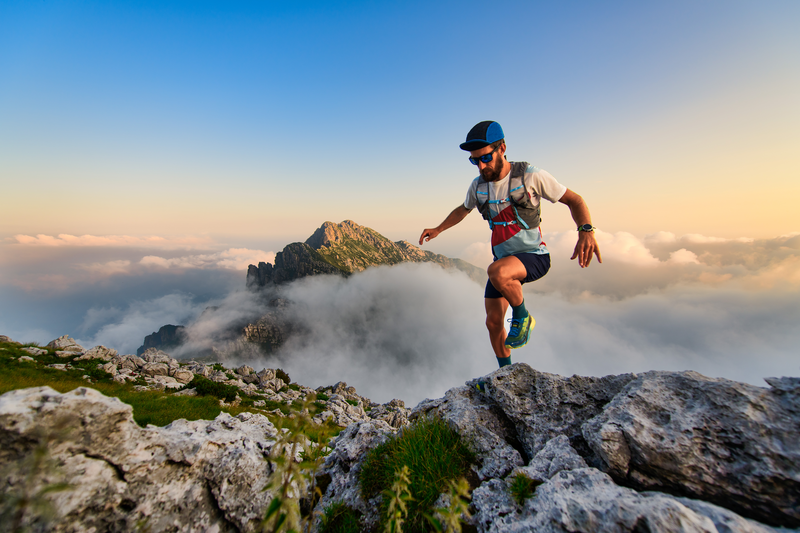
94% of researchers rate our articles as excellent or good
Learn more about the work of our research integrity team to safeguard the quality of each article we publish.
Find out more
PERSPECTIVE article
Front. Mar. Sci. , 11 October 2024
Sec. Global Change and the Future Ocean
Volume 11 - 2024 | https://doi.org/10.3389/fmars.2024.1466968
Marine mammals are integral to global biodiversity and marine health through their roles in coastal, benthic, and pelagic ecosystems. Marine mammals face escalating threats from climate change, pollution, and human activities, which perturb their oceanic environment. The diverse biology and extreme adaptations evolved by marine mammals make them important study subjects for understanding anthropogenic pressures on marine ecosystems. However, ethical and logistical constraints restrict the tractability of experimental research with live marine mammals. Additionally, studies on the effects of changing ocean environments are further complicated by intricate gene-environment interactions across populations and species. These obstacles can be overcome with a comprehensive strategy that involves a systems-level approach integrating genotype to phenotype using rigorously defined experimental conditions in vitro and ex vivo. A thorough analysis of the interactions between the genetics of marine mammals and their exposure to anthropogenic pressures will enable robust predictions about how global environmental changes will affect their health and populations. In this perspective, we discuss four challenges of implementing such non-invasive approaches across scientific fields and international borders: 1) practical and ethical limitations of in vivo experimentation with marine mammals, 2) accessibility to relevant tissue samples and cell cultures; 3) open access to harmonized methods and datasets and 4) ethical and equitable research practices. Successful implementation of the proposed approach has the potential impact to inspire new solutions and strategies for marine conservation.
Marine mammals play essential roles in coastal, benthic, and pelagic ecosystems. Many species occupy high trophic levels and contribute to carbon sequestration, nutrient cycling, and primary productivity. Some marine mammals dive deeply or migrate long distances, enabling vertical and horizontal movement of nutrients. Therefore, declines in marine mammal populations compromise the stability and biodiversity of ocean ecosystems and serve as warning signs of declines in ecosystem health (Fortuna et al., 2024; Estes et al., 2016). Furthermore, the accumulation of contaminants and microplastics in marine mammal tissues provides a bioindicator of pollution in marine food webs and its effects on large, long-lived mammals (Bossart, 2011). This diverse group, comprising whales and dolphins (cetaceans), seals and sea lions (pinnipeds), manatees and dugongs (sirenians), and polar bears and sea otters (marine fissipeds) has convergently evolved adaptations to the aquatic environment on a relatively short time scale, including extreme body size, extraordinary diving ability, and extended fasting capacity (Foote et al., 2015; Jones et al., 2009). During the Anthropocene, marine mammals increasingly confront mounting pressures impacting their health, reproduction, and population viability (Parsons et al., 2015; O’Hara et al., 2021; Halpern et al., 2015; Schaap et al., 2023; Morrison et al., 2022; National Academy of Sciences, Engineering, and Medicine, 2017). As vanguards in a changing world, a more profound understanding of marine mammal adaptations and vulnerabilities to anthropogenic pressures is essential to global health and will allow modeling responses to further change.
Direct and indirect anthropogenic pressures threaten marine mammal populations’ health. Over 40% of the 134 recognized marine mammal species are classified at high risk of global extinction by the International Union for Conservation of Nature (IUCN), an increase from only 25% in 2021 (Nelms et al., 2021; IUCN, 2023). This is likely an underestimate as many species and populations (102 out 209 (IUCN, 2023)) are data-deficient due to uncertain distribution, population status, or taxonomic assignment, and may have an especially high extinction risk (Braulik et al., 2023; Borgelt et al., 2022). While regulatory actions have contributed to the recovery of some populations (Valdivia et al., 2019; Lowry et al., 2014; Herr et al., 2022), others remain under threat due to direct and indirect effects of incidental catch, harvesting, pollution, marine traffic, tourism, urban development, and climate change (Nelms et al., 2021; Davidson et al., 2012; Avila et al., 2018). This is concerning as marine mammals offer diverse ecosystem services critical to ocean health, such as nutrient cycling and food web stability (Estes et al., 2009; McCauley et al., 2015; Hammerschlag et al., 2019; Roman and McCarthy, 2010).
Bycatch in fisheries is a significant threat to whales and dolphins (Cetacea), with at least 300,000 individuals ensnared and killed annually (Read et al., 2006; Elliott et al., 2023). In addition, some fisheries compete with marine mammals for prey, and both fisheries and shipping traffic can cause physical encounters, injuries, and fatalities to marine mammals (Schoeman et al., 2020; International Whaling Commission, 2023; Demaster et al., 2001). Although the number of harvested marine mammals has dropped, several marine mammal species are still consumed worldwide, and direct harvesting is still a threat to tens of species (Avila et al., 2018; Skern-Mauritzen et al., 2022).
Marine mammals are also adversely impacted by the physiological stress responses induced by other oceanic and coastal activities. For example, ecotourism - driven by a fascination with marine mammals - exposes millions of people to these animals globally and is a threat to 21% of the marine mammal species included in the IUCN Red List of Threatened Species (Bejder et al., 2022; IUCN, 2023). Similarly, noise pollution from shipping, naval activities, sonar, and seismic operations is another increasing threat to marine mammals. Noise pollution affects marine mammals’ underwater echolocation and communication strategies, inducing neuroendocrine stress responses that impact energy budgets, diving, foraging, social interactions, reproduction, and survival, and has been linked to whale strandings (Erbe et al., 2019; NOAA, 2023; Southall et al., 2021; Williams et al., 2017).
Atmospheric and oceanic pollution is a worsening global problem impacting marine mammal health (Landrigan et al., 2020). Legacy and emerging chemical pollutants accumulate in marine mammals, particularly affecting predators at high trophic levels, such as polar bears and orcas (Remili et al., 2023). Biomagnification and preferential consumption of fatty tissues that accumulate pollutants such as liver and blubber increase the risk of endocrine and metabolic disruption (Guo et al., 2023; Mortimer and Batley, 2023; Sanganyado et al., 2021; Weijs and Zaccaroni, 2016; Fair and Houde, 2023). Thus, chemical pollutants acting as endocrine and metabolic disruptors impair pre- and post-natal development, reproduction, metabolic and immune health, longevity, and may have further impacts on population dynamics in marine mammals (Landrigan et al., 2020; Sanganyado et al., 2021; Weijs and Zaccaroni, 2016; Desforges et al., 2018; Dietz et al., 2019). Furthermore, plastic pollution - adding around 500,000 metric tons to the oceans annually - poses additional threats through entanglement and ingestion and as a carrier of chemical pollutants (Commission, I.W, 2020; Fossi et al., 2020; Kaandorp et al., 2023). Methodologies to delineate and prioritize chemical pollutant drivers of biological processes are under development but have not yet reached “prime time” regarding policy changes.
Beyond pollution, global climate change is likely to exacerbate anthropogenic impacts and physiological stress in marine mammals by intensifying habitat loss, altering ocean productivity, causing shifts in prey range and abundance, releasing pollutants stored in the sea ice, and increasing the frequency and severity of toxic algal blooms and pathogen outbreaks (Read, 2023; Davidson et al., 2012; Albouy et al., 2020; Gobler, 2020; Mahon et al., 2024). Conversely, the loss of marine mammals may exacerbate the effects of climate change on ecosystems, as large-bodied species play a role in carbon sequestration, nutrient cycling, and other vital ecosystem services (Pearson et al., 2023). Diverse stressors interact in complex ways, as is the case for mixtures of chemical pollutants, challenging the study of their combined effects (Wilson et al., 2016; Bestley et al., 2020; Romero, 2004; Wada, 2019; Tartu et al., 2017; Erbe et al., 2018). Understanding the impacts of multiple stressors on marine mammals is crucial for their conservation (Parsons et al., 2015; National Academy of Sciences, Engineering, and Medicine, 2017). As in vivo experimentation with marine mammals is restricted due to logistic and ethical concerns, correlative field studies investigating the relationships between exposure to a stressor and specific health biomarkers have been widely used (Pallin et al., 2022; Trego et al., 2019; Schaap et al., 2023). These studies have inherent limitations in establishing cause-and-effect relationships and mechanistic understanding (Foote et al., 2015; Dietz et al., 2019).
The extraordinary biology of marine mammals captivates many fields, including evolutionary biology, physiology, and toxicology (Manger, 2022); at the union of these disciplines lie the solutions to understanding and predicting marine mammals’ response and resilience to global change. For example, genomic insights can unravel adaptations to cardiovascular and metabolic diseases and the evolution of cancer resistance in large-bodied, long-lived mammals such as whales (Steimle and Moskowitz, 2017; Sun et al., 2022; Nagy et al., 2007; Silva et al., 2023; Vazquez et al., 2022). The Earth Biogenome Project aims to sequence the genomes of all eukaryotic species to understand the evolution of life at all scales and to drive solutions for preserving biodiversity and sustaining human societies (Lewin et al., 2022). The sequencing of several marine mammal genomes is ongoing worldwide, which increases our knowledge of their adaptive potential and facilitates species’ conservation (Theissinger et al., 2023).
To maximize the impact of marine mammal and environmental research at all levels, we suggest using an approach that simultaneously leverages new and innovative ways to study top-level (phenotype and environmental response) and bottom-level (genomics and molecular biology) phenomena. This “middle-out” approach includes in vitro studies with marine mammal cells, ex vivo experiments with tissue slices and explants, and in silico genome mining and modeling. These approaches can help resolve physiological paradoxes, assess interactions between and impacts of multiple stressors, and have many applications in conservation biology (Schaap et al., 2023; Weijs and Zaccaroni, 2016; Lam et al., 2020; Torres-Velarde et al., 2021; Allen et al., 2024; Goksøyr, 2022). The prospects and challenges involved in implementing such less-invasive approaches are discussed below.
