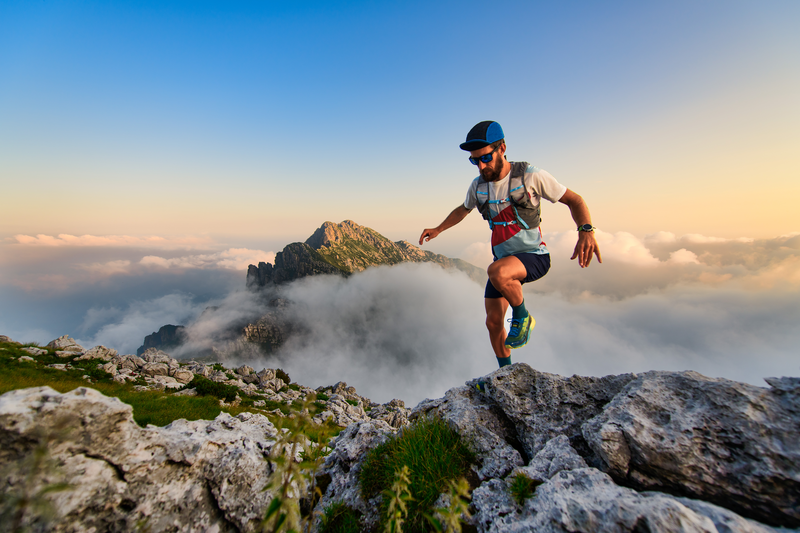
94% of researchers rate our articles as excellent or good
Learn more about the work of our research integrity team to safeguard the quality of each article we publish.
Find out more
ORIGINAL RESEARCH article
Front. Mar. Sci. , 25 November 2024
Sec. Aquatic Physiology
Volume 11 - 2024 | https://doi.org/10.3389/fmars.2024.1490406
Studies of interspecific differences in the physiological performance of animals and their relationship with metabolism may provide insight into how selection influences diversity in phenotypic traits. To investigate the effects of food habits on growth, digestion, specific dynamic action (SDA), and the intestinal microbiota and verify their relationships in freshwater fishes, we measured the specific growth rate (SGR), meal remaining in the gastrointestinal tract, postprandial metabolic response, and intestinal microbial diversity of nine freshwater fish species with different food habits (three carnivorous, four omnivorous, and two herbivorous) fed at 25°C. The SGR, energy ingested (EI), energy efficiency (EE), gastrointestinal evacuation rate (GER), peak metabolic rate (PMR), peak metabolic scope (PMS), and SDA duration values were the highest in carnivores and the lowest in herbivores. The routine metabolic rate (RMR) and SDA coefficient values of carnivores were also significantly greater than those of omnivores and herbivores. The SGR was also significantly and positively correlated with the EI, GER, PMS, and SDA coefficients. These results indicated that growth performance, feeding and digestion abilities, and feeding metabolic responses were the strongest in carnivores and the weakest in herbivores. There was no significant difference in the richness of the intestinal microbiota with different food habits, whereas omnivores presented the highest diversity, followed by herbivores, and carnivores presented the lowest diversity. Moreover, while the phylum composition of the intestinal microbiota in fish with different food habits was similar, significant differences were observed in the composition proportions among carnivores. This might be related to the host’s ability to digest and utilize proteins, fats, and carbohydrates. We suggest that the interspecific differences in growth associated with different food habits are related to their feeding and digestive abilities, metabolic response, and gut microbiota.
Animals can be classified as carnivores, omnivores, or herbivores according to their food habits. The investigation of growth, food habits, and their relationships has a long history in fishes and other animals, as it involves fields related to physiology, ecology, behavior, and evolution (Held and Peterka, 1974; Kooyman and Donald, 1977; Mayntz et al., 2005). Like all other vertebrates, fish need time for digestion, absorption, and assimilation after being fed for energy accumulation. The growth ability of fish is not only influenced by external environmental conditions but also limited by internal physiological factors, such as digestive capacity and metabolism (Pérez-Casanova et al., 2009; Dan et al., 2014). Previous studies suggested that the growth of animals may be more easily limited by their digestive capacity than by the availability of food under conditions of abundant food resources (Kvist and Lindstrom, 2000; Speakman and Król, 2005). Fishes require additional energy consumption during digestion, assimilation, and growth processes (Jobling, 1981a). The characteristics of the feeding metabolism of fishes exhibit wide and consistent interspecific differences (Secor, 2009). For example, after feeding, the duration of feeding metabolism ranges from several hours to more than 10 days, and the energy consumed for feeding metabolism accounts for less than 2% to more than 50% of the energy in the food among fish species (Secor, 2009). Therefore, different fish species might have different growth potentials in terms of their digestive capacity and metabolic response to food and/or feeding habits.
For fish species, the gastrointestinal content weight can be used to estimate daily food rations, and the gastrointestinal evacuation rate (GER, i.e., the reduction in gastrointestinal contents over time after feeding) can be used to estimate the physiological performance of digestion and assimilation (Jobling, 1981b; Miyasaka et al., 2005; Bunnell, 2013). Several previous studies have shown that the GER is correlated with growth ability under different conditions in some fish species (Sveier et al., 1999; Speakman and Król, 2005; Pang et al., 2022). The relationship between growth rate and digestive capacity has also been investigated at the histological, physiological, and biochemical levels, such as gut morphology and size, the hepatosomatic index, and metabolic enzyme activity in the intestines of some fish species (Couture et al., 1998; Stevens et al., 1999; Bélanger et al., 2002; Messina et al., 2023). An increase in the metabolic rate above the routine metabolic rate (RMR) is a physiological response to feeding, a phenomenon commonly referred to as specific dynamic action (SDA) (Jobling, 1981a). The energy consumption of SDA includes parts such as ingesting, digesting, and assimilating food (Jobling, 1981a; Secor, 2009). The energy expended during SDA is determined by the peak metabolic scope [PMS, i.e., the difference between the peak metabolic rate (PMR) and RMR], the SDA duration, and the shape of the feeding metabolic power curve. Several studies have demonstrated that increased growth rates are associated with increased postprandial metabolic rates and vice versa (Carter and Brafield, 1992; Leggatt et al., 2003; Li et al., 2013; Moffatt et al., 2022). Therefore, understanding and testing the complex interactions among growth, digestion, and feeding metabolism are important and of interest to the fields of fish physiology and aquaculture.
