- 1South China Sea Ecological Center of Ministry of Natural Resources (MNR), Nansha Islands Coral Reef Ecosystem National Observation and Research Station, & Key Laboratory of Marine Environmental Survey Technology and Application of MNR, Guangzhou, China
- 2Key Laboratory of Tropical Marine Bio-resources and Ecology, South China Sea Institute of Oceanology, Chinese Academy of Sciences, Guangzhou, China
- 3Guangdong Provincial Key Laboratory of Applied Marine Biology, South China Sea Institute of Oceanology, Chinese Academy of Sciences, Guangzhou, China
- 4Polar Research Institute of China, Ministry of Natural Resources (MNR), Shanghai, China
The behavior and influence mechanisms of trace elements in Antarctic ecosystems are particularly complex and important. This study aims to assess the ecological risk to the Fildes Peninsula by systematically analyzing the distribution of trace elements, and provides a scientific basis for the protection of the Antarctic ecosystem. Trace element concentrations in the soils of the Fildes Peninsula are highest for Fe, Al, and Mg, with notable differences in distribution due to varying topographies and landscapes. Principal component analysis reveals strong correlations among Zn, Fe, Mn, Co, and Cu, and distinct patterns for Na, Ca, As, and Cr. Moss demonstrates a high enrichment capacity for Cu and Zn, often accumulating them to higher levels than soil, with significant spatial variation observed. The bioaccumulation factors (BAF) for K and Ag exceed 1 at all 12 stations, indicating a high capacity for bioaccumulation. Over half of the stations show BAF values greater than 1 for Na, Ca, and Cd, while the other trace elements have BAF values greater than 1 at only limited stations. The geo-accumulation index (Igeo) shows most trace elements in the Fildes Peninsula soils are unpolluted, with Mg, Mn, Cr, and Ni concentrations varying between unpolluted and moderate pollution, and all potential ecological risk index (PERI) values under 150 indicating low ecological risk.
1 Introduction
Trace elements are those that are present at extremely low concentrations in the environment but are essential to the functioning of living organisms and ecosystems (Pan and Wang, 2012). These elements may enter the environment either through natural processes, such as rock weathering, volcanic eruptions or through anthropogenic activities, such as industrial emissions, agricultural activities, and global atmospheric and marine pollution transmission (Ali et al., 2019). Despite their low concentrations in the environment, trace elements may be progressively transferred to all trophic levels of the ecosystem through bioaccumulation and trophic transfer, with profound effects on organism health and ecosystem function (DeForest and Meyer, 2015; Majer et al., 2014; Wang, 2013). Total mercury (THg) and methylmercury (MeHg) were found to biomagnify through the Arctic marine food web (Campbell et al., 2005), and Hg biomagnification through food webs was highest in cold and low productivity systems (Lavoie et al., 2013). Arsenic could also show biomagnification potential in particular coast ecosystems (Du et al., 2021; Huang, 2016).
With global environmental change and the gradual expansion of human activities into the most remote parts of the Earth, Antarctica, as the most pristine continent on Earth and undisturbed by large-scale direct human interference, has become an important area for studying the transport, deposition and bioaccumulation of global pollutants in extreme environments (Fastelli and Renzi, 2019; Perfetti-Bolaño et al., 2022; Thompson et al., 2011; Trevizani et al., 2022; Waller et al., 2017). Over the past few decades, the Antarctic ecosystems has been dramatically altered by human activities, including pollutant transport, scientific research, fishing and tourism (Pritchard et al., 2012; Tin et al., 2009; Vaughan et al., 2003). In the Antarctic region, the behavior of trace elements is particularly complex and important (Gran-Scheuch et al., 2020; Koppel et al., 2020). On the one hand, Antarctica is far from major industrial areas, and natural inputs of pollutants (volcanic activity, wind erosion, dust, etc.) are limited. On the other hand, extreme climatic conditions, such as low temperatures, high wind speeds, prolonged ice and snow cover, and unique light patterns, allow pollutants to persist significantly longer in the Antarctic ecosystem, and their migration and transformation paths in the environment may differ from those in other regions (Szopińska et al., 2018). These characteristics make the study of trace element ecotoxicology a very challenging in the field of environmental science.
In recent years, with the intensification of global climate change and the expansion of human activities, the Antarctic region has faced increasing environmental pressure (Chu et al., 2019; Joju et al., 2024; Xu et al., 2020). Under the long-term global transmission of atmospheric and marine pollution, trace elements may gradually accumulate in the Antarctic ecosystem, thus posing a threat to local biodiversity and ecosystem stability (Signa et al., 2019; Yin et al., 2006). In addition, as glaciers melt due to a warming climate, they may further release pollutants deposited in ice, increasing the potential threat of trace elements to the Antarctic ecosystem. Studies have reported elevated trace elements concentrations in the Antarctic environment due to human activity, which could pose a threat to the Antarctic flora and fauna (Lu et al., 2012; Padeiro et al., 2016).
Mosses constitute one of the main plant groups in the extremely cold environment of Antarctica. They are adapted to extremely low temperatures and arid environments and can survive in harsh conditions (Fabri-Jr et al., 2018). These mosses help maintain soil structure and stability, providing habitats for other organisms (Bhakta et al., 2022; Fabri-Jr et al., 2018). Their growth patterns and chemical composition can reflect past climate conditions, helping scientists understand the impact of climate change on polar ecosystems (Bhakta et al., 2022). Moss can absorb nutrients and water directly from the environment and is a good way to reflect the contamination of trace elements in the environment. Antarctica is one of the most protected ecological areas on Earth and is extremely sensitive to environmental changes (Ogaki et al., 2020). As an important part of the ecosystem, the health of mosses is directly related to the stability of the entire Antarctic ecosystem (Carvallo et al., 2021). Higher trace element contents of Cd, Hg and As were found in bryophytes (Bhakta et al., 2022). Ornithogenic soils, along with moss samples, have proven to be effective bioindicators for environmental monitoring in Antarctica, particularly in regions where human activities may contribute significantly to potential contamination (Alekseev and Abakumov, 2021).
