- 1College of Oceanography and Ecological Science, Shanghai Ocean University, Shanghai, China
- 2College of Geography and Environment, Liaocheng University, Liaocheng, China
- 3Institute of Surface-Earth System Science, College of Earth System Science, Tianjin University, Tianjin, China
Hadal trenches (> 6,000 m water depth) have been revealed as hotspots of organic carbon deposition and mineralization. Here, we present the molecular compositions of porewater dissolved organic matter (DOM) at the “Challenger” Deep (Site MT02; 10,954 m water depth) sediments of the Mariana Trench and the adjacent abyssal plain sediments (Site MT04; 5,800 m water depth) using ultra high-resolution Fourier Transform Ion Cyclotron Resonance Mass Spectrometry (FT-ICR MS). The "Challenger" Deep sediments are characterized by higher abundance of recalcitrant compounds, mainly composed of highly unsaturated compounds (79.7%) and carboxyl-rich alicyclic molecules (57.1%), compared to the abyssal plain sediments (68.3%&51.5%). Principal component analysis suggests that TOC content in the sediments exerts an important control on the molecular characteristics of porewater DOM. It is likely that higher TOC content triggers elevated microbial-mediated organic matter mineralization, thereby forming more refractory organic matter compounds. These results improved the knowledge of the poorly-understood DOM compositions and microbial organic matter degradation in the ultra-deep, extreme ocean environment.
1 Introduction
Dissolved organic matter (DOM) in the ocean is the largest pool of reduced carbon on the Earth, with a carbon stock estimated to be up to 662 Gt (Hansell et al., 2009). As the intermediate product of particulate organic matter (POM) degradation in the sediments, porewater DOM is partially transported to overlying bottom waters, while the rest is accumulated in the sediments (Burdige and Komada, 2015). It has been estimated that the flux of dissolved organic carbon (DOC) from coastal and continental margin sediments to the ocean water is comparable to or even more than that transported by global river systems (Burdige and Komada, 2015; Raymond and Spencer, 2015). Therefore, porewater DOM can be important in regulating marine DOM pool.
Despite of being overlain by oligotrophic surface waters in the Mariana Trench area, the "Challenger" Deep, the deepest point of the Earth’s surface, was shown to host enhanced microbial activity and organic carbon turnover compared to the adjacent abyssal plain (Glud et al., 2013). This is due to the funnel-shaped topography of the trench and frequent mass-wasting events that promote the accumulation of relatively labile organic matter at the trench axis (Oguri et al., 2013; Wenzhöfer et al., 2016), thereby forming a unique and diverse microbial population and hotspot for organic matter degradation (Glud et al., 2013, 2021; Nunoura et al., 2015; Leduc et al., 2016; Luo et al., 2017, 2018; Zheng et al., 2020). The properties of pore water DOM are mainly influenced by the source of POM, its degradation rate, and redox conditions (Blair and Aller, 2012; Burdige, 2007; Chen and Hur, 2015; Hu et al., 2021; Koch et al., 2005; Schmidt et al., 2017), thus offering valuable information about the microbially-mediated organic matter degradation (Burdige and Komada, 2015; Chen and Hur, 2015; Chen et al., 2016; Hu et al., 2023). It has been shown that sedimentary DOM in the laminated diatom mats from the Mariana Trench was characterized by highly degraded, low-molecular-weight, and persistent DOM components (Hu et al., 2024). Using ultraviolet-visible spectrophotometer, Hu et al. (2021) demonstrated an enhanced production of more recalcitrant DOM in the Mariana Trench axis compared to the abyssal plain sediments. However, the understanding of the molecular characteristics of porewater DOM in the Mariana trench axis sediments is lacking.
Here, we applied the ultra-high-resolution Fourier Transform Ion Cyclotron Resonance Mass Spectrometry (FT-ICR MS) technique to analyze the molecular compositions of porewater DOM in the sediments of the "Challenger" Deep (10,954 m water depth) and its adjacent abyssal plain (5,800 m water depth) in the northwest Pacific Ocean. Consistent with the findings derived from optical analysis of porewater DOM, our FT-ICR MS measurements confirmed the production of refractory pore water DOM molecules in the "Challenger" Deep. These new findings improved the understanding of the compositions of porewater DOM and microbial degradation processes of organic matter in the extremely high-pressure marine setting.
2 Materials and methods
2.1 Sampling
During the 15th cruise of the Tansuoyihao launched by the Institute of Deep-Sea Science and Engineering of the Chinese Academy of Sciences in 2019, shallow sediment samples were collected at the "Challenger" Deep Site MT02 at 10,954 m water depth (11.327°N, 142.188°E), and its adjacent abyssal plain Site MT04 at 5,800 m water depth (10.761°N, 142.274°E) in the Mariana Trench using a lander (Figure 1). Short cores were subsampled using a transparent PVC tube on board. The core lengths were 18 cm for core MT02 and 24 cm for core MT04. Sediments in both cores were composed of silt and clay, and the "Challenger" Deep core MT02 contained some diatomaceous debris (Luo et al., 2022; Song et al., 2022). The sediment core was sub-sampled at 1 cm intervals for Site MT02 and at 2 cm intervals for Site MT04. Porewater was collected by using a pre-cleaned Rhizon sampler with a pore size of 0.22 μm attached to a 20 ml syringe. Porewater samples for FT-ICR MS analysis were stored in pre-combusted brown glass bottles and then kept at - 20°C.
2.2 Solid phase extraction of DOM (SPE-DOM)
Due to the small volume of pore water samples, all pore water samples collected at Site MT02 and Site MT04 were combined into two samples each to meet the requirement of at least 100 μg C of sample for FT-ICR MS analysis. Given that the retrieved sediment cores were within the oxic zone (Glud et al., 2013), the change in redox condition had negligible impact on the molecular signature of porewater DOM despite of the mixture of porewater sample at each core. The solid-phase extraction of DOM was carried out by a modified procedure according to Dittmar et al. (2008). Firstly, the pore water samples were acidified to pH = 2 with HCl. Then, the solid-phase extraction columns (Bond Elut-PPL, 100 mg, 3 ml; Agilent Technologies, Germany) of the modified styrene-divinylbenzene polymer were pretreated with ultrapure methanol (Mass Spectrometry grade), ultrapure water, and hydrochloric acid. The entire pore water sample was slowly passed through the extraction columns and then rinsed with acidified water to remove inorganic salt. Finally, the solid-phase extraction columns were eluted with 1 ml of methanol and stored at -20°C in the dark for subsequent FT-ICR MS analysis.
