- Institut Méditerranéen de Biodiversité et d'Ecologie marine et continentale (IMBE), UMR CNRS 7263, IRD 237, Aix Marseille Université, Avignon Université, Station Marine d’Endoume, Chemin de la batterie des lions, Marseille, France
Organisms release and detect molecules for defense, reproduction, feeding strategies and finding suitable habitats. For some migratory species, homing behavior could be related to the recognition of their home chemical fingerprint made of an assemblage of molecules from their habitat. In the marine realm, the functioning of ecosystems such as underwater caves largely depends on trophic interactions between the caves and the outside environment. A key feature of these interactions relies on the circadian migration of small crustaceans (Mysida) from the cave habitat to the open sea. Recently, it has been hypothesized that these migrations could involve chemical mediation. Behavioral experiments using a two-choice system have shown that cave mysids significantly detect cave seawater rather than a control water from the open sea. Here, we used the same experimental system to investigate habitat recognition by two populations of the cave mysid Hemimysis margalefi. Both populations were submitted to a choice between three distinct cave seawaters vs. a control seawater. Additionally, experiments tested the water preference of a non-cave mysid species (Leptomysis sp.) between control and cave seawaters. To evaluate whether the choice of mysids was influenced by chemical cues from conspecifics, a complementary experiment on H. margalefi was conducted. Results demonstrated that each studied mysids population significantly recognizes the water of its own home habitat, and that this behavior is not influenced by the occurrence of H. margalefi’s exudates. Mass spectrometry-based metabolomic analyses revealed that each cave seawater had a specific chemical fingerprint with only a few reproducibly detected signals belonging to different chemical classes: peptides, alkaloids, fatty acids, steroids but also inorganic molecules. Organic pollutants have also been reproducibly detected. Among the detected compounds, one oxylipin derivative and one peptide could be considered as chemical markers of the cave ecosystem. Therefore, we postulate that the chemical seascape of each cave participates to mysid circadian migrations which are analogous to a daily-based homing behavior.
1 Introduction
Chemical mediation is the most widely used mode of communication in the living world and is commonly considered as the “language of life” (Wyatt, 2010, 2014). Exchanges of molecules as chemical cues between organisms are involved in reproduction, defense or feeding and therefore, significantly contribute to the organization and functioning of ecosystems (Burks and Lodge, 2002; Hay, 2009; Hentley and Wade, 2016). Pollination is one of the most famous examples of a chemosensory-mediated network of biotic interactions, plant odors thus structuring the biodiversity of a plethora of arthropods and flying vertebrates (Parachnowitsch and Manson, 2015). More generally, chemical mediation structures the spatial distribution of many animal species capable of orienting themselves within a complex mosaic of odors, often defined as a chemical landscape (Dyer et al., 2018). In the marine environment, the potential existence of a similarly complex chemical seascape, made of a great diversity of molecules transported by water currents, is highly probable. Many of these molecules originate from the metabolic activities of marine organisms. Exudated in the surrounding water, they are named exometabolites and they may carry potential signals for the whole ecosystem (Cassier et al., 2000; Mauduit et al., 2023).
Several studies highlighted that the recruitment of marine species requires the use of chemical cues. For instance, Atema et al. (2002) presented the first evidence that larval reef fishes (primarily apogonids) were able to distinguish between the chemical seascapes from the lagoon and the open ocean. Lecchini et al. (2013) also observed that several fish, crustacean and cephalopod larvae actively selected settlement habitats, assuming the use of chemical cues. The presence of conspecifics, food, the nature of the biodiversity assemblage or the healthy state of an ecosystem, all produce cues susceptible to attract juveniles to a suitable environment for settlement. For example, Coppock et al. (2013) found that juvenile reef fishes exhibited a significant preference for the water conditioned by conspecifics or by some reef-building species. Similarly, Lecchini et al. (2014) showed that various marine larvae were significantly more attracted by the chemical seascape of living corals than by that of dead colonies.