The Fildes Peninsula is located southwest of King George Island in Antarctica, and several national scientific research stations on the island. This area provides an ideal natural laboratory for the study of trace elements in the polar environment because of its unique geographical location, complex climate characteristics and fragile ecosystems. The aim of this study was to character the distribution, transfer and bioaccumulation of trace elements in both environment and moss from the Antarctic ecosystem, as well as their potential ecological risks.
2 Materials and methods
2.1 Sampling sites
The Fildes Peninsula is the largest ice–free area on King George Island. The lack of sunshine, short growing season and other factors severely limit the growth of land plants here, so there are almost no higher plants on the peninsula. The relatively warm and humid climate makes the terrestrial vegetation mainly composed of moss and lichen, and the moss is mainly distributed in the coastal areas with flat terrain, weak freeze–thaw effects and relatively stable surfaces. There are seal colonies on the western side of the peninsula, and Adderley Island on the eastern side of the peninsula is home to the largest penguin colony in the South Shetland Islands (Celis et al., 2023). Moss from 35 stations, soil samples from 21 stations were collected in January 2023; these samples were roughly evenly distributed in Fildes Peninsula (Figure 1).
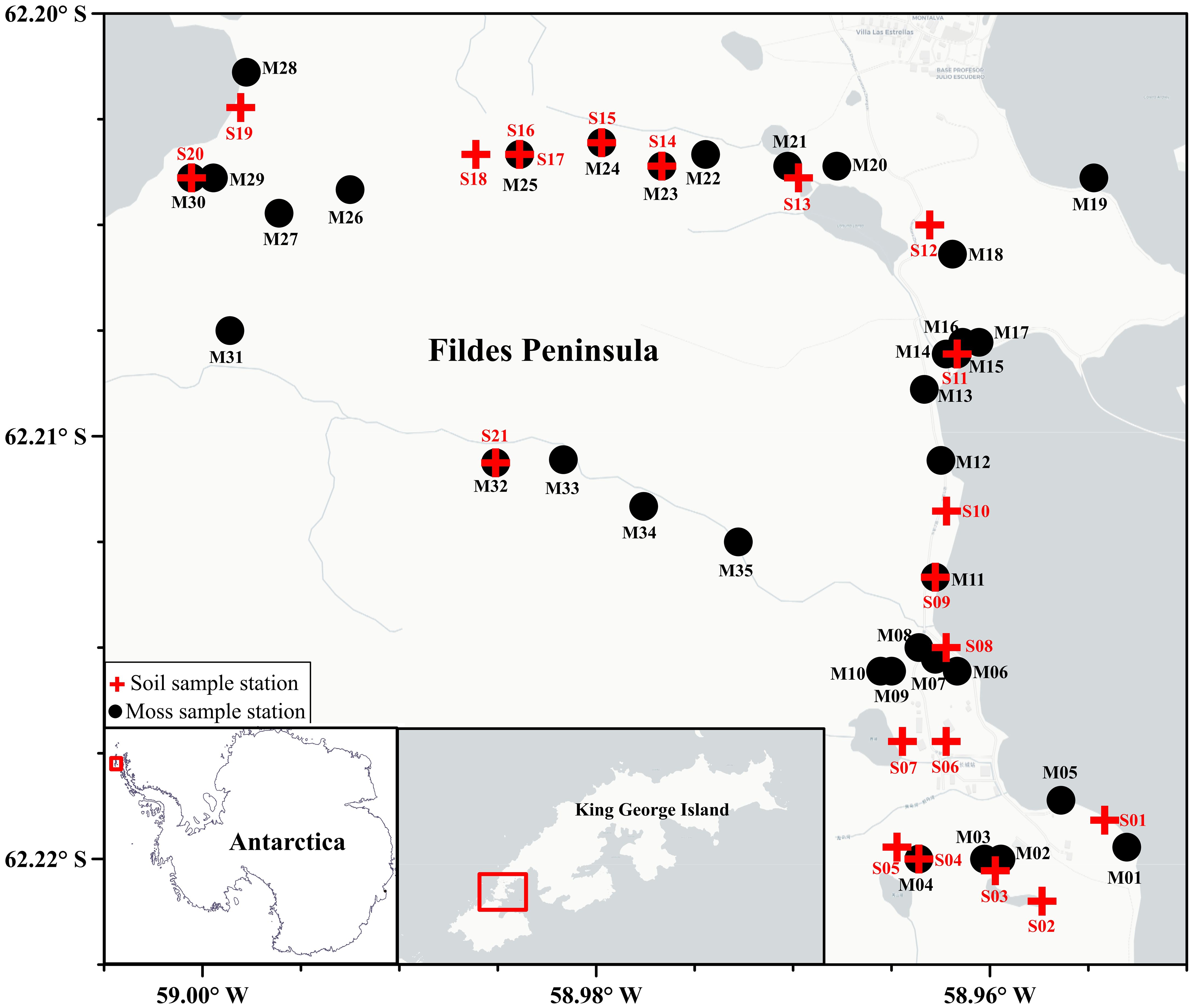
Figure 1. Map of sample stations on the Fildes Peninsula, Antarctic. The solid black circle indicates that the moss samples were collected at the station. The red crosses indicate that the soil samples were collected at this station.
2.2 Sampling
Moss was collected via polythene spades. After being brought back to the laboratory using polyethylene sealing bags, the moss samples were carefully shaken to remove adhering materials, and then rinsed with distilled water to remove small sediment particles. Each moss sample was sealed in a sterile and sealed plastic bag. Moss samples were finally freeze–dried, ground with a mortar, and sieved with nylon filters for trace element analysis.
