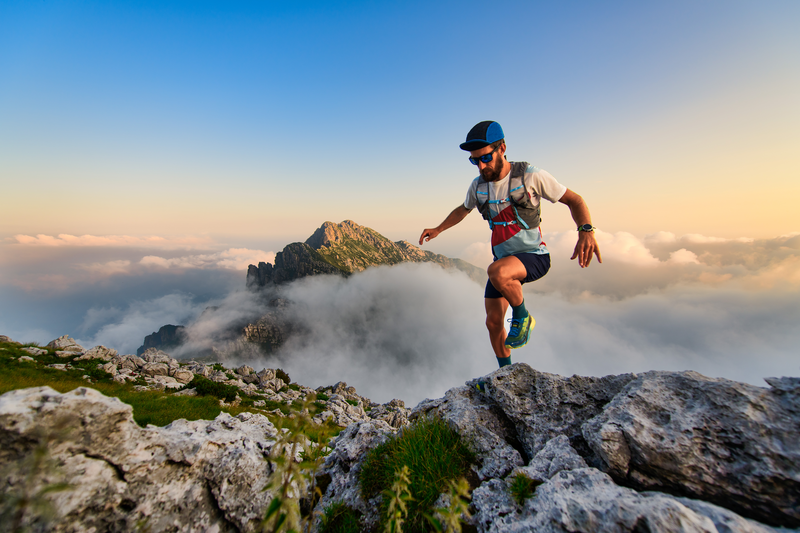
94% of researchers rate our articles as excellent or good
Learn more about the work of our research integrity team to safeguard the quality of each article we publish.
Find out more
ORIGINAL RESEARCH article
Front. Mar. Sci. , 07 January 2025
Sec. Marine Fisheries, Aquaculture and Living Resources
Volume 11 - 2024 | https://doi.org/10.3389/fmars.2024.1473319
This article is part of the Research Topic Towards an Expansion of Sustainable Global Marine Aquaculture View all 13 articles
Introduction: This study evaluates the feasibility of culturing the high-value marine fish Protonibea diacanthus in a polyculture system with Indian pompano (Trachinotus mookalee) using a marine cage culture setup. The study aims to determine growth performance, feed efficiency, and the potential of P. diacanthus as a candidate species for polyculture.
Methods: Wild-caught P. diacanthus were reared in six high-density polyethylene sea cages (Inner Diameter: 6 m; Outer Diameter: 7 m; Depth: 4.5 m; Area: 28.29 m²; Volume: 127.29 m³). Two experimental groups were established: Group-1 with 90 individuals (average weight: 130.15 ± 6.39 g) and Group-2 with 90 individuals (average weight: 287.80 ± 16.90 g). Each group was divided into three cages (30 fish/cage) and reared in triplicate with T. mookalee (2,500 fish/cage, average weight: 31.12 ± 1.17 g). The fish were cultured for 148 days and fed a commercial diet (40% crude protein, 10% crude lipid). Growth indices and feed efficiency indices were analyzed, and growth of the fish was modeled using von Bertalanffy Growth Function (VBGF).
Results: Growth performance indices, including total length gain, body weight gain, and specific growth rate, were significantly higher in Group-1 (P ≤ 0.05). Feed efficiency indices (feed conversion ratio, feed efficiency ratio, and protein efficiency ratio) did not differ significantly between the groups (P > 0.05). VBGF modeling estimated P. diacanthus asymptotic length (L∞) at 164.21 ± 3.58 cm, asymptotic weight (W∞) at 44,070.19 ± 2811 g, growth coefficient (K) at 0.30 ± 0.01 yr−1 and t₀ at -0.005 ± 0.02 yr. The growth performance index (ϕ’) was calculated at 1.91, surpassing values reported for wild populations. Polyculture with P. diacanthus did not significantly affect the growth or feed utilization of T. mookalee (P > 0.05).
Discussion and conclusion: The results indicate that P. diacanthus exhibits superior growth under marine cage culture conditions, with a high growth performance and compatibility in polyculture systems. These findings support the potential of P. diacanthus as a viable candidate for integration into commercial polyculture systems.
Global aquaculture production has been growing at an average annual rate of 5% and, for the first time in 2022, surpassed capture fisheries production, contributing 51% to global aquatic animal production. Excluding algae, global aquaculture achieved a record production of 94.4 million tonnes in 2022, with an estimated value of 295.7 billion USD. Marine aquaculture, which involves the cultivation of marine organisms for food and other products, accounted for approximately 35.3 million tonnes in 2022, representing about 31% of total marine animal production (FAO, 2024). These findings highlight the pivotal role of aquaculture in meeting the growing global demand for seafood. In order to promote the environmental, ecological and socio-economical sustainability of this important sector, continuous research and innovation for the improvisation of the existing farming techniques, refinement of the feed and disease management strategies and diversification of the production and utilization strategies are some of the fundamental aspects that require focused and dedicated attention (Boyd et al., 2020; Carballeira Braña et al., 2021). Species diversification helps to diversify production, reducing the vulnerability of the aquaculture industry to unforeseen challenges from emerging disease outbreaks, environmental challenges such as rising sea temperatures and/or ocean acidification and market dynamics such as change in consumer preference, market demand, culinary and cultural diversity (Harvey et al., 2017; Cai et al., 2022; Chan et al., 2024). Inclusion of species that can rapidly grow and efficiently convert feed into edible protein can make aquaculture practice more resource-efficient and sustainable.
India, the second largest aquaculture producer (excluding algae) of the world, alone contributed about 10.2 million tonnes of aquatic animal production in 2022 which is about 11% of the global aquaculture production and 27% of Asia’s aquaculture production. As far as the marine and coastal aquaculture of aquatic animals is concerned, India ranks the 6th global position with a production of about 1.2 million tonnes against the projected potential of 4–8 million tonnes (FAO, 2024). Nevertheless, marine aquaculture holds significant opportunities in India considering the rapidly increasing demand for seafood, a demand that cannot be satisfied solely by the capture fisheries sector (Parappurathu et al., 2023). Currently, marine aquaculture in India mainly encompasses the cage culture of finfish and shellfish in open seas and internal waters using the hatchery produced and wild collected seeds, bivalve and pearl oyster farming, ornamental fish culture, seaweed cultivation and integrated multi-trophic aquaculture (IMTA) (Parappurathu et al., 2023). Presently, in India, the breeding and seed production techniques have already been standardized for nine marine finfish species (Rachycentron canadum, Epinephelus coioides, Trachinotus blochii, T. mookalee, Lethrinus lentjan, Pomadasys furcatus, Lutjanus johnii, Siganus vermiculatus and Acanthopagrus berda) by ICAR-Central Marine Fisheries Research Institute (Anuraj et al., 2021; Suresh Babu et al., 2022) out of which, many are popularly used for grow-out operation (Aswathy et al., 2020). Apart from this ICAR-CMFRI (India) has also prioritized 76 finfish and shellfish species that could be targeted for future expansion of marine aquaculture production in the country (Ranjan et al., 2017). The Black-spotted croaker, P. diacanthus (Lacepède, 1802) is one such prime species that is presently being evaluated for its marine aquaculture potential in India by ICAR-CMFRI.
