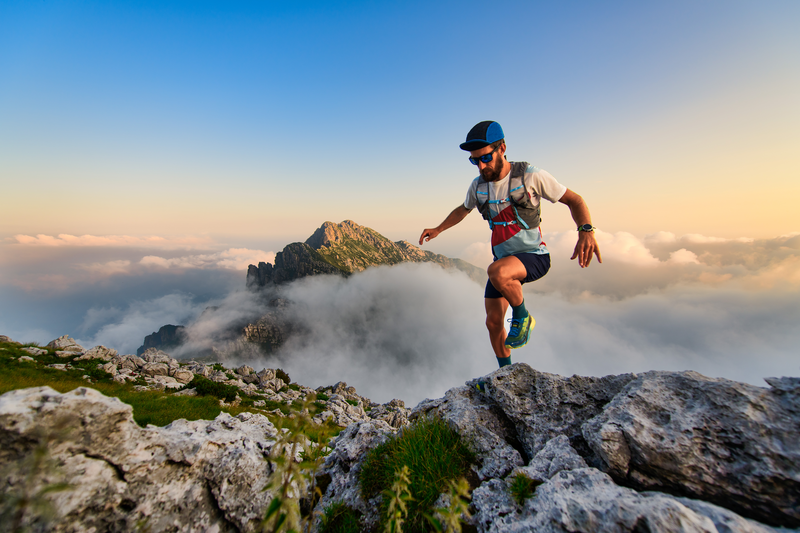
95% of researchers rate our articles as excellent or good
Learn more about the work of our research integrity team to safeguard the quality of each article we publish.
Find out more
ORIGINAL RESEARCH article
Front. Mar. Sci. , 02 December 2024
Sec. Marine Ecosystem Ecology
Volume 11 - 2024 | https://doi.org/10.3389/fmars.2024.1494734
The Arctic is undergoing dramatic changes, including an unprecedented decline in sea ice. Previous studies have shown the severe structuring impact of sea ice scour upon polar intertidal communities. A dramatic example of the influence of Arctic sea ice is the highly depauperate intertidal of Cambridge Bay (Iqaluktuuttiaq) on Victoria Island, Nunavut, Canada. Cambridge Bay intertidal is dominated by a single species of amphipod crustacean, Gammarus setosus, with rare examples of another amphipod, bivalve molluscs, and oligochaetes. Primary producers are limited to a thin algal film, with no macroalgae present shallower than 2 m water depth. This intertidal biodiversity has remained extremely low since it was first surveyed 70 years ago, however, the seasonal sea ice thickness has been in decline for over 50 years. Given the observed dramatic increases in biodiversity and biomass with decreased sea ice cover elsewhere in the Arctic and the presence of the Canadian High Arctic Research Station, we suggest that the intertidal of Cambridge Bay offers an ideal location for a low cost, low effort, and long-term monitoring of biodiversity change and tipping points that may be influenced by sea ice loss in the Arctic as part of a network intertidal monitoring stations.
The biodiversity working group of the Arctic Council has called for the use of science and Traditional Knowledge in long-term, integrated, multi-disciplinary, circumpolar monitoring of the impacts of change in the region through its Arctic Coastal Biodiversity Monitoring Plan (CAFF, 2019). The pace of change in the Arctic is increasing, including unprecedented decline in sea ice and melt of the Greenland ice sheet (Diebold, 2022; Bochow et al., 2023). These changes are likely to have significant impacts on all Arctic ecosystems. In lower latitudes the intertidal zone is often seen as the ‘canary in the coal mine’ since changes in species ranges due to climate change and establishment of invasive species are often observed here first, due to generally easier access and observability than subtidal habitats (Sorte et al., 2017). The Arctic intertidal environment is under pressure from species moving northwards from lower latitudes (Węsławski et al., 2010; Thyrring et al., 2017, 2021). Given their relative accessibility and low cost of monitoring, high latitude intertidal habitats might make useful sentinel habitats to track the impacts of climate change in the shallow marine environment.
Intertidal habitats of the high Arctic differ greatly in observed biodiversity, complexity and abundance depending on location and the local environmental conditions (Ellis and Wilce, 1961; Hansen, 1998; Ronowicz, 2005). Ice scour, ambient sunlight, subaerial exposure to cold temperatures, freshwater input, and high sedimentation regimes all shape Arctic intertidal communities (Renaud et al., 2021; CAFF, 2019) and can be a source of low biodiversity, while, in some areas, sustained human presence has a local impact. The Arctic Coastal Biodiversity Monitoring Plan recognises the high productivity of extensive intertidal zones, with mudflats and rocky shores supporting rich and abundant communities (CAFF, 2019). Ice floes and the vertical movement of fast ice with the tides may have a negative impact on intertidal and subtidal communities, preventing organisms from becoming established (CAFF, 2019; Ellis and Wilce, 1961). Conversely, in some locations an ice foot along the coast might provide a degree of protection from moving ice and insulate against low temperatures (CAFF, 2019). The Coastal Monitoring Plan also identifies and prioritises Focal Ecosystem Components (FECs) to monitor, which for the intertidal included macroalgae (biodiversity and biomass) and macrofauna (biodiversity, abundance, harvest and accessibility and water quality) (CAFF, 2019).
