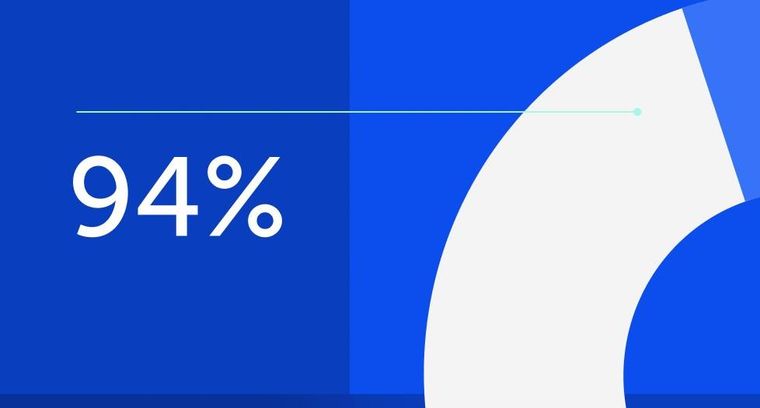
94% of researchers rate our articles as excellent or good
Learn more about the work of our research integrity team to safeguard the quality of each article we publish.
Find out more
ORIGINAL RESEARCH article
Front. Mar. Sci., 09 August 2024
Sec. Marine Pollution
Volume 11 - 2024 | https://doi.org/10.3389/fmars.2024.1450396
Bay ecosystems with unique economic and ecological value are more vulnerable to heavy metal pollution than other marine ecosystems. In South Korea, rapid economic development has exacerbated heavy metal pollution in bay environments. This study analyzed concentrations of seven heavy metals and the structure of macrobenthic community in Asan Bay. Five heavy metal indices (PLI, Pn, RI, TRI, and MERMQ) were used to assess ecological risk. Additionally, spearman correlation, biota–environment matching (BIO-ENV), and redundancy analysis (RDA) were utilized to determine the influence of heavy metals on macrobenthic communities. Although average concentrations of seven heavy metals were below sediment quality guidelines (SQGs), the average concentration of Cd was close to the threshold effects level (TEL). The nemerow pollution index (Pn) and the potential ecological risk index (RI) indicated that Cd was the primary heavy metal contributing to ecological risk. Spearman correlation, BIO-ENV, and RDA indicated that heavy metals were the main environmental factors influencing macrobenthic communities in Asan Bay. However, five heavy metal indices (PLI, Pn, RI, TRI, and MERMQ) did not show a response to the macrobenthic communities. Overall, low concentrations of heavy metals have a certain negative impact on macrobenthic communities in Asan Bay. This study can serve as an important reference for marine environmental protection and policy-making in Asan Bay.
Since the Industrial Revolution, humans have released large amounts of heavy metals into the marine environment (Hu et al., 2015; Mishra et al., 2023). Bay ecosystems have become a concentrated area of human activity due to their unique economic and ecological conditions (Li et al., 2018; He and Silliman, 2019). They are more susceptible to heavy metal pollution than other marine ecosystems (Wang et al., 2023; Guan et al., 2024). Due to heavy metals’ abundance, toxicity, resistance to biodegradation, and bioaccumulation, they have attracted significant attention (Christophoridis et al., 2019; Mani and Kumar, 2014). Among various concerns, fixation of heavy metals in marine sediments has become a global problem (Naifar et al., 2018; Alabssawy and Hashem, 2024; Waqas et al., 2024).
Surface sediments can act as carriers and absorbents of heavy metals (Joksimović et al., 2020). However, fixation of heavy metals in sediments is not permanent. When environmental conditions change, heavy metals can migrate to the sediment-water interface (Zhao et al., 2013; Chen et al., 2017). Therefore, sediments can serve as a source of heavy metals in the marine environment and play a crucial role in the transport and deposition of heavy metals (Bastami et al., 2014; Wang et al., 2018). This makes the assessment of ecological risks posed by heavy metals in sediments particularly important.
Macrobenthos have become effective biological indicators for assessing marine environmental pollution and the ecological quality status of marine ecosystems due to their long lifespans, limited mobility, and species-specific responses to pressure (Rakocinski, 2012; Lam-Gordillo et al., 2020; Pandey et al., 2022; Shi et al., 2023; Liang et al., 2024a). Numerous studies have indicated that macrobenthos are effective indicators for assessing heavy metal pollution (Duzzin et al., 1988; Wang et al., 2022). For example, heavy metal pollution can lead to altered functional traits of macrobenthos in coastal lagoons (Hu et al., 2019).
It is worth noting that although heavy metal indices have been widely used to assess the ecological risks of marine sediments (Halawani et al., 2022), few studies have focused on the relationship between heavy metal indices and macrobenthic communities (Zhao et al., 2023). Moreover, due to the different principles underlying heavy metal indices, ecological risk assessments for the same area can yield varying results depending on the indices chosen (Zhao et al., 2012).
Asan Bay situated between Gyeonggi-do and Chungcheongnam-do provinces in South Korea is a significant industrial and logistics hub with numerous industrial parks (Kim et al., 2021). The bay experiences a spring tidal range of 8 meters and a neap tidal range of 3.5 meters. Approximately 55% of its annual precipitation occurs between June and August (Park et al., 2021). Six artificial lakes near Asan Bay discharge over 2.5 billion m3 of freshwater into the bay annually (Jeong et al., 2016). Park et al. (2020) have indicated that concentrations of heavy metals in the seawater of Asan Bay are inversely proportional to its salinity. This suggests that the influx of industrial effluent, domestic sewage, and agricultural wastewater from nearby artificial lakes is a significant source of heavy metals in Asan Bay.
While progress has been made in understanding spatial distribution of heavy metals and conducting ecological risk assessments along the South Korean coast (Ra et al., 2013), ecological risk assessment of the Asan Bay area based on heavy metal concentration has been overlooked. Moreover, only a few studies have focused on the impact of heavy metals on macrobenthos in South Korea (Lim et al., 2013). Thus, aims of this study were: (1) to evaluate concentrations and spatial distribution of seven heavy metals in surface sediments of Asan Bay, (2) to assess the ecological risk of sediments using five heavy metal indices (PLI, Pn, RI, TRI, and MERMQ), (3) to analyze the impact of heavy metals on macrobenthic community structures.
The study area (A1-A10) was located on the western part of Asan Bay. The southern part of the study area was near the Dangjin Ironworks (Figure 1). At each station, samples were collected three times using a Van Veen grab (0.1 m2) (ChemLab Co., Ltd., Incheon, South Korea) in February, June, September, and November 2014. Two of these samples were designated for macrobenthic analysis, while the third was reserved for environmental factors analysis. The bottom seawater was collected using a Niskin water sampler. Salinity, pH, and dissolved oxygen (DO) were measured using a multiparameter water quality sonde (YSI 6920, YSI Inc., Yellow Springs, OH, USA).
In the field, the first two samples were sieved through a 0.5 mm mesh to collect macrobenthos and preserved in a 4% formalin solution (Samchun Co., Ltd., Seoul, South Korea). The third sample was stored at -20°C and transported to the laboratory for analysis.