Conducting in vivo experiments with marine mammals presents significant challenges driven by logistical and ethical constraints (Parsons et al., 2015; Williams and Hindle, 2021; Hawkins et al., 2017; Hunt et al., 2013). Additionally, experimental manipulations are restricted to captive animals, limited field interventions (Lam et al., 2020; Ensminger et al., 2021a), and hormone and contaminant profiling (O’Hara and Hart, 2018; Ross et al., 1995; De Swart et al., 1996). Our proposal to circumvent these limitations consists of a “middle-out” approach that combines ex vivo, in vitro, and in silico studies, along with comparative functional genomics and computational methods to gain an integrated, systems-level understanding of basic and applied physiology and toxicology of marine mammals (Figure 1) (Torres-Velarde et al., 2021; Bories et al., 2021; Bjørneset et al., 2023; Khudyakov et al., 2022; Penso-Dolfin et al., 2020; Godard et al., 2004; Hindle et al., 2019). This strategy minimizes our impact on animals while facilitating experiments involving multiple treatments and stressors under precisely controlled conditions (Weijs and Zaccaroni, 2016).
Figure 1. “Middle-out” systems approach, which combines ex vivo, in vitro, and in silico methods. The proposed approach is crucial to conduct research with marine mammals where in vivo experimentation is generally not applicable. In this way, an understanding of how marine mammals will respond to anthropogenic pressures such as climate change, noise pollution, and contaminants at the individual and population levels can be achieved through in vitro and ex vivo experimentation and in silico modeling. The proposed approach may be implemented as follows: First, levels of stress hormones, metabolites, or contaminants of interest are measured in tissues from live or recently deceased animals to establish reference ranges (Desforges et al., 2018; Tartu et al., 2020, 2017; Guo et al., 2021). Next, primary cells or tissues are used to test the effects of varying stressor concentrations and combinations on cell and tissue function (Routti et al., 2016; Torres-Velarde et al., 2021; Kashiwabara et al., 2023; Desforges et al., 2018; Rajput et al., 2021; Lühmann et al., 2020). These functional tests may also incorporate other stressors exacerbated by climate change, such as alterations in nutrient levels, oxygen tension, temperature, and pH. The role of specific pathways may be tested using functional tools such as gene overexpression, knockdown, and pharmacological manipulations (Torres-Velarde et al., 2021; Lam et al., 2020). In some cases, in vitro and ex vivo studies can be integrated with in vivo experimentation in amenable systems to determine whether cell and tissue responses are scalable to the organismal level (Ensminger et al., 2021a; McCormley et al., 2018). Functional experiments may also be conducted using in silico modeling to predict responses to genetic and environmental variations (Hanna et al., 2020). Data generated by this approach may be incorporated into models such as the Population Consequences of Multiple Stressors (PCoMS) to predict the impacts on individual survival and reproduction and, by extension, on population stability (Pirotta et al., 2023; Keen et al., 2021). Potential limitations to the proposed approach include the use of primary cells and tissues, which have a finite lifespan, the use of few tissue and cell types due to the limitations of sampling live marine mammals and species-specific differences in tissue structure, amenability to culture, and response to stressors (Lambilotte, 2024). The latter impacts the ability to understand how tissue responses are integrated at the organismal level but may be overcome by coupling in vitro and ex vivo studies with in vivo experimentation in select systems. Furthermore, contaminant and hormone exposure experiments do not always recapitulate the complexity of pollutant cocktails and signaling molecules likely experienced by marine mammals in vivo but offer the advantage of disentangling the interactions between individual components. Figure generated in BioRender; icons from BioRender or noaa.org (orca and elephant seal); phylogeny from (Christmas et al., 2023); crystal structure from Protein Data Bank (2O9I).
A common paradox in biology is that the more endangered the species, the more dire the need for invasive studies to understand and manage their challenges. Over 40% of marine mammal species are at high risk of global extinction (Nelms et al., 2021; IUCN, 2023). Hence, this paradox is especially critical due to the pressing need to understand how environmental hazards threatening marine mammals continue to expand in scope and scale. Handling marine mammals for purposes such as tissue sampling for toxicological studies and measurements for physiological determinations - or even piloting a boat to the sampling site - can induce stress responses that may adversely impact their health (Southall et al., 2021). Moreover, different species present different ethical challenges, which adds another layer of complexity. As such, any approaches that reduce or eliminate intensive in vivo sampling would simultaneously further conservation goals.
In addition to ethical limitations, there are significant logistical challenges to studying marine mammals. These include regional and international regulations that demand specialized knowledge and dedicated efforts to procure, maintain, and coordinate research permits. These regional regulatory apparatuses balance the need for conservation while reducing research redundancy; however, distinct regulatory regimes across regions lead to asymmetric research capacity across the globe. Similarly, some marine mammal populations sometimes span entire oceans and the resources necessary to reach most marine mammals in oceanic environments are either prohibitive or intractable. Even if the resources are in place, logistical challenges often constrain timely sample preservation and laboratory processing. For marine mammal studies specifically and to maximize research efforts more generally, it is critical to facilitate the open sharing of material and develop novel systems to enable mechanistic marine mammal research.
Lack of access to tissue samples and cell cultures severely limits research on the impacts of chemical and other stressors on marine mammals. Critical issues include sample collection, exchange, storage logistics, incomplete source animal data, and research permit requirements. Low animal accessibility, climate, weather patterns, and distance to laboratory facilities often hinder sample collection. Quality and type of tissues and reliable animal access differ among live, stranded, by-caught, euthanized, and hunted animals. Suitable and reproducible storage and preservation of samples, tissues, and cell cultures is critical for research but depends on field logistics and laboratory equipment availability. While metadata from the source animal (e.g., species, sex, age class, body condition, reproductive state, health status, photo ID, specific population, and past exposure to contaminants or other stressors) are essential for functional data interpretation, they are often limited or unavailable. Permits required for sample collection and exchange vary by country and species, usually creating bureaucratic challenges (Parsons et al., 2015). Finally, financial resources needed to provide reliable and equitable storage and access, such as ultralow-temperature freezer equipment, dedicated personnel responsible for inventory and user management, shipping costs, and assistance and training of low-income or traditionally underrepresented researchers or institutions, present an additional challenge.
To be feasible and reproducible at scale, a middle-out approach for endangered marine mammals requires a strategic plan to generate, store, and access a collection of tissues and cells within a framework that allows equitable and cooperative material exchange. Experimentation in cells and tissues offers a bridge between in vivo techniques and functional molecular biology (Godard-Codding et al., 2011; Lam et al., 2020; Madelaire et al., 2022; Debier et al., 2020; Godard et al., 2006; Godard-Codding and Fossi, 2018; Boroda, 2017), providing unique insights into the impacts of contaminant exposure and response to stressors in marine mammals (Kanter et al., 2002). The establishment of tissue cultures, such as precision-cut adipose tissue slices (Debier et al., 2020; Kashiwabara et al., 2023) and other organotypic models would enable the investigation of responses that are a closer reflection of in vivo conditions (Khudyakov et al., 2017), and unveil physiological mechanisms, such as metabolism, fasting, stress physiology while providing a holistic understanding of multi-cellular complexity, extracellular interactions, and organ structure and function (Khudyakov et al., 2022; Godard et al., 2004; Debier et al., 2020; Godard et al., 2006; Tranganida et al., 2023; Deyarmin et al., 2020, 2019; Khudyakov et al., 2018).
Cell, explant, and organotypic cultures can be derived from most marine mammal tissues and have a variety of downstream applications (Lam et al., 2020; Godard et al., 2004, 2006; Kashiwabara et al., 2023; Khudyakov et al., 2017). While dermal fibroblasts are the most used cultures due to skin tissue accessibility, fibroblasts obtained from other tissues, adipocytes, stem cells, myoblasts, endothelial cells, and trophoblasts have been cultured successfully, increasing research opportunities (Torres-Velarde et al., 2021; Allen et al., 2024; Routti et al., 2016; Louis et al., 2015; Griffeth et al., 2014; Johnson et al., 2012). Most marine mammal cell cultures are primary monocultures, but the field is evolving to include directly reprogrammed and immortalized cell lines, induced pluripotent stem cells, organoids, explants, and organotypic (tissue slice) cultures (Godard et al., 2004; Debier et al., 2020; Kashiwabara et al., 2023; Tranganida et al., 2023; Bennett et al., 2017; Robinson et al., 2018). Nevertheless, the scope of available cultures remains limited in the type of source organs and species, while validation and standardization of newer methodologies are still ongoing. Additionally, primary cell cultures, explants, and organotypic cultures have a finite lifespan, limiting experimentation and resource sharing. Developing collaborative research pipelines between researchers with funding and access to animals and laboratories that can derive, store, and share cells and tissues may democratize access to marine mammal tissues for experimentation.
In silico studies, such as simulations and computational genomics, have transformed our ability to approach traditional physiological, toxicological, and evolutionary research questions. However, these studies depend on the availability of the prerequisite data, such as genomic, epigenetic, and experimental data. Furthermore, functional and computational studies highly depend on the experimental methods’ robustness and reproducibility. As the field progresses toward new frontiers, standardizing and generating open-source methods become increasingly crucial.
The need for standardization and standard reference sets extends beyond biological samples to wet and dry lab methodologies. Standardized methods expand the impact of research products across disciplines. Endeavors such as ENCODE, GTEx, and HuBMAP have developed standardized reference datasets across a range of tissues, enabling an era of exponential research and development by democratizing access to high-quality functional data and by standardizing best practices for future data collection (ENCODE Consortium, 2012; Carithers et al., 2015; Jain et al., 2023). Similarly, creating and standardizing bioinformatic datasets and methods across tissues and species for marine mammals will provide a necessary resource for future physiological and toxicological investigations.
Open and collaborative protocol development and sharing would optimize researchers’ time and resources. For example, optimal conditions for establishing and culturing marine mammal cell lines and tissues often deviate from traditional protocols due to their unique metabolic adaptations and tissue structures (Lam et al., 2020; Routti et al., 2016; Louis et al., 2015; Boroda et al., 2020; Burkard et al., 2015; De Miranda et al., 2012). While some modifications are necessary due to cell type- and species-specific quirks, heterogeneity due to trivial technical factors, such as reagent sources, lot-to-lot variability, and environmental controls, results in wasted research effort. Foreseeing these failures requires a space where researchers can share finalized, successful methods and their failed attempts.