The first high level question of the Arctic Coastal Biodiversity Monitoring Plan is: “What is the status and trend of Arctic coastal ecosystems in terms of their native species composition and condition, new and invasive species, geographic distributions, thresholds with respect to climate drivers, phenological norms, and key processes and functions?” (CAFF, 2019). For monitoring to be effective in detecting change, a baseline knowledge from prior to the recorded environmental change is required. The study of the unique and characteristically low biodiversity habitats of the Arctic, driven by the extreme environmental conditions and seasonality (Renaud et al., 2021), might provide a suitable focus for monitoring rapid change in Arctic coastal ecosystems.
Ellis and Wilce (1961) summarised the shores of the true or high central region of the Canadian Arctic as supporting unusually reduced biodiversity from observations made from 1954 to 1957. Little is known about their methodology or number of replicants other than organisms were hand collected, but they did produce inventories for 12 high Arctic beaches. In the Northwest Passage, between Coronation Gulf and Boothia Peninsula, only two species were collected intertidally, with Mesidothea entomon (Linnaeus, 1758) at Kugluktuk (formerly known as Coppermine) and Gammarus setosus at Cambridge Bay (Ellis and Wilce, 1961). The authors remarked that they were both mobile species and “must have arrived on the shores after the ice had melted in summer”, and did not report any other species, including macroalgae, from Cambridge Bay. They surmised that the main reason for this low biodiversity and abundance of intertidal organisms is caused by the relationship between tidal amplitude, thickness of sea ice and soft sandstone substrates, leading to severe ice scour (Ellis and Wilce, 1961).
Cambridge Bay (Iqaluktuuttiaq) is the largest settlement on Victoria Island, Nunavut, in the Canadian high Arctic (Figure 1). The marine environment in Cambridge Bay is both culturally and economically significant, with Cambridge Bay hosting the largest commercial fishery for Arctic char (Salvelinus alpinus) in Canada (Harris et al., 2016). Despite being a centre of commercial and scientific interest in the Canadian Arctic marine environment, the intertidal environment of Cambridge Bay has been largely ignored, due in part to its apparent lack of visible life. It has been over 70 years since the last dedicated intertidal survey of the bay. In recent decades Cambridge Bay has seen impacts of global climate change upon its environment. The bay has experienced warming air temperatures (0.60 ± 0.2 °C/decade-1), negative and significant trends in precipitation (-0.8 ± 0.4 cm/decade-1) and decreasing sea ice thickness (-4.31 ± 1.4 cm/decade-1) over the last 50+ years (Howell et al., 2016). As these trends continue, the intertidal community of Cambridge Bay will have to respond and adapt to warmer and increasingly ice-free conditions and could soon reach a tipping point, allowing dramatic changes in biodiversity and ecosystem structure and function. The aim of this paper is to assess the current state of the Cambridge Bay intertidal community compared to the only previous study from 70 years ago in the context of ongoing and future climate change.
Biodiversity surveys were undertaken on 10 beaches in August of 2022, with organism abundances counted along beach transects perpendicular to the shoreline, with 23 quadrats (50 cm x 50 cm, 0.25 m2) sampled in total (Figure 1; Table 1). Low, mid and high tide marks were sampled where possible (6 beaches). At beaches 1 and 4 the tidal range was so small that one quadrat width represented the entire tidal range and at beaches 5 and 8 two quadrat widths spanned the tidal range. In addition to the quadrats, timed walking surveys of 20 minutes were undertaken to investigate any unsampled habitat types (e.g. tidal pools, different sediment types or potential refugia from ice scour such as large boulders or crevices), species not included in the quadrat samples and to ensure that the quadrat samples were representative of each beach.
Table 1. Location and description of the sampled beaches in and around Cambridge Bay (Iqaluktuuttiaq), Nunavut.
To place the physical samples into a wider context of the distribution of life on the beaches, we mapped beaches 2, 3, 5, and 6 using a small Parrot Bluegrass quadcopter remotely piloted airborne system (RPAS) flown at 40 m above ground level (AGL) and fitted with a multispectral Sequoia sensor (Red, Green, NIR, red edge 5 MP and 14MP RGB) (Figure 1). Multispectral sensors can detect the differences between biological, geological and anthropogenic items on the beach, enabling the mapping of the distribution of large enough living organisms and some differentiation between them. It was only possible to fly the quadcopter at four beaches due to proximity to the airport and weather conditions. A sunlight calibration sensor was used to automatically compensate for variations in cloud cover and sunlight as flights were undertaken on different days and/or different times of day (Figure 1). Calibration images were taken before and after each flight and one metre-square ground control points (GPCs) were placed in up to 8-10 locations in the flightpaths. Multi-spectral and RGB RPAS imagery was processed in-situ in the field and high-resolution structure-from-motion (SfM) 2D RGB and multispectral orthomosaics (maximum 4-5 cm/pixel) were generated using Pix4D Fields software v. 2.6.2.