In the laboratory, macrobenthos were identified to the species level using an Olympus SZX-10 microscope (Olympus Co. Ltd., Tokyo, Japan). For sediment grain size analysis, wet sieving was initially employed to measure particles with a grain size less than 4 Φ. Particles with a grain size greater than 4 Φ were analyzed using a Sedigraph 5100D (Micromeritics Instrument Corp., USA). To determine the ignition loss (IL), a 50 g sediment sample was heated at 550°C for four hours in a muffle furnace (HY-800, Hwa Sueng Ind Co. Ltd., Busan, South Korea). The concentrations of Acid Volatile Sulfide (AVS) and Chemical Oxygen Demand (COD) in the sediment samples were measured using the detection tube method and the titration method, respectively (National Institute of Fisheries Science, 2010). The acid digestion method was used to measure the heavy metal contents (As, Cd, Cr, Cu, Pb, Zn, and Hg) in the sediment (National Institute of Fisheries Science, 2010).
To assess the community structure and diversity of macrobenthos in Asan Bay, four ecological indices, species richness Index (d), Pielou’s Evenness (J’), Simpson Index (1-λ’), and Shannon-Wiener Diversity Index (H’), were calculated using Primer version 7 (PRIMER-E Ltd., Albany, New Zealand) (Clarke et al., 2014). Calculation formulas for these four ecological indices are shown in Supplementary Table S1.
Five widely used heavy metal indices were calculated to accurately assess the ecological risk of heavy metals in marine sediments. Pollution load index (PLI), nemerow pollution index (Pn), and potential ecological risk index (RI) were based on geochemical background values. In addition, the RI considered potential ecological risk coefficient for heavy metals (Liang et al., 2024a). Mean ERM quotient (MERMQ) and toxic risk index (TRI) were based on sediment quality guidelines (SQGs) (Zhao et al., 2023). Formulas for heavy metal indices and categorization of levels of ecological risk for heavy metal indices are shown in Table 1. Geochemical background values, sediment quality guidelines, and ecological risk coefficient for heavy metals are shown in Supplementary Table S2.
Table 1 Formulas for calculating heavy metal indices and categorization of levels of ecological risk for heavy metal indices.
To assess environmental characteristics of Asan Bay, principal component analysis (PCA) was conducted using PRIMER version 7 (PRIMER-E Ltd., Albany, New Zealand). Environmental factor data were log-transformed (log(x+1)) and normalized before performing the PCA. Shapiro-Wilk test was performed to assess the normality of heavy metal concentrations and mean grain size using OriginPro 2023 (OriginLab Inc, Northampton, MA, USA). To assess monthly differences in heavy metal concentrations and mean grain size, One-Way Analysis of Variance (ANOVA) and the Kruskal-Wallis Test (K-W test) were employed using SPSS 29 (IBM Corporation, Armonk, NY, USA). The gidding method was employed to create spatial distribution maps for heavy metals using Surfer 14 software (Golden Software Inc., Golden, CO, USA). To evaluate the community structure of macrobenthic organisms using Analysis of Similarity (ANOSIM) and Similarity Percentage (SIMPER) analyses in PRIMER version 7, the abundance data were log-transformed (log(x+1)) and normalized beforehand.
To evaluate the relationship between dominant species and environmental factors, redundancy analysis (RDA) was used. Dominant species are defined as those with an abundance exceeding 2% of the total abundance. RDA was performed using Canoco 5.0 (http://www.canoco5.com). To evaluate the relationship between macrobenthic communities and environmental factors, biota–environment matching (BIO–ENV) analysis was conducted using Primer version 7 (Walters and Coen, 2006). Spearman correlation analysis was performed using OriginPro 2023 (OriginLab Inc, Northampton, MA, USA) to assess relationships of heavy metals with IL, AVS, COD, mean grain size, abundance of species, number of species, and ecological indices. Additionally, to evaluate the correlation between heavy metal indices and macrobenthic communities, Spearman correlation analysis was employed.
Range and mean values of environmental factors in Asan Bay are shown in Table 2. Values of environmental factors in Asan Bay at each station are shown in Supplementary Table S3. The coefficient of variation for AVS was the highest at 0.45, while the coefficient for salinity was the lowest at 0.03. The sediment type in Asan Bay is primarily silt. Although the highest mean grain size was observed in November and the lowest in June, the mean grain size did not show significant differences across the months (K-W test, p > 0.05).
In principal component analysis (PCA), PC1 and PC2 axes explained 38.2% of the environmental variance. In the PCA plot, stations from November were positioned higher on the PC2 axis than those from other months (Figure 2). This indicated that stations in November had higher As concentration but lower AVS content than stations in other months (Supplementary Table S4).
Mean concentrations of heavy metals in surface sediments were as follows: Zn (44.03mg/kg), Pb (21.98mg/kg), Cr (11mg/kg), Cu (8.64mg/kg), Cd (0.71mg/kg), As (0.45mg/kg), and Hg (0.003mg/kg) (Table 2). The average concentration of Pb in Asan Bay was compared to the Central Area of the East Sea, South Korea (Liang et al., 2024c). Additionally, average concentrations of Cu and Zn in Asan Bay exceeded those in Garolim Bay (Liang et al., 2024b). The average concentration of Cd in Asan Bay was higher than that in the highly urbanized Shenzhen Bay (Huang et al., 2018). Similarly, the average concentration of Cd in Asan Bay was higher than that in the South Yellow Sea (Yuan et al., 2012) (Supplementary Table S5). Notably, the concentration of Cd in Asan Bay was several times higher than background concentrations (Table 2; Supplementary Table S2) (Woo et al., 2019). Cd is a toxic heavy metal that has no physiological function (Genchi et al., 2020). The adsorption and chelation of cadmium Cd by organic matter can lead to increased concentrations of Cd in sediments. This Cd can then be transferred to macroinvertebrates and fish (De Almeida Rodrigues et al., 2022). Future research needs to evaluate the accumulation of Cd in macroinvertebrates and fish in Asan Bay and identify specific sources of Cd.
In February, average concentrations of most heavy metals (except for As) in Asan Bay were the highest (Supplementary Figures S1–S4). Concentrations of As (K-W test, p < 0.05), Cr (ANOVA, p < 0.01), and Cu (ANOVA, p < 0.05) showed significant differences across different months (Supplementary Figure S5). Short-term variations in heavy metal concentrations are typically closely associated with human activities (Vig et al., 2003; Ye et al., 2019). Heavy industrial activities are usually accompanied by substantial emissions of heavy metals (Jeong et al., 2021). For example, Ironworks emit significant amounts of waste gases and wastewater containing heavy metals (Sponza and Karaoǧlu, 2002; Zhu et al., 2023). Therefore, heavy industrial activities might have contributed to As, Cr, and Cu concentrations in Asan Bay. Additionally, artificial lakes near Asan Bay discharge substantial agricultural runoff and domestic wastewater into Asan Bay. Most studies have indicated that domestic sewage and agricultural runoff contain significant heavy metals (Kunhikrishnan et al., 2012; Vardhan et al., 2019). This could also be one of the reasons for concentrations of heavy metals in sediments of Asan Bay.
