- State Key Laboratory of Mariculture Breeding, Fisheries College of Jimei University, Xiamen, China
SIFamide is an amidated neuropeptide with a conserved Ser-Ile-Phe structure at its C-terminal, which is crucial in insect courtship, sleep and feeding in insects. However, little information exists regarding its function in crustaceans. This study aimed to identify the cDNA of Sp-SIFa and its two putative receptors (Sp-SIFaR1 and Sp-SIFaR2) in the mud crab Scylla paramamosain. The expression profiles of Sp-SIFa and its receptors signaling were investigated to explore their potential roles. The results indicated that Sp-SIFa was expressed primarily in the nervous tissues and the midgut, and Sp-SIFaR1 was extensively expressed in the eyestalk ganglion, cerebral ganglion, hepatopancreas and the ovary whereas Sp-SIFaR2 was restricted to the eyestalk ganglion and ovary. The Sp-SIFa expression in the cerebral ganglion was significantly increased at the late vitellogenic stage during the ovarian development. The expression patterns of Sp-SIFaR1 and Sp-SIFaR2 in the ovary were similar to Sp-SIFa, indicating a potential function in regulating ovarian development. Subsequently, in vitro and in vivo experiments were performed to further support this hypothesis. The expression of Sp-Vg and Sp-VgR was significantly induced by Sp-SIFa peptide in vitro and in vivo. Moreover, prolonged injection of the Sp-SIFa peptide caused a significant increase in Sp-VgR expression, oocyte diameter and gonadal development index, demonstrating a possible stimulatory effect on ovarian development. In conclusion, our results suggest that Sp-SIFa may regulate ovarian development by promoting Vg biosynthesis and oocyte uptake in the mud crab S. paramamosain.
1 Introduction
In arthropods, neuropeptides are important signaling molecules to regulate metamorphosis, ecdysis, growth, and reproduction (Charmantier et al., 1997). In crustaceans, past studies have predominantly focus on the neurohormones derived from the eyestalk ganglia, where the X-organ and sinus gland complexes play central roles in the regulation of gonadal development. It has been long known that vitellogenesis inhibiting hormone (VIH) plays an inhibitory role in ovarian development (Tsutsui et al., 2013). Recently, many neuropeptides have been identified in the nervous system of crustaceans using high-throughput sequencing technology; however, their functions in reproduction remain largely unexplored. Therefore, it is intriguing to identify gonadotrophic neuropeptides in crustaceans, which can be applied to the artificial breeding technology of economically significant species.
SIFa is an amidated neuropeptide that characterized by a conserved Ser-Ile-Phe structure in its C-terminal, with 12 amino acid residues in length (Verleyen et al., 2009). There are three isoforms of SIFa that have been identified in arthropods, which are distinguished by a single amino acid residue at the end of the amino (N) terminus: Ala1-SIFa, Gly1-SIFa and Val1-SIFa. AYRKPPFNGSIFamide (Ala1-SIFa), Gly1-SIFa, and Val1-SIFa were first identified in the grey fly Neobellieria bullata (Janssen et al., 1996), the giant tiger prawn Penaeus monodon (Sithigorngul et al., 2002), and the American lobster Homarus americanus (Dickinson et al., 2008), respectively. To date, a number of SIFa and its paralogs have been identified in insects and crustaceans through the application of mass spectrometry and next generation sequencing, for example, the Jonah crab Cancer borealis (Huybrechts et al., 2003) the red swamp crayfish Procambarus clarkii (Yasuda et al., 2004) and the mud crab S. paramamosain (Bao et al., 2015).
The findings regarding physiological effect of SIFa are primarily mediated by insects. In Drosophila melanogaster, SIFa is involved in the regulating various physiological processes, such as courtship (Sellami and Veenstra, 2015), sleep (Huang et al., 2021) and feeding (Martelli et al., 2017). Similarly, the role of SIFa in regulating feeding behavior has been reported in Rhodnius prolixus (Ayub et al., 2020). In Rhyparobia maderae, injection of SIFa delayed locomotor activity rhythms in a circadian time-dependently (Arendt et al., 2015). To date, few studies have documented the biological functions of SIFa in crustacean species. SIFa has been reported to regulate heartbeat in C. borealis and Cancer irroratus (Dickinson et al., 2019), and aggression and dominant behavior in the freshwater prawn Macrobrachium rosenbergii (Vázquez-Acevedo et al., 2009).
The first SIFa receptor (SIFaR) was identified in D. melanogaster (Jørgensen et al., 2006). Subsequently, it was discovered in the genomes of the black-legged tick Ixodes scapularis (Šimo et al., 2013), silkworm Bombyx mori (Fan et al., 2010), red flour beetle Tribolium castaneum (Hauser et al., 2008) and bumblebee Bombus terrestris (Lismont et al., 2018). Two SIFa receptors exist in the American cockroach Periplaneta americana (Veenstra, 2021) and the brown planthopper Nilaparvata lugens (Tanaka et al., 2014). It was observed that activation of its receptor by the SIFa neuropeptide mobilizes Ca2+ accumulation in the cytoplasm, which regulates the expression of downstream genes and induces corresponding physiological effects (Jékely, 2013). SIFa/SIFaR is homologous to the vertebrate gonadotropin inhibitory hormone (GnIH) and its receptor (GnIHR), which is conserved from fish to humans and play a key role in inhibiting reproduction (Ubuka and Tsutsui, 2014). consequently, we hypothesized that SIFa/SIFaR are involved in the regulation of reproduction in crustaceans was proposed in view of this similarity.
The mud crab (S. paramamosain) is an important marine culture crustacean. It takes a long time for female crabs to develop into gonadally mature crabs, which results in high culture costs in aquaculture and constrains the development of mud crab culture industry. In this study, we aimed to investigate the putative role of Sp-SIFa and its receptors in the ovarian development of mud crabs. First, cDNA sequences of Sp-SIFa and its possible receptors were obtained by transcriptome sequencing and molecular cloning. Subsequently, their expression profiles were determined by reverse transcription polymerase chain reaction (RT-PCR) and Real-time Quantitative polymerase chain reaction (qPCR). Finally, in vitro and in vivo experiments were performed to explore the putative effects of Sp-SIFa on ovarian development.
2 Materials and methods
2.1 Animals
Wild-caught female mud crabs (S. paramamosain) were purchased from a local fish market in Xiamen city of Fujian Province, China. They were acclimated for one week at a temperature of 28 ± 1°C and a salinity of 28 ± 0.5 ppt. During this period, the crabs were fed with clams (Ruditapes philippinarum) and the seawater was refreshed daily.
2.2 cDNA cloning and sequences analysis
Total RNA was extracted using TRIzol Reagent (Invitrogen, USA) according to the manufacturer’s instructions. The quantity and quality of RNA were determined using a NanoDrop spectrophotometer (Thermo Fisher Scientific) and 2% agarose gel electrophoresis, respectively. The first-strand cDNA was generated from 1 μg of total RNA using a PrimeScript™ RT reagent Kit with a gDNA Eraser (Taraka, Japan). From the transcriptome library, the sequences encoding Sp-SIFa and its two receptors were screened, and the complete coding sequences were subsequently obtained by PCR.
The online website (https://www.ncbi.nlm.nih.gov/) was used to predicted the open reading frame (ORF) of Sp-SIFa and its two putative receptors. Domains of the protein sequences were predicted using the online website SMART (https://smart.embl-heidelberg.de/). Multiple sequence alignments were performed using an online tool (https://www.bioladder.cn/web/#/chart/29). A phylogenetic tree was constructed using the neighbor-joining (NJ) method with 1000 bootstrap replicates using MEGA (version 11). Since the SIFa receptors (SIFaRs) were only identified in insects, thus, SIFaRs in fifteen insect species and two crustacean species (Portunus trituberculatus and Penaeus indicus) were used for phylogenetic analysis.
2.3 Expression profiles of Sp-SIFa, Sp-SIFaR1 and Sp-SIFaR2
Tissue distribution analysis was performed by RT-PCR. 11 of tissues (eyestalk ganglion, cerebral ganglion, thoracic ganglion, Y-organ, gill, heart, middle gut, ovary, hepatopancreas, muscle and stomach) were collected from crabs at the early vitellogenic stage. The PCR was conducted using the primer pairs of Sp-SIFa-qF/-qR, Sp-SIFaR1-qF/-qR and Sp-SIFaR2-qF/-qR respectively. Additionally, the housekeeping gene β-actin was amplified as an internal control, and the amplification of deionized water was used as a negative control. PCR was performed with Ex-Taq® DNA polymerase (Takara) under the following program: 95°C for 3 min, 35 cycles of 95°C for 30 s, 59°C for 30 s, 72°C for 30 s and followed by 72°C for 10 min. Finally, the PCR products were subjected to 2% agarose gel electrophoresis analysis and imaged using a UV gel imager.
Vitellogenesis of the mud crab S. paramamosain was divided into pre-vitellogenic stage, early vitellogenic stage, and late vitellogenic stage based on the characteristics, including color, gonadal development index, and histological features of the ovary (Huang et al., 2014). At the pre-vitellogenic stage, the ovaries are off white to creamy white, then turn to pale or light yellow at the early vitellogenic stage and finally orange to reddish-orange at the late vitellogenic stage. In this study, the expression profile of Sp-SIFa, Sp-SIFaR1 and Sp-SIFaR2 of the mud crabs (n = 4) at three different stages were detected by qPCR. Primers used in this study were listed in Table 1.