The intestinal microbiota plays an important role in aquatic animal nutrition and immunology (Wang et al., 2024). The intestinal microbiota absorbs host nutrients or decomposes host food to synthesize their own nutrients, and the intestinal microbiota can also secrete digestive enzymes and synthesize vitamins in some fish species (Clements et al., 2014; Chen et al., 2015). In addition, intestinal probiotics can inhibit the increase in pathogenic bacteria and thus protect organisms from pathogen infections in some fish species (Sugita et al., 1997). Previous studies have revealed a potential correlation between intestinal probiotics and food habits, growth, and metabolic responses in some fish species (Moran et al., 2005; Sakyi et al., 2020; Wang et al., 2023).
To evaluate these hypotheses, we measured nine freshwater fish species with different food habits. Three species (Silurus meridionalis, a benthic ambush-feeding species, Micropterus salmoides, a slow-flow species living in the middle and lower layers of water bodies, and Siniperca chuatsi, a benthic still-water species pursuing living prey) are carnivorous; four species (Oreochromis niloticus, a euryphagous species living in the lower layers of water bodies; Cyprinus carpio, a eurytopic and widely distributed species; Carassius auratus, a eurytopic and widely distributed species; and Spinibarbus sinensis, an active fast-flow species) are omnivorous; and two species (Megalobrama amblycephala, a still-water species living in the middle and lower layers of water bodies; and Ctenopharyngodon idella, an active slow-flow species) are herbivorous. S. meridionalis belongs to the order Siluriformes, and the families Siluridae, M. salmoides, S. chuatsi and O. niloticus belong to the order Perciformes and the families Centrarchidae, Serranidae and Cichlidae, respectively. These species are all important aquaculture fish in China. Our goals were to (a) evaluate the interspecific differences and effects of food habits on growth, digestion, feeding metabolism, and the intestinal microbiota, and (b) verify their relationships in freshwater fishes. To achieve these goals, the fishes were subjected to specific growth rate (SGR), GER, and SDA parameters and intestinal microbial diversity measurements.
All nine experimental freshwater fish species (approximately 200 each species) were purchased from local fishermen (aquaculture farms) in Chongqing, China. All the experimental fish were approximately 6–9 months old. Before purchase, these experimental fish were fed mainly formulated food (for carnivores, approximately 45% protein; for omnivores and herbivores, approximately 30% protein). Prior to the experiment, the fish were maintained for 2–3 weeks in laboratory polyvinyl chloride water tanks (200 cm × 120 cm × 70 cm) with recirculating water (to maintain consistency of conditions, all tanks were interconnected). During this period, the temperature of the fresh dechlorinated water used was maintained at 24.5°C–25.5°C, the water oxygen content was above 7.0 mg L−1, the pH ranged from 6.5–7.3, and the ammonia-N content varied from 0.005 to 0.025 ppm. The photoperiod was maintained at 12 h light: 12 h dark. The fish were fed to satiate daily at 12:00 with commercial diets [for carnivores, Guangdong Haid Group, Guangzhou, China, dry composition: 49% protein, 5% lipid, 3% carbohydrate, 18% ash, and 14.055 kJ g−1 bioavailable energy; for omnivores and herbivores, Tongwei Group, Chengdu, China; dry composition: 30% protein, 3% lipid, 12% carbohydrate, 16% ash, and 10.329 kJ g−1 bioavailable energy; the diets were dried to constant weight at 70°C for approximately 48 h, and the dietary bioavailable energy was calculated as protein, 23.6 kJ g−1; lipid, 39.5 kJ g−1; and starch, 17.2 kJ g−1 (Fu et al., 2009)]. The uneaten food and feces were removed, and approximately one quarter of the water in each tank was replaced daily at 13:00 with freshwater to maintain good water quality.
After being held in the water tanks, the similarly sized fish of each species were transferred individually into separate compartments (25 cm × 15 cm × 20 cm) immersed in the tanks for measurements of growth and GER or the separate respirometer chamber (350 ml) of the continuous-flow respirometer (see set up in Fu et al., 2005a) for measurements of the SDA parameters. These individuals were allowed to acclimate for 7 days in these separate compartments or respirometer chambers (more than the rated sample individuals of each species were allowed to acclimate because some experimental fish did not feed in separate compartments or respirometer chambers, and then the rated sample individuals who had completed feeding were selected for any measurements). During the acclimation period, the fish were individually fed food with a maintenance ration daily. To reduce the influence of undigested food on the physiological status of the fish, the S. meridionalis was fasted for 3 days, and the other species were fasted for 2 days before any measurements were taken.
After fasting, growth was measured for a 28-day period. During the experimental period, all the fishes were individually allowed to satiate once daily (at 12:00) with commercial diets of similar size pellets (for carnivores, 0.4850 kJ per pellet, and for omnivores and herbivores, 0.3776 kJ per pellet; see the previous paragraph for dietary composition). Uneaten food and faces were removed 1 h after feeding, and food consumption was calculated on the basis of the number and average weight of the pellets. The other conditions remain consistent with the holding period. At the beginning and end of the 28-day experimental period, the experimental fish were lightly anesthetized (MS222, tricaine methanesulfonate, 50 mg L−1) for approximately 2 min, and body mass was then measured to the nearest 0.1 g. The following growth parameters were calculated:
where M0 and Mt are the initial and final body masses (g) of the experimental fish, respectively, t is the experimental period (d), and Ec represents the total energy (kJ) of food consumed by the fish.
After fasting, the experimental fishes were lightly anesthetized (MS222, 50 mg L−1) for approximately 2 min, after which their body mass was measured. On the next day, the fishes were fed to the average EI per unit weight (the feeding regimen was the same as that used for the fish measured for growth in each species). These experimental fishes, which were able to complete the predetermined feeding amount within 1 h after feeding, were anesthetized (MS222, 100 mg L−1) and sacrificed for dissection of the gastrointestinal tract. Undigested feed contents were collected at 1, 2, and 4 h post feeding and at other time points (after 4 h, samples were taken at 4-h intervals until digestion and absorption were completed, i.e., at a certain time point, some individuals had no meal remaining in the gastrointestinal tract, with six individuals per time point; S. meridionalis for 32 h; M. salmoides and C. carpio for 24 h; S. chuatsi and O. niloticus for 20 h; C. auratus, S. sinensis, and M. amblycephala for 16 h; and C. idella for 12 h). The gastrointestinal contents were dried to constant weight at 70°C for approximately 48 h and then measured to the nearest 0.001 g, and the bioavailable energy value of the remaining meal was calculated. The GER (kJ kg−1 h−1) is the decrease in the remaining energy value of the meal (kJ kg−1) per hour and was obtained from linear regressions of the postfeeding time (h) and remaining energy value of the meal, that is, the absolute value of the slope of the regression equation.
