- 1Department of Biology, Norwegian University of Science and Technology, Trondheim, Norway
- 2Department of Fisheries and New Biomarine Industry, SINTEF Ocean, Trondheim, Norway
- 3Institute of Marine Ecosystem and Fishery Science, University of Hamburg, Hamburg, Germany
Introduction: With the continuous growth of Atlantic salmon (Salmo salar L.) aquaculture, appropriate management of nutrient-rich waste streams from both land- and sea-based production has become increasingly important. Following a circular approach, nutrients contained in aquaculture sludge can be utilized for biomass production of the polychaete Hediste diversicolor. While the potential for upcycling nutrients has been established previously, this study aimed to assess nutrient flows in H. diversicolor fed aquaculture sludge as understanding these will be crucial for optimizing future large-scale production of this species.
Materials and methods: We investigated the effect of low and high feed supply of smolt sludge on the nutrient flows of carbon (C), nitrogen (N), and phosphorus (P) in H. diversicolor on an individual level. Hereby we assessed ingestion, defecation, excretion, respiration, and uptake for growth.
Results and discussion: Nutrient flows differed between the nutrients C, N, and P in the high feed supply group where C uptake for growth was significantly higher than N and P uptake, and N uptake was significantly higher than P uptake. In contrast, no significant differences were observed in the low feed supply group. In the high feed supply group, most of the ingested C, N, and P by H. diversicolor was allocated to uptake for growth (76% C, 83% N, 85% P) with smaller proportions allocated to defecation (7% C, 7% N, 11% P) and respiration/excretion (18% C, 9% N, 5% P). The results from the low feed supply group suggest insufficient nutrient supply as most ingested C was allocated to respiration (84%), while most N was expended on uptake for growth (45%), and ingested P was either taken up for growth (58%) or spent on defecation (34%). Overall, nutrient uptake reflected the smolt sludge composition rather than nutrient requirements for H. diversicolor, which may be a short-term effect and needs to be investigated further. The flow of nutrients was strongly affected by feed supply, as ingestion, defecation, excretion, and uptake for growth were significantly higher in the high feed supply group than in the low feed supply group.
1 Introduction
Aquaculture of fish has become essential in meeting the global seafood demand, which has surged due to the growing world population and increasing per capita consumption of fish (Béné et al., 2015; van Dijk et al., 2021; FAO, 2022). Capture fisheries are unable to meet the growing demand for aquatic products sustainably as most fish stocks are overexploited or at maximum sustainable capture capacity, which further drives the expansion of aquaculture. In 2022, aquaculture accounted for 51% of the fish consumed globally, providing a reliable source of protein and essential nutrients, supporting food security, and contributing to economic development in many regions (Gephart et al., 2020; FAO, 2024).
With an annual production of 1.52 million tonnes in 2023, Norway is the world’s biggest producer of farmed Atlantic salmon (Salmo salar L.) (Shahbandeh, 2020; Fiskeridirektoratet, 2024a), with land-based salmon production using recirculating aquaculture (RAS) gaining importance. It is used for both smolt and post-smolt production, with some companies having a fully land-based production until slaughter (Davidson et al., 2016; Liu et al., 2016; Bjørndal and Tusvik, 2020). While aquaculture is often considered to be a more sustainable way of producing animal protein for the global market than livestock production (Hilborn et al., 2018; Hallström et al., 2019), there are several ecological issues associated with it. The most critical factors impacting growth of the Norwegian salmon industry are arguably a continued supply of high-quality raw ingredients for feed (Tacon and Metian, 2015; Albrektsen et al., 2022) and adequate handling of salmon lice infestations (Lekang et al., 2016; Dempster et al., 2021), while other challenges such as appropriate management of waste streams from both land- and sea-based aquaculture need to be addressed as well (Bannister et al., 2014; Meriac, 2019; Wang and Olsen, 2023). In their review on sustainable intensification of aquaculture, Campanati et al. (2022) highlight the importance of optimization of feed composition (Martins et al., 2010) and better utilization of nutrients (Turchini et al., 2019). According to calculations by Anglade et al. (2024) based on data on feed use (Fiskeridirektoratet, 2024b), feed composition (Olsen et al., 2008; EWOS, 2022a, 2022), and nutrient retention of 38% of feed carbon (C), 43% of nitrogen (N), and 24% of phosphorus (P) by salmon (Wang et al., 2013), the total unutilized nutrients released from Norwegian salmon production in 2023 amounted to 588,000 tonnes C, 66,000 tonnes N, and 22,000 tonnes P in both dissolved and particulate form.
Consequently, sludge from land-based aquaculture systems is rich in nutrients (Sele et al., 2024) and has been demonstrated to be an appropriate feed source for the cultivation of the polychaete Hediste diversicolor following the concept of integrated multi-trophic aquaculture (IMTA) (Pajand et al., 2017; Wang et al., 2019b; Anglade et al., 2023a). In natural habitats, the species plays a significant ecological role in benthic nutrient cycling through bioturbation activities and particle reworking, which facilitates the breakdown and redistribution of organic matter within the sediment (Smith, 1977; Hedman et al., 2011). When cultivated using waste streams from other industries, polychaetes have the potential to upcycle unutilized organic matter and nutrients. At the same time, nutrients from waste products are transformed into valuable polychaete biomass that is high in lipids and protein and could be used in aquafeeds, thus contributing to a circular bioeconomy (Wang et al., 2019a; Anglade et al., 2023b) and reducing the environmental footprint of feed resources (Monteiro et al., 2024).
Building on the promising findings from previous studies, there remains a need to further understand the nutrient flows when cultivating H. diversicolor on aquaculture sludge. Studying these nutrient dynamics will help to improve the efficiency of sludge upcycling by understanding how effectively different nutrients are utilized. This knowledge is crucial for optimizing the utilization of nutrients contained in aquaculture sludge and maximizing polychaete production yield. In this study, we evaluated the flow and uptake of nutrients contained in smolt sludge by polychaetes H. diversicolor that were fed on two different feed levels. The hypotheses hereby were that (1) uptake (mg g-1 DW polychaete) will be different for C, N, and P; (2) uptake efficiency (% of ingested) will follow nutrient requirements of the species rather than diet composition; and (3) uptake (mg g-1 DW polychaete and % of ingested) and nutrient flows of C, N, and P will be affected by the quantity of feed supplied (low feed supply vs. high feed supply).
2 Materials and methods
2.1 Collection and preparation of Hediste diversicolor
Individuals of H. diversicolor were collected from sediment at low tide at the mud flat of Leangen Bay, Trondheim, Norway (63°26′ 24.5′′ N, 10°28′ 27.7′′ E) in October 2021 (Gomes-Dos-Santos et al., 2021) before visible sexual maturation. After retrieval, polychaetes were transported to the laboratory at SINTEF Ocean in plastic containers filled with sediment collected on-site and covered with seaweed, and transferred to holding tanks (52 × 36 × 18 cm L x W x H) with ample water flow and filled with a 10 cm thick layer of sand. Individuals were acclimated in these flow-through tanks for four weeks at 16L:8D and 16°C and fed commercial fish feed (GEMMA DIAMOND 1.0, Skretting AS, Norway) for three weeks, then smolt sludge the week prior to the experiments.
2.2 Feeding trial
The main experiment consisted of three feeding intervals with subsequent retrieval of feces and was run in a climate-controlled room at a temperature of 12°C and a photoperiod of 16L:8D. Prior to the trial, polychaetes were retrieved from the holding tanks and placed in filtered seawater for ≥ 2 h to allow for gut evacuation. Subsequently, adherent water was removed from the polychaete surface by gentle drying with paper tissues, and individuals were weighed (n = 20, 230 ± 58 mg) using a Handy H 120 balance (Satorius AG, Germany). For an acclimatization period of 72 h (Honda and Kikuchi, 2002), the polychaetes were placed in 250 mL beakers, filled with 150 mL filtered seawater (0.22 µm), containing light-proof PU tubes with an inner diameter of 3 mm, cut to a length of 5 cm, which served as artificial burrows to reduce stress (Galasso et al., 2020). Next, the individuals and their respective tubes were transferred into a new, clean beaker containing filtrated seawater and smolt sludge. The sludge (from Lerøy AS, Belsvik) was centrifuged at 5000 rpm for 10 minutes, the supernatant discarded, and the remaining sludge frozen at -20°C to be thawed immediately before feeding. The composition of smolt sludge used in the feeding trial is shown in Table 1. H. diversicolor was fed sludge at two different feed levels using 10 replicates each. As described by Malzahn et al. (2023), feed levels were calculated based on the N content of smolt sludge (60 mg N g-1 DW) and the whole-body N content of the polychaetes (95 N mg g-1 DW polychaete, Wang et al. (2019b)), and the feed supplied per feeding was equivalent to approximately 6% and 50% of N contained in H. diversicolor, which corresponds to feed levels used by Anglade et al. (2023a, 2023b). Individuals received 101 mg sludge g-1 DW polychaete in the low feed supply group and 817 mg sludge g-1 DW polychaete per feeding. Since polychaetes were fed every second day, following previous studies (Wang et al., 2019b; Anglade et al., 2023a, 2023), this corresponds to 3% N d-1 (S3) and 25 N % d-1 (S25). After a feeding period of 3 h, polychaetes and tubes were again transferred to a clean beaker containing filtrated seawater, and the seawater from the old beaker which contained uneaten sludge (SLU) was homogenized using a blender and frozen in PVC sampling bottles at -20°C for later analyses. Polychaetes stayed in the clean beakers for 45 h to allow for defecation. In like manner, the seawater containing feces (FEC) was then collected, homogenized, and stored at -20°C.