We have a responsibility to center ethical and equitable practices in our work. When research is ingrained with these values, its quality and impact are greatly improved; however, scientific systems do not always incentivize ethical practices, and, in many cases, cell culture and genetics work have enacted harm (Beskow, 2016; Forsberg et al., 2013; Watson, 2014; Botkin et al., 2012; Couzin-Frankel, 2010; Mello and Wolf, 2010; Millum, 2010; Beattie et al., 2011; Mackey and Liang, 2012; Tanner et al., 2021). As research with marine mammal cell cultures expands, we must ensure ethics and equitability are ingrained into every aspect of our studies, from engagement with local communities to fostering an inclusive research community.
Scientists must obtain tissue samples to establish marine mammal cell cultures, which may rely on engagement with local communities through fieldwork partnerships. It is imperative to develop equitable rather than extractive relationships with researchers from low-income countries and local Indigenous communities in which input is solicited while generating ideas and before beginning data collection, with the expectation of a bidirectional exchange of knowledge and acknowledgment of contributions (Mackey and Liang, 2012; Hosseini et al., 2022; Buck and Hamilton, 2011). The data and resources generated during these collaborations should be available through open-access platforms and in addition to publishing journal articles, these data should be disseminated in accessible formats such as maps, outreach initiatives, and infographics. As charismatic megafauna, marine mammals have a strong potential to attract public attention and raise environmental awareness (Albert et al., 2018). Science communicators and stakeholders can foster belonging and engagement by identifying shared values.
A constant challenge for wildlife biologists is obtaining data while minimizing harm to the animals and environments studied. Over the past several decades, the ability to collect biological samples from marine mammals with decreasing levels of disturbance has increased (Danovaro et al., 2016; Gilbey et al., 2021; Stat et al., 2017; Suarez-Bregua et al., 2022). These developments include non-invasive sampling of matrices other than tissues for contaminant and hormone analyses and remote (dart) biopsy collection, which does not require direct animal handling (Hunt et al., 2013). This growing field should consider whether to establish consistent ethical practices and norms surrounding sample collection. For example, many tissues are only accessible from freshly deceased individuals, which may be acquired through whaling or Indigenous harvest (Pugliares et al., 2007; Becker et al., 1997). The marine mammal research community has an ethical obligation to consider the impact of its participation in these institutions, and it may be necessary for the community to delineate which sources and circumstances meet the ethical standards of responsible research. Moreover, establishing and sharing cell lines through a research network would allow the opportunity to reduce sampling, environmental footprint, and potential disturbance to focal animals.
Current systems for recruiting and retaining diverse researchers fall short, hindering research development and innovation. Many researchers in marine mammal science begin their careers with unpaid positions, such as full-time internships. Marginalized groups are particularly disadvantaged and burdened by uncompensated work, leading to high rates of attrition (Millum, 2010; Beattie et al., 2011; Mackey and Liang, 2012). Providing fair compensation is critical for increasing the retention of early career scientists; thus, funding for stipends and financial support is crucial to fill these gaps (Spencer et al., 2005; Bruthers et al., 2021; Grady, 2005; Dutz et al., 2023; McGee et al., 2012). Establishing a research coordination network would provide funding support for research and training, connect trainees with mentors within the network, and improve retention for diverse early-career researchers.
A middle-out approach for studying the complex relationship between genotype, environment, and phenotype solves some of the challenges associated with the study of marine mammals while enabling new avenues of experimental research that shine a light on the current and future response of these species to a changing global environment. Recent studies focusing on the biology of the northern elephant seal (Mirounga angustirostris, Inset 1) and the polar bear (Ursus maritimus Inset 2) serve as examples of the success of this approach in addressing complex questions in marine mammal physiology and toxicology. To this end, we propose the creation of repositories for the establishment and distribution of not only validated tissue samples, cells, and other reagents but of detailed and standardized methods and protocols to maximize the utility of these samples.
The goals of the repository are four-fold: 1) promote inter-institutional collaboration; 2) facilitate entry into and research in all facets of marine mammal biology; 3) accelerate the application of cutting-edge techniques to marine mammal species; and 4) maximize the field’s ability to characterize and predict ongoing and future responses of marine mammals to global ocean changes. This repository, and the community supporting it, would detail best practices for isolation, management, and characterization of marine mammal tissue cultures, host comprehensive protocols with detailed information on unfruitful approaches, identify specific reagents in support of these practices, and link samples with tissue- and species-specific omics-level data. Additionally, by coordinating the collection, preservation, storage, and distribution of samples, this community will strengthen the utility of each sample while minimizing redundant or wasteful collection efforts.
Accordingly, this endeavor requires a synchronized effort across disciplines to ensure the construction of complete and accessible methods and sample repositories. Challenges in this process include addressing intellectual property issues, as methods are often developed or applied years before their resulting publications. Additionally, we envision a collaborative research and training network through which the sharing of methodological knowledge and resources will facilitate interdisciplinary efforts to streamline and accelerate the publication of marine mammal cell culture resources. Ultimately, a coordinated approach to solutions founded in principles of open science will provide a path toward a standardized, robust, and equitable international research program poised to address threats and inform regulatory solutions to marine mammals and global ocean health.
The original contributions presented in the study are included in the article/supplementary material, further inquiries can be directed to the corresponding author/s.
JV: Writing – original draft, Writing – review & editing. JK: Writing – original draft, Writing – review & editing. CM: Writing – original draft, Writing – review & editing. CG-C: Writing – original draft, Writing – review & editing. HR: Writing – original draft, Writing – review & editing. EL: Writing – original draft, Writing – review & editing. EP: Writing – original draft, Writing – review & editing. GM: Writing – original draft, Writing – review & editing. JW: Writing – original draft, Writing – review & editing. KA: Writing – original draft, Writing – review & editing. JC: Writing – original draft, Writing – review & editing. PB: Writing – original draft, Writing – review & editing. DS: Writing – original draft, Writing – review & editing. AG: Writing – original draft, Writing – review & editing. JV-M: Writing – original draft, Writing – review & editing.
The author(s) declare financial support was received for the research, authorship, and/or publication of this article. JV was supported by a National Science Foundation Postdoctoral Research Fellowship in Biology (#2109915) and a T32 training grant from the National Institute on Aging (AG000266). JK was supported by ONR Award No. N00014-23-1-2444. PB was supported by the Research Council of Norway grant #335489/SLICE project. AG was supported by the Research Council of Norway grant #334739/Marma-detox project and the Fulbright Norway Program. This manuscript results from a workshop held at the University of California, Berkeley, supported by a Peder Sather Center grant to AG and JV-M. JV-M was supported by the National Institute of General Medical Sciences (R35GM146951) and the National Science Foundation (2020664). DS was supported by the Research Council of Norway grant #334739/Marma-detox project.
We thank Odd André Karlsen, Fekadu Yadetie, Bruce Blumberg, Daniel Schlenk, Allyson Hindle, Amy Klink, Daniel Crocker, Diana Moreno-Santillan, Cathy Debier, Laura Pirard, Magali Houde, Emily Voelkner, Lauren Kashiwabara and Teri Lynn Herbert for participating in our workshop and contributing to helpful discussions.
The authors declare that the research was conducted in the absence of any commercial or financial relationships that could be construed as a potential conflict of interest.
The author(s) declared that they were an editorial board member of Frontiers, at the time of submission. This had no impact on the peer review process and the final decision.
All claims expressed in this article are solely those of the authors and do not necessarily represent those of their affiliated organizations, or those of the publisher, the editors and the reviewers. Any product that may be evaluated in this article, or claim that may be made by its manufacturer, is not guaranteed or endorsed by the publisher.
Albert C., Luque G. M., Courchamp F. (2018). The twenty most charismatic species. PloS One 13, e0199149. doi: 10.1371/journal.pone.0199149
Albouy C., Delattre V., Donati G., Frölicher T. L., Albouy-Boyer S., Rufino M., et al. (2020). Global vulnerability of marine mammals to global warming. Sci. Rep 10, 548. doi: 10.1038/s41598-019-57280-3
Allen K. N., Torres-Velarde J. M., Vazquez J. M., Moreno-Santillán D. D., Sudmant P. H., Vázquez-Medina J. P. (2024). Hypoxia exposure blunts angiogenic signaling and upregulates the antioxidant system in endothelial cells derived from elephant seals. BMC Biol 22, 91. doi: 10.1186/s12915-024-01892-3
Allen K. N., Vázquez-Medina J. P. (2019). Natural tolerance to ischemia and hypoxemia in diving mammals: a review. Front. Physiol 10, 1199. doi: 10.3389/fphys.2019.01199
Andrews R. D., Enstipp M. R. (2016). Diving physiology of seabirds and marine mammals: relevance, challenges and some solutions for field studies. Comp. Biochem. Physiol. Part A: Mol. Integr. Physiol 202, 38–52. doi: 10.1016/j.cbpa.2016.07.004
Avila I. C., Kaschner K., Dormann C. F. (2018). Current global risks to marine mammals: taking stock of the threats. Biol. Conserv 221, 44–58. doi: 10.1016/j.biocon.2018.02.021
Awad M. M., Brown M. A. (2021). Undergraduate research as a high-impact practice for engaging students of color in ecology. JSTOR 102 (2), 1–6. doi: 10.1002/bes2.1816
Beattie A. J., Hay M., Magnusson B., De Nys R., Smeathers J., Vincent J. F. (2011). Ecology and bioprospecting. Austral Ecol 36, 341–356. doi: 10.1111/j.1442-9993.2010.02170.x
Becker P., Wise S., Thorsteinson L., Koster B., Rowles T. (1997). Specimen banking of marine organisms in the United States: current status and long-term prospective. Chemosphere 34, 1889–1906. doi: 10.1016/S0045-6535(97)00051-9
Bejder L., Higham J. E., Lusseau D. (2022). “Tourism and research impacts on marine mammals: A bold future informed by research and technology,” in Marine mammals: the evolving human factor (Gewerbesraβe 11, 6330 - Cham, Switzerland: Springer International Press).