2.3 FT-ICR MS analysis and data processing
The FT-ICR MS analysis of pore water DOM was conducted on a Bruker Solarix equipped with a 7.0 T superconducting magnet at Tianjin University. Before analysis, all SPE-DOM samples were diluted with a mixture of methanol and ultrapure water (1:1) to achieve a final SPE-DOC concentration of 50~100 μg/g. The diluted SPE-DOM samples were ionized using an electrospray ionization source in negative ion mode at a flow rate of 150 μL/h. The capillary voltage was set at 5 kV, and the mass-to-charge (m/z) spectra in the range of 150-800 Da were obtained by scanning each spectrum approximately 256 times, with the data acquisition transient lasting for 4 M. The acquired mass spectra were analyzed using Data Analysis software version 5.0 (Bruker Daltonics). Compounds repeatedly identified in pore water samples within the mass-to-charge ratio range underwent internal calibration, with a root mean square error of internal calibration<1 ppm, ensuring reliable molecular formula assignment: 12C (1-60), 1H (2-120), 16O (0-30), 14N (0-3), and 32S (0-2). Furthermore, the molecular formulas were further validated by limiting them to H/C ≤ 2.25 and O/C ≤ 1.2 based on elemental ratios.
To reduce the complexity of the FT-ICR MS spectral analysis, the molecular formulas were categorized based on their elemental composition into four groups: 1) molecular formulas containing C, H, and O atoms (CHO); 2) molecular formulas containing C, H, O, and N atoms (CHON); 3) molecular formulas containing S (CHOS); and 4) molecular formulas containing N and S (CHONS).
Additionally, a modified aromaticity index (AImod) and the ratio of H/C to O/C were used to classify different categories of molecular formulas into six compound groups (Koch and Dittmar, 2006, 2016): 1) saturated fatty acid compounds (H/C > 2, O/C < 0.9); 2) highly unsaturated compounds (AImod < 0.5, H/C < 1.5, O/C < 0.9); 3) aromatic compounds (0.67 > AImod ≥ 0.5); 4) peptide-like compounds (2 > H/C ≥ 1.5, O/C < 0.9, N > 0); 5) unsaturated aliphatic compounds (2 ≥ H/C > 1.5, O/C < 0.9, N = 0); and 6) the CHO-formulas matching the molecules were identified as carboxyl-rich alicyclic molecules (CRAM) (0.3 < DBE/C < 0.68, 0.2< DBE/H < 0.95, 0.77 < DBE/O < 1.75). Labile components generally exhibit low degree of unsaturation and aromaticity with elevated H/C, which are mostly bioavailable. Recalcitrant DOM molecules are characterized by high aromaticity and unsaturation and an increase in structural complexity, rendering them resistant to microbial degradation. Here, we classified a series of compounds as labile DOM compounds, including saturated fatty acids, peptides, and unsaturated aliphatic compounds that exhibited low aromaticity and unsaturation. In contrast, aromatic compounds, highly unsaturated compounds, and CRAM were classified as recalcitrant compounds (Hertkorn et al., 2006; Koch and Dittmar, 2006; Rossel et al., 2016). Additionally, the “island of stability” (IOS) represents a specific range of elemental combinations (H/C = 1.17 ± 0.13, O/C = 0.52 ± 0.10, MW = 360 ± 28 and 497 ± 51 Da) where compounds persist over geological timescales for 1.5 times the average residence time of DOC (Lechtenfeld et al., 2014). The AImod values were calculated as follows:
The overall stability of DOM was evaluated by calculating the molecular stability index (MLBL, the number of formulas with H/C > 1.5 divided by the total number of formulas) (D’Andrilli et al., 2015). Each sample was normalized to the sum of FT-ICR MS peak intensities. Then, the weighted average values of molecular parameters and the proportions of the relative intensity of different classes of molecular formulas were calculated. The number proportion was obtained by dividing the number of each chemical formula type by the total number of chemical formulas.
3 Results
3.1 Molecular diversity of DOM
3.1.1 Molecular formulas of DOM at the "Challenger" Deep and abyssal plain
A total of 8,716 different molecules were detected, with molecular weights mainly concentrated in the range of 150~600 Da. Porewater DOM at Site MT02 contained 7,405 different molecular formulas, and porewater DOM at Site MT04 contained 6,217 different molecular formulas (Table 1).
The proportions of molecular formulas of the four formula groups (CHO, CHON, CHOS, and CHONS) in the DOM samples from the two sites were similar, but there were significant differences in the relative intensities (Figure 2). The relative intensities of the samples containing CHO, CHNO, CHONS, and CHOS chemical formulas in the pore water DOM of Site MT02 were 68.4%, 21.9%, 2.0%, and 7.7%, while those at Site MT04 were 62.7%, 21.4%, 1.8%, and 14.1%, respectively (Figure 2B). Notably, the relative proportion of CHO chemical formulas of the DOM from both sites was greatly lower than that of CHNO chemical formulas (~11%), while their relative intensities were markedly higher than those of CHNO chemical formulas and other heteroatomic chemical formulas (> 40%). The relative intensity of the CHO chemical formula in the DOM of Site MT02 is significantly higher than that of Site MT04 (Figure 2). The relative intensities of S-containing compounds (CHONS & CHOS) of DOM at Site MT02 were lower than Site MT04, while the relative intensities of N-containing compounds (CHONS & CHNO) of DOM were similar (~23%) (Figure 2B).
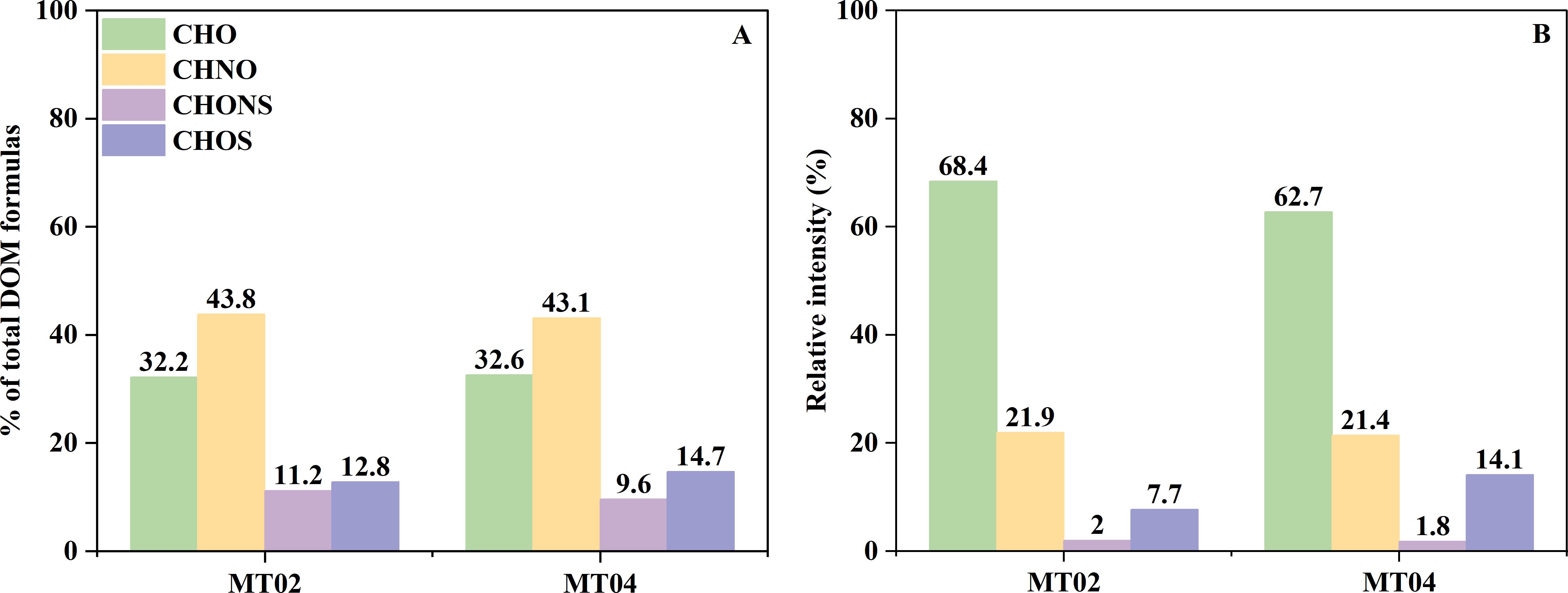
Figure 2. The relative proportions (A) and intensities (B) of the four formula groups (CHO, CHON, CHONS and CHOS) in two sites. Types of molecular formula and relevant parameters at the "Challenger" Deep and abyssal plain sites.