After fasting, the experimental fishes were lightly anesthetized (MS222, 50 mg L−1) for approximately 2 min, after which their body mass was measured. After the measurements were completed, the experimental fish were transferred individually into the respirometer chamber. The next day, the oxygen consumption rate (MO2) was measured six times at 1-h intervals, and the means were used as the RMR (i.e., the prefeeding baseline). The fishes were then fed to the average EI per unit weight (the feeding regimen was the same as that used for the fish measured for growth in each species). After 1 h, the chambers were immediately closed. The postprandial MO2 of those experimental fish, which were able to complete the predetermined feeding amount within 1 h after feeding, was measured at 2 h (S. meridionalis) or 1 h (other species) until the MO2 returned to the RMR level of prefeeding (eight individuals in each species; S. meridionalis for 48 h, M. amblycephala and C. idella for 18 h, and other species for 24 h). The following formula was used to calculate the MO2 (mgO2 kg−1 h−1) of individual fish:
where ΔO2 is the difference in the oxygen concentration (mgO2 L−1) between the experimental chamber and the control chamber (chamber without fish), v is the water flow rate in the experimental chamber (L h−1), and m is the body mass of the fish (kg). The dissolved oxygen concentration was measured at the outlet of the chamber with an oximeter (HQ30, Hach Company, Loveland, CO, USA). The flow rate of water through the respirometer chamber was measured by collecting the water outflow from each tube. The PMR is the postprandial maximum metabolic rate; the PMS is the difference between the PMR and RMR; the SDA duration is the time from feeding to when the metabolic rate returns to within the standard deviation of the RMR of a given fish (Fu et al., 2005b); the energy expended during SDA is the accumulated energy expended above the RMR for the duration of the SDA response, and the oxygen consumption is converted to energy by using a conversion factor of 13.84 J mgO2−1 (Guinea and Fernandez, 1997); the SDA coefficient is the SDA divided by the meal energy.
Fresh whole gut samples were collected after 3 weeks of holding for gut microbial analysis (S. meridionalis were fasted for 3 days, and the other species were fasted for 2 days), and 135 fish were selected from the 9 fish species (3 individuals per measurement, 5 measurements per species). Bacterial genomic DNA was extracted from the contents of the frozen gut samples stored in liquid nitrogen via a QIAamp PowerFecal Pro DNA Kit (QIAGEN, Germany) according to the manufacturer’s instructions. The V3-V4 region of the 16S rRNA gene was amplified via two specific primers (Yan et al., 2020). The PCR products were sequenced on an Illumina HiSeq 2500 platform (Illumina Inc., San Diego, CA) by Biomarker Technologies Corporation (Beijing, China). After demultiplexing and quality filtering, high-quality reads are clustered into amplicon sequence variants (ASVs). Bacterial ASVs were classified via the SILVA database and then denominated at the phylum level (Gupta et al., 2019). The analyses of the correlation between bacterial communities were executed on the online Biomarker Cloud platform (www.biocloud.net). The analysis of microbial diversity data was conducted statistically via SPSS statistical software (IBM® SPSS® Statistics).
STATISTICA 6.0 (StatSoft Inc., Tulsa, OK, USA) was used for data analysis, and P < 0.05 was used as the statistical criterion of significance. The effects of food habits and species on growth parameters (SGR, EI, and EE) and the GER and SDA parameters (RMR, PMR, PMS, duration, energy expended during SDA, and the SDA coefficient) were tested via two-factor analysis of variance (ANOVA), with food habits and species as primary and secondary fixed factors, respectively; the initial body mass was used as a covariate for growth parameters; and body mass was used as a covariate for SDA parameters. ANOVA was followed by Duncan’s multiple range test if a statistical evaluation comparing different food habits and species group values was necessary. The effects of species and time postfeeding on the meal remaining in the gastrointestinal tract were tested via two-factor ANOVA, with both food habits and species as fixed factors and body mass as a covariate. ANOVA was followed by a Duncan’s multiple range test if a statistical evaluation was necessary for comparing different time postfeeding group values for the same species or different species group values for the time postfeeding. The relationship between the amount of meal remaining and the duration of postfeeding was described by a linear equation for each species group. The relationships among the SGR, EI, and EE and among the SGR, GER, and PMS were analyzed via Pearson’s correlation analysis. The effects of the alpha diversity index on the intestinal microbiota were tested via the Kruskal-Wallis H test, with both food habits and species as fixed factors.
Both food habits and species had significant effects on SGR, EI, and EE (P < 0.001; Table 1). The SGR, EI, and EE values were the highest in carnivores and the lowest in herbivores (P < 0.05; Table 1).
Table 1. Specific growth rate (SGR), energy ingested (EI), and energy efficiency (EE) of nine freshwater fish species with different food habits (mean ± S.E., n = 10).
The SGR was significantly and positively correlated with the EI (r = 0.910, P = 0.001; Figure 1A) and EE (r = 0.915, P = 0.001; Figure 1B), and the EI was also significantly and positively correlated with the EE (r = 0.754, P = 0.019; Figure 1C).
Figure 1. Relationships between specific growth rate (SGR) and energy ingested (EI) (A), between SGR and energy efficiency (EE) (B) and between EI and EE (C) among nine freshwater fish species (mean ± S.E.). (1. S. meridionalis, 2. M. salmoides, 3. S.chuatsi, 4. O. niloticus, 5. C. carpio, 6. C. auratus, 7. S. sinensis, 8. M. amblycephala, 9. C. idella).
Both species (F8,315 = 433.947, P < 0.001) and time postfeeding (F9,315 = 656.707, P < 0.001) significantly affected the number of meals remaining in the gastrointestinal tract (Table 2). The remaining meal values significantly differed among the different species groups within each treatment and changed with time postfeeding (interaction effect, F45,315 = 11.290, P < 0.001; Table 2).
Table 2. Meals remaining in the gastrointestinal tract of nine freshwater fish species with different food habits (mean ± S.E., n = 6).
Both food habits and species had significant effects on GER (P < 0.001; Figure 2). The GER values were the highest in carnivores and the lowest in herbivores (P < 0.05; Figure 2J).
Figure 2. Gastrointestinal evacuation rates (GERs) of nine freshwater fish species with different food habits. [(A–I). The relationship between the meal remaining and the duration of postfeeding; (J) The GER is the absolute value of the slope of this relationship. (A–C) Values followed by different capital letters indicate significant differences among the different food habit groups. (a–d) Values followed by different lowercase letters indicate significant differences among species (P < 0.05), (mean ± S.E.)].
Both food habits and species had significant effects on the RMR, PMR, and PMS (P < 0.001; Table 3; Figure 3). The RMR of the carnivores was significantly greater than that of the omnivores and herbivores (P < 0.05), whereas there was no difference between the omnivores and herbivores (Table 3). The PMR and PMS values were the highest in carnivores and the lowest in herbivores (P < 0.05; Table 3).