P. diacanthus, also known as Ghol in India, belongs to the family Sciaenidae and is widely distributed in the coastal waters of the Indo-West Pacific region (Barton, 2018). It is a fast-growing and long-lived large predatory fish species that dwells in the epibenthic near-shore waters and feeds mainly on crustaceans and small fishes (Phelan et al., 2008; Barton, 2018). The species has significant market demand for its swimbladder, which is priced at USD 480-600 per kilogram (Dutta et al., 2014). It is also highly regarded as a food fish, with a market price ranging from USD 25-35 per kilogram (Dutta et al., 2014; Li et al., 2020). All these benefits make it one of the highly lucrative marine species. Due to its high market price, the species has been the prime focus of fishers for quite some time and forms an important component of commercial and subsistence fisheries in India and many countries abroad (Phelan et al., 2008; Dutta et al., 2014). However, its catch depends more on chance due to its rare and patchy (non-uniform) distribution (Dutta et al., 2014). Several studies have reported intra-annual changes in the abundance of P. diacanthus in inshore waters (Bhatt et al., 1964; Ansari et al., 1995), which indicated a seasonal migratory nature of the species. The fish is believed to perform seasonal migration and aggregation probably in response to reproduction cycles (Dhawan, 1971) and/or food availability (Thomas and Kunju, 1981). Unfortunately, fish that show aggregation behaviour are typically vulnerable to overfishing and excessive targeting, especially during spawning aggregation, which could be detrimental for such species (Sadovy and Cheung, 2003), as has been the case in Australia with reduction in total length and an abundance of smaller size groups in the catches, and a declining size at maturity (Phelan et al., 2008; Leigh et al., 2022).
Due to its fisheries’ significance and vulnerability, the species has drawn the attention of researchers in the past, with global studies focusing mostly on growth, food and feeding habits, reproductive biology and fisheries management (Bibby and McPherson, 1997; McPherson, 1997; Phelan et al., 2008; Ghosh et al., 2010; Leigh et al., 2022). Considering the high demand and the challenges involved in its capture fishery in India, alternate production strategies like captive breeding and farming have been suggested. Farming of P. diacanthus in marine cages would not only reduce exploitation pressure on the natural population of this species but also produce a dependable source of production to meet the ever-increasing consumer demand for the species. Due to its fast growth rate, disease resistance, strong adaptability and excellent market value, it is lately being preferred as a lucrative candidate species for commercial aquaculture in China (Li et al., 2016; Rong et al., 2020). Several studies have attempted to understand its ovarian, embryonic and larval development to standardize artificial breeding (Shi et al., 2004; Zhang et al., 2006; Shen et al., 2007). Apart from this, several trials have also been made to study the nutritional requirements of this fish to develop a cost-effective, balanced diet for ensuring better growth under captivity (Li et al., 2017, 2019). Nevertheless, based on the review of accessible literature, it is evident that the aforementioned studies primarily consist of brief trials in which the growth and/or feed utilisation of the species have been assessed within a very short timeframe spanning up to eight weeks. None of the studies presented in the literature portray the growth performance of the species over an adequate timeframe that is necessary for achieving a consumer-acceptable table size (marketable size), which is a crucial factor in determining its suitability as a potential aquaculture species. Therefore, the present study was envisaged (1) To generate in-situ growth data for P. diacanthus by rearing it in floating marine cages under natural captive conditions, (2) To develop a model that simulates the future growth potential of P. diacanthus in floating cages over an extended culture period, and (3) To assess the growth performance of P. diacanthus and evaluate its suitability as a candidate species for aquaculture.
A total of 12 numbers of cages were used for the experiments, and the entire cage units occupied a stretch of approximately 1.5 km neighboring the Bahabalpur Fishing Harbour (210 27.441’N and 870 9.602’S, Odisha, India) (Figure 1). Circular floating cages of 6.0 m inner diameter and 8.0 m outer diameter with 4.5 m net depth (water holding depth) were used for the experiment (area: 28.29 m2; volume: 127.29 m3). The High-Density Polyethylene (HDPE) pipes of Pressure Nominal (PN)-10 and Polyethylene (PE) 100-grade quality were used for the fabrication of the floating cages. HDPE nets were used for better strength; where, inner fish-holding vertical hanging net of 4.5 m water holding depth with 25 mm mesh size was used; outer vertical hanging net of 5.0 m depth with 40.0 mm mesh size was used as predator net; and a horizontal surface net with 80 mm mesh size was used as bird protection net. The inner holding net was supported with two ballast pipes at 2.0 m and 4.0 m below the water surface to maintain the cylindrical shape of the net. The floating cage structure was stabilized in the sea with the help of mooring systems supported by 1.5 t capacity concrete cement blocks (dead weight anchor) and mooring chain (long link alloy steel chain of 13 mm diameter with 22 t shearing strength).
Figure 1. Map showing the experimental location of open sea cage farms established at Bahabalpur (Odisha, India) for the experimental rearing of Protonibea diacanthus.
The live juveniles of Blackspotted croaker (P. diacanthus) were collected from the local fishers fishing in the vicinity of the cage farming demonstration of Indian pompano (Trachinotus mookalee), which was underway in the above-mentioned geographical location. The fish were caught in gill nets (mesh size: 50 mm) from a depth of 5-10 m. The fishes were identified as P. diacanthus (Figure 2) following the morphological description of Fischer and Bianchi (1984). A total of 193 live specimens were collected from the local fishers over a month as and when they were caught, and were transported in 1,000 l onboard water tanks filled with seawater (32 ppt) with continuous aeration. Due to the scarcity of separate and independent offshore farming facilities, the specimens (n = 180) were stocked in six cages, in which culture of the Indian Pompano, T. mookalee of mean total length (TL) 12.29 ± 0.16 cm and mean body weight (BW) of 31.12 ± 1.17 was ongoing at a density of 2,500 individuals per cage. Indian pompano fingerlings, produced in the marine finfish hatchery complex at the Regional Centre of ICAR-CMFRI in Visakhapatnam, India, were transported overnight to the cage farming demonstration site using insulated live-fish carriers. The stocking density used for Indian pompano was determined based on our earlier work (Sekar et al., 2021).
Figure 2. Black-spotted croaker (Protonibea diacanthus) experimentally cultured at Bahabalpur (Odisha, India).
The study was conducted using a Completely Randomized Design (CRD) with two experimental groups, each replicated three times. The experimental groups, Group-1 and Group-2, were classified based on size variations in P. diacanthus specimens.
● Group-1: This group consisted of 90 smaller-sized individuals (n = 90) with a mean total length (TL) of 22.69 ± 0.44 cm (mean ± SE) and a mean body weight (BW) of 130.15 ± 6.39 g (mean ± SE). The fish were distributed across three cages in triplicates, with 30 individuals per cage.
● Group-2: This group included 90 larger-sized individuals (n = 90) with a mean TL of 29.34 ± 0.78 cm and a mean BW of 287.80 ± 16.90 g. These were also distributed across three cages in triplicates, with 30 individuals per cage.
Due to lack of dedicated sea cages for P. diacanthus, both the Group-1 and Group-2 were maintained as polyculture setups, where the 30 numbers of P. diacanthus were added to the existing 2,500 numbers of T. mookalee (a mean TL of 12.18 ± 0.27 cm and a mean BW of 30.90 ± 1.83 g) per cage, resulting in a total stocking density of 2,530 fish per cage in the polyculture setups. Additionally, a monoculture setup comprising six cages stocked only with 2,500 numbers of T. mookalee (mean TL of 12.39 ± 0.24 cm and a mean BW of 31.34 ± 1.84 g) per cage was maintained to compare and evaluate any synergistic or antagonistic effects of P. diacanthus on T. mookalee in the polyculture setups.