Bennett K. A., Robinson K. J., Moss S. E., Millward S., Hall A. J. (2017). Using blubber explants to investigate adipose function in grey seals: glycolytic, lipolytic and gene expression responses to glucose and hydrocortisone. Sci. Rep 7, 7731. doi: 10.1038/s41598-017-06037-x
Beskow L. M. (2016). Lessons from HeLa cells: the ethics and policy of biospecimens. Annu. Rev. Genomics Hum. Genet 17, 395–417. doi: 10.1146/annurev-genom-083115-022536
Bestley S., Ropert-Coudert Y., Bengtson Nash S., Brooks C. M., Cotté C., Dewar M., et al. (2020). Marine ecosystem assessment for the Southern Ocean: birds and marine mammals in a changing climate. Front. Ecol. Evol 8, 566936. doi: 10.3389/fevo.2020.566936
Bjørneset J., Blévin P., Bjørnstad P. M., Dalmo R. A., Goksøyr A., Harju M., et al. (2023). Establishment of killer whale (Orcinus orca) primary fibroblast cell cultures and their transcriptomic responses to pollutant exposure. Environ. Int 174, 107915. doi: 10.1016/j.envint.2023.107915
Blix A. S. (2018). Adaptations to deep and prolonged diving in phocid seals. J. Exp. Biol 221, jeb182972. doi: 10.1242/jeb.182972
Borgelt J., Dorber M., Høiberg M. A., Verones F. (2022). More than half of data deficient species predicted to be threatened by extinction. Commun. Biol 5, 679. doi: 10.1038/s42003-022-03638-9
Bories P., Rikardsen A. H., Leonards P., Fisk A. T., Tartu S., Vogel E. F., et al. (2021). A deep dive into fat: Investigating blubber lipidomic fingerprint of killer whales and humpback whales in northern Norway. Ecol. Evol 11, 6716–6729. doi: 10.1002/ece3.7523
Boroda A. (2017). Marine mammal cell cultures: To obtain, to apply, and to preserve. Mar. Environ. Res 129, 316–328. doi: 10.1016/j.marenvres.2017.06.018
Boroda A. V., Kipryushina Y. O., Golochvastova R. V., Shevchenko O. G., Shulgina M. A., Efimova K. V., et al. (2020). Isolation, characterization, and ecotoxicological application of marine mammal skin fibroblast cultures. In Vitro Cell. Dev. Biology-Animal 56, 744–759. doi: 10.1007/s11626-020-00506-w
Bossart G. D. (2011). Marine mammals as sentinel species for oceans and human health. Veterinary Pathol 48, 676–690. doi: 10.1177/0300985810388525
Botkin J. R., Rothwell E., Anderson R., Stark L., Goldenberg A., Lewis M., et al. (2012). Public attitudes regarding the use of residual newborn screening specimens for research. Pediatrics 129, 231–238. doi: 10.1542/peds.2011-0970
Bourgeon S., Riemer A. K., Tartu S., Aars J., Polder A., Jenssen B. M., et al. (2017). Potentiation of ecological factors on the disruption of thyroid hormones by organo-halogenated contaminants in female polar bears (Ursus maritimus) from the Barents Sea. Environ. Res 158, 94–104. doi: 10.1016/j.envres.2017.05.034
Braathen M., Derocher A. E., Wiig Ø., Sørmo E. G., Lie E., Skaare J. U., et al. (2004). Relationships between PCBs and thyroid hormones and retinol in female and male polar bears. Environ. Health Perspect 112, 826–833. doi: 10.1289/ehp.6809
Braulik G. T., Taylor B. L., Minton G., Notarbartolo Di Sciara G., Collins T., Rojas-Bracho L., et al. (2023). Red-list status and extinction risk of the world's whales, dolphins, and porpoises. Conserv. Biol 37, e14090. doi: 10.1111/cobi.14090
Brint S., Cantwell A. M. (2010). Undergraduate time use and academic outcomes: Results from the University of California Undergraduate Experience Survey 2006. Teachers Coll. Rec 112, 2441–2470. doi: 10.1177/016146811011200908
Bruthers C. B., Hedman E. L., Matyas M. L. (2021). Undergraduate research programs build skills for diverse students. Adv. Physiol. Educ 45, 399–408. doi: 10.1152/advan.00165.2020
Buck M., Hamilton C. (2011). The Nagoya Protocol on access to genetic resources and the fair and equitable sharing of benefits arising from their utilization to the Convention on Biological Diversity. Rev. Eur. Community Int. Environ. Law 20, 47–61. doi: 10.1111/j.1467-9388.2011.00703.x
Burkard M., Whitworth D., Schirmer K., Nash S. B. (2015). Establishment of the first humpback whale fibroblast cell lines and their application in chemical risk assessment. Aquat. Toxicol 167, 240–247. doi: 10.1016/j.aquatox.2015.08.005
Bytingsvik J., Simon E., Leonards P. E., Lamoree M., Lie E., Aars J., et al. (2013). Transthyretin-binding activity of contaminants in blood from polar bear (Ursus maritimus) cubs. Environ. Sci. Technol 47, 4778–4786. doi: 10.1021/es305160v
Carithers L. J., Ardlie K., Barcus M., Branton P. A., Britton A., Buia S. A., et al. (2015). A novel approach to high-quality postmortem tissue procurement: the GTEx project. Biopreservation biobanking 13, 311–319. doi: 10.1089/bio.2015.0032
Christmas M. J., Kaplow I. M., Genereux D. P., Dong M. X., Hughes G. M., Li X., et al. (2023). Evolutionary constraint and innovation across hundreds of placental mammals. Science 380, eabn3943. doi: 10.1126/science.abn3943
COMMISSION I. W. (2020). Report of the IWC workshop on marine debris: the way forward, 3–5 december 2019 (La Garriga, Catalonia, Spain: Paper SC).
COMMISSION I. W. (2023). “International whaling commission,” in Ship strike database. Academic Press, an imprint of Elsevier. Available at: https://portal.iwc.int/shipstrike.
Couzin-Frankel J. (2010). Researchers to return blood samples to the Yanomamö. Science 328 (5983), 1218. doi: 10.1126/science.328.5983.1218
Crocker D. E., Khudyakov J. I., Champagne C. D. (2016). Oxidative stress in northern elephant seals: Integration of omics approaches with ecological and experimental studies. Comp. Biochem. Physiol. Part A: Mol. Integr. Physiol 200, 94–103. doi: 10.1016/j.cbpa.2016.02.011
Danovaro R., Carugati L., Berzano M., Cahill A. E., Carvalho S., Chenuil A., et al. (2016). Implementing and innovating marine monitoring approaches for assessing marine environmental status. Front. Mar. Sci 3. doi: 10.3389/fmars.2016.00213
Davidson A. D., Boyer A. G., Kim H., Pompa-Mansilla S., Hamilton M. J., Costa D. P., et al. (2012). Drivers and hotspots of extinction risk in marine mammals. Proc. Natl. Acad. Sci 109, 3395–3400. doi: 10.1073/pnas.1121469109
Debier C., Pirard L., Verhaegen M., Rzucidlo C., Tinant G., Dewulf C., et al. (2020). In vitro lipolysis and leptin production of elephant seal blubber using precision-cut adipose tissue slices. Front. Physiol 11, 615784. doi: 10.3389/fphys.2020.615784
Del Águila-Vargas A. C., Vázquez-Medina J. P., Crocker D. E., Méndez-Rodríguez L. C., Gaxiola-Robles R., De Anda-Montañez J. A., et al. (2020). Antioxidant response to cadmium exposure in primary skeletal muscle cells isolated from humans and elephant seals. Comp. Biochem. Physiol. Part C: Toxicol. Pharmacol 227, 108641. doi: 10.1016/j.cbpc.2019.108641
Demaster D. P., Fowler C. W., Perry S. L., Richlen M. F. (2001). Predation and competition: the impact of fisheries on marine-mammal populations over the next one hundred years. J. Mammalogy 82, 641–651. doi: 10.1644/1545-1542(2001)082<0641:PACTIO>2.0.CO;2
De Miranda M. A. Jr., Schlater A. E., Green T. L., Kanatous S. B. (2012). In the face of hypoxia: myoglobin increases in response to hypoxic conditions and lipid supplementation in cultured Weddell seal skeletal muscle cells. J. Exp. Biol 215, 806–813. doi: 10.1242/jeb.060681
Desforges J.-P., Hall A., McConnell B., Rosing-Asvid A., Barber J. L., Brownlow A., et al. (2018). Predicting global killer whale population collapse from PCB pollution. Science 361, 1373–1376. doi: 10.1126/science.aat1953
De Swart R. L., Ross P. S., Vos J. G., Osterhaus A. (1996). Impaired immunity in harbour seals (Phoca vitulina) exposed to bioaccumulated environmental contaminants: review of a long-term feeding study. Environ. Health Perspect 104, 823–828. doi: 10.1289/ehp.96104s4823
Deyarmin J., Hekman R., Champagne C., McCormley M., Stephan A., Crocker D., et al. (2020). Blubber proteome response to repeated ACTH administration in a wild marine mammal. Comp. Biochem. Physiol. Part D: Genomics Proteomics 33, 100644. doi: 10.1016/j.cbd.2019.100644
Deyarmin J. S., McCormley M. C., Champagne C. D., Stephan A. P., Busqueta L. P., Crocker D. E., et al. (2019). Blubber transcriptome responses to repeated ACTH administration in a marine mammal. Sci. Rep 9, 2718. doi: 10.1038/s41598-019-39089-2
Dietz R., Letcher R. J., Desforges J.-P., Eulaers I., Sonne C., Wilson S., et al. (2019). Current state of knowledge on biological effects from contaminants on arctic wildlife and fish. Sci. Total Environ 696, 133792. doi: 10.1016/j.scitotenv.2019.133792
Dutz D., Greenstone M., Hortaçsu A., Lacouture S., Mogstad M., Shaikh A. M., et al. (2023). Representation and hesitancy in population health research: evidence from a COVID-19 antibody study. Natl. Bureau Economic Res. doi: 10.3386/w30880
Elliott B., Tarzia M., Read A. J. (2023). Cetacean bycatch management in regional fisheries management organizations: Current progress, gaps, and looking ahead. Front. Mar. Sci 9, 1006894. doi: 10.3389/fmars.2022.1006894
ENCODE Consortium. (2012). An integrated encyclopedia of DNA elements in the human genome. Nature 489, 57. doi: 10.1038/nature11247
Ensminger D. C., Crocker D. E., Lam E. K., Allen K. N., Vázquez-Medina J. P. (2021a). Repeated stimulation of the HPA axis alters white blood cell count without increasing oxidative stress or inflammatory cytokines in fasting elephant seal pups. J. Exp. Biol 224, jeb243198. doi: 10.1242/jeb.243198
Ensminger D. C., Salvador-Pascual A., Arango B. G., Allen K. N., Vázquez-Medina J. P. (2021b). Fasting ameliorates oxidative stress: A review of physiological strategies across life history events in wild vertebrates. Comp. Biochem. Physiol. Part A: Mol. Integr. Physiol 256, 110929. doi: 10.1242/jeb.243198
Ensminger D. C., Somo D. A., Houser D. S., Crocker D. E. (2014). Metabolic responses to adrenocorticotropic hormone (ACTH) vary with life-history stage in adult male northern elephant seals. Gen. Comp. Endocrinol 204, 150–157. doi: 10.1016/j.ygcen.2014.04.024
Erbe C., Dunlop R., Dolman S. (2018). Effects of noise on marine mammals. Effects anthropogenic noise Anim, 277–309. doi: 10.1007/978-1-4939-8574-6_10
Erbe C., Marley S. A., Schoeman R. P., Smith J. N., Trigg L. E., Embling C. B. (2019). The effects of ship noise on marine mammals—a review. Front. Mar. Sci 6, 606. doi: 10.3389/fmars.2019.00606
Estes J. A., Doak D., Springer A., Williams T. (2009). Causes and consequences of marine mammal population declines in southwest Alaska: a food-web perspective. Philos. Trans. R. Soc. B: Biol. Sci 364, 1647–1658. doi: 10.1098/rstb.2008.0231
Estes J. A., Heithaus M., McCauley D. J., Rasher D. B., Worm B. (2016). Megafaunal impacts on structure and function of ocean ecosystems. Annu. Rev. Environ. Resour 41, 83–116. doi: 10.1146/annurev-environ-110615-085622
Fair P. A., Houde M. (2023). “Environmental endocrine-disrupting chemicals and their effects in marine mammals,” in Environmental contaminants and endocrine health (Academic Press, an imprint of Elsevier).
