- 1Independent Researcher, Washington Grove, MD, United States
- 2Institute for Applied Ecology, Santa Fe, NM, United States
Marine harmful algal blooms (HAB) have been implicated in marine mammal die-offs; but the relationship between sub-lethal algal toxicity and marine mammal vulnerability to human activities has not been assessed. HAB toxins can result in compromised neurological or muscular systems and we posit these conditions can expose marine mammals to increased likelihood of entanglement in commercial fishing gear or ship strike. To investigate whether HABs and large whale injuries and deaths were associated, we assessed the spatiotemporal co-occurrence of HAB events and large whale mortalities/injuries in U.S. east (from 2000-2021) and west (2007-2021) coastal waters. The number of mortalities/injuries was frequently higher in years with large-scale or severe HABs. We found statistically significant relationships between the occurrence of HABs and whale mortalities/injuries in west coast waters – at least three additional whale deaths/injuries were detected near an active HAB than in areas where a HAB was not reported. This relationship was similarly positive but weaker for east coast waters, a difference that may be attributable to differing oceanographic features, or approaches used in whale data collection, between coasts. Saxitoxin-producing Alexandrium was the most common causative agent on both the east (64.1%) and west (57.8%) coasts; and domoic acid-producing Pseudo-nitzschia was more common along the west (33.3%) than the east coast (8.7%). Algal toxins can be entrained in marine ecosystems, including in whale prey, and can chronically persist in marine mammals. Given many whale deaths/injuries result from fishing gear entanglement and vessel strikes, algal-induced morbidities may diminish whale capacities to detect or avoid fishing gear and approaching vessels. While there was much interannual variability, general increasing trends were observed in both whale death/injury and HAB datasets which may be attributable to increased monitoring or rising ocean temperatures. HAB prediction modeling has become increasingly sophisticated and could be used as a tool to reduce whale mortality by limiting human activities (e.g., curtailing fishing operations) when HABs, whale occurrence, and maritime activities are expected to overlap. Additional systematic data collection is needed to track and model mechanisms underlying relationships between HABs and incidental whale mortality.
1 Introduction
Marine Harmful Algal Blooms (HAB) are naturally occurring events involving toxic components that can be lethal to marine organisms. Numerous marine alga (as well as diatoms, dinoflagellates, collectively referred to as HABs) can proliferate rapidly under certain conditions, can involve multiple marine trophic levels, and are responsible for various physiological syndromes in humans and marine vertebrates. HABs are commonplace along both coasts of North America especially in spring to fall. Exposure to algal toxins may be quite common as aggregations of marine mammals utilize these waters for feeding, migrating and other purposes. Shellfish and some finfish species may tolerate, but bioaccumulate, algal biotoxins and transfer them to other organisms through food web interactions. Detection of certain thresholds of these substances in shellfish or water column sampling can prompt closures of commercial and recreational fisheries for public safety reasons.
Numerous naturally occurring species of dinoflagellate algal species (including, for example, the genera Alexandrium and Pyrodinium) produce potent saxitoxins (STX) that can result in potentially life-threatening Paralytic Shellfish Poisoning (PSP) in humans and may lead to various neurological symptoms including numbness in extremities and loss of motor control (e.g., Anderson et al., 1990). Human consumption of shellfish contaminated with domoic acid (DA) (from the diatom Pseudo-nitzschia) can, in acute phases (e.g., amnesic shellfish poisoning), cause dizziness, disorientation, lethargy, seizures, memory loss, and a degeneration of neurons controlling sensory and motor functions (Teitelbaum et al., 1990; Fire and Van Dolah, 2012) (see, also, Box 1).
Box 1. Effects of algal toxins.
A suite of naturally occurring dinoflagellate species (including Alexandrium spp., Gonyaulax spp., among others) produce potent saxitoxins (STX) that can lead to life-threatening neurological symptoms in humans. Sub-lethal STX toxicosis can include numbness in extremities, weakening of muscles, and loss of motor control. Human consumption of shellfish contaminated with domoic acid (DA) can result in dizziness, disorientation, lethargy, seizures, memory deficits, and a degeneration of neurons controlling sensory and motor functions (Teitelbaum et al., 1990; Fire and Van Dolah, 2012).
In marine mammals, STX acts by affecting neuromuscular junctions (IWC, 2017) and can result in decreased sensory functions, compromised muscle coordination, or listlessness, among other symptoms (Landsberg, 2002; Scholin et al., 2000. Flewelling et al., 2005). Exposure to DA in marine mammals can result in disorientation, uncoordinated movements, and seizures (McHuron et al., 2013; IWC, 2017). Spatial memory deficits, lethargy, and perseverative (repetitive) behavior have been described in California sea lions following sub-lethal exposures to DA (Goldstein et al., 2008; Cook et al., 2015, 2016). In other mammalian taxa, laboratory trials involving chronic low-level exposure of DA lead to structural and chemical changes in the brain in macaque monkeys (Macaca fascicularis, Petroff et al., 2019) and spatial memory impairment in mice (Lefebvre et al., 2017). Our analysis suggests that debilitating features of these toxins may also contribute to large whale entanglements and ship strikes.
Toxins from HABs have been detected in numerous marine mammal species (e.g., Landsberg, 2002; Danil et al., 2021) and relatively high levels have been reported in sea turtle (e.g., Capper et al., 2013), and seabird species (e.g., Shumway et al., 2003; Gibble et al., 2021). Among marine mammals, adverse effects from toxic algal exposure have been reported for manatees (Bossart et al., 1998; Flewelling et al., 2005; Capper et al., 2013) and cetaceans (e.g., Twiner et al., 2011; Fire et al., 2021).
Mortality and morbidity related to HAB exposure have been reported for hundreds of California sea lions (Zalophus californianus) along U.S. west coast beaches in multiple years in the 1990s to 2010s (Scholin et al., 2000; McCabe et al., 2016; Anderson et al., 2021). Numerous whale, pinniped, and dolphin deaths occurring on the west coast of North America and elsewhere have been attributed (though not always firmly established) to acute HAB exposure (e.g., Scholin et al., 2000; Fire et al., 2010; Häussermann et al., 2017; de la Riva et al., 2009). An early and well-documented mortality event occurred in 1987 in which the deaths of 14 humpback whales (Megaptera novaeangliae) in Massachusetts waters was attributed to whale consumption of saxitoxin in their finfish prey (Geraci et al., 1989).
However, lethal toxicity thresholds, biological pathways, and physiological effects for individuals with sublethal HAB toxin levels are not well understood and doses that can become lethal are not always known. Mechanisms for transmission and accumulation through trophic levels are likely complex as not all HABs result in widespread mammal deaths, and not all mammals that exhibit detectable toxin levels succumb. In addition, a large-scale mammal mortality event (involving primarily sei whales, Balaenoptera borealis) was attributed to a HAB during an El Nino year (Häussermann et al., 2017); but left unexplained are why die-offs of this magnitude do not also occur in other El Nino years or do not consistently occur in the presence of or large scale or particularly toxic HABs. And while symptoms of sublethal morbidity can be severe, apparently scores of marine mammal species chronically carry toxins with HAB origins yet exhibit no clinical signs of acute neurotoxicity (e.g., Lefebvre et al., 2002a; Danil et al., 2021; Fire et al., 2021; Hendrix et al., 2021).
Impaired neurological or sensory systems from sub-lethal effects involving compromised neurological, musculature, and sensory systems may make marine mammals susceptible to additional threats in their habitats. Whale and dolphin exposure to broadly distributed HAB events, on local or regional scales, may, for example, lead to individuals’ elevated vulnerability to fishing gear entanglement or being struck by a moving vessel because of an indifference to the threats, or as a consequence of diminished ability to detect or avoid them.
Entanglement in commercial fishing gear and collisions with sea-going vessels are significant threats to large whale populations (e.g., Reeves et al., 2013; Erbe et al., 2020) and likely inhibit the growth and recovery of some depleted populations (e.g., Thomas et al., 2016; Carretta et al., 2023). Fishing gear entanglements and ship strikes can account for hundreds of whale deaths and injuries each year in U.S. waters; globally, mortality rates are likely in the thousands from these sources (Read et al., 2006; Schoeman et al., 2020). Impacts from these threats could be worsened if large whales are disoriented, are experiencing diminished physiological or sensory systems thereby reducing detection and avoidance of vessels or fishing gear within their habitat.
The goal of this analysis was to determine the extent to which HAB events co-occur with large whale injuries/mortalities from ship strikes or entanglement in fishing gear. Specifically, we sought to test the hypothesis that sub-lethal exposures to HABs may disorient or desensitize large whales, thereby increasing the likelihood that they will suffer injury or death from interactions with human activities. We used existing datasets of HAB events in U.S. east and west coastal waters and records of large whale deaths and injuries to evaluate the timing and locations of recovered or reported whale injuries and deaths from human interaction (e.g., fishing gear entanglement and ship strikes) in relation to timing and locations of HAB events (especially severe or large-scale events). This broad-scale analysis provides compelling evidence, but does not confirm, that large whales may be at higher risk of ship strike or fishing gear entanglement after exposure to a HAB event.
2 Materials and methods
2.1 Acquiring and processing HAB data
Toxic aquatic algae can have adverse consequences for human health; consequently, HAB monitoring programs have been established in hundreds of countries globally. Observations from these programs are provided annually to a central repository, the Harmful Algal Event Database (HAEDAT), curated by UNESCO’s Intergovernmental Oceanographic Commission (HAEDAT; http://haedat.iode.org/). In the United States, routine HAB event monitoring is conducted primarily by state agencies, academic institutions, and coastal municipalities whose data are also provided to the HAEDAT global data base. Standardized HAB monitoring observations generally include, but are not limited to, information on location, dates of observations (and in some cases, the cessation of a bloom), the results of diagnostic testing for the causative agent, and toxic concentrations (generally reported as, for example, ‘mouse unit’ bioassays, or amount per unit of water). In many locations, negative results, i.e., absence of a detectable bloom during routine monitoring, are also reported.
Using the HAEDAT web site search function, we downloaded records in designated grids for all coastal continental U.S. waters from northern Maine to southern Florida, and along the U.S. Pacific Coast from northern Washington to southern California for the period 1969 to 2021.
We excluded HAB observations for the Gulf of Mexico because we were interested in possible impacts of HAB events primarily on large whale species occurring (e.g., in feeding or migrating locations) along the U.S. continental shelves (i.e. records from Alaska were excluded) and coastal margins. However, records from the coastline of eastern Florida were included.
A total of 1,026 records were downloaded (on 14 December 2022).
Of the records accessed, 357 were removed from analysis because they were outside the date ranges of corresponding periods for available whale death and injury data (2000-2021 for the U.S. east coast; 2007-2021 for U.S. west coast records). We removed all HAB event records involving lakes and inland rivers. Records of HAB events at the mouths of estuaries or semi-enclosed bodies of water (e.g., Puget Sound (Washington), the Straits of Juan de Fuca (British Columbia), San Francisco Bay (California), the Chesapeake Bay (Maryland/Virginia)) or large river mouths were retained in our analyses because toxins originating here could be expected to enter coastal marine systems from these sources, and because in some cases large whale species might be expected to enter or utilize some bays (e.g., San Francisco Bay or the Straits of Juan de Fuca) or large river mouths.
Regarding the Chesapeake Bay proper, records involving rivers and their tributaries entering the Bay were eliminated from analysis reasoning that planktonic toxins from a localized HAB event would have to be transported tens (or perhaps to hundreds) of miles to enter a fully marine ecosystem. However, blooms described as having occurred at the mouth of the Chesapeake Bay (e.g., Norfolk/Hampton, Virginia locations) were included in our analysis because these might exit the Bay with tidal fluxes. Records from various monitoring sites with HAB data extending along the length of Long Island Sound (New York) were included because, while some sites involved lagoon systems, they adjoin and readily connect with marine waters and can be areas of relatively high whale concentrations.
Additional records were also eliminated that lacked specificity or sufficient information (e.g., reported an absence of toxins, did not include information about toxin concentration levels), or provided only general information (e.g., merely noted ongoing monitoring programs with no accompanying information), or included comments such as ‘blooms have occurred in this area nearly annually.’ HAB events that involved the occurrence of brown tides (e.g., the algae Aureococcus sp.) were eliminated from our analysis since the occurrence of brown tides have no known toxic effects on vertebrates.
Not all records included specific latitude/longitude coordinate information for sampling locations. For records that lacked specific location data, we determined latitude and longitude information using descriptions for each record (e.g., county, nearest municipality, or other physical landmarks such as named beaches, bays, or islands) as indicated in Google Earth.
Reasoning that concentrations in seafoods that were of sufficient toxicity to be potentially unsafe for human consumption might also be detrimental to marine mammals, all HAB events that involved toxicity levels that exceeded nationally established thresholds that prompt fishery closures (e.g., ≥80 µg/100g STX and >20ppm DA in shellfish tissue; see, for example, standards established by the Food and Agriculture Organization of the United Nations (FAO: https://www.fao.org/3/y5486e/y5486e0d.htm#:~:text=The%20limit%20for%20PSP%20toxins,mg%20STX%20eq%2F100%20g) and elsewhere (e.g., Oregon Department of Fish and Wildlife https://myodfw.com/articles/shellfish-and-biotoxins) were included in our analysis. In addition, all HABs that resulted in a commercial or recreational seafood fishery closure were included in our analysis1.
Following the winnowing processes described here, a total of 194 records of HAB events were used in this analysis.