To indicate the relative abundance of chlorophyll, we used the Modified Chlorophyll Absorption in Reflectance Index (MCARI) which is, based on the R700/R670 nm wavelength ratio, calculated as [((700nm−670nm)−0.2*(700nm−550nm))*(700nm/670nm)] (Daughtry et al., 2000; Cui et al., 2019). The MCARI index provides an indication of the relative abundance of chlorophyll in the shallow nearshore environment, unaffected by differences in illumination during data collection and reflectance from non-photosynthetic materials, such as soil and beach sand, gravel, and cobbles. For comparison, we also generated maps of Normalised Difference Vegetation Index (NDVI), Green Normalised Difference Vegetation Index (GNDVI), and Normalised Difference Red Edge (NDRE) indices, to highlight plant growth and detect areas of stress or low vegetation cover. Our general conclusion was that MCARI performed better than the other indices at detecting changes in photosynthetic activity in shallow water intertidal multi-spectral RPAS mapping (Figure 2).
Figure 2. Multispectral images of beaches 2, 3, 5 and 6 analysed using the Modified Chlorophyll Absorption in Reflectance Index (MCARI). Black and white circles represent where quadrat sampling occurred on each beach, size of circle is proportional to the distance between samples. Low resolution background satellite imagery ©Mapbox and OpenStreetMap, used under Pix4D licence.
G. setosus was recorded on every beach sampled but not at every tidal height (Supplementary Table S1; Figure 3). Low and mid shore zones had the highest abundances of the amphipod G. setosus, with the high shores of beaches 2, 3, 7, 8, 9 and 10 having no amphipods present. The lowest abundances were seen at beach 8, an exposed beach consisting of large heavy boulders and lacking in soft sediments. The mussel (Musculus discors) was found at beach 4 (Supplementary Table S1), near to the small jetty. Small marine oligochaete worms were found under stones at beaches 7 and 9 (Supplementary Table S1). A Principal Component Analysis (not shown), based upon environmental variables (algal cover, substrate type, height on shore, and location inside or outside of the bay), did not reveal any significant relationship between animal abundances and habitat. This is likely due to the high similarities, soft sediments with overlying stones or rocks, between quadrats that contained G. setosus.
Figure 3. Counts of individual Gammarus setosus (pictured) per quadrat (50 cm x 50 cm, 0.25 m2) per tidal zone at each beach.
In addition to the quadrat survey, the walking survey of the beaches found many G. setosus on beach 1 that were not collected by the quadrat survey. The walking survey also collected two additional species, a large amphipod (Gammaracanthus loricatus) at beaches 1 and 5 and blue mussels (Mytilus sp.) at beach 3. One Musculus discors was also collected at beach 3 and oligochaetes at beaches 1 and 5. A green algal film coated most beaches to some degree but no macroalgae were observed in the intertidal, including in pools or crevices. No life, other than the two species of amphipods recorded and some small fish, were observed down to ~2 m below the low water mark. Beyond 2 m depth macroalgae could be seen.
Our RPAS RGB imagery and multispectral mapping give a wider setting for the transect surveys at four beaches (2, 3, 5 and 6) shown in Figure 2. The obvious advantage of this method is that we were able to map large areas of the intertidal zone at cm-scale resolution, producing more detailed multispectral maps of the intertidal zone than is possible using Sentinel and other commercially available satellite imagery. The MACRI indices obtained at different times, and under variable illumination and cloud cover, are broadly comparable between sites (Figure 2). In-situ MCARI analysis of the multispectral RPAS images revealed strong evidence for the impact of ice scour below the low tide mark, with blue/green areas highlighting relatively low chlorophyll for all mapped beaches (Figure 2). The highest chlorophyll levels (red areas in Figure 2) were dominated by terrestrial vegetation. Orange and red areas along the shoreline represent microalgal film-covered rock on the exposed shore. Orange to red areas offshore represent relative increased productivity with increasing depth in some, but not all, deeper subtidal areas. More extensive mapping of deeper subtidal zones with a larger RPAS that has a greater range and water penetration depth, would be highly beneficial.
Cambridge Bay is characterised by extreme environmental conditions, with the sun continuously below the horizon from approximately the 30th of November to the 11th of January, average daily maximum temperature below -20°C, and no month having an average temperature of 10°C or higher. Seawater temperatures range from an average of 2°C in August to -2°C in January. A layer of sea ice covers the bay for most of the year (except August and September) with a mean maximum thickness of 2.11 ± 0.19 m in April (Howell et al., 2016). Cambridge Bay has an annual tidal range of less than a metre (Howell et al., 2016). However, recent studies have shown that the bounding sills, large freshwater inputs and low nutrient loads characteristic of the Kitikmeot Sea region, including Cambridge Bay, are unique within the Canadian Arctic Archipelago (Sims et al., 2023). The surface water layer of the Kitikmeot Sea is particularly nutrient limited and has very low surface chlorophyll a and phytoplankton biomass, compared with deeper water (Ahmed et al., 2019). Cambridge Bay is also the location of the Canadian High Arctic Research Station (CHARS), supporting ecosystem monitoring in the region (Burke and Limousin, 2019).
