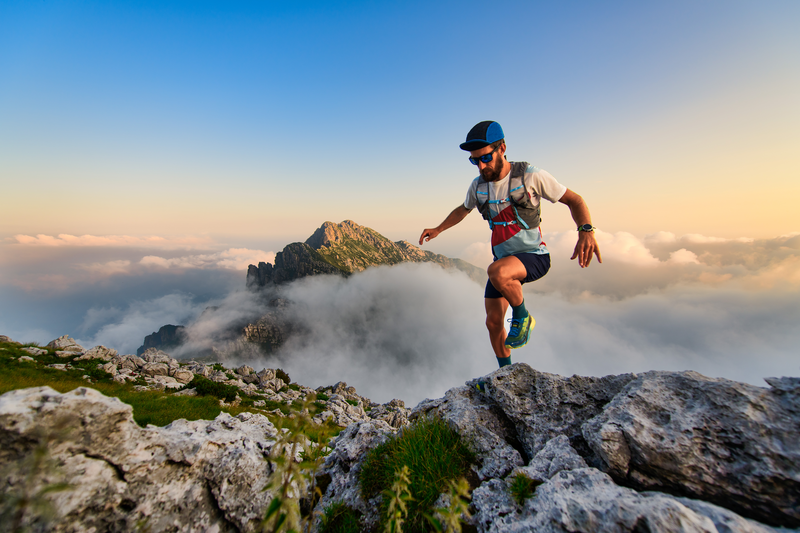
95% of researchers rate our articles as excellent or good
Learn more about the work of our research integrity team to safeguard the quality of each article we publish.
Find out more
REVIEW article
Front. Mar. Sci. , 15 November 2024
Sec. Marine Biotechnology and Bioproducts
Volume 11 - 2024 | https://doi.org/10.3389/fmars.2024.1457500
This article is part of the Research Topic Marine-based Compounds for Sustainable Agriculture View all articles
Seaweeds are a natural marine resource containing many bioactive compounds such as amino acids, lipids, carbohydrates, proteins, phytohormones, and antimicrobial compounds. Since ancient times, seaweeds have been used in various sectors, including medicine, food, and the cosmetic industry. Currently, seaweeds are a promising alternative to reduce the application of harmful chemicals in agriculture. Seaweed and its derived products have been utilized for plant growth promotion, immunity enhancement, and the reduction of biotic and abiotic stresses. In the current global scenario, synthetic fertilizers and chemical pesticides are commonly used to increase agricultural crop production to meet the growing demands of the world population. However, these chemicals pose significant threats to the health of people, livestock, plants, soil, and the entire environment. In contrast, seaweed-based products are emerging as a newer option for stress mitigation and reduction, offering an alternative to synthetic chemicals. This article explains the use of seaweed extracts to increase the tolerance of plants to biotic and abiotic stresses. We also address the functions of various bioactive compounds present in seaweed extracts and the mechanisms by which they promote plant growth and induce defense against different stresses.
Agricultural fertile land is shrinking globally due to the harmful effects of climate change, urbanization, industrialization, random application of harmful chemicals, and several other anthropogenic activities (Mukherjee et al., 2021a, 2021b). Moreover, several biotic stresses like fungal, bacterial, and viral pathogen attacks on crop plants affect the world’s agricultural production (Mukherjee and Patel, 2020). These stresses reduce the quality, quantity, growth, and yield of crops. More than 50% loss in the average yield was reported to be due to abiotic stresses in many crops (Vinocur and Altman, 2005). Additionally, changes in environmental conditions can make the plant susceptible to different plant pathogens (Elad and Pertot, 2014). There are many other factors that threaten crop productivity, such as the development of resistance in plant pathogens against pesticides, which are continuously in use to manage different plant diseases. Therefore, many experiments have been conducted to establish alternative methods for the management of plant biotic and abiotic stresses to enhance crop health and development (Wackett, 2020). The current global scenario is experiencing a demand for organic and agroecological farming, which can avoid the harmful effects of synthetic agrochemicals and focus on natural resources to enhance plant growth attributes. Abiotic factors in plants, including drought and salinity stress, serve as limiting factors for plant development and crop yield, and they also disrupt the physiological characteristics of plants. These changes affect plant morphology and the gene expression responsible for metabolism, stomatal activity, and photosynthesis (Ma et al., 2020). Conversely, several phytohormones, including salicylic acid, ethylene, and abscisic acid, aid in overcoming these adversities and triggering stress tolerance responses (Berens et al., 2019). In addition, farmers rely on the excessive use of synthetic chemicals and fertilizers to protect the crops from biotic stresses. These chemicals leave short-term and long-term harmful effects on the environment (Damalas and Koutroubas, 2016). Therefore, there is a requirement for healthy and natural alternative strategies to reduce the dependency on synthetic chemicals. Seaweeds and products based on seaweed act as biostimulants and have the capacity to lower the enormous use of organic fertilizers and chemicals (Yakhin et al., 2017). The use of biostimulants through natural extracts from seaweeds can be a sustainable technology for the increment in food production without adversely impacting the environment (Hernández-Herrera et al., 2014a). Recently, Gunupuru et al. (2019) reported successful seaweed extract use in controlling several diseases in wheat, including wheat head blight. Similarly, in peas, the infection of powdery mildew was successfully controlled with the application of seaweed extracts (Patel et al., 2020). In addition, the use of seaweed extracts in soybean plants was successful in reducing the harmful effects of abiotic stresses (Shukla et al., 2018). The current analysis in this article is based on the use of seaweed and seaweed-based products for the production and protection of crops.
Seaweeds are macroscopic, multicellular organisms rich in lipids, proteins, carbohydrates, enzymes, and bioactive compounds and have the capacity to tolerate adverse situations such as high temperatures, salinity, and light (Shukla et al., 2016; Gunupuru et al., 2019; Patel et al., 2020). Ascophyllum, Fucus, and Laminaria are the main genera belonging to the brown algae. The use of seaweeds enduring ancient in agriculture as fertilizer, organic matter, and a biostimulant (Sujeeth et al., 2022). Seaweeds contain many constituents, such as sterol, nitrogen-containing compounds, and micro- and macro-nutrients, which show growth-promoting activities (Khan et al., 2009; Craigie, 2011). In the cosmetic and pharmaceutical industries, many bioactive seaweed compounds are identified and used, but less is known about their plant growth-promoting activities. In addition, the focus is now on the use of marine algae in agriculture and their biostimulating activities (Mukherjee and Patel, 2020; Seiber et al., 2014; Ziosi et al., 2012; Ertani et al., 2013; Gunupuru et al., 2019).
Seaweeds can be used for soil application and foliar application in standing crops. Polysaccharides present in seaweed-based products help with water retention, gel formation, and soil aeration. Soil remediation occurs through the exchange and fixation of cations with polyanionic compounds of seaweeds. In suppressive soil, plant growth-promoting activity and antagonistic effect against plant pathogens are stated by soil microflora. Hormone and hormone-like compounds such as gibberellins, auxins, cytokinin, abscisic acid, sterols, and polyamines were reported by immunoassay and bioassay in seaweed extracts (Craigie, 2011). The hormonal effects of the application of seaweed Ascophyllum nodosum in plant tissue are also reported through gene expression studies (Wally et al., 2013). Extract from A. nodosum increases the tolerance to salinity stress and plant growth of Amaranthus tricolor plants under greenhouse conditions (Aziz et al., 2011). The 5% concentration of seaweed liquid extract (Sargassum wightii) was found to be effective in increasing biomass; root and shoot growth; the number of leaves, flowers, and fruits; and finally the yield of brinjal (Divya et al., 2015). Studies have shown that carrot growth, soil microbial communities, and soil respiration were increased after treatment with Ascophyllum extract powder (SAEP) (Alam et al., 2014). Foliar spray with 0.2% A. nodosum extract was found to enhance plant height and fruit yield by 10% and 51%, respectively, as compared to the control. In contrast to reference plants, higher concentrations (0.5%) of A. nodosum extract showed a greater improvement in plant height (37%) and fruit yield (63%). In greenhouse cultivation, the plants used for A. nodosum extract application had greater root structures and improved mineral quantities in the shoots. With an enhancement in quality characteristics such as total soluble solids, ascorbic acid level, size, color, and firmness, tomato fruit yield improvement was noted from treated plants with Ascophyllum seaweed extract in tropical environmental conditions (Ali et al., 2016a).
Plant pathogens are classified as biotrophs, necrotrophs, or hemi-biotrophs (Oliver, 2024). All types of plants have evolved with some resistance mechanisms or immunity to defend against the infection (Pieterse et al., 2009). The defense systems of plants are activated by the recognition of microbial components like flagellin, lipo-polysaccharides, and fungal chitin (Boller and Felix, 2009). The plant defense mechanism is activated by salicylic acid, jasmonic acid, and/or ethylene (Liu et al., 2010). Studies have shown that auxins, abscisic acid, cytokinins, gibberellins, and brassinosteroids are also involved in plant defense response (Pieterse et al., 2009). Seaweed extract can induce cytokinin inside the plants, which enhances the resistance properties against different diseases (Naseem et al., 2014). Some important brown seaweeds contain polysaccharides including laminarin and sulfated fucans, which are mainly water-soluble and act as inducers of plant systemic resistant mechanism. Mayer (2006) showed that seaweed extract can activate the peroxidase (POX) and polyphenol oxidase (PPO), which help in catalyzing the synthesis of lignin and phenolics during pathogen attack. The jasmonic acid/salicylic acid (JA/SA) signaling process helps in induced systemic resistance (ISR) activation in response to various pathogens. Seaweed and its extract help to activate JA/SA signaling, which mainly induces the ISR response against various plant phytopathogens (Figure 1). Seaweed is a good source of different bioactive components with the reported antimicrobial activity (Mukherjee and Patel, 2020).
Figure 1. Seaweed extract used as an elicitor to enhance plant defense mechanisms. The figure illustrates how seaweed-derived biostimulants, which are rich in carbohydrates, proteins, lipids, minerals, phytohormone regulators, and other compounds, enhance plant stress tolerance by activating various defense mechanisms. These stimulants bind to receptor sites on the plant cell membrane, triggering an oxidative burst, calcium ion uptake, and pH changes, which initiate early defense signaling pathways. This leads to the activation of defense genes through transcription factors like WRKYs, stimulating defense pathways such as MAPK cascades and producing key molecules involved in biotic stress resistance, including ethylene (Ets), salicylic acid (SA), and jasmonic acid (JA). As a result, defensive proteins such as defensins and pathogenesis-related (PR) proteins are activated, along with key antioxidant enzymes like phenylalanine ammonia-lyase (PAL), peroxidase (PO), polyphenol oxidase (PPO), and glutathione reductase (GR). Additionally, seaweed stimulants activate abiotic stress response pathways, enhancing the activity of phytohormones like auxins (Aux), gibberellins (GA), cytokinins (CK), and abscisic acid (ABA), as well as antioxidant enzymes such as catalase (CAT), superoxide dismutase (SOD), and peroxidase (POD), which help mitigate oxidative stress. This includes the regulation of osmoprotectants like proline and ion pumps that maintain homeostasis under stressful conditions. Furthermore, seaweed stimulants enhance carbohydrate and lipid metabolism, nutrient uptake, and photosynthesis, which provide the energy and resources required for maintaining plant health and resilience, ultimately improving crop tolerance to both biotic and abiotic stresses, making seaweed biostimulants valuable tools for sustainable agriculture.