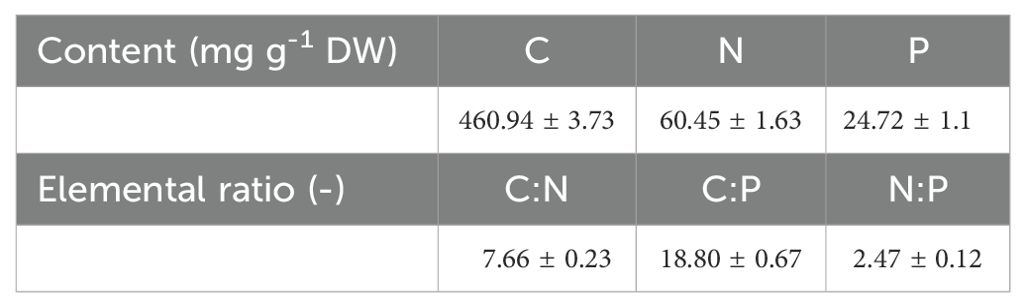
Table 1. Carbon, nitrogen, and phosphorus content (mg g−1 DW polychaete), and C:N, C:P, and N:P ratio (-) smolt sludge (mean ± SE, n = 5).
2.3 Background nutrient levels
Seawater samples were taken and analysed using an autoanalyzer (Flow Solution IV, O.I Analytical) to establish base levels for nitrate + nitrite (NO3-+NO2-), total ammonia nitrogen (TAN), and phosphate (PO43-) concentrations (n = 3). Additionally, two sets of controls were conducted to assess instant leaching (T0) of nutrients from smolt sludge and leaching (L) over a time period of 3 h, which corresponded to the feeding period of the polychaetes. Following the experimental setup, smolt sludge was added on two different levels (corresponding to S3 and S25) that were calculated based on the mean mass and N content of polychaetes. In total, four leaching controls using 4 replicates each were run. Seawater nutrient concentrations were deducted from dissolved nutrient concentrations that were obtained in the polychaete trial and the leaching controls. Results from leaching controls were used to adjust for nutrient leaching from smolt sludge in the polychaete trials. All control samples (seawater and leaching) were homogenized and frozen at -20°C until further analysis.
2.4 Respirometry
Following the setup used by Hansen et al. (2019), a MicroRespiration System (Unisense, Denmark) was used to measure oxygen consumption rates (µmol O2 mg-1 DW h-1), which assuming a respiratory quotient of 1 is equivalent to the release of carbon dioxide (µmol CO2 mg-1 DW h-1). Hereby, the impact of feed level and the feeding state of polychaetes (unfed/fed) was investigated to assess the standard metabolic rate (SMR) and active metabolic rate (AMR), in a similar approach as described by Galasso et al. (2018). In preparation for the measurements, 20 polychaetes were weighed and acclimatized for three days in clean beakers filled with filtered seawater (V = 150 mL) at the same environmental conditions as the main experiment (12°C, 16L:8D), using a climate cabinet (Series KB8000L, Termaks, Sweden). The fed group was given smolt sludge at the same two feed levels as used in the main experiment (S3 and S25) three hours prior to respirometry. The respiration measurement setup included a temperature sensor and two oxygen sensors (OX-MR), which were connected to a MicroSensor Multimeter. These sensors were inserted into MicroRespiration Chambers (MR-Chambers, V = 40 mL), and the chambers were put into a MicroRespiration Rack (MR2-Rack), which was connected to a MicroRespiration Stirrer controller (MR2-Co). To ensure a stable temperature of 12°C during respiration measurements, the MR2-Rack was submerged in a temperature-controlled water bath (300F, Julabo, Germany). The SensorTrace Rate software (application version 2.8.200.21688) was used for sensor calibration, data logging, and calculation of oxygen consumption rates. Respiration chambers were temperature equilibrated for at least 5 min in the water bath before data logging was started. Measurements lasted for 30 min, of which data from at least 5 min was used. The mass of dissolved inorganic carbon (µg DIC mg-1 DW) released by polychaetes during respiration was calculated using the following equation:
where O is the respirometer output showing the reduction in O2 concentration (mmol O2 L-1 h-1), MC is the molar mass of C (12.01 mg mmol-1), V (L) is the volume of the respiration chambers, t is the duration of the experiment (48 h), and m (g DW) is the mass of the subjected polychaetes.
2.5 Chemical analyses
Frozen homogenized samples were thawed and filtered using a vacuum pump (Scanvac VacSafe 15, LaboGene, Denmark) equipped with pre-combusted 47mm glass fiber filters (GF/C, 0.45 μm mesh size, Whatman plc, United Kingdom). Filter punch-outs were used for quantification of particulate C, N, and P contained in SLU and FEC samples. C, N and P content of smolt sludge samples were analysed simultaneously. Content of C and N were analysed by means of gas chromatography using an organic elemental analyser (vario EL cube, Elementar Analysensysteme GmbH, Germany), with acetonitrile as standard. P was oxidized with potassium peroxydisulfate (Koroleff, 1976) and analysed photometrically as phosphate following NS-EN ISO 6878 using an autoanalyzer (Flow Solution IV, O.I Analytical, USA). The filtered seawater samples were collected and analysed for nitrate + nitrite, TAN, and phosphate using the same autoanalyzer.
2.6 Nutrient budget
The nutrient budget of H. diversicolor (Figure 1) was adapted from the mass balance for fish by Olsen et al. (2008) as:
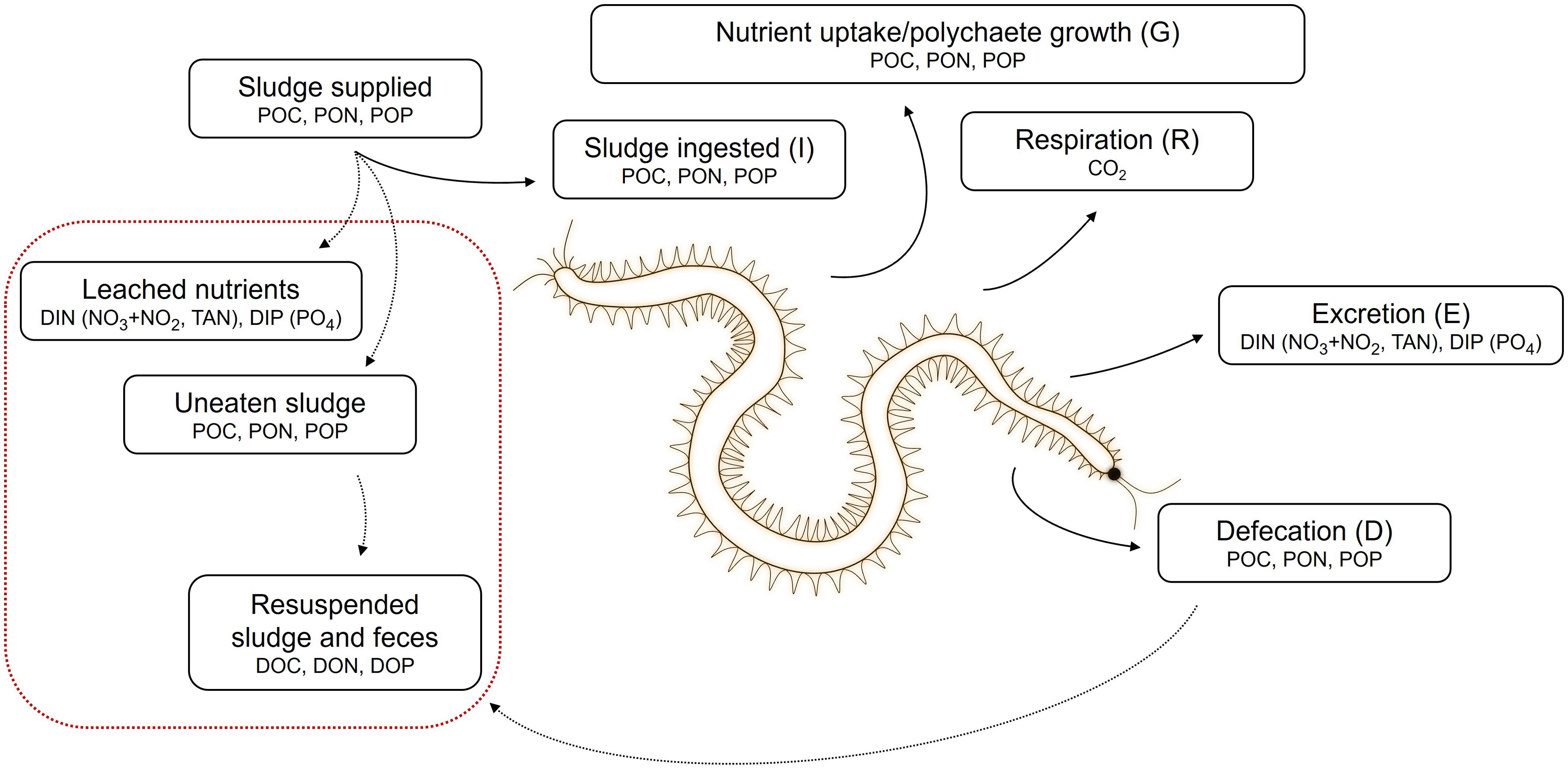
Figure 1. Nutrient budget of H. diversicolor adapted from Olsen et al. (2008). Polychaete illustration by Anglade (2022).
where I is the sludge ingested, A is assimilated nutrients, D is defecation, G is uptake of nutrients allocated for growth, R is respiration, and E is excretion. The uptake for growth was calculated based on the other quantified parameters. Uneaten sludge and leached nutrients were quantified as described previously, while concentrations of resuspended sludge and feces were not measured.