2.4 In vitro effects of Sp-SIFa peptide on Sp-Vg and Sp-VgR expression
The mature Sp-SIFa peptide (GYRKPPFNGSIFamide) was synthesized (GL Biochem Ltd, Shanghai, China) with a purity of 98% for the subsequent experiments. The sequence identity of the synthetic Sp-SIFa peptide was determined by mass spectrometry.
The crabs at the early vitellogenic stage were used for the in vitro experiment. Crabs were placed on ice for 10 minutes to anesthetize, and the ovary samples and hepatopancreas tissues were dissected from the crabs. The samples were washed five times with crab saline containing penicillin G (300 IU/ml) and streptomycin (300 IU/ml). Subsequently, the tissues were cultured in 500 μL of L-15 medium containing penicillin G and streptomycin in 24-well plates at 26°C. After 1 hour, the tissues were treated with synthetic Sp-SIFa peptide at concentrations of 0, 1, 10, and 100 nM. The Sp-SIFa peptide was prepared in an L-15 medium containing penicillin G and streptomycin and each treatment was repeated five times (n = 5). For total RNA extraction and cDNA synthesis, ovary and hepatopancreas samples were collected at the 2nd, 4th, and 6th hour post Sp-SIFa treatment. Finally, the expressions of Sp-VgR (GenBank accession number: KF860893.1) and Sp-Vg (GenBank accession number: FJ812090.1) were detected by qPCR to explore the potential effects of Sp-SIFa on vitellogenesis.
2.5 In vivo effect of Sp-SIFa peptide on ovarian development
After establishing the stimulatory effect of Sp-SIFa on vitellogenesis in S. paramamosain, we performed in vivo experiments to further explore its specific involvement in ovarian development. In the short-term experiment, mud crabs in the early vitellogenic stage were randomly divided into two groups, each has five individuals (n = 5). Crabs were then injected with Sp-SIFa peptide (15 ng/g body mass) prepared in 100 μL crab saline, whereas the control group was received 100 μL crab saline instead. Samples of ovary and hepatopancreas were dissected from the crabs at 12th h post the injection for Sp-Vg and Sp-VgR gene expression analysis.
Based on the results of the short-term experiment, a long-term experiment was conducted by prolonged injection of the Sp-SIFa peptide into mud crabs at the early vitellogenic stage. The crabs were randomly divided into three groups, with five individuals in each group (n = 5). Before the injection, a group of crabs was sampled as a pre-injection control. Mud crabs were injected with Sp-SIFa peptide (15 ng/g body mass) prepared in 100 μL crab saline once every five days, while the control group received 100 μL crab saline instead. On the 16th day, approximately 24 h after the third injection, crabs were put on ice, and samples of ovary and hepatopancreas were collected for gene expression analysis. Moreover, the histological changes of the ovary in response to prolonged injection of Sp-SIFa were determined by hematoxylin and eosin staining and the index of gonadal development (GSI) was measured and calculated following a standard function, as follows: GSI = (gonad weight/body weight) * 100%.
2.6 qPCR assay
The qPCR was performed on a QuantStudio 5 Real-time PCR machine (Applied Biosystems) using PowerUp™ SYBR™ Green Master Mix (Thermo Fisher Scientific) in a total reaction volume of 20 μL. The reaction mixture consisted of PowerUp™ SYBR™ Green Master Mix (10 μL), 10-fold diluted cDNA (2 μL), forward and reverse primers (1 μL each), and deionized water (6 μL). The reaction conditions were as follows: 95°C for 3 min, followed by 40 cycles of 95°C for 15 s, 60°C for 30 s, and 72°C for 30 s, and a subsequent melting curve analysis spanning 60 - 95°C.
2.7 Statistical analyses
The qPCR data were calculated using the 2-ΔΔCt method and presented as mean ± standard error of the mean (SEM). Statistical differences were analyzed using a one-way analysis of variance followed by Duncan’s test or Student’s t-test, using the Statistical Package for the Social Sciences software (version 26.0). Differences were considered statistically significant when p < 0.05.
3 Results
3.1 Cloning and sequence analysis of Sp-SIFa and its putative receptors
The ORF of Sp-SIFa (GenBank accession number: PQ519603) was 237 bp in length, encoding a 78 aa precursor (Figure 1A). The precursor contained a 27 aa signal peptide, a 12 aa mature peptide (GYRKPPFNGSIFamide), a dibasic cleavage site, and a related peptide (Figure 1B). Sequence alignment reveled that Sp-SIFa was highly homologous to SIFa in other crustaceans and insects (Figure 2).
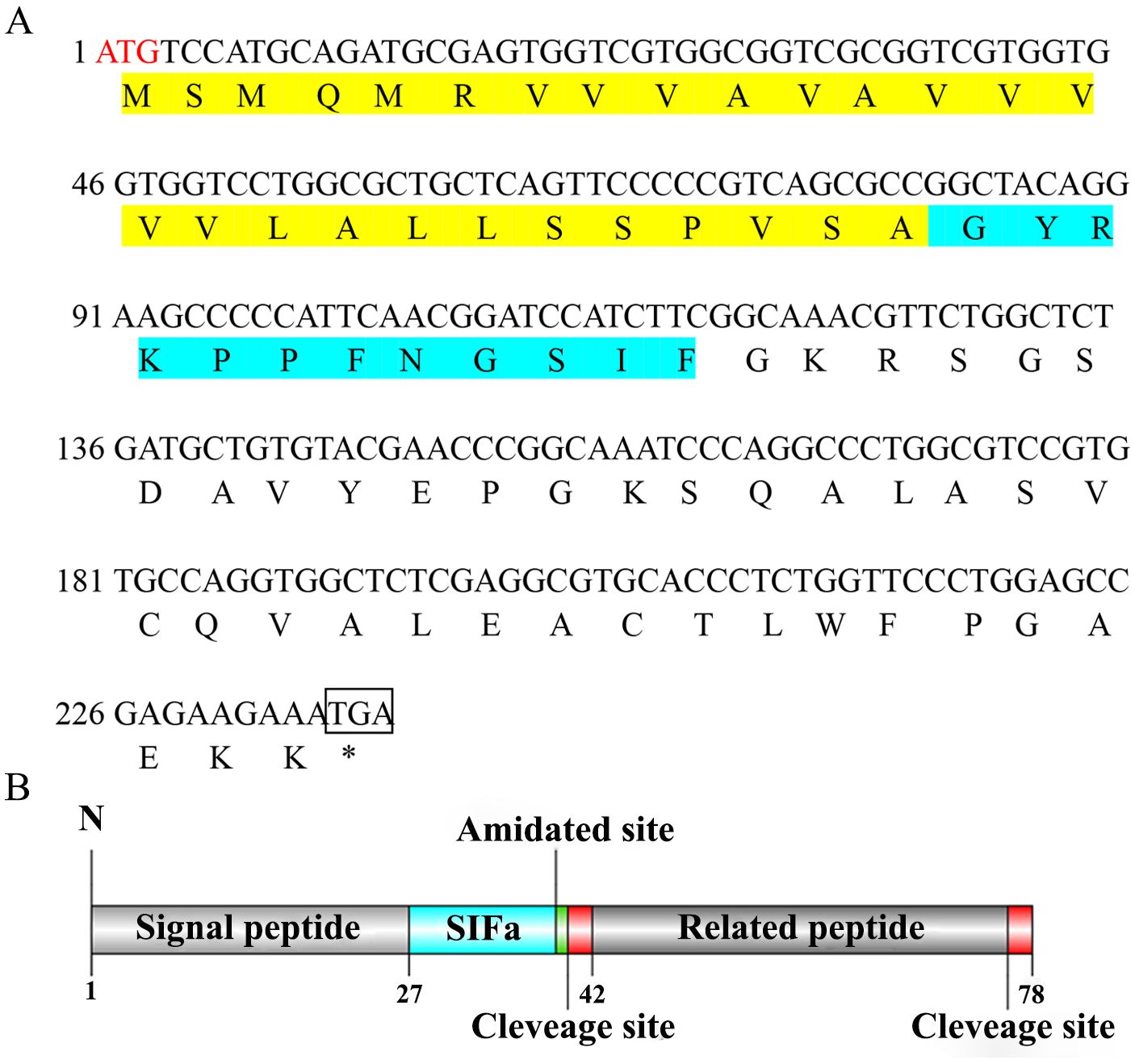
Figure 1. (A) Nucleotide and deduced aa sequence of Sp-SIFa. The ORF is indicated by a single-letter code below the nucleotide sequence. The signal and mature peptides are highlighted in yellow and green, respectively, and the initiation codon, amidated, and dibasic cleavage sites are indicated in red, gray, and blue, respectively. (B) Schematic representation of structural domains of Sp-SIFa.