2.2 Whale injury and mortality data
Whale injury and death data were obtained from programs managed by NOAA’s National Marine Fisheries Service (NMFS) for 2000-2021 for U.S. east coast waters; and from 2007-2021 for U.S. west coast waters. Under authority of the U.S. Marine Mammal Protection Act, NMFS administers the National Marine Mammal Health and Stranding Response Program (https://www.fisheries.noaa.gov/national/marine-life-distress/marine-mammal-health-and-stranding-response-program) which relies on extensive stranding networks involving all U.S. coastal states and other partners. The Program endeavors to, among other things, quantify every marine mammal mortality and injury event in U.S. waters, including dead or injured but not stranded (i.e., offshore ‘floaters’) large whale. Data sources include opportunistic reports and observations and from systematic Federal and state aircraft- and ship-based surveys which are in turn curated by the stranding networks and central repositories. In addition, efforts are made to conduct detailed necropsies of dead whales to determine cause of death, when possible. Determinations are also made of ‘serious’ versus ‘non-serious’ injuries through a review process involving personnel at the NMFS’s Science Centers and Regional Offices, and designated external Scientific Review Group panels as dictated by NMFS policy directives which include specific guidelines for making such determinations (NMFS, 2012; NMFS, 2023; and https://media.fisheries.noaa.gov/dam-migration/02-238.pdf). As such, considerable effort is made to ensure large whale death and injury event data, and subsequent assessments of those records, are systematically collected and managed. However, not all whale fatalities and injuries are detected, reported, or provided to publicly available data repositories. Those limitations notwithstanding, the datasets analyzed here can be viewed as reliable representations of those events for our analytical purposes.
There are some differences between the U.S. west coast and east coast whale data: for example, the likelihood of detecting whale injuries/mortalities distant from shore (primarily off New England) are enhanced by systematic aircraft surveys used to determine abundance, distribution, and incidents of fishing entanglement to aid (primarily) North Atlantic right whale conservation efforts. In addition, the U.S. west coast dataset included exclusively those records in which whale deaths/injuries resulted from known interaction with human activities (e.g., fishing gear entanglement or ship strike); whereas the U.S. east coast data included classifications regarding cause of death/injury as, for example, ‘natural causes’ or ‘unknown.’ We did not include these latter cases in our analysis, using only those records in which interactions with human activities were noted. Doing so rendered the east and west coast datasets more comparable as the west coast data was focused primarily on those cases involving known human interactions. As such, the large whale strandings data analyzed here includes only cases in which interaction with some type of human activity was identified as the source of injury or death.
Using these datasets, we analyzed a total of 850 records of whale injury and mortality occurring in waters off the U.S eastern seaboard, and 566 records for the U.S. west coast.
Locations (i.e., latitudes and longitudes) for most of the whale recovery or observation site were provided in the east coast whale data set (A. Henry, pers comm.; and as indicated, for example, in Henry et al., 2022). For those without location information, we assigned coordinates for these data (for the purposes of plotting the locations) using landmarks that accompanied the data and as indicated in Google Earth. Location data were available for some, but not all, of the whale injury/mortality data from the U.S. west coast (J.V. Carretta, pers comm.), and where location data were absent, we ascribed location information as described above.
Dates and locations generally denoted when and where the injured or dead animal was initially observed or washed ashore. Therefore, locations (and to a certain, but lesser extent, the dates) provided for these records should be regarded as approximations of where the death or injury may have actually occurred. They may represent, for example, the location where a whale beach-stranded, but where it did not necessarily die; where it was first observed entangled in fishing gear whereas the actual entanglement may have occurred some miles distant; or where a whale may have been observed struck by a vessel but succumbed to its injuries elsewhere. Thus, these observations may have lacked the spatial specificity we or others have ascribed to them (i.e., they were translated into specific locations on a map). But because HABs can span tens, hundreds, in some cases thousands, of square miles, we believe approximate locations for whale mortality and injury observations were sufficient for the scales of analysis used here (e.g., up to 100 miles radius, as described below).
Similarly, inclusive dates of potential whale exposure to HAB toxins were assumed to have occurred for the duration of an active HAB, i.e., the dates in which detectable levels of toxins occurred in the environment as determined and reported by various state and federal monitoring programs. The durations and concentrations of exposures that result in serious consequences for marine mammals is not well established; and while not much is known about toxin residency times in mammal tissue, it can be reasonably assumed that marine mammals are exposed for at least the duration of an active bloom (Lefebvre et al., 2002a). Saxitoxin agents can remain in various bivalve species for days or weeks (e.g., Chou et al., 2005; Leblad et al., 2017; Medina et al., 2018). While some organisms, such as blue mussels (Mytilus edulis) are capable of depurating (reducing or eliminating) DA in hours to days (Krogstad et al., 2009), in razor (Siliqua patula), purple varnish clams (Nuttallia obscurata), and other organisms DA can persist much longer (Adams et al., 2000; Lefebvre and Robertson, 2010). Lefebvre et al. (2002a) detected DA in krill near feeding whales as well as in numerous fish species ranging from benthic sanddabs to pelagic anchovies, sardines, and albacore; they concluded that DA permeates benthic as well as pelagic communities. Fish (e.g., anchovies, Engraulis spp.) tend to depurate DA concentrations rapidly but can be potent DA vectors while a bloom is ongoing (Lefebvre et al., 2002b). Therefore, we can reasonably assume that the dates used in our analysis – beginning with the first detection and quantifying of a HAB event – would overlap with whale exposure to HAB toxins for the duration of the bloom.
2.3 HAB and whale data analysis
To determine if whale deaths and injuries were more likely to co-occur with reported HABs, we conducted three analyses. First, we fit a simple linear regression to assess correlations between the number of reported HAB occurrences and the number of human-related large whale deaths and injuries each year in waters off the U.S. west and east coasts. We fit separate models for the east and west coasts, with the number of reported HABs each year as a predictor and the number of reported large whale deaths and injuries each year as the response. In addition, we summarized the average density of whale mortality/injuries that occurred within the reported onset (i.e., first detection) and up to 30 days following conclusion (i.e., reported end date) of a bloom, and in areas of 10, 50, 100, and 200 miles surrounding a HAB event. We estimated the average density as the number of whale injuries/mortalities within each given timeframe and distance for each HAB, then calculated the mean for each timeframe and distance. Finally, we fit a simple linear regression to determine if the occurrence of HAB events was increasing through time along each coast. Analyses were completed in R (version 4.3.1).
3 Results
3.1 Summaries of whale and HAB data sets
A total of 11 algal genera were represented in the HAB database for the study periods (Table 1). Only three of these (Alexandrium, Pseudo-nitzschia, and Dinophysis) occurred on both U.S. coasts. The saxitoxin-producing Alexandrium was the dominate HAB causative agent and comprised 60.1% (n=118 total blooms) of the entire sample from both coasts, followed by the diatom Pseudo-nitzschia (n=42), and various other dinoflagellate species (n=31 combined blooms). Diversity of HAB causative agents was greater in east coast waters than off the west coast; and while Alexandrium blooms were nearly equally represented on both coasts, those involving Pseudo-nitzschia were more common in U.S. west coast waters (n=34) than off the east coast (n=8) (Table 1).
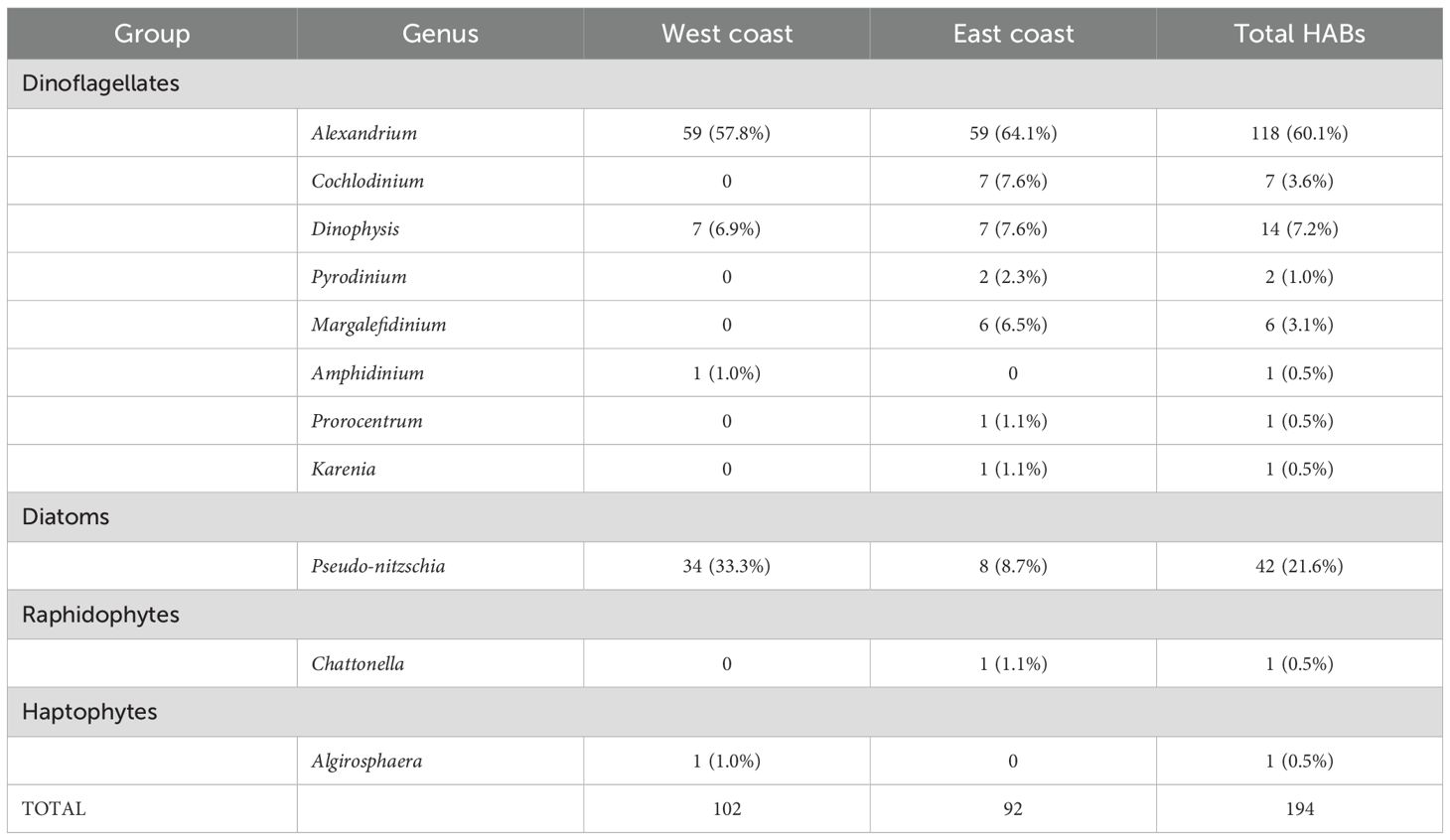
Table 1. Causative agents involved in harmful algal blooms (HAB) and the number and percentage caused by each for U.S. west coast waters, 2007-2021; and U.S. east coast waters, 2000-2021.
A total of nine species appeared in the whale human-related injury and mortality databases analyzed here (Table 2). Interaction with fishing gear and vessel strikes were the most common sources (when the cause was known) of whale injuries and mortalities (Table 3). Of the 556 records of whale mortality and injury for the U.S. west coast, fisheries interactions were involved in 422 (75.9% of all causes; most of these were interactions in which the type of gear could not be identified); 122 (21.9% of all causes) involved vessel strikes. Of the 850 observed east coast injuries and mortalities involving human interactions (723 records were removed from analysis in which the cause of injury/death was ‘unknown’ or resulted from ‘natural causes’), 645 (75.9%) instances involved fishing gear entanglement and 203 (23.9%) resulted from vessel strikes (Table 3). We note that while the cause of death or injury was listed as ‘unknown’ or ‘natural causes’ in many U.S. east coast records, in no instance was exposure to a HAB indicated as a cause of death.
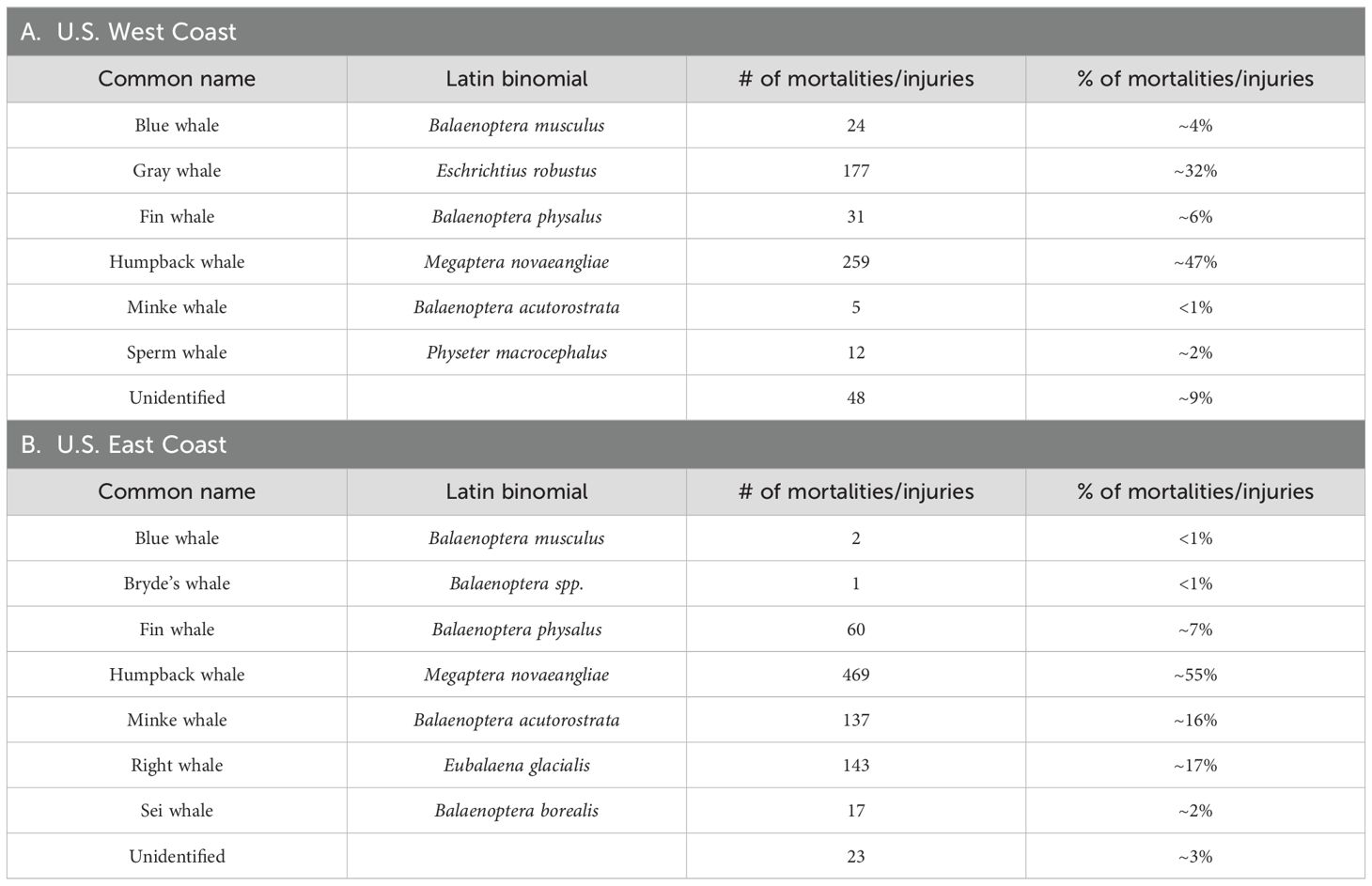
Table 2. Large whale species in U.S. west (A) and east coast (B) mortality and injury data bases and number of mortalities/injuries for each species.