2.7 Statistical analysis
All statistical analyses were conducted using Sigmaplot for Windows Version 15.0 (Systat Software, Inc., USA).
Normal distribution of data was assessed with Shapiro-Wilk tests and variance homogeneity was checked using the Brown-Forsythe test. When comparing two groups, Welch’s t-tests were applied for normally distributed data, while non-normally distributed data was either log-transformed or analysed using the non-parametric Mann-Whitney Rank Sum Test. In the leaching controls, instant leaching (T0) and leaching over time (L) within the high (S25) and low feed supply group (S3) as well as in between those two groups was compared. For each of the nutrients C, N, and P, all measured and calculated parameters that were part of the nutrient budget were tested for significant differences between the S3 and the S25 group.
One-way ANOVA with Pairwise Multiple Comparison (Holm-Sidak post hoc method) was used for comparing data from respirometry. Kruskal-Wallis one-way ANOVA on Ranks for multiple comparisons of non-normally distributed data was used to find significant differences between C, N, and P supplied, ingested, uneaten, contained in feces, assimilation, dissolved inorganic matter, and uptake by polychaetes within feed levels S3 and S25.
Significant differences in data between the three feeding days within feed levels were assessed using a one-way RM ANOVA.
Linear regression followed by one-way ANOVA was conducted to test for correlation between sludge added and seawater nutrient concentrations in control samples, the correlation between polychaete DW and respiration, and correlation between polychaete DW and ingestion, defecation, assimilation, and uptake of C, N, and P.
Statistical analysis was performed at the 95% (p < 0.05) confidence level.
3 Results
3.1 Background nutrient levels
Concentrations of nitrate + nitrite and phosphate in the seawater (mean ± SE) were 136.5 ± 0.9 and 20.7 ± 0.5 µg L-1, respectively, while the concentration of TAN was under the detection limit. No difference was found between instant leaching (T0) of nutrients from smolt sludge and leaching (L) over time (3 h) in neither the low nor the high feed supply control group (Welch’s t-test, p ≥ 0.05), and results were therefore pooled. After adjustment for background nutrient concentrations, leaching of nitrate + nitrite from sludge samples was zero in both the low and the high feed supply control group. Concentrations of TAN and phosphate were significantly higher in the high feed supply control group than in the low feed supply control group (Welch’s t-test, p < 0.05). Linear regression analysis concluded a strong correlation between sludge added (mg DW) and concentrations of phosphate (R2 = 0.932, p < 0.05) and TAN (R2 = 0.865, p < 0.05) in seawater. TAN leaching (mean ± SE) was 3.15 ± 1.28 µg mg-1 DW and 1.86 ± 0.24 µg mg-1 DW for the low and high feed supply control group, respectively, while phosphate leaching was 4.64 ± 0.62 µg mg-1 DW in the low feed supply control group and 2.32 ± 0.43 µg mg-1 DW in the high feed supply control group.
3.2 Respiration in Hediste diversicolor
Respiration in H. diversicolor was not affected by feed supply or feeding state as no significant differences were found between any of the groups (one-way ANOVA, p ≥ 0.05) (Figure 2). In the low feed supply group (S3), the respiratory rate (mean ± SE) was 0.04 ± 0.01 mmol CO2 h-1 g-1 DW (0.45 ± 0.07 mg C h-1 g-1 DW) in unfed polychaetes and 0.03 ± 0.01 mmol CO2 h-1 g-1 DW (0.40 ± 0.07 mg C h-1 g-1 DW) in fed polychaetes. In the high feed supply group (S25), the respiratory rate was 0.05 ± 0.01 mmol CO2 h-1 g-1 DW (0.63 ± 0.06 mg C h-1 g-1 DW) in unfed polychaetes and 0.04 ± 0.01 mmol CO2 h-1 g-1 DW (0.54 ± 0.09 mg C h-1 g-1 DW) in fed polychaetes. Linear regression analysis concluded a strong correlation (R2 = 0.825, p < 0.05) between dry weight and respiration (mmol CO2 h-1) of polychaetes. Consequently, an average respiratory rate of H. diversicolor of 0.50 ± 0.04 mg C h-1 g-1 DW was used for the C budget calculations.
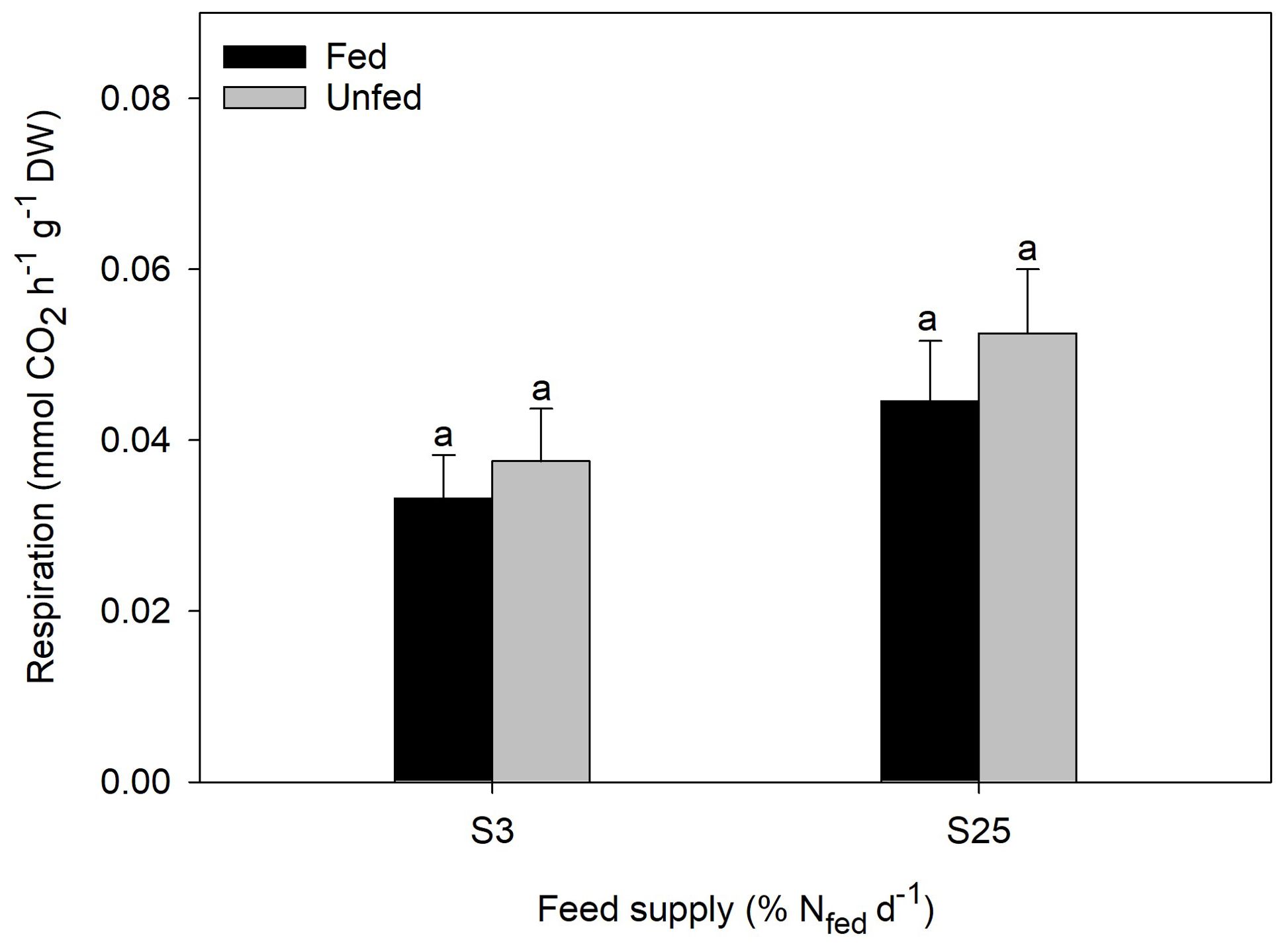
Figure 2. Respiratory rate of H. diversicolor in an unfed and fed state, fed at two feed levels S3 and S25 (n = 10). Same superscripts indicate non-significant differences (p ≥ 0.05).
3.3 Nutrient flows in Hediste diversicolor
Median values (mg g-1 DW polychaete) of supply, ingestion (I), uneaten, defecation (D), assimilation (A), respiration/excretion (R/E), and uptake for growth (G) for the respective nutrients C, N, and P are displayed in Table 2. There was no difference between the three feeding days for any of the measured and calculated parameters (RM ANOVA, p ≥ 0.05).