Multiple studies have shown that plant defense mechanisms triggered during viral infection depend on gene regulation associated with SA/ET-dependent signaling pathways (Ghannam et al., 2013). During viral infection, defense genes such as PR1a, PR2, PR5, PR3, and Def1.2 were activated in plant leaves. Another experiment demonstrated that the mycelial growth of Colletotrichum gloeosporioides was inhibited by the application of κ-carrageenan. Similarly, the administration of κ-carrageenan to Capsicum annuum activated peroxidase (POD) enzymatic activity and regulated genes involved in SA/JA pathways, confirming a defense response against bacterial infection. λ-Carrageenan was identified as the most potent elicitor due to its high sulfur content, inducing systemic resistance against Phytophthora parasitica var. nicotianae in tobacco cells (Mercier et al., 2001). This induced resistance in tobacco cells was linked to the increased expression of sesquiterpene cyclase, a key enzyme in phytoalexin capsidiol synthesis pathways, as well as functional chitinase encoded by PR3 genes. Additionally, λ-carrageenan treatment led to an upregulation of cellular SA and transcripts of lipoxygenase (LOX) and ACC oxidase (ACO), both of which are involved in JA and ET biosynthesis (Mercier et al., 2001). In another study, λ-carrageenan was found to trigger a JA-dependent defense response in Arabidopsis thaliana against Sclerotinia sclerotiorum by inducing the expression of JA-responsive defense-related genes such as AOS, PDF1.2, and PR3 (Brederode et al., 1991).
Laminarins are regarded as standard substrates for β-1,3-glucanases, but their chemical sulfation renders them resistant to degradation by these enzymes (Meénard et al., 2004). The infiltration of PS3 into the leaves of transgenic PR1-β-glucuronidase (GUS) tobacco plants induced GUS expression, further supporting the activation of the SA-dependent signaling pathway (Ménard et al., 2005). However, PS3 treatment of tobacco cells did not induce the expression of acidic PR1 proteins, typically regarded as a hallmark of SAR activation in plant cells (Ménard et al., 2005). Ulvans and methyl jasmonate (MeJA) were found to elicit similar responses in Medicago truncatula (Jaulneau et al., 2010). Microarray analysis revealed a 40% overlap in gene expression in plants treated with MeJA and ulvans. These genes were associated with JA-dependent defense responses, including lipoxygenase, hydroxyproline-rich glycoproteins, proline-rich proteins, cysteine-rich antifungal proteins (e.g., defensin), and wound-induced proteins (Jaulneau et al., 2010).
Brown alga S. wightii has been reported to have antagonistic activity against both Pseudomonas syringae and Xanthomonas oryzae pv. The green alga (Halimeda tuna) extract works against different types of pathogenic fungi (Aspergillus niger, Aspergillus flavus, Penicillium sp., etc.) (Indira et al., 2013; Raj et al., 2018).
There has been a surge in seaweed application as a soil amendment of organic origin lately because of the growing apprehensions about the pernicious repercussions involved with the use of chemical synthetic pesticides (Mazzola, 2004; Sultana et al., 2012). A high fiber composition of seaweeds functions as a conditioner of soils and aids in water retention, while the nutrient content behaves as fertilizer and provides trace elements to the soil. As explained earlier, they have biocontrol properties and are composed of many organics and plant growth regulators like gibberellins, auxins, ethylene, and betaine precursors that affect the growth and development of plants. The seaweed extracts can impart embellished resistance in plants to diseases and pests and can affect the growth of plants, yield, and quality of plant produce (Mat-Atko, 1992). Seaweeds regulate the metabolic pathways of host plant cells by inducing the synthesis of antioxidants, which in turn regulates the growth and resistance of plants (Zhang and Schmidt, 2000). The administration of extracts obtained from different seaweeds leads to a decreased infestation of nematodes in crop plants because of betaine content (Wu et al., 1997). They contain 1-aminocyclopropane-1-carboxylic acid (ACC) and polyphenols that have antimicrobial and antioxidant activities, respectively (Tariq et al., 2011). The application of Solieria robusta, a red seaweed, as an amendment to soil proved to be more efficient in suppressing Fusarium solani than the fungicide Topsin-M (Sultana et al., 2012). Similarly, the use of seaweeds as soil amendment provided convincing root-knot nematode control in addition to promoting plant development under field and plot experimentations. In agricultural fields, there was a reduced incidence of root-knot nematode on the eggplant crop treated with seaweeds, which is otherwise susceptible (Baloch et al., 2013). The concentrated liquid extract obtained from Ecklonia maxima, a brown alga, provided a significant reduction of root-knot infestation and embellished growth in tomato plants. A 0.2% A. nodosum extract significantly reduced the disease severity of two fungal plant pathogens, namely, Botrytis cinerea and Alternaria radicina, in carrot plants under greenhouse conditions at 10 and 25 days after inoculation. The application extract had better efficiency than the salicylic acid application at the 100-µM concentration of the plants. Significant higher activity of various defense-related enzymes, like chitinase, β-1,3-glucanase, phenylalanine ammonia-lyase, peroxidase, and polyphenol oxidase, was found in the seaweed extract treated plants in comparison to the salicylic acid-treated plants. The same study also observed that crop plants treated with seaweed extract had higher levels of transcripts of non-expressing pathogenesis-related protein (NPR-1), lipid transfer protein (Ltp), chitinase, phenylalanine ammonia-lyase, pathogenesis-related protein 1 (PR-1), pathogenesis-related protein 5 (PR-5), and chalcone synthase genes than control plants. All these results suggest that the treatment of seaweed extract onto plants triggers defense responses possibly by inducing the defense genes or defense proteins (Jayaraj et al., 2008). Maxicrop (a commercial seaweed extract) application led to the suppression of powdery mildew spread in turnips and gray mold in strawberries in addition to enhanced yields in both crops (Stephenson, 1966).
Laminarin is present in the liquid formulation obtained from A. nodosum (GYFA 17, Goëmar), which caused an upregulated synthesis of phenylalanine ammonia-lyase, salicylic acid, caffeic acid O-methyl transferase, and lipoxygenase in plants of tobacco crop (Patier et al., 1993; Potin et al., 1999; Klarzynski et al., 2000) and molecules of antifungal characteristics in plants of alfalfa crop (Kobayashi et al., 1993). There was also a considerable development in resistance of cotton seedlings to Xanthomonas campestris when the seeds were primed with Dravya (a commercial aqueous formulation of the extract obtained from S. wightii) at the rate of 1:500 for 12 hours before germination (Raghavendra et al., 2007). When the seaweed extract was applied as a foliar spray, the bacterial blight incidence was also decreased in cotton seedlings, and a maximum reduction of 74% was achieved when the seed priming was coupled with the foliar treatment. The systemic resistance of treated plants was due to the higher level of peroxidase and total phenolic content. The application of seaweed extract had an additional advantage over streptomycin application, as it also caused promotion in plant vigor, increase in stem girth, higher yield of bolls, and no adverse effect on the environment. The molecules of antibacterial characteristics in the chloroform extract of brown and red seaweeds are the alcohols and polyunsaturated esters (Vallinayagam et al., 2009). The treatment of an extract obtained from A. nodosum and humic acid to the creeping bent-grass was shown to have increased superoxide dismutase (SOD) level and a reduced incidence of Sclerotinia homoeocarpa (dollar spot disease) (Zhang et al., 2003). The spraying of soluble extract obtained from A. nodosum to the spinach crop also led to an increased level of phenolics, flavonoids, and antioxidants in the leaves (Fan et al., 2011).
Enhanced tolerance to salt and freezing stresses has also been observed with the foliar application of various seaweed extracts (Mancuso et al., 2006). The application of Seasol (commercial formulation of Ascophyllum spp. extract) was proved to provide a better freezing tolerance in grape plants. A 0.8% solution of the same also showed a reduced osmotic potential in the leaves of grape plants in comparison to the control, which is the main mechanism behind osmotic tolerance (Wilson, 2001). There are many other reports that back the potential of seaweed-derived substances being able to impart abiotic stress tolerance in treated plants and embellish their performance. Until now, the chemistry of bioactive molecules obtained from seaweed and their physiological mechanisms of imparting tolerance in plants is very much unknown. However, there are numerous reports that emphasize the role of cytokinin in providing beneficial anti-stress effects. The combined treatment of humic acid and seaweed extract to the drought-stressed plants led to an increased root mass by 21%–68%, zeatin riboside (endogenous) by 38%, and tocopherol in leaves by 110%. The enzyme-linked immunosorbent assay (ELISA) test of extract obtained from A. nodosum showed the amount of cytokinins of approximately 66 µg g−1 in the form of zeatin riboside, and the use of the same after ashing reduced its effectiveness, which suggested the organic nature of bioactive compounds present in the extract (Zhang and Ervin, 2004). The phytohormone cytokinins are known to alleviate free radicals produced due to various stresses by scavenging directly and by preventing the formation of reactive oxygen species (ROS) through oxidation inhibition of xanthine (Mckersie et al., 1994; Fike et al., 2001). It was hypothesized that the heat tolerance in creeping bentgrass with the application of seaweed extract was mainly due to cytokinin presence (Ervin et al., 2004; Zhang and Ervin, 2008). Kelpak is also proposed for imparting tolerance to abiotic stress in plants by increasing potassium ion (K+) levels.
The infestation level of nematodes reduces when plants are treated with seaweed extracts (Featonby-Smith and Van Staden, 1983; Wu et al., 1997). The amendment of soil with a commercial liquid formulation of E. maxima (sea-bamboo) extract led to a reduced incidence of root-knot nematode in tomato plants (Featonby-Smith and Van Staden, 1983; Crouch and Van Staden, 1993).