The relative intensities of the highly unsaturated and CRAM molecular formulas in the porewater DOM were 79.7% and 57.1% at Site MT02 and 68.3% and 51.5% at Site MT04, respectively (Figure 3B). The proportion of the most stable IOS compounds in the pore water DOM at Site MT02 (5.9%) was lower than that at Site MT04 (6.7%), but the relative intensity at Site MT02 (15.3%) was higher than that at MT04 (12.9%). The proportions and relative intensities of saturated fatty acids and unsaturated aliphatic compounds, which represent two types of relatively labile compounds, were low; however, their relative intensities at Site MT04 are significantly higher than those at Site MT02. Overall, the proportions and relative intensities of refractory compounds of the pore water DOM in Site MT02 are 81.5% and 84.3%, which were higher than those at Site MT04 (74.0% and 73.6%, respectively) (Figures 3C, D).
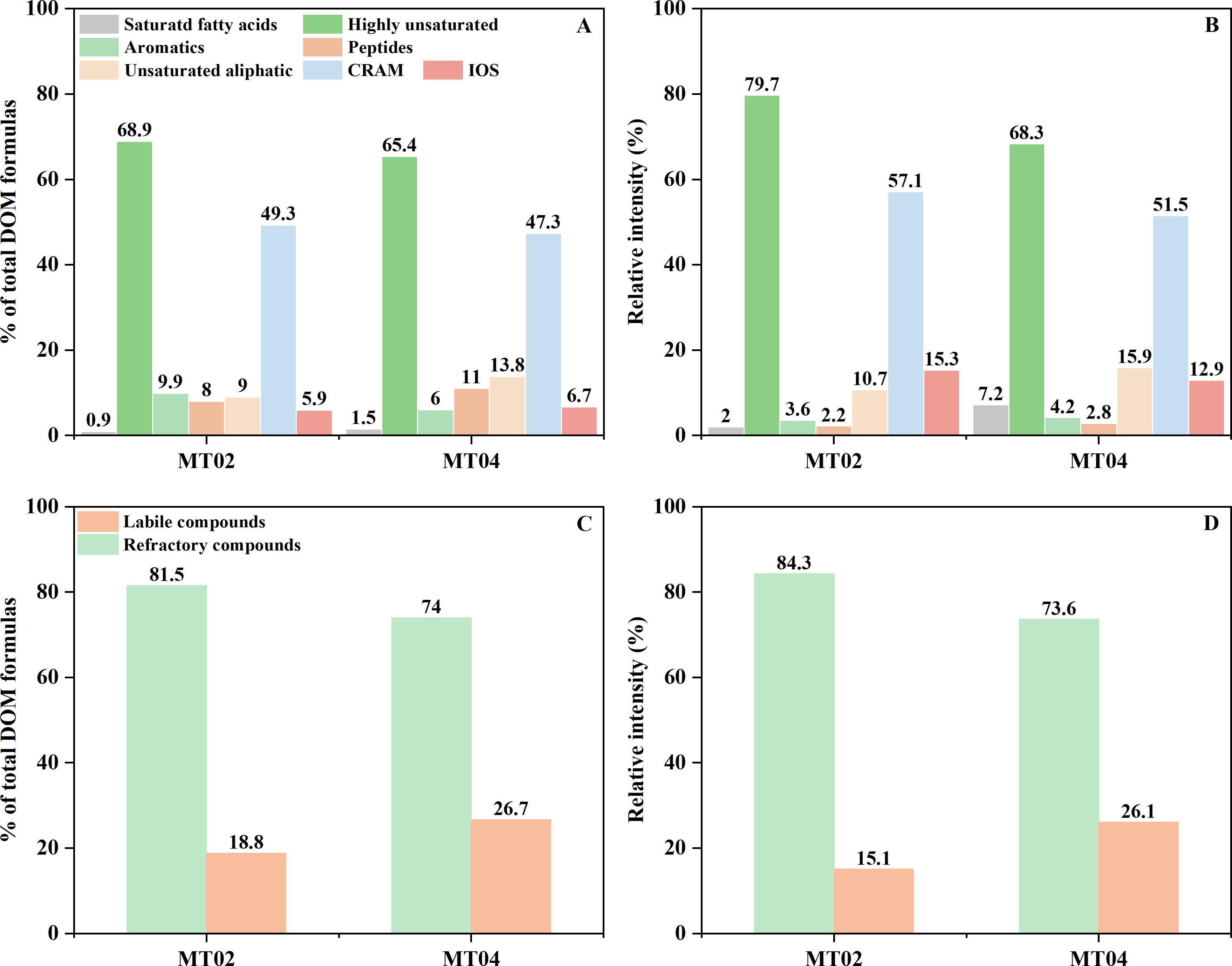
Figure 3. The relative proportions (A) and intensities (B) of compounds at the two cores. The relative proportions (C) and intensities (D) of labile and refractory compounds among all formulas at the two cores. Given that there exists overlap between labile and refractory compounds, the sum of their relative fractions was unequal to 100%.
The Mwa in the pore water DOM samples from Site MT02 was higher than Site MT04. The values of C and N were similar at both sites, but the values of H (Hwa = 22.18) and S (Swa = 0.10) at Site MT02 were lower than those at Site MT04 (Hwa = 23.84; Swa = 0.17), while the value of O (Owa = 8.87) was slightly higher compared to Site MT04 (Owa = 8.11) (Table 1). Compared to Site MT04, the DOM from the "Challenger" Deep Site MT02 had higher values of AImodwa and O/Cwa and lower values of MLBL% and H/Cwa (Table 1).