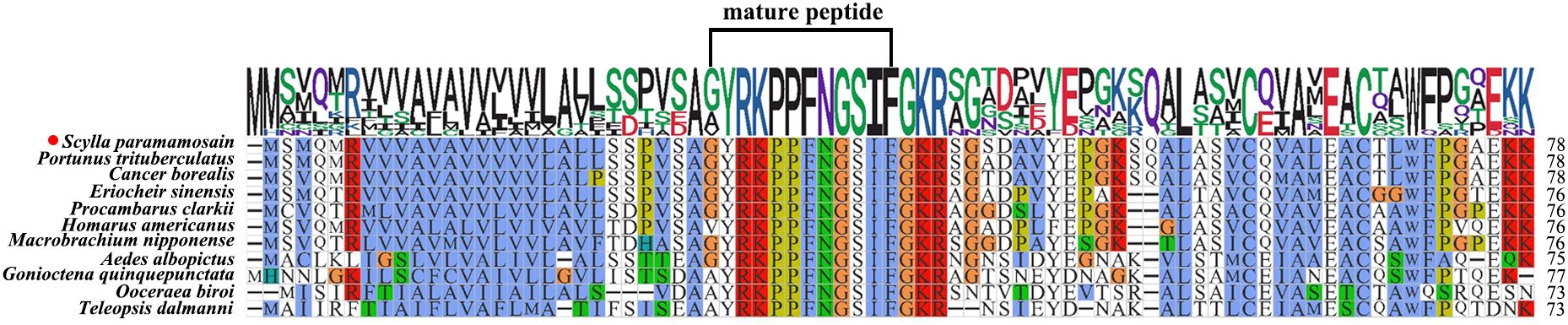
Figure 2. Multiple sequence alignment of Sp-SIFa and its homologs. Portunus trituberculatus (GenBank: XP_045132111.1), Cancer borealis (GenBank: ADO00265.1), Eriocheir sinensis (GenBank: XP_050718719.1), Procambarus clarkii (GenBank: XP_045586683.1), Homarus americanus (GenBank: KAG7158656.1), Macrobrachium nipponense (GenBank: XP_064098545.1), Aedes albopictus (GenBank: XP_019562497.1), Gonioctena quinquepunctata (GenBank: KAG5890203.1), Ooceraea biroi (GenBank: XP_011342125.1), and Teleopsis dalmanni (GenBank: _037954381.1). The aa number is indicated on the right.
In addition, two putative receptors for SIFa were screened from the transcriptome dataset, and further identified from the ovary of S. paramamosian in this study by PCR mothed. They differ in sequence length, Sp-SIFaR1 (GenBank accession number: PQ519601) was 1563 bp in length, which encodes a 520 aa protein, while Sp-SIFaR2 (GenBank accession number: PQ519602) was 1587 bp in length, encoding a 528 aa protein. The sequence analysis indicated that these two receptors possess seven conserved transmembrane structural domains, exhibiting a high similarity to the SIFaRs reported in other crustaceans and insects (Figure 3). However, Sp-SIFaR1 and Sp-SIFaR2 have low sequence similarity between them. The phylogenetic tree demonstrated that Sp-SIFaR1 and Sp-SIFaR2 were categorized into different branches. Sp-SIFaR1 was clustered with SIFaRs in N. lugens, Zootermopsis nevadensis, P. indicus, and Frankliniella occidentalis. Sp-SIFaR2 fit into a clade with SIFaRs in P. trituberculatus, Folsomia candida, Teleopsis dalmanni, and Nasonia vitripennis (Figure 4).
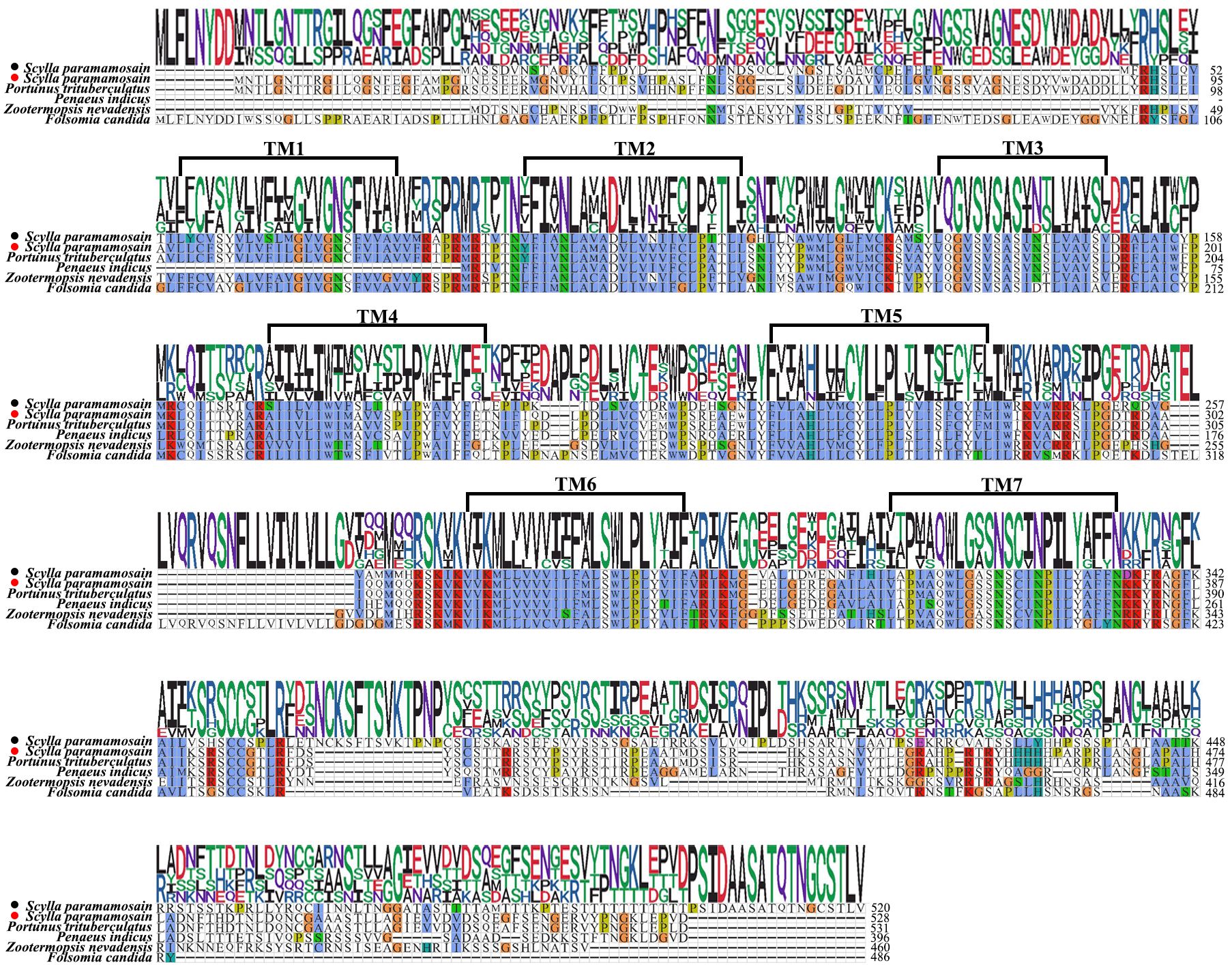
Figure 3. Multiple sequence alignments of Sp-SIFaR 1/SIFaR 2 and their homologs. Portunus trituberculatus (GenBank: XP_045125313.1), Penaeus indicus (GenBank: XP_063588959.1), Zootermopsis nevadensis (GenBank: XP_021917391.1), and Folsomia candida (GenBank: OXA43778.1). The aa number is indicated on the right. The solid black circle represents the Sp-SIFaR1, and the red circle represents the Sp-SIFaR2.