Soil was collected from the top layer using a shovel. Soil samples were freeze–dried at -80°C, with debris such as gravel removed. The samples were ground using an agate mortar and pestle, then sieved through a 160-mesh nylon sieve (with an approximate aperture of 0.01 mm). About 30 g of the prepared sample was quartered and bagged for storage in a -20°C refrigerator as the sample to be tested.
2.3 Trace element analysis
Approximately 0.2 g of dry moss sample was weighed and transferred to a polytetrafluoroethylene digestion tank. First, 2 mL of hydrogen peroxide (Merck) were added and allowed to stand overnight for pre-digestion to prevent excessive reaction. Following this, 5 mL nitric acid (Merck) were added. The digestion process was carried out using a microwave oven (Milestone Ethos 1600). All analyses were performed in triplicate. Quality control was ensured by analyzing a standard material for kelp composition (GBW08517). 0.5 g soil sample was accurately weighed and placed in a polytetrafluoroethylene digestion tank of a microwave digester. To the tank, 6 mL nitric acid, 2 mL perchloric acid, and 2 mL of hydrofluoric acid were added. The mixture was subjected to closed digestion in the CEM microwave system at 200°C for 1 h. After digestion, the sample was cooled to room temperature and then opened. Next, 5 mL boric acid were added to the digested solution to neutralized any residual hydrofluoric acid. The solution was then heated and concentrated to about 1 mL. The contents of digestion tank were rinsed with ultra–pure water. The solution was transferred to a 25 mL tube, and then store it at room temperature until measurement. Quality control was conducted using the certified reference material of offshore marine sediment (GBW07314). The concentrations of trace elements (Na, Mg, Al, K, Ca, Mn, Fe, Cr, Co, Ni, Cu, Zn, As, Se, Ag, Cd and Pb) were determined using inductively coupled plasma mass spectrometry (ICP-MS, Agilent 7900). Internal standards (i.e., 45Sc, 74Ge, 115In, and 209Bi) were selected to correct the sensitivity drift and matrix effects. The quality control samples were analyzed every 20 samples. The measured recovery rate of trace elements for the certified reference was 92.4~109.3%.
2.4 Risk assessment
The bioaccumulation factor (BAF) is used to measure the bioaccumulation capacity of trace elements in moss from soils via the following equation:
where Co is the trace element concentration of moss (μg/g) and CS is the trace element concentration of soils (μg/g). BAF > 1 indicates that the moss has a relatively high bioaccumulation capacity. Conversely, BAF < 1 indicates that the moss has a weak ability to accumulate trace elements or that it may have a strong ability to exclude trace elements.
Geo-accumulation index (Igeo) is widely used in the evaluation of trace element pollution in soils. The index determines the level of trace element pollution in soils by comparing the concentrations of trace elements in actual soils with their geological background values. It was computed via the following equation:
where CS and Cb are the concentrations of trace elements obtained from field measurements and their associated background concentrations (μg/g), respectively. Igeo ≤0 indicates unpolluted; 0< Igeo ≤1 indicates unpolluted to moderate pollution; and 1< Igeo ≤2 indicates moderate pollution. The background concentrations in the soils from the Fildes Peninsula were determined via the data of Lu et al. (2012).
The potential ecological risk index (PERI) is a comprehensive indicator used to assess the potential harm to ecosystems from trace element pollution in sediments or soils. PERI not only considers the degree of contamination of trace elements, but also combines the toxicity and environmental sensitivity of trace elements, which is a relatively comprehensive ecological risk assessment tool. Its calculation equation is as follows:
where Cfi is the contamination factor for i, Eri is the potential ecological risk factor for each trace element, and Tri is the toxic–response factor of trace element i. The Tri values for Cr, Ni, Cu, Zn, As, Cd and Pb are 2, 5, 5, 1, 10, 30 and 5, respectively (Hakanson, 1980).
2.5 Statistical analysis
Statistical analysis was performed via SPSS 20.0. The distribution of trace element in the Fildes Peninsula was performed via surfer. Principal component analysis (PCA) is a dimensionality reduction technique used to identify the main source of trace elements in a variable in a soil or moss sample.
3 Results and discussion
3.1 Trace element analysis of soils in the Fildes Peninsula
The trace element concentrations of the soils from the Fildes Peninsula in descending order are Fe, Al, Mg, Ca, Na, K, Mn, Cu, Zn, Co, Cr, Ni, As, Se, Pb, Cd, and Ag (Figure 2). Na, Ca and Al had high values on the west coast (Supplementary Figure S1). The topography of the Fildes Peninsula is complex, and different topographies and landscapes (such as hillsides, plains, lakes, river valleys, etc.) have different effects on the accumulation and migration of trace elements. Soils in valley areas (S13~S18) present relatively high concentrations of trace elements probably due to pooling and sedimentation effects. The trace element concentrations of soils in this study were not significantly higher than the previous studies (Supplementary Table S2).