3.2 Unique formulas of DOM in the "Challenger" Deep and abyssal plain cores
The pore water DOM of Site MT02 and Site MT04 contained 4,906 common molecular formulas. After excluding the common formulas from the total formulas at each site, there were 2,499 unique formulas at Site MT02 and 1,311 unique formulas at Site MT04. The DOM from Site MT02 had 1,188 unique formulas more than Site MT04, indicating a greater diversity of compound types which were mainly refractory highly unsaturated and CRAM compounds. The relative intensities of these two refractory compounds types with lower H/C and higher O/C ratios (Figure 4) at Site MT02 (70.4% and 51.9%, respectively) were significantly higher than those at Site MT04 (38.9% and 29.7%, respectively) (Figure 5). In contrast to refractory compounds, the relative intensities of peptide and unsaturated aliphatic labile compounds were lower in the unique formulas of Site MT02 compared to those of Site MT04 (Figures 5A, 5B), with higher H/C and lower O/C ratios (Figure 4). Additionally, the relative intensities of refractory compounds with CHO formulas in the unique formulas of the pore water DOM at Site MT02 was 17.2%, and the relative intensities of labile compounds were 4.9% (Figure 5C), which were significantly higher than those formulas composed of the other elements and unique formulas of Site MT04 (Figures 5C, D).
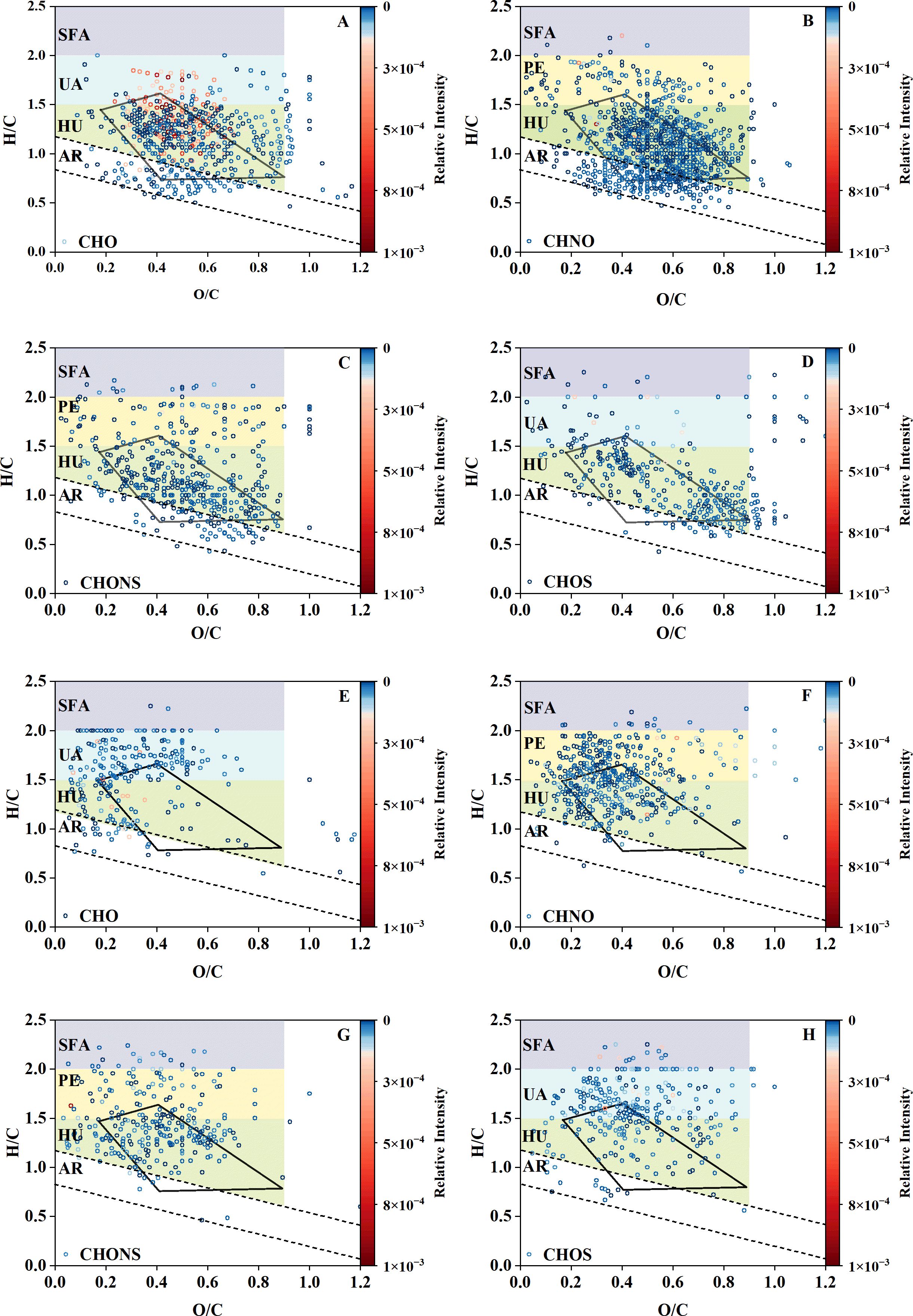
Figure 4. The V-K diagram of the unique formulas at Site MT02 (A-D) and Site MT04 (E-H). The typical compounds were represented by the color shades of different regions (AR, aromatics; HU, highly unsaturated compounds; SFA, saturated fatty acids; UA, unsaturated aliphatic compounds and PE, peptides). The polygon areas delineated by solid lines indicate CRAM compounds. The color code of each datapoint represents the relative intensity of the formulas.
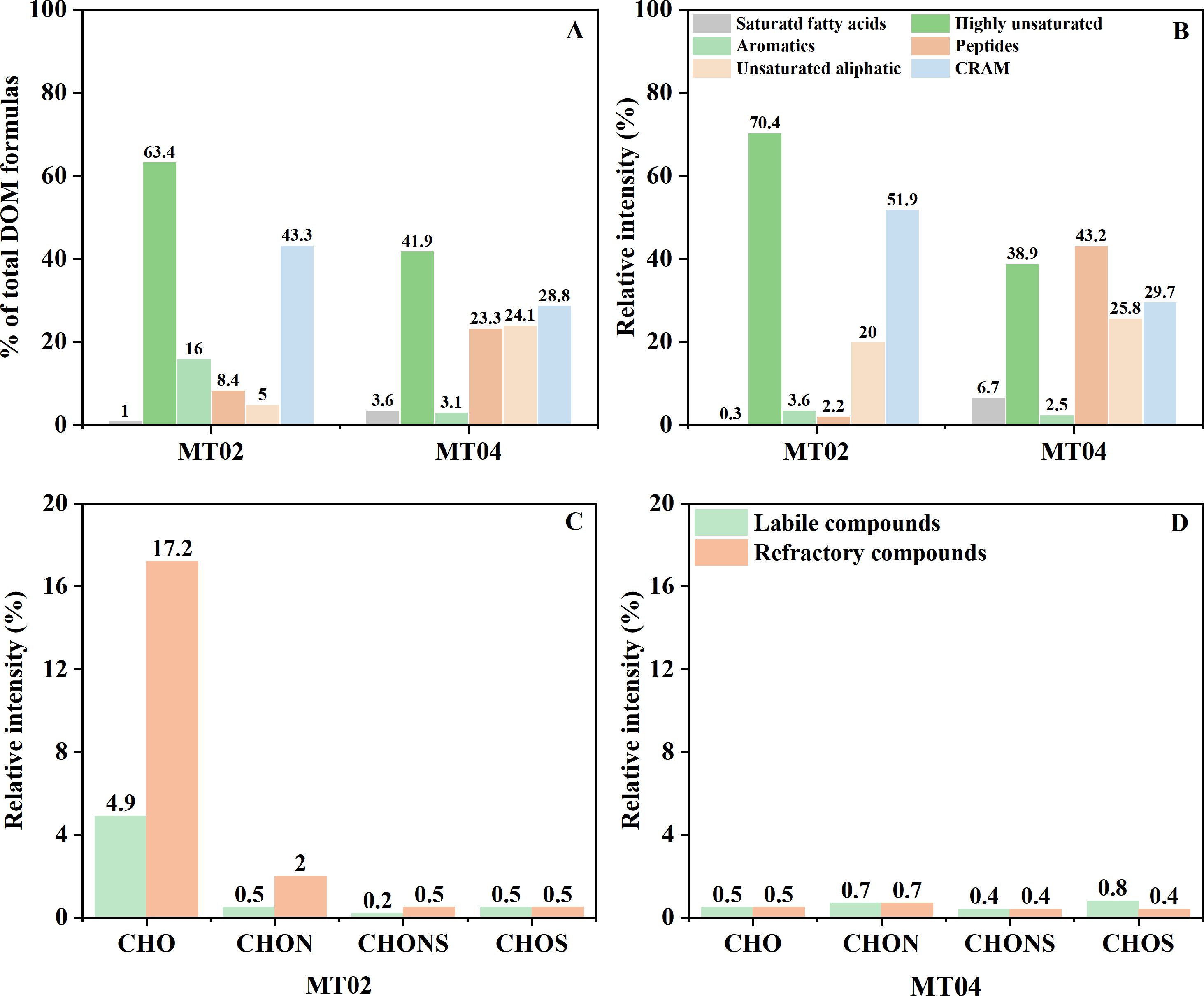
Figure 5. The relative proportions and intensities of unique formulas in the pore water DOM at the two sites MT02 (A) and MT04 (B). The relative intensities of the four formula groups in labile and refractory formulas (C, D).