Foote A. D., Liu Y., Thomas G. W., Vinař T., Alföldi J., Deng J., et al. (2015). Convergent evolution of the genomes of marine mammals. Nat. Genet 47, 272–275. doi: 10.1038/ng.3198
Forsberg J. S., Hansson M. G., Evers K. (2013). International guidelines on biobank research leave researchers in ambiguity: why is this so? Eur. J. Epidemiol 28, 449–451. doi: 10.1007/s10654-013-9815-x
Fortuna C. M., Fortibuoni T., Bueno-Pardo J., Coll M., Franco A., Giménez J., et al. (2024). Top predator status and trends: ecological implications, monitoring and mitigation strategies to promote ecosystem-based management. Front. Mar. Sci 11, 1282091. doi: 10.3389/fmars.2024.1282091
Fossi M. C., Baini M., Simmonds M. P. (2020). Cetaceans as ocean health indicators of marine litter impact at global scale. Front. Environ. Sci 8, 586627. doi: 10.3389/fenvs.2020.586627
Gilbey J., Carvalho G., Castilho R., Coscia I., Coulson M. W., Dahle G., et al. (2021). Life in a drop: Sampling environmental DNA for marine fishery management and ecosystem monitoring. Mar. Policy 124, 104331. doi: 10.1016/j.marpol.2020.104331
Gobler C. J. (2020). Climate change and harmful algal blooms: insights and perspective. Harmful algae 91, 101731. doi: 10.1016/j.hal.2019.101731
Godard C. A., Smolowitz R. M., Wilson J. Y., Payne R. S., Stegeman J. J. (2004). Induction of cetacean cytochrome P4501A1 by β-naphthoflavone exposure of skin biopsy slices. Toxicological Sci 80, 268–275. doi: 10.1093/toxsci/kfh124
Godard C., Wise S., Kelly R., Goodale B., Kraus S., Romano T., et al. (2006). Benzo [a] pyrene cytotoxicity in right whale (Eubalaena glacialis) skin, testis and lung cell lines. Mar. Environ. Res 62, S20–S24. doi: 10.1016/j.marenvres.2006.04.016
Godard-Codding C. A., Clark R., Fossi M. C., Marsili L., Maltese S., West A. G., et al. (2011). Pacific Ocean–wide profile of CYP1A1 expression, stable carbon and nitrogen isotope ratios, and organic contaminant burden in sperm whale skin biopsies. Environ. Health Perspect 119, 337–343. doi: 10.1289/ehp.0901809
Godard-Codding C. A., Fossi M. C. (2018). “Field Sampling Techniques and Ecotoxicologic Biomarkers in Cetaceans,” in Marine Mammal Ecotoxicology: Impacts of Multiple Stressors on Population Health. eds. Fossi M. C., Panti C. (Elsevier), 237–259. doi: 10.1016/B978-0-12-812144-3.00009-7
Goksøyr A. (2022). “Whales and polar bear in a petri dish: decoding marine mammal toxicology through in vitro and in silico approaches” in 32nd ESCPB Conference (European Society for Comparative Physiology and Biochemistry), Naples, Italy, Aug. 28-31, 2022.
Grady C. (2005). Payment of clinical research subjects. J. Clin. Invest 115, 1681–1687. doi: 10.1172/JCI25694
Green A., Larson S. (2016). A review of organochlorine contaminants in nearshore marine mammal predators. J. Environ. Anal. Toxicol 6, 2161–0525.1000370. doi: 10.4172/2161-0525
Griffeth R. J., García-Párraga D., Mellado-López M., Crespo-Picazo J. L., Soriano-Navarro M., Martinez-Romero A., et al. (2014). Platelet-rich plasma and adipose-derived mesenchymal stem cells for regenerative medicine-associated treatments in bottlenose dolphins (Tursiops truncatus). PloS One 9, e108439. doi: 10.1371/journal.pone.0108439
Guo Y., Gui D., Zhang X., Liu W., Xie Q., Yu X., et al. (2021). Blubber cortisol-based approach to explore the endocrine responses of Indo-Pacific humpback dolphins (Sousa chinensis) to diet shifts and contaminant exposure. Environ. Sci. Technol 56, 1069–1080. doi: 10.1021/acs.est.1c04550
Guo Y., Shi W., Liu Z., Sun X., Wu J., Wu Y. (2023). Bisphenol A alternatives continuously contribute to the endocrine disruption in cetaceans. Environ. Int 171, 107679. doi: 10.1016/j.envint.2022.107679
Gutleb A. C., Cenijn P., Velzen M. V., Lie E., Ropstad E., Skaare J. U., et al. (2010). In vitro assay shows that PCB metabolites completely saturate thyroid hormone transport capacity in blood of wild polar bears (Ursus maritimus). Environ. Sci. Technol 44, 3149–3154. doi: 10.1021/es903029j
Halpern B. S., Frazier M., Potapenko J., Casey K. S., Koenig K., Longo C., et al. (2015). Spatial and temporal changes in cumulative human impacts on the world’s ocean. Nat. Commun 6, 1–7. doi: 10.1038/ncomms8615
Hammerschlag N., Schmitz O. J., Flecker A. S., Lafferty K. D., Sih A., Atwood T. B., et al. (2019). Ecosystem function and services of aquatic predators in the Anthropocene. Trends Ecol. Evol 34, 369–383. doi: 10.1016/j.tree.2019.01.005
Hanna E. M., Zhang X., Eide M., Fallahi S., Furmanek T., Yadetie F., et al. (2020). ReCodLiver0. 9: overcoming challenges in genome-scale metabolic reconstruction of a non-model species. Front. Mol. Biosci 7, 591406. doi: 10.3389/fmolb.2020.591406
Hawkins E. R., Harcourt R., Bejder L., Brooks L. O., Grech A., Christiansen F., et al. (2017). Best practice framework and principles for monitoring the effect of coastal development on marine mammals. Front. Mar. Sci 4, 59. doi: 10.3389/fmars.2017.00059
Herr H., Viquerat S., Devas F., Lees A., Wells L., Gregory B., et al. (2022). Return of large fin whale feeding aggregations to historical whaling grounds in the Southern Ocean. Sci. Rep 12, 9458. doi: 10.1038/s41598-022-13798-7
Herst P. M., Aars J., Joly Beauparlant C., Bodein A., Dalvai M., Gagne D., et al. (2020). Adipose tissue transcriptome is related to pollutant exposure in polar bear mother–cub pairs from Svalbard, Norway. Environ. Sci. Technol 54, 11365–11375. doi: 10.1021/acs.est.0c01920
Hindle A. G., Allen K. N., Batten A. J., Hückstädt L. A., Turner-Maier J., Schulberg S. A., et al. (2019). Low guanylyl cyclase activity in Weddell seals: implications for peripheral vasoconstriction and perfusion of the brain during diving. Am. J. Physiology-Regulatory Integr. Comp. Physiol 316, R704–R715. doi: 10.1152/ajpregu.00283.2018
Hosseini H., Al-Jabri H. M., Moheimani N. R., Siddiqui S. A., Saadaoui I. (2022). Marine microbial bioprospecting: Exploitation of marine biodiversity towards biotechnological applications—a review. J. Basic Microbiol 62, 1030–1043. doi: 10.1002/jobm.202100504
Hunt K. E., Moore M. J., Rolland R. M., Kellar N. M., Hall A. J., Kershaw J., et al. (2013). Overcoming the challenges of studying conservation physiology in large whales: a review of available methods. Conserv. Physiol 1, cot006. doi: 10.1093/conphys/cot006
Hwang J.-H., Kannan K., Evans T. J., Iwata H., Kim E.-Y. (2019). Assessment of risks of dioxins for aryl hydrocarbon receptor-mediated effects in polar bear (Ursus maritimus) by in vitro and in silico approaches. Environ. Sci. Technol 54, 1770–1781. doi: 10.1021/acs.est.9b05941
International Whaling Commission. (2023). International Whaling Commission Ship Strike Database. Available online at: https://portal.iwc.int/shipstrike (Accessed June 26, 2023).
IUCN. (2023). THE IUCN RED LIST OF THREATENED SPECIESTM. Available online at: https://www.iucnredlist.org (Accessed November 10, 2023).