The extremely depauperate intertidal community of Cambridge Bay is not typical of other Arctic sites at similar latitudes. Ellis and Wilce (1961) studied 12 high Arctic beaches, with the same methodology of hand collection of macrofauna and megafauna, and only Cambridge Bay and nearby Kugluktuk had single species present, with the others exhibiting diverse communities, macroalgae and zonation. Ellis and Wilce (1961) suggested that the reason for this single species dominance is caused by ice scour due to the relationship between tidal range and thickness of the sea ice and soft sandstone substrates. Sea ice is known to have significant impacts on polar intertidal communities (Waller, 2008, 2013; Petzold et al., 2014) and most of East Antarctica is so impacted by sea ice and glaciers that its intertidal zone is believed to be largely devoid of macroscopic life (Griffiths and Waller, 2016). The multispectral drone images clearly show extensive areas of barren substrate below the low water mark where it is exposed to sea ice scour, compared to the same soft substrates in deeper water with obvious macroalgae and algal films (Figure 2).
In contrast to the intertidal, the subtidal of Cambridge Bay, below 2 m depth, is rich in animal and macroalgal life. The 2015-19 Nearshore Ecological Surveys of Cambridge Bay (Heywood et al., 2016, 2018, 2019; Kent et al., 2015; Schultz et al., 2019), conducted by the Ocean Wise Conservation Association using scuba, found at least 17 morphotypes of macroalgae and >110 morphospecies of benthic marine invertebrates at depths down to a maximum of 35 m. These invertebrate morphospecies comprised at least 8 phyla including: sponges, cnidaria, worms, bryozoans, molluscs, arthropods, echinoderms, and tunicates. Molluscs and echinoderms had the highest numbers of morphospecies, with over 20 of each observed during the surveys. The top ten most abundant invertebrate morphospecies accounted for over 70% of all observations, with the brittlestar, Ophiocten sp., being the most abundant. The diversity and abundance of benthic organisms present in the bay (Dorval, 2017) suggests that there is no restriction caused by food availability or other physical environmental properties, such as temperature or salinity, that might account for the low intertidal biodiversity and biomass. Moreover, at least eight of the macroalgal taxa and over half (at least 65 morphospecies) of the invertebrate morphospecies have been recorded living in the intertidal elsewhere in the Arctic and sub-Arctic, suggesting that conditions on the beaches and upper subtidal of Cambridge Bay are especially challenging. The most likely cause of disturbance is the 2 m thick covering of sea ice for most of the year, scouring the intertidal and shallow subtidal as it rises and falls with the tides. This ice provides some protection from the extreme cold of winter but compacts the beaches and scours the substrate. Despite the presence of a river flowing into the northeast branch of the bay, salinity level remains high in the shallows for all year round, due to brine rejection, except for immediately after the sea ice melt in July (Duke et al., 2021). Recent studies have identified additional species of kelp taxa near Cambridge Bay (Bluhm et al., 2022), but only in deeper waters. Future work in Cambridge Bay should include the identification of the algal film, believed to be colonial diatoms.
Macroalgae and macroinvertebrates are the two main Focal Ecosystem Components to be monitored for the Arctic Coastal Biodiversity Monitoring Plan (CAFF, 2019). Cambridge Bay sits at 69°N and is completely lacking in intertidal macroalgae and most groups of animals, however latitude, including light and nutrient availability and water temperature do not explain the low biodiversity and biomass. In the same publication Ellis and Wilce (1961) found a more diverse intertidal community at Moffet Inlet (72° 15′ N) which comprised macroalgae, fish, bivalves, tunicates, and amphipods. At >69°N, Qeqertarsuaq (historically known as Godhavn) and Akugdlit (Mellemfjord) on the coast of Disko Island on the west coast of Greenland were home to 41 animal taxa and 32 algae (Hansen, 1998). Disko Island experiences a spring tidal range of 2.7 m and had sea ice in the bay for 6–8 months of the year prior to recent warming and weather changes (Taverniers, 2010).
Gammarus setosus was the only abundant macrofaunal species found in this study (Figure 3, Supplementary Table S1) and 60 years ago (Ellis and Wilce, 1961). The other recorded taxa (Gammaracanthus loricatus, Mytilus sp. and Musculus discors) are all common and well distributed throughout the Arctic and further south. The amphipod, G. loricatus, is known to be found in icy environments, including on the underside of sea ice (Buchanan et al., 1977; Grainger et al., 1985; Lønne and Gulliksen, 1991) and in the low intertidal (Ellis and Wilce, 1961). The mussel, M. discors, is known from the shallow waters of Cambridge Bay (Heywood et al., 2016, 2018, 2019; Kent et al., 2015) and is found in the intertidal elsewhere (Ingólfsson, 1996).