Table 3. SDA parameters of nine freshwater fish species with different food habits (mean ± S.E., n = 8).
Both food habits and species had significant effects on SDA duration (P < 0.001; Table 3). The SDA duration was the longest in carnivores and the shortest in herbivores (P < 0.05; Table 3).
Both food habits and species had significant effects on expansion during SDA (P < 0.001; Table 3). The amount expended during SDA was the highest in carnivores and the lowest in herbivores (P < 0.05; Table 3). Food habits had a significant effect on the SDA coefficient (P < 0.001), whereas species had no effect on the SDA coefficient (Table 3). The SDA coefficient of the carnivores was significantly greater than those of the omnivores and herbivores (P < 0.05), whereas there was no difference between the omnivores and herbivores (Table 3).
The SGR was significantly and positively correlated with the GER (r = 0.840, P = 0.005; Figure 4A) and PMS (r = 0.856, P = 0.003; Figure 4B), and the GER was also significantly and positively correlated with the PMS (r = 0.894, P = 0.001; Figure 4C).
Figure 4. Relationships between the specific growth rate (SGR) and gastrointestinal evacuation rate (GER) (A), between the SGR and peak metabolic scope (PMS) (B), and between the GER and PMS (C) among nine freshwater fish species (mean ± S.E.). (1. S. meridionalis, 2. M. salmoides, 3. S. chuatsi, 4. O. niloticus, 5. C. carpio, 6. C. auratus, 7. S. sinensis, 8. M. amblycephala, 9. C. idella).
The high-quality sequences were clustered into 6,257 ASVs to analyze the similarity in bacterial communities among the different groups via a Venn diagram. Only three ASVs were clustered together across all species. The number of ASVs in carnivores ranged from 339 to 603, that in omnivores ranged from 427 to 1,191, and that in herbivores ranged from 307 to 875, with crucian having the highest number of ASVs (Figure 5A). The number of ASVs in omnivores differed significantly from that in carnivores and herbivores.
Figure 5. Intestinal microbial diversity in freshwater fishes with different feeding habits. (A) Venn diagram of 100% amplicon sequence variants (ASVs) of each group. (B) Venn diagram of 100% ASVs associated with each feeding habit. (C) Principal coordinate analysis (PCoA) based on the binary Jaccard distance. (D) Alpha diversity index of each group (mean ± S.E., P value determined by the Kruskal-Wallis H test). ACE: abundance-based coverage estimator; Chao1: number of ASVs index; Simpson: Simpson’s index of diversity (1-Dsimpson); Shannon: Shannon’s entropy. The greater the Simpson index and Shannon’s entropy values are, the greater the diversity of the microorganisms. (E) Hierarchical clustering and heatmap showing the percentages of different groups by abundance. Species of high and low abundance are grouped into blocks to reflect the similarities and differences in the compositions of multiple sample communities via color gradients and varying degrees of similarity. (F) Microorganism relative abundance at the phylum level. (G) Microorganism relative abundance at the genus level. (H) BugBase predicts organism-level microbiome phenotypes. (A. S. meridionalis, B M. salmoides, C S. chuatsi, D O. niloticus, E C. carpio, F C. auratus, G S. sinensis, H M. amblycephala, I C. idella, X. carnivorous, Y. omnivorous, Z. herbivorous).
The results of principal coordinate analysis (PCoA) revealed that the bacterial communities were diverse, as they clustered separately in the plot and presented different degrees of similarity in terms of species abundance among all the samples (Figure 5B). Although crucian carp presented the highest species richness, the alpha diversity index revealed no significant differences in species richness. Moreover, there was a significant difference in the diversity of the intestinal microbiota, with omnivores displaying the highest diversity, followed by herbivores, and carnivores showing the least diversity (Figure 5C). Hierarchical clustering and heatmaps also support this point (Figure 5F).
While the phylum and genus compositions of the intestinal microbiota in fish with different feeding habits were similar, significant differences were observed in the composition proportions among the groups. Proteobacteria were dominant, although there were exceptions among S. meridionalis and S. chuatsi (Figure 5D). Cetobacterium occupied a dominant position in most genus groups, except for S. meridionalis and C. auratus. Mycoplasma had the highest proportion in S. meridionalis, whereas Aeromonas had the highest proportion in C. auratus (Figure 5E). Moreover, BugBase can be used to predict microbiome phenotypes at the organism level. These findings indicate that most fish intestinal microbiome phenotypes are similar, except for S. chuatsi, which presents more anaerobic and gram-positive phenotypes (Figure 5G).
In this study, we measured the growth, digestion, SDA, and gut microbiota parameters of nine freshwater fish species with different food habits. This study revealed that the growth (SGR, EI, and EE), digestion (GER), and feeding metabolism (PMR and PMS) values were the highest in carnivores and the lowest in herbivores. The SGR was significantly and positively correlated with EI, GER, and PMS. Analysis of intestinal microbial diversity revealed a significant difference in the diversity of the intestinal microbiota, with omnivores showing the highest diversity, followed by herbivores, and carnivores showing the lowest diversity. We suggest that the different growth performances of freshwater fishes with different food habits are related to their feeding and digestive abilities, metabolic response, and gut microbiota.
Research on the relationships between food habits and growth has been highly common in recent decades in animals (Held and Peterka, 1974; Kooyman and Donald, 1977; Mayntz et al., 2005). From the perspectives of physiology and nutriology, it is generally believed that the food (i.e., prey) composition is nutritionally balanced for carnivores, which consequently should be limited by the availability of food (Galef, 1996), but omnivores and herbivores should face a heterogeneous nutritional environment and therefore possess a well-developed ability to balance their intake of multiple nutrients (Raubenheimer and Simpson, 1997). In addition, carnivores have separate stomachs in their digestive system, whereas omnivores (except for O. niloticus) and herbivores have no such structure in this study. Consequently, under conditions of abundant food resources (e.g., under culture conditions), carnivores have a higher energy intake rate than omnivores and herbivores do. Our results revealed that the SGR and EI values were the highest in carnivores and the lowest in herbivores and that the SGR was also positively correlated with EI among the different freshwater fish species. Therefore, the greater the energy intake, the faster the growth of freshwater fish. For omnivores and herbivores, increasing their feeding frequency not only ensures nutritional balance but also compensates for growth (Raubenheimer and Simpson, 1997; Wu et al., 2021). In fact, omnivores need much time to obtain food, and the current feeding regime (once daily) is insufficient, which is also an important factor that affects their growth.