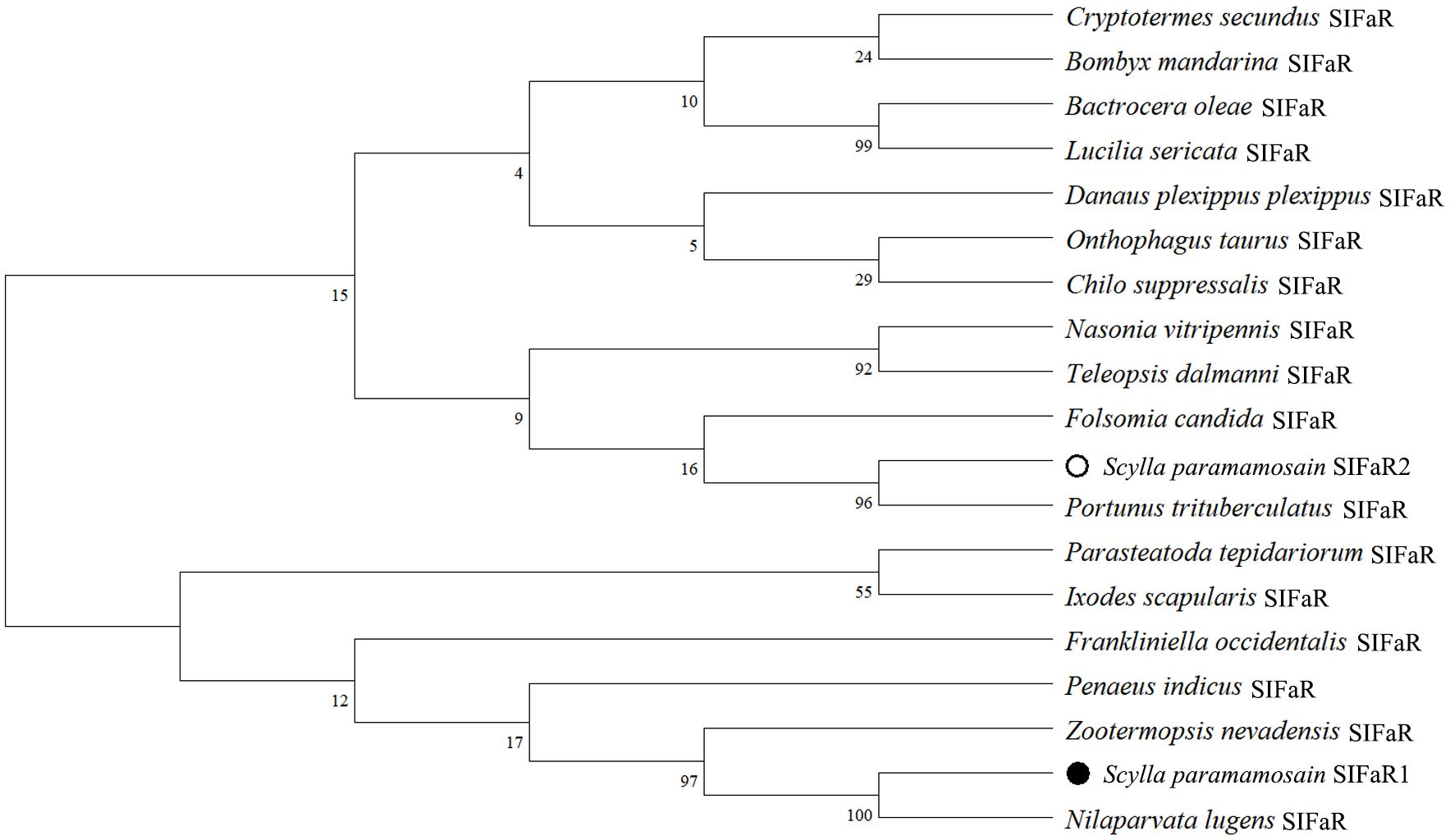
Figure 4. Phylogenetic analysis of SIFaR in crustaceans and insects. Danaus plexippus (GenBank: XP_032516043.1), Chilo suppressalis (GenBank: ALM88339.1), Bombyx mandarina (GenBank: XP_028044101.1), Nasonia vitripennis (GenBank: XP_0016000098.3), Onthophagus taurus (GenBank: XP_022919670.1), Cryptotermes secundus (GenBank: XP_023712423.2), Nilaparvata lugens (GenBank: BAO01053.1), Lucilia sericata (GenBank: XP_037808011.1), Bactrocera oleae (GenBank: XP_014089790.2), Teleopsis dalmanni (GenBank: XP_037955898.1), Parasteatoda tepidariorum (GenBank: XP_015924497.2), Ixodes scapularis (GenBank: XP_002406273.3), Folsomia candida (GenBank: OXA43778.1), Zootermopsis nevadensis (GenBank: XP_021917391.1) and Frankliniella occidentalis (GenBank: XP_052132360.1), Portunus trituberculatus (GenBank: XP_045125313.1), Penaeus indicus (GenBank: XP_063588959.1). The solid circle represents the Sp-SIFaR1, and the hollow circle represents the Sp-SIFaR2.
3.2 Expression profiles of Sp-SIFa and its putative receptors in female S. paramamosain
RT-PCR was conducted to determine the tissue expression of Sp-SIFa and its receptors in female S. paramamosain. Sp-SIFa exhibited high expression levels in the cerebral ganglion, followed by the eyestalk ganglion, middle gut, and thoracic ganglion. Additionally, Sp-SIFaR1 was highly expressed in the cerebral ganglion and ovary and moderately expressed in the eyestalk ganglion and hepatopancreas. However, the Sp-SIFaR2 expression was confined to the ovary and eyestalk ganglion (Figure 5A).
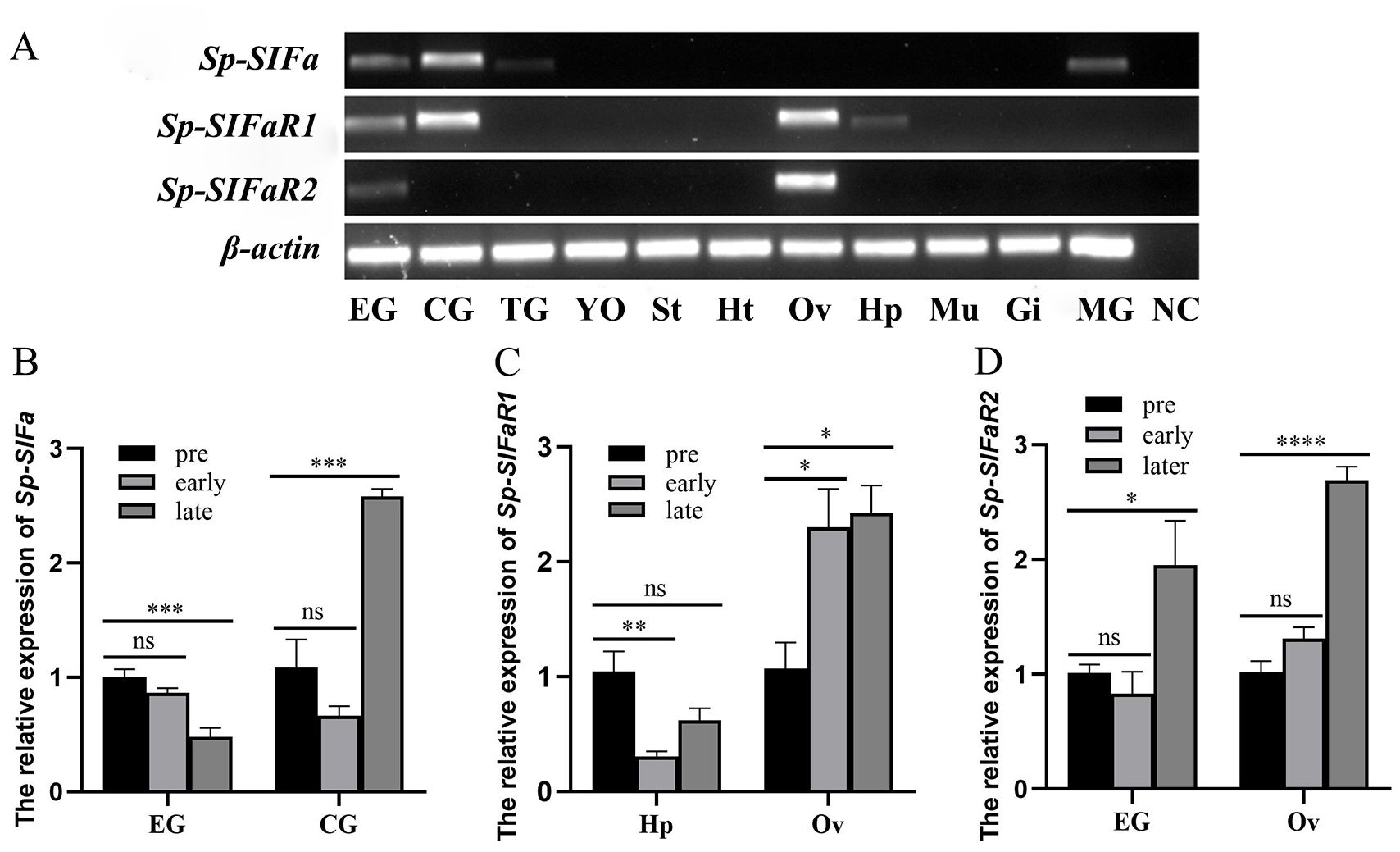
Figure 5. Expression profiles of Sp-SIFa and its putative receptors in female S. paramamosain. (A) Tissue distribution of Sp-SIFa and its putative receptors. β-actin was included as an internal reference. EG, eyestalk ganglion; CG, cerebral ganglion; TG, thoracic ganglion; YO, Y-organ; St, stomach; Ht, heart; Ov, ovary; Hp, hepatopancreas; Mu, muscle; Gi, gill; MG, middle gut; NC, negative control (amplification of deionized water). (B-D) The expression pattern of Sp-SIFa in the eyestalk ganglion and cerebral ganglion, Sp-SIFaR1 in the hepatopancreas and ovary, and Sp-SIFaR2 in the eyestalk ganglion and ovary during vitellogenesis. Pre: pre-vitellogenic stage; early: early vitellogenic stage; late: late vitellogenic stage. The data are presented as mean ± SEM (n = 4). Asterisks (*P < 0.05, **P < 0.01, ***P < 0.001, ****P < 0.0001) indicate significant differences from the pre-vitellogenic stage. ns indicates no significant difference from the pre-vitellogenic stage.
The expression profiles of Sp-SIFa and its two receptors at three different vitellogenic stages were determined using qPCR. Sp-SIFa was highly expressed in the eyestalk ganglion at the pre-vitellogenic stage, and its expression was significantly down-regulated at the late stage of vitellogenesis (P < 0.001; Figure 5B). However, an opposite expression profile was observed in the cerebral ganglion, which revealed that the level of Sp-SIFa transcript was low at the pre-vitellogenic stage and subsequently increased at the late stage of vitellogenesis (P < 0.001; Figure 5B). Sp-SIFaR1 was highly expressed in the pre-vitellogenic hepatopancreas, and its expression significantly decreased in the early vitellogenic stage (P < 0.01; Figure 5C). In contrast, its expression level in the ovary was low at the pre-vitellogenic stage, significantly increased at the early vitellogenic stage (P < 0.05), and subsequently reached the highest level at the late vitellogenic stage (Figure 5C). Additionally, the level of Sp-SIFaR2 transcript in the eyestalk ganglion and ovary was low at the pre-and early vitellogenic stages but increased significantly in the late stage of vitellogenesis (P < 0.05 in the eyestalk ganglion; P < 0.0001 in the ovary; Figure 5D).