G. setosus is a gammarid amphipod found on the northern coasts of both the Atlantic and Pacific oceans, with multiple records from the Canadian Arctic (Steele and Steele, 1975), Svalbard (Węsławski et al., 2018, 2021; Grabowski et al., 2019), Franz Josef Land (Węsławski, 1995) and the Barents Sea (Ikko and Lyubina, 2010). This species is typically found in the benthic subtidal or low intertidal regions (Steele and Steele, 1970). G. setosus has also been observed on the underside of sea ice in Resolute Bay and Bridport Inlet, Nunavut (Green and Steele, 1975; Buchanan et al., 1977) and as a component of the “cryopelagic biocenosis” in Franz Josef Land (Golikov and Scarlato, 1973). G. setosus dominated the biomass on four species-poor gravel beaches in Hornsund Fjord, West Spitsbergen, where the intertidal zone is covered by an ice foot from autumn to late June (Ronowicz, 2005). The wider tidal range of the beaches, ~5 m, supported a more diverse community (12 macrofaunal taxa) than Cambridge Bay. Communities dominated by G. setosus on Svalbard are generally found among and under loose stones and boulders in soft sediments on sheltered beaches (Węsławski et al., 1993, 1997, 2021; Alonso, 2016), similar to the conditions observed in Cambridge Bay.
Amphipod abundances varied greatly among different beaches and tidal heights, with the mid-tide zone of beach 10 having the highest abundances (Figure 3). Beaches 2, 6 and 10 had the highest abundances of G. setosus, with habitats consisting of stones on soft sediment and a wide enough tidal range for three zones (high, mid and low tide) to be sampled. The low abundances of beach 1 are likely due to the local freshwater inputs from the sewage outflow and very narrow tidal range (Figures 1, 3, Supplementary Tables S1; S2). Beach 8 had very low abundances and was an exposed location with large boulders and lacking soft sediments and smaller rocks for the amphipods to hide under. The very low abundance or absence of amphipods at high water is likely driven by exposure to predation from birds (Grabowski et al., 2019) and probable avoidance of dehydration. Adult G. setosus are opportunistic feeders (Dischereit et al., 2024) and are likely to be feeding on any organic matter they find in the intertidal of Cambridge Bay.
Climate change is impacting and changing the structure and function of the Arctic intertidal. The rocky shores of Sorkappland (77°N and a tidal range of 1.8 m), Svalbard, were home to 19 species when surveyed in 1988 and had increased to 43 species in 2008 (Węsławski et al., 2010), with an expansion of Subarctic boreal species, while Arctic species retreated. In 1988 the bay was devoid of macroalgae and there was long-lasting sea ice cover. However, in 2008 there was a clear increase in overall intertidal biomass and Fucus distychus and barnacles were more widespread along the shore. Environmental changes within the bay over the 20 years included reductions in pack and fast ice and increased turbidity from melting glaciers (Węsławski et al., 2010). On the west coast of Greenland, a study including biodiverse intertidal habitats dominated by macroalgae across a range of latitudes found that climate change may lead to an increase in intertidal biomass in north Greenland, but it is unlikely to drive functional or structural ecosystem change in the near future (Thyrring et al., 2021). Macroalgal canopies and the geomorphology of the rocky substrate can heavily influence the structure of sub-Arctic intertidal communities by reducing thermal stress and increasing habitat complexity (Ørberg et al., 2018). Whilst Cambridge Bay has maintained its low biodiversity, probably due to the heavy and persistent sea ice cover, it is not immune to the impacts of climate change and is likely to experience the ecological impacts of warming in the coming decades. The unusually low biodiversity of the Cambridge Bay intertidal may be one of the first and most obvious signs of a tipping point being breached.
As has been seen in the last 20 years in Svalbard, changes to sea ice conditions, caused by global climate change, have dramatic impacts on high Arctic intertidal habitats. The Cambridge Bay intertidal and immediate subtidal represent an extreme example of a sea ice impacted community, under a monitored and changing sea ice regime. The lack of macroalgae and dominance of a single species of macroinvertebrate make monitoring any change in Focal Ecosystem Components for the Arctic Coastal Biodiversity Monitoring Plan simple and accessible without expert taxonomic skills (CAFF, 2019). In contrast, the deeper subtidal of the bay is already known to be home to over 70 species of macroalgae and invertebrates capable of surviving in the intertidal elsewhere. These local species are likely to become new colonists of the Cambridge Bay intertidal should conditions become more favourable due to climate change. Given its relatively accessible location and the presence of the Canadian High Arctic Research Station, the Cambridge Bay intertidal offers the ideal location for a low cost, low effort and long-term monitoring of biodiversity change associated with sea ice loss and other environmental change and should form part of the ongoing monitoring efforts in the region. Annual transect and RPAS surveys during summer months to detect increased biodiversity and range expansions (especially the presence of sessile animals or macroalgae), between the high tide mark and ~2 m below low tide, could produce real-time monitoring and evidence of tipping points and change in the ecosystem. Such monitoring should include Inuit Qaujimajatuqangit and school science activities and allow for greater interaction between researchers and the local community.
The original contributions presented in the study are included in the article/Supplementary Material, further inquiries can be directed to the corresponding author/s.
HG: Conceptualization, Data curation, Formal analysis, Funding acquisition, Investigation, Methodology, Project administration, Writing – original draft, Writing – review & editing. CW: Conceptualization, Data curation, Formal analysis, Funding acquisition, Investigation, Methodology, Writing – original draft, Writing – review & editing. SR: Conceptualization, Data curation, Formal analysis, Funding acquisition, Investigation, Methodology, Writing – original draft, Writing – review & editing. AJ: Formal analysis, Writing – original draft. DH: Writing – original draft, Writing – review & editing.
