- 1Marine Mammal and Turtle Division, Southeast Fisheries Science Center, National Marine Fisheries Service, Miami, FL, United States
- 2Marine Mammal and Sea Turtle Conservation Division, Office of Protected Resources, National Marine Fisheries Service, Silver Spring, MD, United States
- 3Cooperative Institute of Marine and Atmospheric Studies, University of Miami, Miami, FL, United States
Vessel strikes are a substantial source of mortality for large whales worldwide and may pose conservation threats for small populations. Model-based estimates of mortality rates, which inform management strategies to reduce vessel strike mortality, typically assume a reduced likelihood that a whale-vessel collision will be lethal to the whale at slower vessel speeds. In this study, we reviewed and updated available data on observed whale-vessel interactions in U.S. waters and developed a new model characterizing the probability that an interaction will be lethal to the whale as a function of vessel speed, length (as a proxy for mass), and whale taxon. We found a significant effect of vessel size class on the probability of lethality. In addition, decreasing vessel speeds reduced the likelihood of a lethal outcome for all vessel size classes, but this effect was strongest for vessels less than 108m in length. The probability that a strike by a very large ocean-going vessel will be lethal exceeded 0.80 at all speeds above 5 knots. Whale taxon also affected both the likelihood of a lethal strike and the effect of vessel speed. Humpback whales (Megaptera novaeangliae) had significantly lower rates of lethal strikes compared to other large whales. This difference may be associated with data limitations, differing behavioral responses between species, varying vessel types between regions or differences in body composition and blubber thickness. The model is consistent with biophysical models that demonstrate a high rate of strike lethality for large vessels with high masses. Vessel speed restrictions are one of the primary approaches to reduce the risk of vessel strikes to whales in the face of continued industrialization of the oceans, and the model presented here will help better inform management efforts.
1 Introduction
Vessel strikes of cetaceans have long been documented throughout the world’s oceans. Rapid increases in commercial shipping between 1950 and 1980 coincided with an increase in the number of vessel strikes of large whales (Laist et al., 2001), and the number of case records in the International Whaling Commission (IWC) ship strike database increased in the early 1990s and has remained high through the present (Winkler et al., 2020). Vessel strikes impact at least 36 species of cetaceans (Winkler et al., 2020). Mysticetes account for the majority of the reported vessel strikes in the IWC’s database, with fin whales (Balaenoptera physalus) and humpback whales (Megaptera novaeangliae) representing the most commonly reported species (Winkler et al., 2020). A global review documented vessel strike mortality to at least 11 species of Mysticetes (Schoeman et al., 2020) including several species listed as Endangered under the International Union for Conservation of Nature (IUCN): blue whales (Balaenoptera musculus, e.g., Redfern et al., 2019), sei whales (Balaenoptera borealis, e.g., Carrillo and Ritter, 2023), and North Atlantic right whales (Eubalaena glacialis, e.g., Sharp et al., 2019). This source of anthropogenic mortality remains a significant threat to the conservation of small and endangered Mysticete populations around the world (IWC, 2022).
In both Atlantic and Pacific waters of the United States, vessel strikes are a significant source of mortality for populations of Mysticete whales including several listed under the U.S. Endangered Species Act (Carretta et al., 2022; Hayes et al., 2022). Assessments of the spatial and temporal risk of vessel strikes have been conducted for humpback whale, blue whale, and fin whale populations in regions along the U.S. west coast (Rockwood et al., 2017; Redfern et al., 2020; Rockwood et al., 2020) using encounter theory models (Martin et al., 2016) to estimate mortality due to vessel strikes. For the eastern North Pacific blue whale stock, model studies suggest that annual vessel strike mortality is approximately 18 whales per year, which is substantially higher than the number of detected vessel strikes of approximately 0.8 whales per year (Carretta et al., 2022). Interannual variability in both vessel traffic patterns and whale spatial distribution can result in significant variation in the magnitude and location of vessel strike risk (Blondin et al., 2020; Redfern et al., 2020). Voluntary reductions in vessel speeds associated with incentives to reduce greenhouse gas emissions are expected to decrease the risk of vessel strikes; however, expanded areas with reduced vessel speeds and routing measures to reduce spatiotemporal overlap between whales and vessels may be needed to further reduce mortality rates (Redfern et al., 2020; Rockwood et al., 2020).
On the east coast of the U.S., mortalities due to vessel strikes are significant for the Gulf of Maine stock of humpback whales and North Atlantic right whales (Hayes et al., 2022). For humpback whales, vessel strikes accounted for 36% of the documented human caused mortalities and serious injuries during 2013-2017 (Henry et al., 2020). Vessel strikes also remain a significant source of mortality for North Atlantic right whales accounting for 29% of documented human caused mortalities and serious injuries between 2016-2020 (Hayes et al., 2022). Observed counts of mortalities and serious injuries underestimate the total human caused mortality by 2.8 times, and differences in the detection rates between entanglements in fishing gear and vessel strikes make it difficult to accurately apportion mortality between these two sources (Pace et al., 2021). However, even the observed mean annual number of documented vessel strike mortalities exceeds the Potential Biological Removal benchmark for this small and endangered population (Hayes et al., 2022).
In an effort to reduce the impacts of vessel strikes on North Atlantic right whales, the U.S. National Marine Fisheries Service (NMFS) implemented regulations establishing seasonal management areas (SMAs) along the U.S. east coast that restrict the speed of most vessels of length 19.8 m (65 feet) and longer to 10 knots or less in specific spatial areas during specified times of year when right whales are likely to be present (National Oceanic and Atmospheric Administration, 2008). The NMFS also implemented mandatory and recommended routing measures and voluntary dynamic speed reduction zones concurrent with these regulations (National Oceanic and Atmospheric Administration, 2008). Several subsequent analyses suggested that these efforts reduced mortality inside SMAs (Laist et al., 2014; van der Hoop et al., 2015). Encounter theory models, similar to those in Rockwood et al. (2017), also indicate that speed reductions reduce lethal vessel strikes for North Atlantic right whales (Crum et al., 2019; Garrison et al., 2022; Blondin et al., 2025). A recent analysis by Redfern et al. (2024) using a similar modeling approach demonstrated that expanding the coverage of SMAs to include more of the primary habitat of North Atlantic right whales could reduce the risk of lethal vessel strikes by 18%.
The expected positive relationship between vessel speed and the probability a vessel strike of a large whale will be lethal underlies all of these analytical approaches and associated management actions. Laist et al. (2001) first reviewed global data on vessel strikes of large whales that included information on the vessel type, vessel speed, and the fate of the whale after the strike and concluded that lethal interactions were positively correlated with higher vessel speeds. Vanderlaan and Taggart (2007) further explored these data, and additional records from an expanded dataset (Jensen and Silber, 2003), and developed a logistic regression model to quantify the relationship between vessel speed and strike lethality. Conn and Silber (2013) added additional data and developed a similar model that has since been used as the basis for evaluating the impact of reducing vessel speeds on reducing mortalities of large whales (e.g., Nichol et al., 2017; Rockwood et al., 2017; Crum et al., 2019; Garrison et al., 2022; Redfern et al., 2024).
While these previous studies provided an understanding of the relationship between vessel speed and strike lethality, they did not assess factors aside from vessel speed that may influence whether a vessel strike of a large whale is lethal. In particular, these studies did not assess vessel size or differences between species, largely because of limited sample size and the resulting limited ability to draw inferences. However, recent biophysical models have demonstrated the importance of considering the contribution of vessel mass to the causes of blunt force injuries to large whales (Kelley et al., 2021). This approach suggests that the forces involved in collisions with smaller vessels (i.e., < 20m length) are sufficient at speeds of 10 knots to result in a significant probability of mortality, and that collisions with larger vessels are likely to result in a high probability of a lethal interaction at all speeds (Kelley et al., 2021).
In this study, we gathered and reviewed additional data on vessel strikes of large whales in U.S. waters of the Pacific and Atlantic oceans and developed an updated model of the lethality of vessel strikes to large whales. Our study follows a similar approach to that in Conn and Silber (2013) and builds on their existing dataset. Here we obtain and review additional records of vessel strikes that include information on the fate of the whale following the interaction along with information about the vessels involved (e.g., vessel speed, type, size, etc.). Using these updated data, we developed a logistic regression model and evaluated the importance of several explanatory factors, including vessel speed, vessel size, and whale species in predicting the likelihood of lethality for a given whale-vessel interaction. The resulting updated model can be used to better characterize the risk of lethal vessel strikes in encounter theory models and other approaches used to inform conservation strategies.
2 Materials and methods
2.1 Data acquisition and verification
The data for this analysis consist of reports of whale-vessel interactions in U.S. waters from a range of sources including stranding networks, mariners, and the public. We first obtained the dataset used in Conn and Silber (2013), which included records reported in Laist et al. (2001), Jensen and Silber (2003), and Vanderlaan and Taggart (2007). In addition to these sources, the Conn and Silber (2013) study included 38 additional records derived from Neilson et al. (2012) and additional records from regional National Marine Fisheries Service strandings databases for a total of 90 records through September 2012 (Conn and Silber, 2013). We obtained these records from the study authors (P. Conn, pers. comm.); however, many of the records did not have sufficient information included to match them to records in the strandings databases or the database compiled in Jensen and Silber (2003). We were able to verify the source and link 75 of the 90 records in Conn and Silber (2013) to a current data source and gather additional information on the interaction from these sources.