4 Discussion
4.1 Production and accumulation of more refractory DOM components in the "Challenger" Deep sediments caused by intensified microbial activity
Sedimentary DOM is produced by microbial utilization of particulate organic matter (Komada et al., 2013; Burdige and Komada, 2015). Highly unsaturated compounds and CRAM are ubiquitous because they are resistant to be degraded (Medeiros et al., 2015; Martínez-Pérez et al., 2017). The axis of the Mariana Trench exhibited a higher mineralization rate and stronger microbial activity compared to the abyssal plain (Glud et al., 2013; Wenzhöfer et al., 2016; Luo et al., 2018). The elevated microbial carbon turnover led to a production of more highly degraded and persistent DOM fractions as indicated by the increase in humification indices and absorbance ratios at the trench axis cores (Hu et al., 2021).
The porewater SPE-DOM at both sites consisted mainly of highly unsaturated compounds and CRAM (Figures 3A, B). However, both the proportion of total molecular numbers and relative intensity of these two groups of recalcitrant compounds were significantly higher at the "Challenger" Deep Site MT02 than those in the abyssal plain Site MT04 (Figures 3C, D), especially the relative intensities of the extremely recalcitrant IOS compounds (Figures 3A, B). This suggests that the enhanced microbial activity at the trench axis may lead to increased production and accumulation of refractory DOM.
Studies have shown that complex substrates and microbial diversity contributed to the molecular diversity of DOM (Chen et al., 2022). The number of pore water DOM unique formulas at the "Challenger" Deep Site MT02 is significantly higher than the abyssal plain Site MT04. Moreover, the unique formulas types of DOM in the trench axis sediments were mainly highly unsaturated compounds and CRAM (Figures 4A-D), while he abyssal sediments contained more relatively labile peptides and unsaturated aliphatic compounds (Figures 4E-H). The difference in the porewater DOM molecular compositions may be associated with the diverse microbial communities between the trench axis and abyssal plain sediments (Glud et al., 2013; Nunoura et al., 2015; Wu et al., 2023). The alternative explanation could be the presence of giant diatom debris in the trench axis sediments. It has been suggested that the hydrolysis and degradation of hydrocarbon compounds in the giant diatom-bearing sediments in the southern Mariana Trench sediments could lead to the release of CHO-containing DOM components into porewater (Hu et al., 2024). Therefore, the enhanced microbial activity in the "Challenger" Deep likely generate a higher abundance of refractory compounds, mainly CHO-containing highly unsaturated compounds and CRAM.
4.2 The molecular characteristics of SPE-DOM is likely impacted by sedimentary TOC contents
The molecular composition of sediment pore water DOM at different sites and water depths is highly diverse (Schmidt et al., 2017). Understanding the potential controlling factors of these molecular compositions is crucial as it can provide insights into the biogeochemical processes and environmental conditions in different marine settings. We performed principal component analysis (PCA) >of our DOM data with published data at water depths from 30 to 2,300 m (Schmidt et al., 2017; Hu et al., 2023) and those from the seaward slope of the Mariana Trench at ~7,000 m water depth (Hu et al., 2024) (Table 2; Figures 6A, B). Molecular parameters incorporated in PCA were all abundance-weighted averages. Considering the potential offset in molecular parameters due to instrument differences, prior to PCA analysis, we applied independent sample t-tests to identify parameters that may have been greatly affected by different instruments (see Supplementary materials). To ensure that PCA analysis mainly reflects the real differences between samples, parameters significantly affected by instrument differences were not used in PCA analysis. Ultimately, H/Cwa, AImodwa, and Nwa were included in PCA analysis.