3.2 Coincidence of HABs and whale mortalities/injuries
Graphical representation of HAB events and whale deaths/injuries indicate an overlap of these on both coasts of the United States (Figure 1). HABs tended to be relatively evenly distributed along the U.S. west coast but were spatially more like to occur in relatively higher latitudes (i.e., Chesapeake Bay mouth, north) along the U.S. east coast. They were frequently (i.e., in multiple years in our study period) reported in coastal Maine and Gulf of Maine waters; and reported in most years along the Massachusetts coasts, along the southern coasts of Long Island, and near the mouth of the Chesapeake Bay. Whale deaths/injuries were generally evenly distributed along the U.S. west coast coastal waters (Figure 1). Records of whale deaths/injuries on the U.S. east coast were weighted toward New England waters and in some cases were reported tens of miles from the shoreline.
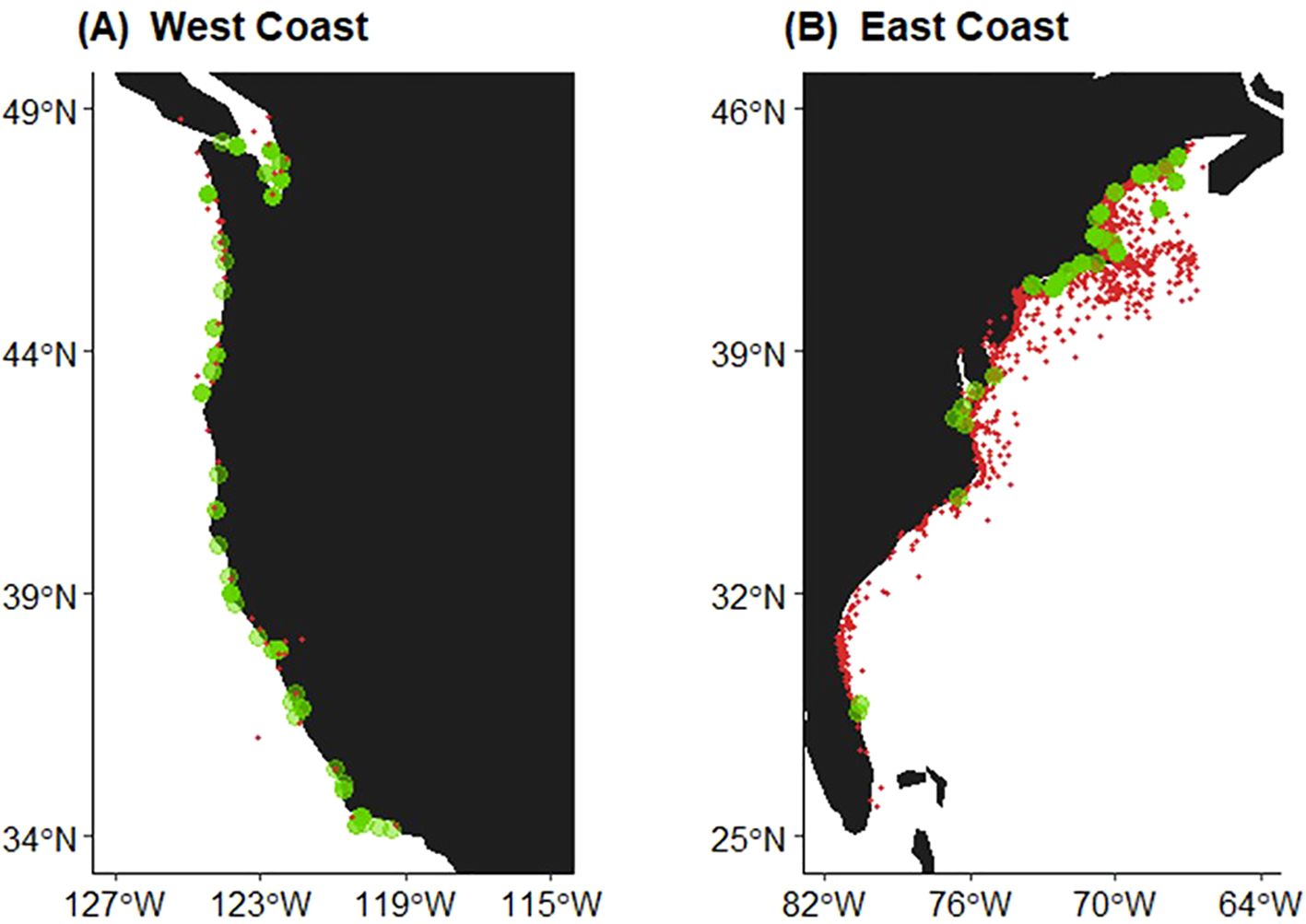
Figure 1. Map of harmful algal bloom (HAB) events (denoted by green circles) and reported large whale deaths and injury locations (red dots) for the (A) U.S. west coast, 2007-2021, and (B) U.S. east coast, 2000-2021.
We found a positive relationship between the occurrence of HABs and large whale deaths and injuries in waters off the U.S. west coast and east coast (Figure 2). This relationship was statistically significant in west coast waters () but not in east coast waters (). These results suggest an increase of at least three large whale deaths or injuries for each additional HAB off the west coast. There was much interannual variation in both the number of HABs and whale injuries/mortalities (Figure 3).
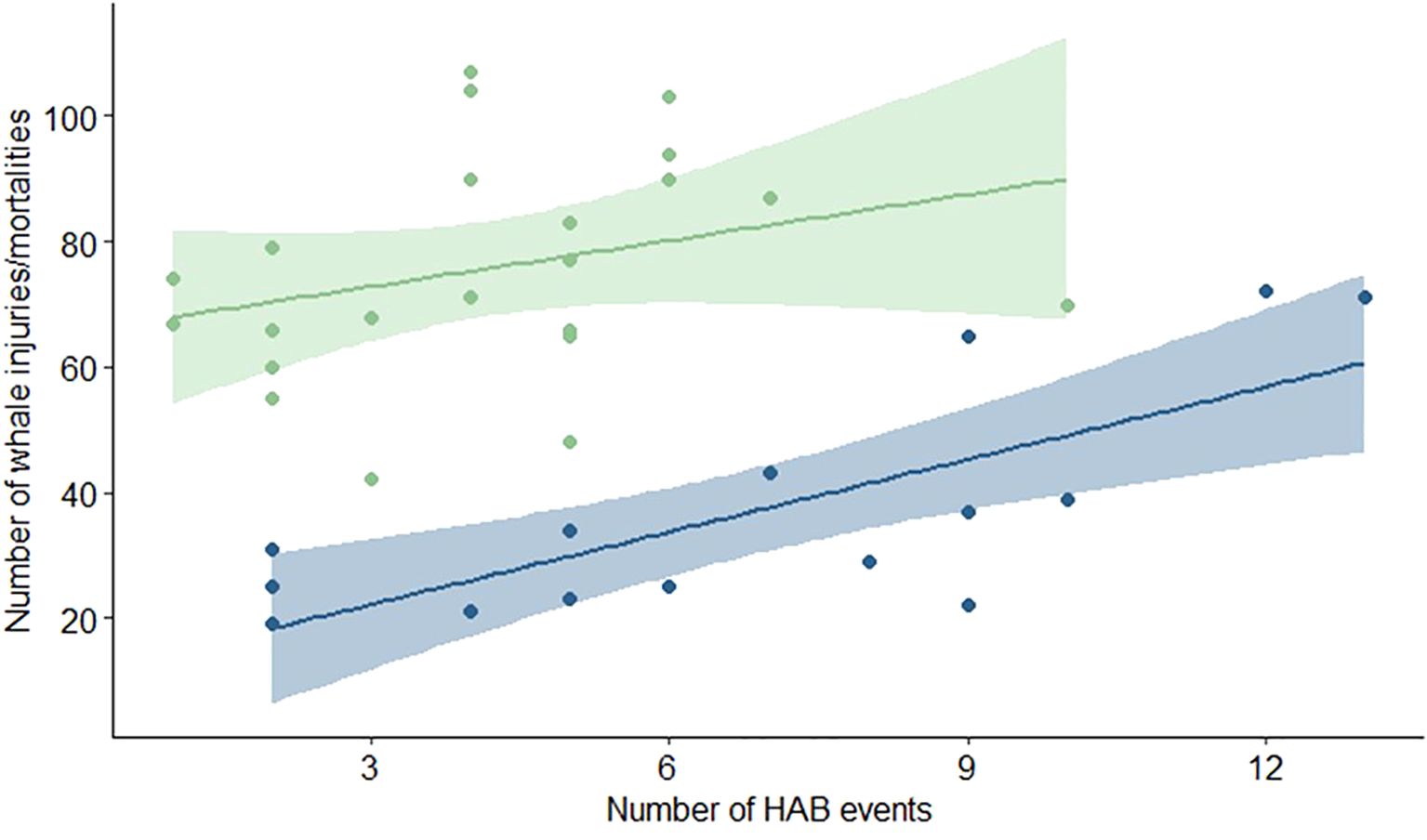
Figure 2. Co-occurrence of harmful algal bloom (HAB) events and whale injury/deaths. Linear regressions for the number of HAB events and large whale injuries/deaths per year are shown for U.S. west (in blue) and east coasts (in green). The relationship between the annual number of HABs and whale deaths/injuries is statistically significant (p = 0.002) for U.S. west coast waters, but not significant for the U.S. east coast.
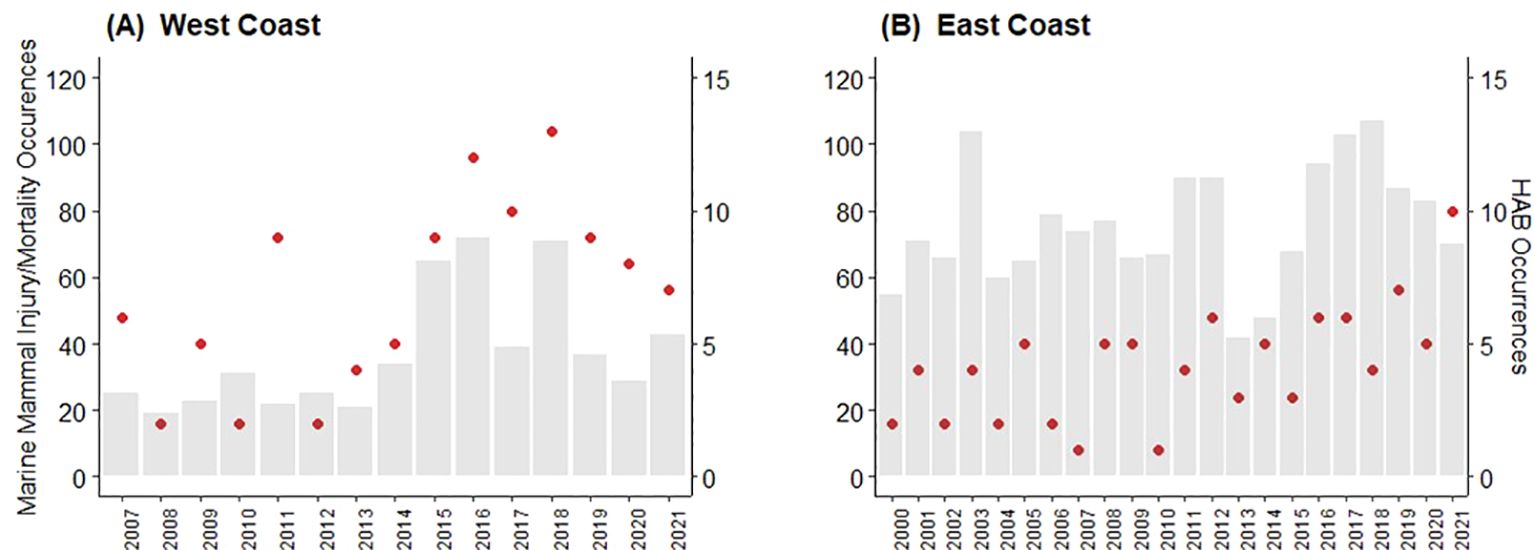
Figure 3. Number of reported harmful algal blooms (HAB) per year (gray bars) and frequency of reported large whale deaths and injuries (red circles) for waters off the U.S. west [(A) 2007-2021] and east [(B) 2000-2021] coasts.
On average, about two human-caused whale injuries/deaths occurred within 10 miles of an active HAB in west coast waters (Figure 4). This number decreased with time and distance; only about one injury/death occurred within 200 miles of an active west coast HAB and almost no whale injuries/deaths occurred after 10 days of an active west coast HAB. On the east coast, patterns are more ambiguous, likely due to the manner in which whale injury/mortality data were collected there (data are collected distant from shore in some locations) and the spatial extents of HAB and whale monitoring efforts poorly align spatially (see Methods). More whale injuries/deaths occurred within 200 miles of an active HAB than within 10 miles of an active HAB, and almost no injuries/deaths occurred after 10 days of an active HAB (Figure 4).