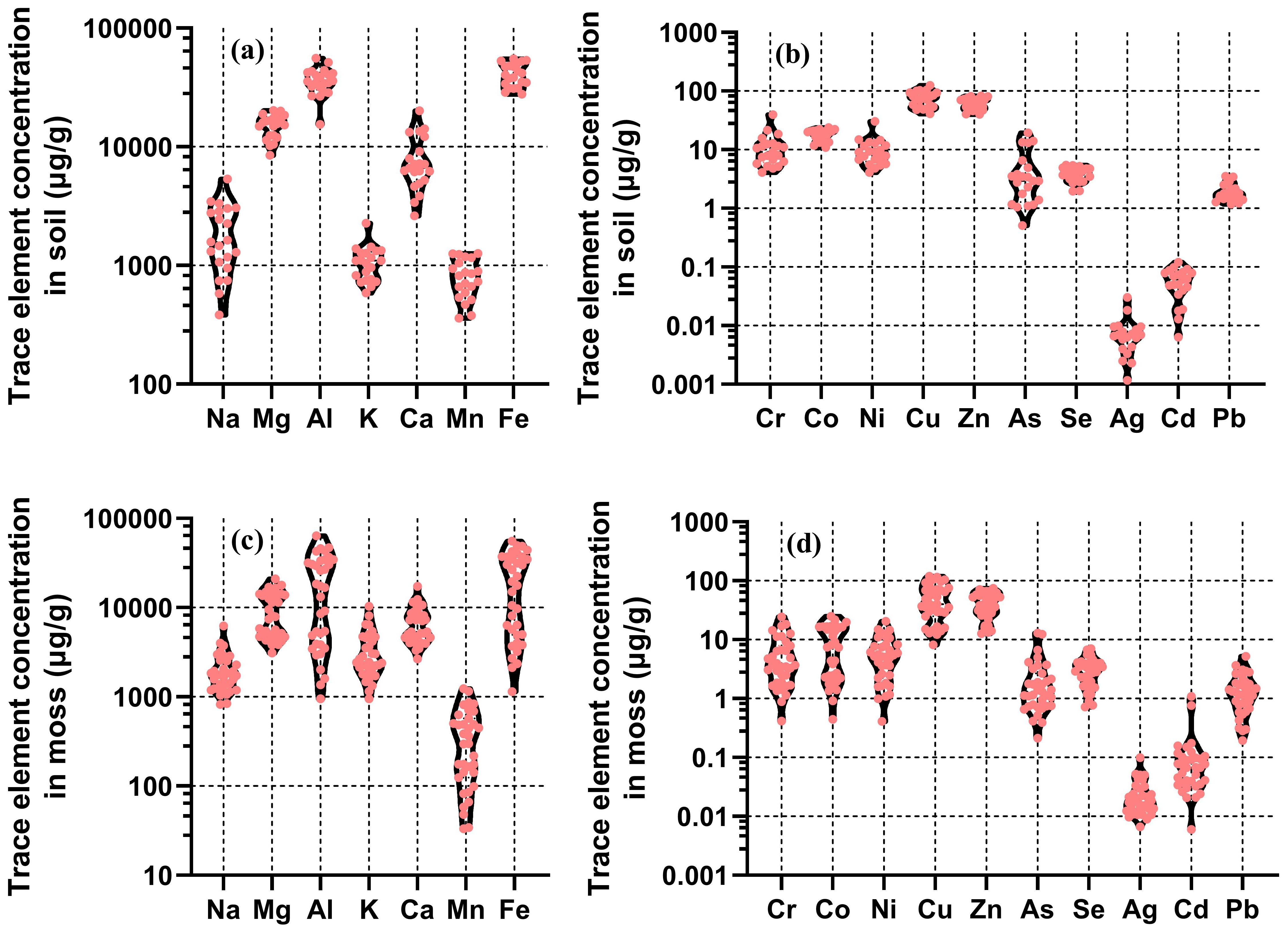
Figure 2. Violin plot of trace element concentrations in soil (A, B) and moss (C, D) from the Fildes Peninsula, Antarctic. All points are shown in the plot.
Principal component analysis (PCA) of the trace elements in the soils from the Fildes Peninsula (Figure 3) revealed that the X-axis explained 46.2% of the total variation, which represented the maximum variation in the trace elements in the soil samples. The Y-axis explains 20.1% of the total variation. There can be strong correlations between Zn, Fe, Mn, Co, and Cu. Stations of S13, S14 and S21 have similar trace element compositions mentioned above. Al, Fe, and Mn are shown to be crustal-derived, and Na, Mg, K, and Ca originated depends on the distance from the sea identified by Antarctic snows (Boutron and Martin, 1980). S16 and S17 are relatively independent, indicating that their trace element characteristics are significantly different from those of the other samples and that they may have higher Cu concentrations. As and Cr have different distribution trends, as do Ni and Ag. Na and Ca are different from those of the other elements, and S19 and S20 may be rich in Na and Al. S03 is further removed from the other samples, which indicates a unique trace element composition compared with that of the other samples. The distributions of Cr, Cu, and Zn are linked to weathering processes, while the distributions of As and Pb are related to vehicle usage and unloading activities at the wharfs on Mirror Peninsula (Xu et al., 2020).
Trace elements generally show significant spatial differences in the soils of the Fildes Peninsula (Slukovskii and Guzeva, 2024). Trace elements tend to be found in soils near areas of intense human activity, such as research stations, airports and fuel storage facilities (Chu et al., 2019). There are several research stations on the Fildes Peninsula (such as the Great Wall Station, Bellings-Goggin Station, etc.), and these research stations and their ancillary facilities (such as fuel storage and power generation equipment) are among the main sources of trace element pollution. Scientific research activities, vehicle emissions, and improper waste disposal all increase the concentrations of trace elements in the soil. The highest levels of trace elements were found for Cr, Cu, Ni and Zn, which were well above baseline levels at two sites located near previously identified contamination sources in the Fildes Peninsula (Pereira et al., 2017). Historical issues such as past fuel use (such as leaded gasoline), waste, and improper wastewater treatment can also lead to the accumulation of trace elements in the soil. Long–distance air pollutants (such as industrial emissions and trace element particles from fuel combustion) can fall on the Fildes Peninsula through atmospheric sedimentation (Reindl et al., 2024). The presence of penguins increases Cu concentrations in Antarctic soils (Perfetti-Bolaño et al., 2018). Seabirds can also biotransport Cu, Pb, and Zn from the ocean to the Antarctic continent, and Cu is the trace metal most biotransported by seabirds (Castro et al., 2021). Although Antarctica is far from pollution sources, wind and air currents can carry pollutants to the region, leading to local enrichment of trace elements in the soil. The Ardley Cove area on the Fildes Peninsula, Antarctica, has significant human impact with contamination hotspots for Zn, Pb, Cd, Cr, and Ni, as confirmed by enrichment factors and sequential extractions, highlighting the need for contamination control and remediation (Padeiro et al., 2016).