Feed efficiency is influenced by feed intake; when feed intake is below a rated level, feed efficiency increases with feed intake (Storebakken and Austreng, 1987; Hung et al., 1989; Tuene and Nortvedt, 1995; Du et al., 2006). Our results revealed that the EI and EE values were the highest in carnivores and the lowest in herbivores, and the EI was positively correlated with the EE among the different freshwater fish species. These results indicate that species with high energy intake rates have relatively high feed conversion efficiencies. In addition, maintenance energy consumption might also affect feed efficiency; for example, among three carnivorous species, the RMR of S. chuatsi was greater than those of S. meridionalis and M. salmoides and therefore had a lower EE in this study (Table 1).
Several previous studies reported that the gastrointestinal evacuation time increased and that the absolute GER (food reduction per unit time in the gastrointestinal tract) increased with increasing meal size in some fish species (Persson, 1981; Andersen, 1998; Pääkkönen et al., 2010; Smith et al., 2011). Food is digested from the surface, and the specific surface area (the ratio of surface area to volume) increases with increasing meal size; therefore, the absolute GER also increases with increasing meal size (Andersen, 1998; Pääkkönen et al., 2010; Smith et al., 2011). Our results revealed that the GER and EI values were the highest in carnivores and the lowest in herbivores, and the GER was also positively correlated with the EI among the different freshwater fish species (r = 0.913, n = 9, P = 0.001). In the present study, the food habits of the fish were closely related to their digestive enzyme activity and digestive tract structure (Párrizas et al., 1994; Hidalgo et al., 1999; Rong et al., 2023). The protease and lipase activities of carnivorous fish are greater than those of herbivorous and omnivorous fish; however, carnivorous fish present lower amylase activity (Párrizas et al., 1994; Rong et al., 2023). Compared with omnivorous and herbivorous individuals, carnivorous individuals have longer and wider intestinal microvilli and thicker intestinal muscle layers (Rong et al., 2023). These results indicated that the digestive ability (for protein and fat digestion) was the strongest in carnivores (3% carbohydrate in the diets of carnivores in this study) and the weakest in herbivores.
During the processes of SDA, digestion, and nutrient assimilation, animals experience a postprandial increase in metabolic rate and increased energy consumption (Jobling, 1981a; McCue, 2006; Secor, 2009). In the process of digestion, the energy cost is related to the mechanical and chemical breakdown of food macromolecules (secretion of digestive enzymes and digestive juices) and the absorption of the resulting nutrients from the gut into the blood (Reenstra and Forte, 1981; Secor, 2009; Moffatt et al., 2022). Our results revealed that the PMS and GER values were highest in carnivores and lowest in herbivores, and the PMS was also positively correlated with the GER and EI (r = 0.874, n = 9, P = 0.002) among the different freshwater fish species. Therefore, similar to GER, the difference in EI is the primary contributor to the difference in PMS (Fu et al., 2005b, 2009). These results suggested that the higher PMS species had a greater feeding metabolic response because of their greater energy intake and stronger digestive ability, and vice versa.
Some previous studies have suggested that a larger SDA and/or higher postprandial metabolic rate are associated with a higher rate of protein synthesis in some fish species and thus faster growth (Hidalgo and Alliot, 1988; Carter and Brafield, 1992; McCarthy et al., 1994, 1999; Leggatt et al., 2003; Li et al., 2013; Moffatt et al., 2022). Our results revealed that the SGR and PMS values were the highest in carnivores and the lowest in herbivores, the SDA coefficient of carnivores was greater than those of omnivores and herbivores, and the SGR was also positively correlated with the PMS and SDA coefficients (r = 0.823, n = 9, P = 0.006) among the different freshwater fish species. These results suggested that the faster growing species presented a greater feeding metabolic response and a greater SDA for digestion and assimilation.
In this study, we compared the intestinal microbiota of individuals with different food habits. Although the main phyla of bacteria are not the same, a similar community composition was observed, consisting of four dominant phyla: Proteobacteria, Firmicutes, Fusobacteria, and Bacteroidota. The richness of the intestinal microbiota in fish with different food habits was relatively consistent. However, there was a significant difference in the diversity of the intestinal microbiota, with omnivores showing the highest diversity, followed by herbivores, and carnivores showing the lowest diversity. These results were similar to those of previous studies (Jiao et al., 2023). In general, carnivorous fish species have shorter intestines (Day et al., 2014) and are unable to utilize carbohydrates (Ma et al., 2019), which might lead to a greater GER. Consequently, the intestinal microbiota in carnivores tends to increase the absorption of proteins and fats in the diet. In contrast, omnivores need to obtain nutrients from a variety of foods, which may lead to a more complex diversity of their intestinal microbiota. Acinetobacter and Pseudomonas species might help the host digest protein, whereas Bacteroidetes species may help the host digest cellulose (Day et al., 2014). In addition, our results revealed that both Simpson (r = 0.835, n = 9, P = 0.005) and Shannon (r = 0.797, n = 9, P = 0.010) diversities were negatively correlated with the SDA coefficient among the different freshwater fish species. Therefore, the high gut microbiota diversity of fish species could reduce energy consumption during feeding metabolism. Together, these factors may lead to differences in the intestinal microbial diversity and composition of different food habits of different fish species. Although we found correlations between gut microbiota diversity and fish physiology, these correlations do not imply causation. Further studies are needed to establish the causal relationships between these variables.
However, this study has several limitations: (a) the macronutrient composition (i.e., the relative contents of proteins, carbohydrates, and lipids) might ultimately affect the growth, digestion, feeding metabolism, and gut microbiota of some fish species (Naik et al., 2000; Fu and Xie, 2005; Monentcham et al., 2010; Fu et al., 2005a; Pang et al., 2022). Fish with different food habits have different nutritional requirements and tolerances (Fu and Xie, 2005; Monentcham et al., 2010; Chen et al., 2020). Therefore, the macronutrient compositions of the diets used by carnivores, omnivores, and herbivores were different in this study. (b) Genetic background has a profound effect on physiological ability. In fact, Siluriformes (S. meridionalis), Perciformes (M. salmoides, S. chuatsi, and O. niloticus), and Cypriniformes (C. carpio, S. sinensis, M., amblycephala, and C. idella) presented the highest, moderate, and lowest growth and digestive performance, respectively. (c) To ensure consistency of experimental conditions, it is not appropriate to use consistent feeding methods (feeding time points and feeding frequency), for example, for omnivores and herbivores, feeding once a day is not sufficient for growth (Raubenheimer and Simpson, 1997; Wu et al., 2021), aquaculture conditions (temperature and photoperiod), for example, the optimal feeding temperature for S. meridionalis is 29°C rather than 25°C (Xie and Sun, 1992), or fish behaviors (feeding behavior and social interaction); for example, for benthic fish, nighttime is more suitable for feeding than daytime is for species with different feeding and ecological habits. Specifically, the response of freshwater fish growth, digestion, metabolic reactions, and so forth, to environmental conditions and the integration of production practices with laboratories are worthy of further research.