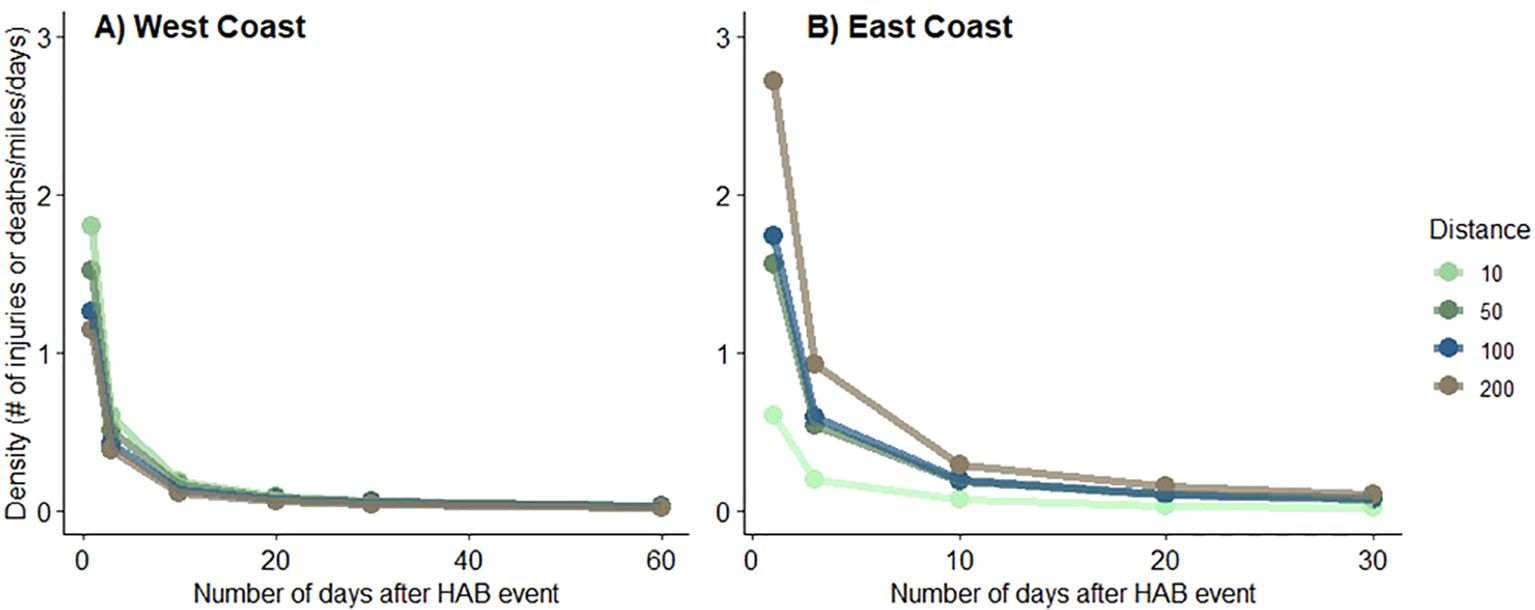
Figure 4. Average density of whale injuries and deaths in waters off the U.S. west [(A) 2007-2021] and east [(B) 2000-2021] coasts during and after HABs (days) and across four distances (miles).
Reported whale deaths and mortalities were coincident with only five toxic algal species on the U.S. east coast (Table 4) and only four algal species on the west coast (Table 5). Most injuries/mortalities were coincident – occurring in the vicinity (at ranges of 10-100 miles) – with Alexandrium and Pseudo-nitzschia blooms (Tables 4, 5).
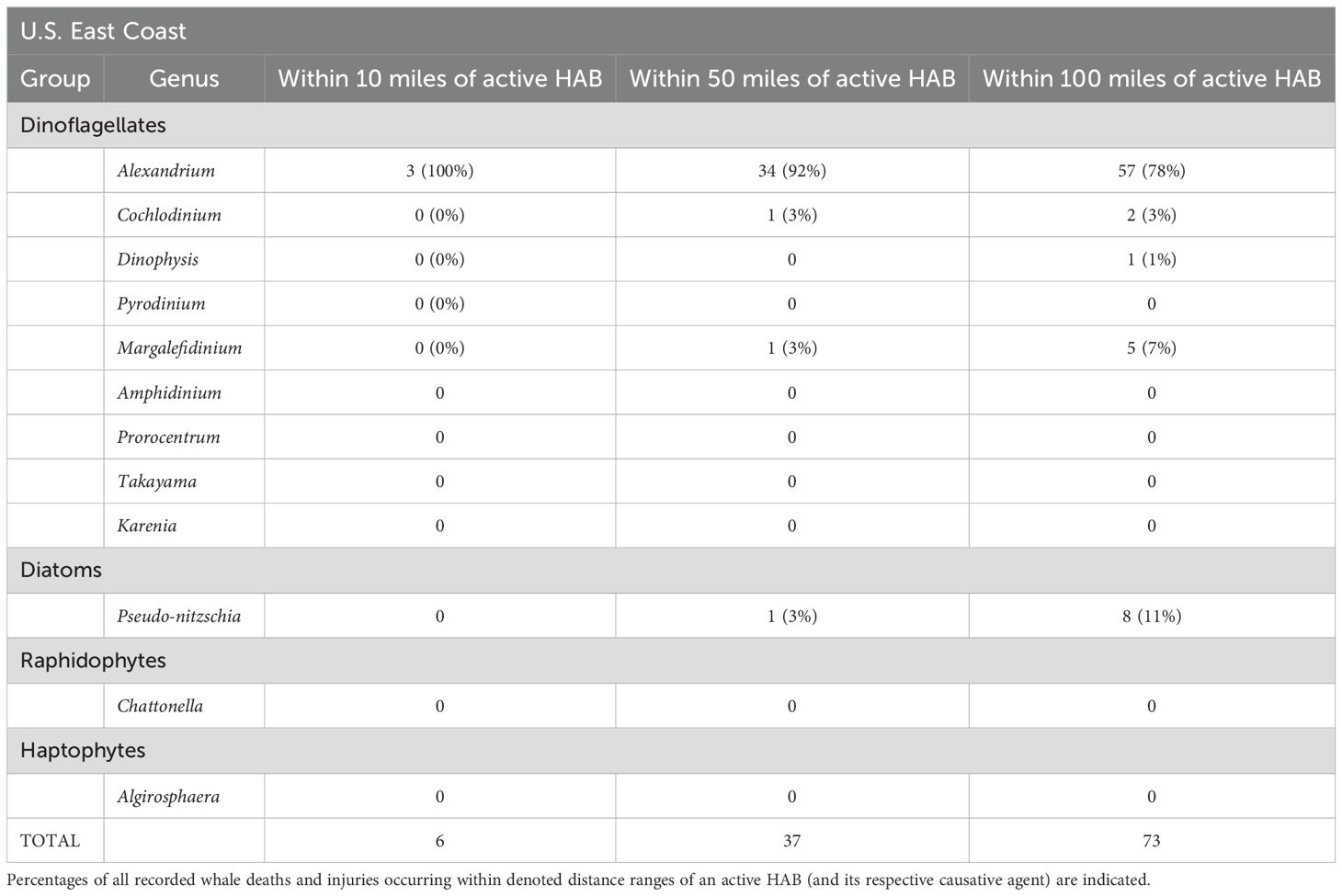
Table 4. Occurrence of whale mortalities and injuries within 10, 50 and 100 miles of U.S. east coast harmful algal blooms (HAB).
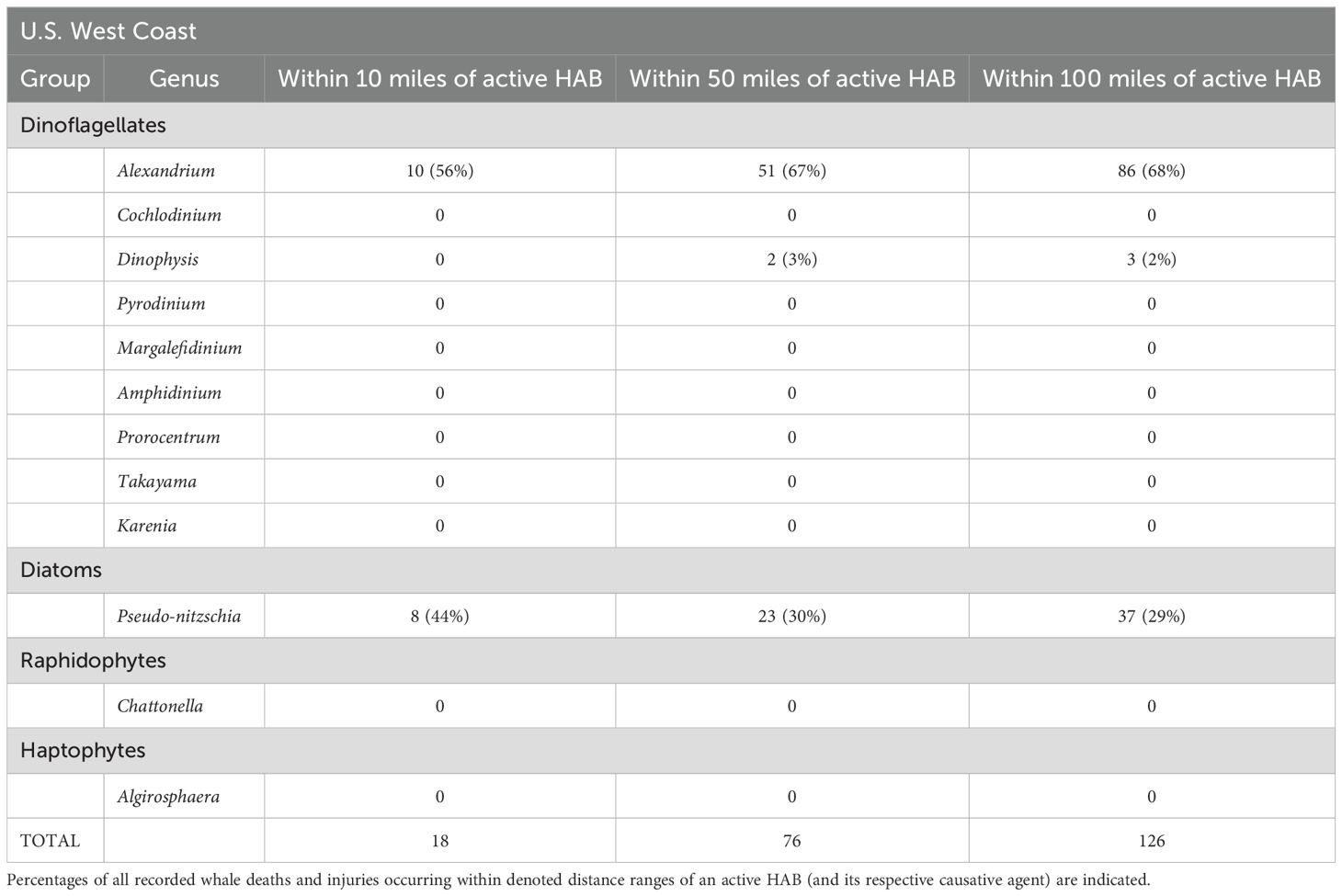
Table 5. Occurrence of whale mortalities and injuries within 10, 50 and 100 miles of U.S. west coast harmful algal blooms (HAB).
The number of HAB events was highly variable between years, a feature common to the waters of both coasts (Figure 2). Nonetheless, the rate of reported HAB occurrence increased in waters of both the east () and west coasts () of the United States in the respective periods of our study (Figure 5). While the rates of increase were statistically significant for data from both coasts, we found that the increase was more rapid on the U.S. west coast than on the east coast; roughly five additional reported HABs per decade on the west coast and about two additional HABs per decade on the east coast. Seasonally, the occurrence of both HABs and whale mortalities/injuries were generally highest in spring to fall (i.e., April-September) (Tables 6, 7).
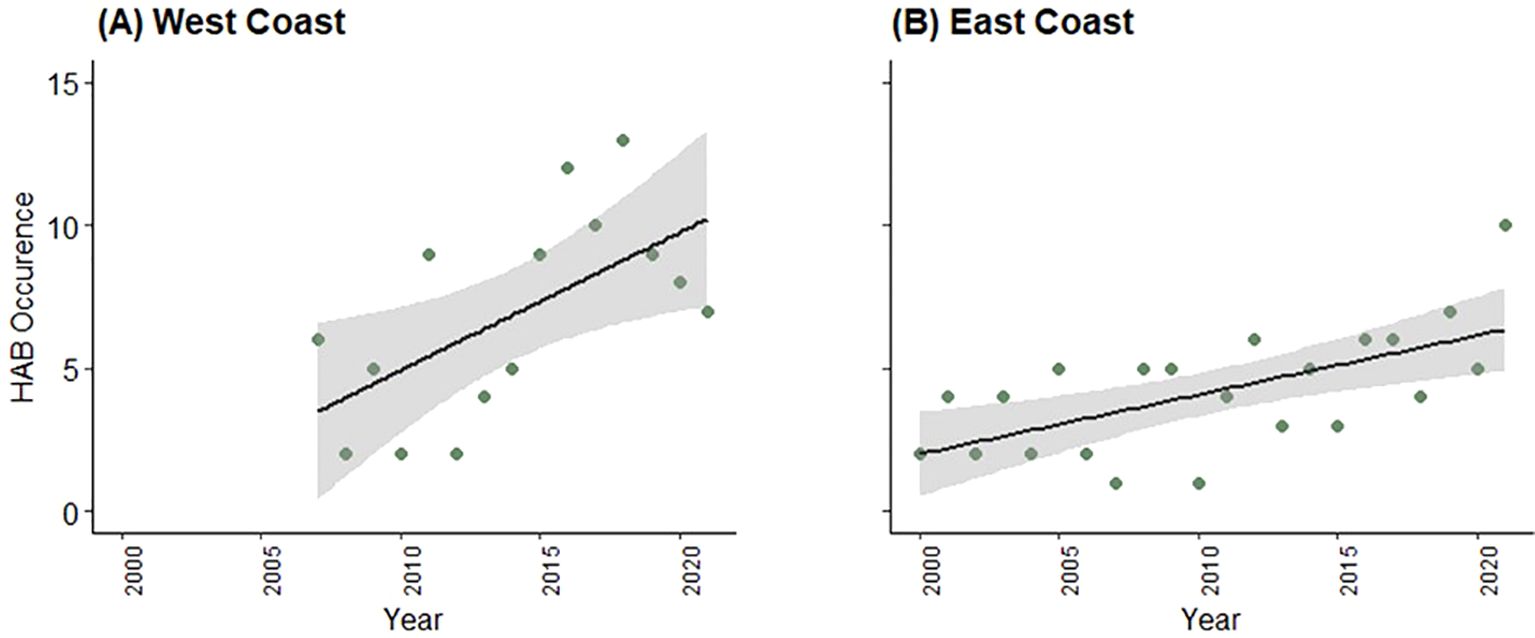
Figure 5. Rates of increase in harmful algal blooms (HAB) (green circles) in U.S. west coast waters, 2007-2021 (A) and U.S. east coast waters, 2000-2021 (B). Black lines represent predictions from linear model (HAB occurrence ~ year), and gray shaded area indicates 95% CIs.