Additional records on whale-vessel interactions from U.S. waters were obtained from reports and records maintained at the NMFS Northeast Fisheries Science Center (NEFSC; Henry et al., 2015, 2017, 2019, 2020, 2021), Pacific Islands Fisheries Science Center (PIFSC; Lammers et al., 2013; Bradford and Lyman, 2015, 2018, 2019), Alaska Fisheries Science Center (AFSC), and Southwest Fisheries Science Center (SWFSC; Carretta et al., 2020, 2021, 2022). In each case, these are records maintained by the respective offices to support Marine Mammal Protection Act stock assessment reports and determination of serious injuries and mortalities of large whales in the respective regions. Where appropriate, these records are also linked to the NMFS National Marine Mammal Strandings Database (https://www.fisheries.noaa.gov/national/marine-life-distress/national-stranding-database-public-access). Data available through October 2022 were considered for the current analysis and included a total of 291 records (Supplementary Table S1). This dataset included 266 interactions with individual whales, 10 interactions with mother-calf pairs, and two interactions with groups of whales (3 and 2 individuals, respectively). In the case of mother-calf pairs and groups, the same vessel potentially struck more than one individual in the same event.
2.2 Case review and assignment of outcomes
We further refined this dataset by reviewing the associated records and, in some cases, seeking additional information on vessel characteristics to maximize the number of records that included information on the vessel size and type, speed at the time of the interaction, and information about the outcome for the whale. Fourteen records, 13 from the Conn and Silber (2013) dataset and one humpback whale interaction in Hawaii, were excluded because a clear source with supporting documentation could not be determined. Because this analysis focused on U.S. waters, and we did not obtain a complete accounting of international records, we excluded 16 records from outside of U.S. waters. Finally, an additional two records were excluded because they contained no information to identify the vessel type or size category, and one remaining record from the year 1885 was excluded due to concerns with the age of the record and whether or not it is representative of current whale-vessel interactions.
We reviewed the remaining 258 cases (individuals) to evaluate the information on the interaction and to determine if the outcome could be assigned as a severe injury/mortality (“mortality”) or no severe injury/mortality (Figure 1). First, the case was reviewed to determine if a whale-vessel interaction could be confirmed; this resulted in excluding five cases that did not indicate that the whale had actually been struck. These cases included 4 individuals and the adult female from one mother-calf pair. Second, the description of each incident was evaluated to determine if the whale was sufficiently observed following the interaction to allow for an assessment of any injury; this resulted in excluding 52 cases (47 individuals, 2 mother-calf pairs, and the calf from one mother-calf pair) where there was insufficient observation after the interaction. Of the remaining 201 cases, the description was reviewed to assess the evidence for a severe injury or mortality. For this assessment, we followed the same set of criteria described in Vanderlaan and Taggart (2007), Neilson et al. (2012), and Conn and Silber (2013). In particular, if a carcass was observed, blood was observed in the water, or lacerations (aside from those described as superficial) were observed, then the case was categorized as a severe injury/mortality. As in these earlier studies, we considered severe injuries and mortalities to be equivalent since there is limited opportunity for follow up observations in the vast majority of these cases. It should be noted that some injuries, particularly impacts from blunt force trauma, may not have been apparent at the time of observation, and therefore would not be captured in this analysis. Each case was reviewed independently by the study authors for these determinations. In 115 cases, there was no evidence of a severe injury/mortality. For the remaining 86 cases, there were six cases where the individual whale was known through photo-identification data, and the whale was observed weeks to months after the interaction with no apparent injury. As a result, there were 80 cases where a severe injury/mortality was observed, 121 cases where no severe injury/mortality was observed, and 57 cases with an unknown outcome (Figure 1). Details on each case are provided in Supplementary Material (Supplementary Table S1).

Figure 1. Flow chart depicting steps to review available cases (n = 291 individual whales) describing potential whale-vessel interactions. See text for additional detail on each step in the review process. After review, the dataset included 80 cases where a severe injury or mortality occurred (“Yes”), 121 cases with no severe injury or mortality (“No”), and 57 cases where a determination could not be made (“Unknown”).
2.3 Logistic regression analysis
We employed logistic regression (McCullagh and Nelder, 1989; Hosmer et al., 2013) to model the probability that a given vessel-whale interaction will be lethal (i.e., result in a severe injury or mortality or “lethal”) versus non-lethal (e.g., Vanderlaan and Taggart, 2007; Conn and Silber, 2013). Due to the increased number of available individuals (201 with known outcomes) compared to previous analyses, we were able to explore a larger number of potential explanatory variables including vessel speed, vessel size (as measured by vessel length), species/taxon, and region of the U.S. (Atlantic vs. Pacific [including Hawaii and Alaska]).
The 201 individuals included 10 mother-calf pairs and 2 adult groups (2 and 3 individuals, respectively) that interacted with the same vessel at the same time. The outcome for individuals in these groups cannot be considered statistically independent. Therefore, we combined them into independent “events” for the logistic regression analysis. The outcome was the same for all of the individuals in 8 of these events (6 mother-calf pairs, 2 adult groups). For the remaining two mother-calf pairs, an injury could be confirmed for one individual, but it was unknown whether or not the other individual was injured. For these two events, the outcome was assigned as “severe injury/mortality” since at least one animal was observed with an injury. The grouped dataset included 113 events where no mortality/injury occurred and 79 events where at least one individual suffered mortality/injury.
For vessel size, we grouped vessels into four size categories based on reported length or vessel type: 4.6 -12.1 m (<40 feet, “Small”), 12.2 – 19.7 m (≥40-65 feet, “Medium”), 19.8 – 108 m (≥65 - 350 feet, “Large”), and ≥ 108 m (≥ 350 ft, “Extra Large”). The Small and Medium categories align with U.S. Coast Guard small vessel categories (i.e., Class 2 and Class 3 motorboats, respectively; 46 CFR 90.10-23, U.S. Federal Register 30:251 FR 16790), the Large category corresponds to a range of vessel types, and the Extra Large category corresponds to tankers, cargo vessels, and other very large ocean-going vessels (OGV). In cases where the vessel length was not provided (or provided as a range), we placed the vessel into a size category using vessel type or other descriptive information.
Eight species of large whales are represented in our dataset plus an unidentified large whale category (Table 1). Of the 201 individuals where severe injury/mortality could be assigned, 133 were interactions with humpback whales. Therefore, species were categorized as humpback vs. other species in the logistic regression model.
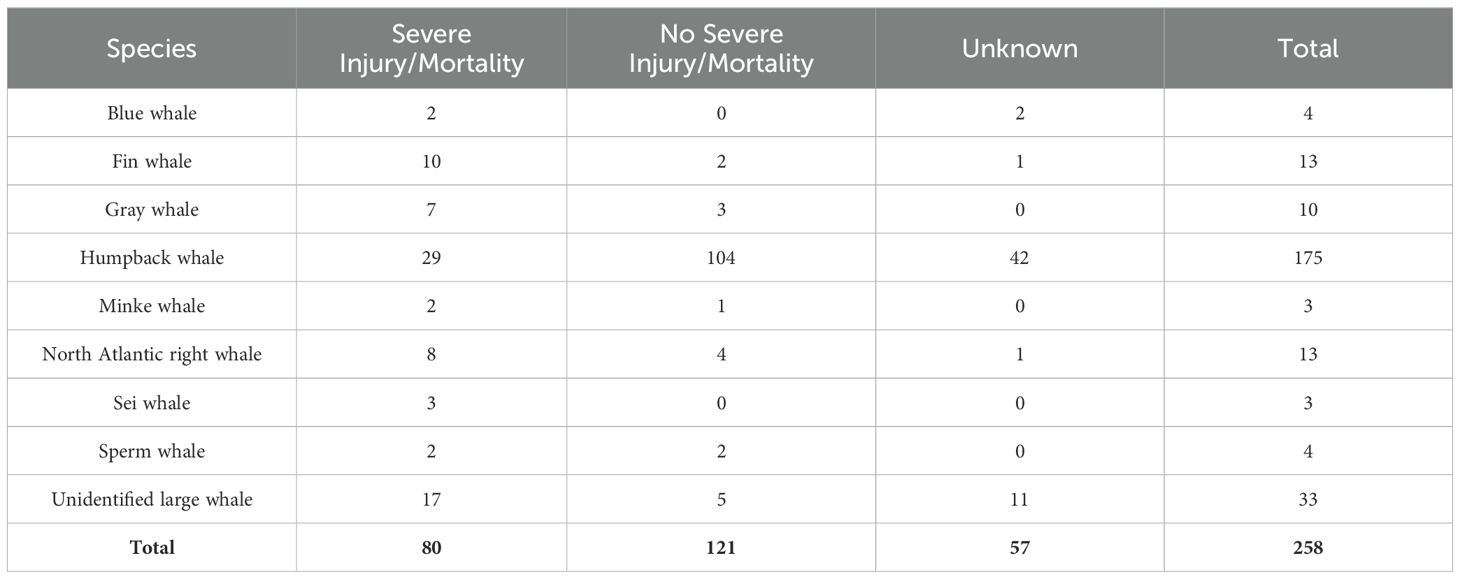
Table 1. Whale-vessel interactions and assigned severe injury or mortality status by large whale species.