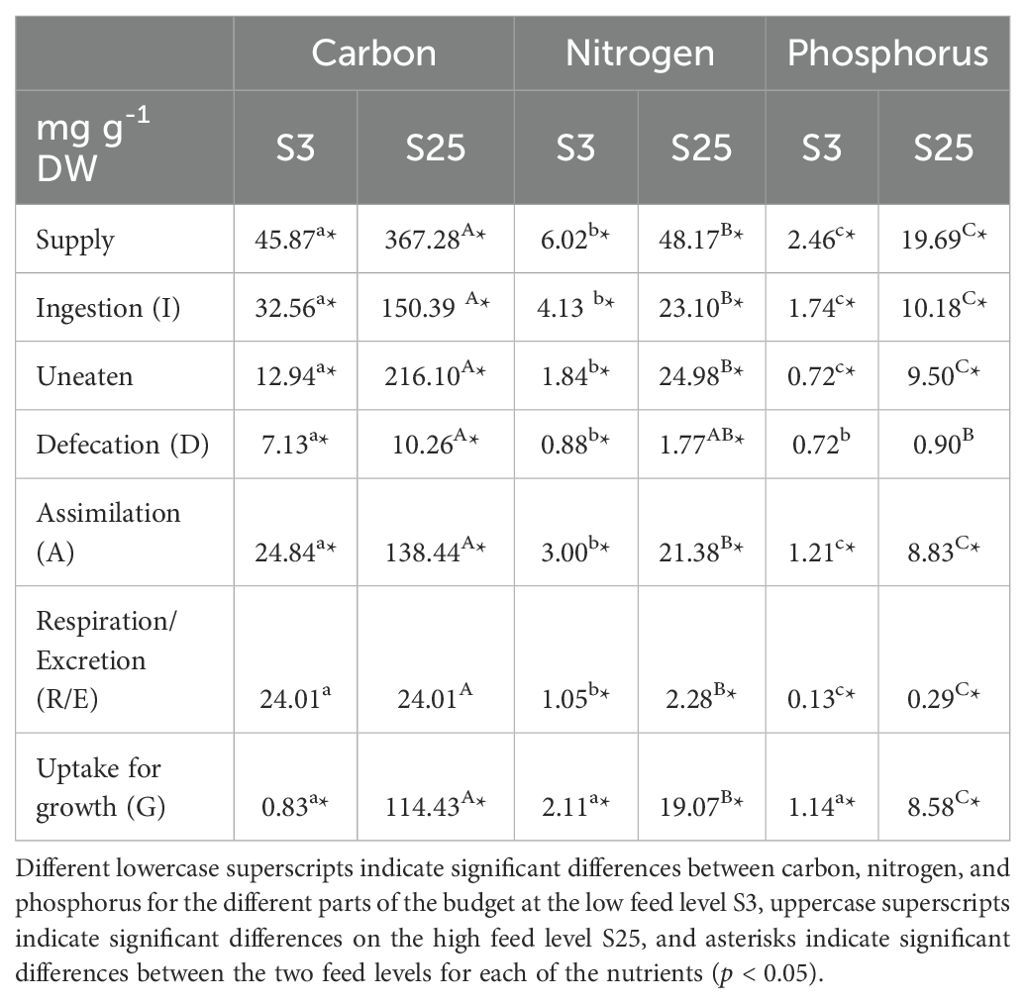
Table 2. Nutrient budget and carbon, nitrogen, and phosphorus flow (median, mg g-1 DW polychaete) in H. diversicolor fed two different feed levels S3 and S25 (n = 10) of smolt sludge.
Apart from uptake for growth, all parameters (supply, ingestion, uneaten, defecation, assimilation, respiration/excretion) presented in Table 2 were significantly higher for C than for N and P, and higher for N than for P (one-way ANOVA on ranks, p < 0.05). With regards to uptake, polychaetes in the low feed supply group (S3) showed no difference in uptake when comparing C, N, and P (one-way ANOVA on ranks, p ≥ 0.05). In the high feed supply group (S25), however, C uptake was higher than that of N and P, and N uptake was higher than that of P (one-way ANOVA on ranks, p < 0.05).
When comparing the same parameters (C, N, and P supply, ingestion, uneaten, defecation, assimilation, respiration/excretion, uptake) for H. diversicolor between feed levels S3 and S25, median values in the high feed supply group (S25) were significantly higher than those in the low feed supply group (S3) for all three nutrients C, N, and P (Mann-Whitney Rank Sum Test, p < 0.05), apart from P contained in feces, where no difference between the high and low feed supply group was found (Mann-Whitney Rank Sum Test, p ≥ 0.05).
Proportions (mg g-1 DW polychaete and %) of ingested C, N, and P (mean ± SE) are shown in Figure 3A–F. In polychaetes in the low feed supply group (S3), the largest proportion of ingested C was allocated to respiration while uptake was around zero (Figures 3A, B). In the high feed supply group (S25), C uptake had the largest share. The assimilation (uptake + respiration) of C was 82% and 93% in the low and high feed supply group, respectively (Figure 3B). The majority of ingested N in polychaetes fed a low feed quantity (S3) was taken up for growth, with lower percentages allocated to defecation and excretion (TAN-N). Polychaetes in the high feed supply group (S25) allocated most of the ingested N to uptake for growth (Figures 3C, D). The assimilation (uptake + excretion) of N was 74% and 93% in the low and high feed supply group, respectively (Figure 3D). The largest proportions of ingested P were allocated to uptake for growth and defecation by polychaetes in the low feed supply group (S3), while uptake accounted for the largest proportion of ingested P in the high feed supply group (S25) (Figures 3E, F). The assimilation (uptake + excretion) of P was 66% and 89% in the low and high feed supply group, respectively (Figure 3F).
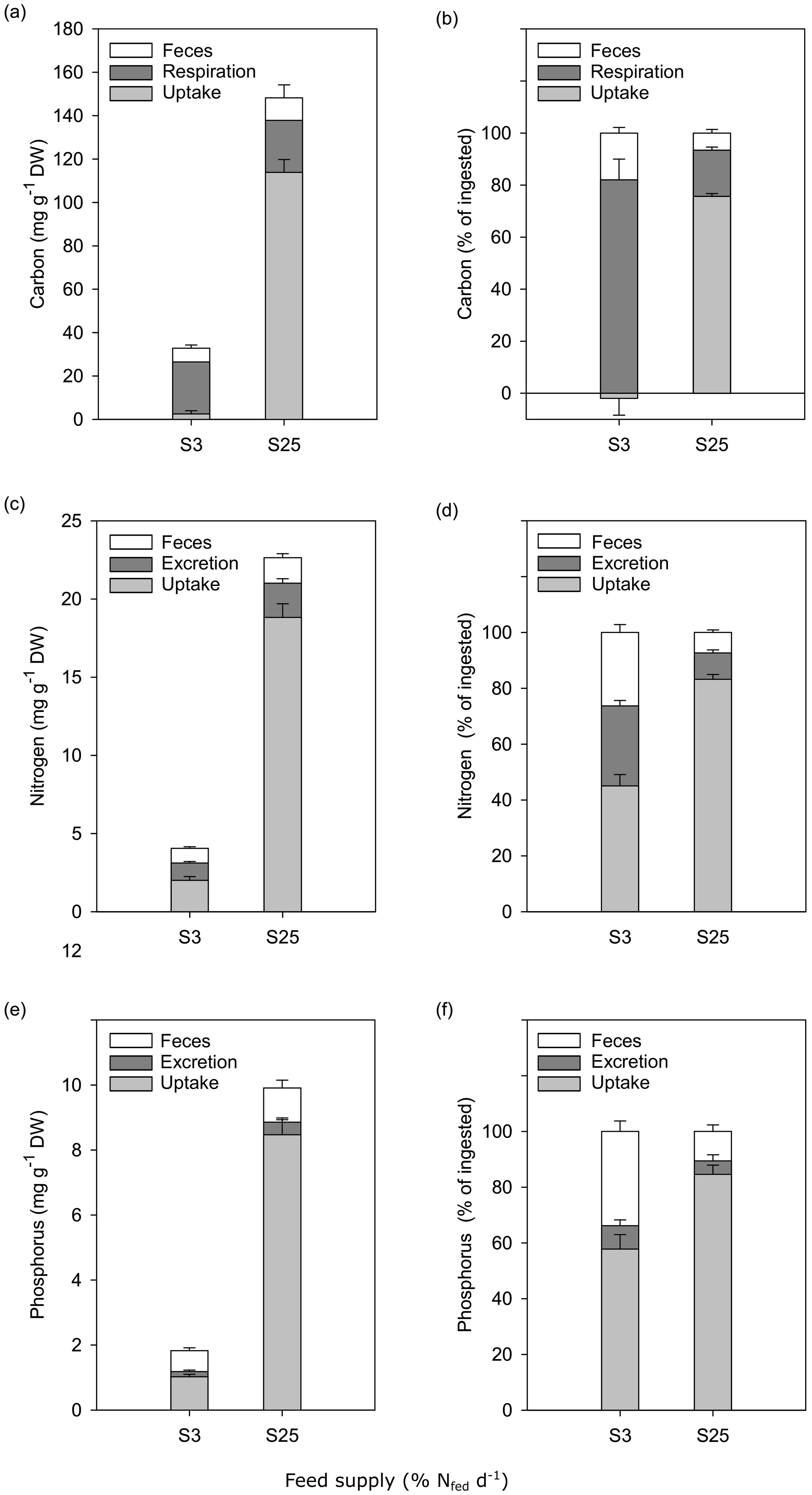
Figure 3. Allocation of ingested carbon, nitrogen, and phosphorus (mean ± SE) in polychaetes H. diversicolor fed at two feed levels S3 and S25 (n = 10). Carbon in mg g-1 DW polychaete (A) and % of ingested (B), nitrogen in mg g-1 DW polychaete (C) and % of ingested (D), and phosphorus in mg g-1 DW polychaete (E) and % of ingested (F).
Linear regression analysis revealed no correlation (R2 < 0.2, p ≥ 0.05) between C, N, and P ingestion, defecation, assimilation, and uptake (mg g-1 DW polychaete) and biomass (g DW) of H. diversicolor for neither of the feed levels S3 and S25 (Supplementary Tables 1–3). A weak negative correlation (0.2 ≤ R2 < 0.4; p < 0.05) was found for C, N, and P defecation by polychaetes in the high feed supply group (S25) and N defecation by polychaetes in the low feed supply group (S3).