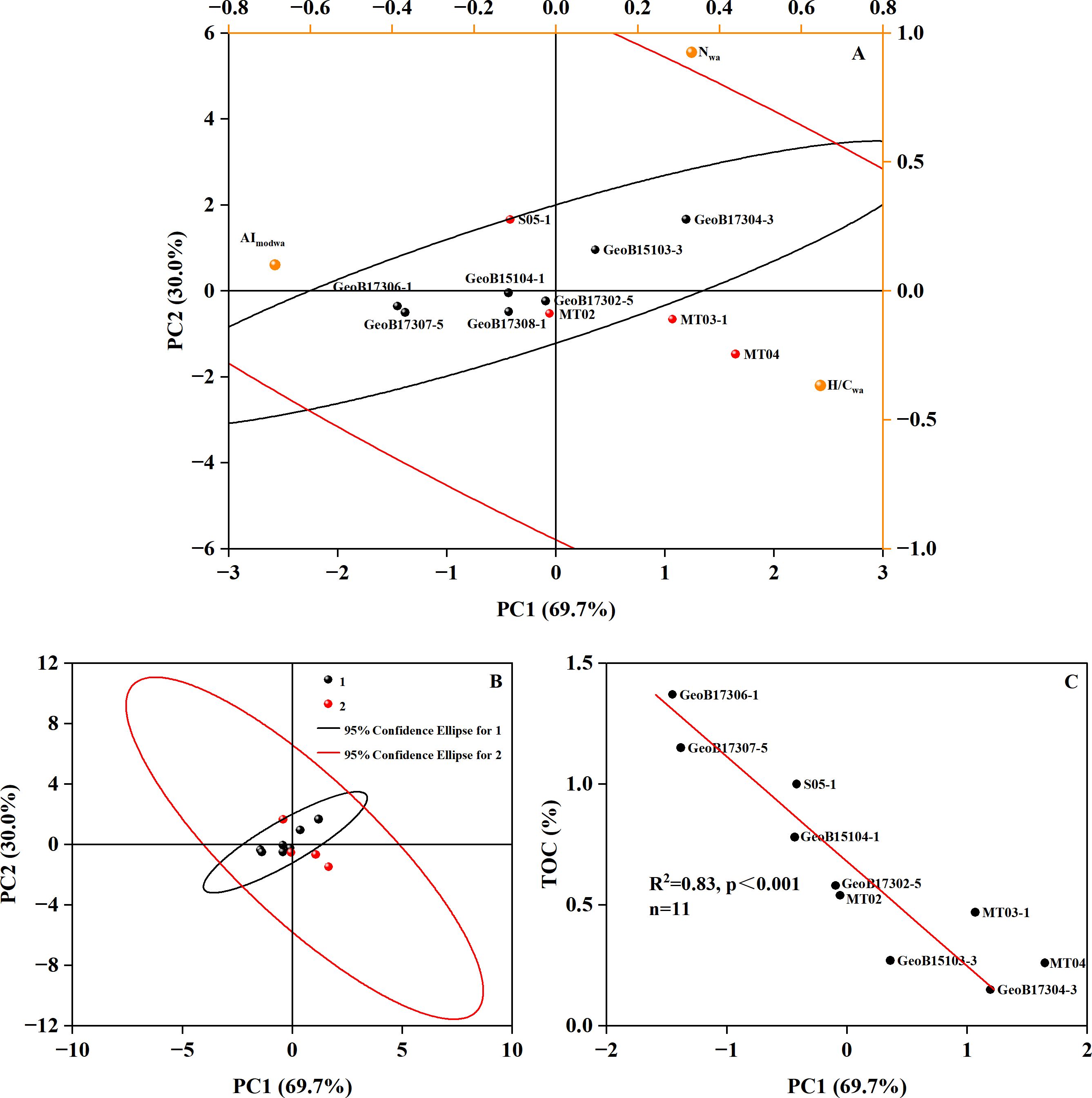
Figure 6. Principal component analysis of molecular parameters of pore water DOM. Biplot of PCA (A), score plot of PCA (B), and relationship between TOC and PC1 score (C). The black dots are the data reported by Schmidt et al. (2017) (Group 1). The red dots represent data from Hu et al. (2024, 2023), the "Challenger" Deep Site MT02 and the adjacent abyssal plain Site MT04 (Group 2).
The PCA results show that our data and those reported in Schmidt et al. (2017) do not exhibit obvious grouping (Figure 6); both located within the 95% confidence interval ellipse of Group 2, likely suggesting a small influence of instrument differences on analytical results. In addition, K-means cluster analysis was used to verify that three parameters used for PCA analysis were not impacted by artefacts among instruments (see Supplementary materials). The sample from the "Challenger" Deep core MT02 is distributed within the confidence interval ellipse of Group 1, while MT04 and MT03-1 are outside (Figures 6A, B). This implies that the "Challenger" Deep sample is more similar to samples from shallower water (<2,300 m) than to those from the abyssal plain (5,800 m) and seaward slope (~7,000 m). The correlation analysis shows that the TOC content in surface sediments at different water depths has a significantly negative correlation with the first principal component (PC1) (Figure 6C). This suggests that the TOC content may be a key factor leading to the differences in the molecular composition of the pore water DOM among different marine environments.
Correlation analysis of the nine datapoints located within the confidence interval ellipse of Group 1 showed that H/Cwa was significantly negatively correlated with TOC content, and that AImodwa was significantly positively correlated with TOC content (Figure 7; red lines). This further suggests that TOC exerts an important control on the molecular composition of DOM. However, when the two datapoints outside the confidence interval ellipse of Group 1 were included in the regression analysis, the overall correlations were significantly reduced (Figure 7; black lines). This is mostly due to higher H/Cwa ratios and lower AImodwa in the abyssal plain (MT04) and seaward slope (MT03) than those in the trench axis (MT02), which is likely associated enhanced microbial activity and transformation of organic matter (Glud et al., 2013, 2021; Wenzhöfer et al., 2016; Hu et al., 2021). Through comparisons with published data, we suggest that TOC content is likely a key controlling factor of pore water DOM characteristics among different environments and that microbial transformation can also play a role.
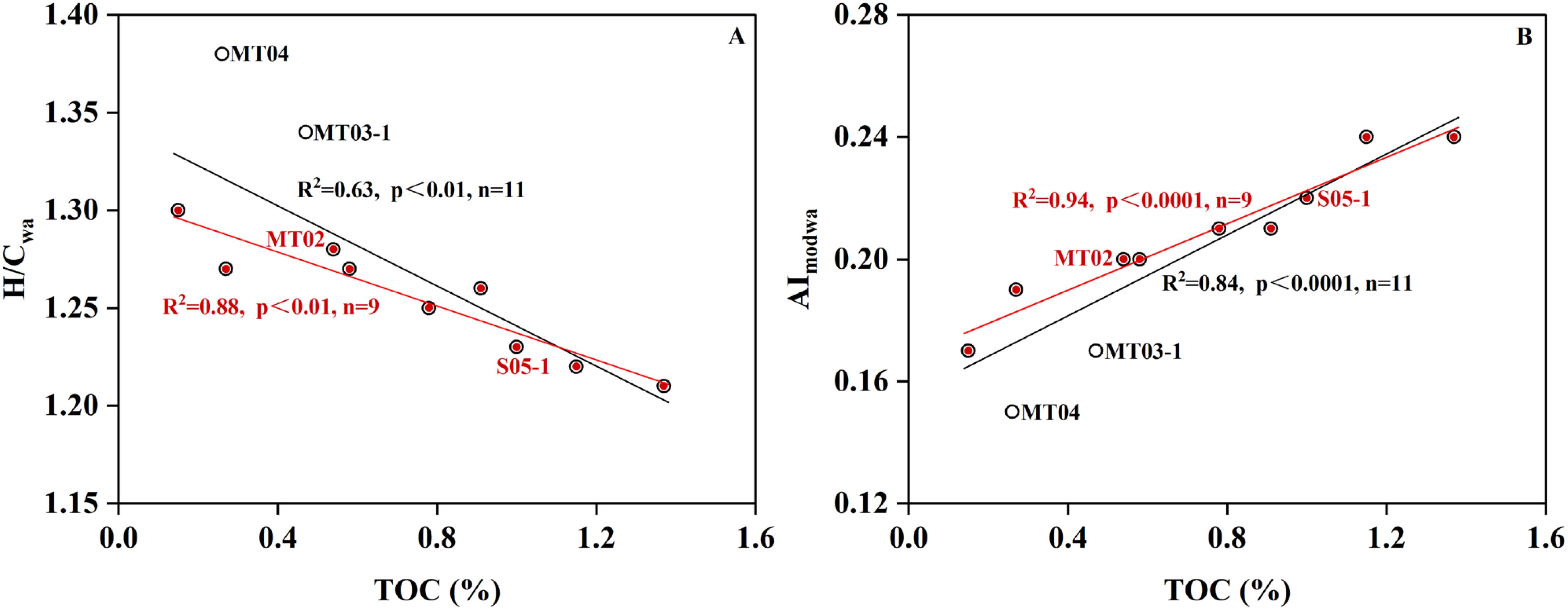
Figure 7. Relationship between TOC and H/Cwa (A) and AImodwa(B). Data from Hu et al. (2023, 2024) and Schmidt et al. (2017) were compiled for regression analysis. The red and black lines represent linear regressions of data with or without MT04 and MT03-1.