While it would be desirable to explore all possible combinations of factors and their interactions to select the best model, the small sample size limited the possible set of models that could be considered. We therefore evaluated a suite of 35 potential models that included vessel speed, vessel size category, taxon (humpback vs. other species), and region (Atlantic vs. Pacific coast) as main effects and sets of two-term interaction effects of interest (Supplementary Table S2). There were insufficient data to test higher-order interaction terms. Vessel speed was included in the majority of the models evaluated as were interaction terms with vessel speed and vessel size category given that these were the primary variables of interest for this analysis. The best model was selected based on the lowest value of the Akiake’s Information Criterion (AIC) among the candidate models (Burnham and Anderson, 2002). Additional model selection metrics were calculated including AIC corrected for small sample size (AICc), Bayesian Information Criterion (BIC), BIC corrected for small sample size (BICc), and the model R2 (the coefficient of determination) appropriate for a logistic regression model. Predictive skill was evaluated through model mean root-mean-squared error (RMSE) using K-fold cross validation with 10 folds (James et al., 2013). The overall model explanatory power was assessed using likelihood ratio tests on the residual deviance, and the significance of individual explanatory variables was tested using chi-square tests based on single deletion of terms (Wald tests; Hosmer et al., 2013). Statistical significance for these tests was interpreted at an alpha = 0.1. This selection of alpha is justified by 1) our limited power to detect biologically meaningful differences due to small sample size, 2) the desire to avoid excluding potential confounding effects between variables (as discussed in Hosmer et al., 2013), and 3) consistency with model selection via AIC (Sutherland et al., 2023). Analyses were conducted in function “glm” of the R statistical language (R Core Team, 2023) and packages “aod” (version 1.3.3, Lesnoff and Lancelot, 2023), “greybox” (Svetunkov, 2024), and “caret” (version 6.0-94, Kuhn, 2008). Residual patterns were evaluated to assess deviation from assumptions about the underlying statistical distribution through simulated residuals in package DHARMa (version 0.4.7, Hartig, 2024).
3 Results
The analyzed dataset for U.S. waters included 199 new records in addition to 59 records from the Conn and Silber (2013) data set. This included eight new records collected prior to the year 2001, 68 new records collected between 2001-2010, and 123 records of interactions that occurred after 2010 (Figure 2). Records of interactions since 2011 included a larger proportion of non-lethal interactions compared to earlier records. The proportion of lethal interactions (excluding those with unknown outcomes) was 0.34 for 2011-2022 and 0.49 for the period from 2001-2010. This difference reflects a higher proportion of records from medium sized vessels (0.34 during 2011-2022 vs. 0.20 during 2001-2010) and a lower proportion of records from extra-large vessels (0.09 during 2011-2022 vs. 0.12 during 2001-2010). The more recent data also includes a greater proportion of records of humpback whales from the Pacific coast (see below). Finally, there has been concerted effort in recent years within NMFS to document large whale injuries to support stock assessments (e.g., Henry et al., 2021), and this improved documentation could result in detection of more non-lethal interactions that are less obvious than mortalities or major injuries.
Records from the Pacific coast were more representative of smaller vessel classes, where 68% of the interactions reported occurred with small and medium sized vessels compared to the Atlantic coast where 46% of reported records occurred with these size classes (Figure 3). The proportion of lethal interactions was lower for each vessel size class on the Pacific coast compared to the Atlantic coast (Figure 3). However, this regional difference is confounded by differences in the species reported in each region. The Pacific coast records are dominated by humpback whales (119/147 records, Figure 4), and the proportion of lethal interactions is notably lower for humpback whales compared to all other species groups. This lower lethality for humpbacks is consistent across regions. For the Atlantic coast, 21.4% of interactions were lethal for humpbacks while 78.0% were lethal for other species. On the Pacific coast, 21.8% of interactions with humpbacks were lethal, and 71.4% were lethal for other species (Figure 4).
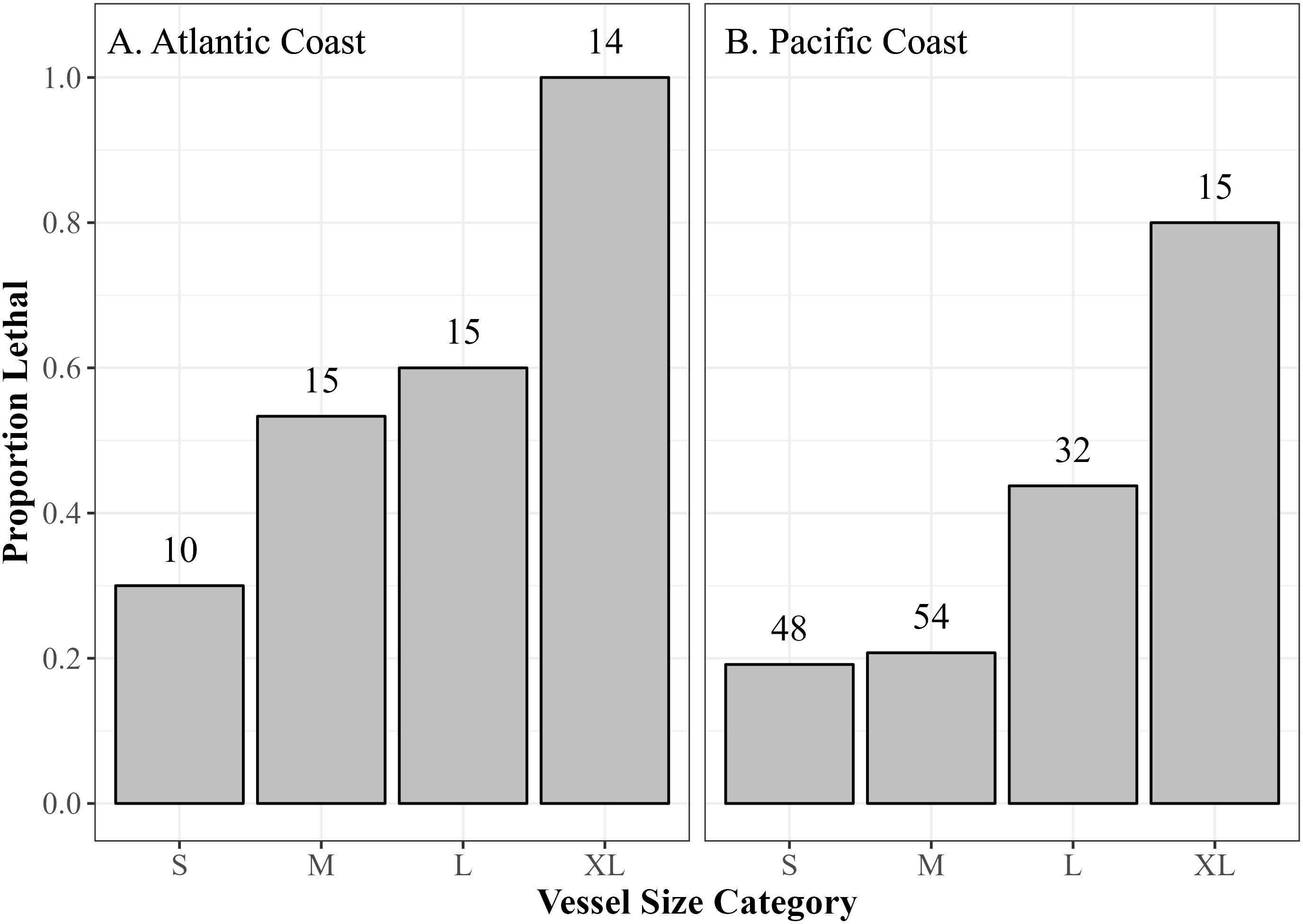
Figure 3. Proportion of lethal whale-vessel interactions by vessel size category for the (A) Atlantic and (B) Pacific coasts of the U.S. The number of individual whales in each category are indicated. Vessel size categories are: S, Small (<12.1 m); M, Medium (12.1 – 19.7 m); L, Large (19.8 – 108 m); XL, Extra-large (> 108 m).
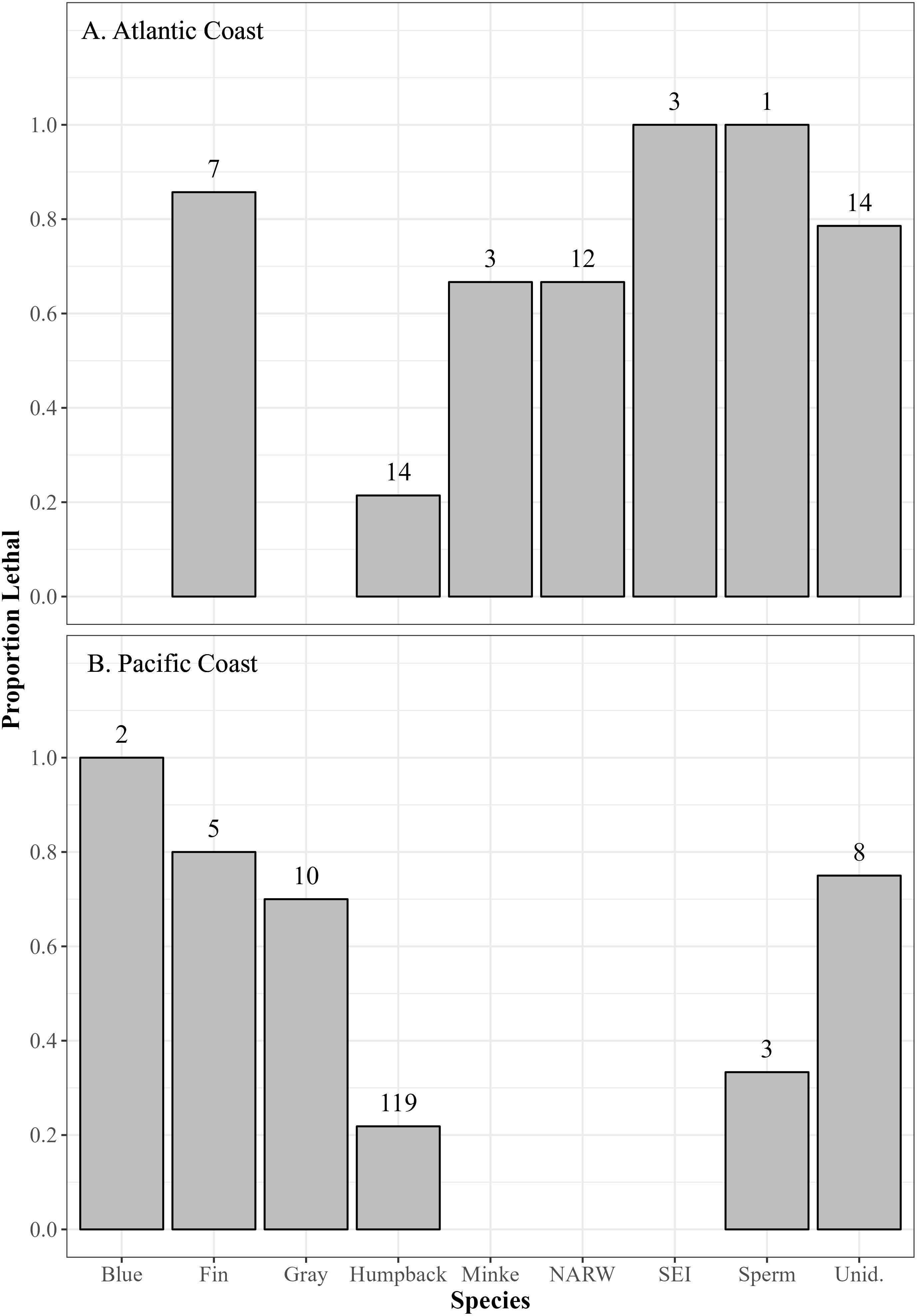
Figure 4. Proportion of lethal whale-vessel interactions by species for the (A) Atlantic and (B) Pacific coasts of the U.S. Sample sizes in each category are indicated. NARW, North Atlantic right whale; Unid, unidentified large whale.