The feeding regime followed for T. mookalee using a commercial diet (Nutrila Marine Fish Feed, Growel, Andhra Pradesh, India; Ingredients: fish meal, soybean meal, wheat products, rice products, fish oil, vegetable oil, soya lecithin, amino acid, vitamins and minerals; Nutritional Composition: 40% crude protein, 10% crude fat, 3% crude fiber, and 12% crude moisture; Pellet size: 1.5 mm, 3.0 mm and 6.0 mm) was continued unchanged in both the experimental groups; and was at 6% of the combined body weight initially, which was gradually reduced to 3% of the combined body weight. The fish were grown for a maximum duration of 148 days. Feeding trials with P. diacanthus were conducted during the acclimatization phase using the specified feed to evaluate its acceptability by the species. The entire ration was divided into 3 equal portions and broadcasted in early morning (06:00 hr), mid-day (12:00 hr) and evening (18:00 hr) in the middle of the cage to make it available for all the fish. Adequate measures were employed to control feed wastage from the cage, where the inner fish-holding cage net was stitched with a zero-mesh size nylon net at the water and air interface to retain the feed completely inside the cage. The growth was monitored fortnightly and the feeding rate and ration were adjusted accordingly.
The growth performance of the fish (P. diacanthus) during the culture duration was evaluated using the indices such as total length gain (TLG%), body weight gain (BWG%), specific growth rate (SGR) and absolute growth rate (AGR) which were assessed using the following formula.
Fulton’s condition factor was calculated according to Htun-Han (1978) equation, as per the formula given below:
Where, BW is the body weight of fish in g; TL is the total length of fish in cm.
The feed utilization performance of the fish (P. diacanthus) during the culture duration was evaluated using the indices such as feed conversion ratio (FCR), protein conversion rate (PCR) and protein efficiency rate (PER) which were assessed using the following formula. The average protein content of the commercial feed was used for the calculation of PCR and PER.
The survival rate (%) was calculated using the following formulae
The above-mentioned indices on growth and feed utilization performances were also assessed for Indian Pompano grown in both the monoculture and polyculture setups and compared to assess any possible impact of P. diacanthus on the standalone growth and feed utilization performances of T. mookalee.
To simulate and project the growth potential of the fish beyond the actual culture duration of the present study, the available growth data was modeled using the fortnightly collected periodic length (mm) and weight (g) increment data. The growth was modeled using the non-linear von Bertalanffy’s growth equation (von Bertalanffy, 1934) using ‘nls’ routine in the R statistical software package.
Where Lt and Wt are the length (cm) and weight (g) of fish, respectively, that can be expected at age t (years); L∞ and W∞ are asymptotic length (cm) and weight (g) of the fish, respectively; k is the growth coefficient of the fitted curve; t0 is the age (year) when the size of the fish was theoretically zero. It is also the point where the fitted growth curve intersects the x-axis and is also used as a proxy for the gestation or incubation period (hatching duration). The weight form of the von Bertalanffy’s growth equation (Equation 2) necessitates another parameter, i.e., b, which is the power of the length-weight relationship of the fish. The relationship between length and weight (LWR) was established using the power law suggested by Le Cren (1951).
Where BW is the body weight of fish in g; TL is the total length of fish in cm; ‘a’ and ‘b’ are model coefficients. A Wald test was performed to check if the growth is isometric (b=3.0) or allometric (b≠3.0).
The Shapiro-Wilk test (Shapiro and Wilk, 1965) was performed on growth performance and feed utilization performance data to check the normality of the data. An F-test was performed to check the equality or homogeneity of variance (homoscedasticity). When the assumption of normality of the data was not met, the Welch t-test was performed to determine if there were statistically significant differences between the means of the experimental groups. All the statistical analyses in the present study were performed using the R statistical software package (R Core Team, 2021).
The growth performance and feed utilization efficiency indicators and their comparisons between the two experimental groups (Group-1 vs. Group-2) are shown in Figure 3 and Supplementary Table S1. Both the relative growth indicators, i.e., total length gain (TLG%, Figure 3A), body weight gain% (BWG%, Figure 3B) and the instantaneous growth indicator, i.e., specific growth rate (SGR, Figure 3D) were significantly higher (P ≤ 0.05) in Group-1 compared to the Group-2. Contrary to this a significantly lower (P ≤ 0.05) absolute growth rate (AGR) (Figure 3F) was recorded in the Group-1 compared to the Group-2. The condition factor (CF) (Figure 3C) did not show any significant difference (P > 0.05) among the experimental groups.
Figure 3. Comparison of growth performance indices, (A) total length gain (TLG%), (B) body weight gain (BWG%), (C) condition factor (CF), (D) specific growth rate (SGR), (E) modeled growth (increment in body weight) during culture, (F) absolute growth rate (AGR); and feed utilization indices, (G) feed conversion ratio (FCR), (H) feed efficiency ratio (FER), and (I) protein efficiency ratio (PER) between the fish (Protonibea diacanthus) reared in two experimental groups (Group-1 vs. Group-2).
The modeled growth in terms of absolute increase in body weight is presented in Figure 3E. None of the feed efficiency indicators, i.e., feed conversion ratio (FCR, Figure 3G), feed efficiency ratio (FER, Figure 3H) as well as the protein efficiency ratio (PER, Figure 3I) showed any signficant difference (P > 0.05) among the experimental groups. The survival was 100% in both the experimental groups.
The growth performance and feed utilization efficiency indicators and their comparisons between the two culture systems (polyculture and monoculture) are shown in Figure 4 and Supplementary Table S2. The relative growth indicators, i.e., TLG% (Figure 4A), BWG% (Figure 4B) and CF (Figure 4C) of T. mookalee grown in polyculture mode with P. diacanthus did not vary significantly (P> 0.05) from that of the fish grown in monoculture mode. Similarly, there was no significant difference (P> 0.05) in the SGR (Figure 4D) and AGR (Figure 4F) of T. mookalee grown in polyculture and monoculture modes. The von Bertalanffy’s growth function (VBGF) parameters derived by fitting the growth model using fortnightly weight increment data of T. mookalee is shown in Figure 4E. The asymptotic length (L∞) and weight (W∞) for the fish were derived as 95.06 ± 1.24 cm and 11574.49 ± 377.90 g, respectively for polyculture mode and 95.17 ± 1.30 cm and 11850.12 ± 387.80 g, respectively for monoculture mode. The modeled growth coefficient (k) and t0 were 0.48 ± 0.01 yr-1 and -0.004 ± 0.01 yr, respectively for polyculture mode and 0.48 ± 0.01 yr-1 and -0.003 ± 0.01 yr, respectively for monoculture mode. The simulated growth trajectories for T. mookalee grown in polyculture mode with P. diacanthus did not vary significantly (P> 0.05) from that of the T. mookalee grown alone in monoculture mode. Similarly, none of the feed utilization efficiency indices viz., FCR ('Figure 4G), FER (Figure 4H) and PER (Figure 4I) of T. mookalee were significantly different beween the polyculture and monoculture setups.
Figure 4. Comparison of growth performance indices, (A) total length gain (TLG%), (B) body weight gain (BWG%), (C) condition factor (CF), (D) specific growth rate (SGR), (E) modeled growth (increment in body weight) during culture, (F) absolute growth rate (AGR); and feed utilization indices, (G) feed conversion ratio (FCR), (H) feed efficiency ratio (FER), and (I) protein efficiency ratio (PER) between the fish (Trachinotus mookalee) reared in two culture setups (Polyculture vs. Monoculture).