5 Conclusion
This is the first study that documented the molecular composition of porewater DOM in the sediments of the ocean’s deepest part. Compared to its adjacent abyssal plain at a water depth of 5,800 m, higher abundance of highly unsaturated compounds and CRAM in DOM contributes to the enhanced refractory nature of porewater DOM in the "Challenger" Deep sediments. This phenomenon is related to the stronger microbial respiration likely caused by high TOC content. Our findings have implications for understanding the microbial-mediated POM degradation processes and the biogeochemical cycling of organic carbon in hadal trench settings.
Data availability statement
The original contributions presented in the study are included in the article/Supplementary Material. Further inquiries can be directed to the corresponding authors.
Author contributions
KZ: Writing – original draft, Visualization, Methodology, Investigation, Formal analysis, Data curation. TH: Validation, Methodology, Formal analysis, Writing – review & editing, Investigation. ML: Writing – review & editing, Supervision, Investigation, Funding acquisition. LC: Writing – review & editing, Methodology, Formal analysis. YQ: Writing – review & editing, Methodology, Formal analysis. JX: Writing – review & editing, Formal analysis. DC: Writing – review & editing, Supervision, Funding acquisition.
Funding
The author(s) declare financial support was received for the research, authorship, and/or publication of this article. This work was financially supported by the National Natural Science Foundation of China (42176069, 41806126 and 42406036), Shuguang Program (grant 23SG44) of Shanghai Education Development Foundation and Shanghai Municipal Education Commission, and the Science and Technology Innovation Fund for College Students of Luo Zhaorao College of Shanghai Ocean University (A1-2004-22-201317).
Acknowledgments
We thank the captain and crews of RV Tansuoyihao for their invaluable help with coring during the expedition. Thanks to Chen Xiaoxia from Xiamen University for her suggestions on data processing and analysis. Prof. Harunur Rashid from Shanghai Ocean University is thanked for carefully reading the initial version of the manuscript.
Conflict of interest
The authors declare that the research was conducted in the absence of any commercial or financial relationships that could be construed as a potential conflict of interest.
Publisher’s note
All claims expressed in this article are solely those of the authors and do not necessarily represent those of their affiliated organizations, or those of the publisher, the editors and the reviewers. Any product that may be evaluated in this article, or claim that may be made by its manufacturer, is not guaranteed or endorsed by the publisher.
Supplementary material
The Supplementary Material for this article can be found online at: https://www.frontiersin.org/articles/10.3389/fmars.2024.1469547/full#supplementary-material
References
Blair N., Aller R. (2012). The fate of terrestrial organic carbon in the marine environment. Annu. Rev. Mar. Sci. 4, 401–423. doi: 10.1146/annurev-marine-120709-142717
Burdige D. J. (2007). Preservation of organic matter in marine sediments: controls, mechanisms, and an imbalance in sediment organic carbon budgets? Chem. Rev. 107, 467. doi: 10.1021/cr050347q
Burdige D. J., Komada T. (2015). “Chapter 12 - sediment pore waters,” in Biogeochemistry of marine dissolved organic matter, 2nd. Eds. Hansell D. A., Carlson C. A (Academic Press, Boston), 535–577. doi: 10.1016/B978-0-12-405940-5.00012-1
Chen M., Hur J. (2015). Pre-treatments, characteristics, and biogeochemical dynamics of dissolved organic matter in sediments: A review. Water Res. 79, 10–25. doi: 10.1016/j.watres.2015.04.018
Chen M., Kim J.-H., Nam S.-I., Niessen F., Hong W.-L., Kang M.-H., et al. (2016). Production of fluorescent dissolved organic matter in Arctic Ocean sediments. Sci. Rep. 6, 39213. doi: 10.1038/srep39213
Chen Q., Lønborg C., Chen F., Gonsior M., Li Y., Cai R., et al. (2022). Increased microbial and substrate complexity result in higher molecular diversity of the dissolved organic matter pool. Limnology Oceanography 67, 2360–2373. doi: 10.1002/lno.12206
D’Andrilli J., Cooper W. T., Foreman C. M., Marshall A. G. (2015). An ultrahigh-resolution mass spectrometry index to estimate natural organic matter lability. Rapid Commun. Mass Spectrometry 29, 2385–2401. doi: 10.1002/rcm.7400
Dittmar T., Koch B., Hertkorn N., Kattner G. (2008). A simple and efficient method for the solid-phase extraction of dissolved organic matter (SPE-DOM) from seawater. Limnology Oceanography: Methods 6, 230–235. doi: 10.4319/lom.2008.6.230
Glud R. N., Berg P., Thamdrup B., Larsen M., Stewart H. A., Jamieson A. J., et al. (2021). Hadal trenches are dynamic hotspots for early diagenesis in the deep sea. Commun. Earth Environ. 2, 1–8. doi: 10.1038/s43247-020-00087-2
Glud R. N., Wenzhöfer F., Middelboe M., Oguri K., Turnewitsch R., Canfield D. E., et al. (2013). High rates of microbial carbon turnover in sediments in the deepest oceanic trench on Earth. Nat. Geosci. 6, 284–288. doi: 10.1038/ngeo1773
Hansell D. A., Carlson C. A., Repeta D. J., Schlitzer R. (2009). Dissolved organic matter in the ocean: A controversy stimulates new insights. Oceanography 22, 202–211. doi: 10.5670/oceanog.2009.109
Hertkorn N., Benner R., Frommberger M., Schmitt-Kopplin P., Witt M., Kaiser K., et al. (2006). Characterization of a major refractory component of marine dissolved organic matter. Geochimica Cosmochimica Acta 70, 2990–3010. doi: 10.1016/j.gca.2006.03.021
Hu T., Luo M., Qi Y., He D., Chen L., Xu Y., et al. (2023). Molecular evidence for the production of labile, sulfur-bearing dissolved organic matter in the seep sediments of the South China Sea. Water Res. 233, 119732. doi: 10.1016/j.watres.2023.119732
Hu T., Luo M., Wünsch U. J., He D., Gieskes J., Xu Y., et al. (2021). Probing sedimentary DOM in the deepest sector of Earth’s surface. Mar. Chem. 237, 104033. doi: 10.1016/j.marchem.2021.104033