3.3 In vitro effects of Sp-SIFa peptide on Sp-Vg and Sp-VgR expression
To elucidate the potential role of Sp-SIFa in vitellogenesis, synthetic Sp-SIFa was applied to the hepatopancreatic and ovarian explants. Upon stimulation with Sp-SIFa, dynamic changes in the levels of Sp-Vg and Sp-VgR transcripts were detected using qPCR. The expression of Sp-Vg in the hepatopancreatic explants was significantly induced after 4 h of incubation with 10 nM Sp-SIFa peptide (P < 0.01; Figure 6B). The Sp-VgR transcript levels in the ovarian explants increased significantly when stimulated by Sp-SIFa peptide at 1 nM and 10 nM concentrations (Figure 6D) for 2 h (P < 0.001) and after treatment with Sp-SIFa for 4 h at concentrations of 10 nM (P < 0.001) and 100 nM (P < 0.01; Figure 6E).
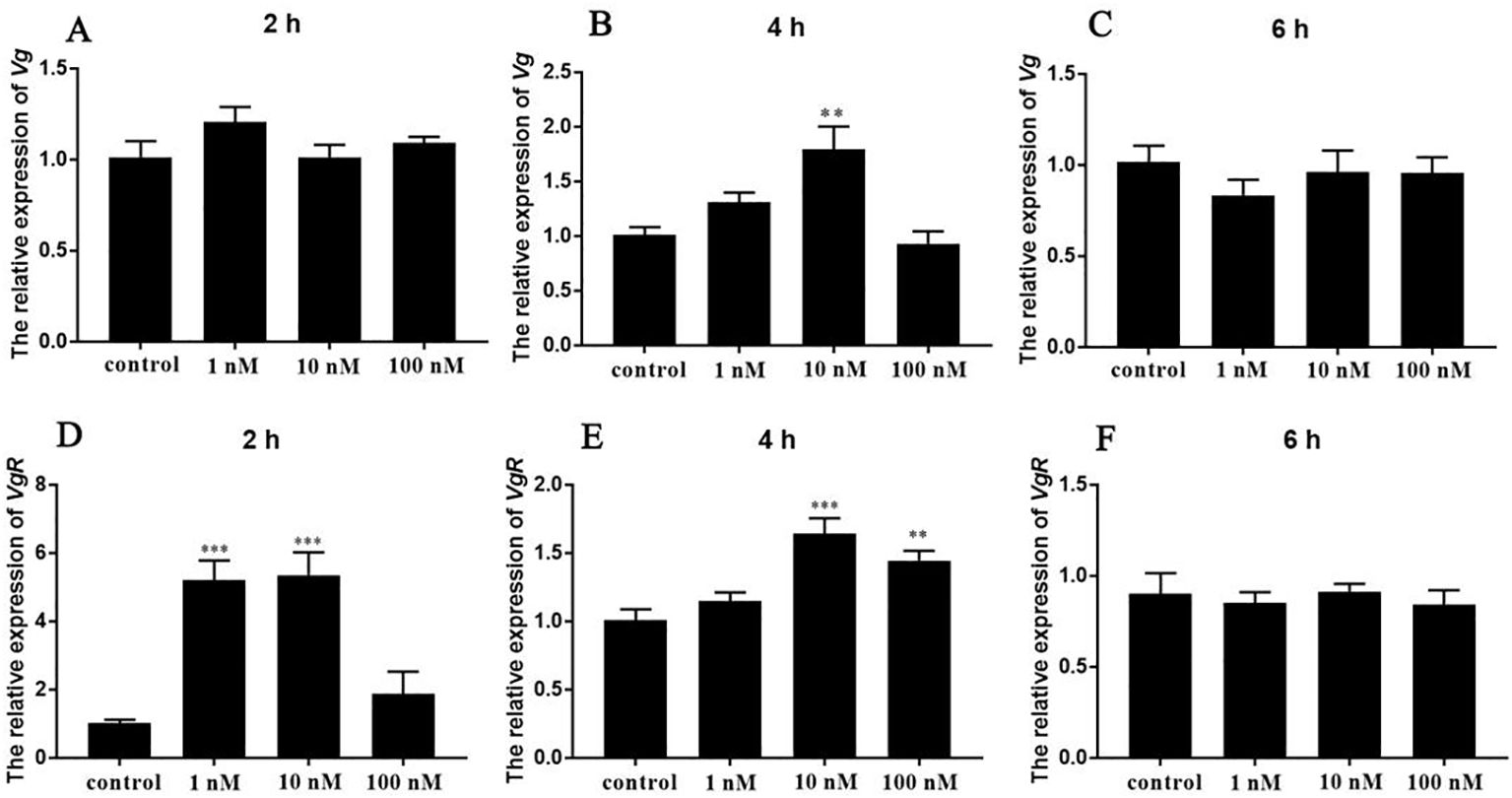
Figure 6. In vitro effects of synthetic Sp-SIFa peptide on the expression of Sp-Vg and Sp-VgR. (A-C) Relative expression of Sp-Vg in the hepatopancreas in response to the Sp-SIFa peptide. (D-F) Relative expression of Sp-VgR in the ovary in response to the Sp-SIFa peptide. Data are presented as mean ± SEM (n = 5). Asterisks (**P < 0.01; ***P < 0.001) indicate significant differences from the control.
3.4 In vivo effects of Sp-SIFa peptide on ovarian development
Experiments by injection of Sp-SIFa peptide into mud crab at stage III were performed to further confirm its role in ovarian development. The results of the short-term experiment revealed that the expression of Sp-Vg in the hepatopancreas and Sp-VgR in the ovary was significantly induced by a 12-h injection of the Sp-SIFa peptide (P < 0.05; Figure 7).
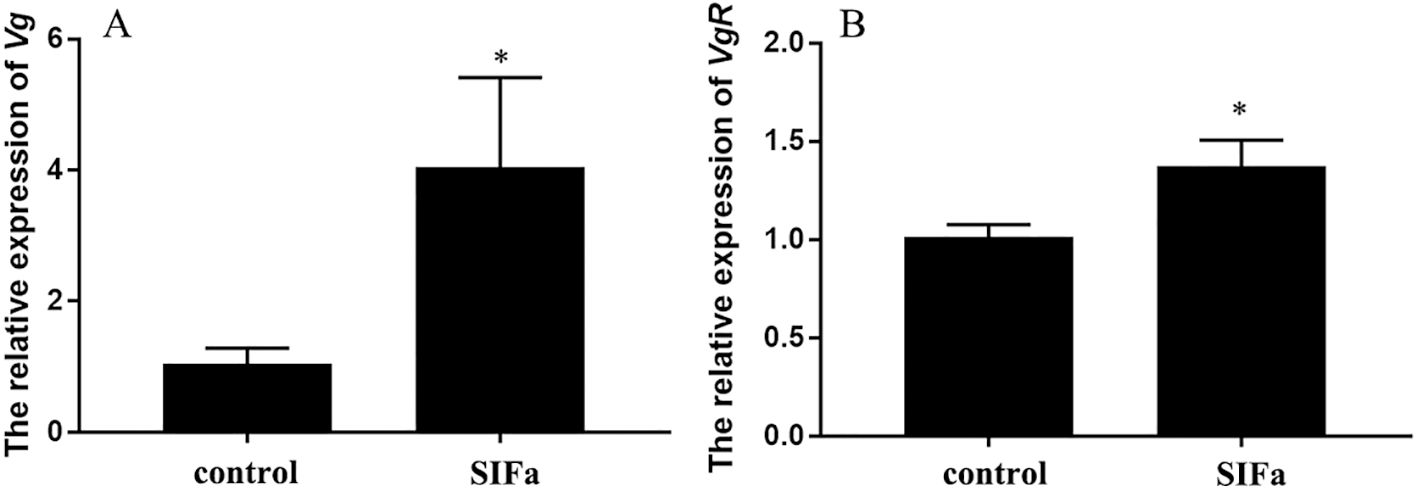
Figure 7. Relative expression of Sp-Vg (A) in the hepatopancreas and Sp-VgR (B) in the ovary after 12 h injection of Sp-SIFa peptide. Data are presented as mean ± SEM (n = 5). Asterisks (*P < 0.05) on the error bar indicate significant differences from the control.
In the long-term experiments, prolonged injection of Sp-SIFa significantly induced the expression of Sp-VgR (P < 0.05) (Figure 8B) and increased the GSI (P < 0.05) of S. paramamosain (Figure 8C). However, the level of Sp-Vg expression in hepatopancreas did not change significantly at the sampling point (Figure 8A). Concurrently, histologic analysis further showed that oocyte diameter and the number of yolk granules were significantly increased (P < 0.001) (Figure 9). These results indicate that Sp-SIFa may promote ovarian development in mud crabs by facilitating oocyte uptake of Vg and oocyte growth.