In Spearman correlation analysis, As was positively correlated with Cr. Additionally, Cr was positively correlated with Cu and COD. Cu also showed a positive correlation with Zn, COD, and mean grain size. In contrast, Zn was negatively correlated with Hg and IL. In addition, As was negatively correlated with AVS (Figure 3). These correlation analysis results suggest that As, Cr, Cu, and Zn may have similar sources and migration patterns. The negative correlation between AS and AVS indicated that as AVS accumulates in the sediment, As was transformed into other metal forms (Peng et al., 2009). Positive correlations of COD with Cr and Cu and Pb with IL indicated that Cr, Cu, and Pb were present in sediments in forms associated with organic matter. The weak correlation between mean grain size and heavy metals content might be attributed to limited variation in mean grain size within the study area.
Figure 3 Spearman correlation analysis for heavy metals, IL, AVS, COD, and mean grain size. IL, ignition loss; AVS, acid volatile sulfide; COD, chemical oxygen demand.
A total of 109 species of macrobenthos were identified, with annelids (51 species) showing the highest number, followed by molluscs (25 species), arthropods (21 species), echinoderms (8 species), and other animals (4 species) (Supplementary Figure S6). Numbers of macrobenthic species identified in February, June, September, and November were 74, 68, 70, and 46, respectively (Figure 4; Supplementary Figure S6). Macrobenthic species abundance values in February, June, September, and November were 2035.6, 2438.8, 1867.1, and 807.1, respectively (Figure 5). In studies by Yu et al. (2011) and Paik et al. (2008), the macrobenthic community in Asan Bay was similarly dominated by polychaetes. Likewise, macrobenthic communities in Cheonsu Bay and Garolim Bay, South Korea, were predominantly polychaetes (Liang et al., 2024b, d, e).
Figure 5 Abundance of species (ind./m2) at each station. F, February; J, June; S, September; N, November.
In SIMPER analysis, Tharyx sp had the highest contribution percentage in group a; Heteromastus filiformis had the highest contribution percentage in groups b, c, and f; Amphiodia craterodmeta had the highest contribution percentage in groups d and g; while Ampharete arctica had the highest contribution percentage in group e (Figure 6). ANOSIM analysis indicated that there are significant differences between groups a, b, c, d, e, and f (R= 0.859, p = 0.1%). Overall, Amphiodia craterodmeta is the most abundant species in Asan Bay (Figure 6).
Figure 6 Shade plot for the top fifty most significant macrobenthic species in Asan Bay. F, February; J, June; S, September; N, November.
Species Richness Index (d), Pielou’s Evenness (J’), Simpson Index (1-λ’), and Shannon-Wiener Diversity Index (H’) ranged from 1.02 to 5.48 (mean 3.06), 0.16 to 0.94 (mean 0.67), 0.5 to 4.21 (mean 2.93), and 0.12 to 0.93 (mean 0.74), respectively. The highest average d was observed in February, while the lowest was in November. The highest average J’ occurred in November and the lowest one occurred in February. The highest average of 1-λ’ was found in September and the lowest one was found in February. Similarly, the highest average H’ was recorded in September and the lowest one was recorded in February (Supplementary Figures S7–S10). Overall, three ecological indices (J’, 1-λ’, and H’) indicated that the diversity of the macrobenthic community was the lowest in February. This lower diversity was attributed to Amphiodia craterodmeta, which accounted for 37.3% of total individuals in February. Some studies have shown that echinoderms are indicator species for trawl fisheries (Shephard et al., 2010; Jørgensen et al., 2016; Rosellon-Druker and Stokesbury, 2019). In our study, Amphiodia craterodmeta predominantly appeared at coastal stations (i.e., A1-A3) or within bays (i.e., A4). Future research should explore the relationship between the population size of Amphiodia craterodmeta and trawl fisheries.
In our study, average concentrations of all heavy metals were below the Sediment Quality Guidelines (SQGs), including Threshold Effects Level (TEL), Probable Effects Level (PEL), Effects Range Low (ERL), and Effects Range Median (ERM) (Supplementary Table S2) (Buchman, 2008; Jin et al., 2022). Notably, the average concentration of Cd was 0.71 mg/kg, close to the TEL value of 0.75 mg/kg. This suggests that the concentration of Cd is approaching the threshold below which adverse effects on the ecosystem are rarely observed (Jung et al., 2024). In future studies of Asan Bay, it is essential to focus on the concentration of Cd.
Although SQGs are widely used for assessing ecological risks, some issues are still associated with these guidelines (McCauley et al., 2000; Kwok et al., 2014). For example, most SQGs were developed or recalibrated for specific regions without accounting for fluctuating environmental factors (such as particle size and pH) (Hübner et al., 2009). The Ministry of Oceans and Fisheries in South Korea has established SQGs that include only TEL and PEL. We recommend that the Ministry to further enhance SQGs of South Korea by incorporating additional criteria to provide a more comprehensive assessment framework. This enhancement would improve the accuracy and effectiveness of environmental monitoring and management.
PLI, Pn, and RI based on geochemical background concentrations ranged from 0.37 to 0.55 (mean 0.48), 2.97 to 5.43 (mean 3.98), and 136.23 to 241.54 (mean 179.45), respectively. The ecological risk based on PLI values was unpolluted at each station (Figure 7). The ecological risk based on Pn values was heavy pollution at each station (except for station 2 in November) (Figure 8). The ecological risk based on RI values was moderate at each station (Figure 9). Although PLI, Pn, and RI have been widely used to assess heavy metal contamination in marine sediments (Jafarabadi et al., 2017; Rezaei et al., 2021), the comparability of these assessments is often reduced due to different methods used for calculating geochemical background concentrations and the lack of a unified standard (Tian et al., 2017; Zhang et al., 2023). We recommend South Korea to establish a standardized geochemical background values framework to address this issue. These three indices’ different calculation methods and principles might have led to varying results in assessing ecological risk (Huang et al., 2020; Yang et al., 2020). The Pn considers the highest ratio of heavy metal content in sediments to geochemical background values (Table 1). In our study, the average value of Cd was 5.4 times the geochemical background concentration, resulting in the ecological risk assessment indicating heavy pollution at each station. Notably, Cd concentration exceeding geochemical background concentrations in marine sediments is a global phenomenon. For example, Cd concentrations have been found to exceed geochemical background concentrations in the Andaman Islands of India, Zhejiang Province of China, and Chabahar Bay, Makoran of Iran (Nobi et al., 2010; Agah, 2021; Zhao et al., 2023). Human activities have led to the enrichment of Cd in surface marine sediments (Apeti et al., 2009; Huang et al., 2014). When environmental conditions change, accumulated Cd in sediments can be released into the pore water, posing a threat to aquatic organisms (Hu et al., 2017). The RI considers heavy metals’ potential ecological risk coefficient (Table 1). The RI index indicates the potential for ecological risk due to heavy metal contamination in Asan Bay. In the calculation of RI, overall contribution percentages of Cd and Hg were relatively high in Asan Bay. This result is similar to findings of Yang et al. (2020) for rivers of the southwestern coastal region of South Korea.