Jain S., Pei L., Spraggins J. M., Angelo M., Carson J. P., Gehlenborg N., et al. (2023). Advances and prospects for the human bioMolecular atlas program (HuBMAP). Nat. Cell Biol 25, 1089–1100. doi: 10.1038/s41556-023-01194-w
Jelincic J., Tift M., Houser D., Crocker D. (2017). Variation in adrenal and thyroid hormones with life-history stage in juvenile northern elephant seals (Mirounga angustirostris). Gen. Comp. Endocrinol 252, 111–118. doi: 10.1016/j.ygcen.2017.08.001
Johnson S. P., Catania J. M., Harman R. J., Jensen E. D. (2012). Adipose-derived stem cell collection and characterization in bottlenose dolphins (Tursiops truncatus). Stem Cells Dev 21, 2949–2957. doi: 10.1089/scd.2012.0039
Jones M. T., Barlow A. E., Villarejo M. (2010). Importance of undergraduate research for minority persistence and achievement in biology. J. Higher Educ 81, 82–115. doi: 10.1080/00221546.2010.11778971
Jones K. E., Bielby J., Cardillo M., Fritz S. A., O'dell J., Orme C. D. L., et al. (2009). PanTHERIA: a species-level database of life history, ecology, and geography of extant and recently extinct mammals: Ecological Archives E090-184. Ecology 90, 2648–2648. doi: 10.1890/08-1494.1
Kaandorp M. L., Lobelle D., Kehl C., Dijkstra H. A., Van Sebille E. (2023). Global mass of buoyant marine plastics dominated by large long-lived debris. Nat. Geosci 16, 689–694. doi: 10.1038/s41561-023-01216-0
Kanter R. D., Monshouwer M., Meijer D., Groothuis G. (2002). Precision-cut organ slices as a tool to study toxicity and metabolism of xenobiotics with special reference to non-hepatic tissues. Curr. Drug Metab 3, 39–59. doi: 10.2174/1389200023338071
Kashiwabara L., Pirard L., Debier C., Crocker D., Khudyakov J. (2023). Effects of cortisol, epinephrine, and bisphenol contaminants on the transcriptional landscape of marine mammal blubber. Am. J. Physiology-Regulatory Integr. Comp. Physiol 325, R504–R522. doi: 10.1152/ajpregu.00165.2023
Keen K. A., Beltran R. S., Pirotta E., Costa D. P. (2021). Emerging themes in population consequences of disturbance models. Proc. R. Soc. B 288, 20210325. doi: 10.1098/rspb.2021.0325
Khudyakov J., Allen K. N., Crocker D. E., Trost N. S., Roberts A. H., Pirard L., et al. (2022). Comprehensive molecular and morphological resolution of blubber stratification in a deep-diving, fasting-adapted seal. Front. Physiol 13, 1057721. doi: 10.3389/fphys.2022.1057721
Khudyakov J., Champagne C., Meneghetti L., Crocker D. (2017). Blubber transcriptome response to acute stress axis activation involves transient changes in adipogenesis and lipolysis in a fasting-adapted marine mammal. Sci. Rep 7, 42110. doi: 10.1038/srep42110
Khudyakov J. I., Champagne C. D., Preeyanon L., Ortiz R. M., Crocker D. E. (2015). Muscle transcriptome response to ACTH administration in a free-ranging marine mammal. Physiol. Genomics 47, 318–330. doi: 10.1152/physiolgenomics.00030.2015
Khudyakov J. I., Deyarmin J. S., Hekman R. M., Pujade Busqueta L., Maan R., Mody M. J., et al. (2018). A sample preparation workflow for adipose tissue shotgun proteomics and proteogenomics. Biol. Open 7, bio036731. doi: 10.1242/bio.036731
Lühmann K., Lille-Langøy R., Øygarden L., Kovacs K. M., Lydersen C., Goksøyr A., et al. (2020). Environmental pollutants modulate transcriptional activity of nuclear receptors of whales in vitro. Environ. Sci. Technol 54, 5629–5639. doi: 10.1021/acs.est.9b06952
Lam E. K., Allen K. N., Torres-Velarde J. M., Vázquez-Medina J. P. (2020). Functional studies with primary cells provide a system for genome-to-phenome investigations in marine mammals. Integr. Comp. Biol 60, 348–360. doi: 10.1093/icb/icaa065
Lambilotte G. (2024). Development of an ex vivo adipose tissue model to study the impact of multiple stressors in arctic marine mammals. In: Faculté des bioingénieurs (Prom. Debier, Cathy: Spinel, Alexandra). Available online at:http://hdl.handle.net/2078.1/thesis:46465.
Landrigan P. J., Stegeman J. J., Fleming L. E., Allemand D., Anderson D. M., Backer L. C., et al. (2020). Human health and ocean pollution. Ann. Global Health 86 (1), 151, 1–64. doi: 10.5334/aogh.2831
Le Boeuf B. J., Condit R., Morris P. A., Reiter J. (2011). The northern elephant seal (Mirounga angustirostris) rookery at Año Nuevo: a case study in colonization. Aquat. Mammals 37, 486. doi: 10.1578/AM.37.4.2011.486
Lewin H. A., Richards S., Lieberman Aiden E., Allende M. L., Archibald J. M., Bálint M., et al. (2022). The earth BioGenome project 2020: Starting the clock. Natl. Acad. Sci. 119 (4). doi: 10.1073/pnas.2115635118
Lille-Langøy R., Goldstone J. V., Rusten M., Milnes M. R., Male R., Stegeman J. J., et al. (2015). Environmental contaminants activate human and polar bear (Ursus maritimus) pregnane X receptors (PXR, NR1I2) differently. Toxicol. Appl. Pharmacol 284, 54–64. doi: 10.1016/j.taap.2015.02.001
Linn M. C., Palmer E., Baranger A., Gerard E., Stone E. (2015). Undergraduate research experiences: Impacts and opportunities. Science 347, 1261757. doi: 10.1126/science.1261757
Louis C., Tift M. S., Crocker D. E., Alexander D., Smith D. R., Debier C. (2015). Isolation of progenitor cells from the blubber of northern elephant seals (Mirounga angustirostris) in order to obtain an in vitro adipocyte model-preliminary results. Mar. Mamm. Sci 31, 764–773. doi: 10.1111/mms.12176
Lowry M. S., Condit R., Hatfield B., Allen S. G., Berger R., Morris P. A., et al. (2014). Abundance, distribution, and population growth of the northern elephant seal (Mirounga angustirostris) in the United States from 1991 to 2010. Aquat. Mammals 40, 20–31. doi: 10.1578/AM.40.1.2014.20
Mackey T. K., Liang B. A. (2012). Integrating biodiversity management and indigenous biopiracy protection to promote environmental justice and global health. Am. J. Public Health 102, 1091–1095. doi: 10.2105/AJPH.2011.300408
Madelaire C. B., Klink A. C., Israelsen W. J., Hindle A. G. (2022). Fibroblasts as an experimental model system for the study of comparative physiology. Comp. Biochem. Physiol. Part B: Biochem. Mol. Biol 260, 110735. doi: 10.1016/j.cbpb.2022.110735
Mahon M. B., Sack A., Aleuy O. A., Barbera C., Brown E., Buelow H., et al. (2024). A meta-analysis on global change drivers and the risk of infectious disease. Nature 629, 830–836. doi: 10.1038/s41586-024-07380-6
Manger P. R. (2022). Grand challenges in mammal science. Front. Mammal Sci 1. doi: 10.3389/fmamm.2022.1057311
McCauley D. J., Pinsky M. L., Palumbi S. R., Estes J. A., Joyce F. H., Warner R. R. (2015). Marine defaunation: animal loss in the global ocean. Science 347, 1255641. doi: 10.1126/science.1255641
McCormley M. C., Champagne C. D., Deyarmin J. S., Stephan A. P., Crocker D. E., Houser D. S., et al. (2018). Repeated adrenocorticotropic hormone administration alters adrenal and thyroid hormones in free-ranging elephant seals. Conserv. Physiol 6, coy040. doi: 10.1093/conphys/coy040
McGee R. Jr., Saran S., Krulwich T. A. (2012). Diversity in the biomedical research workforce: developing talent. Mount Sinai J. Medicine: A J. Trans. Personalized Med 79, 397–411. doi: 10.1002/msj.21310
Meir J. U., Champagne C. D., Costa D. P., Williams C. L., Ponganis P. J. (2009). Extreme hypoxemic tolerance and blood oxygen depletion in diving elephant seals. Am. J. Physiology-Regulatory Integr. Comp. Physiol 297, R927–R939. doi: 10.1152/ajpregu.00247.2009
Mello M. M., Wolf L. E. (2010). The Havasupai Indian tribe case—lessons for research involving stored biologic samples. New Engl. J. Med 363, 204–207. doi: 10.1056/NEJMp1005203
Millum J. (2010). How should the benefits of bioprospecting be shared? Hastings Center Rep 40, 24–33. doi: 10.1353/hcr.0.0227
Morris A., Letcher R., Dyck M., Chandramouli B., Cosgrove J. (2019). Concentrations of legacy and new contaminants are related to metabolite profiles in Hudson Bay polar bears. Environ. Res 168, 364–374. doi: 10.1016/j.envres.2018.10.001
Morrison M., Trevisan R., Ranasinghe P., Merrill G. B., Santos J., Hong A., et al. (2022). A growing crisis for One Health: Impacts of plastic pollution across layers of biological function. Front. Mar. Sci 9, 980705. doi: 10.3389/fmars.2022.980705
Mortimer M., Batley G. (2023). “Marine Contaminants of Emerging Concern.” in Marine Pollution – Monitoring, Management and Mitigation, ed. Reichelt-Brushett A. (Cham: Springer), 285–303. doi: 10.1007/978-3-031-10127-4_13
Nagy J. D., Victor E. M., Cropper J. H. (2007). Why don't all whales have cancer? A novel hypothesis resolving Peto's paradox. Integr. Comp. Biol 47, 317–328. doi: 10.1093/icb/icm062
National Academy of Sciences, Engineering, and Medicine (2017). Approaches to understanding the cumulative effects of stressors on marine mammals. Washington, DC: The National Academies Press. doi: 10.17226/23479
Nelms S. E., Alfaro-Shigueto J., Arnould J. P., Avila I. C., Nash S. B., Campbell E., et al. (2021). Marine mammal conservation: over the horizon. Endangered Species Res 44, 291–325. doi: 10.3354/esr01115
NOAA. (2023). Ocean noise strategy Available online at: https://oceannoise.noaa.gov. (Accessed June 30, 2023).
Northey A. D., Holser R. R., Shipway G. T., Costa D. P., Crocker D. E. (2023). Adrenal response to ACTH challenge alters thyroid and immune function and varies with body reserves in molting adult female northern elephant seals. Am. J. Physiology-Regulatory Integr. Comp. Physiol 325, R1–R12. doi: 10.1152/ajpregu.00277.2022
O’Hara C. C., Frazier M., Halpern B. S. (2021). At-risk marine biodiversity faces extensive, expanding, and intensifying human impacts. Science 372, 84–87. doi: 10.1126/science.abe6731
O’Hara T. M., Hart L. (2018). “Environmental toxicology,” in CRC handbook of marine mammal medicine. Ed. Gulland D. A. W. (6000 Broken Sound Parkway, NW, (Suite 300), Boca Raton, FL, USA 33487: CRC Press).