The marine algal organisms can be a major component of plant defense elicitor molecules (Cluzet et al., 2004). Naturally, plants are known to protect themselves from the phytopathogen by recognizing the signal molecules called elicitors, which are vastly varied molecules from oligosaccharides, polysaccharides, proteins, peptides, and even lipids, which are the composing elements of phytopathogenic organisms’ cell wall (Boller, 1995; Côté et al., 1998). There are a variety of polysaccharides that can be found in the seaweed extracts, out of which many serve as elicitor molecules, thereby imparting enhanced defense responses to the phytopathogens (Kloareg and Quatrano, 1988). The cell wall of Rhodophyta (red algae) species mainly contains carrageenans and agars; the Phaeophyta (brown algae) algal extracts contain laminarins, sulfated fucans, alginates, and other composite mucilages. In contrast, the extracts from green algae contain mucilages that are made up of uronic acid, xylose, and rhamnose (Cluzet et al., 2004). In alfalfa and tobacco plants, laminarin and sulfated fucans, which are present in the extract from brown algae, are known to elicit multiple defense responses (Kobayashi et al., 1993; Klarzynski et al., 2000, 2003). The extract of Rhodophyta (red algae) contains a family of linear sulfated galactans, altogether known as carrageenans, which are also effectively capable of eliciting various defense mechanisms in tobacco plants (Mercier et al., 2001). The foliar application of A. nodosum extracts is known to reduce the incidence of Plasmopara viticola in grape and Phytophthora capsici infection in chili (Lizzi et al., 1998). The application of seaweed extract in liquid form to the soil accelerated the population and activities of antagonistic microorganisms of Pythium ultimum in cabbage (Dixon and Walsh, 2004). In an experiment, it was shown that the extract from Ulva spp. enhanced resistance to Colletotrichum trifolii (anthracnose disease) in M. truncatula (alfalfa), and there was no necrotic lesion elicitation. It also increased the level of resistance in alfalfa plants when the treatment was conducted before the pathogen challenge. The genomic analysis showed an upregulated expression of 152 genes in the plants treated with algal extract; most of them were related to defense pathways (Cluzet et al., 2004). The extract from Ulva spp. also causes elicitation PR-10 gene expression, which is a part of the defense mechanism against the phytopathogenic attack (Van Loon et al., 2006).
Bacterial quorum sensing is a communication mechanism employed by bacterial populations to coordinate and regulate gene expression based on cell density, influencing various physiological activities and functions (Brelles-Marino and Bedmar, 2001; Winzer and Williams, 2001; Dong and Zhang, 2005). This process is primarily mediated by acylated-homoserine lactone (acyl-HSL), a small signaling molecule. Quorum sensing plays a crucial role in determining the virulence of phytopathogenic bacteria, making it a target for controlling bacterial pathogenicity. Delisea pulchra, a red alga found in marine environments, produces halogenated enones and furanones that are structurally similar to acyl-HSL. These compounds inhibit quorum sensing by binding to the LuxR receptor, which normally interacts with acyl-HSL, thus preventing signal transduction. Naturally occurring furanones are known to disrupt quorum sensing in several marine bacterial species, including Vibrio fischeri, Serratia liquefaciens, and Vibrio harveyi (Rasmussen et al., 2000; Manefield et al., 2002).
Concerning the surging demand for organic cultivation owing to the disastrous effects of synthetic chemicals and fertilizers, the sector of seaweed fertilizer is growing rapidly around the globe. Additionally, seaweeds are also known as naturally occurring promoters of plant growth and resistance to plant diseases (Table 1). Seaweeds have always been the entities that are affluent in providing a very wide variety of natural compounds that are mainly secondary metabolites that they produce. These secondary metabolites are composed of lipids, steroids, terpenes, amino acid derivatives, phlorotannins, aromatic compounds, acetogenins, and various other polymeric substances (Zbakh et al., 2012; Thinakaran and Sivakumar, 2013; Paul, 2014; Pérez et al., 2016). Although there has been an insufficient amount of research evidence about the activity of green algal products against microbes; numerous experiments have proved the antimicrobial activities of extracts obtained from seaweeds. Reports have suggested that this antimicrobial characteristic of seaweeds is mainly because of terpenes and their derivatives (Paulert et al., 2009; Peres et al., 2012). There are many pieces of evidence that establish different algal extracts as an antifungal substance to various fungal phytopathogens (Coşoveanu et al., 2010; Thinakaran and Sivakumar, 2013). In an experiment, it was observed that different organic extracts obtained from five species of seaweeds of the Phaeophyta group, viz., Colpomenia sinuosa, Dictyota dichotoma, Cystoseira barbata, Dictyopteris membranacea, and Sargassum vulgare, were antagonistic to eight fungal species (Alternaria alternata, Fusarium oxysporum, A. flavus, A. niger, Cladosporium cladosporioides, Aspergillus ochraceus, Epicoccum nigrum, and Penicillium citrinum) (Khallil and Daghman, 2015). Also, seaweed’s potential as a biocide came into light when extracts from Ulva fasciata and Ulva lactuca proved to be effective against the adults and nymphs of Dysdercus cingulatus, a pest of cotton (Asha et al., 2012). There are various species of brown seaweed that have also been proven to be efficient in the management of phytopathogens (Peres et al., 2012). Various organic extracts obtained from discrete species of marine algae like Melanothamnus afaqhusainii, Padina tetrastromatica, and Sargassum tenerrimum possessed very efficient nematicidal activity when were tested against Meloidogyne javanica (root-knot nematode), showing approximately 99% larval mortality and 96% less egg hatching (Khan et al., 2015). There are certain seaweeds that have less efficient antifungal activity. Still, when the same was used in consortium with some plant growth-promoting bacteria, their efficiency as an antifungal agent increased very significantly. This phenomenon was observed when some seaweed species were studied in association with Paecilomyces lilacinus and Bradyrhizobium japonicum for sunflower root protection (Ara et al., 1996). Therefore, it can be very rightly said that the application of seaweeds as a biocontrol agent could become a very advanced research domain for biotechnological and phytopathological studies (Abdel-Raouf et al., 2012). Lately, it has been confirmed that the compounds obtained from green, brown, and red algal species have potential antimicrobial activities. The cumulative use of the green, brown, and red seaweeds was proven to be immensely effective in controlling the phytopathogenic fungi of okra seedlings (Sultana et al., 2005). The application of extracts obtained from seaweeds as foliar sprays inhibited the incidence of fruit rot disease, enhanced the production of strawberry crops, and additionally efficiently reduced the development of gray mold (Washington et al., 1999). The extract obtained from several seaweeds in ethanol and water solution proved to be a significantly effective nematicide against M. javanica. The efficiency screening of three seaweeds, viz., H. tuna, M. afaqhusainii, and Spatoglossum variabile, against the nematicide Carbofuran and the fungicide Topsin-M, was carried out under field as well as greenhouse conditions. The results showed that seaweeds caused similar or more suppression of the root pathogens in tomato and sunflower plants. They reduced the number of galls on the roots and resulted in lesser nematode penetration. In the tomato plants under field application, it was observed that the mixed application of Carbofuran with S. variabile had a maximum reduction in penetration of roots by nematodes and increased the fresh weight of shoot, root length, and yield (Sultana et al., 2012). In another study, it was shown that extracts and powder obtained from red alga Gracilaria confervoides were assessed for their biocontrol activity against three soil-borne phytopathogenic fungi of cucumber, viz., Fusarium solani, Macrophomina phaseolina, and Rhizoctonia solani. The maximum reduction (100%) of mycelial growth was observed for Rhizoctonia solani under chloroform extract, which was followed by 50% ethyl acetate extraction (Soliman et al., 2018).
The use of plant biostimulants in place of synthetic pesticides and fertilizers provides better and alternative options as traditional agrochemical input, and in many cases, plant biostimulants are also found to decrease the rate of application by increasing the efficacy of synthetic pesticides and fertilizers (Calvo et al., 2014; Van Oosten et al., 2017; Yakhin et al., 2017). The positive impact of macroalga-based products on salinity and other abiotic stresses that cause severe losses to crop yields has been reported a number of times (Schroeder et al., 2001; Semenov and Shewry, 2011; Graves, 1995) (Table 2).
There is a great concern about growing new products with different bioactive compounds that help to improve plant health. The benefits involve increased plant growth, such as root structure, flowering and leaf growth, fruit set, enhanced crop yield, and greater capacity to withstand plant disease and stresses. Application of aqueous extract of Kappaphycus alvarezii in wheat (Triticum durum) plants stimulated plant growth and development, reducing damage to membrane permeability, reducing electrolyte leakage, reducing ROS, and increasing the activity of superoxide dismutase enzymes, and osmoprotectants such as proline, sugars, and phytohormones were also regulated by the applied extract (Patel et al., 2018). Red alga K. alvarezii (Rhodophyta) is one of the five most effective cultivated species in the world according to the WHO and Food and Agriculture Organization (FAO) (2022). This species contains 3.3% protein, 3.3% lipids, 3.0% insoluble aromatic compounds, 15.6% ash, and 12.4% sulfates, in addition to carbohydrates on a dry mass basis (Solorzano-Chavez et al., 2019) and known to improve photosynthetic efficiency transport and adaptive response to the stress in rice. Root application of K. alvarezii extract with rice plants enhanced N and K uptake efficiency, leading to increased amino N levels in plants. In contrast, enhanced soil microbial quantity, soil composition, and water-holding capability were also increased by the use of seaweed extract. Different types of plant growth regulators, i.e., ethylene, abscisic acid, gibberellins, auxins, and cytokinins, have been found in seaweed extract (Khan et al., 2009; Kurepin et al., 2014; Stephen et al., 1985). Response of seaweed extract found equivalent to specific plant hormones was characterized by plant callus-inducing assay at an early stage of the work (Craigie, 2011). The existence of cytokinin within seaweed had first been recorded using Seasol™ liquid seaweed extract derived from Durvillaea potatorum, commercially sold in Australia (Tay et al., 1985). In the South African kelp extract from Ecklonia maxima, brassinosteroids were identified along with gibberellins and abscisic acid (Stirk et al., 2014). Osmoprotectants had been found to play an important role in plant stress conditions and were reported to accumulate in increased stress tolerance. Betaines are a quaternary ammonium molecule isolated and characterized from seaweed extract and found to work against major osmotic changes. Several genera of brown algae such as Ascophyllum, Fucus, and Laminaria have been reported to yield betaine. There is growing evidence from in vivo and in vitro plant physiology, genetics, and biochemistry analyses that strongly indicate the role of glycine betaine against abiotic stresses in plants. It protects sub-cellular structures and plays a role in mediating osmotic adjustment under stressed conditions. There are several crops, like rice, which in stressful conditions do not produce or accumulate glycine betaine. Therefore, exogenous application and activation of biosynthesis through genetic engineering are a good strategy to enhance stress tolerance (Wani et al., 2013; Karabudak et al., 2014; Calvo et al., 2014; Craigie, 2011; Blunden et al., 1986a; Blunden et al., 1986b). Diverse polysaccharides, alginates, and some sulfates have been characterized and reported to play roles in root growth and development, both directly and indirectly, and induce plant defense responses (Xu et al., 2003; Khan et al., 2012; González et al., 2013; Subramanian et al., 2011; Vera et al., 2011). Trace components, lipid-based minerals such as sterols, have been documented to enhance nutrition and play a significant role in plant growth (Mancuso et al., 2006; Rayirath et al., 2009).