These taxon specific differences are also apparent when examining changes in the observed proportion of lethal strikes within vessel speed classes. The sample size becomes limiting in some cases, in particular as there were very few records with vessel speeds greater than 20 knots for humpback whales (Figure 5). However, where direct comparisons can be made, the proportion of lethal interactions for humpback whales was lower than that for other species in large and extra-large vessels at speeds between 11-25 knots (Figure 5). For other species, the proportion of lethal interactions exceeded 0.8 for vessel speeds greater than 10 knots in the large and extra-large vessel classes, and lethality was typically lower at speeds less than 10 knots (Figure 5), consistent with previous studies.
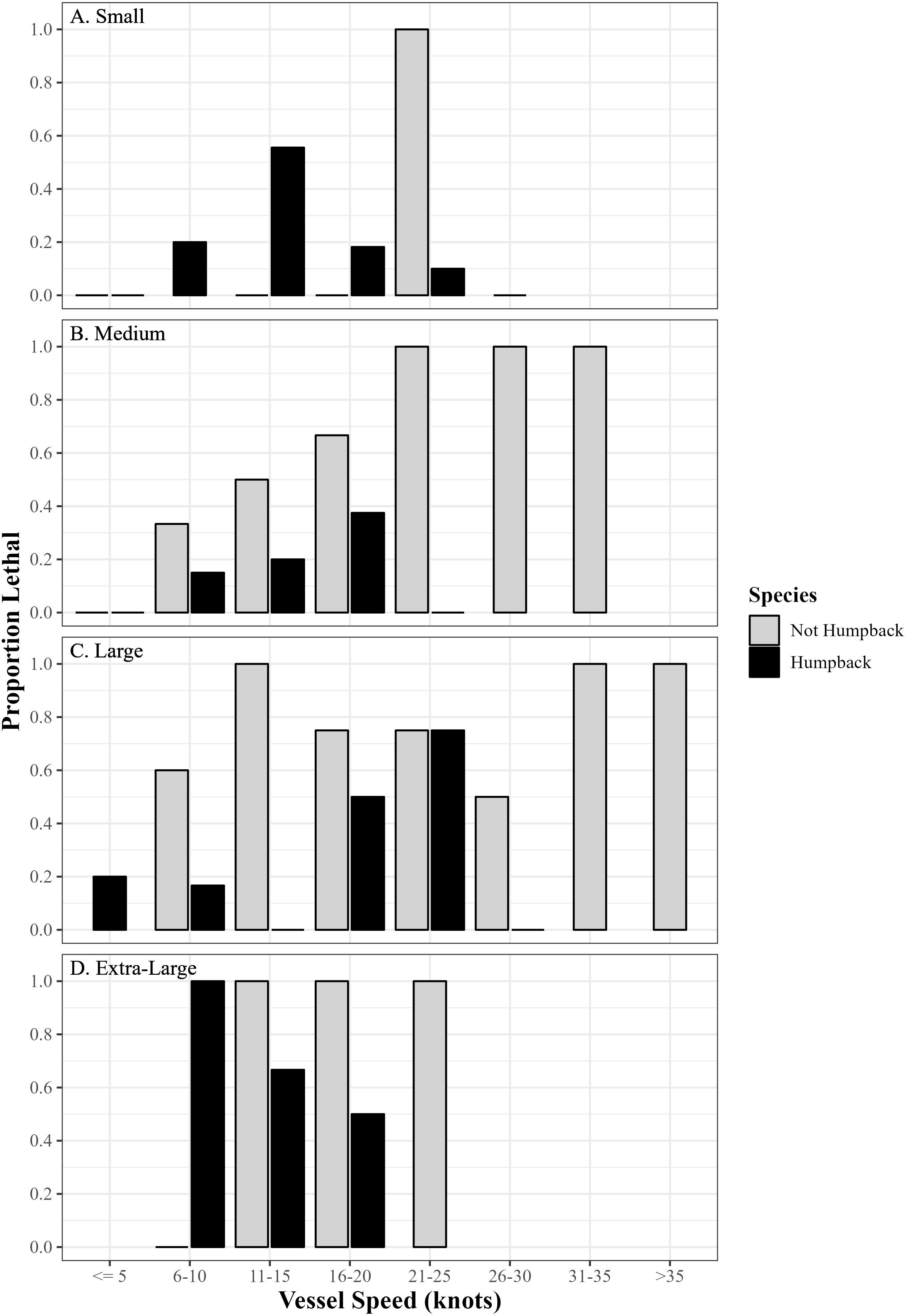
Figure 5. Proportion of lethal whale-vessel interactions by vessel speed by species category and vessel size category. Vessel size categories are: (A) Small: <12.1 m, (B) Medium: 12.1 – 19.7 m, (C) Large: 19.8 – 108 m, and (D) Extra-large: > 108 m.
The best supported logistic regression model included terms for vessel speed, vessel size category, whale taxon, and the interaction term between speed and taxon (Table 2). This model was selected because it had the lowest value of AIC and AICc, the highest R2, and low RMSE compared to other candidate models. It should be noted that the BIC and BICc scores were higher for the model including this interaction term compared to the model excluding the interaction (Table 2), which is to be expected since these metrics penalize model complexity more heavily than the AIC metrics. The model was highly significant (overall likelihood ratio test Chi-squared = 75.7, df = 6, p < 0.0001), and Wald tests for individual terms indicated significant effects of species, vessel size category, and vessel speed on the likelihood of a whale-vessel interaction being lethal (Table 3). There were no deviations in residual patterns that would indicate mis-specification or mis-fit of the selected model. The low proportion of total deviance explained by the model (R2 = 0.291) likely reflects the high amount of variability in the data associated with differences in observation platforms, conditions, and the opportunistic nature of the data collection. Comparisons between levels of the vessel size category variable indicate that the small, medium, and large vessel size classes are not statistically different from each other, but are each different from the extra-large size class (Table 3). The speed by species interaction term was statistically significant at alpha = 0.1 (Wald test Chi-squared = 2.8, df = 1, p = 0.094). This term indicates that the effect of vessel speed on lethality is different between humpback whales and other species. The reduction in AIC and AICc through the addition of this term suggests improved explanatory capability of the model, and the lower RMSE prediction error does not suggest that including this term results in overfitting (Table 2). The apparent differences in the effects of vessel speed on lethality between taxa further supports the inclusion of this interaction term in the selected model to avoid confounding effects.