The length-weight relationship (LWR) modeled for the fish is shown in Figure 5. The weight increment appears to be isometric as the exponent (b) is not significantly different from 3 (Wald-test, F-value=0.2292, P=0.6339). The von Bertalanffy’s growth function (VBGF) parameters derived by fitting the growth model using fortnightly length and weight increment data are shown in Figure 6 and their comparison with earlier studies is given in Table 1, Supplemetary Table S3 and Figure 7. The asymptotic length (L∞) and weight (W∞) for the fish were derived as 164.21 ± 3.58 cm and 44070.19 ± 2811 g, respectively. The modeled growth coefficient (K) and t0 were 0.30 ± 0.01 yr-1 and -0.005 ± 0.02 yr, respectively. The fortnightly and annual growth projections from previously established growth models using samples from the natural feral (wild) condition and the presently fitted growth model using samples from the natural captive (culture) condition are shown in Figure 7 and Supplementary Table S3. The projected age for sexual maturity (TM50) appears to be earlier (approximately 2.5 years) under natural captivity compared to the wild condition (Figure 7), which indicates that the species grows faster and matures earlier when cultured in a natural captive condition. The growth performance index (ϕ’=1.91) was also observed to be higher in the fish cultured in sea cage farms compared to the fish in the wild (Table 1).
Figure 5. Relationship between the total length (cm) and body weight (g) of Protonibea diacanthus (A: observed data; B: modeled projection).
Figure 6. Growth modeling of Protonibea diacanthus using fortnightly length and weight increment data. (A) represents length-based VBGF model, with the blue arrow indicating a zoomed view of the actual length increment data fitted by the model. (B) represents weight-based VBGF model, with the red arrow indicating a zoomed view of the actual weight increment data fitted by the model.
Table 1. Von Bertalanffy’s Growth Function (VBGF) parameters and Length-Weight Relationship (LWR) parameters (Mean ± SE) modeled for Protonibea diacanthus reared in sea cage farms at Bahabalpur (Odisha, India), and comparison with earlier studies.
Figure 7. Comparison of growth performance of Protonibea diacanthus in natural wild conditions, derived from length-at-age keys modeled in earlier studies, with the growth performance of P. diacanthus cultured in captive conditions modeled using periodic length increment data from sea cage farms at Bahabalpur (Odisha, India).
The fast growth and high market price, especially due to the demand for swimbladders and the nutritional quality of its meat make P. diacanthus a potential candidate species for marine aquaculture (Li et al., 2016; Rong et al., 2020). The data available in the public domain at present describes the growth of the species over short-duration trials conducted for a maximum of up to 56 days. Furthermore, there is no predictive model available to understand the growth of this species in captive conditions for a longer timeframe, which is essential to assess the culture performance of the species for aquaculture. The experiment has generated distinctive in situ length increment data, which has been applied to develop models to understand the growth of the species under captive conditions beyond the duration of culture in this study to assess its suitability for aquaculture. The modeled growth performance of the species will pave the way for future research to further refine the technological interventions to develop the species as an ideal candidate species for aquaculture.
From a thorough review of the accessible literature available in the public domain, it appears that in most of the world including India, the breeding and seed production technologies, along with grow-out technology for the species, are yet to be standardized. Therefore, the present study was attempted by using wild-caught juveniles of P. diacanthus and integrating it to the culture set-up originally developed for the grow-out of T. mookalee. Uniformity in initial stocking size could not be ensured for both the culture groups of P. diacanthus (Group-1 and Group-2) due to the sole dependency on nature for seed materials. As the T. mookalee, the originally mandated species of the ongoing demonstration program, were harvested after a culture period of 5 months (148 days), it was economically not viable to further continue the culture experiment with P. diacanthus. Nevertheless, the experiment could generate the much-needed and rarely available length and weight increment data for 148 days necessary to model the growth performance of P. diacanthus under natural captive conditions, which is of paramount importance to evaluate the species for its aquaculture potential so that future research for its artificial propagation, seed production and grow-out technology can be envisaged.
The few short-duration (56-day) sea-based in-situ culture trials conducted by earlier studies have reported the growth performance of P. diacanthus either in terms of absolute growth rate (length or weight increase) or relative growth rate (length or weight gain percentage compared to initial length or weight, respectively) or as an instantaneous growth rate (specific growth) (Li et al., 2016, 2017, 2019; Rong et al., 2020). However, these indices have certain inherent limitations in their underlying assumptions. For example, the absolute growth rate (AGR) in the present study was observed to be significantly higher (P< 0.05) in Group-2 (mean weight ± SE: 287.80 ± 16.90 g) compared to the Group-1 (mean weight ± SE: 130.15 ± 6.39 g). In aquaculture studies, the AGR, though considered a standard method or index for expressing and comparing the growth rate, it assumes that growth is linear and constant throughout the entire life history of fish, which is not true (Hopkins, 1992). Furthermore, AGR measures the total change in the mass of cultured animals over a specified time period, but it assumes that the individuals being compared should have the same initial sizes. Otherwise, the periodic increase in absolute mass of a slow growing larger individual could be higher than the fast-growing smaller individuals. Therefore, AGR could give biased results if the cultured animals have different initial sizes. One way to address this issue (different initial stocking size or biomass) is to calculate the growth rate of the cultured animals in terms of relative growth rate index (RGR) that derives the growth as a ratio of final to initial mass. It assumes that the animals grow in equal proportion throughout their entire life stage which is not true. Fish usually grow slower during the initial and late stages and faster during the intermediate stage, which is entirely ignored by the above-mentioned growth performance indices and therefore, could be less informative when such indices are used to compare growth over a longer duration. In the present study, contrary to the AGR, the RGRs (i.e., TLG and BWG), were significantly higher (P< 0.05), in the Group-1 (mean weight ± SE: 130.15 ± 6.39 g) compared to the Group-2 (mean weight ± SE: 287.80 ± 16.90 g), which is due to faster growth rate of the smaller individuals compared to the larger. Another way of expressing the growth rate is the instantaneous growth rate, a form of which is expressed as the specific growth rate (SGR). Since it assumes the weight of fish grows exponentially, it is only valid to compare the growth of fast-growing young fish cultured for a short-duration but not valid for larger fish cultured for a long duration (Hopkins, 1992). In the present study, just like the AGRs, the SGR was found to be higher for smaller sized Group-1 compared to the large sized Group-2. Therefore, all these indices may not always be suitable to compare the growth performance of the fish in experimental set-ups, especially which depends on feral fish as it is difficult to ensure same culture size and duration among the experimental groups due to the sole dependency on wild for seed. Another way of expressing the growth rate is the instantaneous growth rate, a form of which is expressed as the specific growth rate (SGR). Since it assumes the weight of fish grows exponentially, it is only valid for young fish cultured for a short-duration but not valid for larger fish cultured for a long duration (Hopkins, 1992).