3.2 Trace element analysis of mosses on the Fildes Peninsula
Moss has a strong enrichment capacity for some trace elements, especially Cu and Zn (Figure 2), which may be enriched to a greater degree in moss than in soil. Like the distribution of trace elements in soil, the concentration of trace elements in moss also shows significant spatial differences. The distribution trend of Mg, Mn, Fe, Co, Cu, Zn and Se was similar, which the highest concentration in the middle (M32~M35) and the lower on both sides. The concentrations of Pb in moss are high among fuel storage facilities areas. Figure 4 shows the results of the principal component analysis of trace elements in mosses on the Fildes Peninsula. The X-axis explains 80.9% of the total variation. Y-axis, explaining 5.8% of the total variation. There may be a correlation between K and Ag, indicating that these elements are enriched together in the same or similar samples. Cd has a uniquely high concentration associated with the first principal component direction. M02 and M09 have a higher Cd concentration in these samples. The more likely sources of Cd appear to be marine aerosols, seabird guano, and volcanic emissions (Bargagli et al., 1998). Na and Ca have different distributions than most other trace elements. Fuel combustion, accidental oil spills, waste incineration and sewage disposal are amongst the primary sources of heavy metal contaminants in Antarctica, besides natural sources such as animal excrements and volcanism (Chu et al., 2019). The distributions of Al, Mg, Fe, Cu, Zn, Mn, Ni, Cr, Se, As, Pb and other trace elements are similar (Supplementary Figure S2), indicating that these trace elements are enriched in some samples. In addition to atmospheric deposition, the primary pathway for metals to enter mosses is likely through evapotranspiration at their surface, which facilitates the upward migration of ions and their subsequent bioaccumulation (Bargagli et al., 1998).
Moss samples and corresponding soil samples were collected simultaneously at 12 stations. The bioaccumulation factor of the moss samples (Figure 5) revealed that the BAFs of K and Ag at all 12 stations were greater than 1, which indicates a high bioaccumulation capacity. Mosses typically have a high surface area and an abundance of adsorption sites, so they can efficiently adsorb and accumulate trace elements from the environment. More than half of the stations have BAF values of Na, Ca, and Cd greater than 1, and the other trace elements have BAF values greater than 1 at only a few stations. The metal bioaccumulation in the mosses is mainly influenced by the altitude, urban emissions and the species specific accumulation capacities of the different sampled moss species (Holy et al., 2009). The concentrations of Cr, Cu, Ni, Zn, and V in soil, lichens, and moss at Fildes Peninsula, Antarctica, may be elevated due to intense human activities, including fossil fuel use and scientific station operations (Fabri-Jr et al., 2018). The soil pH, redox state and soil mineral composition may affect the availability of trace elements and the enrichment efficiency of moss (Lazo et al., 2018; Wang et al., 2019). Despite most contamination being in anthropogenically affected areas, elevated soil pollution indices in natural soils were also linked to higher element accumulation in guano–derived and moss materials (Alekseev and Abakumov, 2021). Stations away from human activity, on the other hand, may mainly reflect natural background levels and therefore have a lower BAF (Liu et al., 2018). Different stations may have different types of mosses or different physiological states, although mosses that look the same may have different abilities to absorb and accumulate trace elements due to different growth conditions. In addition, physiological characteristics such as the growth density and health status of moss also affect the BAF. In general, the differences in BAF among different stations provide a better way to understand trace element pollution in the Antarctic region and help further explore the sources, transmission routes and ecological impacts of pollution.
3.3 Trace element risk assessment
The geo-accumulation index indicated that most trace elements were unpolluted in the Fildes Peninsula (Figure 6). The Mg concentration was between unpolluted and moderate pollution. The Mn, Cr, and Ni concentrations at some stations were also between unpolluted and moderate pollution. The PERIs of all the stations were less than 150, which means that the trace element concentrations in the soils posed a low ecological risk. Trace element levels in the South Ocean ecosystem of Antarctica over the past 1500 years have revealed a sharp increase in lead since the late 1800s due to industrial contamination, followed by a subsequent decline after the 1980s from reduced leaded gasoline use, whereas copper levels vary with weathering and climate, and cadmium, arsenic, and zinc show no clear temporal trends (Yin et al., 2006). Metal concentrations analysis of surface soils on the Fildes Peninsula, King George Island, Antarctica, revealed significant anthropogenic contamination with lead, cadmium, and mercury, while showing lower magnesium and nickel levels but higher copper levels than those in adjacent areas, on the basis of cumulative frequency, enrichment factors, and principal component analysis (Lu et al., 2012). Using the geo-accumulation index, all the pristine soils of the King George and Elephant Islands and ornithogenic soils of the Fildes Peninsula were classified as unpolluted, and the human–affected soils were identified mainly as moderately polluted (Abakumov et al., 2017).
4 Conclusion
This research explores the presence of trace elements and their potential ecological impacts in the Fildes Peninsula, Antarctica. Fe, Al, and Mg are the most abundant trace elements, with their distribution affected by the area’s diverse topographies and microenvironments. Principal component analysis highlights significant correlations between Zn, Fe, Mn, Co, and Cu, while some stations, like S16 and S17, exhibit distinctive trace element profiles, including elevated Cu levels. Additionally, variations in trace element patterns are observed for Na, Ca, As, and Cr across different locations.