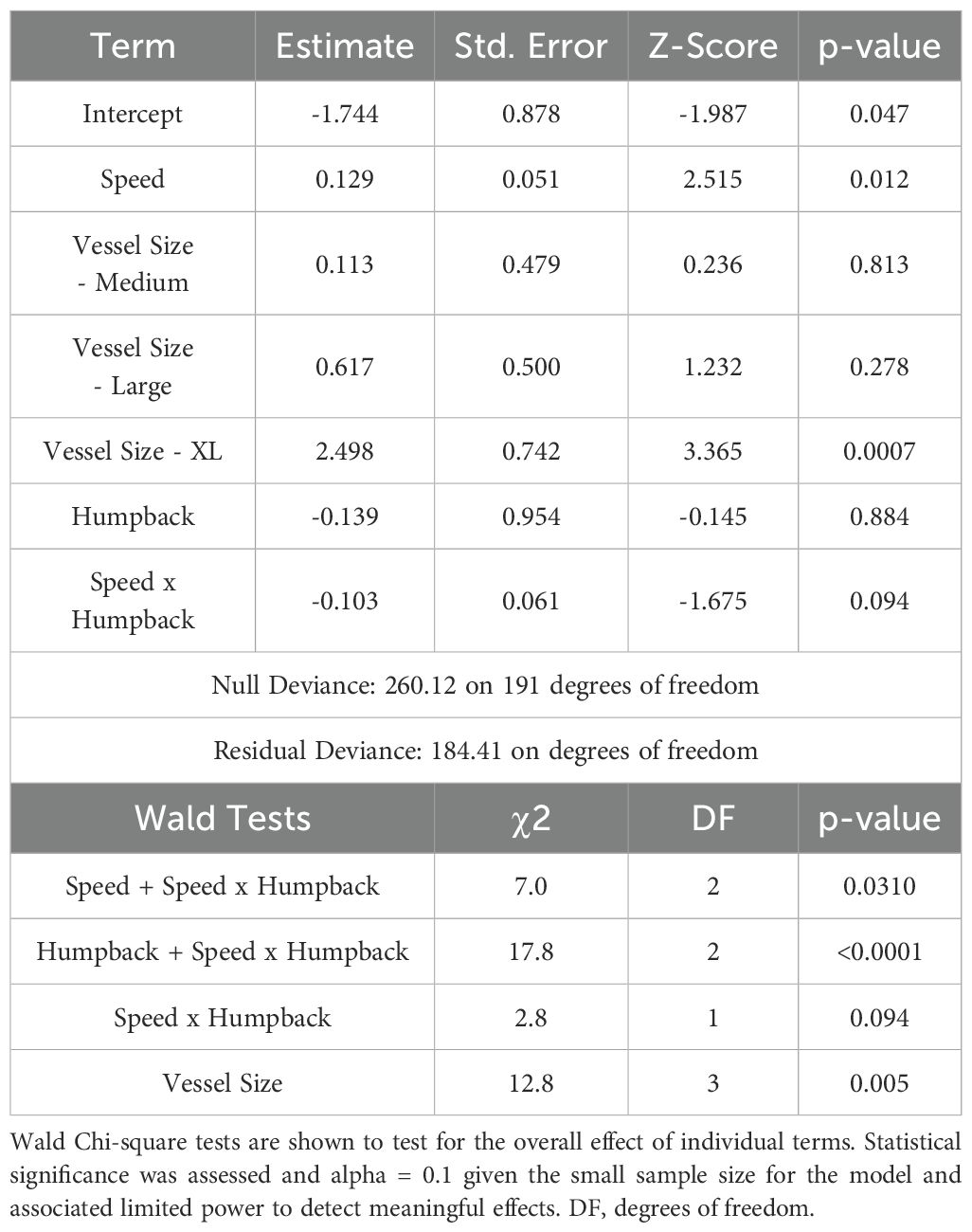
Table 3. Parameter estimates and significance tests for individual terms of the best (lowest AIC) logistic regression model.
The predicted effects of vessel speed, vessel size, and taxon from the selected model are shown in Figure 6. The interpretation of these predictions at vessel speeds <5 knots should be treated with caution as there are very few observations of interactions at low speeds within our dataset. The relationship between vessel speed and lethality for humpback whales is weaker than that for the other species. However, this may be influenced by the limited sample size for humpbacks at high vessel speeds. Probability of lethality for non-humpback species is significantly higher than that for humpbacks at all vessel speeds and sizes. For extra-large vessels, the estimated probability of lethality for non-humpback taxa exceeds 0.79 for vessel speeds greater than 5 knots, and there is a greater reduction in lethality for the other size classes as a function of vessel speed (Figure 6).
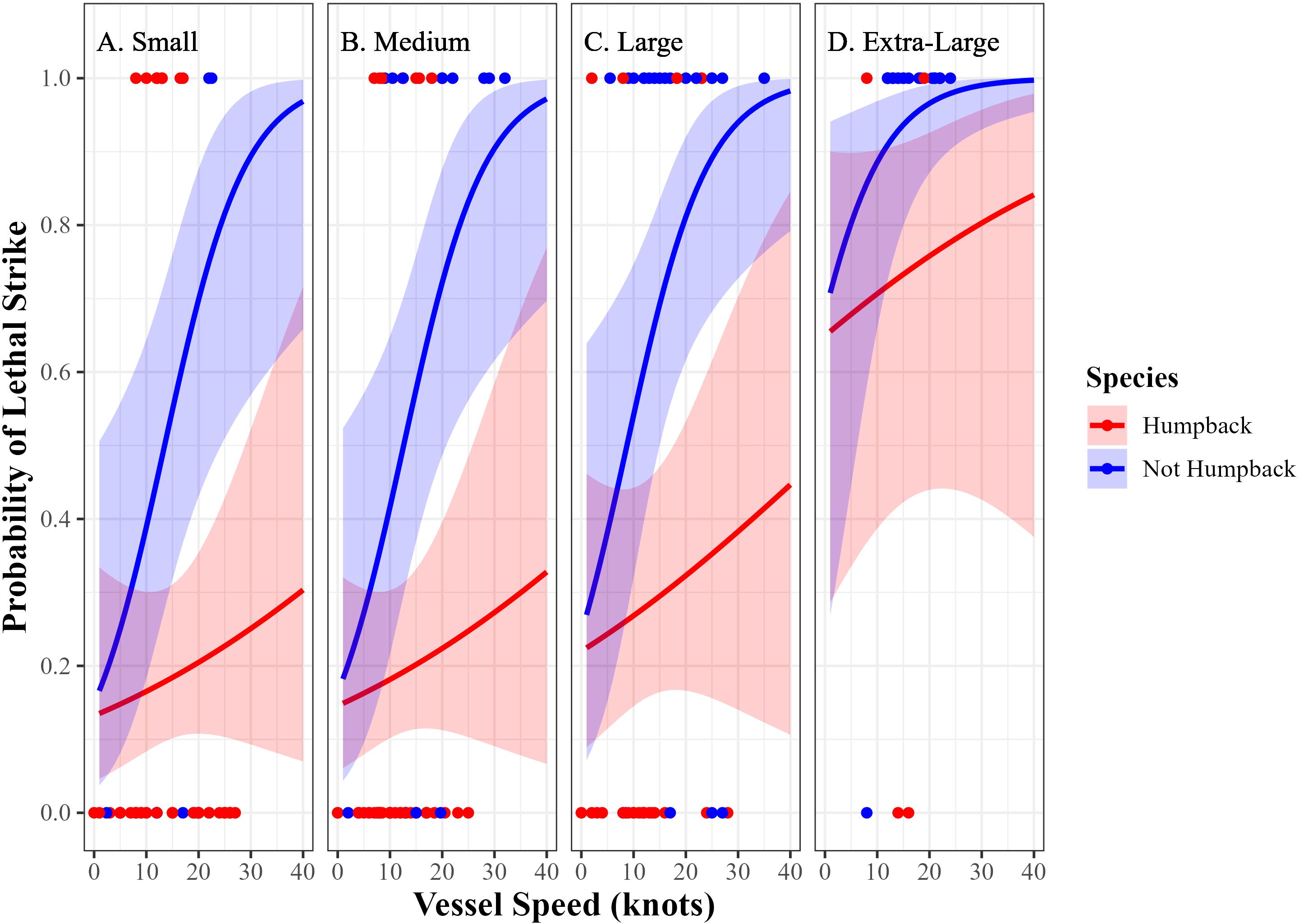
Figure 6. Predicted relationship between vessel speed and the probability of a lethal strike by vessel size category and species. Envelopes indicate 95% confidence interval of the prediction. Vessel size categories are: (A) Small: <12.1 m, (B) Medium: 12.1 – 19.7 m, (C) Large: 19.8 – 108 m, and (D) Extra-large: > 108 m.
4 Discussion
The updated and expanded dataset used in this study allowed us to develop an improved model for evaluating the factors influencing the lethality of vessel interactions with large whales. In addition to increasing sample size, our updated dataset included increased representation of smaller vessels, interactions with vessel types from both the Atlantic and Pacific waters of the U.S., and sufficient data to examine taxon-specific effects. The updated model can be used to inform and improve the predictions from encounter theory models (e.g., Rockwood et al., 2017; Garrison et al., 2022; Blondin et al., 2025) and similar vessel strike models (e.g., Wiley et al., 2011; Nichol et al., 2017; Redfern et al., 2024). Accounting for the effects of vessel size, which can be considered a proxy for vessel mass, is particularly important for these analyses. For North Atlantic right whales, six documented mortalities and serious injuries have been associated with vessels less than 19.8 m (65 feet) in length between 2010-2024 (National Marine Fisheries Service, 2020; Hayes et al., 2022; National Marine Fisheries Service, 2025). The results from this study improve the information available to quantify the potential benefits of reducing speeds in these smaller vessel classes and to differentiate these effects from very large vessels where speed reductions appear to have a lesser, though still beneficial, effect on the probability of severe injury/death given a collision. It should be noted that reduced vessel speeds may influence other aspects of whale-vessel interactions, including the probability of avoiding a collision as discussed below.
The effect of vessel size on lethality is not surprising, and the results presented here, that very large vessels have a high lethality rate across all operational speeds, are supported by studies that consider the physics of these whale-vessel interactions. The forces involved in a direct, perpendicular collision between a large whale and vessels of various masses and bow configurations were considered in detail in Kelley et al. (2021). These authors focused their analyses on blunt force trauma involving contact with a vessel’s bow, the forces and acceleration involved, and the compression of the whale’s blubber layer and underlying musculature. They included an analysis of a subset of vessel strikes with known detailed vessel characteristics and interaction outcome to identify the threshold force at which a strike was likely to be lethal. The study authors noted that their model predicted higher lethality rates at all speeds than the previous Conn and Silber (2013) models. In a detailed analysis of vessel strike mortality risk for fin whales and humpback whales on the west coast of Canada, Keen et al. (2023) applied the Kelley et al. (2021) models for this reason. Our study results suggest that the lower lethality at low vessel speeds predicted by the Conn and Silber (2013) model may have reflected a hidden effect of vessel size. Many of the records included in prior lethality studies at low speeds were also smaller vessels. Our expanded dataset allows us to account for vessel speed and size effects and allows a more direct comparison to the results of [Kelley et al. (2021); Table 4]. The lethality rates predicted for our “Large” and “Extra-Large” vessel size categories are generally consistent with those from Kelley et al. (2021). As expected from the masses involved, the impacts of collisions with very large ships are likely to be lethal at any operational speed. For the “Medium” vessel classes, our model predictions are lower than those for Kelley et al. (2021); however, given the variation in the sizes, bow configuration, and masses of vessels in this class, direct comparisons between our studies are difficult. It should be noted that the Kelley et al. (2021) study did not consider the possible impacts of lacerations from propellers or other parts of the vessel. Lacerations that result in severe injuries and mortalities are observed regularly in whale interactions with both large and small vessels (Wiley et al., 2016; Moore et al., 2021), and are documented in the data and resulting model for the current study.