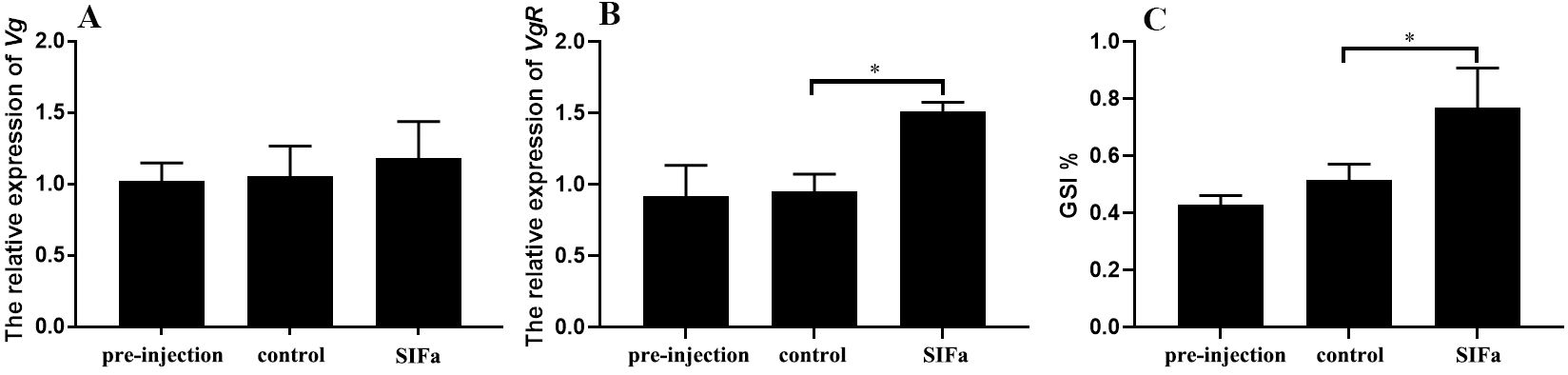
Figure 8. Relative expression of Sp-Vg (A) in the hepatopancreas, Sp-VgR (B) in the ovary, and GSI % (C) after 15 days of administration of Sp-SIFa peptide. Data are presented as mean ± SEM (n = 5). Asterisks (*P < 0.05) on the error bar indicate significant differences from the control.
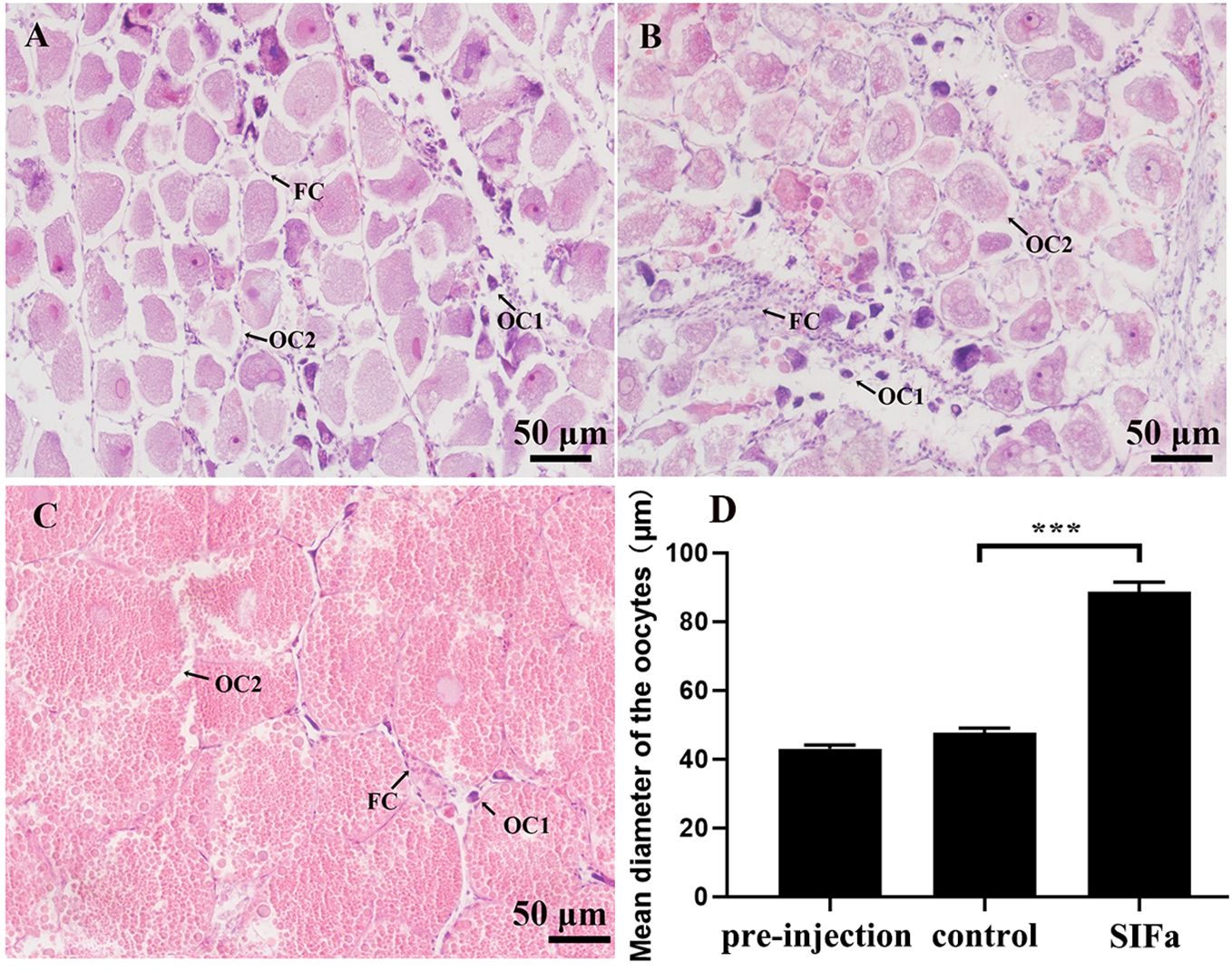
Figure 9. Histological changes in S. paramamosain ovary at the early vitellogenic stage in response to administration of synthetic Sp-SIFa peptide. (A) Pre-injection control. (B) Crab saline treatment (saline control). (C) Sp-SIFa peptide treatment. OC1, pre-vitellogenic oocyte; OC2, early vitellogenic oocyte; FC, follicular cell. Scale bars: 50 μm. (D) Mean oocyte diameter. Asterisk ( *** P < 0.001) indicates significant differences from the control.
4 Discussion
SIFa is extensively present in the central nervous systems of arthropods, and its conservation across various species suggests its potential significance (Verleyen et al., 2009). Leveraging advancements in high-throughput sequencing technology, SIFa has been widely recognized in several crustaceans (Sithigorngul et al., 2002; Dickinson et al., 2008; Huybrechts et al., 2003; Yasuda et al., 2004; Ma et al., 2009); however, its physiological functions remain largely unexplored. This study characterized SIFa and its two putative receptors and described their expression profiles in the mud crab S. paramamosain, illustrating a potential stimulatory role in ovarian development.
In the mud crab S. paramamosain, sequences encoding an Sp-SIFa precursor and its two potential SIFa receptors were identified. The Sp-SIFa precursor exhibited a characteristic similarity with the documented insect SIFa, comprising a signal peptide, mature peptide, and precursor-related peptide (Ayub et al., 2020). There was an amidated signal at the C-terminus of the mature peptide (Figure 1), suggesting it was an amidated hormone. Sequence alignment revealed that the crustacean SIFa precursor exhibited high sequence similarity to that in insects, suggesting that SIFa is highly conserved among arthropods (Figure 2). Previous research has demonstrated that SIFaR is structurally related to the GnIHR; however, there is low similarity between their ligands SIFa and GnIH (Ubuka and Tsutsui, 2014; Elphick and Mirabeau, 2014). It has been proposed that GnIH/GnIHR and SIFa/SIFaR may be distinct from the ancestral Famide peptide system, which is a family of peptides that have independently co-evolved in vertebrates and insects, including FMRFamide, neuropeptide F, short neuropeptide F, sulfakin, myosuppressin and SIFa (Ubuka and Tsutsui, 2014). G protein-coupled receptors (GPCRs) are the largest family of receptors characterized by seven α-helical transmembrane structural domains, which are involved in regulating a wide variety of physiological processes (Horn et al., 1998; Pierce et al., 2002). The GPCRs are classified into six major classes, and SIFaR belongs to class A, Rhodopsin-like receptors (Šimo et al., 2013). Sequence alignment revealed that SIFa receptors from crustaceans and insects exhibited relatively high conservation in the seven transmembrane structural domains (Figure 3). In S. paramamosain, the two SIFaRs (Sp-SIFaR1 and Sp-SIFaR2) exhibited low sequence identity (less than 32%), implying that they may be involved in a distinct physiological process.