Median values (%) of C, N, and P ingestion, assimilation, and uptake for growth as a proportion of supplied C, N, and P are portrayed in Table 3. There was no significant difference in the percentage of C, N, and P ingestion by polychaetes in the low feed supply group (S3) (one-way ANOVA on ranks, p ≥ 0.05). In the high feed supply group (S25), the percentage of C ingestion was significantly lower than that of P ingestion (one-way ANOVA on ranks, p < 0.05), while the percentage of N ingestion was not significantly different from C or P (one-way ANOVA on ranks, p ≥ 0.05). The percentage of C, N, and P ingestion was significantly higher for polychaetes with a low feed supply (S3) than those with a high feed supply (S25) (Mann-Whitney Rank Sum Test, p < 0.05).
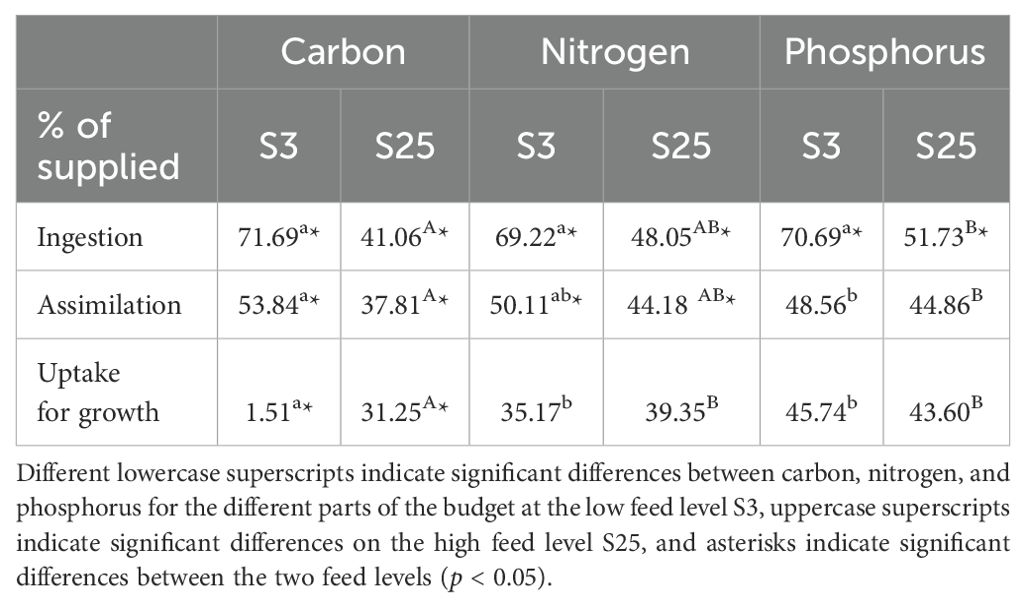
Table 3. Percentages (median) of carbon, nitrogen, and phosphorus ingestion, assimilation, and uptake (median, % of supplied) by H. diversicolor fed two different feed levels S3 and S25 (n = 10) of smolt sludge.
In the low feed supply group (S3), the percentage of assimilated C was significantly higher than assimilated P, while in the high feed supply group (S25), the percentage of C assimilation was significantly lower than P assimilation (one-way ANOVA on ranks, p < 0.05). N assimilation was not different from C and P assimilation in either of the groups (one-way ANOVA on ranks, p ≥ 0.05). The percentage of C and N assimilation was significantly higher in the S3 group than in the S25 group (Mann-Whitney Rank Sum Test, p < 0.05).
The percentage of C uptake was significantly lower than that of N and P, both in polychaetes with low (S3) and high feed supply (S25) (one-way ANOVA on ranks, p < 0.05). When comparing low (S3) and high feed supply (S25), uptake by H. diversicolor as a proportion of nutrients supplied was only significantly different for C, with C uptake being significantly higher in the S25 group (Mann-Whitney Rank Sum Test, p < 0.05). There was no difference in N and P uptake by polychaetes between the two feed levels S3 and S25 (Mann-Whitney Rank Sum Test, p ≥ 0.05).
4 Discussion
Our results show that nutrient flows in H. diversicolor depend both on the type of nutrient and the supplied quantity. While there was no difference between C, N, and P uptake (mg g-1 DW polychaete) in the low feed supply group (S3), differences were pronounced in the high feed supply group (S25). The uptake of C was significantly higher than that of N and P, and N uptake was significantly higher than P uptake, which is in line with results published by Anglade et al. (2023b). Fang et al. (2016) analysed nutrient flows in the nereid polychaete Perinereis aibuhitensis fed fish feces and evaluated the overall assimilation of the diet, unlike in this trial where it was assessed for each of the nutrients C, N, and P individually. They found an assimilation of ca. 55% which is substantially lower than the assimilation of C, N, and P that we observed in H. diversicolor at both feed levels. The uptake of C (% of ingested feed) in the low feed supply group, however, was close to zero, being that all the C ingested was expended spent on respiration and defecation, and the individuals were likely starved. This threshold for starvation has been described previously by Anglade et al. (2023a) where growth was zero below a feed concentration of 5.8% Nfed d-1. A feed concentration that was approximately half of that was used in the present study, resulting in all energy supplied being invested in basic metabolic processes rather than somatic growth. While the feed supplied to H. diversicolor in the low feed supply group (S3) was limiting with regards to C uptake, it was more appropriate for N and P of which 45% and 58%, respectively, were taken up for growth. In the high feed supply group (S25), uptake of all nutrients was significantly higher, with uptake rates ranging between 75% and 84% of ingested nutrients. Lower percentages for C uptake (8%-27%) and N uptake (24%-63%) by nereids have been published previously (Honda and Kikuchi, 2002; Fang et al., 2016). To the authors’ best knowledge, only Clavero et al. (1994) has previously described the P uptake by H. diversicolor with regard to its role in phosphate fluxes between sediment and seawater, however, the individual P uptake was not quantified in their study. The uptake of C, N, and P as a proportion of supplied sludge was higher than reported by Anglade et al. (2023b). When assessing these numbers, it is important to consider the cultivation system and how feed was administered. Omitting the use of sediment in this study not only allowed for the quantification of uneaten sludge and polychaete feces, but also ensured efficient sludge ingestion, with little energy being spent on foraging as there was no need to search for feed within the sediment. Further, there was no competition for feed as each individual was kept separately.
Another important aspect to consider is the smolt sludge used in this trial. The suitability of aquaculture sludge as a feed for H. diversicolor has been demonstrated in multiple studies (Pajand et al., 2017; Wang et al., 2019b; Anglade et al., 2023a). However, as a waste product from salmon production, its composition is subject to change. The wide range of sludge composition has been described by Sele et al. (2024) and depends on several factors, such as salmon feed composition, type of system, fish health, appetite, season, and the stage of the production cycle as well as storage and preservation of sludge (Del Campo et al., 2010; Galasso et al., 2017; Aas and Åsgård, 2017). Although obtained from the same smolt production facility, the composition of smolt sludge used in this trial was somewhat different from that used in a study by Anglade et al. (2023a). While C and N contents (mg g-1 DW) in this study were slightly higher, P content was nearly half. Consequently, the C:P and N:P ratios were approximately double. Nutrient uptake by H. diversicolor depends on different factors, with feed composition arguably being the most important. Hereby, the composition of an organism itself provides important insights into the nutrient requirements and the associated elemental ratios (Sterner and Elser, 2003; Wagner et al., 2013). For H. diversicolor, Anglade et al. (2023b) reported a C content between 419 and 454 mg g-1 DW, an N content of 84-90 mg g-1 DW, and a P content around 8 mg g-1 DW, giving a C:N ratio of 4.6 to 5.3, a C:P ratio of 44.0 to 59.9, and an N:P ratio of 9.7 to 12.0. When comparing these values with the composition of smolt sludge that was used as a diet in the present study, it becomes evident that the somewhat low N and very high P content in sludge would make efficient utilization of all nutrients simultaneously challenging. Remarkably, the elemental ratios when evaluating the uptake of C, N, and P (mg g-1) in our trial were quite similar to those of the fed smolt sludge, which opposes the findings of Anglade et al. (2023b) where uptake followed polychaete composition and appeared mostly independent of diet composition. Whether this discrepancy is due to the shorter duration of the experiment would be recommended to be evaluated in future studies.
Assessing the potential of polychaetes for upcycling of nutrients contained in aquaculture sludge is strongly linked to feeding behavior and feed ingestion, especially when considering large-scale production. The percentage of ingested feed plays a pivotal role in determining the ideal feed concentration, which is key to minimizing the accumulation of residual feed over time as a surplus of feed can contribute to organic buildup and subsequent degradation in water quality. When comparing the absolute values (mg g-1 DW polychaete) of ingested C, N, and P by polychaetes in the different treatment groups, it becomes evident that ingestion was correlated with supply and smolt sludge composition, being that the factor between absolute ingestion values remains constant for all three nutrients. As a result, the percent values of ingestion were similar for C, N, and P. Around 70% of supplied nutrients were ingested in the low feed supply group (S3) and 42-52% in the high feed supply group (S25). While Anglade et al. (2023b) did not quantify ingestion, they found increased growth with increased feed supply, which suggests a similar trend between ingestion and uptake. Honda and Kikuchi (2002) found an N ingestion by Pereinereis vallata corresponding to 28 mg g-1 DW which is similar to N ingestion by H. diversicolor in the high feed supply group (S25). Anglade et al. (2023a) found the highest growth for H. diversicolor of comparable size as individuals used in this study and fed an equivalent to the high feed supply group (S25), with a further increase in feed supply not resulting in additional growth. When imagining larger-scale production scenarios, it will be crucial to balance maximizing polychaete growth while at the same time minimizing residual feed and thus increasing feeding efficiency. Based on our findings it appears that a feed supply close to that used in the high feed supply group (S25) would be suited. Hereby, it will be important also to assess the effect of polychaete size and production temperature on feed ingestion, as these are known to impact growth (Heip and Herman, 1979; Fang et al., 2016) which is linked strongly to feed intake. Our study permitted ingestion only for a limited time, to prevent individuals from defecation during the feeding period, which would have resulted in feces being incorrectly quantified as uneaten sludge. It would be recommended to allow for a longer feeding period in large-scale production.