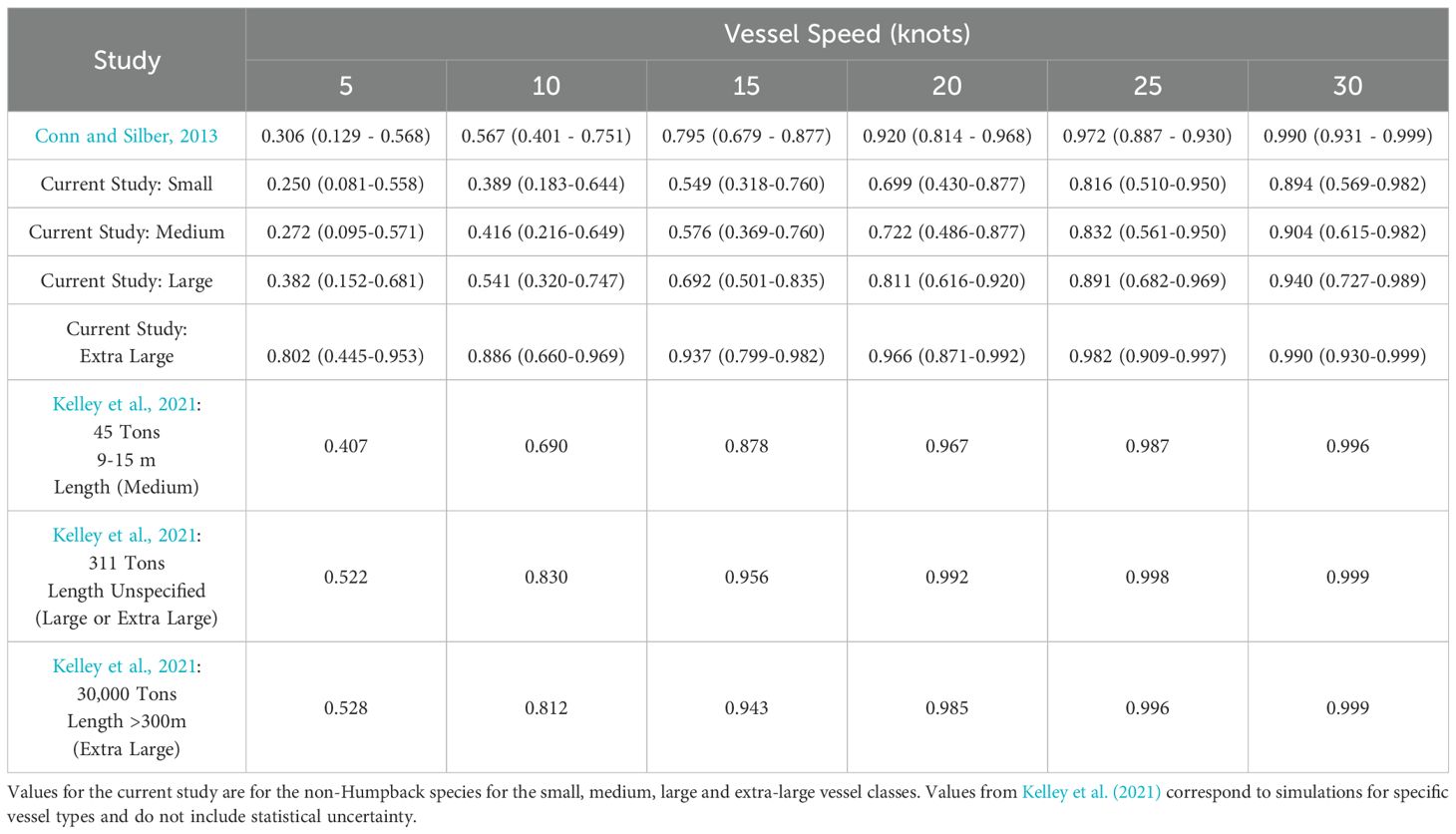
Table 4. Comparison of predicted lethality (95% confidence interval) of whale-vessel interactions between the current study, Conn and Silber (2013), and Kelley et al. (2021).
The observed difference in lethality across all vessel size classes for humpback whales compared to other species is an important finding. Prior to this study, all species have been grouped and treated equally in encounter theory models (e.g., Rockwood et al., 2017, 2020). There may be confounding effects of the nature of the data collected on humpback whales that require some caution in interpreting these findings. It is notable that there were no interactions reported at high vessel speeds (greater than 20 knots) for humpback whales, and there were very few reported interactions with Extra Large vessels. Many of the interactions were observed in habitats with relatively high small vessel use and associated with whale-watching activities (e.g., Currie et al., 2017). It is possible that with frequent close interactions with smaller vessels, these whales reacted in a way to reduce the lethality of collisions when they occurred. Both Currie et al. (2017) in Hawaii and Gende et al. (2011) demonstrated an effect of vessel speed on the probability of close encounters (i.e., within 100-300m) for humpback whales. These results could suggest a behavioral response for this species that allowed them to avoid slower moving vessels. It is possible that humpback whales, especially in high traffic habitats, are more responsive than other species, and that this behavior, in addition to reducing close approaches, also reduces the likelihood of a lethal strike when avoidance is unsuccessful. Finally, blubber layer thickness and musculature of humpback whales may differ from the other species in this study. Kelley et al. (2021) evaluated blubber thickness as a factor influencing the force required to cause a lethal injury because the blubber absorbs some of the force of the collision and protects bones and vital organs. If the humpback whales in this study have thicker blubber layers in the habitats where they were observed, that may also influence their vulnerability to lethal strikes. However, observed lacerations of only the blubber layer would have been coded as severe injuries in this study. The species differences observed here are intriguing and require additional study, particularly considering the limited sample size and potential confounding effects, as there are important management considerations for humpback whales in the U.S. and elsewhere related to vessel strike mortality.
A primary application of the model developed here is to use the estimated probability of lethality given a vessel strike as a parameter within encounter theory models of vessel strike mortality (e.g., Blondin et al., 2025; Rockwood et al., 2020; Garrison et al., 2022), similar approaches that evaluate relative risk (e.g., Nichol et al., 2017; Redfern et al., 2024), and alternative vessel-strike impact assessment frameworks (e.g., Keen et al., 2022, 2023). Each of these approaches rely on an underlying probability of lethality given that a vessel strikes a whale. These models include other components of vessel strike interactions that are influenced by vessel speed, but which are not addressed in the current study. Vessel speed can influence the probability that a whale and vessel will come into close enough proximity for a collision to occur (e.g., Martin et al., 2016; Keen et al., 2022; Blondin et al., 2025). In addition, whales may take evasive maneuvers or dive to avoid oncoming vessels, and vessel speed directly affects the time available for successful avoidance (e.g., McKenna et al., 2015). The frequency of active avoidance by the whale, detection and reaction distances, and the nature of reactions are significant sources of uncertainty for vessel strike assessment models (Blondin et al., 2025; Rockwood et al., 2017; Garrison et al., 2022). However, assuming whales attempt to avoid imminent vessel strikes, slower vessel speeds most likely improve the chances of a successful avoidance (Wiley et al., 2016). This potential benefit of reduced vessel speed is beyond the scope of this analysis.
Reducing the impact of vessel strikes on large whales is essential for the conservation of impacted species and represents a significant challenge to balance both conservation and economic priorities. Increased vessel traffic in offshore habitats associated with new industrial activities such as offshore renewable energy installations and aquaculture may serve to increase or alter the nature of the threat of vessel strikes to large whales. Vessel speed reductions in habitats where whales and vessels overlap are one of the primary tools to reduce the risk to whales in the face of growing use and industrialization of the oceans. This study updates and improves the information available to evaluate the impacts of management efforts focused on vessel speeds. However, the high rate of lethality at all speeds for large ocean-going vessels indicates that avoiding strikes entirely (as opposed to minimizing injury from a strike) is essential to whale conservation outcomes. In combination with routing measures to reduce the spatial overlap between vessels and whales (e.g., Van Der Hoop et al., 2015; Fonnesbeck et al., 2008) and dynamic approaches that notify mariners of whale presence and prompt a response, reducing vessel speeds can improve conservation by reducing the lethality of whale-vessel interactions as well as increasing the opportunity for avoidance by both the whale and the vessel operator.
Data availability statement
The original contributions presented in the study are included in the article/Supplementary Material. Further inquiries can be directed to the corresponding author.
Author contributions
LG: Conceptualization, Formal analysis, Methodology, Supervision, Visualization, Writing – original draft, Writing – review & editing. NL: Data curation, Methodology, Writing – original draft, Writing – review & editing. MG: Data curation, Methodology, Writing – original draft, Writing – review & editing. EP: Conceptualization, Data curation, Investigation, Methodology, Project administration, Resources, Writing – original draft, Writing – review & editing. HB: Writing – original draft, Writing – review & editing. CG: Data curation, Methodology, Writing – original draft, Writing – review & editing.