The raw data supporting the conclusions of this article will be made available by the authors, without undue reservation.
The animal study was approved by Animal Care and Use Committee of the Key Laboratory of Animal Biology of Chongqing. The study was conducted in accordance with the local legislation and institutional requirements.
XP: Conceptualization, Methodology, Visualization, Data curation, Formal analysis, Investigation, Software, Writing – original draft. JY: Data curation, Investigation, Methodology, Writing – review & editing. S-AX: Investigation, Methodology, Writing – review & editing. HS: Investigation, Methodology, Writing – review & editing. S-JF: Conceptualization, Methodology, Resources, Supervision, Visualization, Writing – review & editing.
The author(s) declare that no financial support was received for the research, authorship, and/or publication of this article.
We thank the reviewers and the associate editor (Yafei Duan) for their constructive comments.
The authors declare that the research was conducted in the absence of any commercial or financial relationships that could be construed as a potential conflict of interest.
All claims expressed in this article are solely those of the authors and do not necessarily represent those of their affiliated organizations, or those of the publisher, the editors and the reviewers. Any product that may be evaluated in this article, or claim that may be made by its manufacturer, is not guaranteed or endorsed by the publisher.
Andersen N. ,. G. (1998). The effect of meal size on gastric evacuation in whiting. J. Fish. Biol. 52, 743–755. doi: 10.1111/j.1095-8649.1998.tb00817.x
Bélanger F., Blier P. U., Dutil J. D. (2002). Digestive capacity and compensatory growth in Atlantic cod (Gadus morhua). Fish. Physiol. Biochem. 26, 121–128. doi: 10.1023/A:1025461108348
Bunnell M. (2013). Gastric evacuation rate, index of fullness, and daily ration of Lake Michigan slimy (Cottus cognatus) and deepwater sculpin (Myoxocephalus thompsonii). J. Great. Lakes. Res. 39, 327–335. doi: 10.1016/j.jglr.2013.03.007
Carter C. G., Brafield A. E. (1992). The relationship between specific dynamic action and growth in grass carp, Ctenopharyngodon idella. J. Fish. Biol. 40, 895–907. doi: 10.1111/j.1095-8649.1992.tb02635.x
Chen J. X., Feng J. Y., Zhu J., Luo L., Lin S. M., Wang D. S., et al. (2020). Starch to protein ratios in practical diets for genetically improved farmed Nile tilapia Oreochromis niloticus: Effects on growth, body composition, peripheral glucose metabolism and glucose tolerance. Aquaculture 515, 734538. doi: 10.1016/j.aquaculture.2019.734538
Chen Y. J., Yuan R. M., Liu Y. J., Yang H. J., Liang G. Y., Tian L. X. (2015). Dietary vitamin c requirement and its effects on tissue antioxidant capacity of juvenile largemouth bass, Micropterus Salmoides. Aquaculture 435, 431–436. doi: 10.1016/j.aquaculture.2014.10.013
Clements K. D., Angert E. R., Montgomery W. L., Choat J. H. (2014). Intestinal microbiota in fishes: what’s known and what’s not. Mol. Ecol. 23, 1891–1898. doi: 10.1111/mec.2014.23.issue-8
Couture P., Dutil J. D., Guderley H. (1998). Biochemical correlates of growth and condition in juvenile Atlantic cod from Newfoundland. Can. J. Fish. Aquat. Sci. 55, 1591–1598. doi: 10.1139/f98-045
Dan X. M., Yan G. J., Zhang A. J., Cao Z. D., Fu S. J. (2014). Effects of stable and diel-cycling hypoxia on hypoxia tolerance, postprandial metabolic response, and growth performance in juvenile qingbo (Spinibarbus sinensis). Aquaculture 428-429, 21–28. doi: 10.1016/j.aquaculture.2014.02.025
Day R. D., Tibbetts I. R., Secor S. M. (2014). Physiological responses to short-term fasting among herbivorous, omnivorous, and carnivorous fishes. J. Comp. Physiol. B. 184, 497–512. doi: 10.1007/s00360-014-0813-4
Du Z. Y., Liu Y. J., Tian L. X., He J. G., Cao J. M., Liang G. Y. (2006). The influence of feeding rate on growth, feed efficiency and body composition of juvenile grass carp (Ctenopharyngodon idella). Aquacult. Int. 14, 247–257. doi: 10.1007/s10499-005-9029-7
Fu S. J., Xie X. J. (2005). Effect of dietary carbohydrate levels on growth performance in Silurus meridionalis Chen. Acta Hydro. Sin. 29, 393–398. doi: 10.1360/biodiv.050121
Fu S. J., Xie X. J., Cao Z. D. (2005a). Effect of dietary composition on specific dynamic action in southern catfish Silurus meridionalis Chen. Aquac. Res. 36, 1384–1390. doi: 10.1111/j.1365-2109.2005.01356.x
Fu S. J., Xie X. J., Cao Z. D. (2005b). Effect of feeding level and feeding frequency on specific dynamic action in Silurus meridionalis. J. Fish. Biol. 67, 171–181. doi: 10.1111/j.0022-1112.2005.00722.x
Fu S. J., Zeng L. Q., Li X. M., Pang X., Cao Z. D., Peng J. L., et al. (2009). The behavioural, digestive and metabolic characteristics of fishes with different foraging strategies. J. Exp. Biol. 212, 2296–2302. doi: 10.1242/jeb.027102
Galef B. G. (1996). Food selection: problems in understanding how we choose foods to eat. Neurosci. Biobehav. Rev. 20, 67–73. doi: 10.1016/0149-7634(95)00041-C
Guinea J., Fernandez F. (1997). Effect of feeding frequency, relative meal size and temperature on energy metabolism in Sparus aurata. Aquaculture 148, 125–142. doi: 10.1016/S0044-8486(96)01424-X