Soil salinity is a global issue affecting the production of agriculture in over 800 million hectares of land. Salinity stress at a mild level affects cell–water relations, inhibits cell expansion, reduces growth rate, and, consequently, causes physiological drought in plants (Hussain et al., 2020). Ionic stress is caused by the long-term result of high salinity by disrupting intracellular ion homeostasis, resulting in the attenuation of metabolic activity, membrane dysfunction, plant growth inhibition, and improvement in cell death (Yadav et al., 2012; Hasegawa, 2013; Shukla et al., 2012, 2015). Osmotic as well as ionic stresses induced by salinity stress limit the growth and productivity of plants (Agarwal et al., 2013). Plants have evolved strategies to respond at genetic, biochemical, and physiological levels to salinity stress (Agarwal et al., 2013; Hasegawa, 2013; Ferchichi et al., 2018). Improved tolerance and increase of plant growth parameters such as number of leaves, root and stem length, fresh and dry leaf, and root and stem weight have been observed after spraying plants with seaweed extract against salinity stress (Aziz et al., 2011). Betaine and betaine-like compounds found in the extract are responsible for improving tolerance to abiotic stresses such as drought and salinity (Wang et al., 2003). Betaines protect plant cells through cytoplasmic osmolytes from drought, high temperature, salinity, and osmotic stress (Blunden, 1977; Blunden et al., 1996). Macroalgal treatment for abiotic stress management was found effective during research in North America especially against cold, low soil moisture, salinity, and heat (Zhang and Schmidt, 2000; Nabati et al., 1994). Salinity stress in avocados is alleviated by improving Ca+ and K+ nutrient uptake after the application of A. nodosum extract (Bonomelli et al., 2018). Further, A. nodosum extract (ANE) maintains the level of K+/Na+ content and enhances turf grass growth under salt stress (Elansary et al., 2017). Seaweed extract significantly improved the above- and below-ground part of turf grass while increasing survival under salinity stress (Nabati et al., 1994).
Temperature stress limits the production and productivity of crops by adversely affecting the development and growth of plants (Miura and Furumoto, 2013). A. thaliana also showed enhanced tolerance under freezing situations after the application of a lipophilic fraction of A. nodosum extract (Rayirath et al., 2009). Bioactive compounds in seaweed extract have been reported to enhance the freezing stress tolerance of plants after spray applications (Mancuso et al., 2006). In grapes, freezing temperature tolerance was improved after 9 days of treatment with seaweed extract with a decrease in the osmotic ability of leaves (Wilson, 2001). Priming the seeds with commercial A. nodosum extract was found to enhance the germination of seed and increase tolerance in lettuce and creeping bentgrass during high-temperature stress, and the author documented this effect because of the “cytokinin-like” substance in extract including an increase in K+ uptake (Möeller and Smith, 1998; Ervin et al., 2004; Zhang and Ervin, 2008).
Drought negatively affects the physiology of plants, thereby the productivity of the crop, by interfering with water and nutrient relations and photosynthesis (Fahad et al., 2017; Shukla et al., 2018). Drought stress increases the temperature of the leaf by a reduction in transpiration cooling (Yordanov et al., 2000). A. nodosum commercial extract Acadian® supports soybean plants to survive in drought conditions by preserving leaf temperature and turgor with the regulation of stress-responsive genes (Martynenko et al., 2016; Shukla et al., 2018). Under drought stress, Acadian® extract showed enhanced stomatal conductivity, which is a main physiological factor of plants affected during drought stress (Shukla et al., 2018; Manavalan et al., 2009). A. nodosum bioactive compound lowers the harmful effect of drought stress through intervention with cellular, molecular, and physiological responses. They also regulate several genes, resulting in the improvement of the antioxidant system, osmolyte accumulation, and an increase in gaseous exchange with stomatal regulation. Many past studies have strongly suggested the role of ANEs in the management of drought stress in Glycine max, Spiraea nipponica, Phaseolus vulgaris, A. thaliana, Citrus sinensis, Lycopersicon esculentum, Spinacea oleracea, and Pittosporum eugenioides (Spann and Little, 2011; Xu and Leskovar, 2015; Elansary et al., 2016; Santaniello et al., 2017; Carvalho et al., 2018; Goñi et al., 2018; Shukla et al., 2018). In the greenhouse, vegetable production with seaweed commercial extract of A. nodosum was found to reduce wilting caused by drought stress while improving water use efficiency and leaf water content as compared to untreated control plants (Neily et al., 2010; Neily et al., 2008). Ascophyllum commercial extract root applications in almonds at 2-week intervals improved the water potential of plants (Neily et al., 2010).
Based on the findings, it is evident that seaweed extracts not only promote plant growth but also activate plant defense mechanisms against both biotic and abiotic stresses. The excessive use of synthetic chemicals and pesticides has led to significant global challenges, including the development of resistance to pathogens and pests, the contamination of food with chemical residues, increased production costs, and severe environmental and human health impacts. Algal extracts, with their phytostimulating and phytoeliciting properties, offer a promising alternative as biostimulants. The potential of various marine algal species as plant biostimulants has been well-documented, and seaweed extracts can be effectively utilized alongside or as substitutes for synthetic fertilizers, thereby reducing chemical input in agriculture—a crucial aspect of sustainable farming practices. Given the current global context and the growing public interest, especially in developing nations, in reducing the use of toxic products like pesticides and fertilizers, environmentally friendly, non-chemical alternatives for agricultural crop production are becoming increasingly vital. Future research should focus on unraveling the molecular mechanisms behind the interaction of specific seaweed compounds with plant receptors to better understand the signaling pathways activated by these biostimulants. Additionally, comparative studies on different seaweed species and their specific bioactive components will be essential in determining the most potent combinations for stress tolerance and growth promotion in various crops. Understanding the optimal application methods, dosage, and timing for different environmental conditions and crop types is also critical for maximizing the benefits of seaweed-based biostimulants. Exploring the synergistic effects of seaweed extracts with other organic biostimulants, microorganisms, or reduced synthetic inputs could further enhance their effectiveness in integrated pest and stress management systems. Furthermore, large-scale field trials across diverse agroecological zones are needed to assess the long-term impact of seaweed extracts on soil health, crop productivity, and ecosystem services. The development of innovative extraction technologies and formulation strategies that maintain the stability and bioactivity of seaweed compounds is another promising area for enhancing their commercial viability and adoption in sustainable agriculture.
GK: Conceptualization, Investigation, Writing – original draft, Writing – review & editing. SN: Writing – original draft, Writing – review & editing. SS: Writing – original draft, Writing – review & editing. SK: Writing – original draft, Writing – review & editing. DS: Writing – original draft, Writing – review & editing. BS: Writing – original draft, Writing – review & editing. AM: Conceptualization, Investigation, Supervision, Writing – original draft, Writing – review & editing.
The author(s) declare financial support was received for the research, authorship, and/or publication of this article. The author AM thanks the PMRF fellowship scheme for financial support for research studies.
The authors declare that the research was conducted in the absence of any commercial or financial relationships that could be construed as a potential conflict of interest.
All claims expressed in this article are solely those of the authors and do not necessarily represent those of their affiliated organizations, or those of the publisher, the editors and the reviewers. Any product that may be evaluated in this article, or claim that may be made by its manufacturer, is not guaranteed or endorsed by the publisher.
Abdel-Raouf N., Al-Homaidan A. A., Ibraheem I. B. M. (2012). Agricultural importance of algae. J. Biotechnol. 11, 11648–11658.
Abkhoo J., Sabbagh S. K. (2016). Control of Phytophthora melonis damping-off, induction of defense responses, and gene expression of cucumber treated with commercial extract from Ascophyllum nodosum. J. App. Phycol. 28, 1333–1342. doi: 10.1007/s10811015-0693-3
Agarwal P., Patel K., Das A. K., Ghosh A., Agarwal P. K. (2016). Insights into the role of seaweed Kappaphycus alvarezii sap towards phytohormone signalling and regulating defence responsive genes in Lycopersicon esculentum. J. App. Phycol. 28, 25292537. doi: 10.1007/s10811-015-0784-1
Agarwal P. K., Shukla P. S., Gupta K., Jha B. (2013). Bioengineering for salinity tolerance in plants: state of the art. Mol. Biotechnol. 54, 102–123.
Aguado A., Pastrana A. M., De Los Santos B., Romero F., Sánchez M. C., Capote N. (2012). Efficiency of natural products in the control of Colletotrichum acutatum monitored by real-time PCR. In. VII Int. Strawberry Symposium 1049, 329–334. doi: 10.17660/ActaHortic.2014.1049.44
Alam M. Z., Braun G., Norrie J., Mark Hodges D. (2014). Ascophyllum extract application can promote plant growth and root yield in carrot associated with increased root-zone soil microbial activity. Can. J. Plant Sci. 94, 337–348. doi: 10.4141/cjps2013-135
Ali N., Farrell A., Ramsubhag A., Jayaraman J. (2016a). The effect of Ascophyllum nodosum extract on the growth, yield and fruit quality of tomato grown under tropical conditions. J. App. Phycol. 28, 1353–1362. doi: 10.1007/s10811-015-0608-3
Ali N., Ramkissoon A., Ramsubhag A., Jayaraj J. (2016b). Ascophyllum extract application causes reduction of disease levels in field tomatoes grown in a tropical environment. Crop Prot. 83, 67–75. doi: 10.1016/j.cropro.2016.01.016
Ara J., Ehteshamul-Haque S., Sultana V., Qasim R., Ghaffar A. (1996). Effect of Sargassum seaweed and microbial antagonists in the control of root rot disease of sunflower. Pak. J Bot., 219–223.
Arunkumar K., Selvapalam N., Rengasamy R. (2005). The antibacterial compound sulphoglycerolipid 1-0 palmitoyl-3-0 (6′-sulpho-α-quinovopyranosyl)-glycerol from Sargassum wightii Greville (Phaeophyceae). doi: 10.1515/BOT.2005.058
Asha A., Rathi J. M., Raja D. P., Sahayaraj K. (2012). Biocidal activity of two marine green algal extracts against third instar nymph of Dysdercus cingulatus (Fab.) (Hemiptera: Pyrrhocoridae). J. Biopest 5, 129–134.
Aziz N. A., Mahgoub M. H., Siam H. S. (2011). Growth, flowering and chemical constituents performance of Amaranthus tricolor plants as influenced by seaweed (Ascophyllum nodosum) extract application under salt stress conditions. J. Appl. Sci. Res. 7, 1472–1484.
Baloch G. N., Tariq S., Ehteshamul-Haque S., Athar M., Sultana V., Ara J. (2013). Management of root diseases of eggplant and watermelon with the application of asafoetida and seaweeds. J. Appl. Bot. Food Qual. 86, 138–142. doi: 10.5073/JABFQ.2013.086.019
Berens M. L., Wolinska K. W., Spaepen S., Ziegler J., Nobori T., Nair A., et al. (2019). Balancing trade-offs between biotic and abiotic stress responses through leaf age-dependent variation in stress hormone cross-talk. PNAS 116, 2364–2373. doi: 10.1073/pnas.18172331
Blunden G. (1977). “Cytokinin activity of seaweed extracts,” in Marine natural products chemistry (Springer US, Boston, MA), 337–344.