Funding
The author(s) declare that financial support was received for the research, authorship, and/or publication of this article. Funding was provided through the National Marine Fisheries Service Office of Protected Resources and Southeast Fisheries Science Center, North Atlantic Right Whale Conservation and Research Funds.
Acknowledgments
The authors would like to thank the data providers at NMFS science centers and regional offices who maintain large whale injury records and the National Marine Mammal Stranding database including Allison Henry, Jim Carretta, Ed Lyman, Amanda Bradford, Nancy Young, Sarah Wilkin, Deborah Fauquier and Phinn Onens. We also thank Paul Conn for providing the original data files from his prior analyses. Tim Tinker and Yong Chen provided valuable reviews of an early version of this manuscript.
Conflict of interest
The authors declare that the research was conducted in the absence of any commercial or financial relationships that could be construed as a potential conflict of interest.
Publisher’s note
All claims expressed in this article are solely those of the authors and do not necessarily represent those of their affiliated organizations, or those of the publisher, the editors and the reviewers. Any product that may be evaluated in this article, or claim that may be made by its manufacturer, is not guaranteed or endorsed by the publisher.
Supplementary material
The Supplementary Material for this article can be found online at: https://www.frontiersin.org/articles/10.3389/fmars.2024.1467387/full#supplementary-material
References
Blondin H., Abrahms B., Crowder L. B., Hazen E. L. (2020). Combining high temporal resolution whale distribution and vessel tracking data improves estimates of ship strike risk. Biol. Cons. 250, 108757. doi: 10.1016/j.biocon.2020.108757
Blondin H., Garrison L. P., Adams J. D., Roberts J. J., Good C. P., Gahm M., et al. (2025). Vessel strike encounter risk model informs mortality risk for endangered North Atlantic Right Whales along the United States east coast. Nat. Sci. Rep. 15, 736. doi: 10.1038/s41598-024-84886-z
Bradford A. L., Lyman E. (2015). Injury determinations for humpback whales and other cetaceans reported to NOAA response networks in the Hawaiian Islands during 2007- 2012. U.S. Department of Commerce. NOAA Tech Memo, NMFS-PIFSC-45. doi: 10.7289/V5TX3CB1
Bradford A. L., Lyman E. (2018). Injury determinations for humpback whales and other cetaceans reported to the Hawaiian Islands Disentanglement and Pacific Islands Marine Mammal Response Networks during 2013–2016. U.S. Department of Commerce. NOAA Tech Memo, NMFS-PIFSC-75. doi: 10.25923/7n69-jh50
Bradford A. L., Lyman E. (2019). Injury determinations for humpback whales and other cetaceans reported to the Hawaiian Islands Disentanglement and Pacific Islands Marine Mammal Response Networks during 2017. U.S. Department of Commerce. NOAA Tech Memo, NMFS-PIFSC-81. doi: 10.25923/7csm-h961
Burnham K., Anderson D. (2002). Model selection and multimodel inference: a practical information-theoretic approach (New York, NY: Springer-Verlag).
Carretta J. V., Delean B., Helker V., Muto M. M., Greenman J., Wilkinson K., et al. (2020). Sources of human-related injury and mortality for U.S. Pacific west coast marine mammal stock assessments 2015-2018. U.S. Department of Commerce. NOAA Tech. Memo, NMFS-SWFSC-631. doi: 10.25923/j73c-6q78
Carretta J. V., Greenman J., Wilkinson K., Freed J., Saez L., Lawson D., et al. (2021). Sources of human-related injury and mortality for U.S. Pacific west coast marine mammal stock assessments 2015-2019. U.S. Department of Commerce. NOAA Tech. Memo, NMFS-SWFSC-643. doi: 10.25923/cwre-v564
Carretta J. V., Oleson E. M., Forney K. A., Weller D. W., Lang A. R., Baker J., et al. (2022). U.S. Pacific marine mammal stock assessments: 2022. U.S. Department of Commerce. NOAA Tech. Memo, NMFS-SWFSC-684. doi: 10.25923/5ysf-gt95
Carrillo M., Ritter F. (2010). Increasing numbers of ship strikes in the Canary Islands: proposals for immediate action to reduce risk of vessel-whale collisions. J. Cetacean Res. Manage. 11, 131–138. doi: 10.47536/jcrm.v11i2.618
Conn P. B., Silber G. K. (2013). Vessel speed restrictions reduce risk of collision-related mortality for North Atlantic right whales. Ecosphere 4, 43. doi: 10.1890/ES13-00004.1
Crum N., Gowan T., Krzystan A., Martin J. (2019). Quantifying risk of whale–vessel collisions across space, time, and management policies. Ecosphere 10, e02713. doi: 10.1002/ecs2.2713
Currie J. J., Stack S. H., Kaufman G. D. (2017). Modelling whale-vessel encounters: the role of speed in mitigating collisions with humpback whales (Megaptera novaengliae). J. Cetacean Res. Manage. 17, 57–64. doi: 10.47536/jcrm.v17i1.431
Fonnesbeck C. J., Garrison L. P., Ward-Geiger L. I., Baumstark R. D. (2008). Bayesian hierarchical model for evaluating the risk of vessel strikes on North Atlantic right whales in the SE United States. Endang. Spec. Res. 6, 87–94. doi: 10.3354/esr00134
Garrison L. P., Adams J., Patterson E. M., Good C. P. (2022). Assessing the risk of vessel strike mortality in North Atlantic right whales along the U.S. East Coast. U.S. Department of Commerce. NOAA Tech. Memo, NMFS-SEFSC-757. doi: 10.25923/pcpj-0k72
Gende S. M., Hendrix A. N., Harris K. R., Eichenlaub B., Nielsen J., Pyare S. (2011). A Bayesian approach for understanding the role of ship speed in whale-ship encounters. Ecol. Applic. 21, 2232–2240. doi: 10.1890/10-1965.1
Hartig F. (2024). _DHARMa: Residual Diagnostics for Hierarchical (Multi-Level/ Mixed) Regression Models_. R package version 0.4.7. doi: 10.32614/CRAN.package.DHARMa
Hayes S. A., Josephson E., Maze-Foley K., Rosel P. E., McCordic J., Wallace J. (2022). U.S. Atlantic and Gulf of Mexico marine mammal stock assessments. U.S. Department of Commerce. NOAA Tech Memo, NMFS-NE-304. doi: 10.25923/42zk-w456
Henry A. G., Cole T. V. N., Garron M., Ledwell W., Morin D., Reid A. (2017). Serious injury and mortality determinations for baleen whale stocks along the Gulf of Mexico, United States East Coast, and Atlantic Canadian Provinces 2011-2015. National Marine Fisheries Service, Northeast Fisheries Science Center. Center Reference Document 17-19. doi: 10.7289/V5C53HTB
Henry A. G., Cole T. V. N., Hall L., Ledwell W., Morin D., Reid A. (2015). Mortality and serious injury determinations for baleen whale stocks along the Gulf of Mexico, United States east coast and Atlantic Canadian provinces 2009-2013. National Marine Fisheries Service, Northeast Fisheries Science Center. Center Reference Document 15-10. doi: 10.7289/V5C53HTB
Henry A. G., Cole T. V. N., Hall L., Ledwell W., Morin D., Reid A. D. (2020). Mortality and Serious Injury determinations for baleen whale stocks along the Gulf of Mexico, United States East Coast and Atlantic Canadian Provinces 2013–2017 National Marine Fisheries Service, Northeast Fisheries Science Center. Center Reference Document 20-06. doi: 10.25923/fbc7-ky15
Henry A. G., Cole T. V. N., Hall L., Ledwell W., Morin D., Reid A. (2021). Mortality and serious injury determinations for baleen whale stocks along the Gulf of Mexico, United States East Coast and Atlantic Canadian Provinces 2014–2018. National Marine Fisheries Service, Northeast Fisheries Science Center. Center Reference Document 21-07. doi: 10.25923/ey0b-fw22
Henry A., Garron M., Reid A., Morin D., Ledwell W., Cole T. V. N. (2019). Serious injury and mortality determinations for baleen whale stocks along the Gulf of Mexico, United States East Coast, and Atlantic Canadian Provinces 2012-2016. National Marine Fisheries Service, Northeast Fisheries Science Center. Center Reference Document 19-13. doi: 10.25923/121e-z310
Hosmer D. W. Jr., Lemeshow S., Sturdivant R. X. (2013). Applied Logistic Regression. 3rd Edition (Hoboken, NJ: John Wiley & Sons). doi: 10.1002/9781118548387
International Whaling Commission (2022). Strategic Plan to Mitigate the Impacts of Ship Strikes on Cetacean Populations: 2022-2032 (International Whaling Commission, Conservation Committee). Available at: https://iwc.int/management-andconservation/ship-strikes (Accessed January 10, 2025).
James G., Witten D., Hastie T., Tibshirani R. (2013). An Introduction to Statistical Learning: with Applications in R. Springer Texts in Statistics (New York: Springer).
Jensen A. S., Silber G. K. (2003). Large whale ship strike database. U.S. Department of Commerce. NOAA Tech. Memo, NMFS-OPR-25. Available at: https://repository.library.noaa.gov/view/noaa/23127 (Accessed January 10, 2025).