Depending on their fate (e.g. adsorption on particles, solubility), molecules may be transported over long distances and be perceived by chemo-sensitive organisms (Mollo et al., 2014, 2017). Lecchini et al. (2014) found that molecules emitted by corals can be transported over 2 km in the ocean and that they could be detected more than 1 km away by fish larvae searching for their recruitment habitat. Distant chemical cues also mediate habitat selection for migratory species such as salmonid fish and turtles (Stabell, 1992; Endres et al., 2016). They follow chemical corridors to accurately return to their natal habitat, a specific behavior known as homing (Scholz et al., 1976).
However, none of these pioneer studies in marine chemical ecology has addressed the chemical nature of these seascapes, influencing the behavior of many mobile marine organisms. Access to this chemical information is even more crucial as global change alters the environmental quality, and thus, putatively chemical mediation and ecosystem functioning (Munday et al., 2009; Saha et al., 2019; Wilke, 2021; Roggatz et al., 2022). Advances in mass spectrometry (MS)-based metabolomics, including data processing and spectral annotation, have improved the characterization of mixtures of unknown molecules originating from different environments, such as seawater. The knowledge gained from such analyses greatly benefits marine chemical ecology research and provides new opportunities to understand the marine ecosystem functioning (Santonja et al., 2018; Wegley Kelly et al., 2022; Mauduit et al., 2023; Nelson et al., 2023; Quinlan et al., 2023).
Underwater cave ecosystems allow working on simplified processes compared to the open sea, thus providing experimental conditions such as natural mesocosms to study chemical mediation. Of reduced size, they provide a stable environment with little or no light or water movement leading to a low food supply (Harmelin et al., 1985). Among the most successful inhabitants of marine caves, sponges (Porifera) are known to produce a great diversity of metabolites (Carroll et al., 2022), some of them being released into the environment (Ternon et al., 2016; Mauduit et al., 2022, Mauduit et al., 2023) and contributing to a complex chemical seascape. Mobile dwellers of the cave ecosystem include teleost fishes and various crustaceans, such as mysids (Crustacea: Mysida) (Rastorgueff et al., 2011). Mysids can form dense swarms with millions of individuals in a single cave (Passelaigue and Bourdillon, 1986; Coma et al., 1997; David et al., 1999).
Cave mysids including Hemimysis species display circadian horizontal migrations outside their caves to find food and avoid predation at night (Macquart-Moulin and Passelaigue, 1982; Passelaigue and Bourdillon, 1986; Riera et al., 1991). At the end of the night, they return from the open-sea to their cave habitat. In their study, Santonja et al. (2018) used the context of underwater marine caves to address the potential role of sponge-produced metabolites on the migratory behavior of Hemimysis margalefi. MS-based metabolomics led to the determination of molecular formulae of chemical cues suspected to participate in the swimming behavior of cave mysids as well as of shrimps (Crustacea: Decapoda). These compounds were found both in experimental waters potentially containing sponge exometabolites and also naturally occurring in seawater collected in an underwater cave. They were thus hypothesized as possible chemical cues guiding cave mysids during their nocturnal migrations. Furthermore, as the behavioral responses were most pronounced during morning experiments, Santonja et al. (2018) hypothesized that sponge metabolites might act as recognition cues when H. margalefi return to their cave at dawn. Given that each marine cave harbors an original combination of sponge assemblages (Grenier et al., 2018) and that H. margalefi populations display high spatial genetic structuring (Lejeusne and Chevaldonné, 2006; Rastorgueff et al., 2014), it could even be conceivable that a given H. margalefi population could recognize the chemical composition of its own cave water. This would constitute an interesting homing behavior (Brönmark and Hansson, 2000), a case worth of investigation.
The aim of the present study was to evaluate individual cave seawater recognition by cave-dwelling mysids and to compare the overall chemical composition of each tested cave seawater in an attempt to correlate a choice behavior with reproducibly detected molecules. The behavioral response of different populations of two mysid species was evaluated on seawaters naturally occurring in three different underwater caves. The selected species are the cave-dwelling H. margalefi and a non-cave mysid, Leptomysis sp. living in nearby shallow water environments. Hence, the two species have contrasting ecological habits.