Moss in the studied area exhibits a remarkable ability to enrich trace elements, especially Cu and Zn, often accumulating them at concentrations exceeding those in the soil. This enrichment exhibits significant spatial variability. Principal component analysis reveals a strong association between K and Ag, elevated Cd levels in specific samples, and generally consistent enrichment trends for numerous trace elements. Furthermore, bioaccumulation factors for K and Ag surpass 1 at all 12 stations, indicating a high capacity for bioaccumulation in moss.
According to the geo-accumulation index, most trace elements in the soils of the Fildes Peninsula are not polluted, with Mg, Mn, Cr, and Ni showing concentrations that range from unpolluted to moderately polluted. Additionally, all PERI values are below 150, reflecting a low level of ecological risk across the area.
Data availability statement
The original contributions presented in the study are included in the article/Supplementary Material. Further inquiries can be directed to the corresponding authors.
Author contributions
ZN: Conceptualization, Funding acquisition, Investigation, Project administration, Resources, Supervision, Writing – review & editing, Data curation. XC: Writing – review & editing, Data curation, Formal analysis, Methodology, Software. SD: Writing – review & editing, Formal analysis, Validation, Methodology, Software, Visualization, Writing – original draft. CL: Writing – review & editing, Data curation, Formal analysis, Project administration, Supervision, Validation. GL: Writing – review & editing, Investigation, Resources, Supervision. PZ: Writing – review & editing, Formal analysis, Methodology, Resources, Supervision. WMD: Writing – review & editing, Investigation. LQ: Writing – review & editing, Investigation. CC: Writing – review & editing, Data curation, Formal analysis. WD: Writing – review & editing, Formal analysis. AD: Writing – review & editing, Data curation.
Funding
The author(s) declare that financial support was received for the research, authorship, and/or publication of this article. This work was supported by the Director’s Foundation of South China Sea Bureau of Ministry of Natural Resources (230201), National Key Research and Development Program of China (2022YFC3102001), the Science and Technology Planning Project of Guangdong Province, China (2023B1212060047), Hanjiang Estuary–Nanao Island Marine Ecosystem Observation and Research Station (2021B1212050025).
Acknowledgments
The authors would like to thank the 39th Chinese National Antarctic Research Expedition by Ministry of Natural Resources and also appreciate the expedition team members in the Chinese Antarctic Great Wall Station for their help with sampling.
Conflict of interest
The authors declare that the research was conducted in the absence of any commercial or financial relationships that could be construed as a potential conflict of interest.
Publisher’s note
All claims expressed in this article are solely those of the authors and do not necessarily represent those of their affiliated organizations, or those of the publisher, the editors and the reviewers. Any product that may be evaluated in this article, or claim that may be made by its manufacturer, is not guaranteed or endorsed by the publisher.
Supplementary material
The Supplementary Material for this article can be found online at: https://www.frontiersin.org/articles/10.3389/fmars.2024.1485062/full#supplementary-material
References
Abakumov E., Lupachev A., Andreev M. (2017). Trace element content in soils of the King George and Elephant islands, maritime Antarctica. Chem. Ecol. 33, 856–868. doi: 10.1080/02757540.2017.1384821
Alekseev I., Abakumov E. (2021). Content of trace elements in soils of eastern Antarctica: variability across landscapes. Arch. Environ. Contam. Toxicol. 80, 368–388. doi: 10.1007/s00244-021-00808-4
Ali H., Khan E., Ilahi I. (2019). Environmental chemistry and ecotoxicology of hazardous heavy metals: Environmental persistence, toxicity, and bioaccumulation. J. Chem. 2019, 6730305. doi: 10.1155/2019/6730305
Bargagli R., Sanchez-Hernandez J. C., Martella L., Monaci F. (1998). Mercury, cadmium and lead accumulation in Antarctic mosses growing along nutrient and moisture gradients. Polar Biol. 19, 316–322. doi: 10.1007/s003000050252
Bhakta S., Rout T. K., Karmakar D., Pawar C., Padhy P. K. (2022). Trace elements and their potential risk assessment on polar ecosystem of Larsemann Hills, East Antarctica. Polar Sci. 31, 100788. doi: 10.1016/j.polar.2022.100788
Boutron C., Martin S. (1980). Sources of twelve trace metals in Antarctic snows determined by principal component analysis. J. Geophysical Research: Oceans 85, 5631–5638. doi: 10.1029/JC085iC10p05631
Campbell L. M., Norstrom R. J., Hobson K. A., Muir D. C. G., Backus S., Fisk A. T. (2005). Mercury and other trace elements in a pelagic Arctic marine food web (Northwater Polynya, Baffin Bay). Sci. Total Environ. 351–352, 247–263. doi: 10.1016/j.scitotenv.2005.02.043
Carvallo C., Godoy N., Aguilar B., Egas C., Fuentealba R., Préndez M. (2021). Long-term monitoring of atmospheric pollution in the Maritime Antarctic with the lichen Usnea aurantiaco-atra (Jacq.) Bory: a magnetic and elemental study. Antarct. Sci. 33, 660–673. doi: 10.1017/S0954102021000419