The author(s) declare financial support was received for the research, authorship, and/or publication of this article. The research leading to these results has received funding from the European Union’s Horizon 2020 project INTERACT, under grant agreement No 730938.
All fieldwork was carried out under a licence to Fish for Scientific Purposes issued pursuant to Section 52 of the Fishery (General) Regulations, licence number S-22/23-1038-NU. We would like to thank the people of Cambridge Bay, Ekaluktutiak Hunters & Trappers Organization, Government of Nunavut Department of Environment, Polar Knowledge Canada, Jennifer Hubbard, Christopher Arko, Martin Léger, Hannele Savela, and all the staff at the Canadian High Arctic Research Station (CHARS) for making our fieldwork possible and for making us feel welcome.
The authors declare that the research was conducted in the absence of any commercial or financial relationships that could be construed as a potential conflict of interest.
All claims expressed in this article are solely those of the authors and do not necessarily represent those of their affiliated organizations, or those of the publisher, the editors and the reviewers. Any product that may be evaluated in this article, or claim that may be made by its manufacturer, is not guaranteed or endorsed by the publisher.
The Supplementary Material for this article can be found online at: https://www.frontiersin.org/articles/10.3389/fmars.2024.1494734/full#supplementary-material
Ahmed M., Else B. G. T., Burgers T. M., Papakyriakou T. N. (2019). Variability of surface water pCO2 in the Canadian Arctic Archipelago from 2010 to 2016. J. Geophys Res. Oceans 124, 1876–1896. doi: 10.1029/2018JC014639
Alonso M. V. (2016). ‘Bioaccumulation and effects of parent and alkylated PAHs in an Arctic marine amphipod (Gammarus setosus) originating from pristine and historically contaminated sites in Svalbard. Implications for sensitivity and adaptation’. Master's thesis (UiT The Arctic University of Norway) 2016.
Bluhm B. A., Brown K., Rotermunc L., Williams W., Danlelsen S., Carmack E. C. (2022). New distribution records of kelp in the Kitikmeot Region, Northwest Passage, Canada, fill a pan-Arctic gap. Polar Biol. 45, 719–736. doi: 10.1007/s00300-022-03007-6
Bochow N., Poltronieri A., Robinson A., Montoya M., Rypdal M., Boers N. (2023). Overshooting the critical threshold for the Greenland ice sheet. Nature 622, 528–536. doi: 10.1038/s41586-023-06503-9
Buchanan R. A., Cross W. E., Thomson D. H. (1977). Survey of the marine environment of Bridport Inlet, Melville Island (LGL Limited, Environmental Research Associates).
Burke D. C., Limousin M. (2019). An interview with Marla Limousin. Arctic 72, 329–334. doi: 10.14430/arctic69026
CAFF (2019). Arctic Coastal Biodiversity Monitoring Plan (Akureyri, Iceland: Conservation of Arctic Flora and Fauna International Secretariat), ISBN: ISBN 978-9935-431-76-9.
Cui B., Zhao Q., Huang W., Song X., Ye H., Zhou X. (2019). A new integrated vegetation index for the estimation of winter wheat leaf chlorophyll content. Remote Sens. 11, 974. doi: 10.3390/rs11080974
Daughtry C. S., Walthall C. L., Kim M. S., De Colstoun E. B., McMurtrey Iii J. E. (2000). Estimating corn leaf chlorophyll concentration from leaf and canopy reflectance. Remote Sens. Environ. 74, 229–239. doi: 10.1016/S0034-4257(00)00113-9
Diebold F. X. (2022). When will arctic sea ice disappear?: projections of area, extent, thickness, and volume. J. Econometrics 236, 105479. doi: 10.3386/w30732
Dischereit A., Beermann J., Lebreton B., Wangensteen O. S., Neuhaus S., Havermans C. (2024). DNA metabarcoding reveals a diverse, omnivorous diet of Arctic amphipods during the polar night, with jellyfish and fish as major prey. Front. Mar. Sci. 11, 1327650. doi: 10.3389/fmars.2024.1327650
Dorval J. (2017). Variation temporelle à haute résolution de la dynamique des assemblages benthiques infratidales à Cambridge Bay, NU (Arctique) (Canada: Universite du Quebec a Rimouski).