Gupta S., Mortensen M. S., Schjørring S., Trivedi U., Vestergaard G., Stokholm J., et al. (2019). Amplicon sequencing provides more accurate microbiome information in healthy children compared to culturing. Commun. Biol. 2, 291. doi: 10.1038/s42003-019-0540-1
Held J. W., Peterka J. J. (1974). Age, growth, and food habits of the fathead minnow, Pimephales promelas, in North Dakota Saline Lakes. Trans. Am. Fish. Soc 103, 743–756. doi: 10.1577/1548-8659(1974)103<743:AGAFHO>2.0.CO;2
Hidalgo F., Alliot E. (1988). Influence of water temperature on protein requirement and protein utilization in juvenile sea bass, Dicentrarchus labrax. Aquaculture 72, 115–129. doi: 10.1016/0044-8486(88)90152-4
Hidalgo M. C., Urea E., Sanz A. (1999). Comparative study of digestive enzymes in fish with different nutritional habits. proteolytic and amylase activities. Aquaculture 170, 267–283. doi: 10.1016/S0044-8486(98)00413-X
Hung S. S. O., Lutes P. B., Conte F. S., Storebakken T. (1989). Growth and feed efficiency of whit sturgeon (Acipenser transmontanus) sub-yearling at different feeding rates. Aquaculture 80, 147–153. doi: 10.1016/0044-8486(89)90280-9
Jiao F., Zhang L., Limbu S. M., Yin H., Xie Y., Yang Z., et al. (2023). A comparison of digestive strategies for fishes with different feeding habits: Digestive enzyme activities, intestinal morphology, and gut microbiota. Ecol. Evol. 13, e10499. doi: 10.1002/ece3.10499
Jobling M. (1981a). The influences of feeding on themetabolic rate of fishes: a short review. J. Fish. Biol. 18, 385–400. doi: 10.1111/j.1095-8649.1981.tb03780.x
Jobling M. (1981b). Mathematical models of gastric emptying and the estimation of daily rates of food consumption for fish. J. Fish. Biol. 19, 245–257. doi: 10.1111/j.1095-8649.1981.tb05829.x
Kooyman D. B., Donald A. H. (1977). Food, feeding habits, and growth of goldeye, Hiodon alosoides (Rafinesque), in waters of the Peace-Athabasca Delta. Can. J. Zool. 55, 1038–1047. doi: 10.1139/z77-132
Kvist A., Lindstrom A. (2000). Maximum daily energy intake: it takes time to lift the metabolic ceiling. Physiol. Biochem. Zool. 73, 30–36. doi: 10.1086/316719
Leggatt R. A., Devlin R. H., Farrell A. P., Randall D. J. (2003). Oxygen uptake of growth hormone transgenic coho salmon during starvation and feeding. J. Fish. Biol. 62, 1053–1066. doi: 10.1046/j.1095-8649.2003.00096.x
Li X. M., Yu L. J., Cao Z. D., Fu S. J., Zhang Y. G. (2013). The effects of exhaustive chasing training on the growth performance and postprandial metabolic response in Spinibarbus sinensis and Procypris rabaudi. Freshwat. Fisher 43, 63–68. doi: 10.3969/j.issn.1000-6907.2013.01.012
Ma H. J., Mou M. M., Pu D. C., Lin S. M., Chen Y. J., Luo L. (2019). Effect of dietary starch level on growth, metabolism enzyme and oxidative status of juvenile largemouth bass, Micropterus salmoides. Aquaculture 498, 482–487. doi: 10.1016/j.aquaculture.2018.07.039
Mayntz D., Raubenheimer D., Salomon M., Toft S., Simpson S. (2005). Nutrient-specific foraging in invertebrate predators. Science 307, 111–113. doi: 10.1126/science.1105493
McCarthy I. D., Houlihan D., Carter C. G. (1994). Individual variation in protein turnover and growth efficiency in rainbow trout, Oncorhynchus mykiss (Walbaum). Proc. R. Soc Lond. B. Biol. Sci. 257, 141–147. doi: 10.1098/rspb.1994.0107
McCarthy I. D., Moksness E., Pavlov D. A., Houlihan D. F. (1999). Effects of water temperature on protein synthesis and protein growth in juvenile Atlantic wolffish (Anarhichas lupus). Can. J. Fish. Aquat. Sci. 56, 231–241. doi: 10.1139/f98-171
McCue M. D. (2006). Specific dynamic action: a century of investigation. Comp. Biochem. Physiol. A. 144, 381–394. doi: 10.1016/j.cbpa.2006.03.011
Messina M., Iacumin L., Pascon G., Tulli F., Tibaldi E., Cardinaletti G. (2023). Effect of feed restriction and refeeding on body condition, digestive functionality and intestinal microbiota in rainbow trout (Oncorhynchus mykiss). Fish. Physiol. Biochem. 49, 169–189. doi: 10.1007/s10695-023-01170-z
Miyasaka H., Kawaguchi Y., Genkai-Kato M., Yoshino K., Ohnishi H., Kuhara N., et al. (2005). Thermal changes in the gastric evacuation rate of the freshwater sculpin Cottus nozawae Snyder. Limnology 6, 169–172. doi: 10.1007/s10201-005-0158-5
Moffatt K., Rossi M., Park E., Svendsen J. C., Wilson J. M. (2022). Inhibition of gastric acid secretion with omeprazole affects fish specific dynamic action and growth rate: Implications for the development of phenotypic stomach loss. Front. Physiol. 13, 966447. doi: 10.3389/fphys.2022.966447
Monentcham S. E., Pouomigne V., Kestemont P. (2010). Influence of dietary protein levels on growth performance and body composition of African bonytongue fingerlings Heteriostis niloticus (Cuvier 1829). Aquacult. Nutr. 16, 144–152. doi: 10.1111/j.1365-2095.2008.00646.x
Moran D., Turner S. J., Clements K. D. (2005). Ontogenetic development of the gastrointestinal microbiota in the marine herbivorous fish Kyphosus sydneyanus. Microb. Ecol. 49, 590–597. doi: 10.1007/s00248-004-0097-4
Naik M. K., Reddy H., Annappaswamy T. S. (2000). Influence of diet composition, starvation and feeding frequency on gastric evacuation rates in Catla, Catla Catla (Hamilton) fingerlings. Indian J. Anim. Sci. 70, 1090–1093.