Castro M. F., Neves J. C. L., Francelino M. R., Schaefer C. E. G. R., Oliveira T. S. (2021). Seabirds enrich Antarctic soil with trace metals in organic fractions. Sci. Total Environ. 785, 147271. doi: 10.1016/j.scitotenv.2021.147271
Celis J. E., Espejo W., Chiang G., Kitamura D., Kashiwada S., O’Driscoll N. J. (2023). Concentration of fifty-six elements in excreta of penguins from the Antarctic Peninsula area. Mar. pollut. Bull. 192, 115133. doi: 10.1016/j.marpolbul.2023.115133
Chu W.-L., Dang N.-L., Kok Y.-Y., Ivan Yap K.-S., Phang S.-M., Convey P. (2019). Heavy metal pollution in Antarctica and its potential impacts on algae. Polar Sci. 20, 75–83. doi: 10.1016/j.polar.2018.10.004
Chu Z., Yang Z., Wang Y., Sun L., Yang W., Yang L., et al. (2019). Assessment of heavy metal contamination from penguins and anthropogenic activities on Fildes Peninsula and Ardley Island, Antarctic. Sci. Total Environ. 646, 951–957. doi: 10.1016/j.scitotenv.2018.07.152
DeForest D. K., Meyer J. S. (2015). Critical review: toxicity of dietborne metals to aquatic organisms. Crit. Rev. Environ. Sci. Technol. 45, 1176–1241. doi: 10.1080/10643389.2014.955626
Du S., Zhou Y., Zhang L. (2021). The potential of arsenic biomagnification in marine ecosystems: A systematic investigation in Daya Bay in China. Sci. Total Environ. 773, 145068. doi: 10.1016/j.scitotenv.2021.145068
Fabri-Jr R., Krause M., Dalfior B. M., Salles R. C., de Freitas A. C., da Silva H. E., et al. (2018). Trace elements in soil, lichens, and mosses from Fildes Peninsula, Antarctica: spatial distribution and possible origins. Environ. Earth Sci. 77, 124. doi: 10.1007/s12665-018-7298-5
Fastelli P., Renzi M. (2019). Exposure of key marine species to sunscreens: Changing ecotoxicity as a possible indirect effect of global warming. Mar. pollut. Bull. 149, 110517. doi: 10.1016/j.marpolbul.2019.110517
Gran-Scheuch A., Ramos-Zuñiga J., Fuentes E., Bravo D., Pérez-Donoso J. M. (2020). Effect of co-contamination by PAHs and heavy metals on bacterial communities of diesel contaminated soils of south shetland islands, Antarctica. Microorganisms 8, 1749. doi: 10.3390/microorganisms8111749
Hakanson L. (1980). An ecological risk index for aquatic pollution control.a sedimentological approach. Water Res. 14, 975–1001. doi: 10.1016/0043-1354(80)90143-8
Holy M., Leblond S., Pesch R., Schröder W. (2009). Assessing spatial patterns of metal bioaccumulation in French mosses by means of an exposure index. Environ. Sci. pollut. Res. 16, 499–507. doi: 10.1007/s11356-009-0146-0
Huang J. H. (2016). Arsenic trophodynamics along the food chains/webs of different ecosystems: a review. Chem. Ecol. 32, 803–828. doi: 10.1080/02757540.2016.1201079
Joju G. S., Warrier A. K., Sali A. S. Y., Chaparro M. A. E., Mahesh B. S., Amrutha K., et al. (2024). An assessment of metal pollution in the surface sediments of an east antarctic lake. Soil. Sediment. Contam 33, 1–22. doi: 10.1080/15320383.2024.2323516
Koppel D. J., King C. K., Brown K. E., Price G. A. V., Adams M. S., Jolley D. F. (2020). Assessing the risk of metals and their mixtures in the antarctic nearshore marine environment with diffusive gradients in thin-films. Environ. Sci. Technol. 54, 306–315. doi: 10.1021/acs.est.9b04497
Lavoie R. A., Jardine T. D., Chumchal M. M., Kidd K. A., Campbell L. M. (2013). Biomagnification of mercury in aquatic food webs: a worldwide meta-analysis. Environ. Sci. Technol. 47, 13385–13394. doi: 10.1021/es403103t
Lazo P., Steinnes E., Qarri F., Allajbeu S., Kane S., Stafilov T., et al. (2018). Origin and spatial distribution of metals in moss samples in Albania: A hotspot of heavy metal contamination in Europe. Chemosphere 190, 337–349. doi: 10.1016/j.chemosphere.2017.09.132
Liu Y., Du G., Mao Y. (2018). Trace Metals Analysis Along the Fildes Peninsula Coastline Using Two Red Algae, Rhodymenia Antarctica and Iridaea cordata, as Monitors. J. Ocean Univ. China 17, 1487–1491. doi: 10.1007/s11802-018-3593-8
Lu Z., Cai M., Wang J., Yang H., He J. (2012). Baseline values for metals in soils on Fildes Peninsula, King George Island, Antarctica: the extent of anthropogenic pollution. Environ. Monit. Assess. 184, 7013–7021. doi: 10.1007/s10661-011-2476-x
Majer A. P., Petti M. A. V., Corbisier T. N., Ribeiro A. P., Theophilo C. Y. S., Ferreira P. A. D. L., et al. (2014). Bioaccumulation of potentially toxic trace elements in benthic organisms of Admiralty Bay (King George Island, Antarctica). Mar. pollut. Bull. 79, 321–325. doi: 10.1016/j.marpolbul.2013.12.015
Ogaki M. B., Vieira R., Muniz M. C., Zani C. L., Alves T. M. A., Junior P. A. S., et al. (2020). Diversity, ecology, and bioprospecting of culturable fungi in lakes impacted by anthropogenic activities in Maritime Antarctica. Extremophiles 24, 637–655. doi: 10.1007/s00792-020-01183-z
Padeiro A., Amaro E., dos Santos M. M. C., Araújo M. F., Gomes S. S., Leppe M., et al. (2016). Trace element contamination and availability in the Fildes Peninsula, King George Island, Antarctica. Environ. Sci.-Process Impacts 18, 648–657. doi: 10.1039/C6EM00052E
Pan K., Wang W. X. (2012). Trace metal contamination in estuarine and coastal environments in China. Sci. Total Environ. 421-422, 3–16. doi: 10.1016/j.scitotenv.2011.03.013