Blunden G., Cripps A. L., Gordon S. M., Mason T. G., Turner C. H. (1986a). The characterisation and quantitative estimation of betaines in commercial seaweed extracts. doi: 10.1515/botm.1986.29.2.155
Blunden G., Gordon S. M., Crabb T. A., Roch O. G., Rowan M. G., Wood B. (1986b). NMR spectra of betaines from marine algae. Magnetic Res. Chem. 24, 965–971. doi: 10.1002/mrc.1260241108
Blunden G., Yang M. H., Yuan Z. X., Smith B. E., Patel A., Cegarra J. A., et al. (1996). Betaine distribution in the labiatae. Bioch. Syst. Ecol. 24, 71–81. doi: 10.1016/0305-1978(95)00098-4
Boller T. (1995). Chemoperception of microbial signals in plant cells. Ann. Rev. Plant Physiol. Plant Mol. Biol. 46, 189–214. doi: 10.1146/annurev.pp.46.060195.001201
Boller T., Felix G. (2009). A renaissance of elicitors: perception of microbe-associated molecular patterns and danger signals by pattern-recognition receptors. Ann. Rev. Plant Boil. 60, 379–406. doi: 10.1146/annurev.arplant.57.032905.105346
Bonomelli C., Celis V., Lombardi G., Mártiz J. (2018). Salt stress effects on avocado (Persea americana Mill.) plants with and without seaweed extract (Ascophyllum nodosum) application. Agronomy. 8, 64. doi: 10.3390/agronomy8050064
Bradáčová K., Weber N. F., Morad-Talab N., Asim M., Imran M., Weinmann M., et al. (2016). Micronutrients (Zn/Mn), seaweed extracts, and plant growth-promoting bacteria as cold-stress protectants in maize. Chem. Biol. Technol. Agriol. 3, 1–10. doi: 10.1186/s40538-016-0069-1
Brederode F. T., Linthorst H. J., Bol J. F. (1991). Differential induction of acquired resistance and PR gene expression in tobacco by virus infection, ethephon treatment, UV light and wounding. Plant Mol. Biol. 17, 1117–1125. doi: 10.1007/BF00028729
Brelles-Marino G., Bedmar E. J. (2001). Detection, purification and characterisation of quorum-sensing signal molecules in plant-associated bacteria. J. @ Biotechnol. 91, 197–209. doi: 10.1016/S0168-1656(01)00330-3
Calvo P., Nelson L., Kloepper J. W. (2014). Agricultural uses of plant biostimulants. Plant Soil 383, 3–41. doi: 10.1007/s11104-014-2131-8
Carvalho M. E. A., de Camargo P. R., Gaziola S. A., Azevedo R. A. (2018). Is seaweed extract an elicitor compound? Changing proline content in drought-stressed bean plants. Comunicata Scientiae 9, 292–297. doi: 10.14295/cs.v9i2.2134
Cluzet S., Torregrosa C., Jacquet C., Lafitte C., Fournier J., Mercier L., et al. (2004). Gene expression profiling and protection of Medicago truncatula against a fungal infection in response to an elicitor from green algae Ulva spp. Plant Cell Environ. 27, 917–928. doi: 10.1111/j.1365-3040.2004.01197.x
Coşoveanu A., Axine O., Iacomi B. (2010). Antifungal activity of macroalgae extracts. Sci. Papers UASVM Bucharest Ser. A 53, 442–447.
Côté F., Ham K. S., Hahn M. G., Bergmann C. W. (1998). Oligosaccharide elicitors in hostpathogen interactions: generation, perception, and signal transduction. Plant-Microbe Interact., 385–432. doi: 10.1007/978-1-4899-1707-2_13
Craigie J. S. (2011). Seaweed extract stimuli in plant science and agriculture. J. @ Appl. Phycol 23, 371–393. doi: 10.1007/s10811-010-9560-4
Crouch I. J., Van Staden J. (1993). Effect of seaweed concentrate from Ecklonia maxima (Osbeck) Papenfuss on Meloidogyne incognita infestation on tomato. J. Appl. Phycol. 5, 37–43. doi: 10.1007/BF02182420
Damalas C. A., Koutroubas S. D. (2016). Farmers’ exposure to pesticides: toxicity types and ways of prevention. Toxics. 4, 1. doi: 10.3390/toxics4010001
De Corato U., Salimbeni R., De Pretis A., Avella N., Patruno G. (2017). Antifungal activity of crude extracts from brown and red seaweeds by a supercritical carbon dioxide technique against fruit postharvest fungal diseases. Postharvest Biol. Technol. 131, 16–30. doi: 10.1016/j.postharvbio.2017.04.011
Divya K., Roja M. N., Padal S. B. (2015). Effect of seaweed liquid fertilizer of Sargassum wightii on germination, growth and productivity of brinjal. Int. J. Adv. Res. Sci. Eng. Technol. 2, 868–871.
Dixon G. R., Walsh U. F. (2004). Suppressing Pythium ultimum induced damping-off in cabbage seedlings by biostimulation with proprietary liquid seaweed extracts. Acta Hortic. 635, 103–106. doi: 10.17660/ActaHortic.2004.635.13
Dong Y. H., Zhang L. H. (2005). Quorum sensing and quorum-quenching enzymes. J. Microbiol. 43, 101–109.
Elad Y., Pertot I. (2014). Climate change impacts on plant pathogens and plant diseases. J. @ Crop Improvement 28, 99–139. doi: 10.1080/15427528.2014.865412
Elansary H. O., Skalicka-Woźniak K., King I. W. (2016). Enhancing stress growth traits as well as phytochemical and antioxidant contents of Spiraea and Pittosporum under seaweed extract treatments. Plant Physiol. Biochem. 105, 310–320. doi: 10.1016/j.plaphy.2016.05.024
Elansary H. O., Yessoufou K., Abdel-Hamid A. M., El-Esawi M. A., Ali H. M., Elshikh M. S. (2017). Seaweed extracts enhance salam turfgrass performance during prolonged irrigation intervals and saline shock. Front.Plant Sci. 8. doi: 10.3389/fpls.2017.00830
El Modafar C., Elgadda M., El Boutachfaiti R., Abouraicha E., Zehhar N., Petit E., et al. (2012). Induction of natural defence accompanied by salicylic acid-dependant systemic acquired resistance in tomato seedlings in response to bioelicitors isolated from green algae. Scientia Horticul. 138, 55–63. doi: 10.1016/j.scienta.2012.02.011
Ertani A., Schiavon M., Muscolo A., Nardi S. (2013). Alfalfa plant-derived biostimulant stimulate short-term growth of salt stressed Zea mays L. plants. Plant soil. 364, 145–158. doi: 10.1007/s11104-012-1335-z
Ervin E. H., Zhang X., Fike J. H. (2004). Ultraviolet-B radiation damage on Kentucky Bluegrass II: Hormone supplement effects. Hortic. Sci. 39, 1471–1474. doi: 10.21273/HORTSCI.39.6.1471
Fahad S., Bajwa A. A., Nazir U., Anjum S. A., Farooq A., Zohaib A., et al. (2017). Crop production under drought and heat stress: plant responses and management options. Front. Plant Sci I, 1147. doi: 10.3389/fpls.2017.01147
Fan D., Hodges D. M., Zhang J., Kirby C. W., Ji X., Locke S. J., et al. (2011). Commercial extract of the brown seaweed Ascophyllum nodosum enhances phenolic antioxidant content of spinach (Spinacia oleracea L.) which protects Caenorhabditis elegans against oxidative and thermal stress. Food Chem. 124, 195–202. doi: 10.1016/j.foodchem.2010.06.008
Featonby-Smith B. C., Van Staden J. (1983). The effect of seaweed concentrate and fertilizer on the growth of Beta vulgaris. Z. für Pflanzenphysiologie. 112, 155–162. doi: 10.1016/S0044-328X(83)80030-0
Ferchichi S., Hessini K., Dell’Aversana E., D’Amelia L., Woodrow P., Ciarmiello L. F., et al. (2018). Hordeum vulgare and hordeum maritimum respond to extended salinity stress displaying different temporal accumulation pattern of metabolites. Funct. Plant Biol. 45, 1096–1109.