The percent C and N defecation of H. diversicolor in the low feed supply group (S3) valued at 18% and 26%, respectively, and was similar to C (22-27%) and N (24-28%) defecation reported by Fang et al. (2016) and overall defecation (22%) found by Honda and Kikuchi (2002). No reference values for P were found. While the absolute C, N, and P defecation increased between the low and high feed supply, the percent defecation decreased due to a higher absolute uptake by polychaetes in the high feed supply group (S25).
Galasso et al. (2020) reported an ammonia excretion rate of approximately 0.06 µmol N h-1 for 230 mg (WW) individuals that were fed ad libitum. Honda and Kikuchi (2002) found an N excretion rate of 0.1 mg ind-1 d-1 for P. vallata weighing 600 mg (WW). Applied to our experiment, with a duration of 48 h, this corresponds to 0.90 mg N g-1 DW (Galasso et al., 2020) and 1.70 mg N g-1 DW (Honda and Kikuchi, 2002) which are comparable with the values found for N excretion in the low (S3) and high feed supply group (S25), (1.05 mg g-1 DW and 2.28 mg g-1 DW, respectively). Fang et al. (2016) showed that N excretion in P. aibuhitensis is highly affected by temperature and food quality, with rates ranging between 9% and 70% of ingested N, which the values of 29% (S3) and 9% (S25), that were found for H. diversicolor in this study, fall within.
Overall, the respiration of H. diversicolor was in line with previously reported literature data. The values we found in this trial, ranging from 0.033 mmol CO2 g-1 DW h-1 to 0.052 mmol CO2 g-1 DW h-1 were well within reported values. At 12°C, Kristensen (1983a) found a respiratory rate of 0.039 mmol CO2 g-1 DW h-1 in H. diversicolor, while Nielsen et al. (1995) reported respiration data for individuals cultivated at 15°C that was equivalent to values between 0.023 mmol CO2 g-1 DW h-1 and 0.052 mmol CO2 g-1 DW h-1. Galasso et al. (2018) found an SMR equivalent to 0.006 mmol CO2 g-1 DW h-1, suggesting that our results correspond to the AMR rather than the SMR. Among others, Kristensen (1981) and Nielsen et al. (1995) describe respiration as an exponential function of weight, however, linear regression gave a better fit for our data, meaning the allometric coefficient had a value that was close to one. According to Riisgård (1998), this is more common for young and fast-growing stages. Both Kristensen (1983a) and (Nielsen et al., 1995) found that H. diversicolor ventilated ca 70-80% of the time at a temperature of 12°C. Nereid polychaetes, like most organisms, typically engage in respiration during ventilation, as the two processes are closely linked, and Kristensen (1981) reported that respiration during active ventilation was five to six times higher than in a resting state. Additionally, Fang et al. (2016) found that feed consumption and quality affected the C metabolism with a higher fraction of C being respired when more high-quality feed was ingested by P. aibuhitensis. Findings by Malzahn et al. (2010) suggest that excess diet C is eliminated through respiration in the calanoid copepod Acartia tonsa. The present study aimed to find a difference in respiration between a fed and an unfed state of individuals as well as a difference between low and high feed supply, however, this was not achieved likely due to an overall low feeding activity (visual assessment). As a result, an average value for respiration (which corresponded to literature values of the AMR) was used. Considering differences between resting and active ventilation phases as well as the AMR and SMR, and the effect of ingested feed quantity would significantly impact the calculated number of C uptake for growth by H. diversicolor as respiration accounted for the largest share of energy spent in the form of C, especially in polychaetes in the low feed supply group (S3), and the respired mass of C may have been overestimated.
Previous studies have shown that resuspension and leaching of DOM (DOC, DON, and DOP) may account for 15% of the respective nutrients in salmonid feces (Chen et al., 2003; Sugiura et al., 2006; Wang et al., 2012). DOM was not quantified in our trial, however, the smolt sludge that was used was collected from the drum filter of a land-based smolt production facility where the largest fraction of DOM would already have been eluted. Additionally, the sludge was treated via centrifugation preceding the experiment, with the supernatant being discarded, therefore, the quantity of DOM that could still leach from the sludge is likely minuscule. Furthermore, the release of DOC and DON by H. diversicolor through mucus production was not taken into account in this study. Likely, the portion of nutrients allocated to mucus production and the contribution of these to the overall nutrient budget was limited as no excessive mucus production was observed. Nonetheless, mucus production will contribute to the C and N budget in the form of DOC and DON to a small degree and may vary with increased feed intake and stress (Mouneyrac et al., 2003; Dale et al., 2019).
Coprophagy has previously been observed in nereid polychaetes with feces from other species (Frankenberg and Smith, 1967, Bradshaw et al., 1990). Accordingly, it is possible that individuals in this study consumed their own feces as they were left without feed for 45 h at a time. While this does not affect the final nutrient budget, it is important to note that the nutrient flows may not be as unambiguous as presented in Figure 1.
To evaluate nutrient flows in H. diversicolor, it was essential to conduct our experiments without sediment. While the tubes used as artificial burrows in this study offered a stable and controlled environment, they could potentially restrict the natural behavior of the polychaetes, decrease physiological performance, and affect their feed intake (Kristensen, 1983b). Especially the change in environment from sediment to artificial burrows can contribute to a certain level of stress. To minimize this, we allowed for polychaetes to acclimatize for 72 h prior to the first feeding, following published procedures (Kristensen, 1983c; Honda and Kikuchi, 2002; Galasso et al., 2018). Gas exchange and respiration in H. diversicolor may have been decreased due to the impermeable material of the tubes used in our trial. Levels of dissolved oxygen were not assessed; however, the use of permeable tubes would ensure sufficient circulation of oxygen-rich water through and around the tubes. Even though the transfer of H. diversicolor and tubes between beakers was executed with great care, the handling of polychaetes likely further added stress on individuals which would affect nutrient flows.
5 Conclusion
Overall, we found that nutrients that were contained in smolt sludge were efficiently taken up for growth by polychaetes H. diversicolor and could validate results from previous studies that smolt sludge constitutes an adequate feed source for this species. Further, we could confirm our hypothesis that absolute uptake (mg g-1 DW polychaete) would vary among different nutrients. In the high feed supply group (S25), C uptake was significantly higher than N and P uptake, and N uptake was significantly higher than P uptake. No such differences were observed in the low feed supply group (S3) where the highest percentage of ingested C was allocated to respiration, while most ingested N was used for uptake, with smaller proportions being allocated to defecation and excretion. Most of the P ingested was either taken up or used for defecation. The allocation of nutrients by polychaetes in the low feed supply group suggests an insufficient nutrient supply where most energy that was ingested was spent on basic metabolic processes. In contrast, the largest share of C, N, and P ingested by polychaetes in the high feed supply group was taken up, with smaller proportions allocated to defecation and respiration/excretion. Opposing our second hypothesis, uptake of C, N, and P (% of ingested) seemed to reflect the composition of smolt sludge rather than the composition of H. diversicolor which is closely linked to the nutrient requirements of the species. Whether this is a short-term effect needs to be investigated in future studies. Lastly, we could confirm that nutrient flows and uptake of C, N, and P were significantly affected by the quantity of supplied feed as nearly all assessed parameters (i.e. ingestion, defecation, assimilation, excretion, and uptake) were significantly higher in individuals in the high feed supply group compared with those in the low feed supply group, whereas respiration in H. diversicolor was not affected by feed supply in this study.
Data availability statement
The original contributions presented in the study are included in the article/Supplementary Material. Further inquiries can be directed to the corresponding author.
Ethics statement
The manuscript presents research on animals that do not require ethical approval for their study.
Author contributions
IA: Writing – review & editing, Writing – original draft, Investigation, Formal analysis, Data curation, Conceptualization. HS: Writing – review & editing, Investigation, Formal analysis, Conceptualization. AH: Writing – review & editing, Project administration, Investigation, Funding acquisition, Conceptualization. KR: Writing – review & editing, Supervision, Project administration, Investigation, Funding acquisition, Conceptualization. AM: Writing – review & editing, Supervision, Project administration, Investigation, Funding acquisition, Conceptualization.
Funding
The author(s) declare financial support was received for the research, authorship, and/or publication of this article. We acknowledge funding by the RCN projects POLYCHAETE (#280836), MIND-P (#268338), and the H2020 ERA-NET BlueBio COFUND Project “SIDESTREAM -Secondary bio-production of low trophic organisms utilizing side streams from the Blue and Green sectors to produce novel feed ingredients for European aquaculture” (Grant ID 68), co-funded through national funds provided by the Norwegian Research Council (#311701). Further, we acknowledge internal funding by SINTEF Ocean from the strategic project “RACE Sludge to Feed” with support from SINTEF ACE. The project was carried out in the framework of the national research infrastructure “Norwegian Center for Plankton Technology” (Norwegian Research Council #245937/F50) hosted by SINTEF and NTNU.
Conflict of interest
The authors declare that the research was conducted in the absence of any commercial or financial relationships that could be construed as a potential conflict of interest.
Publisher’s note
All claims expressed in this article are solely those of the authors and do not necessarily represent those of their affiliated organizations, or those of the publisher, the editors and the reviewers. Any product that may be evaluated in this article, or claim that may be made by its manufacturer, is not guaranteed or endorsed by the publisher.