Pereira J. L., Pereira P., Padeiro A., Gonçalves F., Amaro E., Leppe M., et al. (2017). Environmental hazard assessment of contaminated soils in Antarctica: Using a structured tier 1 approach to inform decision-making. Sci. Total Environ. 574, 443–454. doi: 10.1016/j.scitotenv.2016.09.091
Perfetti-Bolaño A., Araneda A., Muñoz K., Barra R. O. (2022). Occurrence and distribution of microplastics in soils and intertidal sediments at fildes bay, maritime Antarctica. Front. Mar. Sci. 8. doi: 10.3389/fmars.2021.774055
Perfetti-Bolaño A., Moreno L., Urrutia R., Araneda A., Barra R. (2018). Influence of pygoscelis penguin colonies on cu and pb concentrations in soils on the ardley peninsula, maritime Antarctica. Water Air Soil pollut. 229, 390. doi: 10.1007/s11270-018-4042-4
Pritchard H. D., Ligtenberg S. R. M., Fricker H. A., Vaughan D. G., van den Broeke M. R., Padman L. (2012). Antarctic ice-sheet loss driven by basal melting of ice shelves. Nature 484, 502–505. doi: 10.1038/nature10968
Reindl A. R., Wolska L., Piotrowicz-Cieślak A. I., Saniewska D., Bołałek J., Saniewski M. (2024). The impact of global climate changes on trace and rare earth elements mobilization in emerging periglacial terrains: Insights from western shore of Admiralty Bay (King George Island, Antarctic). Sci. Total Environ. 926, 171540. doi: 10.1016/j.scitotenv.2024.171540
Signa G., Calizza E., Costantini M. L., Tramati C., Sporta Caputi S., Mazzola A., et al. (2019). Horizontal and vertical food web structure drives trace element trophic transfer in Terra Nova Bay, Antarctica. Environ. pollut. 246, 772–781. doi: 10.1016/j.envpol.2018.12.071
Slukovskii Z. I., Guzeva A. V. (2024). Trace elements in the sediment cores of lakes on king george island, Antarctica. Dokl. Earth Sci. 516, 803–810. doi: 10.1134/S1028334X24600981
Szopińska M., Szumińska D., Bialik R. J., Chmiel S., Plenzler J., Polkowska Ż. (2018). Impact of a newly-formed periglacial environment and other factors on fresh water chemistry at the western shore of Admiralty Bay in the summer of 2016 (King George Island, Maritime Antarctica). Sci. Total Environ. 613-614, 619–634. doi: 10.1016/j.scitotenv.2017.09.060
Thompson D. W. J., Solomon S., Kushner P. J., England M. H., Grise K. M., Karoly D. J. (2011). Signatures of the Antarctic ozone hole in Southern Hemisphere surface climate change. Nat. Geosci 4, 741–749. doi: 10.1038/ngeo1296
Tin T., Fleming Z. L., Hughes K. A., Ainley D. G., Convey P., Moreno C. A., et al. (2009). Impacts of local human activities on the Antarctic environment. Antarct. Sci. 21, 3–33. doi: 10.1017/S0954102009001722
Trevizani T. H., Montone R. C., Figueira R. C. L. (2022). Temporal distribution of arsenic and metals in soil from king george island, Antarctica. Front. Mar. Sci. 8. doi: 10.3389/fmars.2021.772742
Vaughan D. G., Marshall G. J., Connolley W. M., Parkinson C., Mulvaney R., Hodgson D. A., et al. (2003). Recent rapid regional climate warming on the antarctic peninsula. Clim. Change 60, 243–274. doi: 10.1023/A:1026021217991
Waller C. L., Griffiths H. J., Waluda C. M., Thorpe S. E., Loaiza I., Moreno B., et al. (2017). Microplastics in the Antarctic marine system: An emerging area of research. Sci. Total Environ. 598, 220–227. doi: 10.1016/j.scitotenv.2017.03.283
Wang W. X. (2013). Dietary toxicity of metals in aquatic animals: Recent studies and perspectives. Chin. Sci. Bull. 58, 203–213. doi: 10.1007/s11434-012-5413-7
Wang X., Yuan W., Feng X., Wang D., Luo J. (2019). Moss facilitating mercury, lead and cadmium enhanced accumulation in organic soils over glacial erratic at Mt. Gongga, China. Environ. pollut. 254, 112974. doi: 10.1016/j.envpol.2019.112974
Xu Q., Chu Z., Gao Y., Mei Y., Yang Z., Huang Y., et al. (2020). Levels, sources and influence mechanisms of heavy metal contamination in topsoils in Mirror Peninsula, East Antarctica. Environ. pollut. 257, 113552. doi: 10.1016/j.envpol.2019.113552
Keywords: soil, moss, trace elements, Fildes Peninsula, risk assessment
Citation: Ni Z, Chen X, Du S, Lu C, Luo G, Zhou P, Du W, Qi L, Chen C, Deng W and Dang A (2024) The evaluation of trace elements and associated risk in the polar ecosystem of the Fildes Peninsula, Antarctica. Front. Mar. Sci. 11:1485062. doi: 10.3389/fmars.2024.1485062
Received: 23 August 2024; Accepted: 18 September 2024;
Published: 04 October 2024.
Edited by:
Xiutang Yuan, Chinese Academy of Sciences (CAS), ChinaCopyright © 2024 Ni, Chen, Du, Lu, Luo, Zhou, Du, Qi, Chen, Deng and Dang. This is an open-access article distributed under the terms of the Creative Commons Attribution License (CC BY). The use, distribution or reproduction in other forums is permitted, provided the original author(s) and the copyright owner(s) are credited and that the original publication in this journal is cited, in accordance with accepted academic practice. No use, distribution or reproduction is permitted which does not comply with these terms.
*Correspondence: Sen Du, ZHVzZW5Ac2NzaW8uYWMuY24=; Chuqian Lu, bHVjaHVxYW5AMTYzLmNvbQ==; Guangfu Luo, bHVvZ3VhbmdmdUBwcmljLm9yZy5jbg==; Peng Zhou, c2FubXpob3UyQDEyNi5jb20=
†These authors have contributed equally to this work and share first authorship