Figure 7 Values of Pollution load index (PLI) and ecological risk level at each station. F, February; J, June; S, September; N, November.
Figure 8 Values of nemerow pollution index (Pn) and ecological risk level at each station. F, February; J, June; S, September; N, November.
Figure 9 Values of potential ecological risk index (RI) and ecological risk level at each station. F, February; J, June; S, September; N, November.
TRI and MERMQ based on SQGs ranged from 1.74 to 2.6 (mean 2.0) and from 0.04 to 0.07 (mean 0.05), respectively. The ecological risk based on TRI values was not toxic at each station (Figure 10). The ecological risk based on MERMQ values was a 9% probability of toxicity at each station (Figure 11). Overall, TRI and MERMQ indicate that macrobenthos in Asan Bay face a low risk and that all sediment samples pose no toxic risk to the benthos.
Figure 10 Values of toxic risk index (TRI) and ecological risk level at each station. F, February; J, June; S, September; N, November.
Figure 11 Values of mean ERM quotient (MERMQ) and ecological risk level at each station. F, February; J, June; S, September; N, November.
Based on assessment results of comprehensive SQGs and five heavy metal indices, surface sediments of Asan Bay were evaluated as polluting. This result is likely due to anthropogenic pollution from nearby industrial complexes (especially ironworks) and agricultural activities in Asan Bay. Although pollution levels might not be severe, continuous monitoring and management are still necessary.
In Spearman correlation analysis, As was negatively correlated with the number of species, d’, H’, and 1-λ’. Additionally, Cr was negatively correlated with d’, J’, H’, and 1-λ’. In contrast, Zn was positively correlated with d’ (Figure 12). Metal elements are essential for the growth and development of marine organisms (Yılmaz et al., 2017). However, when metal concentrations reach certain levels, they can become toxic to marine organisms (Hall and Frid, 1995). In this study, concentrations of all heavy metals were below the SQGs. Different forms of metals can alter their toxicities to organisms (Das et al., 2014; Zhang et al., 2018). For example, Dauvin (2008) has noted that the toxicity of copper to estuarine invertebrates increases with decreasing salinity and increasing temperature. In our study, higher concentrations of As and Cr were associated with reduced abundance and diversity of macrobenthos. However, it was perplexing that Zn was positively correlated with d. Different benthos have varying tolerances for heavy metals (Webb et al., 2020). For example, crustaceans have lower Cd tolerance than annelids (Gonzalez, 2004). Additionally, Zn was negatively correlated with Hg and IL. This indicates that points with high Zn concentrations have low levels of organic matter and Hg, which might explain the positive correlation between Zn and d.
Figure 12 Spearman correlation analysis for heavy metals, number of species, abundance of species, and ecological indices. d, Species Richness index., J’, Pielou’s Evenness index., 1-λ’, Simpson index., H’, Shannon-Wiener Diversity index.
In redundancy analysis, Axis 1 and Axis 2 explained 43.52% of the variance in species-environment relationships (Figure 13). Pb and Zn exhibited positive correlations with five dominant species, except for Heteromastus filiformis. Conversely, As, Cd, Cr, and Hg showed negative correlations with five dominant species, except for Amphiodia craterodmeta. Notably, only Heteromastus filiformis displayed a positive correlation with Cu. The study by Zan et al. (2015) indicated that As and Hg exerted stress on polychaetes. Similarly, in our study, Heteromastus filiformis and Ampharete arctica were negatively correlated with these two heavy metals. In the study by Tang et al. (2023), Cu concentrations in seawater had a negative impact on Corophium sp. In this study, Corophium sp. was negatively correlated with Cu concentrations in sediments.
Figure 13 Redundancy analysis for dominant species (>2% of total abundance) and environmental factors. AmplBock, Ampelisca bocki; AmphArct, Ampharete arctica; AmphCrat, Amphiodia craterodmeta; CoropSP, Corophium sp; HetrFilf, Heteromastus filiformis; TheoFrag, Theora fragilis; IL, ignition loss; AVS, acid volatile sulfide; COD, chemical oxygen demand; DO, dissolved oxygen.
In BIO-ENV analysis, COD, As, Zn, and mean grain size were the best environmental variable combinations influencing the macrobenthic community structure. In February, the macrobenthic community structure was most affected by heavy metals (As and Zn) (Table 3). In China’s Jiaozhou Bay, coastal waters of Singapore, and Tunisian harbors, macrobenthic community structures are similarly influenced by grain size and heavy metals (Lu, 2005; Zhang et al., 2013; Rebai et al., 2022). Integrating results of Spearman correlation analysis and BIO-ENV analysis, it is evident that the macrobenthos in Asan Bay have already been affected by heavy metals (i.e., As, Cr, and Zn) in surface sediments.
In spearman correlation analysis, all heavy metal indices performed poorly in correlation with the number of species and abundance of species (Figure 14). PLI, Pn, and RI are based on geochemical background concentrations. They primarily reflect environmental pollution due to human activities (Cuevas et al., 2023). TRI and MERMQ are based on SQGs, emphasizing the likelihood of harmful substances in sediments negatively affecting benthos (Radomirović et al., 2021). In a study by Zhao et al. (2023) on the south coast of Zhejiang province, China, TRI and MERMQ showed an excellent linear relationship with benthos. In the present study, the poor performance of TRI and MERMQ might be attributed to heavy metal concentrations in the research area being below the SQGs. Additionally, compared to the south coast of Zhejiang province, the differences in the structure of the macrobenthic community in Asan Bay might also contribute to the poor performance of TRI and MERMQ with the macrobenthic community.
Figure 14 Spearman correlation analysis for heavy metals indices, number of species, and abundance of species. Pn, nemerow pollution index; PLI, Pollution load index; RI, potential ecological risk index; MERMQ, Mean ERM quotient; TRI, toxic risk index.
1. In this study, mean concentrations of heavy metals in surface sediments in Asan Bay were ranked as follows: Zn > Pb > Cr > Cu > Cd > As > Hg. Although average concentrations of these seven heavy metals were below the sediment quality guidelines (SQGs), the mean concentration of Cd was close to the threshold effects level (TEL). The mean concentration of Cd was several times higher than the geochemical background concentration. As, Cr, and Cu concentrations showed significant monthly variations (p < 0.05).
2. Based on three heavy metal indices (i.e., PLI, Pn, and RI) and geochemical background concentration, surface sediments in Asan Bay have been polluted with heavy metals. However, based on two indices (i.e., TRI and MERMQ) and sediment quality guidelines (SQGs), heavy metals in surface sediments pose no toxic risk to the macrobenthos. Overall, surface sediments of Asan Bay are polluted, likely due to anthropogenic activities. Although pollution levels are not severe, continuous monitoring and management are necessary.