Supplementary material
The Supplementary Material for this article can be found online at: https://www.frontiersin.org/articles/10.3389/fmars.2024.1458426/full#supplementary-material
References
Aas T. S., Åsgård T. E. (2017). Estimated content of nutrients and energy in feed spill and faeces in Norwegian salmon culture 19, 2017, 1–8. Nofima rapportserie.
Albrektsen S., Kortet R., Skov P. V., Ytteborg E., Gitlesen S., Kleinegris D., et al. (2022). Future feed resources in sustainable salmonid production: A review. Rev. Aquac. 14, 1790–1812. doi: 10.1111/raq.12673
Anglade I. (2022). Illustration of the common ragworm Hediste diversicolor (OF Müller 1776) (Annelida: Nereididae).
Anglade I., Dahl T. H., Kristensen B. S. B., Hagemann A., Malzahn A. M., Reitan K. I. (2023a). Biochemical composition of Hediste diversicolor (OF Müller 1776) (Annelida: Nereidae) reared on different types of aquaculture sludge. Front. Mar. Sci. 10. doi: 10.3389/fmars.2023.1197052
Anglade I., Kristensen B. S. B., Dahl T. H., Hagemann A., Malzahn A. M., Reitan K. I. (2023b). Upcycling of carbon, nitrogen, and phosphorus from aquaculture sludge using the polychaete Hediste diversicolor (OF Müller 1776) (Annelida: Nereididae). Front. Sustain. Food Syst. 7. doi: 10.3389/fsufs.2023.1278586
Anglade I., Krogli T. M., Reitan K. I. (2024). Sludge from sea−based Atlantic salmon (Salmo salar L.) production: quantification, composition, and potential application in integrated multi−trophic aquaculture. Aquac. Int. 32, 6707–6726. doi: 10.1007/s10499-024-01485-5
Bannister R. J., Valdemarsen T., Hansen P. K., Holmer M., Ervik A. (2014). Changes in benthic sediment conditions under an Atlantic salmon farm at a deep, well-flushed coastal site. Aquac. Environ. Interact. 5, 29–47. doi: 10.3354/aei00092
Béné C., Barange M., Subasinghe R., Pinstrup-Andersen P., Merino G., Hemre G.-I., et al. (2015). Feeding 9 billion by 2050 – Putting fish back on the menu. Food Secur. 7, 261–274. doi: 10.1007/s12571-015-0427-z
Bjørndal T., Tusvik A. (2020). Economic analysis of on-growing of salmon post-smolts. Aquac. Econ. Manage. 24, 355–386. doi: 10.1080/13657305.2020.1737272
Bradshaw S. A., O'Hara S. C. M., Corner E. D. S., Eglinton G. (1990). Dietary lipid changes during herbivory and coprophagy by the marine invertebrate. Nereis diversicolor. J. Mar. Biol. Assoc. U.K. 70, 771–787. doi: 10.1017/S0025315400059051
Campanati C., Willer D., Schubert J., Aldridge D. C. (2022). Sustainable intensification of aquaculture through nutrient recycling and circular economies: more fish, less waste, blue growth. Rev. Fish. Sci. Aquac. 30, 143–169. doi: 10.1080/23308249.2021.1897520
Chen Y. S., Beveridge M., Telfer T., Roy W. (2003). Nutrient leaching and settling rate characteristics of the faeces of Atlantic salmon (Salmo salar L.) and the implications for modelling of solid waste dispersion. J. Appl. Ichthyol. 19, 114–117. doi: 10.1046/j.1439-0426.2003.00449.x
Clavero V., Xavier Niell F., Fernandez J. (1994). A laboratory study to quantify the influence of Nereis Diversicolor O.F. Müller in the exchange of phosphate between sediment and water. J. Exp. Mar. Biol. Ecol. 176, 257–267. doi: 10.1016/0022-0981(94)90188-0
Dale H., Taylor J. D., Solan M., Lam P., Cunliffe M. (2019). Polychaete mucopolysaccharide alters sediment microbial diversity and stimulates ammonia-oxidising functional groups. FEMS Microbiol. Ecol. 95. doi: 10.1093/femsec/fiy234
Davidson J., May T., Good C., Waldrop T., Kenney B., Terjesen B. F., et al. (2016). Production of market-size North American strain Atlantic salmon Salmo salar in a land-based recirculation aquaculture system using freshwater. Aquac. Eng. 74, 1–16. doi: 10.1016/j.aquaeng.2016.04.007
Del Campo L. M., Ibarra P., Gutiérrez X., Takle H. (2010). Utilization of sludge from recirculation aquaculture Vol. 9 (Norway: Nofima), 1–63. Nofima rapportserie.
Dempster T., Overton K., Bui S., Stien L. H., Oppedal F., Karlsen Ø., et al. (2021). Farmed salmonids drive the abundance, ecology and evolution of parasitic salmon lice in Norway. Aquac. Environ. Interact. 13, 237–248. doi: 10.3354/aei00402
EWOS (2022a). EWOS CLEAR 200 Extruded feed for salmon and trout in fresh water (Bergen, Norway: EWOS AS).
Fang J., Zhang J., Jiang Z., Du M., Liu Y., Mao Y., et al. (2016). Environmental remediation potential of Perinereis aibuhitensis (Polychaeta) based on the effects of temperature and feed types on its carbon and nitrogen budgets. Mar. Biol. Res. 12, 583–594. doi: 10.1080/17451000.2016.1177653
FAO (2022). “The state of world fisheries and aquaculture 2022,” in Towards Blue Transformation (FAO, Rome, Italy). doi: 10.4060/cc0461en
FAO (2024). “The state of world fisheries and aquaculture 2024,” in Blue Transformation in Action (FAO, Rome, Italy). doi: 10.4060/cd0683en
Fiskeridirektoratet (2024a). Uttak av slaktet fisk fordelt på art 2017-2023 (produksjonsområde). Available online at: https://www.fiskeridir.no/Akvakultur/Tall-og-analyse/Biomassestatistikk/Biomassestatistikk-etter-produksjonsomraade (Accessed 28.01.2024).
Fiskeridirektoratet (2024b). Forbruk av fôr fordelt på art 2017-2023 (produksjonsområde). Available online at: https://www.fiskeridir.no/Akvakultur/Tall-og-analyse/Biomassestatistikk/Biomassestatistikk-etter-produksjonsomraade (Accessed 22.01.2024).
Frankenberg D., Smith J. K. (1967). Coprophagy in marine animals. Limnol. Oceanogr. 12, 443–450. doi: 10.4319/lo.1967.12.3.0443
Galasso H. L., Callier M. D., Bastianelli D., Blancheton J.-P., Aliaume C. (2017). The potential of near infrared spectroscopy (NIRS) to measure the chemical composition of aquaculture solid waste. Aquaculture 476, 134–140. doi: 10.1016/j.aquaculture.2017.02.035
Galasso H. L., Lefebvre S., Aliaume C., Sadoul B., Callier M. D. (2020). Using the Dynamic Energy Budget theory to evaluate the bioremediation potential of the polychaete Hediste diversicolor in an integrated multi-trophic aquaculture system. Ecol. Model. 437, 109296. doi: 10.1016/j.ecolmodel.2020.109296
Galasso H. L., Richard M., Lefebvre S., Aliaume C., Callier M. D. (2018). Body size and temperature effects on standard metabolic rate for determining metabolic scope for activity of the polychaete Hediste (Nereis) diversicolor. PeerJ 6, e5675. doi: 10.7717/peerj.5675
Gephart J. A., Golden C. D., Asche F., Belton B., Brugere C., Froehlich H. E., et al. (2020). Scenarios for global aquaculture and its role in human nutrition. Rev. Fish. Sci. Aquac. 29, 122–138. doi: 10.1080/23308249.2020.1782342
Gomes-Dos-Santos A., Hagemann A., Valente L., Malzahn A. M., Monroig Ó., Froufe E., et al. (2021). Complete mitochondrial genome of the ragworm annelid Hediste diversicolor (OF Müller 1776) (Annelida: Nereididae). Mitochondrial DNA B 6, 2849–2851. doi: 10.1080/23802359.2021.1970644
Hallström E., Bergman K., Mifflin K., Parker R., Tyedmers P., Troell M., et al. (2019). Combined climate and nutritional performance of seafoods. J. Clean. Prod. 230, 402–411. doi: 10.1016/j.jclepro.2019.04.229
Hansen B. H., Malzahn A., Hagemann A., Farkas J., Skancke J., Altin D., et al. (2019). Acute and sub-lethal effects of an anionic polyacrylamide on sensitive early life stages of Atlantic cod (Gadus morhua). Sci. Total Environ. 652, 1062–1070. doi: 10.1016/j.scitotenv.2018.10.310
Hedman J. E., Gunnarsson J. S., Samuelsson G., Gilbert F. (2011). Particle reworking and solute transport by the sediment-living polychaetes Marenzelleria neglecta and Hediste diversicolor. J. Exp. Mar. Biol. Ecol. 407, 294–301. doi: 10.1016/j.jembe.2011.06.026
Heip C., Herman R. (1979). Production of Nereis diversicolor O. F. Müller (Polychaeta) in a shallow brackish-water pond. Estuar. Coast. Mar. Sci. 8, 297–305. doi: 10.1016/0302-3524(79)90047-1
Hilborn R., Banobi J., Hall S. J., Pucylowski T., Walsworth T. E. (2018). The environmental cost of animal source foods. Front. Ecol. Environ. 16, 329–335. doi: 10.1002/fee.1822
Honda H., Kikuchi K. (2002). Nitrogen budget of polychaete Perinereis nuntia vallata fed on the feces of Japanese flounder. Fish. Sci. 68, 1304–1308. doi: 10.1046/j.1444-2906.2002.00568.xiv
Kristensen E. (1981). Direct measurement of ventilation and oxygen uptake in three species of tubicolous polychaetes (Nereis spp.). J. Comp. Physiol. 145, 45–50. doi: 10.1007/bf00782592
Kristensen E. (1983a). Ventilation and oxygen uptake by three species of Nereis (Annelida: Polychaeta). II. Effects of temperature and salinity changes. Mar. Ecol. Prog. Ser. 12, 299–306. doi: 10.3354/meps012299
Kristensen E. (1983b). Comparison of polychaete (Nereis spp.) ventilation in plastic tubes and natural sediment. Mar. Ecol. Prog. Ser. 12, 307–309. doi: 10.3354/meps012307
Kristensen E. (1983c). Ventilation and oxygen uptake by three species of Nereis (Annelida: Polychaeta). I. Effects of hypoxia. Mar. Ecol. Prog. Ser. 12, 289–297. doi: 10.3354/meps012289
Koroleff F. (1976). Determination of nutrients. In: GRASSHOF E., KREMLING E. (eds.) Methods of seawater analysis. New York, USA: Verlag Chemie Weinhein, 117–181.