While there was substantial interannual variation, there also appeared to be a general increase in the number of human-caused whale mortalities/injuries during the respective study periods (Figure 2). In addition, in some years in which large numbers of (or severe) HABs were reported, whale mortalities/injuries were also high. Among these were, notably, 2016 and 2018 for the U.S. west coast whale data. In U.S. east coast waters both the number of HABs and whale death/injury records were among the highest in the data sets for the years 2012, 2016, 2017, 2019, and 2020 (Tables 6, 7). However, this pattern did not consistently hold for all years, i.e., years in which large numbers of HABs were reported were not necessarily accompanied by high numbers of whale mortality and injury events, or vice versa (e.g., west coast, 2011; east coast 2008) (Figure 2). These co-occurrences are discussed more fully in Section 4.7, below.
4 Discussion
Our analysis provides further evidence that toxic algal blooms of varying scales and severity are increasingly common in U.S. coastal waters. As such, marine mammals using these habitats are likely routinely exposed to HAB toxins, incurring a higher risk of mortality through direct exposure and loss of neurological function. The established co-occurrence of HABs and whale morbidity/mortality resulting from commercial fishing and shipping interactions suggests, but does not confirm, that non-fatal concentrations of algal neurotoxins may render whales more susceptible to injury from commercial activities by making them less able to respond to those activities. Additional work is needed to further elucidate the relative importance of this relationship compared to direct relationships between HABs and marine mammal mortality, primarily through ongoing and systematic collection of HAB occurrence and severity data, and through additional and systematic tissue sampling from dead whales.
4.1 Direct and indirect effects of HABs on marine mammals
Events involving the deaths of scores of marine mammals have been attributed to exposure to HABs. Algal toxins have been implicated (but generally not fully established) in various large whale UMEs (https://smdp.com/2015/09/28/increase-whale-deaths-gulf-alaska/; https://repository.library.noaa.gov/view/noaa/17715), in the deaths of hundreds of California sea lions in multiple years in the last three decades 2015 (Scholin et al., 2000; Di Liberto, 2015; Anderson et al., 2021), and in other large-scale marine mammal mortality events (de la Riva et al., 2009). Yet, considering the extent and frequency of occurrences of HABs, marine mammal die-offs from exposure to HAB toxins appear to be remarkably rare events (Starr et al., 2017). Nonetheless, marine toxicologists continue to seek associations between HABs and marine mammal die-offs. Instead, we posit that debilitating consequences from sub-lethal concentrations of HAB toxins may be the source of indirect, and perhaps more serious population-level consequences for marine mammals when also exposed to existing threats such as commercial fishing and vessel operations.
To our knowledge, there are only two references (one now over two decades old) that alluded to associations between HAB agent toxicosis and increased vulnerability to human activities: Durbin et al. (2002) concluded that toxic algae ingestion by North Atlantic right whales could increase whale susceptibility to collisions with ships or fouling in fishing gear resulting primarily from diminished respiratory capabilities (and increased periods at the water’s surface); the other posited a possible link between reduced whale avoidance of vessels resulting from synergies or co-morbidities following toxic algal exposure (Savage, 2017). Understanding the role of toxic algal morbidity in marine mammals relative to human commercial activities may help provide a means to limit the negative impacts from those activities.
4.2 Impacts of principal causative agents
Alexandrium was the most common type of bloom, and overall, it may also be the type of bloom putting whales at greatest risk. Pseudo-nitzschia blooms were more prevalent in U.S. west coast (34% of all reported blooms) than east coast waters; only 8 blooms were reported (7% of all blooms) for east coast waters. However, 11% of whale deaths and injuries occurred<100 miles of active blooms involving this species in east coast waters, and up to 29% of all U.S. Pacific coast whale mortalities and injuries. Thus, while blooms involving Pseudo-nitzschia occurred less commonly than those involving Alexandrium, the former may also represent a potent agent involved in indirect effects on marine mammal populations.
4.3 Differences in east and west coast HAB and whale data sets
In U.S. west coast waters, large whale injuries and mortalities occurred in higher densities near an active HAB than in times and locations when HABs were not reported. However, this relationship is not apparent along the east coast. Inconsistent patterns between the two coasts may reflect differences in the coastal and nearshore biological oceanography and ecology. For example, the east coast has relatively shallower, warmer waters, which may foster disparate conditions for algal blooms. Patterns may also differ because of variance in algal species composition and diversity in the data sets from the two coasts.
In addition, differences between coasts may result from differences in the extent of marine mammal and/or HAB monitoring between the two coasts. Marine mammal surveys off the east coast are more numerous, systematic, and larger in spatial coverage (including farther from the shore) than in west coast waters. Furthermore, HAB monitoring near areas of human recreation and nearshore fisheries in numerous bays, estuaries, and near municipalities appears to have occurred at a greater extent along the eastern seaboard relative to the west. Systematically collected data across space and time could better support models that predict the overlap of whale and HAB occurrence and distribution and help shape management actions to reduce indirect marine mammal injuries and deaths. In addition, further research on this subject, and related subjects, would benefit from standardization of whale mortality data reporting and curation across the various stranding programs, and in providing records to central repositories that provide for transparency and are generally available for analysis.
4.4 Toxin pathways and whale natural histories
HAB toxins are capable of persisting in components of marine ecosystems and can be transmitted to cetaceans and other marine mammal species via prey items such as krill and finfish (Lefebvre et al., 1999). Schooling fish such as the Spanish mackerel (Scomberomorus spp.) have been identified as the vector for STX and was the prey responsible for a large-scale mortality event involving humpback whales, and researchers concluded that the toxin had been transported considerable distances by the mackerel (Geraci et al., 1989). The northern anchovy (Engraulis mordax) was identified as the primary trophic vector of DA in the death of a minke whale (Balaenoptera acutorostrata) in waters off California (Fire et al., 2010). Durbin et al. (2002) concluded that ingestion of PSP-ladened zooplankton was the source of neuropathology (including muscle paralysis) in North Atlantic right whales with the potential to adversely affect the whales’ respiratory capabilities and feeding behavior. Lefebvre et al. (2002a) found algal toxins in blue and humpback whale fecal material as well as in the krill and small bait fish they were consuming and concluded that DA can permeate pelagic as well as benthic communities.
It appears scores of marine mammal species – as indicated in diets, fecal samples and by other means – are capable of carrying chronic levels of HAB toxins with no clinical signs of acute neurotoxicity (e.g., Lefebvre et al., 2002a; Lefebvre et al., 2016); and toxicity, at levels that are not fatal, appear to be permanent or semi-permanent features of ecosystems (e.g., Danil et al., 2021; Hendrix et al., 2021). In a long-term study involving 23 species in waters off New England, marine mammals experienced significant and widespread exposure to HAB toxins, but little correlation was found between HABs and HAB-associated marine mammal mortality events (Fire et al., 2021). Nonetheless, of the 458 species sampled, 19% tested positive for either DA or STX, or both; humpback whales exhibited the highest rates of the presence of DA of all species tested; and DA was also most prevalent in whale and dolphin species bycaught in commercial fishing gear and in those sampled from waters distant from the coast. Similar findings were reported in a 2003 study involving Gulf of Maine and Georges Bank waters in which high concentrations of DA were found in water samples as well as dead whales (primarily humpback whales) (https://www2.whoi.edu/site/andersonlab/past-projects/pseudo-nitzschia-emerging-hab-threat-in-the-gulf-of-maine/). These findings appear to support the contention that marine mammals may tolerate low-level toxicities that (while not lethal) may be detrimental during interactions with human activities.
In our study, blooms involving DA were relatively infrequently reported for the U.S. east coast waters, making it noteworthy that DA has been found in animals sampled in offshore habitats and in bycaught individuals. East coast DA blooms may occur primarily far from shore, making them less likely to be sampled by coastal monitoring programs but at the same time having adverse effects on cetacean species feeding in offshore habitats.
In our study, the humpback whale was the species most often appearing in whale injury/mortality databases, comprising nearly half of all records for both coasts (Table 4). This may reflect, primarily, the abundance of this species relative to other baleen whale species – humpback whales are increasing in abundance, globally, and the species can be rather common in many parts of its range (Betteridge et al., 2015). Minke whale injuries/mortalities were likewise commonly represented (ca. 23% of the records) in the U.S. east coast data set (but much less frequently occurring in the west coast data). Humpback and minke whales are primarily piscivores, while less represented species (e.g., right and blue whales) in the database feed primarily at lower trophic levels (e.g., zooplankton). In feeding mostly on schooling fish species (although they also consume zooplankton), humpback and minke whales are likely exposed to higher levels of algal toxins through ecosystemic bioaccumulation than whales feeding at lower trophic levels ‘(e.g., blue and right whales; although gray whales are also likely exposed to high levels of bio-accumulated HAB toxin concentrations when feeding on bivalves and other benthic organisms), and this may account, at least in part, for their frequency of occurrence in the data sets.
Conditions leading to elevated internal toxin loads are likely dependent on numerous factors – the duration, scale, and severity of the bloom, the duration and extent of mammal exposure to the ocean-borne toxins, and the natural history of the mammals (if they are primarily piscivores, for example) primary among them. Oceanic and environmental conditions that drive HAB occurrences and trajectories in turn likely involve the interaction of multiple physical (e.g., upwelling) and chemical (e.g., introduced nutrients) oceanic processes. While marine mammals may routinely carry non-lethal toxin loads, symptoms of chronic toxicosis may be exacerbated by environmental perturbations, in response to additional or novel environmental stressors, or newly or already compromised immune systems.
4.5 Population-level consequences of threats
Abundances of most large whale populations in U.S. waters are steadily increasing (https://www.fisheries.noaa.gov/national/marine-mammal-protection/marine-mammal-stock-assessment-reports-species-stock), notably those of the humpback whale (Betteridge et al., 2015). Nonetheless, all large whale species continue to be negatively affected by entanglement in fishing gear (Reeves et al., 2013; Thomas et al., 2016) and ship strikes worldwide (Jensen and Silber 2003). Nearly all large whale species remain listed on the U.S. Endangered Species Act’s List of Endangered and Threatened Wildlife and Plants, and the rates of entanglement in commercial fishing gear and collisions with vessels remain significant challenges for large whale species recovery. For some populations, including the depleted and probably declining North Atlantic right whale, reducing interactions with human activities remain significant conservation challenges.
In the two data bases examined in this study alone, 556 U.S. west coast whale injuries and mortalities were recorded and 1,667 occurred along the U.S. east coast involving at least seven species (also, see, for example, Henry et al., 2022; Carretta et al., 2023). (note: in the analysis presented here, we removed records from this dataset in which human-interaction, i.e., ship strike or fishing gear entanglement, was not indicated.) Put another way, that is ca. 64 such incidents per year, or about (and at least) 5-6 per month for U.S. east and west coast continental margins. These records do not include, for example, whales that were struck or entangled, died at sea, yet their carcasses were not recovered, or their deaths otherwise not observed, or records that may exist elsewhere but are not, for various reasons, included in these datasets. Threats from those human activities may be substantially magnified if the sensorimotor capabilities of animals that encounter fishing gear or ships in their habitats are diminished or debilitated.
Given the scope and densities of commercial fishing gear (e.g., Moore et al., 2021; Richardson et al., 2022) and vessel traffic (e.g., Pirotta et al., 2019; Silber et al., 2021) in U.S. coastal waters – which now encompass staggeringly large areas of marine habitats – it is perhaps remarkable that entanglement and vessel strike rates are not far greater than reported as whales attempt to navigate these conditions.
Numerous factors likely play roles in whales’ negative interactions with fishing lines and gear or oncoming ships. Very little is known about faculties – if any – a whale might have to detect or avoid fishing gear in the water column or the approach of a vessel. Visual systems, for example, are of limited utility in low-light conditions or at depth; and the sound of oncoming vessels is almost certainly detected by a whale, but the animal may perceive the stimuli as benign until it is too late. Whales engaged in vital behavior such as feeding or mating may have little regard for threats such as a moving vessel or entangling gear. Nonetheless, even relatively rudimentary detection and avoidance strategies may be diminished by sensory, neurological, or motor disfunction thereby heightening vulnerability to threats from human activities – a situation that further confounds conservation efforts.
Toxic algal species have existed for tens of millions of years and were a part of marine ecosystems in which whales evolved. Perhaps, as noted, marine mammals can tolerate chronic low levels of toxins – and this may explain why large-scale die-offs are relatively rare considering the extent to which marine mammals are routinely exposed to the toxins. However, motorized ships and large-scale fishing enterprises are relatively new components of whale habitats and represent novel threats to which these animals are not adapted.
4.6 Coincidence of severe blooms and whale deaths & injuries
In multiple years, notably high levels of whale mortalities/injuries coincided with large-scale, persistent, or particularly potent HAB events. Occurrences of HABs and high levels of whale deaths/injuries did not align in all years. For example, although blooms of Pseudo-nitzschia in waters off southern California in 2007, 2011 and 2017 involved concentrations that were among the highest values reported in the literature (Smith et al., 2018) we found no evidence of elevated west coast whale deaths/injuries in those years (Tables 6, 7). Nonetheless, in the sections that follow, we describe several illustrative cases during which high levels of whale injuries/mortalities coincided with severe HAB events.