3. Spearman correlations and BIO-ENV analysis indicated that heavy metals (i.e., As, Cr, and Zn) were primary environmental factors influencing the macrobenthic community structure. RDA indicated that most heavy metals had a negative impact on dominant species. In Spearman correlation analysis, five heavy metal indices (PLI, Pn, RI, TRI, and MERMQ) showed poor performances with the macrobenthic community.
The raw data supporting the conclusions of this article will be made available by the authors, without undue reservation.
The manuscript presents research on animals that do not require ethical approval for their study.
JL: Writing – original draft, Writing – review & editing, Conceptualization, Formal analysis, Project administration, Software. C-WM: Data curation, Funding acquisition, Project administration, Resources, Supervision, Validation, Visualization, Writing – review & editing. K-BK: Conceptualization, Investigation, Writing – review & editing.
The author(s) declare financial support was received for the research, authorship, and/or publication of this article. This work was supported by the Soonchunhyang University Research Fund. Although we received funding from the university, it was not associated with a specific grant number.
The authors declare that the research was conducted in the absence of any commercial or financial relationships that could be construed as a potential conflict of interest.
All claims expressed in this article are solely those of the authors and do not necessarily represent those of their affiliated organizations, or those of the publisher, the editors and the reviewers. Any product that may be evaluated in this article, or claim that may be made by its manufacturer, is not guaranteed or endorsed by the publisher.
The Supplementary Material for this article can be found online at: https://www.frontiersin.org/articles/10.3389/fmars.2024.1450396/full#supplementary-material
Agah H. (2021). Ecological risk assessment of heavy metals in sediment, fish, and human hair from Chabahar Bay, Makoran, Iran. Mar. pollut. Bull. 169, 112345. doi: 10.1016/j.marpolbul.2021.112345
Alabssawy A. N., Hashem A. H. (2024). Bioremediation of hazardous heavy metals by marine microorganisms: a recent review. Arch. Microbiol. 206, 103. doi: 10.1007/s00203-023-03793-5
Apeti D. A., Lauenstein G. G., Riedel G. F. (2009). Cadmium distribution in coastal sediments and mollusks of the US. Mar. pollut. Bull. 58, 1016–1024. doi: 10.1016/j.marpolbul.2009.02.013
Bastami K. D., Bagheri H., Kheirabadi V., Zaferani G. G., Teymori M. B., Hamzehpoor A., et al. (2014). Distribution and ecological risk assessment of heavy metals in surface sediments along southeast coast of the Caspian Sea. Mar. pollut. Bull. 81, 262–267. doi: 10.1016/j.marpolbul.2014.01.029
Buchman M. F. (2008). Screening Quick Reference Tables (SQuiRTs) (NOAA). Available online at: https://repository.library.noaa.gov/view/noaa/9327. (accessed March 15, 2024)
Chen M., Ding S., Zhang L., Li Y., Sun Q., Zhang C. (2017). An investigation of the effects of elevated phosphorus in water on the release of heavy metals in sediments at a high resolution. Sci. Total Environ. 575, 330–337. doi: 10.1016/j.scitotenv.2016.10.063
Christophoridis C., Bourliva A., Evgenakis E., Papadopoulou L., Fytianos K. (2019). Effects of anthropogenic activities on the levels of heavy metals in marine surface sediments of the Thessaloniki Bay, Northern Greece: Spatial distribution, sources and contamination assessment. MICROCHEM. J. 149, 104001. doi: 10.1016/j.microc.2019.104001
Clarke K. R., Gorley R. N., Somerfield P. J., Warwick R. M. (2014). Changes in Marine Communities: An Approach to Statistical Analysis and Interpretation. (Auckland: PRIMER-E Ltd).
Cuevas J. G., Faz A., Martínez-Martínez S., Gabarrón M., Beltrá J. C., Martínez J., et al. (2023). Spatial distribution and pollution evaluation in dry riverbeds affected by mine tailings. Environ. Geochem. Health 45, 9157–9173. doi: 10.1007/s10653-022-01469-5
Das S., Raj R., Mangwani N., Dash H. R., Chakraborty J. (2014). Heavy metals and hydrocarbons. Microbial. Biodegrad. Bioremed., 23–54. doi: 10.1016/B978-0-12-800021-2.00002-9
Dauvin J.-C. (2008). Effects of heavy metal contamination on the macrobenthic fauna in estuaries: The case of the Seine estuary. Mar. pollut. Bull. 57, 160–169. doi: 10.1016/j.marpolbul.2007.10.012
De Almeida Rodrigues P., Ferrari R. G., Kato L. S., Hauser-Davis R. A., Conte-Junior C. A. (2022). A systematic review on metal dynamics and marine toxicity risk assessment using crustaceans as bioindicators. Biol. Trace. Elem. Res. 200, 881–903. doi: 10.1007/s12011-021-02685-3
Dong J.-Y., Wang X., Zhang X., Bidegain G., Zhao L. (2023). Integrating multiple indices based on heavy metals and macrobenthos to evaluate the benthic ecological quality status of Laoshan Bay, Shandong Peninsula, China. Ecol. Indic. 153, 110367. doi: 10.1016/j.ecolind.2023.110367
Duzzin B., Pavoni B., Donazzolo R. (1988). Macroinvertebrate communities and sediments as pollution indicators for heavy metals in the river Adige (Italy). Water. Res. 22, 1353–1363. doi: 10.1016/0043-1354(88)90091-7
Genchi G., Sinicropi M. S., Lauria G., Carocci A., Catalano A. (2020). The effects of cadmium toxicity. Int. J. Environ. Res. Public. Health 17, 3782. doi: 10.3390/ijerph17113782
Gonzalez J. L. (2004). Le cadmium: Comportement d’un contaminant métallique en estuaire. Versailles: Editions Quae.