Fike J. H., Allen V. G., Schmidt R. E., Zhang X., Fontenot J. P., Bagley C. P., et al. (2001). Tasco-Forage: I. Influence of a seaweed extract on antioxidant activity in tall fescue and in ruminants. J. Anim. Sci. 79, 1011–1021. doi: 10.2527/2001.7941011x
Ghannam A., Abbas A., Alek H., Al-Waari Z., Al-Ktaifani M. (2013). Enhancement of local plant immunity against tobacco mosaic virus infection after treatment with sulphated-carrageenan from red alga (Hypnea musciformis). Physiol. Mol. Plant Pathol. 84, 19–27. doi: 10.1016/j.pmpp.2013.07.001
Goñi O., Quille P., O'Connell S. (2018). Ascophyllum nodosum extract biostimulants and their role in enhancing tolerance to drought stress in tomato plants. Plant Physiol. Biochem. 126, 63–73. doi: 10.1016/j.plaphy.2018.02.024
González A., Castro J., Vera J., Moenne A. (2013). Seaweed oligosaccharides stimulate plant growth by enhancing carbon and nitrogen assimilation, basal metabolism, and cell division. J. Plant Growth Regul. 32, 443–448. doi: 10.1007/s00344-012-9309-1
Graves W. R. (1995). Stress and stress coping in cultivated plants: BD McKersie and YY Leshem. Kluwer Academic Publishers, Dordrecht, The Netherlands 1994. ISBN 0-79232827-2, 256 pp., Hardback, Dfl. 220, sP 88. Field Crops Res. 42, 143–144. doi: 10.1016/0378-4290(95)90040-3
Guinan K. J., Sujeeth N., Copeland R. B., Jones P. W., O'brien N. M., Sharma H. S. S., et al. (2012). Discrete roles for extracts of Ascophyllum nodosum in enhancing plant growth and tolerance to abiotic and biotic stresses. I World Congress Use Biostimulants Agric. 1009, 127–135. doi: 10.17660/ActaHortic.2013.1009.15
Gunupuru L. R., Patel J. S., Sumarah M. W., Renaud J. B., Mantin E. G., Prithiviraj B. (2019). A plant biostimulant made from the marine brown algae Ascophyllum nodosum and chitosan reduce Fusarium head blight and mycotoxin contamination in wheat. PLoS One 14, e0220562. doi: 10.1371/journal.pone.0220562
Hankins S. D., Hockey H. P. (1990). The effect of a liquid seaweed extract from Ascophyllum nodosum (Fucales, Phaeophyta) on the two-spotted red spider mite Tetranychus urticae. Hydrobiologia 204, 555–559. doi: 10.1007/BF00040286
Hasegawa P. M. (2013). Sodium (Na+) homeostasis and salt tolerance of plants. Environ. Experim. Bot. 92, 19–31. doi: 10.1016/j.envexpbot.2013.03.001
Hegazi A. M., El-Shraiy A. M., Ghoname A. A. (2015). Alleviation of salt stress adverse effect and enhancing phenolic anti-oxidant content of eggplant by seaweed extract. Gesunde Pflanzen. 67, pp.21–pp.31. doi: 10.1007/s10343-014-0333-x
Hernández-Herrera R. M., Santacruz-Ruvalcaba F., Ruiz-López M. A., Norrie J., Hernández-Carmona G. (2014a). Effect of liquid seaweed extracts on growth of tomato seedlings (Solanum lycopersicum L.). J. Appl. Phycol. 26, 619–628. doi: 10.1007/s10811-013-0078-4
Hernández-Herrera R. M., Virgen-Calleros G., Ruiz-López M., Zañudo-Hernández J., Délano-Frier J. P., Sánchez-Hernández C. (2014b). Extracts from green and brown seaweeds protect tomato (Solanum lycopersicum) against the necrotrophic fungus Alternaria solani. J. Appl. Phycol. 26, 1607–1614. doi: 10.1007/s10811-013-01932
Hussain S., Zhu C., Huang J., Huang J., Zhu L., Cao X., et al. (2020). Ethylene response of salt stressed rice seedlings following Ethephon and 1-methylcyclopropene seed priming. Plant Growth Regul. 92, 219–231. doi: 10.1007/s10725-020-00632-1
Ibrahim W. M., Ali R. M., Hemida K. A., Sayed M. A. (2014). Role of Ulva lactuca extract in alleviation of salinity stress on wheat seedlings. Sci. World J. 1), 847290. doi: 10.1155/2014/847290
Indira K., Balakrishnan S., Srinivasan M., Bragadeeswaran S., Balasubramanian T. (2013). Evaluation of in vitro antimicrobial property of seaweed (Halimeda tuna) from Tuticorin coast, Tamil Nadu, Southeast coast of India. Afr. J. @ Biotechnol. 12, 284–289. doi: 10.5897/AJB12.014
Jaulneau V., Lafitte C., Jacquet C., Fournier S., Salamagne S., Briand X., et al. (2010). Ulvan, a sulfated polysaccharide from green algae, activates plant immunity through the jasmonic acid signaling pathway. BioMed. Res. Int. 2010, 525291. doi: 10.1155/2010/525291
Jayaraj J., Wan A., Rahman M., Punja Z. K. (2008). Seaweed extract reduces foliar fungal diseases on carrot. Crop Prot. 27, 1360–1366. doi: 10.1016/j.cropro.2008.05.005
Jayaraman J., Norrie J., Punja Z. K. (2011). Commercial extract from the brown seaweed Ascophyllum nodosum reduces fungal diseases in greenhouse cucumber. J. @ Appl. Phycol 23, 353–361. doi: 10.1007/s10811-010-9547-1
Karabudak T., Bor M., Özdemir F., Türkan İ. (2014). Glycine betaine protects tomato (Solanum lycopersicum) plants at low temperature by inducing fatty acid desaturase and lipoxygenase gene expression. Mol. Boil. Rep. 41, 1401–1410. doi: 10.1007/s11033-013-2984-6
Khallil A. M., Daghman I. M. (2015). Fadyaa antifungal potential in crude extracts of five selected brown seaweeds collected from the western Libya coast. J. Micro. Creat. 1, 103. doi: 10.15744/2575-5498.1.103
Khan S. A., Abid M., Hussain F. (2015). Nematicidal activity of seaweeds against Meloidogyne javanica. Pakistan J. @ Nematol 33, 195–203.
Khan W., Rayirath U. P., Subramanian S., Jithesh M. N., Rayorath P., Hodges D. M., et al. (2009). Seaweed extracts as biostimulants of plant growth and development. J. @ Plant Growth Regul. 28, 386–399. doi: 10.1007/s00344-009-9103-x
Khan W., Zhai R., Souleimanov A., Critchley A. T., Smith D. L., Prithiviraj B. (2012). Commercial extract of Ascophyllum nodosum improves root colonization of alfalfa by its bacterial symbiont Sinorhizobium meliloti. Commun. Soil Sci. Plant Anal. 43, 2425–2436. doi: 10.1080/00103624.2012.708079
Klarzynski O., Descamps V., Plesse B., Yvin J. C., Kloareg B., Fritig B. (2003). Sulfated fucan oligosaccharides elicit defense responses in tobacco and local and systemic resistance against tobacco mosaic virus. Mol. Plant-Micr. Int. 16, 115–122. doi: 10.1094/MPMI.2003.16.2.115
Klarzynski O., Plesse B., Joubert J. M., Yvin J. C., Kopp M., Kloareg B., et al. (2000). Linear β-1, 3 glucans are elicitors of defense responses in tobacco. Plant Physiol. 124, 1027–1038. doi: 10.1104/pp.124.3.1027
Kloareg B., Quatrano R. S. (1988). Structure of the cell walls of marine algae and ecophysiological functions of the matrix polysaccharides. Oceanogr. Mar. Biol.: Annu. Review. 26, 259–315.
Kobayashi A., Tai A., Kanzaki H., Kawazu K. (1993). Elicitor-active oligosaccharides from algal laminaran stimulate the production of antifungal compounds in alfalfa. Z. für Naturforschung C 48, 575–579. doi: 10.1515/znc-1993-7-808
Kumar C. S., Sarada D. V., Rengasamy R. (2008). Seaweed extracts control the leaf spot disease of the medicinal plant Gymnema sylvestre. Indian J. Sci. Technol. 1, 1–5. doi: 10.17485/ijst/2008/v1i3/8
Kurepin L. V., Zaman M., Pharis R. P. (2014). Phytohormonal basis for the plant growth promoting action of naturally occurring biostimulators. J. @ Sci. Food Agriol 94, 1715–1722. doi: 10.1002/jsfa.6545
Liu P. P., Yang Y., Pichersky E., Klessig D. F. (2010). Altering expression of benzoic acid/salicylic acid carboxyl methyltransferase 1 compromises systemic acquired resistance and PAMP-triggered immunity in Arabidopsis. Mol. Plant-Microbe Interact. 23, 82–90. doi: 10.1094/MPMI-23-1-0082
Lizzi Y., Coulomb C., Polian C., Coulomb P. J., Coulomb P. O. (1998). Seaweed and mildew: what does the future hold? Phytoma La Defense Des. Vegetaux 508, 29–30.
Loureiro R. R., Reis R. P., Marroig R. G. (2014). Effect of the commercial extract of the brown alga Ascophyllum nodosum Mont. on Kappaphycus alvarezii (Doty) Doty ex PC Silva in situ submitted to lethal temperatures. J. @ Appl. Phycol 26, 629–634. doi: 10.1007/s10811-013-0085-5
Ma Y., Dias M. C., Freitas H. (2020). Drought and salinity stress responses and microbe-induced tolerance in plants. Front. Plant Sci. 11. doi: 10.3389/fpls.2020.591911
Manavalan L. P., Guttikonda S. K., Phan Tran L. S., Nguyen H. T. (2009). Physiological and molecular approaches to improve drought resistance in soybean. Plant Cell Physiol. 50, 1260–1276. doi: 10.1093/pcp/pcp082
Mancuso S., Azzarello E., Mugnai S., Briand X. (2006). Marine bioactive substances (IPA extract) improve foliar ion uptake and water stress tolerance in potted Vitis vinifera plants. Adv. Hortic. Sci. 20, 156–161. Available at: http://www.jstor.org/stable/4288247.
Manefield M., Rasmussen T. B., Henzter M., Andersen J. B., Steinberg P., Kjelleberg S., et al. (2002). Halogenated furanones inhibit quorum sensing through accelerated LuxR turnover. Microbiol. 148, 1119–1127. doi: 10.1099/00221287-148-4-1119
Martynenko A., Shotton K., Astatkie T., Petrash G., Fowler C., Neily W., et al. (2016). Thermal imaging of soybean response to drought stress: the effect of ascophyllum nodosum seaweed extract. Springerplus 5, 1393. doi: 10.1186/s40064-016-3019-2
Mayer A. M. (2006). Polyphenol oxidases in plants and fungi: going places? A review. Phytochem. 67, 2318–2331. doi: 10.1016/j.phytochem.2006.08.006
Mazzola M. (2004). Assessment and management of soil microbial community structure for disease suppression. Annu. Rev. Phytopathol. 42, 35–59. doi: 10.1146/annurev.phyto.42.040803.140408
Mckersie B. D., Leshem Y. A. Y., Mckersie B. D., Leshem Y. A. Y. (1994). Desiccation. Stress and stress coping in cultivated plants. Springer 132–147.
Meénard R., Alban S., de Ruffray P., Jamois F., Franz G., Fritig B., et al. (2004). β-1, 3 glucan sulfate, but not β-1, 3 glucan, induces the salicylic acid signaling pathway in tobacco and Arabidopsis. Plant Cell. 16, 3020–3032. doi: 10.1105/tpc.104.024968
Ménard R., de Ruffray P., Fritig B., Yvin J. C., Kauffmann S. (2005). Defense and resistance-inducing activities in tobacco of the sulfated β-1, 3 glucan PS3 and its synergistic activities with the unsulfated molecule. Plant Cell Physiol. 46, 1964–1972. doi: 10.1093/pcp/pci212
Mercier L., Lafitte C., Borderies G., Briand X., Esquerré-Tugayé M. T., Fournier J. (2001). The algal polysaccharide carrageenans can act as an elicitor of plant defence. New Phytol. 149, 43–51. doi: 10.1046/j.1469-8137.2001.00011.x
Miura K., Furumoto T. (2013). Cold signaling and cold response in plants. Int. J. Mol. Sci. 14, 5312–5337. doi: 10.3390/ijms14035312
Möller M., Smith M. L. (1998). The significance of the mineral component of seaweed suspensions on lettuce (Lactuca sativa L.) seedling growth. J. Plant Physiol. 153, 658–663. doi: 10.1016/S0176-1617(98)80217-4
Mukherjee A., Bhowmick S., Yadav S., Rashid M. M., Chouhan G. K., Vaishya J. K., et al. (2021a). Re-vitalizing of endophytic microbes for soil health management and plant protection. 3 Biotech. 11, 1–17. doi: 10.1007/s13205-021-02931-4
Mukherjee A., Gaurav A. K., Patel A. K., Singh S., Chouhan G. K., Lepcha A., et al. (2021b). Unlocking the potential plant growth-promoting properties of chickpea (Cicer arietinum L.) seed endophytes bio-inoculants for improving soil health and crop production. Land Degrad. Dev. 32, 4362–4374. doi: 10.1002/ldr.4042
Mukherjee A., Patel J. S. (2020). Seaweed extract: biostimulator of plant defense and plant productivity. Int. J. Environ. Sci. Technol. 17, 553–558. doi: 10.1007/s13762-019-02442-z
Nabati D. A., Schmidt R. E., Parrish D. J. (1994). Alleviation of salinity stress in Kentucky bluegrass by plant growth regulators and iron. Crop Sci. 34, 198–202. doi: 10.2135/cropsci1994.0011183X003400010035x
Nair P., Kandasamy S., Zhang J., Ji X., Kirby C., Benkel B., et al. (2012). Transcriptional and metabolomic analysis of Ascophyllum nodosum mediated freezing tolerance in Arabidopsis thaliana. BMC Genet. 13, 1–23. doi: 10.1186/1471-2164-13-643
Naseem M., Kunz M., Dandekar T. (2014). Probing the unknowns in cytokinin-mediated immune defense in Arabidopsis with systems biology approaches. BBI 8, BBIS13462. doi: 10.4137/BBI.S13462
Neily W., Shishkov L., Nickerson S., Titus D., Norrie J. (2010). Commercial extract from the brown seaweed Ascophyllum nodosum (Acadian (R)) improves early establishment and helps resist water stress in vegetable and flower seedlings. HortScience 45, S105–S106.