Furthermore, the conventional growth indices have very limited applicability as they can only describe the growth that can be observed during a culture experiment, mostly by differentiating the growth at the end by comparing it with the growth at the beginning of the experiment. To visualize what is happening at intermediate points and beyond the observable experimental data and make any predictions about further growth development, it is essential to use complex non-linear models. The von Bertalanffy growth function (VBGF) is one such nonlinear model that is popularly used in fishery biology to describe the growth of fish (Hopkins, 1992; Lugert et al., 2016; Lugert et al., 2017). Furthermore, the majority of the studies conducted to assess the life history parameters of feral populations of P. diacanthus have modeled the growth parameters using the non-linear von Bertalanffy growth function (VBGF) and therefore, the same was adopted in the present study so that the results can be compared. The present study is unique in that the growth performance of P. diacanthus has been modeled using the length and weight increment data collected over the longest available culture duration of 148 days through the VBGF model, which could be considered scientifically sound and more reliable.
According to Leigh et al. (2022), P. diacanthus is a large species which can attain lengths of at least 150 cm in total length and weights of at least 42 kg, and can live up to at least 15 years. The growth performance modeled using von Bertalanffy’s growth function (VBGF) revealed an asymptotic length (L∞) of 164.21 cm and a growth rate of 0.30 yr-1 for the species. By comparing the L∞ derived in the present study with that of the earlier studies mentioned in Table 1, though it appears to be higher, it is reasonable as the back calculated Lmax (i.e., 156 cm) using the empirical thumb rule of the relationship between L∞ and Lmax (i.e., L∞ = Lmax/0.95) recommended by Pauly (1984) and Taylor (1958), is similar to the observed Lmax of 156 cm and 152 cm for this species that have previously been reported from Maharashtra and Gujarat states of India (Hotagi, 1994; Mohammed et al., 2009). The present estimate is also similar to the Lmax of 154 cm (Bibby and McPherson, 1997) reported from the Queensland Gulf (Australia). However, the present estimate of maximum length (Lmax = 156 cm) and maximum body weight derived using the Lmax and length-weight relationship (Wmax = 37925 g) are smaller compared to the Lmax of 176 cm and Wmax of 45000 g reported by Grant (1982) from Queensland (Australia), though the latter was almost similar to the asymptotic weight (W∞=44070 g) modeled in the present study. Similarly, there are studies from India (Rao, 1966, 1968; Ghosh et al., 2010) as well as Australia (Erzini, 1991; Bibby and McPherson, 1997; Leigh et al., 2022) where the derived L∞ can be observed to be lower compared to the present study. As the Lmax (and so does the dependent L∞) depends mostly on the exploitation parameters, it tends to decrease when there is targeted fishery for the species, which is evident in the P. diacanthus fishery of Queensland (Australia), where the Lmax has decreased drastically from 176 cm in 1982 to 92.5 cm in 2000, and then subsequently revived to 103.5 cm in 2002-2003 (Phelan et al., 2008). In the present study, the dissimilarity observed in the derived Lmax (and therefore the modeled L∞) with that of earlier studies could be due to the spatiotemporal differences in the fishery-related factors. However, the growth coefficient (K) modeled for the species in the present study appears to be similar or higher compared to most of the earlier studies mentioned in Table 1, except for those conducted by Erzini (1991) and Leigh et al. (2022). However, careful scrutiny reveals that for these studies, the reported L∞ is very low (L∞< 120 cm) compared to the present study. Due to its compensatory behaviour, the K increases as the L∞ decreases and vice versa, so that the overall growth performance remains more or less constant for a species (Pauly and Munro, 1984). Therefore, a merely high K value does not necessarily mean a better growth rate and hence the growth performance index (ϕ) should rather be compared to understand the growth performance of the species. As ϕ’ is based on body length rather than body weight, it is less liable to bias. It has the dimensions of both length and time and is usually normally distributed with smaller standard deviations compared to other growth indices and therefore, it has been recommended as the most flexible and precise estimator of growth performance (Mathews and Samuel, 1990). The higher ϕ’ (=1.91) observed in the present study illustrates the superior growth performance of the species under natural captive conditions, which can also be seen in Supplementary Table S2 and Figure 7. As the growth of the animals in a culture system is strongly boosted in a time-bound manner by technological interventions like highly proteinaceous feed and augmented environmental conditions, they may outperform their wild counterparts in terms of growth performance. It has been substantiated that fish grown in sea-based cage culture set-up exhibit better growth, probably due to the acquisition of supplementary nutrition (apart from highly proteinaceous feed) from naturally occurring organisms growing in/on the culture system, as well as plankton, invertebrates and other live prey items that move through the culture system (Simon and James, 2007; Perez-Benavente et al., 2010). Furthermore, fish reared in sea-based culture systems with adequate water exchanges are likely to receive environmental (physical) enrichment that attenuates stress and improves overall welfare (Johnsson et al., 2014; Näslund and Johnsson, 2016; Jones et al., 2021).
McPherson (1997) and Phelan et al. (2008) have reported the length at first maturity to be 79 cm TL in Northern Cape York Peninsula waters and 92 cm TL in Queensland waters respectively, that approximately corresponds to the 3 and 4 years age classes using the length/age keys developed by Bibby and McPherson (1997). The findings of Phelan et al. (2008) also match with the observations by Rao (1963) from Indian waters, who found that 5% of the fish were mature in the 75–80 cm TL, 36.8% in 80–85 cm TL, 91% in 85–90 cm TL, and 100% in 90–95 cm TL, in which the first length at maturity is attained at an approximate age of 3 years using the VBGF modeled for the species by Rao (1966). The spatiotemporal variations in the size of maturity observed in these studies could be attributed to fisheries related exploitation factors that influence the maturity of the targeted population, which decreases as the targeted exploitation increases (Phelan et al., 2008). According to the growth model developed in the present study, these lengths of maturity, i.e., 79 cm and 92 cm TL would be attained at about 2.2 years and 2.7 years respectively if the fish are allowed to grow under natural captive conditions. This implies that the fish matures a year earlier in the natural captive condition (which can be verified in Figure 6) as it grows faster compared to the wild ones that grow in natural conditions. The information has great implications as it can be used to design future experiments to standardize artificial breeding and seed production of P. diacanthus.
A comparison with previous captive-rearing studies conducted in China provides valuable insights into growth performance. As growth performance indicators (GPIs) like WG% and SGR depend on the initial size or body weight of fish, the indices generated in the present study cannot be compared directly with those from the earlier studies. To enable an unbiased comparison, initial body weight of the present study was simulated to match with the initial body weights of the previous studies and the final body weight after 56 days of culture (which is the culture duration of the previous studies) was derived using the established VBGF growth model. The simulated GPIs, i.e., 160.81 WG% and 1.71 SGR obtained in the present study by feeding the fish a commercial diet containing 40% crude protein were marginally lower compared to the GPIs (171.74 WG% and 1.78 SGR) obtained by Li et al. (2016) when fish were fed a commercial diet having 41% crude protein. The GPIs were also observed to be higher compared to the present study when the fish were fed a commercial diet having higher percentage (≥ 45%) of crude protein (Li et al., 2016, 2017, 2019 and Li et al., 2020). It is well established that carnivorous fish generally exhibit enhanced growth performance when provided with diets containing high levels of good-quality protein (McGoogan and Gatlin, 1999). As a carnivorous species, P. diacanthus exhibits a limited ability to utilize non-protein energy sources, and relies primarily on protein oxidation to fulfill its cellular energy requirements (Li et al., 2017).