Pallin L., Botero-Acosta N., Steel D., Baker C., Casey C., Costa D., et al. (2022). Variation in blubber cortisol levels in a recovering humpback whale population inhabiting a rapidly changing environment. Sci. Rep 12, 20250. doi: 10.1126/science.adk4244
Parsons E. C. M., Baulch S., Bechshoft T., Bellazzi G., Bouchet P., Cosentino A. M., et al (2015). Key research questions of global importance for cetacean conservation. Endanger. Species Res. 27, 113–118. doi: 10.3354/esr00655
Pearson H. C., Savoca M. S., Costa D. P., Lomas M. W., Molina R., Pershing A. J., et al. (2023). Whales in the carbon cycle: can recovery remove carbon dioxide? Trends Ecol. Evol 38, 238–249. doi: 10.1016/j.tree.2022.10.012
Penso-Dolfin L., Haerty W., Hindle A., Di Palma F. (2020). microRNA profiling in the Weddell seal suggests novel regulatory mechanisms contributing to diving adaptation. BMC Genomics 21, 1–17. doi: 10.1186/s12864-020-6675-0
Peterson S. H., Ackerman J. T., Holser R. R., Mcdonald B. I., Costa D. P., Crocker D. E. (2023). Mercury bioaccumulation and cortisol interact to influence endocrine and immune biomarkers in a free-ranging marine mammal. Environ. Sci. Technol 57, 5678–5692. doi: 10.1021/acs.est.2c08974
Pirard L., Khudyakov J. I., Crocker D. E., Van Hassel L., Scholl G., Eppe G., et al. (2023). Cortisol and epinephrine alter the adipose functions and the mobilization of PCBs in adipose tissue slices from elephant seal. Front. Mar. Sci 10, 1290472. doi: 10.3389/fmars.2023.1290472
Pirotta E., Schick R. S., Hamilton P. K., Harris C. M., Hewitt J., Knowlton A. R., et al. (2023). Estimating the effects of stressors on the health, survival and reproduction of a critically endangered, long-lived species. Oikos 2023, e09801. doi: 10.1111/oik.09801
Pugliares K. R., Bogomolni A., Touhey K. M., Herzig S. M., Harry C. T., Moore M. J. (2007). Marine mammal necropsy: an introductory guide for stranding responders and field biologists. Woods Hole, MA: Woods Hole Oceanographic Institution, 133pp. (WHOI Technical Report 2007-06). doi: 10.1575/1912/1823
Pujade Busqueta L., Crocker D. E., Champagne C. D., McCormley M. C., Deyarmin J. S., Houser D. S., et al. (2020). A blubber gene expression index for evaluating stress in marine mammals. Conserv. Physiol 8, coaa082. doi: 10.1093/conphys/coaa082
Rajput I. R., Yaqoob S., Yajing S., Sanganyado E., Wenhua L. (2021). Polybrominated diphenyl ethers exert genotoxic effects in pantropic spotted dolphin fibroblast cell lines. Environ. pollut 271, 116131. doi: 10.1016/j.envpol.2020.116131
Read A. J. (2023). The ecology of whales in a changing climate. Science 382, 159–160. doi: 10.1126/science.adk4244
Read A. J., Drinker P., Northridge S. (2006). Bycatch of marine mammals in US and global fisheries. Conserv. Biol 20, 163–169. doi: 10.1111/j.1523-1739.2006.00338.x
Remili A., Dietz R., Sonne C., Samarra F. I., Rikardsen A. H., Kettemer L. E., et al. (2023). Quantitative fatty acid signature analysis reveals a high level of dietary specialization in killer whales across the North Atlantic. J. Anim. Ecol 92, 1216–1229. doi: 10.1111/1365-2656.13920
Robinson K. J., Hall A. J., Debier C., Eppe G., Thomé J.-P., Bennett K. A. (2018). Persistent organic pollutant burden, experimental POP exposure, and tissue properties affect metabolic profiles of blubber from gray seal pups. Environ. Sci. Technol 52, 13523–13534. doi: 10.1021/acs.est.8b04240
Roman J., McCarthy J. J. (2010). The whale pump: marine mammals enhance primary productivity in a coastal basin. PloS One 5, e13255. doi: 10.1371/journal.pone.0013255
Romero L. M. (2004). Physiological stress in ecology: lessons from biomedical research. Trends Ecol. Evol 19, 249–255. doi: 10.1016/j.tree.2004.03.008
Ross P. S., De Swart R. L., Reijnders P., Van Loveren H., Vos J. G., Osterhaus A. (1995). Contaminant-related suppression of delayed-type hypersensitivity and antibody responses in harbor seals fed herring from the Baltic Sea. Environ. Health Perspect 103, 162–167. doi: 10.1289/ehp.95103162
Routti H., Berg M. K., Lille-Langøy R., Øygarden L., Harju M., Dietz R., et al. (2019). Environmental contaminants modulate the transcriptional activity of polar bear (Ursus maritimus) and human peroxisome proliferator-activated receptor alpha (PPARA). Sci. Rep 9, 6918. doi: 10.1038/s41598-019-43337-w
Routti H., Lille-Langøy R., Berg M. K., Fink T., Harju M., Kristiansen K., et al. (2016). Environmental chemicals modulate polar bear (Ursus maritimus) peroxisome proliferator-activated receptor gamma (PPARG) and adipogenesis in vitro. Environ. Sci. Technol 50, 10708–10720. doi: 10.1021/acs.est.6b03020
Sanganyado E., Bi R., Teta C., Buruaem Moreira L., Yu X., Yajing S., et al. (2021). Toward an integrated framework for assessing micropollutants in marine mammals: Challenges, progress, and opportunities. Crit. Rev. Environ. Sci. Technol 51, 2824–2871. doi: 10.1080/10643389.2020.1806663
Schaap I., Buedenbender L., Johann S., Hollert H., Dogruer G. (2023). Impact of chemical pollution on threatened marine mammals: A systematic review. J. Hazardous Materials, 132203. doi: 10.1016/j.jhazmat.2023.132203
Schoeman R. P., Patterson-Abrolat C., Plön S. (2020). A global review of vessel collisions with marine animals. Front. Mar. Sci 7, 292. doi: 10.3389/fmars.2020.00292
Silva F. A., Souza É.M., Ramos E., Freitas L., Nery M. F. (2023). The molecular evolution of genes previously associated with large sizes reveals possible pathways to cetacean gigantism. Sci. Rep 13, 67. doi: 10.1038/s41598-022-24529-3
Simon E., Bytingsvik J., Jonker W., Leonards P. E., De Boer J., Jenssen B. M., et al. (2011). Blood plasma sample preparation method for the assessment of thyroid hormone-disrupting potency in effect-directed analysis. Environ. Sci. Technol 45, 7936–7944. doi: 10.1021/es2016389
Skern-Mauritzen M., Lindstrøm U., Biuw M., Elvarsson B., Gunnlaugsson T., Haug T., et al. (2022). Marine mammal consumption and fisheries removals in the Nordic and Barents Seas. ICES J. Mar. Sci 79, 1583–1603. doi: 10.1093/icesjms/fsac096
Southall B. L., Nowacek D. P., Bowles A. E., Senigaglia V., Bejder L., Tyack P. L. (2021). Marine mammal noise exposure criteria: assessing the severity of marine mammal behavioral responses to human noise. Aquat. Mammals 47, 421–464. doi: 10.1578/AM.47.5.2021.421
Spencer M. B., Noll E., Cassidy E. (2005). Monetary incentives in support of academic achievement: Results of a randomized field trial involving high-achieving, low-resource, ethnically diverse urban adolescents. Eval. Rev 29, 199–222. doi: 10.1177/0193841X04273329
Stanfield E., Slown C. D., Sedlacek Q., Worcester S. E. (2022). A course-based undergraduate research experience (CURE) in biology: Developing systems thinking through field experiences in restoration ecology. CBE—Life Sci. Educ 21, ar20. doi: 10.1187/cbe.20-12-0300
Stat M., Huggett M. J., Bernasconi R., Dibattista J. D., Berry T. E., Newman S. J., et al. (2017). Ecosystem biomonitoring with eDNA: metabarcoding across the tree of life in a tropical marine environment. Sci. Rep 7, 12240. doi: 10.1038/s41598-017-12501-5
Steimle J., Moskowitz I. (2017). TBX5: a key regulator of heart development. Curr. topics Dev. Biol 122, 195–221. doi: 10.1016/bs.ctdb.2016.08.008
Suarez-Bregua P., Alvarez-Gonzalez M., Parsons K. M., Rotllant J., Pierce G. J., Saavedra C. (2022). Environmental DNA (eDNA) for monitoring marine mammals: Challenges and opportunities. Front. Mar. Sci 9, 987774. doi: 10.3389/fmars.2022.987774
Sun L., Rong X., Liu X., Yu Z., Zhang Q., Ren W., et al. (2022). Evolutionary genetics of flipper forelimb and hindlimb loss from limb development-related genes in cetaceans. BMC Genomics 23, 797. doi: 10.1186/s12864-022-09024-3
Tanner R. L., Grover N., Anderson M. L., Crocker K. C., Dutta S., Horner A. M., et al. (2021). Examining cultural structures and functions in biology. Integr. Comp. Biol 61, 2282–2293. doi: 10.1093/icb/icab140
Tartu S., Fisk A. T., Götsch A., Kovacs K. M., Lydersen C., Routti H. (2020). First assessment of pollutant exposure in two balaenopterid whale populations sampled in the Svalbard Archipelago, Norway. Sci. Total Environ 718, 137327. doi: 10.1016/j.scitotenv.2020.137327
Tartu S., Lille-Langøy R., Størseth T. R., Bourgeon S., Brunsvik A., Aars J., et al. (2017). Multiple-stressor effects in an apex predator: combined influence of pollutants and sea ice decline on lipid metabolism in polar bears. Sci. Rep 7, 16487. doi: 10.1038/s41598-017-16820-5
Theissinger K., Fernandes C., Formenti G., Bista I., Berg P. R., Bleidorn C., et al. (2023). How genomics can help biodiversity conservation. Trends Genet 39, 545–559. doi: 10.1016/j.tig.2023.01.005
Torres-Velarde J. M., Kolora S. R. R., Khudyakov J. I., Crocker D. E., Sudmant P. H., Vázquez-Medina J. P. (2021). Elephant seal muscle cells adapt to sustained glucocorticoid exposure by shifting their metabolic phenotype. Am. J. Physiology-Regulatory Integr. Comp. Physiol 321, R413–R428. doi: 10.1152/ajpregu.00052.2021
Tranganida A., Hall A. J., Armstrong H. C., Moss S. E., Bennett K. A. (2023). Consequences of in vitro benzyl butyl phthalate exposure for blubber gene expression and insulin-induced Akt activation in juvenile grey seals. Environ. pollut 316, 120688. doi: 10.1016/j.envpol.2022.120688
Trego M. L., Hoh E., Whitehead A., Kellar N. M., Lauf M., Datuin D. O., et al. (2019). Contaminant exposure linked to cellular and endocrine biomarkers in Southern California bottlenose dolphins. Environ. Sci. Technol 53, 3811–3822. doi: 10.1021/acs.est.8b06487
Valdivia A., Wolf S., Suckling K. (2019). Marine mammals and sea turtles listed under the US Endangered Species Act are recovering. PloS One 14, e0210164. doi: 10.1371/journal.pone.0210164
Vazquez J. M., Kraft M., Lynch V. J. (2022). A CDKN2C retroduplication in Bowhead whales is associated with the evolution of extremely long lifespans and alerted cell cycle dynamics. BioRxiv. , 2022.09. 07.506958. doi: 10.1101/2022.09.07.506958
Vázquez-Medina J. P., Zenteno-Savín T., Tift M. S., Forman H. J., Crocker D. E., Ortiz R. M. (2011). Apnea stimulates the adaptive response to oxidative stress in elephant seal pups. J. Exp. Biol 214, 4193–4200. doi: 10.1242/jeb.063644
Wada H. (2019). Damage-fitness model: the missing piece in integrative stress models. Stress 22, 548–562. doi: 10.1080/10253890.2019.1614556
Watson P. H. (2014). Biobank classification: communicating biorepository diversity. Biopreserv Biobank 12, 163–164. doi: 10.1089/bio.2014.1231
Weijs L., Zaccaroni A. (2016). Toxicology of marine mammals: new developments and opportunities. Arch. Environ. contamination Toxicol 70, 1–8. doi: 10.1007/s00244-015-0233-9
Wiig Ø., Amstrup S., Atwood T., Laidre K., Lunn N., Obbard M., et al. (2015). Ursus maritimus. IUCN Red List Threatened Species 2015, e. doi: 10.2305/IUCN.UK.2015-4.RLTS.T22823A14871490.en
Williams T. M., Blackwell S. B., Richter B., Sinding M.-H. S., Heide-Jørgensen M. P. (2017). Paradoxical escape responses by narwhals (Monodon monoceros). Science 358, 1328–1331. doi: 10.1126/science.aao2740
Williams C. L., Hindle A. G. (2021). Field physiology: studying organismal function in the natural environment. Compr. Physiol 11, 1979–2015. doi: 10.1002/cphy.c200005
Williams C. L., Ponganis P. J. (2021). Diving physiology of marine mammals and birds: the development of biologging techniques. Philos. Trans. R. Soc. B 376, 20200211. doi: 10.1098/rstb.2020.0211
Wilson J., Berntsen H. F., Zimmer K. E., Verhaegen S., Frizzell C., Ropstad E., et al. (2016). Do persistent organic pollutants interact with the stress response? Individual compounds, and their mixtures, interaction with the glucocorticoid receptor. Toxicol. Lett 241, 121–132. doi: 10.1016/j.toxlet.2015.11.014
Zhao Y., Zhang K., Giesy J. P., Hu J. (2015). Families of nuclear receptors in vertebrate models: characteristic and comparative toxicological perspective. Sci. Rep 5, 8554. doi: 10.1038/srep08554
Northern elephant seals (Mirounga angustirostris) provide unparalleled research accessibility among marine mammals. Since their recovery from a near total population collapse in the 1800s, northern elephant seals have re-colonized much of their historical range along the California coast (Lowry et al., 2014). Fortuitously for Challenge 1, animals established large coastal rookeries near major research institutions (Año Nuevo State Reserve, Point Reyes National Seashore) in the mid to late 20th century (Le Boeuf et al., 2011). By providing easy access to these animals, these rookeries have facilitated extensive research on elephant seal behavior, physiology, and life history while also enabling conservation management and monitoring of responses to changing environmental conditions.