Keen E., Hendricks B., Shine C., Wray J., Picard C. R., Alidina H. M. (2022). A simulation-based tool for predicting whale-vessel encounter rates. Ocean Coast. Manage. 224, 106183. doi: 10.1016/j.ocecoaman.2022.106183
Keen E. M., O’Mahony É., Nichol L. M., Wright B. M., Shine C., Hendricks B., et al. (2023). Ship-strike forecast and mitigation for whales in Gitga’at First Nation territory. Endang. Spec. Res. 51, 31–58. doi: 10.3354/esr01244
Kelley D. E., Vlasic J. P., Brillant S. W. (2021). Assessing the lethality of ship strikes on whales using simple biophysical models. Mar. Mamm. Sci. 37, 251–267. doi: 10.1111/mms.12745
Kuhn M. (2008). Building predictive models in R using the caret package. J. Stat. Softw. 28, 1–26. doi: 10.18637/jss.v028.i05
Laist D. W., Knowlton A. R., Mead J. G., Collet A. S., Podesta M. (2001). Collisions between ships and whales. Mar. Mamm. Sci. 17, 35–75. doi: 10.1111/j.1748-7692.2001.tb00980.x
Laist D. W., Knowlton A. R., Pendleton D. (2014). Effectiveness of mandatory vessel speed limits for protecting North Atlantic right whales. Endang. Spec. Res. 23, 133–147. doi: 10.3354/esr00586
Lammers M. O., Pack A. A., Lyman E. G., Espiritu L. (2013). Trends in collisions between vessels and North Pacific humpback whales (Megaptera novaeangliae) in Hawaiian waters, (1975-2011). J. Cetacean Res. Manage. 13, 73–80. doi: 10.47536/jcrm.v13i1.557
Lesnoff M., Lancelot R. (2012). aod: Analysis of Overdispersed Data. R package version 1.3.3. Available online at: http://cran.r-project.org/package=aod.
Martin J., Sabatier Q., Gowan T. A., Giraud C., Gurarie E., Calleson. C. S., et al. (2016). A quantitative framework for investigating risk of deadly collisions between marine wildlife and boats. Methods Ecol. Evol. 7, 42–50. doi: 10.1111/2041-210X.12447
McCullagh P., Nelder J. A. (1989). Generalized Linear Models. 2nd Edition (London: Chapman and Hall).
McKenna M., Calambokidis J., Oleson E., Laist D. W., Goldbogen J. A. (2015). Simultaneous tracking of blue whales and large ships demonstrates limited behavioral responses for avoiding collision. Endang. Species Res. 27, 219–32. doi: 10.3354/esr00666
Moore M., Rowles T., Fauquier D., Baker J., Biedron I., Durban J. W., et al. (2021). REVIEW Assessing North Atlantic right whale health: threats, and development of tools critical for conservation of the species. Dis. Aquat. Organ. 143, 205–226. doi: 10.3354/dao03578
National Marine Fisheries Service (2025). 2017-2025 North Atlantic Right Whale Unusual Mortality Event. Available at: https://www.fisheries.noaa.gov/national/marine-life-distress/2017-2025-north-atlantic-right-whale-unusual-mortality-event (Accessed January 15, 2025).
National Marine Fisheries Service (2020). North Atlantic right whale (Eubalaena glacialis) vessel speed rule assessment (National Marine Fisheries Service, Office of Protected Resources, Silver Spring, MD). Available at: https://media.fisheries.noaa.gov/2021-01/FINAL_NARW_Vessel_Speed_Rule_Report_Jun_2020.pdf?null.
National Oceanic and Atmospheric Administration (2008). Endangered fish and wildlife; final rule to implement speed restrictions to reduce the threat of ship collisions with North Atlantic right whales. U.S. Fed. Regist. 73, 60173–60191.
Neilson J. L., Gabriele C. M., Jensen A. S., Jackson K., Straley J. M. (2012). Summary of reported whale-vessel collisions in Alaskan waters. J. Mar. Biol. 2012, 106282. doi: 10.1155/2012/106282
Nichol L., Wright B., O’Hara P., Ford J. (2017). Risk of lethal vessel strikes to humpback and fin whales off the west coast of Vancouver Island, Canada. Endang. Spec. Res. 32, 373–390. doi: 10.3354/esr00813
Pace R. M., Williams R., Kraus S. D., Knowlton A. R., Pettis H. M. (2021). Cryptic mortality of North Atlantic right whales. Conserv. Sci. Pract. 3, e346. doi: 10.1111/csp2.346
R Core Team (2023). _R: A Language and Environment for Statistical Computing_ (Vienna, Austria: R Foundation for Statistical Computing). Available at: https://www.R-project.org/ (Accessed January 10, 2025).
Redfern J. V., Becker E. A., Moore T. J. (2020). Effects of variability in ship traffic and whale distributions on the risk of ships striking whales. Front. Mar. Sci. 6. doi: 10.3389/fmars.2019.00793
Redfern J. V., Hodge B. C., Pendleton D. E., Knowlton A. R., Adams J., Patterson E. M., et al. (2024). Estimating reductions in the risk of vessels striking whales achieved by management strategies. Biol. Cons. 290, 110427. doi: 10.1016/j.biocon.2023.110427
Redfern J. V., Moore T. J., Becker E. A., Calambokidis J., Hastings S. P., Irvine L. M., et al. (2019). Evaluating stakeholder-derived strategies to reduce the risk of ships striking whales. Diver. Distr. 25, 1575–1585. doi: 10.1111/ddi.12958
Rockwood R., Adams J., Silber G., Jahncke J. (2020). Estimating effectiveness of speed reduction measures for decreasing whale-strike mortality in a high-risk region. Endang. Spec. Res. 43, 145–166. doi: 10.3354/esr01056
Rockwood R. C., Calambokidis J., Jahncke J. (2017). High mortality of blue, humpback and fin whales from modeling of vessel collisions on the U.S. West coast suggests population impacts and insufficient protection. PloS One 12, e0183052. doi: 10.1371/journal.pone.0183052
Schoeman R. P., Patterson-Abrolat C., Plön S. (2020). A global review of vessel collisions with marine animals. Front. Mar. Sci. 7. doi: 10.3389/fmars.2020.00292
Sharp S. M., McLellan W. A., Rotstein D. S., Costidis A. M., Barco S. G., Durham K., et al. (2019). Gross and histopathological diagnoses from North Atlantic right whale Eubalaena glacialis mortalities between 2003 and 2018. Dis. Aquat. Organ. 135, 1–31. doi: 10.3354/dao03376
Sutherland C., Hare D., Johnson P. J., Linden D. W., Montgomery R. A., Droge E. (2023). Practical advice on variable selection and reporting using Akaike information criterion. Proc. R. Soc B 290, 20231261. doi: 10.1098/rspb.2023.1261
Svetunkov I. (2023). greybox: Toolbox for Model Building and Forecasting. R package version 2.0.3. doi: 10.32614/CRAN.package.greybox
van der Hoop J. M., Vanderlaan A. S. M., Cole T. V. N., Henry A. G., Hall L., Mase-Guthrie B., et al. (2015). Vessel strikes to large whales before and after the 2008 Ship Strike Rule. Conserv. Lett. 8, 24–32. doi: 10.1111/conl.2015.8.issue-1
Vanderlaan A. S. M., Taggart C. T. (2007). Vessel collisions with whales: the probability of lethal injury based on vessel speed. Mar. Mamm. Sci. 23, 144–156. doi: 10.1111/j.1748-7692.2006.00098.x
Wiley D. N., Mayo C. A., Maloney E. M., Moore M. J. (2016). Vessel strike mitigation lessons from direct observations involving two collisions between noncommercial vessels and North Atlantic right whales (Eubalaena glacialis). Mar. Mamm. Sci. 32, 1501–1509. doi: 10.1111/mms.2016.32.issue-4
Wiley D. N., Thompson M., Pace R. M., Levenson J. (2011). Modeling speed restrictions to mitigate lethal collisions between ships and whales in the Stellwagen Bank National Marine Sanctuary, USA. Biol. Conserv. 144, 2377–2381. doi: 10.1016/j.biocon.2011.05.007
Winkler C., Panigada S., Murphy S., Ritter F. (2020). Global numbers of ship strikes: An assessment of collisions between vessels and cetaceans using available data in the IWC Ship Strike Database (International Whaling Commission). Document Number 68B/SC/HIM/09. Available at: https://archive.iwc.int/pages/view.php?ref=17562&k= (Accessed January 10, 2025)
Keywords: mysticete whales, vessel strike, conservation, endangered species, logistic regression
Citation: Garrison LP, Lisi NE, Gahm M, Patterson EM, Blondin H and Good CP (2025) The effects of vessel speed and size on the lethality of strikes of large whales in U.S. waters. Front. Mar. Sci. 11:1467387. doi: 10.3389/fmars.2024.1467387
Received: 19 July 2024; Accepted: 24 December 2024;
Published: 05 February 2025.
Edited by:
Todd Atwood, U.S. Geological Survey, Alaska, United StatesReviewed by:
Mingli Lin, Chinese Academy of Sciences (CAS), ChinaMarc Lammers, Hawaiian Islands Humpback Whale National Marine Sanctuary, United States
Copyright © 2025 This work is authored by Garrison LP, Lisi NE, Gahm M, Patterson EM, Blondin H and Good CP on behalf of the U.S. Government and as regards Garrison LP, Lisi NE, Gahm M, Patterson EM, Blondin H and Good CP and the U.S. Government, is not subject to copyright protection in the United States. Foreign and other copyrights may apply. This is an open-access article distributed under the terms of the Creative Commons Attribution License (CC BY). The use, distribution or reproduction in other forums is permitted, provided the original author(s) and the copyright owner(s) are credited and that the original publication in this journal is cited, in accordance with accepted academic practice. No use, distribution or reproduction is permitted which does not comply with these terms.
*Correspondence: Lance P. Garrison, TGFuY2UuR2Fycmlzb25Abm9hYS5nb3Y=