A comparison of feed utilization indicators across the experimental groups (Group-1 vs. Group-2) revealed no significant differences in FCR, FER, and PER. However, the feed efficiency indicators obtained in this study (i.e., FCR of 5.15, FER of 0.19, and PER of 0.49) were inferior to those reported by Li et al. (2016), who observed an FCR of 1.59, FER of 0.63, and PER of 1.55 by feeding P. diacanthus a diet containing 41% crude protein, primarily supplemented with fishmeal. Similarly, better feed utilization indicators were noted in studies where diets with higher protein content (≥45%) were provided (Li et al., 2019, 2020). The considerable deviation in feed efficiency parameters between the present study and earlier investigations could be attributed to the differences in initial stocking size. Additionally, the present study did not specifically focus on evaluating feed utilization efficiency of P. diacanthus. This was due to the limited availability of wild-caught P. diacanthus seeds, resulting in its rearing alongside T. mookalee in small proportions. Consequently, the same feeding regime was applied to both species, making it challenging to monitor and optimize the actual feed intake of P. diacanthus. Instead, this experiment served as an opportunity to generate growth data for P. diacanthus under natural captive conditions (marine cages). The primary aim was to assess the feasibility of captive rearing for this species rather than to optimize feed utilization.
The condition factor (CF) reported in the present study, i.e., 1.13 g cm-3 and 1.14 g cm-3 for Group-1 and Group-2, respectively, though not significantly different between the groups, are less compared to the CF of 1.74 g cm-3 reported by Li et al. (2016), 1.61 g cm-3 reported by Li et al. (2017) and 1.65 g cm-3 reported by Li et al. (2020). This indicates that the fish grown in the present study were lean and slender compared to the fishes grown in these above-mentioned earlier studies. As the crude lipid content in most of the previous studies was slightly higher, it might have influenced the fish to become fatty and pulpier. Furthermore, as both the feed utilization indicators and condition factors were lower in the present study, the possibility of growth interference due to the intense competition from the dominant T. mookalee for feed and space cannot be entirely ruled out. This also indicates that there is a possibility in which P. diacanthus could perform better (both in growth and feed utilization) if allowed to grow in a monoculture setup. Therefore, further studies should be envisaged to optimize the feed efficiency of the species by growing them alone in the laboratory as well as in natural captive conditions. Nevertheless, the study indicates that the species grows faster in natural captive conditions (marine cages) and therefore, can be considered as a probable candidate species for aquaculture.
Interestingly, neither the growth performance indices (TLG, BWG, SGR and AGR) nor the feed utilization indices (FCR, FER and PER) of T. mookalee were significantly affected when P. diacanthus was introduced. The SGR (≈1.55% day-1), AGR (≈1.91 g day-1) and FCR (2.29) of T. mookalee observed in the present study are also found to be in congruence with the earlier reported values of SGR (1.47% day-1), AGR (1.89 g day-1) and FCR (2.19) obtained by growing T. mookalee in marine cages off Visakhapatnam coast in India (Sekar et al., 2021). This indicates that in the present study, the inclusion of P. diacanthus in the existing monoculture setups of T. mookalee has not adversely or synergistically impacted the growth performance and feed utilization of T. mookalee. However, as the number of P. diacanthus was lower compared to T. mookalee, it would be too premature to comment on this aspect of polyculture.
The present study assessed the survival and actual growth of P. diacanthus and modeled its potential growth in captive conditions within floating marine cages in a polyculture system with T. mookalee. The results, based on length and weight increments analyzed and modeled using the von Bertalanffy Growth Function (VBGF), indicate that P. diacanthus exhibits superior growth performance in marine cages compared to wild conditions. Additionally, the study found that co-culturing P. diacanthus with Indian Pompano (T. mookalee) does not negatively affect the growth or feed utilization efficiency of T. mookalee. These findings highlight the potential of P. diacanthus to be used as a candidate species in marine cage farming.
The original contributions presented in the study are included in the article/Supplementary Material. Further inquiries can be directed to the corresponding author.
The animal study was approved by ICAR-CMFRI, Central Marine Fisheries Research Institute, Kochi, India. The study was conducted in accordance with the local legislation and institutional requirements.
SG: Conceptualization, Methodology, Project administration, Supervision, Writing – original draft. GD: Conceptualization, Methodology, Software, Writing – original draft, Writing – review & editing. BD: Data curation, Methodology, Writing – original draft. RKP: Data curation, Writing – original draft. SM: Data curation, Writing – original draft. PRB: Data curation, Writing – review & editing. RR: Data curation, Writing – review & editing. SS: Formal analysis, Writing – original draft. MD: Data curation, Writing – original draft. SVR: Writing – review & editing. GA: Conceptualization, Supervision, Writing – review & editing. JJ: Conceptualization, Supervision, Writing – review & editing.
The author(s) declare financial support was received for the research, authorship, and/or publication of this article. In-house Project Research Grant provided by Indian Council of Agricultural Research, New Delhi, India.
The authors are thankful to the Director, ICAR-Central Marine Fisheries Research Institute, Kochi for providing the requisite infrastructure and facilities to carry out the work.
The authors declare that the research was conducted in the absence of any commercial or financial relationships that could be construed as a potential conflict of interest.
All claims expressed in this article are solely those of the authors and do not necessarily represent those of their affiliated organizations, or those of the publisher, the editors and the reviewers. Any product that may be evaluated in this article, or claim that may be made by its manufacturer, is not guaranteed or endorsed by the publisher.
The Supplementary Material for this article can be found online at: https://www.frontiersin.org/articles/10.3389/fmars.2024.1473319/full#supplementary-material
Ansari Z. A., Chatterji A., Ingole B. S., Sreepada R. A., Rivonkar C., Parulekar A. H. (1995). Community structure and seasonal variation of an inshore demersal fish community at Goa. West Coast of India. Estuarine. Coast. Shelf Sci. 41, 593–610. doi: 10.1016/0272-7714(95)90029-2
Anuraj A., Suresh Babu P. P., Loka J., Ignatius B., Santhosh B., Ramudu K. R., et al. (2021). Induced breeding and larval rearing of vermiculated spinefoot, Siganus vermiculatus (Valenciennes 1835) in indoor conditions. Aquaculture 539, 736600. doi: 10.1016/j.aquaculture.2021.736600
Aswathy N., Joseph I., Ignatius B., Joseph S. (2020). Economic Viability of Cage Fish Farming in India Vol. 134 (Kochi: Central Marine Fisheries Research Institute). CMFRI Special Publication.
Barton D. P. (2018). Notes on the diet of the Black-spotted Croaker (Protonibea diacanthus) across northern Australia. Northern Territory Nat. 28, 61–69. doi: 10.5962/p.374206
Bhatt Y. M., Kutty M. N., Rao K.V., Punwani D. M. (1964). Ghol-Dara" fishery off Bedi port in the Gulf of Kutch. Indian J. Fisheries 11A, 135–156. Available online at: https://eprints.cmfri.org.in/2128.
Bibby J. M., McPherson G. R. (1997). “Age and growth of five target fish species in the Gulf of Carpentaria inshore gillnet fishery,” in Biology and Harvest of Tropical Fishes in the Queensland Gulf of Carpentaria Gillnet Fishery. Ed. Garrett R. N. (Department of Primary Industries, Queensland), 61–85. QI98018.