Duke P. J., Else B. G. T., Jones S. F., Marriot S., Ahmed M. M. M., Nandan V., et al. (2021). Seasonal marine carbon system processes in an Arctic coastal landfast sea ice environment observed with an innovative underwater sensor platform. Elementa: Sci. Anthropocene 9, 00103. doi: 10.1525/elementa.2021.00103
Ellis D. V., Wilce R. T. (1961). Arctic and subarctic examples of intertidal zonation. Arctic 14, 224–235. doi: 10.14430/arctic3676
Golikov A. N., Scarlato O. A. (1973). Comparative characteristics of some ecosystems of the upper regions of the shelf in tropical, temperate and Arctic waters. Helgoländer wissenschaftliche Meeresuntersuchungen 24, 219–234. doi: 10.1007/BF01609513
Grabowski M., Jabłońska A., Weydmann-Zwolicka A., Gantsevich M., Strelkov P., Skazina M., et al. (2019). Contrasting molecular diversity and demography patterns in two intertidal amphipod crustaceans reflect Atlantification of High Arctic. Mar. Biol. 166, 155. doi: 10.1007/s00227-019-3603-4
Grainger E. H., Mohammed A. A., Lovrity J. E. (1985). The sea ice fauna of Frobisher Bay, Arctic Canada. Arctic 38, 23–30. doi: 10.14430/arctic2103
Green J. M., Steele D. H. (1975). “Observations on marine life beneath sea ice, Resolute Bay,” in NWT proceedings, circumpolar conference on northern ecology national research council of Canada II. 77–86.
Griffiths H. J., Waller C. L. (2016). The first comprehensive description of the biodiversity and biogeography of Antarctic and Sub-Antarctic intertidal communities. J. biogeography 43, 1143–1155. doi: 10.1111/jbi.2016.43.issue-6
Hansen L. (1998). “The intertidal macrofauna and macroalgae at five Arctic localities (Disko, West Greenland),” in The 1998 Danish-German excursion to Disko Island, West Greenland, Book 330. Eds. Brandt A., Thomsen H. A., Heide-Jorgensen H., Kristensen R. M., Ruhberg H. (Alfred Wegener Institut für Polar und Meeresforschung, Bremerhaven), 92–110.
Harris L. N., Moore J. -S., Bajno R., Tallman R. F. (2016). [amp]]lsquo;Genetic stock structure of Anadromous arctic char in Canada’s central arctic: potential implications for the management of Canada’s largest arctic char commercial fishery. North Am. J. Fisheries Manage. 36, 1473–1488. doi: 10.1080/02755947.2016.1227399
Heywood J., Kent D., Gibbs D., Borden L., Neale M., Lisaingo J., et al. (2016). 2016 Nearshore Ecological Survey (Vancouver Aquarium Marine Science Centre).
Heywood J. D., Borden L., Schultz J., Kent D., Neale M., Martinello C., et al. (2018). 2018 Nearshore Ecological Survey (Ocean Wise Conservation Association).
Heywood J., Kulcsar C., Hung B., Weltman A., Acharya-Patel N. (2019). 2019 Nearshore Ecological Survey (Ocean Wise Conservation Association).
Howell S. E., Laliberté F., Kwok R., Derksen C., King J. (2016). Landfast ice thickness in the Canadian Arctic Archipelago from observations and models. cryosphere 10, 1463–1475. doi: 10.5194/tc-10-1463-2016
Ikko N. V., Lyubina O. S. (2010). Distribution of the genus Gammarus (Crustacea, Amphipoda) along the coast of Arctic fjords as an indicator of prevailing environmental conditions. Doklady Biol. Sci. 431, 149–151. doi: 10.1134/S0012496610020213
Ingólfsson A. (1996). The distribution of intertidal macrofauna on the coasts of Iceland in relation to temperature. Sarsia 81, 29–44. doi: 10.1080/00364827.1996.10413609
Kent D., Gibbs Neale M., Heywood J. (2015). 2015 Nearshore Ecological Survey (Vancouver Aquarium Marine Science Centre).
Lønne O. J., Gulliksen B. (1991). Sympagic macro-fauna from multiyear sea-ice near Svalbard. Polar Biol. 11, 471–477. doi: 10.1007/BF00233082
Ørberg S. B., Krause-Jensen D., Mouritsen K. N., Olesen B., Marbà N., Larsen M. H., et al. (2018). Canopy-forming macroalgae facilitate recolonization of sub-arctic intertidal fauna and reduce temperature extremes. Front. Mar. Sci. 5, 332. doi: 10.3389/fmars.2018.00332
Petzold W., Willers M. T., Scrosati R. A. (2014). Visual record of intertidal disturbance caused by drift ice in the spring on the Atlantic coast of Nova Scotia. F1000Research 3, 112. doi: 10.12688/f1000research
Renaud P. E., Węsławski J. M., Conlan K. (2021). "Ecology of Arctic shallow subtidal and intertidal benthos", in Arctic Ecology ed. Thomas D. N. (Hoboken, NJ: Wiley), 289–324. doi: 10.1002/9781118846582.ch11
Ronowicz M. (2005). Species diversity of Arctic gravel beach: case study for species poor habitats. Polish Polar Res. 26, 287–297.