Pääkkönen J.-P. J., Myyrä R., Marjomäki. T. J. (2010). The effect of meal size on the rate of gastric evacuation of burbot, Lota lota (L.). Ecol. Freshw. Fish. 8, 49–54. doi: 10.1111/j.1600-0633.1999.tb00052.x
Pang X., Tan G., Sun H., Shi H. Y., Su S. Q., Li Y., et al. (2022). Effect of feeding different diets on postprandial metabolic response, digestive capacity and growth performance in juvenile southern catfish (Silurus meridionalis). Aquacult. Rep. 27, 101413. doi: 10.1016/j.aqrep.2022.101413
Párrizas M., Planas J., Plisetskaya E. M., Gutiérrez. J. (1994). Insulin binding and receptor tyrosine kinase activity in skeletal muscle of carnivorous and omnivorous fish. Am. J. Physiol. R. 266, 1944–1950. doi: 10.1152/ajpregu.1994.266.6.R1944
Pérez-Casanova J. C., Lall S. P., Gamperl A. K. (2009). Effect of feed composition and temperature on food consumption, growth and gastric evacuation of juvenile Atlantic cod (Gadus morhua L.) and haddock (Melanogrammus aeglefinus L.). Aquaculture 294, 228–235. doi: 10.1016/j.aquaculture.2009.06.005
Persson L. (1981). The effects of temperature and meal size on the rate of gastric evacuation in perch (Perca fluviatilis) fed on fish larvae. Freshw. Biol. 11, 131–138. doi: 10.1111/j.1365-2427.1981.tb01249.x
Raubenheimer D., Simpson S. J. (1997). Integrative models of nutrient balancing: application to insects and vertebrates. Nutr. Res. Rev. 10, 151–179. doi: 10.1079/NRR19970009
Reenstra W. W., Forte J. G. (1981). H+/ATP stoichiometry for the gastric (K++H+)-ATPase. J. Membr. Biol. 61, 55–60. doi: 10.1007/BF01870752
Rong H., Zhang L., Wang X. W., Wu X. W., Wang J. H., Hu Q., et al. (2023). Comparative study on digestive enzyme activity and intestinal tissue morphology of four fishes with different feeding habits. Freshw. Fisher. 53, 29–35. doi: 10.13721/j.cnki.dsyy.2023.02.008
Sakyi M. E., Cai J., Tang J., Abarike E. D., Jian J. (2020). Effects of starvation and subsequent re-feeding on intestinal microbiota, and metabolic responses in Nile tilapia, Oreochromis niloticus. Aquacul. Rep. 17, 100370. doi: 10.1016/j.aqrep.2020.100370
Secor S. M. (2009). Specific dynamic action: a review of the postprandial metabolic response. J. Comp. Physiol. B. 179, 1–56. doi: 10.1007/s00360-008-0283-7
Smith R. L., Paul J. M., Paul A. J. (2011). Gastric evacuation in walleye pollock, Theragra chalcogramma. Can. J. Fish. Aquat. Sci. 46, 489–493. doi: 10.1139/f89-065
Speakman J. R., Król E. (2005). Limits to sustained energy intake ix: a review of hypotheses. J. Comp. Physiol. B. 175, 375–394. doi: 10.1007/s00360-005-0013-3
Stevens E. D., Wagner G. N., Sutterlin A. (1999). Gut morphology in growth hormone transgenic Atlantic salmon. J. Fish. Biol. 55, 517–526. doi: 10.1111/j.1095-8649.1999.tb00696.x
Storebakken T., Austreng E. (1987). Ration level for salmonids growth, survival, body composition, and feed conversion in Atlantic salmon fry and fingerlings. Aquaculture 60, 189–206. doi: 10.1016/0044-8486(87)90287-0
Sugita H., Shibuya K., Shimooka H., Deguchi Y. (1997). Antibacterial abilities of intestinal bacteria in freshwater cultured fish. Aquaculture 145, 195–203. doi: 10.1016/S0044-8486(96)01319-1
Sveier H., Wathne E., Lied E. (1999). Growth, feed and nutrient utilisation and gastrointestinal evacuation time in Atlantic salmon (Salmo salar L.): the effect of dietary fish meal particle size and protein concentration. Aquaculture 180, 265–282. doi: 10.1016/S0044-8486(99)00196-9
Tuene S., Nortvedt R. (1995). Feed intake, growth and feed conversion efficiency of Atlantic halibut, Hippoglossus hippoglossus (L.). Aquacult. Nutr. 1, 27–35. doi: 10.1111/j.1365-2095.1995.tb00032.x
Wang S., Li E. C., Yu Q. R., Luo Z. Y., Li W., Wang X. D., et al. (2024). Supplementation of yeast culture to low-fishmeal diets improves growth, intestinal health, and heat stress resistance in juvenile Chinese mitten crab (Eriocheir sinensis). Aquaculture 593, 741308. doi: 10.1016/j.aquaculture.2024.741308
Wang C. Q., Luo X., Zhang Y. X., Zhou Y., Xiao Q. W., Huang X., et al. (2023). Triploidization modulates intestinal microbiota and promotes growth in Carassius auratus. Aquaculture 571, 739480. doi: 10.1016/j.aquaculture.2023.739480
Wu B. L., Huang L., Chen J., Zhang Y., Chen X. J., Wu C. C., et al. (2021). Effects of feeding frequency on growth performance, feed intake, metabolism and expression of fgf21 in grass carp (Ctenopharyngodon idellus). Aquaculture 545, 737196. doi: 10.1016/j.aquaculture.2021.737196
Xie X. J., Sun R. Y. (1992). Maximum ration level in the southern catfish (Silurus meridionalis Chen) in relation to body weight and temperature. Acta Ecol. Sin. 12, 225–231. doi: 10.1111/j.1095-8649.1992.tb02619.x
Keywords: freshwater fishes, gastric evacuation rate, intestinal microbiota, nutritional physiology, specific dynamic action, specific growth rate
Citation: Pang X, Yang J, Xiang S-A, Sun H and Fu S-J (2024) Interspecific differences in growth, digestion, and feeding metabolism among freshwater fishes with different food habits. Front. Mar. Sci. 11:1490406. doi: 10.3389/fmars.2024.1490406
Received: 03 September 2024; Accepted: 29 October 2024;
Published: 25 November 2024.
Edited by:
Yafei Duan, South China Sea Fisheries Research Institute, ChinaReviewed by:
Basanta Kumar Das, Central Inland Fisheries Research Institute (ICAR), IndiaCopyright © 2024 Pang, Yang, Xiang, Sun and Fu. This is an open-access article distributed under the terms of the Creative Commons Attribution License (CC BY). The use, distribution or reproduction in other forums is permitted, provided the original author(s) and the copyright owner(s) are credited and that the original publication in this journal is cited, in accordance with accepted academic practice. No use, distribution or reproduction is permitted which does not comply with these terms.
*Correspondence: Shi-Jian Fu, c2hpamlhbmZ1OUBob3RtYWlsLmNvbQ==
Disclaimer: All claims expressed in this article are solely those of the authors and do not necessarily represent those of their affiliated organizations, or those of the publisher, the editors and the reviewers. Any product that may be evaluated in this article or claim that may be made by its manufacturer is not guaranteed or endorsed by the publisher.
Research integrity at Frontiers
Learn more about the work of our research integrity team to safeguard the quality of each article we publish.