Boyd C. E., D'Abramo L. R., Glencross B. D., Huyben D. C., Juarez L. M., Lockwood G. S., et al. (2020). Achieving sustainable aquaculture: Historical and current perspectives and future needs and challenges. J. World Aquaculture Soc. 51, 578–633. doi: 10.1111/jwas.12714
Cai J. N., Yan X., Leung P. S. (2022). Benchmarking species diversification in global aquaculture (Rome: FAO), 52. doi: 10.4060/cb8335en
Carballeira Braña C. B., Cerbule K., Senff P., Stolz I. K. (2021). Towards environmental sustainability in marine finfish aquaculture. Front. Mar. Sci. 8. doi: 10.3389/fmars.2021.666662
Chan H. L., Cai J., Leung P. S. (2024). Aquaculture production and diversification: What causes what? Aquaculture 583, 740626. doi: 10.1016/j.aquaculture.2024.740626
Dhawan R. M. (1971). On an unusual abundance of Pseudosciaena diacanthus off Goa. Indian J. Fisheries 18, 191–193. Available online at: https://epubs.icar.org.in/index.php/IJF/article/view/13184.
Dutta S., Giri S., Dutta J., Hazra S. (2014). Blackspotted Croaker, Protonibea diacanthus (Lacepède 1802): A new dimension to the fishing pattern in West Bengal, India. Croatian J. Fisheries 72, 41–44. doi: 10.14798/72.1.684
Erzini K. (1991). A compilation of data on variability in length-age in marine fishes (Collaborative Research Support Program, University of Rhode Island), 36. Working Paper 77.
FAO. (2024). The State of World Fisheries and Aquaculture 2022 – Blue Transformation in action (FAO: Rome), 236. doi: 10.4060/cc0461en
Fischer W., Bianchi G. (1984). FAO species identification sheets for fishery purposes Vol. 4 (Rome, Italy: FAO).
Ghosh S., Mohanraj G., Asokan P. K., Dhokia H. K., Zala M. S., Bhint H. M., et al. (2010). Fishery and population dynamics of Protonibea diacanthus (Lacepede) and Otolithoides biauritus (Cantor) landed by trawlers at Vanakbara, Diu along the west coast of India'. Indian J. Fisheries 57, 15–20. Available online at: https://epubs.icar.org.in/index.php/IJF/article/view/61757.
Grant E. M. (1982). Guide to fishes. 5th edition (Brisbane, Queensland, Australia: Dep. Harbours Mar.), 896.
Harvey B., Soto D., Carolsfeld J., Beveridge M., Bartley D. M. (2017). Planning for aquaculture diversification: The importance of climate change and other drivers (Rome: FAO), 50–91.
Hopkins K. D. (1992). Reporting fish growth: a review of the basics. J. World Aquaculture Soc. 23, 173–179. doi: 10.1111/j.1749-7345.1992.tb00766.x
Hotagi J. S. (1994). Unusual high catch of 'Ghol' at Basein Kolliwada, Thane district, Maharashtra. Mar. Fisheries Inf. Service Tech. Extension Ser. 126. pp, 16–17. Available online at: https://eprints.cmfri.org.in/4184.
Htun-Han M. (1978). The reproductive biology of the dab Limanda limanada (L.) in the North Sea: gonadosomatic index, hepatosomatic index and condition factor. J. Fish Biol. 13, 351–377. doi: 10.1111/j.1095-8649.1978.tb03445.x
Johnsson J. I., Brockmark S., Näslund J. (2014). Environmental effects on behavioural development consequences for fitness of captive-reared fishes in the wild. J. Fish Biol. 85, 1946–1971. doi: 10.1111/jfb.2014.85.issue-6
Jones N. A. R., Webster M. M., Salvanes A. G. V. (2021). Physical enrichment research for captive fish: Time to focus on the DETAILS. J Fish Biol. 99, 704–725. doi: 10.1111/jfb.14773
Lacepède B. G. E. (1802). Histoire naturelle des poissons: IV Vol. 4 (Paris, France: Chez Plassan), 728. Available at: https://www.biodiversitylibrary.org/page/6705733.
Le Cren C. D. (1951). The length-weight relationship and seasonal cycle in gonad weight and condition in perch, Perca fluviatilis. J. Anim. Ecol. 20, 201–219. doi: 10.2307/1540
Leigh G. M., Janes R., Williams S. M., Martin T. S. H. (2022). Stock Assessment of Queensland East Coast black jewfish (Protonibea diacanthus), Australia, with data to December 2021 (State of Queensland, Brisbane: Department of Agriculture and Fisheries, Qeensland Government). Technical Report.
Li W. J., Wen X. B., Zhao J., Li S. K., Zhu D. S. (2016). Effects of dietary protein levels on growth, feed utilization, body composition and ammonia–nitrogen excretion in juvenile Nibea diacanthus. Fisheries Sci. 82, 137–146. doi: 10.1007/s12562-015-0945-9
Li W., Wen X., Huang Y., Zhao J., Li S., Zhu D. (2017). Effects of varying protein and lipid levels and protein-to-energy ratios on growth, feed utilization and body composition in juvenile Nibea diacanthus. Aquaculture Nutr. 23, 1035–1047. doi: 10.1111/anu.12471
Li W., Xu B., Wei F., Li S., Wang S., Wen X. (2020). Effects of partial substitution of dietary fishmeal by fermented soybean meal on growth, amino acid and protein metabolism of juvenile Nibea diacanthus. Aquaculture Nutr. 26, 2147–2158. doi: 10.1111/anu.13153
Li Z., Zhang X., Aweya J. J., Wang S., Hu Z., Li S., et al. (2019). Formulated diet alters gut microbiota compositions in marine fish Nibea coibor and Nibea diacanthus. Aquaculture Res. 50, 126–138. doi: 10.1111/are.2019.50.issue-1
Lugert V., Tetens J., Thaller G., Schulz C., Krieter J. (2017). Finding suitable growth models for turbot (Scophthalmus maximus L.) in aquaculture 1 (length application). Aquaculture Res. 48, 24–36. doi: 10.1111/are.12857
Lugert V., Thaller G., Tetens J., Schulz C., Krieter J. (2016). A review on fish growth calculation: multiple functions in fish production and their specific application. Rev Aquacult. 8, 30–42. doi: 10.1111/raq.12071
Mathews C. P., Samuel M. (1990). Using the growth performance index Phi to choose species aquaculture: an example from Kuwait. Aquabyte 3, 2–4. Available online at: https://hdl.handle.net/20.500.12348/3201.
McGoogan B. B., Gatlin D. M. III. (1999). Dietary manipulations affecting growth and nitrogenous waste production of red drum Sciaenops ocellatus: I. Effects of dietary protein and energy levels. Aquaculture 178, 333–348. doi: 10.1016/S0044-8486(99)00137-4
McPherson G. R. (1997). “Reproductive biology of five target fish species in the gulf of Carpentaria inshore gillnet fishery,” in Biology and Harvest of Tropical Fishes in the Queensland Gulf of Carpentaria Gillnet Fishery. Ed. Garrett R. N. (Department of Primary Industries, Queensland), 86–103. QI98018.
Mohammed G., Ghosh S., Makadia B. V. (2009). “Unusual heavy landing of otolithoides biauritus and protonibea diacanthus at salaya landing centre, jamnagar, gujarat,” in Marine fisheries information service, technical and extension series (200) (Kochi, Kerala, India: CMFRI), 22. Available at: https://eprints.cmfri.org.in/8188/1/MFIS_-_200_English.pdf.