Lekang O. I., Salas-Bringas C., Bostock J. C. (2016). Challenges and emerging technical solutions in on-growing salmon farming. Aquac. Int. 24, 757–766. doi: 10.1007/s10499-016-9994-z
Liu Y., Rosten T. W., Henriksen K., Hognes E. S., Summerfelt S., Vinci B. (2016). Comparative economic performance and carbon footprint of two farming models for producing Atlantic salmon (Salmo salar): Land-based closed containment system in freshwater and open net pen in seawater. Aquac. Eng. 71, 1–12. doi: 10.1016/j.aquaeng.2016.01.001
Malzahn A. M., Hantzsche F., Schoo K. L., Boersma M., Aberle N. (2010). Differential effects of nutrient-limited primary production on primary, secondary or tertiary consumers. Oecologia 162, 35–48. doi: 10.1007/s00442-009-1458-y
Malzahn A. M., Villena-Rodríguez A., Monroig Ó., Johansen Å., Castro L. F. C., Navarro J. C., et al. (2023). Diet rather than temperature determines the biochemical composition of the ragworm Hediste diversicolor (OF Müller 1776) (Annelida: Nereidae). Aquaculture 569, 739368. doi: 10.1016/j.aquaculture.2023.739368
Martins C. I. M., Eding E. H., Verdegem M. C. J., Heinsbroek L. T. N., Schneider O., Blancheton J. P., et al. (2010). New developments in recirculating aquaculture systems in Europe: A perspective on environmental sustainability. Aquac. Eng. 43, 83–93. doi: 10.1016/j.aquaeng.2010.09.002
Meriac A. (2019). Smolt production and the potential for solid waste collection in Norway 25, 2019, 1–12. Nofima rapportserie.
Monteiro M., Costa R. S., Sousa V., Marques A., Sá T., Thoresen L., et al. (2024). Towards sustainable aquaculture: Assessing polychaete meal (Alitta virens) as an effective fishmeal alternative in European seabass (Dicentrarchus labrax) diets. Aquaculture 579, 740257. doi: 10.1016/j.aquaculture.2023.740257
Mouneyrac C., Mastain O., Amiard J.-C., Amiard-Triquet C., Beaunier P., Jeantet A. Y., et al. (2003). Trace-metal detoxification and tolerance of the estuarine worm Hediste diversicolor chronically exposed in their environment. Mar. Biol. 143, 731–744. doi: 10.1007/s00227-003-1124-6
Nielsen A., Eriksen N., Iversen J., Riisgård H. (1995). Feeding, growth and respiration in the polychaetes Nereis diversicolor (facultative filter-feeder) and N. virens (omnivorous)-a comparative study. Mar. Ecol. Prog. Ser. 125, 149–158. doi: 10.3354/meps125149
Olsen L. M., Holmer M., Olsen Y. (2008). “Perspectives of nutrient emission from fish aquaculture in coastal waters,” in Literature review with evaluated state of knowledge (FHF project). doi: 10.13140/RG.2.1.1273.8006
Pajand Z. O., Soltani M., Bahmani M., Kamali A. (2017). The role of polychaete Nereis diversicolor in bioremediation of wastewater and its growth performance and fatty acid composition in an integrated culture system with Huso huso (Linnaeus 1758). Aquac. Res. 48, 5271–5279. doi: 10.1111/are.13340
Riisgård H. U. (1998). No foundation of a “3/4 power scaling law“ for respiration in biology. Ecol. Lett. 1, 71–73. doi: 10.1046/j.1461-0248.1998.00020.x
Sele V., Ali A., Liland N., Lundebye A.-K., Tibon J., Araujo P., et al. (2024). Characterization of nutrients and contaminants in fish sludge from Atlantic salmon (Salmo salar L.) production sites - A future resource. J. Environ. Manage. 360, 121103. doi: 10.1016/j.jenvman.2024.121103
Shahbandeh M. (2020). Distribution of salmon production worldwide in 2018, by leading country. Available online at: https://www.statista.com/statistics/1182142/leading-salmon-producers-worldwide/ (Accessed 09.12.2023).
Smith R. (1977). The physiological and reproductive adaptations of Nereis diversicolor to life in the Baltic Sea and adjacent waters (Los Angeles, USA: Allan Hancock Press).
Sterner R. W., Elser J. J. (2003). Ecological stoichiometry: the biology of elements from molecules to the biosphere (Princeton, New Jersey, USA: Princeton University Press).
Sugiura S. H., Marchant D. D., Kelsey K., Wiggins T., Ferraris R. P. (2006). Effluent profile of commercially used low-phosphorus fish feeds. Environ. pollut. 140, 95–101. doi: 10.1016/j.envpol.2005.06.020
Tacon A. G. J., Metian M. (2015). Feed matters: satisfying the feed demand of aquaculture. Rev. Fish. Sci. Aquac. 23, 1–10. doi: 10.1080/23308249.2014.987209
Turchini G. M., Trushenski J. T., Glencross B. D. (2019). Thoughts for the future of aquaculture nutrition: realigning perspectives to reflect contemporary issues related to judicious use of marine resources in aquafeeds. N. Am. J. Aquacult. 81, 13–39. doi: 10.1002/naaq.10067
van Dijk M., Morley T., Rau M. L., Saghai Y. (2021). A meta-analysis of projected global food demand and population at risk of hunger for the period 2010–2050. Nat. Food 2, 494–501. doi: 10.1038/s43016-021-00322-9
Wagner N. D., Hillebrand H., Wacker A., Frost P. C. (2013). Nutritional indicators and their uses in ecology. Ecol. Lett. 16, 535–544. doi: 10.1111/ele.12067
Wang X., Andresen K., Handå A., Jensen B., Reitan K. I., Olsen Y. (2013). Chemical composition and release rate of waste discharge from an Atlantic salmon farm with an evaluation of IMTA feasibility. Aquac. Environ. Interact. 4, 147–162. doi: 10.3354/aei00079
Wang H., Hagemann A., Reitan K., Ejlertsson J., Wollan H., Handå A., et al. (2019a). Potential of the polychaete Hediste diversicolor fed on aquaculture and biogas side streams as an aquaculture food source. Aquac. Environ. Interact. 11, 551–562. doi: 10.3354/aei00331
Wang C., Olsen Y. (2023). Quantifying regional feed utilization, production and nutrient waste emission of Norwegian salmon cage aquaculture. Aquac. Environ. Interact. 15, 231–249. doi: 10.3354/aei00463
Wang X., Olsen L. M., Reitan K. I., Olsen Y. (2012). Discharge of nutrient wastes from salmon farms: environmental effects, and potential for integrated multi-trophic aquaculture. Aquac. Environ. Interact. 2, 267–283. doi: 10.3354/aei00044
Wang H., Seekamp I., Malzahn A. M., Hagemann A., Carvajal A. K., Slizyte R., et al. (2019b). Growth and nutritional composition of the polychaete Hediste diversicolor (OF Müller 1776) cultivated on waste from land-based salmon smolt aquaculture. Aquaculture 502, 232–241. doi: 10.1016/j.aquaculture.2018.12.047
Keywords: Hediste diversicolor, nutrient upcycling, polychaeta, salmon aquaculture, aquaculture sludge, nutrient uptake, nutrient budget
Citation: Anglade I, Sæther HOE, Hagemann A, Reitan KI and Malzahn AM (2024) Nutrient upcycling and flows of carbon, nitrogen, and phosphorus in Hediste diversicolor (OF Müller, 1776) (Annelida: Nereididae) fed aquaculture sludge. Front. Mar. Sci. 11:1458426. doi: 10.3389/fmars.2024.1458426
Received: 02 July 2024; Accepted: 30 August 2024;
Published: 18 September 2024.
Edited by:
Ana Pombo, Center for Marine and Environmental Sciences (MARE- IPLeiria), PortugalReviewed by:
Ingrid Lupatsch, AB Agri Ltd, United KingdomD. K. Meena, Central Inland Fisheries Research Institute (ICAR), India
Copyright © 2024 Anglade, Sæther, Hagemann, Reitan and Malzahn. This is an open-access article distributed under the terms of the Creative Commons Attribution License (CC BY). The use, distribution or reproduction in other forums is permitted, provided the original author(s) and the copyright owner(s) are credited and that the original publication in this journal is cited, in accordance with accepted academic practice. No use, distribution or reproduction is permitted which does not comply with these terms.
*Correspondence: Inka Anglade, aW5rYS5zZWVrYW1wQG50bnUubm8=