Guan C., Zhao X., Qu T., Sun B., Zhong Y., Hou C., et al. (2024). Quantitative and predictive evaluation of heavy metal pollution in bay ecosystems of northern China: characteristics, accumulation, and risk assessment. ACS. EST. Water. 4, 57–67. doi: 10.1021/acsestwater.3c00334
Halawani R. F., Wilson M. E., Hamilton K. M., Aloufi F. A., Taleb A., Al-Zubieri A. G., et al. (2022). Spatial distribution of heavy metals in near-shore marine sediments of the Jeddah, Saudi Arabia region: enrichment and associated risk indices. J. Mar. Sci. Eng. 10, 614. doi: 10.3390/jmse10050614
Hall J. A., Frid C. L. J. (1995). Responses of estuarine benthic macrofauna in copper-contaminated sediments to remediation of sediment quality. Mar. pollut. Bull. 30, 694–700. doi: 10.1016/0025-326X(95)00051-N
He Q., Silliman B. R. (2019). Climate change, human impacts, and coastal ecosystems in the anthropocene. Curr. Biol. 29, R1021–R1035. doi: 10.1016/j.cub.2019.08.042
Hu G., Bi S., Xu G., Zhang Y., Mei X., Li A. (2015). Distribution and assessment of heavy metals off the Changjiang River mouth and adjacent area during the past century and the relationship of the heavy metals with anthropogenic activity. Mar. pollut. Bull. 96, 434–440. doi: 10.1016/j.marpolbul.2015.05.009
Hu C., Dong J., Gao L., Yang X., Wang Z., Zhang X. (2019). Macrobenthos functional trait responses to heavy metal pollution gradients in a temperate lagoon. Environ. pollut. 253, 1107–1116. doi: 10.1016/j.envpol.2019.06.117
Hu N., Huang P., Zhang H., Wang X., Zhu A., Liu J., et al. (2017). Geochemical source, deposition, and environmental risk assessment of cadmium in surface and core sediments from the Bohai Sea, China. Environ. Sci. Pollut. Res. 24, 827–843. doi: 10.1007/s11356-016-7800-0
Huang P., Li T., Li A., Yu X., Hu N.-J. (2014). Distribution, enrichment and sources of heavy metals in surface sediments of the North Yellow Sea. Cont. Shelf. Res. 73, 1–13. doi: 10.1016/j.csr.2013.11.014
Huang L., Rad S., Xu L., Gui L., Song X., Li Y., et al. (2020). Heavy metals distribution, sources, and ecological risk assessment in Huixian wetland, South China. Water 12, 431. doi: 10.3390/w12020431
Huang F., Xu Y., Tan Z., Wu Z., Xu H., Shen L., et al. (2018). Assessment of pollutions and identification of sources of heavy metals in sediments from west coast of Shenzhen, China. Environ. Sci. pollut. Res. 25, 3647–3656. doi: 10.1007/s11356-017-0362-y
Hübner R., Astin K. B., Herbert R. J. H. (2009). Comparison of sediment quality guidelines (SQGs) for the assessment of metal contamination in marine and estuarine environments. J. Environ. Monit. 11, 713–722. doi: 10.1039/b818593j
Jafarabadi A. R., Riyahi Bakhtiyari A., Shadmehri Toosi A., Jadot C. (2017). Spatial distribution, ecological and health risk assessment of heavy metals in marine surface sediments and coastal seawaters of fringing coral reefs of the Persian Gulf, Iran. Chemosphere 185, 1090–1111. doi: 10.1016/j.chemosphere.2017.07.110
Jeong Y. H., Cho M. K., Lee D. G., Doo S. M., Choi H. S., Yang J. S. (2016). Seasonal variations in seawater quality due to freshwater discharge in Asan bay. J. Korean Soc Mar. Environ. Saf. 22, 454–467. doi: 10.7837/kosomes.2016.22.5.454
Jeong H., Choi J. Y., Ra K. (2021). Heavy metal pollution assessment in stream sediments from urban and different types of industrial areas in South Korea. Soil. Sediment. Contam. 30, 804–818. doi: 10.1080/15320383.2021.1893646
Jin D. R., Lee M., Yang H. J., Kim S., Lee J.-S., Moon S.-D. (2022). Evaluation of metal contamination in brackish area sediments South Korea, using receiver operation characteristic curve. Mar. pollut. Bull. 184, 114175. doi: 10.1016/j.marpolbul.2022.114175
Joksimović D., Perošević A., Castelli A., Pestorić B., Šuković D., Đurović D., et al. (2020). Assessment of heavy metal pollution in surface sediments of the Montenegrin coast: a 10-year review. J. Soils. Sediments. 20, 2598–2607. doi: 10.1007/s11368-019-02480-7
Jørgensen L. L., Planque B., Thangstad T. H., Certain G. (2016). Vulnerability of megabenthic species to trawling in the Barents Sea. ICES. J. Mar. Sci. 73, i84–i97. doi: 10.1093/icesjms/fsv107
Jung J. -M., Kim C. -J., Chung C. -S., Kim T., Gu H. -S., Kim H. -E., et al. (2024). Applying new regional background concentration criteria to assess heavy metal contamination in deep-sea sediments at an ocean dumping site, Republic of Korea. Mar. Pollut. Bull. 200, 116065. doi: 10.1016/j.marpolbul.2024.116065
Kim K.-B., Jung Y.-J., Oh J.-K., Kang H., Son D.-S., Ma C.-W. (2021). Macrobenthic community and benthic health assessment of central area in Asan bay. J. Korean Soc Fish. Mar. Sci. Edu. 33, 903–917. doi: 10.13000/JFMSE.2021.8.33.4.903
Kunhikrishnan A., Bolan N. S., Müller K., Laurenson S., Naidu R., Kim W.-I. (2012). The influence of wastewater irrigation on the transformation and bioavailability of heavy metal(Loid)s in soil. Adv. Agron. 115, 215–297. doi: 10.1016/B978-0-12-394276-0.00005-6
Kwok K. W. H., Batley G. E., Wenning R. J., Zhu L., Vangheluwe M., Lee S. (2014). Sediment quality guidelines: challenges and opportunities for improving sediment management. Environ. Sci. pollut. Res. 21, 17–27. doi: 10.1007/s11356-013-1778-7
Lam-Gordillo O., Baring R., Dittmann S. (2020). Ecosystem functioning and functional approaches on marine macrobenthic fauna: A research synthesis towards a global consensus. Ecol. Indic. 115, 106379. doi: 10.1016/j.ecolind.2020.106379
Li J., Liu Y., Pu R., Yuan Q., Shi X., Guo Q., et al. (2018). Coastline and landscape changes in bay areas caused by human activities: A comparative analysis of Xiangshan Bay, China and Tampa Bay, USA. J. Geogr. Sci. 28, 1127–1151. doi: 10.1007/s11442-018-1546-1
Liang J., Huang H.-R., Ma C.-W., Son D.-S., Kim S.-K. (2024b). Using the heavy metal indices and benthic indices to assess the ecological quality in the tidal flats of Garolim bay, South Korea. Water 16, 736. doi: 10.3390/w16050736
Liang J., Ma C.-W., Kim S.-K., Park S.-H. (2024d). Assessing the benthic ecological quality in the intertidal zone of Cheonsu bay, Korea, using multiple biotic indices. Water 16, 272. doi: 10.3390/w16020272
Liang J., Ma C.-W., Kim K.-B., Son D.-S. (2024e). Can the ecological quality of several bays in South Korea be accurately assessed using multiple benthic biotic indices? J. Mar. Sci. Eng. 12, 1179. doi: 10.3390/jmse12071179
Liang J., Ma C.-W., Son D.-S. (2024c). Using the heavy metal and biotic indices to assess ecological quality in the central area of the east sea, South Korea. Water 16, 1230. doi: 10.3390/w16091230
Liang J., Shu M.-Y., Huang H.-R., Ma C.-W., Kim S.-K. (2024a). Using benthic indices to assess the ecological quality of sandy beaches and the impact of urbanisation on sandy beach ecosystems. J. Mar. Sci. Eng. 12, 487. doi: 10.3390/jmse12030487
Lim D., Choi J.-W., Shin H. H., Jeong D. H., Jung H. S. (2013). Toxicological impact assessment of heavy metal contamination on macrobenthic communities in southern coastal sediments of Korea. Mar. pollut. Bull. 73, 362–368. doi: 10.1016/j.marpolbul.2013.05.037
Lu L. (2005). The relationship between soft-bottom macrobenthic communities and environmental variables in Singaporean waters. Mar. pollut. Bull. 51, 1034–1040. doi: 10.1016/j.marpolbul.2005.02.013
Mani D., Kumar C. (2014). Biotechnological advances in bioremediation of heavy metals contaminated ecosystems: an overview with special reference to phytoremediation. Int. J. Environ. Sci. Technol. 11, 843–872. doi: 10.1007/s13762-013-0299-8
McCauley D. J., DeGraeve G. M., Linton T. K. (2000). Sediment quality guidelines and assessment: overview and research needs. Environ. Sci. Policy. 3, 133–144. doi: 10.1016/S1462-9011(00)00040-X
Mishra R., Singh E., Kumar A., Singh A. K., Madhav S., Shukla S. K., et al. (2023). Metal pollution in marine environment: sources and impact assessment. Metals Water, 175–193. doi: 10.1016/B978-0-323-95919-3.00006-9
Naifar I., Pereira F., Zmemla R., Bouaziz M., Elleuch B., Garcia D. (2018). Spatial distribution and contamination assessment of heavy metals in marine sediments of the southern coast of Sfax, Gabes Gulf, Tunisia. Mar. pollut. Bull. 131, 53–62. doi: 10.1016/j.marpolbul.2018.03.048
National Institute of Fisheries Science Notification of Marine Environmental Process Test Standards (2010). Available online at: https://www.nifs.go.kr/board/actionBoard0052List.do?BBS_CL_CD=B.