In insects, SIFa is mainly expressed in the nervous system (Ayub et al., 2020). In crustaceans, including H. americanus and P. clarkii, SIFa is predominantly expressed in the stomatogastric nervous system and the middle gut (Dickinson et al., 2008). Similarly, the Sp-SIFa transcript is confined to nervous tissues (eyestalk, cerebral, and thoracic ganglion) and the middle gut of S. paramamosain (Figure 5A), suggesting that SIFa functions as a brain-gut hormone in most crustaceans. SIFa is present in the nerve fibers of the olfactory lobes of certain insect species, suggesting a possible neuromodulatory role for SIFa in the olfactory system (Verleyen et al., 2004). In Drosophila, SIFa is expressed in only four medial neurons of the brain (Terhzaz et al., 2007), which can inhibit sexual behavior (Dickson, 2008), promote sleep (Park et al., 2014), and induce appetitive and feeding behaviors (Martelli et al., 2017). In R. prolixus, SIFa is released by the interneurons and dorsal vessel, where it also has a role in facilitating ingestion and inducing heart rate in a dose-dependent manner (Ayub et al., 2020). Similarly, SIFa functions as a cardioactive peptide in C. borealis and C. irroratus to promote cardiac output, however, this regulation differs from that of R. prolixus. SIFa appears to be synthesized in the middle gut and delivered to the heart to regulate C. borealis and C. irroratus (Dickinson et al., 2019). In this study, Sp-SIFa was highly expressed in the cerebral ganglion, implying that it may be involved in similar physiological activities, including reproduction, sleeping, and feeding, which was further supported by the wide expression of Sp-SIFaR1 (Figure 5A). Sp-SIFaR1 was extensively expressed in the eyestalk ganglion, cerebral ganglion, ovary, and hepatopancreas, whereas the Sp-SIFaR2 expression was restricted to the eyestalk ganglion and ovary (Figure 5A). This outcome is broadly comparable to the tissue distribution of SIFaR in insects (Sellami and Veenstra, 2015; Lismont et al., 2018; Veenstra, 2021). Moreover, the Sp-SIFa expression in the eyestalk and cerebral ganglia was significantly altered during vitellogenesis, implying its possible role in ovarian development (Figure 5B). The presence of two Sp-SIFa receptors in the ovary of S. paramamosain further supports their significance in regulating ovarian development. Sp-SIFaR1 levels was significantly elevated in the early and late vitellogenic stages (Figure 5C), while Sp-SIFaR2 was significantly increased at the late vitellogenic stage (Figure 5D). These results indicated that Sp-SIFaR1 and Sp-SIFaR2 may involve in different regulation during ovarian development, which warrants future study. Two receptors for SIFa in I. scapularis collaborate to regulate salivary secretion (Guerrib et al., 2023).
Vitellogenesis, the process by which Vg synthesized by the hepatopancreas and ovary is endocytosed into the oocyte mediated by the Vg receptor, is essential for ovarian development in crustaceans (Subramoniam, 2010). Although vitellogenesis in crustaceans has been extensively studied, the mechanisms underlying its regulation remain incompletely comprehended. Multifunctional neuropeptides, neurotransmitters, neuromodulations, and other factors also regulate ovarian development in crustaceans (Subramoniam, 2010). VIH, crustacean hyperglycaemic hormone (CHH), and molt-inhibiting hormone (MIH) are eyestalk peptides of the CHH family. VIH inhibits the Vg production in the hepatopancreas and the Vg uptake by oocytes during vitellogenesis (Chen et al., 2014; Qiao et al., 2015). In contrast, CHH and MIH may act as positive regulators of vitellogenesis and ovarian development (de Kleijn et al., 1998; Luo et al., 2015). 5-HT is a neurotransmitter that significantly contributes to ovarian development by modulating downstream endocrine factors, hormones, and reproduction-associated proteins (Jayasankar et al., 2020). The present study revealed the role of Sp-SIFa in the vitellogenesis of mud crabs by detecting the effects of Sp-SIFa on the expression of Sp-Vg and Sp-VgR. The in vitro results demonstrated that Sp-SIFa significantly induced Sp-Vg expression in the hepatopancreas and Sp-VgR expression in the ovary (Figure 6). Short-term injection of SIFa into female crabs consolidated its stimulatory effects on vitellogenesis by promoting Sp-Vg and Sp-VgR expression (Figure 7). A similar effect was observed with the addition of hEGF protein, which can induce Sp-VgR expression (Lu et al., 2020). In the long-term experiment, the Sp-Vg level did not change significantly (Figure 8), which may have been caused by the sampling point. However, the level of Sp-VgR (Figure 8), GSI, and oocyte diameter (Figure 9) were significantly increased by the prolonged injection of Sp-SIFa, suggesting a stimulatory role in ovarian development.
In conclusion, this study isolated Sp-SIFa and its two putative receptors from mud crabs. It delineated their expression patterns in females, offering insight into their physiological activities. This study further confirmed the stimulatory role of Sp-SIFa in ovarian development by promoting Vg biosynthesis and oocyte uptake.
Data availability statement
The datasets presented in this study can be found in online repositories. The names of the repository/repositories and accession number(s) can be found in the article/supplementary material.
Ethics statement
The animal studies were approved by Animal Care and Use Committee of the Fisheries College of Jimei University (Approval Code: 2021-04; Approval Date: January 22, 2021). The studies were conducted in accordance with the local legislation and institutional requirements. Written informed consent was obtained from the owners for the participation of their animals in this study.
Author contributions
SC: Formal analysis, Investigation, Writing – original draft. YT: Formal analysis, Investigation, Writing – original draft. LL: Formal analysis, Investigation, Writing – original draft. SG: Investigation, Writing – original draft. AL: Conceptualization, Funding acquisition, Project administration, Supervision, Validation, Writing – original draft, Writing – review & editing. HY: Conceptualization, Funding acquisition, Writing – review & editing.
Funding
The author(s) declare that financial support was received for the research, authorship, and/or publication of this article. This study was supported by the National Natural Science Foundation of China (grant numbers: 32102787), and the Natural Science Foundation of Fujian Province (grant numbers: 2021J05160).
Acknowledgments
We sincerely thank the members of the laboratory for their invaluable input and eagerness to help during the experimental and writing processes.
Conflict of interest
The authors declare that the research was conducted in the absence of any commercial or financial relationships that could be construed as a potential conflict of interest.
Publisher’s note
All claims expressed in this article are solely those of the authors and do not necessarily represent those of their affiliated organizations, or those of the publisher, the editors and the reviewers. Any product that may be evaluated in this article, or claim that may be made by its manufacturer, is not guaranteed or endorsed by the publisher.
References
Arendt A., Neupert S., Schendzielorz J., Predel R., Stengl M. (2015). The neuropeptide SIFamide in the brain of three cockroach species. J. Comp. Neurol. 524, 1337–1360. doi: 10.1002/cne.23910
Ayub M., Hermiz M., Lange A. B., Orchard I. (2020). SIFamide influences feeding in the Chagas disease vector, Rhodnius prolixus. Front. Neurosci. 14. doi: 10.3389/fnins.2020.00134
Bao C. C., Yang Y. N., Huang H. Y., Ye H. H. (2015). Neuropeptides in the cerebral ganglia of the mud crab, Scylla paramamosain: transcriptomic analysis and expression profiles during vitellogenesis. Sci. Rep. 5, 17055. doi: 10.1038/srep17055
Charmantier G., Charmantier-Daures M., Van Herp F. (1997). “Hormonal regulation of growth and reproduction in crustaceans,” in Recent Advances in Marine Biotechnology, vol. I . Eds. Fingerman M., Nagabhushanan R., Thompson M. F. (Science Publishers, New York), 109–161.
Chen T., Zhang L. P., Wong N. K., Zhong M., Ren C. H., Hu C. Q. (2014). Pacific white shrimp (Litopenaeus vannamei) vitellogenesis-inhibiting hormone (VIH) is predominantly expressed in the brain and negatively regulates hepatopancreatic vitellogenin (VTG) gene expression. Biol. Reprod. 47, 1–10. doi: 10.1095/biolreprod.113.115030
de Kleijn D. P., Janssen K. P., Waddy S. L., Hegeman R., Lai W. Y., Martens G. J., et al. (1998). Expression of the crustacean hyperglycaemic hormones and the gonad-inhibiting hormone during the reproductive cycle of the female American lobster Homarus americanus. J. Endocrinol. 156, 291–298. doi: 10.1677/joe.0.1560291
Dickinson P. S., Samuel H. M., Stemmler E. A., Christie A. E. (2019). SIFamide peptides modulate cardiac activity differently in two species of Cancer crab. Gen. Comp. Endocrinol. 282, 113204. doi: 10.1016/j.ygcen.2019.06.008