Näslund J., Johnsson J. I. (2016). Environmental enrichment for fish in captive environments: effects of physical structures and substrates. Fish Fisheries 17, 1–30. doi: 10.1111/faf.2016.17.issue-1
Parappurathu S., Menon M., Jeeva C., Belevendran J., Anirudhan A., Lekshmi P. S. S., et al. (2023). Sustainable intensification of small-scale mariculture systems: Farm-level insights from the coastal regions of India. Front. Sustain. Food Syst. 7. doi: 10.3389/fsufs.2023.1078314
Pauly D. (1984). Fish population dynamics in tropical waters: a manual for use with programmable calculators. ICLARM Stud. Rev. 8, 1–325. Available online at: https://hdl.handle.net/20.500.12348/3445.
Pauly D., Munro J. L. (1984). Once more on the comparison of growth in fish and invertebrates. Fishbyte 2, 21. Available online at: https://EconPapers.repec.org/RePEc:wfi:wfbyte:38103.
Perez-Benavente G., Uglem I., Browne R., Mariño-Balsa C. (2010). Culture of juvenile European lobster (Homarus gammarus L.) in submerged cages. Aquac. Int. 18, 1177–1189. doi: 10.1007/s10499-010-9332-9
Phelan M. J., Gribble N. A., Garrett R. N. (2008). Fishery biology and management of Protonibea diacanthus (Sciaenidae) aggregations in far northern Cape York Peninsula waters. Continental Shelf Res. 28, 2143–2151. doi: 10.1016/j.csr.2008.03.022
Ranjan R., Muktha M., Ghosh S., Gopalakrishnan A., Gopakumar G., Joseph I. (Eds.) (2017). Prioritised species for mariculture India (Kochi: ICAR-Central Marine Fisheries Research Institute), 450.
Rao K. V. S. (1963). Some aspects of the biology of ‘Ghol’, Pseudosciaena diacanthus (Lacépéde). Indian J. Fisheries 10, 413–459. Available online at: https://eprints.cmfri.org.in/10402.
Rao K. V. S. (1966). Age and growth of 'Ghol', Pseudosciaena diacanthus (Lacepède) in Bombay and Saurashtra waters. Indian J. Fisheries 13, 251–292. Available online at: https://epubs.icar.org.in/index.php/IJF/article/view/13535.
Rao K. S. (1968). Studies on the scales of Pseudosciaena diacanthus (Lacepède) for estimating growth parameters. Indian J. Fisheries 15, 127–144. Available online at: https://epubs.icar.org.in/index.php/IJF/article/view/13282.
R Core Team. (2021). R: A language and environment for statistical computing (Vienna, Austria: R Foundation for Statistical Computing). Available online at: https://www.R-project.org/ (Accessed December 06, 2022).
Rong H., Lin F., Zhang Y., Bi B., Dou T., Wu X., et al. (2020). The TOR pathway participates in the regulation of growth development in juvenile spotted drum (Nibea diacanthus) under different dietary hydroxyproline supplementation. Fish Physiol. Biochem. 46, 2085–2099. doi: 10.1007/s10695-020-00863-z
Sadovy Y., Cheung W. L. (2003). Near extinction of a highly fecund fish: the one that nearly got away. Fish Fisheries 4, 86–99. doi: 10.1046/j.1467-2979.2003.00104.x
Sekar M., Ranjan R., Xavier B., Ghosh S., Pankyamma V., Ignatius B., et al. (2021). Species validation, growth, reproduction and nutritional perspective of Indian pompano, Trachinotus mookalee–A candidate species for diversification in coastal mariculture. Aquaculture Volume 545 2021, 737212. doi: 10.1016/j.aquaculture.2021.737212
Shapiro S. S., Wilk M. B. (1965). An analysis of variance test for normality (Complete samples). Biometrika 52, 591–611. doi: 10.2307/2333709
Shen Z., Chen H., Yao R., Zhao H. (2007). Seasonal cycles of ovary development of Nibea diacanthus. J. Guangdong Ocean Univ. 27, 7–11. Available online at: https://caod.oriprobe.com/articles/11843330/Seasonal_Cycles_of_Ovary_Development_of_Nibea_diacanthus.html.
Shi Z. H., Xia L. J., Wang J. G. (2004). A study on artificial propagation technique of Nibea diacanthus (Lacepede). Mar. Sci. 28, 34–37.
Simon C. J., James P. J. (2007). The effect of different holding systems and diets on the performance of spiny lobster juveniles, Jasus edwardsii (Hutton 1875). Aquaculture 266, 166–178. doi: 10.1016/j.aquaculture.2007.02.050
Suresh Babu P. P., Anuraj A., Shilta M. T., Asokan P. K., Vinod K., Praveen N. D., et al. (2022). Broodstock development, breeding and larval rearing of Acanthopagrus berda (Forsskal 1775), a suitable species for farming in tropical waters. Aquacult. Res. 53, 6439–6453. doi: 10.1111/are.v53.18
Taylor C. C. (1958). Cod growth and temperature. J. du Conseil 23, 366–370. doi: 10.1093/icesjms/23.3.366
Thomas P. A., Kunju M. M. (1981). On an unusual catch of Ghol Pseudosciaena diacanthus off Goa. Indian J. Fisheries 25, 266–268. Available online at: https://eprints.cmfri.org.in/789.
von Bertalanffy K. L. (1934). Untersuchungen Über die gesetzlichkeit des wachstums. W. Roux' Archiv f. Entwicklungsmechanik 131, 613–652. doi: 10.1007/BF00650112
Zhang Y., Hu S., Xu S., Xie Y., Hu J. (2006). Study on early development of Nibea diacanthus. J. Jimei Univ. 11, 13–17. Available online at: https://caod.oriprobe.com/articles/10185936/Study_on_Early_Development_of_Nibea_diacanthus.html.
Keywords: captive rearing, growth modeling, feed utilization, cage farming, sustainable aquaculture
Citation: Ghosh S, Dash G, Dash B, Pradhan RK, Megarajan S, Behera PR, Ranjan R, Sen S, Das M, Suresh VR, Gopalakrishnan A and Jena J (2025) From ocean to cage: evaluating the culture feasibility of Black-spotted croaker (Protonibea diacanthus). Front. Mar. Sci. 11:1473319. doi: 10.3389/fmars.2024.1473319
Received: 30 July 2024; Accepted: 06 December 2024;
Published: 07 January 2025.
Edited by:
Yngvar Olsen, Norwegian University of Science and Technology, NorwayReviewed by:
Pranaya Kumar Parida, Central Inland Fisheries Research Institute (ICAR), IndiaCopyright © 2025 Ghosh, Dash, Dash, Pradhan, Megarajan, Behera, Ranjan, Sen, Das, Suresh, Gopalakrishnan and Jena. This is an open-access article distributed under the terms of the Creative Commons Attribution License (CC BY). The use, distribution or reproduction in other forums is permitted, provided the original author(s) and the copyright owner(s) are credited and that the original publication in this journal is cited, in accordance with accepted academic practice. No use, distribution or reproduction is permitted which does not comply with these terms.
*Correspondence: Gyanaranjan Dash, Z3lhbnJhbmphbmRhc2hjbWZyaUBnbWFpbC5jb20=; Z3lhbnJhbmphbi5kYXNoQGljYXIub3JnLmlu
Disclaimer: All claims expressed in this article are solely those of the authors and do not necessarily represent those of their affiliated organizations, or those of the publisher, the editors and the reviewers. Any product that may be evaluated in this article or claim that may be made by its manufacturer is not guaranteed or endorsed by the publisher.
Research integrity at Frontiers
Learn more about the work of our research integrity team to safeguard the quality of each article we publish.