Nobi E. P., Dilipan E., Thangaradjou T., Sivakumar K., Kannan L. (2010). Geochemical and geo-statistical assessment of heavy metal concentration in the sediments of different coastal ecosystems of Andaman Islands, India. Estuar. Coast. Shelf Sci. 87, 253–264. doi: 10.1016/j.ecss.2009.12.019
Paik S. G., Yun S. G., Park H. S., Lee J. H., Ma C. W. (2008). Effects of sediment disturbance caused by bridge construction on macrobenthic communities in Asan Bay, Korea. J. Environ. Biol. 29, 559–566.
Pandey V., Jha D. K., Kumar P. S., Santhanakumar J., Venkatnarayanan S., Prince Prakash Jebakumar J., et al. (2022). Effect of multiple stressors on the functional traits of sub-tidal macrobenthic fauna: A case study of the southeast coast of India. Mar. pollut. Bull. 175, 113355. doi: 10.1016/j.marpolbul.2022.113355
Park S., Choi M., Jang D., Joe D., Park K., Lee H., et al. (2020). Spatiotemporal distribution of dissolved heavy metals in Gyeonggi bay, Korea. Ocean. Sci. J. 55, 69–84. doi: 10.1007/s12601-020-0002-1
Park S. J., Kim K., Kim T. I. (2021). Vertical profiles of suspended sediment transport integrating bottom boundary layer in the channel and port of Asan bay, Korea. J. Korean Soc Mar. Environ. Energy. 24, 236–248. doi: 10.7846/JKOSMEE.2021.24.4.236
Peng J., Song Y., Yuan P., Cui X., Qiu G. (2009). The remediation of heavy metals contaminated sediment. J. Hazard. Mater 161, 633–640. doi: 10.1016/j.jhazmat.2008.04.061
Ra K., Kim E.-S., Kim K.-T., Kim J.-K., Lee J.-M., Choi J.-Y. (2013). Assessment of heavy metal contamination and its ecological risk in the surface sediments along the coast of Korea. J. Coast. Res. 65, 105–110. doi: 10.2112/SI65-019.1
Radomirović M., Tanaskovski B., Pezo L., Ceccotto F., Cantaluppi C., Onjia A., et al. (2021). Spatial and temporal distribution of pollution indices in marine surface sediments—a chemometric approach. Environ. Sci. Pollut. Res. 28, 42496–42515. doi: 10.1007/s11356-021-13644-9
Rakocinski C. F. (2012). Evaluating macrobenthic process indicators in relation to organic enrichment and hypoxia. Ecol. Indic. 13, 1–12. doi: 10.1016/j.ecolind.2011.04.031
Rebai N., Mosbahi N., Dauvin J.-C., Neifar L. (2022). Ecological risk assessment of heavy metals and environmental quality of Tunisian harbours. J. Mar. Sci. Eng. 10, 1625. doi: 10.3390/jmse10111625
Rezaei M., Mehdinia A., Saleh A., Modabberi S., Mansouri Daneshvar M. R. (2021). Environmental assessment of heavy metal concentration and pollution in the Persian Gulf. Model. Earth. Syst. Environ. 7, 983–1003. doi: 10.1007/s40808-020-00913-8
Rosellon-Druker J., Stokesbury K. D. E. (2019). Quantification of echinoderms (Echinodermata) on Georges Bank, and the potential influence of marine protected areas on these populations. Invert. Biol. 138, e12243. doi: 10.1111/ivb.12243
Shephard S., Brophy D., Reid D. G. (2010). Can bottom trawling indirectly diminish carrying capacity in a marine ecosystem? Mar. Biol. 157, 2375–2381. doi: 10.1007/s00227-010-1502-9
Shi Y., He Y., Shin P. K. S., Guo Y., Zhang G., Wen Y., et al. (2023). Responses of biological traits of macrobenthic fauna to a eutrophication gradient in a semi-enclosed bay, China. Mar. Environ. Res. 189, 106072. doi: 10.1016/j.marenvres.2023.106072
Sponza D., Karaoǧlu N. (2002). Environmental geochemistry and pollution studies of Aliaǧa metal industry district. Environ. Int. 27, 541–553. doi: 10.1016/S0160-4120(01)00108-8
Tang Z., Liu X., Niu X., Yin H., Liu M., Zhang D., et al. (2023). Ecological risk assessment of aquatic organisms induced by heavy metals in the estuarine waters of the Pearl River. Sci. Rep. 13, 9145. doi: 10.1038/s41598-023-35798-x
Tian K., Huang B., Xing Z., Hu W. (2017). Geochemical baseline establishment and ecological risk evaluation of heavy metals in greenhouse soils from Dongtai, China. Ecol. Indic. 72, 510–520. doi: 10.1016/j.ecolind.2016.08.037
Vardhan K. H., Kumar P. S., Panda R. C. (2019). A review on heavy metal pollution, toxicity and remedial measures: Current trends and future perspectives. J. Mol. Liq. 290, 111197. doi: 10.1016/j.molliq.2019.111197
Vig K., Megharaj M., Sethunathan N., Naidu R. (2003). Bioavailability and toxicity of cadmium to microorganisms and their activities in soil: a review. Adv. Environ. Res. 8, 121–135. doi: 10.1016/S1093-0191(02)00135-1
Walters K., Coen L. D. (2006). A comparison of statistical approaches to analyzing community convergence between natural and constructed oyster reefs. J. Exp. Mar. Biol. Ecol. 330, 81–95. doi: 10.1016/j.jembe.2005.12.018
Wang W., Huo Y., Wang L., Lin C., Liu Y., Huang H., et al. (2023). Integrated assessment of trace elements contamination in sediments of a typical aquaculture bay in China: Ecological toxicity, sources and spatiotemporal variation. J. Clean. Prod. 425, 139122. doi: 10.1016/j.jclepro.2023.139122
Wang Q., Shi S., Liu X. (2022). Functional diversity of macrofaunal assemblages as indicators to assess heavy metal pollution in the Bohai Sea, China. Mar. pollut. Bull. 185, 114265. doi: 10.1016/j.marpolbul.2022.114265
Wang C., Zou X., Feng Z., Hao Z., Gao J. (2018). Distribution and transport of heavy metals in estuarine–inner shelf regions of the East China Sea. Sci. Total. Environ. 644, 298–305. doi: 10.1016/j.scitotenv.2018.06.383