Dickinson P. S., Stemmler E. A., Cashman C. R., Brenan H. R., Dennison B., Huber K. E., et al. (2008). SIFamide peptides in clawed lobsters and freshwater crayfish (Crustacea, Decapoda, Astacidea): A combined molecular, mass spectrometric and electrophysiological investigation. Gen. Comp. Endocrinol. 156, 347–360. doi: 10.1016/j.ygcen.2008.01.011
Dickson B. (2008). Wired for sex: The neurobiology of Drosophila mating decisions. Science 322, 904–909. doi: 10.1126/science.1159276
Elphick M. R., Mirabeau O. (2014). The evolution and variety of RFamide-type neuropeptides: Insights from deuterostomian invertebrates. Front. Endocrinol. 5. doi: 10.3389/fendo.2014.00093
Fan Y., Sun P., Wang Y., He X. B., Deng X. Y., Chen X. P., et al. (2010). The G protein-coupled receptors in the silkworm, Bombyx mori. Insect Biochem. Mol. Biol. 40, 581–591. doi: 10.1016/j.ibmb.2010.05.005
Guerrib F., Ning C., Mateos-Hernandez L., Rakotobe S., Park Y., Hajdusek O., et al. (2023). Dual SIFamide receptors in Ixodes salivary glands. Insect Biochem. Mol. Biol. 158, 103963. doi: 10.1016/j.ibmb.2023.103963
Hauser F., Cazzamali G., Williamson M., Park Y., Li B., Tanaka Y., et al. (2008). A genome-wide inventory of neurohormone GPCRs in the red flour beetle Tribolium castaneum. Front. Neuroendocrinol. 29, 142–165. doi: 10.1016/j.yfrne.2007.10.003
Horn F., Weare J., Beukers M. W., Hörsch S., Bairoch A., Chen W., et al. (1998). GPCRDB: an information system for G protein coupled receptors. Nucleic Acids Res. 26, 275–279. doi: 10.1093/nar/26.1.275
Huang H. Y., Possidente D. R., Vecsey C. G. (2021). Optogenetic activation of SIFamide (SIFa) neurons induces a complex sleep-promoting effect in the fruit fly Drosophila melanogaster. Physiol. Behav. 239, 113507. doi: 10.1016/j.physbeh.2021.113507
Huang X. S., Ye H. H., Huang H. Y., Yang Y. N., Gong J. (2014). An insulin-like androgenic gland hormone gene in the mud crab, Scylla paramamosain, extensively expressed and involved in the processes of growth and female reproduction. Gen. Comp. Endocrinol. 204, 229–238. doi: 10.1016/j.ygcen.2014.06.002
Huybrechts J., Nusbaum M. P., Bosch L. V., Baggerman G., Loof A. D., Schoofs L. (2003). Neuropeptidomic analysis of the brain and thoracic ganglion from the Jonah crab, Cancer borealis. Biochem. Biophys. Res. Commun. 308, 535–544. doi: 10.1016/S0006-291X(03)01426-8
Janssen I., Schoofs L., Spittaels K., Neven H., Broeck J., Vanden, et al. (19961996). Isolation of NEB-LFamide, a novel myotropic neuropeptide from the grey flesh fly. Mol. Cell. Endocrinol. 117, 157–165. doi: 10.1016/0303-7207(95)03746-2
Jayasankar V., Tomy S., Wilder M. N. (2020). Insights on molecular mechanisms of ovarian development in decapod crustacea: focus on vitellogenesis-stimulating factors and pathways. Front. Endocrinol. 11. doi: 10.3389/fendo.2020.577925
Jékely G. (2013). Global view of the evolution and diversity of metazoan neuropeptide signaling. Proc. Natl. Acad. Sci. 110, 8702–8707. doi: 10.1073/pnas.1221833110
Jørgensen L. M., Hauser F., Cazzamali G., Williamson M., Grimmelikhuijzen C. J. P. (2006). Molecular identification of the first SIFamide receptor. Biochem. Biophys. Res. Commun. 340, 696–701. doi: 10.1016/j.bbrc.2005.12.062
Lismont E., Mortelmans N., Verlinden H., Vanden Broeck J. (2018). Molecular cloning and characterization of the SIFamide precursor and receptor in a hymenopteran insect, Bombus terrestris. Gen. Comp. Endocrinol. 258, 39–52. doi: 10.1016/j.ygcen.2017.10.014
Lu B., Jiang Q. L., Liu A., Huang H. Y., Ye H. H. (2020). Stimulatory roles of epidermal growth factor receptor (EGFR) in ovarian development of mud crab Scylla paramamosain. Gen. Comp. Endocrinol. 299, 113616. doi: 10.1016/j.ygcen.2020.113616
Luo X., Chen T., Zhong M., Jiang X., Zhang L. P., Ren C. H., et al. (2015). Differential regulation of hepatopancreatic vitellogenin (VTG) gene expression by two putative molt-inhibiting hormones (MIH1/2) in Pacific white shrimp (Litopenaeus vannamei). Peptides 68, 58–63. doi: 10.1016/j.peptides.2014.11.002
Ma M. M., Bors E. K., Dickinson E. S., Kwiatkowski M. A., Sousa G. L., Henry R. P., et al. (2009). Characterization of the Carcinus maenas neuropeptidome by mass spectrometry and functional genomics. Gen. Comp. Endocrinol. 161, 320–334. doi: 10.1016/j.ygcen.2009.01.015
Martelli C., Pech U., Kobbenbring S., Pauls D., Bahl B., Sommer M. V., et al. (2017). SIFamide translates hunger signals into appetitive and feeding behavior in Drosophila. Cell Rep. 20, 464–478. doi: 10.1016/j.celrep.2017.06.043
Park S. J., Sonn J. Y., Oh Y. K., Lim C. H., Choe J. H. (2014). SIFamide and SIFamide receptor define a novel neuropeptide nignaling to promote sleep in Drosophila. Molecules Cells 37, 295–301. doi: 10.14348/molcells.2014.2371
Pierce K. L., Premont R. T., Lefkowitz R. J. (2002). Signalling: seven-transmembrane receptors. Nat. Rev. Mol. Cell Biol. 3, 639–650. doi: 10.1038/nrm908
Qiao H., Xiong Y. W., Zhang W. Y., Fu H. T., Jiang S. F., Sun S. M., et al. (2015). Characterization, expression, and function analysis of gonad-inhibiting hormone in Oriental River prawn, Macrobrachium nipponense and its induced expression by temperature. Comp. Biochem. Physiol. A: Mol. Integr. Physiol. 185, 1–8. doi: 10.1016/j.cbpa.2015.03.005
Sellami A., Veenstra J. A. (2015). SIFamide acts on fruitless neurons to modulate sexual behavior in Drosophila melanogaster. Peptides 74, 50–56. doi: 10.1016/j.peptides.2015.10.003
Šimo L., Koči J., Park Y. (2013). Receptors for the neuropeptides, myoinhibitory peptide and SIFamide, in control of the salivary glands of the blacklegged tick Ixodes scapularis. Insect Biochem. Mol. Biol. 43, 376–387. doi: 10.1016/j.ibmb.2013.01.002
Sithigorngul P., Pupuem J., Krungkasem C., Longyant S., Chaivisuthangkura P., Sithigorngul W., et al. (2002). Seven novel FMRFamide-like neuropeptide sequences from the eyestalk of the giant tiger prawn Penaeus monodon. Comp. Biochem. Physiol. Part B: Biochem. Mol. Biol. 131, 325–337. doi: 10.1016/S1096-4959(01)00499-7
Subramoniam T. (2010). Mechanisms and control of vitellogenesis in crustaceans. Fisheries Sci. 77, 1–21. doi: 10.1007/s12562-010-0301-z
Tanaka Y., Suetsugu Y., Yamamoto K., Noda H., Shinoda T. (2014). Transcriptome analysis of neuropeptides and G-protein coupled receptors (GPCRs) for neuropeptides in the brown planthopper Nilaparvata lugens. Peptides 53, 125–133. doi: 10.1016/j.peptides.2013.07.027
Terhzaz S., Rosay P., Goodwin S. F., Veenstra J. A. (2007). The neuropeptide SIFamide modulates sexual behavior in Drosophila. Biochem. Biophys. Res. Commun. 352, 305–310. doi: 10.1016/j.bbrc.2006.11.030
Tsutsui N., Ohira T., Okutsu T., Shinji J., Bae S. H., Kang B. J., et al. (2013). Molecular cloning of a cDNA encoding vitellogenesis-inhibiting hormonein the whiteleg shrimp Litopenaeus vannamei and preparation of its recombinant peptide using an E. coli expression system. Fisheries Sci. 79, 357–365. doi: 10.1007/s12562-013-0603-z
Ubuka T., Tsutsui K. (2014). Evolution of gonadotropin-inhibitory hormone receptor and its ligand. Gen. Comp. Endocrinol. 209, 148–161. doi: 10.1016/j.ygcen.2014.09.002
Vázquez-Acevedo N., Rivera N. M., Torres-González A. M., Rullan-Matheu Y., Ruíz-Rodríguez E. A., Sosa M. A. (2009). GYRKPPFNGSIFamide (Gly-SIFamide) modulates aggression in the freshwater prawn Macrobrachium rosenbergii. Biol. Bull. 217, 313–326. doi: 10.1086/bblv217n3p313
Veenstra J. A. (2021). The neuropeptide SMYamide, a SIFamide paralog, is expressed by salivary gland innervating neurons in the American cockroach and likely functions as a hormone. Peptides 136, 170466. doi: 10.1016/j.peptides.2020.170466
Verleyen P., Huybrechts J., Baggerman G., Lommel A. V., Loof A. D., Schoofs L. (2004). SIFamide is a highly conserved neuropeptide: a comparative study in different insect species. Biochem. Biophys. Res. Commun. 320, 334–341. doi: 10.1016/j.bbrc.2004.05.173
Verleyen P., Huybrechts J., Schoofs L. (2009). SIFamide illustrates the rapid evolution in arthropod neuropeptide research. Gen. Comp. Endocrinol. 162, 27–35. doi: 10.1016/j.ygcen.2008.10.020
Keywords: SIFa, SIFa receptors, Scylla paramamosain, ovarian development, mud crab
Citation: Chen S, Tang Y, Lu L, Gong S, Liu A and Ye H (2024) SIFa and its receptors play a possibly stimulatory role during ovarian development of the mud crab Scylla paramamosain. Front. Mar. Sci. 11:1494264. doi: 10.3389/fmars.2024.1494264
Received: 10 September 2024; Accepted: 30 October 2024;
Published: 18 November 2024.
Edited by:
Yafei Duan, South China Sea Fisheries Research Institute, ChinaCopyright © 2024 Chen, Tang, Lu, Gong, Liu and Ye. This is an open-access article distributed under the terms of the Creative Commons Attribution License (CC BY). The use, distribution or reproduction in other forums is permitted, provided the original author(s) and the copyright owner(s) are credited and that the original publication in this journal is cited, in accordance with accepted academic practice. No use, distribution or reproduction is permitted which does not comply with these terms.
*Correspondence: An Liu, bGl1YW5Aam11LmVkdS5jbg==; Haihui Ye, aGh5ZUBqbXUuZWR1LmNu