4.6.1 2015-2016 U.S. West coast bloom – Pseudo-nitzschia
In 2015-2016 an unprecedented Pseudo-nitzschia bloom extended from Alaska to southern California (Di Liberto, 2015; McCabe et al., 2016). From May 2015 to at least September 2015, DA concentrations were among the highest recorded to that time (McCabe et al., 2016). Harvest closures for numerous shellfish species were enacted from May-November 2015 at various locations in British Columbia, Washington, Oregon, and California (Ryan et al., 2017).
DA was detected in harbor seals (Phoca vitulina), Northern fur seals (Callorhinus ursinus), porpoises, dolphins, and whales in samples through late 2015 (Di Liberto, 2015) in an area that extended from the Pacific Northwest to southern California. In California over 200 sea lions died from exposure to Pseudo-nitzschia; an additional 83 sea lion deaths were reported in 2016. Large-scale seabird deaths occurred off California in the same period (Gibble et al., 2018). An ‘Unusual Mortality Event’ (UME) was designated for large whale species from May to December 2015 in the Gulf of Alaska, and from April 2015 to April 2016 in British Columbia (over 50 whales deaths, primarily fin and humpback whales, were reported) (https://www.fisheries.noaa.gov/national/marine-life-distress/2015-2016-large-whale-unusual-mortality-event-western-gulf-alaska). While the HAB was cited as a possible contributing factor in the large number of whale deaths, no definitive cause of the UME was identified (Bates et al., 2018).
In the large whale data set analyzed here, a total of 63 whale deaths/injuries were reported for the U.S. west coast in 2015, the third highest death/injury toll of all years considered in this study (2016 being the overall highest at 71 deaths/injuries; nearly one-quarter of all reported U.S. west coast whale deaths/injuries occurred in 2015 and 2016; Table 6). In May-November 2015 alone, at the height of the bloom, 46 whale deaths/injuries resulting from human interactions were reported in Washington, Oregon, and California, namely vessel strikes (n=3) and gillnet, pot/trap, or unidentified fisheries entanglements (n=43).
4.6.2 2016-2019 East coast blooms – Pseudo-nitzschia
In the fall/winter of 2016-2017 an anomalous bloom of Pseudo-nitzschia resulted in the closure of hundreds of miles of Maine coastline to shellfish harvests – thus becoming the first regional (i.e., Gulf of Maine) DA-induced shellfishery closure (Clark et al., 2019). In September 2016, nearly 30 tons of commercially harvested shellfish were recalled by the state of Maine (Lewis et al., 2017; https://www.bigelow.org/news/articles/2018-09-19.html), and the Canadian Food and Inspection Agency prohibited shellfish harvesting in swaths of the Bay of Fundy (Canada) in October 2016 (Bates et al., 2018). In its entry in the HAB data base, Maine’s Department of Marine Resources reported that although ‘low levels of DA had been detected [in this region] in the past … toxicity of this scale had never before been recorded.’ This bloom apparently began in the Bay of Fundy and progressed southwestward along the coasts of Maine, Massachusetts, and Rhode Island; consequently, precautionary closures were implemented in significant portions of Massachusetts and Rhode Island state waters in October 2016 (Sterling et al., 2022). Bloom toxicity had a rapid onset, with shellfish rapidly accumulating 3-4 times the DA closure limit in (https://www.bigelow.org/news/articles/2018-09-19.html). The blooms were apparently fueled by an influx of Scotian Shelf water (Clark et al., 2021), suggesting its toxic effects may have been widespread with oceanic origins. Collectively, these waters are also key summer/fall feeding habitats for humpback and right whales, among other species.
Further, recurring patterns began to emerge. Anomalously high DA levels were detected in waters off Rhode Island in the summers/falls of 2017, 2018 and 2019, including a particularly concerning toxic species, P. australis (Sterling et al., 2022); high DA levels in spring 2017 again closed shellfish harvests in Narragansett Bay, Rhode Island. In 2017, the first Karenia mikimotoi bloom was identified in Maine waters, and a rare late fall Pseudo-nitzschia bloom closed Casco Bay, Maine to shellfish harvests (https://www.bigelow.org/news/articles/2018-09-19.html). Shellfish closures were re-established in fall 2017 through winter 2018 in eastern and western Maine (Bates et al., 2018; http://www.pressherald.com/2017/09/14/algae-bloom-forces-suspension-of-shellfishing-in542parts-of-down-east-maine/), and again in the falls of 2018 and 2019 (Clark et al., 2021).
From January 2016 to the present, elevated humpback whale mortalities have occurred along the Atlantic coast from Maine through Florida (https://www.fisheries.noaa.gov/national/marine-life-distress/2016-2024-humpback-whale-unusual-mortality-event-along-atlantic-coast). In April 2017 a humpback whale UME was designated for the U.S. east coast for the period beginning in January 2016 (https://www.fisheries.noaa.gov/national/marine-life-distress/2016-2024-humpback-whale-unusual-mortality-event-along-atlantic-coast). The UME is ongoing. While, to date, the cause(s) of the UME have not been (officially) determined, of those whales for which partial or full necropsy examinations were conducted (a total of about 90 individuals) 40% exhibited evidence of human interaction, meaning either ship strike or entanglement mortality.
The whale mortality/injury database indicates that 103, 106, and 119 whale deaths and injuries were recorded along the U.S. east coast in 2016, 2017, and 2018, respectively – among the highest numbers for any year in the sample (Figures 2, 4; Table 7). A cluster of unusually high number of whale death/injuries occurred in August-October in 2017, 2018, and 2019, including the highest number in the entire database in August and September 2018 (Table 7). The 19 whale deaths and injuries in October 2017 were the highest for that month in the entire sample and was 3-times higher than the 22-year monthly average for that month (Table 7).
4.6.3 2018 U.S. West coast bloom – Alexandrium
While apparently receiving far less attention in the literature than Pseudo-nitzschia blooms described above, the HAB data records documented a geographically extensive Alexandrium bloom that occurred January to August 2018 in California waters. The bloom initially developed off central California, occurred subsequently in northern California waters, and was then detected in southern California. Numerous records indicate that toxicity levels far exceeded toxicity threshold standards, in some cases by greater than one order of magnitude.
Whale mortalities and injuries (n=70) on the U.S. west coast in 2018 were the second highest of all years in our sample (Figures 2, 3). The monthly average (5.8 whale deaths and injuries per month) for the year was nearly two-fold greater than the monthly average (X=3.0) in the 15-year period for which data were available; and the highest monthly total number of whale injuries/mortalities in the entire sample occurred in May of that year (Figure 3; Tables 6, 7). Most (n=55) 2018 whale injuries/deaths were attributed to gillnet and pot fisheries interactions (followed by vessel strikes, n=14, and marine debris, n=1); and most of these (n=50) occurred in California waters.
4.6.4 2003 U.S. East coast bloom – Alexandrium and Pseudo-nitzschia
Records in the HAB database documented high levels of Alexandrium in spring through fall 2003 (in some areas detected as early in the year as January) in New England waters. Numerically, reported HABs were moderate (i.e., only four blooms were reported in 2003 (all involving Alexandrium). However, the geographic extent and the severity of the bloom were noteworthy: extending from Massachusetts Bay, including Cape Cod and the North Shore (Massachusetts), to western and eastern Maine.
In 2003, STX concentrations sampled from shellfish tissue were some of the highest indicated in the database; in some instances, levels were many-fold greater than prescribed ‘alert’ levels for human consumption. Multi-state shellfish harvest closures were established as a result. Most shellfish closures were relaxed in the region by fall 2003, but one record indicated that the Maine offshore quahog (Mercenaria mercenaria) fishery remained partially closed into 2004.
The U.S. east coast whale database contains 105 records of whale injuries and deaths for the year 2003, among the highest for all years represented in the sample (Tables 7). In cases in which source of the interaction was known (those constituting 38% of those reported in 2003), fishing gear entanglements accounted for 35 instances and ship strikes for five of them. In May-September alone, 68 whale mortalities/injuries were reported (Tables 7). Of these, over 20 whales (mostly humpback whales) died near Georges Bank (a large area offshore and east of Massachusetts) and off the Maine coast in July 2003 (https://www2.whoi.edu/site/andersonlab/past-projects/pseudo-nitzschia-emerging-hab-threat-in-the-gulf-of-maine/). Plankton sampling in those same waters indicated the presence of Pseudo-nitzschia spp., and domoic acid was detected in most of the whales sampled, some exhibiting high concentrations (https://www2.whoi.edu/site/andersonlab/past-projects/pseudo-nitzschia-emerging-hab-threat-in-the-gulf-of-maine/).
4.7 Increasing occurrence of HABs
Numerous studies have indicated that the HAB frequency, duration, and severity are increasing on national (e.g., Anderson et al., 2021) and global scales (e.g., Van Dolah, 2000; Anderson et al. 2012; IWC, 2017; Broadwater et al., 2018); and these rates have often been attributed to rising ocean temperatures (e.g., Hinder et al., 2012; IWC, 2017), acidification, and nutrient pollution, among other things (e.g., Gobler, 2020; Anderson et al., 2021). Our analysis, too, indicated (apparent) generally increasing trends in HAB occurrence in the last two or so decades (Figure 5); but we acknowledge this may reflect increased monitoring efforts. Yet, it is possible that perceived increases in HAB occurrence may reflect, at least in part, increased awareness about the detriments of HABs on public health and a subsequent increase in monitoring and reporting efforts (e.g., Sellner et al., 2003; Hallegraeff, 2010; Hallegraeff et al., 2021).
However, increased monitoring efforts would not account for apparent interannual variability in the occurrence of HABs (Figure 3). Between year variability, instead, suggests that complex oceanographic processes, such as variation in physical (e.g., upwelling, water temperatures) and chemical (e.g., nutrient levels) conditions, have important roles in inducing HABs. Some of these processes have been identified (e.g., McCabe et al., 2016; Sandoval-Belmar et al., 2023) but a full discussion of these factors remains beyond the scope of our study. If, however, HAB frequency is in fact rising as related to warming temperatures and other (human-induced) factors, greater direct and indirect marine mammal vulnerability to HAB events might be expected. For example, shifts in distributions of marine mammals and their prey in response to a changing climate (e.g., Silber et al., 2017; Hudak et al., 2023) or other environmental features (e.g., Santora et al., 2020) could result in increased threats to marine mammals from increased or novel exposure to fixed fishing gear or other threats in new aggregation locations (e.g., Santora et al., 2020; Ingman et al., 2021; Meyer-Gutbrod et al., 2023). These are subject areas for further research.
4.8 Management implications – the importance of HAB predictions to whale conservation
Environmental monitoring and various predictive modeling approaches are being used worldwide, and with increasing sophistication, to identify the interplay of conditions that are antecedent to blooms (e.g., Dabrowski et al., 2016; Glibert et al., 2018; Litchman, 2022; Sandoval-Belmar et al., 2023). Satellite imagery and other types of remote sensing are being employed on large spatial scales to monitor oceanographic features that can lead to harmful blooms (e.g., Stumpf, 2001; Kahn et al., 2021). Similarly, modeling exercises that predict large whale spatiotemporal occurrence are now established analytical practices in marine mammalogy (e.g., Roberts et al., 2016; Redfern et al., 2017). And the locations and spatial extent of human activities such as vessel traffic densities (e.g., Silber et al., 2021; Silber and Adams, 2019) and commercial fishing operations (derived, for example, from fishing regulation data, on-board observer data) can be quantified on various scales. Therefore, by coupling predictive analyses of the occurrence and distribution of HABs, large whale occurrence information, and human activities, whale vulnerability to threats from human maritime uses could be reduced. For example, fishing gear deployment could be temporarily limited (in geographic scope or effort) to minimize threats to large whale populations potentially exposed to harmful blooms. Any such modeling that seeks to characterize whale, human activity, and HAB distributional overlap would also need to account for possible shifts (especially those that are climate related) in the occurrence of whales or their prey (see, for example, Hare et al., 2010; Wright et al., 2023), anomalous warm water masses (e.g., Santora et al., 2020), and other types of oceanographic variability.
Ample precedent exists for management actions that curtail, relocate, or otherwise modify fishing operations (e.g. NOAA, 2021, 2023; Cole et al., 2021) and shipping activities (e.g., Silber et al., 2014; Rockwood et al., 2017) through policy actions or rulemaking to limit whale entanglement and ship strikes. It may be possible to modify or expand such efforts to reduce whale mortality by anticipating HAB events, thereby enhancing whale conservation.
5 Conclusions
We posit that sub-lethal concentrations of HAB toxins increase marine mammal vulnerability to some human activities. We found compelling, but not necessarily definitive, evidence that exposure (possibly even at chronic low levels) to HAB toxins can increase the chances of large whale injury and death from interactions with moving vessels and commercial fishing gear. Evolutionarily, large whales are not well-equipped to detect and avoid such hazards and faculties they may have – if any at all – to avoid such hazards may be compromised by symptoms associated with HAB toxins. Increasing capabilities to forecast the occurrence of HABs based on knowledge of oceanic conditions that prompt large-scale or severe blooms may likewise provide an ability to predict the occurrence of fatal interactions with human activities. Predicting bloom occurrence could be a useful tool in developing whale conservation measures, such as curbing or moving vessel or fishing operations to alternative locations or times when and where blooms are expected to occur. Additional work is needed to further determine if whale mortalities/injuries are associated with the blooms – and at scales with finer resolution than those used in our study. Additional work is also needed to determine if HAB predictive and forecast modeling approaches can also be used to reduce the impacts of human commercial activities to large whale population recovery. If so, such an approach could become a potent conservation tool.
Data availability statement
Publicly available datasets were analyzed in this study. This data can be found here: Harmful Algal Event Database (HAEDAT), UNESCO Intergovernmental Oceanographic Commission (HAEDAT; http://haedat.iode.org/); whale mortality data: https://www.fisheries.noaa.gov/national/marine-mammal-protection/marine-mammal-stock-assessment-reports-species-stock#cetaceans—large-whales.
Author contributions
GS: Writing – review & editing, Writing – original draft, Methodology, Investigation, Data curation, Conceptualization. KS: Writing – review & editing, Writing – original draft, Methodology, Investigation, Formal analysis, Data curation.
Funding
The author(s) declare that no financial support was received for the research, authorship, and/or publication of this article.