Waqas W., Yuan Y., Ali S., Zhang M., Shafiq M., Ali W., et al. (2024). Toxic effects of heavy metals on crustaceans and associated health risks in humans: a review. Environ. Chem. Lett. 22, 1391–1411. doi: 10.1007/s10311-024-01717-3
Webb A. L., Hughes K. A., Grand M. M., Lohan M. C., Peck L. S. (2020). Sources of elevated heavy metal concentrations in sediments and benthic marine invertebrates of the western Antarctic Peninsula. Sci. Total. Environ. 698, 134268. doi: 10.1016/j.scitotenv.2019.134268
Woo J., Lee H., Park J., Park K., Cho D., Jang D., et al. (2019). Background concentration and contamination assessment of heavy metals in Korean coastal sediments. J. Korean Soc Oceanogr. 24, 64–78. doi: 10.7850/JKSO.2019.24.1.064
Yang H. J., Jeong H. J., Bong K. M., Jin D. R., Kang T.-W., Ryu H.-S., et al. (2020). Organic matter and heavy metal in river sediments of southwestern coastal Korea: Spatial distributions, pollution, and ecological risk assessment. Mar. pollut. Bull. 159, 111466. doi: 10.1016/j.marpolbul.2020.111466
Ye C., Butler O. M., Du M., Liu W., Zhang Q. (2019). Spatio-temporal dynamics, drivers and potential sources of heavy metal pollution in riparian soils along a 600 kilometre stream gradient in Central China. Sci. Total Environ. 651, 1935–1945. doi: 10.1016/j.scitotenv.2018.10.107
Yılmaz A. B., Yanar A., Alkan E. N. (2017). Review of heavy metal accumulation on aquatic environment in Northern East Mediterrenean Sea part I: some essential metals. Rev. Environ. Health 32, 119–163. doi: 10.1515/reveh-2016-0065
Yu O.-H., Lee H.-G., Lee J.-H. (2011). The influence of environmental variables on macrobenthic communities after dike construction in Asan Bay, Korea. Korean J. Environ. Biol. 29, 326–344.
Yuan H., Song J., Li X., Li N., Duan L. (2012). Distribution and contamination of heavy metals in surface sediments of the South Yellow Sea. Mar. pollut. Bull. 64, 2151–2159. doi: 10.1016/j.marpolbul.2012.07.040
Zan X., Zhang C., Xu B., Xue Y., Ren Y. (2015). Distribution of polychaete assemblage in relation to natural environmental variation and anthropogenic stress. J. Ocean Univ. China 14, 749–758. doi: 10.1007/s11802-015-2650-9
Zhang J., Guo W., Li Q., Wang Z., Liu S. (2018). The effects and the potential mechanism of environmental transformation of metal nanoparticles on their toxicity in organisms. Environ. Sci.: Nano. 5, 2482–2499. doi: 10.1039/C8EN00688A
Zhang Y., Lv Z., Guan B., Liu Y., Li F., Li S., et al. (2013). Status of macrobenthic community and its relationships to trace metals and natural sediment characteristics. CLEAN. Soil. Air. Water. 41, 1027–1034. doi: 10.1002/clen.201200575
Zhang J., Peng W., Lin M., Liu C., Chen S., Wang X., et al. (2023). Environmental geochemical baseline determination and pollution assessment of heavy metals in farmland soil of typical coal-based cities: A case study of Suzhou City in Anhui Province, China. Heliyon 9, e14841. doi: 10.1016/j.heliyon.2023.e14841
Zhao S., Feng C., Wang D., Liu Y., Shen Z. (2013). Salinity increases the mobility of Cd, Cu, Mn, and Pb in the sediments of Yangtze Estuary: Relative role of sediments’ properties and metal speciation. Chemosphere 91, 977–984. doi: 10.1016/j.chemosphere.2013.02.001
Zhao S., Feng C., Yang Y., Niu J., Shen Z. (2012). Risk assessment of sedimentary metals in the Yangtze Estuary: New evidence of the relationships between two typical index methods. J. Hazard. Mater. 241–242, 164–172. doi: 10.1016/j.jhazmat.2012.09.023
Zhao Z., Liu Q., Liao Y., Yu P., Tang Y., Liu Q., et al. (2023). Ecological risk assessment of trace metals in sediments and their effect on benthic organisms from the south coast of Zhejiang province, China. Mar. pollut. Bull. 187, 114529. doi: 10.1016/j.marpolbul.2022.114529
Keywords: ecological risk, heavy metal, macrobenthic, sediment, Asan Bay
Citation: Liang J, Ma C-W and Kim K-B (2024) Ecological risk assessment of heavy metals in surface sediments and their impact on macrobenthos in Asan Bay, South Korea. Front. Mar. Sci. 11:1450396. doi: 10.3389/fmars.2024.1450396
Received: 17 June 2024; Accepted: 24 July 2024;
Published: 09 August 2024.
Edited by:
Catherine Tsangaris, Hellenic Centre for Marine Research (HCMR), GreeceReviewed by:
Nikolaos Stamatis, Research and Religious Affairs, GreeceCopyright © 2024 Liang, Ma and Kim. This is an open-access article distributed under the terms of the Creative Commons Attribution License (CC BY). The use, distribution or reproduction in other forums is permitted, provided the original author(s) and the copyright owner(s) are credited and that the original publication in this journal is cited, in accordance with accepted academic practice. No use, distribution or reproduction is permitted which does not comply with these terms.
*Correspondence: Chae-Woo Ma, Y3dvb21hQHNjaC5hYy5rcg==
Disclaimer: All claims expressed in this article are solely those of the authors and do not necessarily represent those of their affiliated organizations, or those of the publisher, the editors and the reviewers. Any product that may be evaluated in this article or claim that may be made by its manufacturer is not guaranteed or endorsed by the publisher.
Research integrity at Frontiers
Learn more about the work of our research integrity team to safeguard the quality of each article we publish.