Schultz J., Heywood J., Gibbs D., Borden L., Kent D., Neale M., et al. (2019). Arctic marine ecology benchmarking program: Monitoring biodiversity using scuba. Polar Knowledge 1, 39–45. doi: 10.35298/pkc.2018.05
Sims R. P., Ahmed M. M., Butterworth B. J., Duke P. J., Gonski S. F., Jones S. F., et al. (2023). High interannual surface pCO2 variability in the southern Canadian Arctic Archipelago’s Kitikmeot Sea. Ocean Sci. 19, 837–856. doi: 10.5194/os-19-837-2023
Sorte C. J. B., Davidson V. E., Franklin M. C., Benes K. M., Doellman M. M., Etter R. J., et al. (2017). Long-term declines in an intertidal foundation species parallel shifts in community composition. Global Change Biol. 23, 341–352. doi: 10.1111/gcb.2017.23.issue-1
Steele V. J., Steele D. H. (1970). The biology of Gammarus (Crustacea, Amphipoda) in the northwestern Atlantic. II. Gammarus setosus Dementieva. Can. J. zoology 48, 659–671. doi: 10.1139/z70-124
Steele D. H., Steele V. J. (1975). The biology of Gammarus (Crustacea, Amphipoda) in the northwestern Atlantic. XI. Comparison and discussion. Can. J. zoology 53, 1116–1126. doi: 10.1139/z75-131
Taverniers P. (2010). “Weather variability and changing sea ice use in qeqertaq, west Greenland 1987–2008,” in SIKU: Knowing Our Ice: Documenting Inuit Sea Ice Knowledge and Use. Ed. Krupnik I., et al (Springer Netherlands, Dordrecht), 31–44. doi: 10.1007/978-90-481-8587-0_2
Thyrring J., Blicher M. E., Sørensen J. G., Wegeberg S., Sejr M. K. (2017). Rising air temperatures will increase intertidal mussel abundance in the Arctic. Mar. Ecol. Prog. Ser. 584, 91–104. doi: 10.3354/meps12369
Thyrring J., Wegeberg S., Blicher M. E., Krause‐Jensen D., Høgslund S., Olesen B., et al. (2021). Latitudinal patterns in intertidal ecosystem structure in West Greenland suggest resilience to climate change. Ecography 44, 1156–1168. doi: 10.1111/ecog.2021.v44.i8
Węsławski J. M., Wiktor J., Zajączkowski M., Swerpel S. (1995). Gammarus (Crustacea, Amphipoda) from Svalbard and Franz Josef land. Distribution and density. Oceanographic Literature Rev. 7, 559. doi: 10.1080/00364827.1994.10413553
Węsławski J. M., Wiktor J., Zajączkowski M., Swerpel S. (1993). Intertidal zone of Svalbard. Polar Biol. 13, 73–79. doi: 10.1007/BF00238538
Węsławski J. M., Wiktor J., Szymelfenig M. (1997). Intertidal zone of Svalbard3. Littoral of a subarctic, oceanic island: Bjornoya. Polar Biol. 18, 45–52. doi: 10.1007/s003000050157
Węsławski J. M., Dragańska-Deja K., Legeżyńska J., Walczowski W. (2018). Range extension of a boreal amphipod Gammarus oceanicus in the warming Arctic. Ecol. Evol. 8, 7624–7632. doi: 10.1002/ece3.2018.8.issue-15
Węsławski J. M., Legeżyńska J., Kotwicki L., Mazurkiewicz M., Olenin S. (2021). Gammarus (Amphipoda) species competitive exclusion or coexistence as a result of climate change in the Arctic? Polish Polar Res. 42, 287–302. doi: 10.24425/ppr.2021.138586
Węsławski J. M., Wiktor J., Kotwicki L. (2010). Increase in biodiversity in the arctic rocky littoral, Sorkappland, Svalbard, after 20 years of climate warming. Mar. biodiversity 40, 123–130. doi: 10.1007/s12526-010-0038-z
Waller C. L. (2008). Variability in intertidal communities along a latitudinal gradient in the Southern Ocean. Polar Biol. 31, 809–816. doi: 10.1007/s00300-008-0419-y
Keywords: intertidal, Arctic, low biodiversity, amphipod, climate change, sea ice
Citation: Griffiths HJ, Waller CL, Roberts SJ, Jażdżewska AM and Hik DS (2024) Extremely low biodiversity Arctic intertidal habitats as sentinels for environmental change. Front. Mar. Sci. 11:1494734. doi: 10.3389/fmars.2024.1494734
Received: 11 September 2024; Accepted: 13 November 2024;
Published: 02 December 2024.
Edited by:
Susana Carvalho, King Abdullah University of Science and Technology, Saudi ArabiaReviewed by:
Jan Marcin Weslawski, Polish Academy of Sciences, PolandCopyright © 2024 Griffiths, Waller, Roberts, Jażdżewska and Hik. This is an open-access article distributed under the terms of the Creative Commons Attribution License (CC BY). The use, distribution or reproduction in other forums is permitted, provided the original author(s) and the copyright owner(s) are credited and that the original publication in this journal is cited, in accordance with accepted academic practice. No use, distribution or reproduction is permitted which does not comply with these terms.
*Correspondence: Huw J. Griffiths, aGpnQGJhcy5hYy51aw==
Disclaimer: All claims expressed in this article are solely those of the authors and do not necessarily represent those of their affiliated organizations, or those of the publisher, the editors and the reviewers. Any product that may be evaluated in this article or claim that may be made by its manufacturer is not guaranteed or endorsed by the publisher.
Research integrity at Frontiers
Learn more about the work of our research integrity team to safeguard the quality of each article we publish.