Hu T., Zheng K., Luo M., Xie J., Qi Y., Xu Y., et al. (2024). Probing the optical and molecular properties of sedimentary dissolved organic matter in the laminated diatom mats from the southern Mariana Trench. Global Planetary Change 234, 104386. doi: 10.1016/j.gloplacha.2024.104386
Koch B. P., Dittmar T. (2006). From mass to structure: an aromaticity index for high-resolution mass data of natural organic matter. Rapid Commun. Mass Spectrometry 20, 926–932. doi: 10.1002/rcm.2386
Koch B. P., Dittmar T. (2016). From mass to structure: an aromaticity index for high-resolution mass data of natural organic matter. Rapid Commun. Mass Spectrometry 30, 250–250. doi: 10.1002/rcm.7433
Koch B. P., Witt M., Engbrodt R., Dittmar T., Kattner G. (2005). Molecular formulae of marine and terrigenous dissolved organic matter detected by electrospray ionization Fourier transform ion cyclotron resonance mass spectrometry. Geochimica Cosmochimica Acta 69, 3299–3308. doi: 10.1016/j.gca.2005.02.027
Komada T., Burdige D. J., Crispo S. M., Druffel E. R. M., Griffin S., Johnson L., et al. (2013). Dissolved organic carbon dynamics in anaerobic sediments of the Santa Monica Basin. Geochimica Cosmochimica Acta 110, 253–273. doi: 10.1016/j.gca.2013.02.017
Lechtenfeld O. J., Kattner G., Flerus R., McCallister S. L., Schmitt-Kopplin P., Koch B. P. (2014). Molecular transformation and degradation of refractory dissolved organic matter in the Atlantic and Southern Ocean. Geochimica Cosmochimica Acta 126, 321–337. doi: 10.1016/j.gca.2013.11.009
Leduc D., Rowden A. A., Glud R. N., Wenzhöfer F., Kitazato H., Clark M. R. (2016). Comparison between infaunal communities of the deep floor and edge of the Tonga Trench: Possible effects of differences in organic matter supply. Deep Sea Res. Part I: Oceanographic Res. Papers 116, 264–275. doi: 10.1016/j.dsr.2015.11.003
Luo M., Gieskes J., Chen L., Shi X., Chen D. (2017). Provenances, distribution, and accumulation of organic matter in the southern Mariana Trench rim and slope: Implication for carbon cycle and burial in hadal trenches. Mar. Geology 386, 98–106. doi: 10.1016/j.margeo.2017.02.012
Luo M., Glud R. N., Pan B., Wenzhöfer F., Xu Y., Lin G., et al. (2018). Benthic carbon mineralization in hadal trenches: insights from in situ determination of benthic oxygen consumption. Geophysical Res. Lett. 45, 2752–2760. doi: 10.1002/2017GL076232
Luo M., Li W., Geilert S., Dale A. W., Song Z., Chen D. (2022). Active silica diagenesis in the deepest hadal trench sediments. Geophysical Res. Lett. 49, e2022GL099365. doi: 10.1029/2022GL099365
Martínez-Pérez A. M., Nieto-Cid M., Osterholz H., Catalá T. S., Reche I., Dittmar T., et al. (2017). Linking optical and molecular signatures of dissolved organic matter in the Mediterranean Sea. Sci. Rep. 7, 3436. doi: 10.1038/s41598-017-03735-4
Medeiros P. M., Seidel M., Powers L. C., Dittmar T., Hansell D. A., Miller W. L. (2015). Dissolved organic matter composition and photochemical transformations in the northern North Pacific Ocean. Geophysical Res. Lett. 42, 863–870. doi: 10.1002/2014GL062663
Nunoura T., Takaki Y., Hirai M., Shimamura S., Makabe A., Koide O., et al. (2015). Hadal biosphere: Insight into the microbial ecosystem in the deepest ocean on Earth. Proc. Natl. Acad. Sci. 112, E1230–E1236. doi: 10.1073/pnas.1421816112
Oguri K., Kawamura K., Sakaguchi A., Toyofuku T., Kasaya T., Murayama M., et al. (2013). Hadal disturbance in the Japan Trench induced by the 2011 Tohoku–Oki Earthquake. Sci. Rep. 3, 1915. doi: 10.1038/srep01915
Raymond P. A., Spencer R. G. M. (2015). “Chapter 11 - riverine DOM,” in Biogeochemistry of marine dissolved organic matter, 2nd. Eds. Hansell D. A., Carlson C. A. (Academic Press, Boston), 509–533. doi: 10.1016/B978-0-12-405940-5.00011-X
Rossel P. E., Bienhold C., Boetius A., Dittmar T. (2016). Dissolved organic matter in pore water of Arctic Ocean sediments: Environmental influence on molecular composition. Organic Geochemistry 97, 41–52. doi: 10.1016/j.orggeochem.2016.04.003
Schmidt F., Koch B. P., Goldhammer T., Elvert M., Witt M., Lin Y. S., et al. (2017). Unraveling signatures of biogeochemical processes and the depositional setting in the molecular composition of pore water DOM across different marine environments. Geochimica Cosmochimica Acta 207, 57–80. doi: 10.1016/j.gca.2017.03.005
Song Z., Meng F., Li W., Chen L., Luo M. (2022). Preliminary study on source and sedimentary environment in the Mariana Trench. Mar. Geology Quaternary Geology 42, 1–17. doi: 10.16562/j.cnki.0256-1492.2022031801 (in Chinese with English abstract).
Wenzhöfer F., Oguri K., Middelboe M., Turnewitsch R., Toyofuku T., Kitazato H., et al. (2016). Benthic carbon mineralization in hadal trenches: Assessment by in situ O2 microprofile measurements. Deep Sea Res. Part I: Oceanographic Res. Papers 116, 276–286. doi: 10.1016/j.dsr.2016.08.013
Wu J., Wang L., Du J., Liu Y., Hu L., Wei H., et al. (2023). Biogeographic distribution, ecotype partitioning and controlling factors of Chloroflexi in the sediments of six hadal trenches of the Pacific Ocean. Sci. Total Environ. 880, 163323. doi: 10.1016/j.scitotenv.2023.163323
Keywords: molecular characteristics, dissolved organic matter, “Challenger” Deep, Mariana Trench, FT-ICR MS
Citation: Zheng K, Hu T, Luo M, Chen L, Qi Y, Xie J and Chen D (2024) Molecular characteristics of dissolved organic matter in the porewater of "Challenger" Deep sediments, Mariana Trench. Front. Mar. Sci. 11:1469547. doi: 10.3389/fmars.2024.1469547
Received: 24 July 2024; Accepted: 07 October 2024;
Published: 12 November 2024.
Edited by:
Guangchao Zhuang, Ocean University of China, ChinaCopyright © 2024 Zheng, Hu, Luo, Chen, Qi, Xie and Chen. This is an open-access article distributed under the terms of the Creative Commons Attribution License (CC BY). The use, distribution or reproduction in other forums is permitted, provided the original author(s) and the copyright owner(s) are credited and that the original publication in this journal is cited, in accordance with accepted academic practice. No use, distribution or reproduction is permitted which does not comply with these terms.
*Correspondence: Min Luo, bWx1b0BzaG91LmVkdS5jbg==; Duofu Chen, ZGZjaGVuQHNob3UuZWR1LmNu