Acknowledgments
U.S. NOAA Fisheries’ Allison Henry graciously provided a comprehensive, carefully assembled, and vetted U.S. east coast whale death and injury database spreadsheet; and James V. Carretta graciously provided the same information for the U.S. west coast. We commend their dedication to their work. We are grateful to all those who consistently and tirelessly gather data on algal blooms and whale strandings.
Conflict of interest
The authors declare that the research was conducted in the absence of any commercial or financial relationships that could be construed as a potential conflict of interest.
Publisher’s note
All claims expressed in this article are solely those of the authors and do not necessarily represent those of their affiliated organizations, or those of the publisher, the editors and the reviewers. Any product that may be evaluated in this article, or claim that may be made by its manufacturer, is not guaranteed or endorsed by the publisher.
Footnotes
- ^ While criteria were developed for inclusion of HAB records for in the analysis in this study, they were not dissimilar from those used by Anderson et al. (2021) in a study of past and current HABs, namely the bloom was to have been ‘…associated with a negative impact or management action. These could include: 1) toxin accumulation in seafood above levels considered safe for human consumption; 2) water discoloration, scum or foam sufficient to cause ecosystem damage or a socioeconomic impact; 3) any event where humans, animals or other organisms are negatively affected by the bloom; or 4) precautionary closures of harvesting areas based on predefined thresholds of toxic phytoplankton cells in the water.’
References
Adams N. G., Lesoing M., Trainer V. L. (2000). Environmental conditions associated with domoic acid in razor clams on the Washington coast. J. Shellfish Res. 19, 1007–1015.
Anderson D. M., Cembella A. D., Hallegraeff G. M. (2012). Progress in understanding harmful algal blooms: paradigm shifts and new technologies for research, monitoring, and management. Annu. Rev. Mar. Sci. 4, 143–176. doi: 10.1146/annurev-marine-120308-081121
Anderson D. M., Fensin E., Gobler C. J., Hoeglund A. E., Hubbard K. A., Kulis D. M., et al. (2021). Marine harmful algal blooms (HABs) in the United States: History, current status and future trends. Harmful Algae. 102, 101975. doi: 10.1016/j.hal.2021.101975
Anderson D. M., Kulis D. M., Sullivan J. J., Hall S., Lee C. (1990). Dynamics and physiology of saxitoxin production by the dinoflagellates Alexandrium spp. Mar. Biol. 104, 511–524. doi: 10.1007/BF01314358
Bates S. S., Hubbard K. A., Lundholm N., Montresor M., Leaw C. P. (2018). Pseudo-nitzschia, Nitzschia, and domoic acid: New research since 2011. Harmful Algae 79, 3–43. doi: 10.1016/j.hal.2018.06.001
Bettridge S., Baker C. S., Barlow J., Clapham P. J., Ford M., Gouveia D., et al. (2015). Status review of the humpback whale (Megaptera novaeangliae) under the Endangered Species Act. US Dept of Commer NOAA Technical Memorandum. NMFS; NOAA-TM-NMFS-SWFSC-540. Available at: https://repository.library.noaa.gov/view/noaa/4883/noaa_4883_DS1.pdf.
Bossart G. D., Baden D. G., Ewing R. Y., Roberts B., Wright S. D. (1998). Brevetoxicosis in manatees (Trichechus manatus latirostris) from the 1996 epizootic: gross, histologic, and immunohistochemical features. Toxicological Pathol. 26, 276–282. doi: 10.1177/019262339802600214
Broadwater M. H., Van Dolah F. M., Fire S. E. (2018). “Vulnerabilities of marine mammals to harmful algal blooms,” in Harmful algal blooms, vol. 8 . Eds. Shumway S. E., Burkholder J. M., Morton S. L. (John Wiley & Sons, Ltd, Hoboken, NJ), 191–222. doi: 10.1002/9781118994672.ch5
Capper A., Flewelling L. J., Arthur K. (2013). Dietary exposure to harmful algal bloom (HAB) toxins in the endangered manatee (Trichechus manatus latirostris) and green sea turtle (Chelonia mydas) in Florida, USA. Harmful Algae. 28, 1–9. doi: 10.1016/j.hal.2013.04.009
Carretta J. V., Oleson E. M., Forney K. A., Weller D. W., Lang A. R., Baker J., et al. (2023). U.S. Pacific marine mammal stock assessments: 2022. U.S. Department of Commerce, NOAA Technical Memorandum NMFS-SWFSC-684. (La Jolla, CA: NMFS Southwest Fisheries Science Center). doi: 10.25923/5ysf-gt95
Chou H. N., Huang C., P and Chen C. Y. (2005). Accumulation and depuration of paralytic shellfish poisoning toxins by laboratory cultured purple clam Hiatula diphos Linnaeus. Toxicon 46 5, 587–590. doi: 10.1016/j.toxicon.2005.07.010
Clark S., Hubbard K. A., Anderson D. M., McGillicuddy D. J. J., Ralston D. K., Townsen D. W. (2019). Pseudo-nitzschia bloom dynamics in the Gulf of Maine: 2012 – 2016. Harmful Algae 88, 101656. doi: 10.1016/j.hal.2019.101656
Clark S., Hubbard K. A., McGillicuddy D. J. Jr., Ralston D. K., Shankar S. (2021). Investigating Pseudo-nitzschia australis introduction to the Gulf of Maine with observations and models. Cont Shelf Res. 228, 104493. doi: 10.1016/j.csr.2021.104493
Cole A. K., Brillant S. W., Boudreau S. A. (2021). Effects of time-area closures on the distribution of snow crab fishing effort with respect to entanglement threat to North Atlantic right whales. ICES J. Mar. Sci. 78, 2109–2119. doi: 10.1093/icesjms/fsab103
Cook P. F., Reichmuth C., Rouse A., Dennison S., Van Bonn B., Gulland F. (2016). Natural exposure to domoic acid causes behavioral perseveration in wild sea lions: Neural underpinnings and diagnostic application. Neurotoxicol Teratol. 57, 95–105. doi: 10.1016/j.ntt.2016.08.001
Cook P. F., Reichmuth C., Rouse A. A., Libby L. A., Dennison S. E., Carmichael O. T., et al. (2015). Algal toxin impairs sea lion memory and hippocampal connectivity, with implications for strandings. Science. 350, 1545–1547. doi: 10.1126/science.aac5675
Dabrowski T., Lyons K., Nolan G., Berry A., Cusack C., Silke J. (2016). Harmful algal bloom forecast system for SW Ireland. Part I: Description and validation of an operational forecasting model. Harmful Algae 53, 64–76. doi: 10.1016/j.hal.2015.11.015
Danil K., Berman M., Frame E., Preti A., Fire S. E., Leighfield T., et al. (2021). Marine algal toxins and their vectors in southern California cetaceans. Harmful Algae 103, 102000. doi: 10.1016/j.hal.2021.102000
de la Riva G. T., Johnson C. K., Gulland F. M., Langlois G. W., Heyning J. E., Rowles T. K., et al. (2009). Association of an unusual marine mammal mortality event with Pseudo-nitzschia spp. blooms along the southern California coastline. J. Wildl Dis. 45, 1, 109–121. doi: 10.7589/0090-3558-45.1.109
Di Liberto T. (2015). California closures Dungeness and razor clam fisheries due to algal toxin. NOAA News. Available at: https://www.climate.gov/news-features/event-tracker/california-closes-dungeness-and-razor-clam-fisheries-due-algal-toxin.
Durbin E. E., Teegarden G., Campbell R., Cembella A., Baumgartner M. F., Mate B. R. (2002). North Atlantic right whales, Eubalaena glacialis, exposed to paralytic shellfish poisoning (PSP) toxins via a zooplankton vector, Calanus finmarchicus. Harmful Algae 1, 243–251. doi: 10.1016/S1568-9883(02)00046-X
Erbe C., Smith J. N., Redfern J. V., Peel D. (2020). Editorial: Impacts of shipping on marine fauna. Front. Mar. Sci. 7. doi: 10.3389/fmars.2020.00637
Fire S. E., Bogomolni A., DiGiovanni R. A. Jr., Early G., Leighfield T. A., Matassa K., et al. (2021). An assessment of temporal, spatial and taxonomic trends in harmful algal toxin exposure in stranded marine mammals from the U.S. New England coast. PloS One 16, e0243570. doi: 10.1371/journal.pone.0243570
Fire S. E., Van Dolah F. M. (2012). “Marine biotoxins: emergence of harmful algal blooms as health threats to marine wildlife,” in New directions in conservation medicine: applied cases in ecological health. Eds. Aguirre A. A., Ostfield R. S., Daszak P. (Oxford University Press, New York), 374–389. Available at: https://core.ac.uk/download/pdf/77945422.pdf.
Fire S., Wang Z., Berman M., Langlois G. W., Morton S. L., Sekula-Wood E., et al. (2010). Trophic transfer of the harmful algal toxin domoic acid as a cause of death in a minke whale (Balaenoptera acutorostrata) stranding in southern California. Aquat Mamm 36, 342–350. doi: 10.1578/AM.36.4.2010.342
Flewelling L. J., Naar J. P., Abbott J. P., Baden D. G., Barros N. B., Bossart G. D., et al. (2005). Brevetoxicosis: red tides and marine mammal mortalities. Nature. 435, 755–756. doi: 10.1038/nature435755a
Geraci J. R., Anderson D. M., Timperi R. J., St. Aubin D. J., Early G. A., Prescott J. H., et al. (1989). Humpback whales (Megaptera novaeangliae) fatally poisoned by Dinoflagellate toxin. Can. Aquat. Sci. 46, 1895–1898. doi: 10.1139/f89-238
Gibble C., Duerr R., Bodenstein B., Lindquist K., Lindsey J., Beck J., et al. (2018). Investigation of a largescale Common Murre (Uria aalge) mortality event in California, USA, in 2015. J. Wildl Dis. 54, 569–574. doi: 10.7589/2017-07-179
Gibble C. M., Kudela R. M., Knowles S., Bodenstein B., Lefebvre K. A. (2021). Domoic acid and saxitoxin in seabirds in the United States between 2007 and 2018. Harmful Algae. 103, 101981. doi: 10.1016/j.hal.2021.101981
Glibert P. M., Berdalet E., Burford M. A., Pitcher G. C., Zhou M. (2018). “Advancements and continuing challenges of emerging technologies and tools for detecting harmful algal blooms, their antecedent conditions and toxins, and applications in predictive models,” in Global ecology and oceanography of harmful algal blooms. Ecological studies (Analysis and synthesis). Ed. Glibert P., et al (Springer, Cham).
Gobler C. J. (2020). Climate change and harmful algal blooms: insights and perspective. Harmful Algae. 91 January 2020, 101731. doi: 10.1016/j.hal.2019.101731
Goldstein T., Mazet J. A., Zabka T. S., Langlois G., Colegrove K. M., Silver M., et al. (2008). Novel symptomatology and changing epidemiology of domoic acid toxicosis in California sea lions (Zalophus californianus): an increasing risk to marine mammal health. Proc. Biol. Sci. 275, 267–276. doi: 10.1098/rspb.2007.1221
Hallegraeff G. M. (2010). Ocean climate change, phytoplankton community responses, and harmful algal blooms: a formidable predictive challenge. J. Phycol 46, 220–235. doi: 10.1111/j.1529-8817.2010.00815.x
Hallegraeff G. M., Anderson D. M., Belin C., Bottein M. Y. D., Bresnan E., Chinain M., et al. (2021). Perceived global increase in algal blooms is attributable to intensified monitoring and emerging bloom impacts. Commun. Earth Environ. 2, 117. doi: 10.1038/s43247-021-00178-8
Hare J. A., Alexander M., Fogarty M., Williams E., Scott J. (2010). Forecasting the dynamics of a coastal fishery species using a coupled climate-population model. Ecol. App 20, 452–464. doi: 10.1890/08-1863.1
Häussermann V., Gutstein C. S., Beddington M., Cassis D., Olavarria C., Dale A. C., et al. (2017). Largest baleen whale mass mortality during strong El Niño event is likely related to harmful toxic algal bloom. PeerJ 5, e3123. doi: 10.7717/peerj.3123
Hendrix A. M., Lefebvre K. A., Quakenbush L., Bryan A., Stimmelmayr R., Sheffield G., et al. (2021). Ice seals as sentinels for algal toxin presence in the Pacific Arctic and subarctic marine ecosystems. Mar. Mamm Sci. 37, 1292–1308. doi: 10.1111/mms.12822
Henry A., Smith A., Garron M., Morin D., Reid A., Ledwell W., et al. (2022). Serious injury and mortality determinations for baleen whale stocks along the Gulf of Mexico, United States East Coast, and Atlantic Canadian Provinces 2016-2020. US Dept Commer Northeast Fish Sci Cent Ref Doc. 22-13, (Woods Hole, MA USA: NMFS Northeast Fisheries Science Center) Vol. 61. doi: 10.25923/7a57-9d36
Hinder S. L., Hays G. C., Edwards M., Roberts E. C., Walne A. W., Gravenor M. B. (2012). Changes in marine dinoflagellate and diatom abundance under climate change. Nature Clim Change 2, 271–275. doi: 10.1038/nclimate1388
Hudak C. A., Stamieszkin K., Mayo C. A. (2023). North Atlantic right whale Eubalaena glacialis prey selection in Cape Cod Bay. Endang Species Res. 51, 15–29. doi: 10.3354/esr01240
Ingman K., Hines E., Mazzini P. L. F., Rockwood R. C., Nur N., Jahncke J. (2021). Modeling changes in baleen whale seasonal abundance, timing of migration, and environmental variables to explain the sudden rise in entanglements in California. PloS One 16 4, e0248557. doi: 10.1371/journal.pone.0248557
IWC (International Whaling Commission) (2017). Report of the workshop on harmful algal blooms (HABs) and associated toxins. 7-8 may 2017, BLED, Slovenia. SC/67a/rep09. Available online at: https://www.webapps.nwfsc.noaa.gov/assets/11/9142_03012019_100314_IWC2017_SC_67A_REP_09.pdf (accessed June 23, 2023).
Jensen A. S., Silber G. K. (2003). Large whale ship strike database. U.S. Department of commerce, NOAA technical memorandum, NMFS- F/OPR-25. Available online at: https://repository.library.noaa.gov/view/noaa/23127 (accessed January 25, 2024).