Neily W., Shishkov L., Tse T., Titus D. (2008). Acadian LSC helps reduce salinity stress in pepper seedlings cv. California Wonder. PGRSA Newsl. 1, 14.
Oliver R. P. (2024). “Classification of phytopathogens and diseases,” in Agrios' Plant Pathology. Elsevier, 333–338. doi: 10.1016/B978-0-12-822429-8.00012-1
Patel J. S., Selvaraj V., Gunupuru L. R., Rathor P. K., Prithiviraj B. (2020). Combined application of Ascophyllum nodosum extract and chitosan synergistically activates hostdefense of peas against powdery mildew. BMC Plant Biol. 20, 1–10. doi: 10.1186/s12870-020-2287-8
Patel K., Agarwal P., Agarwal P. K. (2018). Kappaphycus alvarezii sap mitigates abiotic-induced stress in triticum durum by modulating metabolic coordination and improves growth and yield. J. Appl. Phycol 30, 2659–2673. doi: 10.1007/s10811-018-1423-4
Patier P., Yvin J. C., Kloareg B., Liénart Y., Rochas C. (1993). Seaweed liquid fertilizer from Ascophyllum nodosum contains elicitors of plant D-glycanases. J. Appl. Phycol. 5, 343–349. doi: 10.1007/BF02186237
Paul J. (2014). Influence of seaweed liquid fertilizer of Gracilaria dura (AG.) J. AG.(red seaweed) on Vigna radiata (l.) r. wilczek., in thoothukudi, tamil nadu, India. World J. Pharm. Res. 3, 968–978.
Paulert R., Talamini V., Cassolato J. E. F., Duarte M. E. R., Noseda M. D., Smania A., et al. (2009). Die Wirkung von sulfonierten Polysacchariden und alkoholischen Extrakten aus der Grünalge Ulva fasciata auf den Anthracnosebefall und das Wachstum von Buschbonenpflanzen (Phaseolus vulgaris L.). J. Plant Dis. Prot. 116, 263–270. doi: 10.1007/BF03356321
Peres J. C. F., Carvalho L. R. D., Gonçalez E., Berian L. O. S., Felicio J. D. (2012). Evaluation of antifungal activity of seaweed extracts. Ciênc. Agrotec. 36, 294–299. doi: 10.1590/S1413-70542012000300004
Pérez M. J., Falqué E., Domínguez H. (2016). Antimicrobial action of compounds from marine seaweed. Mar. Drugs 14, 52. doi: 10.3390/md14030052
Pieterse C. M., Leon-Reyes A., van der Ent S., Van Wees S. C. (2009). Networking by small-molecule hormones in plant immunity. Nat. Chem. Biol. 5, 308–316. doi: 10.1038/nchembio.164
Potin P., Bouarab K., Küpper F., Kloareg B. (1999). Oligosaccharide recognition signals and defence reactions in marine plant-microbe interactions. Curr. Opin. Microbiol. 2, 276–283. doi: 10.1016/S1369-5274(99)80048-4
Raghavendra V. B., Lokesh S., Prakash H. S. (2007). Dravya, a product of seaweed extract (Sargassum wightii), induces resistance in cotton against Xanthomonas campestris pv. malsvacearum. Phytoparasitica. 35, 442–449. doi: 10.1007/BF03020602
Raj T. S., Graff K. H., Arumukapravin A., Suji H. A. (2016). Effect of various red seaweed extracts on controlling sheath blight in rice caused by Rhizoctonia solani Kuhn. I. J. Trop. Agric. 34, 2265–2278.
Raj T. S., Graff K. H., Suji H. A. (2018). Evaluation of various marine products against Rhizoctonia solani under in vitro condition. Afr. J. Microbiol. Res. 12, 46–51. doi: 10.5897/AJMR2016.8036
Ramkissoon A., Ramsubhag A., Jayaraman J. (2017). Phytoelicitor activity of three Caribbean seaweed species on suppression of pathogenic infections in tomato plants. J. Appl. Phycol. 29, 3235–3244. doi: 10.1007/s10811-017-1160-0
Rasmussen T. B., Manefield M., Andersen J. B., Eberl L., Anthoni U., Christophersen C., et al. (2000). How Delisea pulchra furanones affect quorum sensing and swarming motility inSerratia liquefaciens MG1. Microbiol. 146, 3237–3244. doi: 10.1099/00221287-146-12-3237
Rayirath P., Benkel B., Mark Hodges D., Allan-Wojtas P., MacKinnon S., Critchley A. T., et al. (2009). Lipophilic components of the brown seaweed, Ascophyllum nodosum, enhance freezing tolerance in Arabidopsis thaliana. Planta 230, 135147. doi: 10.1007/s00425-009-0920-8
Rekanović E., Potočnik I., Milijašević-Marčić S., Stepanović M., Todorović B., Mihajlović M. (2010). Efficacy of seaweed concentrate from Ecklonia maxima (Osbeck) and conventional fungicides in the control of Verticillium wilt of pepper. Pesticidii fitomedicina 25, 319–324. doi: 10.2298/PIF1004319R
Roberti R., Righini H., Reyes C. P. (2016). Activity of seaweed and cyanobacteria water extracts against Podosphaera xanthii on zucchini. Ital. J. Mycol. 45, 66–77. doi: 10.6092/issn.2531-7342/6425
Santaniello A., Scartazza A., Gresta F., Loreti E., Biasone A., Di Tommaso D., et al. (2017). Ascophyllum nodosum seaweed extract alleviates drought stress in Arabidopsis by affecting photosynthetic performance and related gene expression. Front. Plant Sci. 8. doi: 10.3389/fpls.2017.01362
Schroeder J. I., Kwak J. M., Allen G. J. (2001). Guard cell abscisic acid signalling and engineering drought hardiness in plants. Nature. 410, 327–330. doi: 10.1038/35066500
Seiber J. N., Coats J., Duke S. O., Gross A. D. (2014). Biopesticides: state of the art and future opportunities. J. Agric. Food Chem. 62, 11613–11619. doi: 10.1021/jf504252n
Semenov M. A., Shewry P. R. (2011). Modelling predicts that heat stress, not drought, will increase vulnerability of wheat in Europe. Sci. Rep. 1, 66. doi: 10.1038/srep00066
Shukla P. S., Agarwal P. K., Jha B. (2012). Improved salinity tolerance of Arachis hypogaea (L.) by the interaction of halotolerant plant-growth-promoting rhizobacteria. J. Plant Growth Regul. 31, 195–206. doi: 10.1007/s00344-011-9231-y
Shukla P. S., Borza T., Critchley A. T., Prithiviraj B. (2016). Carrageenans from red seaweeds as promoters of growth and elicitors of defense response in plants. Front. Mar. Sci. 3. doi: 10.3389/fmars.2016.00081
Shukla P. S., Gupta K., Agarwal P., Jha B., Agarwal P. K. (2015). Overexpression of a novel SbMYB15 from Salicornia brachiata confers salinity and dehydration tolerance by reduced oxidative damage and improved photosynthesis in transgenic tobacco. Planta. 242, 1291–1308. doi: 10.1007/s00425-015-2366-5
Shukla P. S., Shotton K., Norman E., Neily W., Critchley A. T., Prithiviraj B. (2018). Seaweed extract improve drought tolerance of soybean by regulating stress-response genes. AoBP. 10, plx051. doi: 10.1093/aobpla/plx051
Soliman A. S., Ahmed A. Y., Abdel-Ghafour S. E., El-Sheekh M. M., Sobhy H. M. (2018). Antifungal bio-efficacy of the red algae Gracilaria confervoides extracts against three pathogenic fungi of cucumber plant. Middle East J. Appl. Sci. 8, 727–735.
Solorzano-Chavez E. G., Paz-Cedeno F. R., de Oliveira L. E., Gelli V. C., Monti R., de Oliveira S. C., et al. (2019). Evaluation of the kappaphycus alvarezii growth under different environmental conditions and efficiency of the enzymatic hydrolysis of the residue generated in the carrageenan processing. Biomass Bioenergy 127, 105254.
Spann T. M., Little H. A. (2011). Applications of a commercial extract of the brown seaweed Ascophyllum nodosum increases drought tolerance in container-grown ‘Hamlin’sweet orange nursery trees. Hortic. Sci. 46, 577–582. doi: 10.21273/HORTSCI.46.4.577
Stephen A. B., John K. M. T., Palnia L. M. S., Lethama D. S. (1985). Detection of cytokinins in a seaweed extract. Phytochem. 24, 2611–2614. doi: 10.1016/S0031-9422(00)80679-2
Stephenson W. M. (1966). “The effect of hydrolysed seaweed on certain plant pests and diseases,” in Proceedings of the Fifth International Seaweed Symposium, Halifax, August 25–28. 405–415. doi: 10.1016/B978-0-08-011841-3.50064-1
Stirk W. A., Tarkowská D., Turečová V., Strnad M., Van Staden J. (2014). Abscisic acid, gibberellins and brassinosteroids in Kelpak®, a commercial seaweed extract made from Ecklonia maxima. J. Appl. Phycol. 26, 561–567. doi: 10.1007/s10811-013-0062-z
Subramanian S., Sangha J. S., Gray B. A., Singh R. P., Hiltz D., Critchley A. T., et al. (2011). Extracts of the marine brown macroalga, Ascophyllum nodosum, induce jasmonic acid dependent systemic resistance in Arabidopsis thaliana against Pseudomonas syringae pv. tomato DC3000 and Sclerotinia sclerotiorum. Eur. J. Plant Pathol. 131, 237–248. doi: 10.1007/s10658-011-9802-6
Sujeeth N., Petrov V., Guinan K. J., Rasul F., O’Sullivan J. T., Gechev T. S. (2022). Current insights into the molecular mode of action of seaweed-based biostimulants and the sustainability of seaweeds as raw material resources. Int. J. Mol. Sci. 23, 7654. doi: 10.3390/ijms23147654
Sultana V., Baloch G. N., Ara J., Ehteshamul-Haque S., Tariq R. M., Athar M. (2012). Seaweeds as an alternative to chemical pesticides for the management of root diseases of sunflower and tomato. J. Appl. Bot. Food Qual. 84, 162.