Comprehensive in vivo studies of elephant seal physiology have provided a wealth of data for future work, including biologging, endocrine and metabolic challenges, isotopic tracer and metabolic flux experiments, contaminant analyses, translocation, and various “omic” investigations (Crocker et al., 2016; Green and Larson, 2016; Williams and Ponganis, 2021; Blix, 2018; Andrews and Enstipp, 2016; Allen and Vázquez-Medina, 2019; Ensminger et al., 2021b). Research in elephant seals over the past decade has also examined variables influencing baseline variability in stress hormone levels and organismal and tissue-specific responses to stress and low oxygen levels during various life history stages (Ensminger et al., 2021a; Deyarmin et al., 2019; Vázquez-Medina et al., 2011; Meir et al., 2009; Peterson et al., 2023; Pujade Busqueta et al., 2020; Jelincic et al., 2017; Northey et al., 2023; McCormley et al., 2018; Ensminger et al., 2014; Khudyakov et al., 2015, 2017).
More recently, in vivo studies in elephant seals have been complemented by in vitro and ex vivo approaches, which have enabled genetic manipulations, subcellular resolution of stress pathways and contaminant responses, and combinatorial stress exposure experiments (Lam et al., 2020; Debier et al., 2020; Del Águila-Vargas et al., 2020; Pirard et al., 2023). For example, the function of a cortisol-responsive gene previously identified in vivo was examined in cultured elephant seal muscle cells (myotubes) using RNA-seq, pharmacological manipulations, and siRNA gene knockdowns, and found to regulate mitochondrial function and organelle dynamics during prolonged glucocorticoid elevations, providing a mechanistic explanation underlying the resilience of elephant seal muscle tissue to corticosteroids (Torres-Velarde et al., 2021; Khudyakov et al., 2015). Similarly, previously identified cytoprotective pathways conferring hypoxemic tolerance to elephant seals were found to be induced during experimental hypoxia exposure in cultured vascular endothelial cells (Allen et al., 2024; Allen and Vázquez-Medina, 2019; Vázquez-Medina et al., 2011). Moreover, precision-cut adipose tissue slices were recently used to disentangle the role of cortisol and epinephrine in the transcriptional response of blubber to stress, which was previously described in vivo, as well as to determine how the presence of bisphenol contaminants alters such responses (Kashiwabara et al., 2023; Pirard et al., 2023; Khudyakov et al., 2017).
These and other studies in elephant seals may be used as a proof-of-concept to illustrate the biological relevance of in vitro and ex vivo systems in marine mammal research (e.g., many of the same stress-responsive genes were identified using both in vivo and in vitro/ex vivo experiments). The accessibility of free-ranging elephant seals for tissue sampling facilitates the development of cell lines, genetic manipulation techniques, and tissue culture approaches relevant to Challenge 2. These resources, which should be broadly disseminated to the marine mammal community (Challenge 3), can then be applied to other marine mammal systems from which tissue collection is limited. Most importantly, the accessibility of elephant seals on beaches near large population centers and universities, rather than in remote locations or at sea, facilitates the inclusion of diverse researchers, including undergraduate students as outlined in Challenge 4. Involving undergraduate students in research in ecology and other fields - including elephant seal research - has resulted in significant gains in inclusivity and diversity in post-graduate research programs and STEM fields more broadly (Jones et al., 2010; Brint and Cantwell, 2010; Linn et al., 2015; Awad and Brown, 2021; Stanfield et al., 2022). However, as stipulated in Challenge 4, continuous efforts must be made to minimize research impacts on these animals, and all work with elephant seals must be conducted in agreement with Indigenous communities and state and national parks in which their rookeries are located.
The polar bear (Ursus maritimus) offers another compelling example of the value of the middle-out approach in advancing our understanding of how pollutants disrupt endocrine function in marine mammals. The polar bear is a top predator with a circumpolar distribution, inhabiting ice-covered Arctic waters. Classified as vulnerable by the IUCN Red List of Threatened Species, polar bears face numerous stressors, including climate change, pollution, pathogens, and increasing Arctic resource exploration and development (Wiig et al., 2015). Despite logistical and practical challenges in studying polar bears (Challenge 1), sample collection has been possible due to national population monitoring programs and subsistence harvest.
Related to Challenge 2, skin biopsies collected during polar bear monitoring in Svalbard, Norway, allowed the establishment of adipose tissue-derived stem cells (Routti et al., 2016). Further studies showed that exposure to chemical mixtures influenced the transition of these cells to adipocytes (Routti et al., 2016). In addition, skin biopsies from live-captured bears and liver samples from subsistence harvests were instrumental in mechanistic in vitro studies on nuclear receptors, which act as transcription factors that regulate essential physiological processes, including metabolism, the endocrine and immune systems, and reproduction (Zhao et al., 2015). Research on polar bears has revealed that various chemical pollutants modulate nuclear receptors in vitro, particularly those involved in lipid metabolism and detoxification (Lille-Langøy et al., 2015; Routti et al., 2019; Hwang et al., 2019). Comparative testing on human transcription factors showed species-specific differences, further supported by in silico protein structure modeling (Lille-Langøy et al., 2015; Routti et al., 2019). The binding affinities of pollutants to polar bear nuclear receptors have also been predicted using in silico methods followed by in vitro testing (Routti et al., unpublished (Hwang et al., 2019).
In parallel with in vitro experiments, samples were collected from over a hundred free-ranging adult female polar bears to explore the relationship between pollutant exposure and various parameters related to energy metabolism, including gene expression, circulating hormones, lipids and lipoproteins, and metabolites (Tartu et al., 2017). Additionally, transcriptomic analyses were performed on adipose tissue samples from polar bear mother-cub pairs (Herst et al., 2020). Also, a study in male polar bears from Canada investigated changes in the liver metabolome in relation to contaminant exposure (Morris et al., 2019). Altogether, these findings suggest that pollutant exposure disrupts lipid metabolism in polar bears. Both in vitro and correlative field approaches provide strong evidence of pollutant-mediated thyroid disruption in these animals (Braathen et al., 2004; Gutleb et al., 2010; Simon et al., 2011; Bytingsvik et al., 2013; Bourgeon et al., 2017).
Training the next generation to develop and utilize in vitro tools is crucial for polar bear studies (Challenge 4). Young researchers have been trained to analyze complex field data, whereas experienced researchers have primarily carried out sample collection following the guidelines of national authorities. In conclusion, these studies highlight how in vitro and in silico research on nuclear receptors, experimental studies on stem cells, and correlative field studies provide valuable insights into the mechanisms by which pollutants impact polar bears and their physiological condition in the wild. Ongoing research on cellular models and the development of tissue-based cultures will further enhance our understanding of how pollutant exposure disrupts biological processes in polar bears while providing resources and material for the wider marine mammal community (Challenge 3).
Keywords: marine mammals, systems biology, toxicology, physiology, climate change, predictive modeling, functional genomics, endocrine-metabolic disruptors
Citation: Vazquez JM, Khudyakov JI, Madelaire CB, Godard-Codding CA, Routti H, Lam EK, Piotrowski ER, Merrill GB, Wisse JH, Allen KN, Conner J, Blévin P, Spyropoulos DD, Goksøyr A and Vázquez-Medina JP (2024) Ex vivo and in vitro methods as a platform for studying anthropogenic effects on marine mammals: four challenges and how to meet them. Front. Mar. Sci. 11:1466968. doi: 10.3389/fmars.2024.1466968
Received: 18 July 2024; Accepted: 10 September 2024;
Published: 11 October 2024.
Edited by:
Mario Lebrato, Bazaruto Center for Scientific Studies (BCSS), MozambiqueReviewed by:
Wei Liu, Shanghai University, ChinaCopyright © 2024 Vazquez, Khudyakov, Madelaire, Godard-Codding, Routti, Lam, Piotrowski, Merrill, Wisse, Allen, Conner, Blévin, Spyropoulos, Goksøyr and Vázquez-Medina. This is an open-access article distributed under the terms of the Creative Commons Attribution License (CC BY). The use, distribution or reproduction in other forums is permitted, provided the original author(s) and the copyright owner(s) are credited and that the original publication in this journal is cited, in accordance with accepted academic practice. No use, distribution or reproduction is permitted which does not comply with these terms.
*Correspondence: Juan Manuel Vazquez, anVhbkB2YXpxdWV6LmJpbw==; Anders Goksøyr, YW5kZXJzLmdva3NveXJAdWliLm5v; José Pablo Vázquez-Medina, anB2LW1AYmVya2VsZXkuZWR1
Disclaimer: All claims expressed in this article are solely those of the authors and do not necessarily represent those of their affiliated organizations, or those of the publisher, the editors and the reviewers. Any product that may be evaluated in this article or claim that may be made by its manufacturer is not guaranteed or endorsed by the publisher.
Research integrity at Frontiers
Learn more about the work of our research integrity team to safeguard the quality of each article we publish.