Khan R. M., Salehi B., Mahdianpari M., Mohammadimanesh F., Mountrakis G., Quackenbush L. J. (2021). A meta-analysis on harmful algal bloom (HAB) detection and monitoring: a remote sensing perspective. Remote Sens. 13, 4347. doi: 10.3390/rs13214347
Krogstad F. T. O., Griffith W. C., Vigoren E. M., Faustman E. M. (2009). Re-evaluating blue mussel depuration rates in ‘Dynamics of the phycotoxin domoic acid: accumulation and excretion in two commercially important bivalves’. J. Appl. Phycol. 21, 745–746. doi: 10.1007/s10811-009-9410-4
Landsberg J. H. (2002). The effects of harmful algal blooms on aquatic organisms. Rev. Fish Sci. 10, 2, 113–390. doi: 10.1080/20026491051695
Leblad B. R., Nhhala H., Daoudi M., Marhraoui M., Abdellah M. O., Véron B., et al. (2017). Contamination and depuration of Paralytic Shellfish Poisoning by Acanthocardia tuberculata cockles and Callista chione clams in Moroccan waters. J. Materials Env. Sci. 8, 4634–4641.
Lefebvre K. A., Bargu S., Kieckhefer T., Silver M. W. (2002a). From sanddabs to blue whales: The pervasiveness of domoic acid. Toxicon 40, 971–977. doi: 10.1016/S0041-0101(02)00093-4
Lefebvre K. A., Kendrick P. S., Ladiges W., Hiolski E. M., Ferriss B. E., Smith D. R., et al. (2017). Chronic low-level exposure to the common seafood toxin domoic acid causes cognitive deficits in mice. Harmful Algae 64, 20–29. doi: 10.1016/j.hal.2017.03.003
Lefebvre K. A., Powell C. L., Busman M., Doucette G. J., Moeller P. D., Silver J. B., et al. (1999). Detection of domoic acid in northern anchovies and California sea lions associated with an unusual mortality event. Nat. Toxins 7, 85–92. doi: 10.1002/(sici)1522-7189(199905/06)7:3<85::aid-nt39>3.0.co;2-q
Lefebvre K. A., Quakenbush L., Frame E., Huntington K. B., Sheffield G., Stimmelmayr R. (2016). Prevalence of algal toxins in Alaskan marine mammals foraging in a changing arctic and subarctic environment. Harmful Algae. 55, 13–24. doi: 10.1016/j.hal.2016.01.007
Lefebvre K. A., Robertson A. (2010). Domoic acid and human exposure risks: a review. Toxicon 15 56, 218–230. doi: 10.1016/j.toxicon.2009.05.034
Lefebvre K. A., Silver M. W., Coale S. L., Tjeerdema R. S. (2002b). Domoic acid in planktivorous fish in relation to toxic Pseudo-nitzschia cell densities. Mar. Biol. 140, 625–631. doi: 10.1007/s00227-001-0713-5
Lewis B., MacLeod J., Hubbard K. (2017). The Gulf of Maine domoic acid event of 2016: an emerging public health concern. 9th U.S. HAB Symposium. 11-17 November 2017, Baltimore, MD. Available online at: https://www.9thushab.com/ (accessed September 18, 2023).
Litchman E. (2022). Understanding and predicting harmful algal blooms in a changing climate: A trait-based framework. Limnology Oceanography Lett. (IF 8.507). 8, 229-246. doi: 10.1002/lol2.10294
McCabe R. M., Hickey B. M., Kudela R. M., Lefebvre K. A., Adams N. G., Bill B. D., et al. (2016). An unprecedented coastwide toxic algal bloom linked to anomalous ocean conditions. Geophys. Res. Lett. 43, 10, 366–10,376. doi: 10.1002/2016GL070023
McHuron E. A., Greig D. J., Colegrove K. M., Fleetwood M., Spraker T. R., Gulland F. M. D. (2013). Domoic acid exposure and associated clinical signs and histopathology in Pacific harbor seals (Phoca vitulina richardii). Harmful Algae 23, 28–33. doi: 10.1016/j.hal.2012.12.008
Medina-Elizalde J., García-Mendoza E., Turner A. D., Sánchez-Bravo Y. A., Murillo-Martínez R. (2018). Transformation and depuration of paralytic shellfish toxins in the Geoduck clam Panopea globosa from the Northern Gulf of California. Front. Mar. Sci. 5, 335. doi: 10.3389/fmars.2018.00335
Meyer-Gutbrod E. L., Davies K. T. A., Johnson C. L., Plourde S., Sorochan K. A., Kenney R. D., et al. (2023). Redefining North Atlantic right whale habitat use patterns under climate change. Limnol Oceanogr 68, S71–S86. doi: 10.1002/lno.12242
Moore J. E., Heinemann D., Francis T. B., Hammond P. S., Long K. J., Punt A. E., et al. (2021). Estimating bycatch mortality for marine mammals: concepts and best practices. Front. Mar. Sci. 8. doi: 10.3389/fmars.2021.752356
NOAA (National Oceanic and Atmospheric Administration) (2021). Final rule to amend the Atlantic Large Whale Take Reduction Plan to reduce risk of serious injury and mortality to North Atlantic right whales caused by entanglement in northeast crab and lobster trap/pot fisheries. U.S. 86 FR 51970. Available online at: https://www.federalregister.gov/documents/2021/09/17/2021-19040/taking-of-marine-mammals-incidental-to-commercial-fishing-operations-atlantic-large-whale-take (accessed November 17, 2023).
NOAA (National Oceanic and Atmospheric Administration) (2023). Taking of Marine Mammals Incidental to Commercial Fishing Operations; Atlantic Large Whale Take Reduction Plan Regulations. Temporary rule; emergency final rule. 88 FR 7362. Available online at: https://www.federalregister.gov/documents/2023/02/03/2023-02185/taking-of-marine-mammals-incidental-to-commercial-fishing-operations-atlantic-large-whale-take (accessed November 17, 2023).
Pirotta V., Grech A., Jonsen I. D., Laurance W. F., Harcourt R. G. (2019). Consequences of global shipping traffic for marine giants. Front. Ecol. Environ. 17, 39–47. doi: 10.1002/fee.1987
Petroff R., Richards T., Crouthamel B., McKain N., Stanley C., Grant K. S. (2019). Chronic, low-level oral exposure to marine toxin, domoic acid, alters whole brain morphometry in nonhuman primates. Neurotoxic 72, 114-124. doi: 10.1016/j.neuro.2019.02.016
Read A. J., Drinker P., Northridge S. (2006). Bycatch of marine mammals in U.S. Global fisheries. Conserv. Biol. 20 1, 163–169. doi: 10.1111/j.1523-1739.2006.00338.x
Redfern J. V., Moore T. J., Fiedler P. C., de Vos A., Brownell R. L., Forney K. A., et al. (2017). Predicting cetacean distributions in data-poor marine ecosystems. Diversity Distributions. 23, 394–408. doi: 10.1111/ddi.2017.23.issue-4
Reeves R. R., McClellan K., Werner T. B. (2013). Marine mammal bycatch in gillnet and other entangling net fisheries 1990 to 2011. Endang Species Res. 20, 71–97. doi: 10.3354/esr00481
Richardson K., Hardesty B. D., Vince J., Wilcox C. (2022). Global estimates of fishing gear lost to the ocean each year. Sci. Adv. 8, eabq0135. doi: 10.1126/sciadv.abq0135
Roberts J. J., Best B. D., Mannocci L., Fujioka E., Halpin P. N., Palka D. L., et al. (2016). Habitat-based cetacean density models for the U.S. Atlantic Gulf Mexico. Sci. Rep. 6, 22615. doi: 10.1038/srep22615
Rockwood R. C., Calambokidis J., Jahncke J. (2017). High mortality of blue, humpback and fin whales from modeling of vessel collisions on the U.S. West Coast suggests population impacts and insufficient protection. PloS One 12, 8, e0183052. doi: 10.1371/journal.pone.0183052
Ryan J. P., Kudela R. M., Birch J. M., Blum M., Bowers H. A., Chavez F. P., et al. (2017). Causality of an extreme harmful algal bloom in Monterey Bay, California, during the 2014–2016 northeast Pacific warm anomaly. Geophys. Res. Lett. 44, 5571–5579. doi: 10.1002/2017GL072637
Sandoval-Belmar M., Smith J., Moreno A. R., Anderson C., Kudela R. M., Sutula M., et al. (2023). A cross-regional examination of patterns and environmental drivers of Pseudo-nitzschia harmful algal blooms along the California coast. Harmful Algae 126, 102435. doi: 10.1016/j.hal.2023.102435
Santora J. A., Mantua N. J., Schroeder I. D., Field J. C., Hazen E. L., Bograd S. J., et al. (2020). Habitat compression and ecosystem shifts as potential links between marine heatwave and record whale entanglements. Nat Commun. 11, 536. doi: 10.1038/s41467-019-14215-w
Savage K. (2017). Alaska and British Columbia large whale unusual mortality event summary report. NOAA fisheries, protected resources division, Juneau, AK. Available online at: https://repository.library.noaa.gov/view/noaa/17715 accessed April 8, 2024.
Schoeman R. P., Patterson-Abrolat C., Plön S. (2020). A global review of vessel collisions with marine animals. Front. Mar. Sci. 7. doi: 10.3389/fmars.2020.00292
Scholin C. A., Gulland F., Doucette G. J., Benson S., Busman M., Chavez F. P., et al. (2000). Mortality of sea lions along the central California coast linked to a toxic diatom bloom. Nature 403, 80–84. doi: 10.1038/47481
Sellner K. G., Doucette G. J., Kirkpatrick G. J. (2003). Harmful algal blooms: causes, impacts and detection. J. Ind. Microbiol. Biotechnol. 30, 383–406. doi: 10.1007/s10295-003-0074-9
Shumway S. E., Allen S. M., Boersma P. D. (2003). Marine birds and harmful algal blooms: sporadic victims or under-reported events? Harmful Algae 2, 1–17. Available at: https://www.sciencedirect.com/science/article/pii/S1568988303000027.
Silber G. K., Adams J. D. (2019). Characteristics and trends of Arctic vessel operation 2015-2017. Front. Mar. Sci. 6, 573. doi: 10.3389/fmars.2019.00573
Silber G. K., Adams J. D., Fonnesbeck C. J. (2014). Compliance with vessel speed restrictions to protect North Atlantic right whales. PeerJ 2, e399. doi: 10.7717/peerj.399
Silber G. K., Lettrich M. D., Thomas P. O., Baker J. D., Baumgartner M., Becker E. A., et al. (2017). Projecting marine mammal distribution in a changing climate. Front. Mar. Sci. 4. doi: 10.3389/fmars.2017.00413
Silber G. K., Weller D. W., Reeves R. R., Adams J. D., Moore T. J. (2021). Co-occurrence of gray whales and vessel traffic in the North Pacific Ocean. Endang Species Res. 44, 177–201. doi: 10.3354/esr01093
Smith J., Connell P., Evans R. H., Gellene A. G., Howard M. D. A., Jones B. H., et al. (2018). A decade and a half of Pseudo-nitzschia spp. and domoic acid along the coast of southern California. Harmful Algae 79, 87–104. doi: 10.1016/j.hal.2018.07.007
Starr M., Lair S., Michaud S., Scarratt M., Quilliam M., Lefaivre D., et al. (2017). Multispecies mass mortality of marine fauna linked to a toxic dinoflagellate bloom. PloS One 12, e0176299. doi: 10.1371/journal.pone.0176299
Sterling A. R., Kirk R. D., Bertin M. J., Rynearson T. A., Borkman D. G., Caponi M. C., et al. (2022). Emerging harmful algal blooms caused by distinct seasonal assemblages of a toxic diatom. Limnology Oceanography 67, 2341–2359. doi: 10.1002/lno.12189
Stumpf R. P. (2001). Applications of satellite ocean color sensors for monitoring and predicting harmful algal blooms. Hum. Ecol. Risk Assessment: Int. J. 7, 1363–1368. doi: 10.1080/20018091095050
Teitelbaum J. S., Zatorre R. J., Carpenter S., Gendron D., Evans A. C., Gjedde A., et al. (1990). Neurologic sequelae of domoic acid intoxication due to the ingestion of contaminated mussels. N Engl. J. Med. 322, 1781–1787. doi: 10.1056/NEJM199006213222505
Thomas P. O., Reeves R. R., Brownell R. L. (2016). Status of the world’s baleen whales. Mar. Mamm. Sci. 32, 682–734. doi: 10.1111/mms.2016.32.issue-2
Twiner M. J., Fire S., Schwacke L., Davidson L., Wang Z., Morton S., et al. (2011). Concurrent exposure of bottlenose dolphins (Tursiops truncatus) to multiple algal toxins in Sarasota Bay, Florida, USA. PloS One 6, e17394. doi: 10.1371/journal.pone.0017394
Van Dolah F. M. (2000). Marine algal toxins: Origins, health effects, and their increased occurrence. Environ. Health Perspectives. Rev. Environ. Health 108, Supplement 1, 133–141. doi: 10.1289/ehp.00108s1133
Keywords: harmful algal blooms, whale deaths, whale entanglement, ship strikes, marine algal toxicity, climate change
Citation: Silber GK and Silber KM (2024) Co-occurrence of harmful algal blooms and whale deaths. Front. Mar. Sci. 11:1454656. doi: 10.3389/fmars.2024.1454656
Received: 25 June 2024; Accepted: 25 October 2024;
Published: 22 November 2024.
Edited by:
Rochelle Diane Seitz, College of William & Mary, United StatesReviewed by:
Kathi Lefebvre, National Oceanic and Atmospheric Administration (NOAA), United StatesJessica Huggins, Cascadia Research Collective, United States
Copyright © 2024 Silber and Silber. This is an open-access article distributed under the terms of the Creative Commons Attribution License (CC BY). The use, distribution or reproduction in other forums is permitted, provided the original author(s) and the copyright owner(s) are credited and that the original publication in this journal is cited, in accordance with accepted academic practice. No use, distribution or reproduction is permitted which does not comply with these terms.
*Correspondence: Gregory K. Silber, gregsilber2@gmail.com