Sultana V., Ehteshamul-Haque S., Ara J., Athar M. (2005). Comparative efficacy of brown, green and red seaweeds in the control of root infecting fungi and okra. Int. J. Environ. Sci. Technol. 2, 129132. doi: 10.1007/BF03325866
Tariq A., Ara J., Sultana V., Ehteshamul-Haque S., Athar M. (2011). Antioxidant potential of seaweeds occurring at Karachi coast of Pakistan. J. Appl. Bot. Food Qual 84, 207–212.
Tay S. A. B., MacLeod J. K., Palni L. M. S., Letham D. S. (1985). Detection of cytokinins in a seaweed extract. Phytochemistry 24, 2611–2614.
Thinakaran T., Sivakumar K. (2013). Antifungal activity of certain seaweeds from Puthumadam coast. Int. J. Res. Rev. Pharm. Appl. Sci. 3, 341–350.
Uppal A. K., El Hadrami A., Adam L. R., Tenuta M., Daayf F. (2008). Biological control of potato Verticillium wilt under controlled and field conditions using selected bacterial antagonists and plant extracts. Biocontrol. 44, 90–100. doi: 10.1016/j.biocontrol.2007.10.020
Vallinayagam K., Arumugam R., Kannan R. R. R., Thirumaran G., Anantharaman P. (2009). Antibacterial activity of some selected seaweeds from Pudumadam coastal regions. Global J. Pharmacol. 3, 50–52.
Van Loon L. C., Rep M., Pieterse C. M. (2006). Significance of inducible defense-related proteins in infected plants. Annu. Rev. Phytopathol. 44, 135–162. doi: 10.1146/annurev.phyto.44.070505.143425
Van Oosten M. J., Pepe O., De Pascale S., Silletti S., Maggio A. (2017). The role of biostimulants and bioeffectors as alleviators of abiotic stress in crop plants. Chem. Biol. Technol. Agric. 4, 1–12. doi: 10.1186/s40538-017-0089-5
Vera J., Castro J., Contreras R. A., González A., Moenne A. (2012). Oligo-carrageenans induce a long-term and broad-range protection against pathogens in tobacco plants (var. Xanthi). Physiol. Mol. Plant Pathol. 79, 31–39. doi: 10.1016/j.pmpp.2012.03.005
Vera J., Castro J., Gonzalez A., Moenne A. (2011). Seaweed polysaccharides and derived oligosaccharides stimulate defense responses and protection against pathogens in plants. Mar. Drugs 9, 2514–2525. doi: 10.3390/md9122514
Vinocur B., Altman A. (2005). Recent advances in engineering plant tolerance to abiotic stress: achievements and limitations. Curr. Opin. Biotechnol. 16, 123–132. doi: 10.1016/j.copbio.2005.02.001
Wackett L. P. (2020). Microbial biocontrols in agriculture: An annotated selection of World Wide Web sites relevant to the topics in microbial biotechnology. Microb. Biotechnol. 13, 814. doi: 10.1111/17517915.13569
Wally O. S., Critchley A. T., Hiltz D., Craigie J. S., Han X., Zaharia L. I., et al. (2013). Regulation of phytohormone biosynthesis and accumulation in Arabidopsis following treatment with commercial extract from the marine macroalga Ascophyllum nodosum. J. Plant Growth Regul. 32, 324–339. doi: 10.1007/s00344012-9301-9
Wang W., Vinocur B., Altman A. (2003). Plant responses to drought, salinity and extreme temperatures: towards genetic engineering for stress tolerance. Planta 218, 1–14. doi: 10.1007/s00425-003-1105-5
Wani H. S., Singh B., Haribhushan A., Iqbal Mir J. (2013). Compatiblesolute engineering in plants for abiotic stress tolerance-role of glycine betaine. Cur. Genom. 14, 157–165. doi: 10.1007/s10811-013-0193-2
Washington W. S., Engleitner S., Boontjes G., Shanmuganathan N. (1999). Effect of fungicides, seaweed extracts, tea tree oil, and fungal agents on fruit rot and yield in strawberry. Aust. J. Exp. Agric. 39, 487–494. doi: 10.1071/EA98164
Wilson S. (2001). Frost management in cool climate vineyards Vol. 99 (Australia: University of Tasmania research report UT).
Winzer K., Williams P. (2001). Quorum sensing and the regulation of virulence gene expression in pathogenic bacteria. Int. J. Med. Microbiol. 291, 131–143. doi: 10.1078/1438-422100110
Wite D., Mattner S. W., Porter I. J., Arioli T. (2015). The suppressive effect of a commercial extract from Durvillaea potatorum and Ascophyllum nodosum on infection of broccoli by Plasmodiophora brassicae. J. Appl. Phycol. 27, 2157–2161. doi: 10.1007/s10811015-0564-y
Wu Y., Jenkins T., Blunden G., von Mende N., Hankins S. D. (1998). Suppression of fecundity of the root-knot nematode, Meloidogyne javanica, in monoxenic cultures of Arabidopsis thaliana treated with an alkaline extract of Ascophyllum nodosum. J. Appl. Phycol. 10, 91–94. doi: 10.1023/A:1008067420092
Wu Y., Jenkins T., Blunden G., Whapham C., Hankins S. D. (1997). The role of betaines in alkaline extracts of Ascophyllum nodosum in the reduction of Meloidogyne javanica and M. incognita infestations in tomato plants. Fund. Appl. Nematol. 20, 99–102.
Xu X., Iwamoto Y., Kitamura Y., Oda T., Muramatsu T. (2003). Root growth-promoting activity of unsaturated oligomeric uronates from alginate on carrot and rice plants. Biosci. Biotechnol. Biochem. 67, 2022–2025. doi: 10.1271/bbb.67.2022
Xu C., Leskovar D. I. (2015). Effects of A. nodosum seaweed extracts on spinach growth, physiology and nutrition value under drought stress. Sci. Hortic. 183, 39–47. doi: 10.1016/j.scienta.2014.12.004
Yadav N. S., Shukla P. S., Jha A., Agarwal P. K., Jha B. (2012). The SbSOS1 gene from the extreme halophyte Salicornia brachiata enhances Na+ loading in xylem and confers salt tolerance in transgenic tobacco. BMC Plant Bio. 12, 1–18. doi: 10.1186/1471-222912-188
Yakhin O. I., Lubyanov A. A., Yakhin I. A., Brown P. H. (2017). Biostimulants in plant science: a global perspective. Front. Plant Sci. 7. doi: 10.3389/fpls.2016.02049
Yordanov I., Velikova V., Tsonev T. (2000). Plant responses to drought, acclimation, and stress tolerance. Photosynthetica 38, 171–186.
Zbakh H., Chiheb H., Bouziane H., Riadi H. (2012). Antibacterial activity of benthicmarine algae extracts from the Mediterranean coast of Morocco. J. Microbiol. Biotechnol. Food Sci. 2, 219–228.
Zhang X., Ervin E. H. (2004). Cytokinin-containing seaweed and humic acid extracts associated with creeping bentgrass leaf cytokinins and drought resistance. Crop Sci. 44, 1737–1745. doi: 10.2135/cropsci2004.1737
Zhang X., Ervin E. H. (2008). Impact of seaweed extract-based cytokinins and zeatin riboside on creeping bentgrass heat tolerance. Crop Sci. 48, 364–370. doi: 10.2135/cropsci2007.05.0262
Zhang X., Ervin E. H., Schmidt R. E. (2003). Physiological effects of liquid applications of a seaweed extract and a humic acid on creeping bentgrass. J. Amer. Soc Hortic. Sci. 128, 492–496. doi: 10.21273/JASHS.128.4.0492
Zhang X., Schmidt R. E. (2000). Hormone-containing products' impact on antioxidant status of tall fescue and creeping bentgrass subjected to drought. Crop Sci. 40, 1344–1349. doi: 10.2135/cropsci2000.4051344x
Ziosi V., Zandoli R., Di Nardo A., Biondi S., Antognoni F., Calandriello F. (2012). Biological activity of different botanical extracts as evaluated by means of an array of in vitro and in vivo bioassays. I World Congress Use Biostimulants Agric. 1009, 61–66. doi: 10.17660/ActaHortic.2013.1009.5
Zodape S. T., Gupta A., Bhandari S. C., Rawat U. S., Chaudhary D. R., Eswaran K., et al. (2011). Foliar application of seaweed sap as biostimulant for enhancement of yield and quality of tomato (Lycopersicon esculentum Mill.). J. Sci. Indus. Res. 0975-1084, 215–219. Available at: http://nopr.niscpr.res.in/handle/123456789/11089.
Keywords: abiotic stress, agricultural productivity, biotic stress, plant defense, seaweeds
Citation: Kumar G, Nanda S, Singh SK, Kumar S, Singh D, Singh BN and Mukherjee A (2024) Seaweed extracts: enhancing plant resilience to biotic and abiotic stresses. Front. Mar. Sci. 11:1457500. doi: 10.3389/fmars.2024.1457500
Received: 30 June 2024; Accepted: 01 October 2024;
Published: 15 November 2024.
Edited by:
Maria Fernanda Lopez Climent, University of Jaume I, SpainReviewed by:
Paulo Cardoso, University of Aveiro, PortugalCopyright © 2024 Kumar, Nanda, Singh, Kumar, Singh, Singh and Mukherjee. This is an open-access article distributed under the terms of the Creative Commons Attribution License (CC BY). The use, distribution or reproduction in other forums is permitted, provided the original author(s) and the copyright owner(s) are credited and that the original publication in this journal is cited, in accordance with accepted academic practice. No use, distribution or reproduction is permitted which does not comply with these terms.
*Correspondence: Arpan Mukherjee, YXJwYW4ubXVraGVyamVlNTVAZ21haWwuY29t
Disclaimer: All claims expressed in this article are solely those of the authors and do not necessarily represent those of their affiliated organizations, or those of the publisher, the editors and the reviewers. Any product that may be evaluated in this article or claim that may be made by its manufacturer is not guaranteed or endorsed by the publisher.
Research integrity at Frontiers
Learn more about the work of our research integrity team to safeguard the quality of each article we publish.