Previous studies highlighted the chemical influence of conspecifics on habitat selection for crustaceans such as barnacle larvae (Matsumura et al., 1998), shrimp larvae (Lecchini et al., 2017) and spiny lobsters, where sex pheromones such as conspecific urine of both sexes acted as aggregation signals (Horner et al., 2006). Considering the gregarious behavior of mysids, one might conceive that conspecific chemical signals could play a role in orienting H. margalefi migrations. Therefore, we also tested their choice behavior on seawater originating from aquaria where the same species of mysids were kept. To conduct the experiments, we combined the use of (i) an experimental two-choice device to assess the behavioral response of the crustaceans, and (ii) MS-based metabolomics to decipher the chemical composition of each tested seawater condition.
2 Material and methods
2.1 Study sites
The three underwater caves Fauconnière (43°9.3’N, 5°41.0’E), 3PP (43°9.8’N, 5°36.0’E) and Jarre (43°11.8’N, 5°21.9’E) are distributed from the bay of La Ciotat to the Calanques coast near Marseille (Calanques National Park, South of France). These caves are 11 to 24 m deep and harbor a specialized biodiversity where sponges dominate the sessile benthic assemblages (see Gerovasileiou and Bianchi, 2021 for a list of representative sponge species; Gerovasileiou and Bianchi, 2021). Hemimysis spp. populations have been well studied in these three caves (Chevaldonné and Lejeusne, 2003; Lejeusne and Chevaldonné, 2005, Lejeusne and Chevaldonné, 2006; Rastorgueff et al., 2011, 2014) and the relative abundance of H. margalefi is particularly high in Jarre and Fauconnière.
2.2 Behavioral experiments
Behavioral laboratory experiments were conducted using the aquarium facilities of the Marine Station of Endoume in Marseille (France).
2.2.1 Sample collection and seawater preparation
The different seawaters were collected by SCUBA diving in each of the selected underwater caves with a 20 L polyvinyl chloride tube. The experimental control seawater (Control) was collected in shallow waters (8 m depth) dominated by macrophytes such as Posidonia oceanica and devoid of any characteristic cave benthic organisms. Hence, this control, collected nearby the Marine Station (43°16.80’N, 5°20.95’E), was expected to harbor a totally different chemical composition.
Hemimysis margalefi individuals were collected by SCUBA diving in the Jarre and Fauconnière caves. Although the 3PP cave seawater was collected and used as a “generic” cave water, different from either Jarre or Fauconnière cave waters, H. margalefi were not harvested in 3PP because of the risk to mistake them with a very similar species (H. speluncola) co-occurring in that cave. Indeed, only an examination under a dissecting microscope can tell them apart, which would cause a significant stress to each individual. Leptomysis sp. individuals were sampled from the shallow waters adjacent to the Marine Station. Prior to the experiments, the three mysid populations were kept separately in aquaria, under a controlled circadian cycle, and fed with Artemia salina nauplii every 2 days.
To prepare the seawater conditioned by H. margalefi, individuals from Jarre were maintained in a 30 L aerated tank during 24 h in order to enrich seawater with the products of their metabolic activities, herein called exometabolites (H. margalefi seawater).
2.2.2 Chemosensory experiments
A Y-shaped glass flume providing a two-channel choice system was set up to assess the crustacean behavioral response when faced with two different seawaters (see Santonja et al. (2018) for more details). Two 10 L tanks were connected to the flume by pipes, creating a constant gravity-driven flow of 50 mL min−1 in the Y-channels. The mysids were introduced at the downstream end of the apparatus, where they were free to remain or to swim towards and select one of the two channels connected to the tanks. Each assay was performed with a single specimen which was maintained for a 1−min acclimation period followed by a 5−min testing period. During the test, the position of the mysid in the downstream compartment or in the right/left channels was recorded at 5-sec intervals. After each test, the flume was emptied and rinsed with the Control water. The seawater treatments were then switched between the two-channel system, preventing any memory effect due to previously tested seawater or any system bias. After that switch, the entire assay was repeated with new individuals, each individual being used in a single assay. The time (in seconds) spent by the individual in each of the three compartments of the flume (i.e. the downstream compartment and the two channels) was recorded.
A total of 286 mysid individuals were tested, in 9 experiments (Table 1) conducted both in the morning (7 – 11 AM) and in the afternoon (2 – 6 PM) in order to encompass the daily variability of the crustacean response to the seawater treatments.
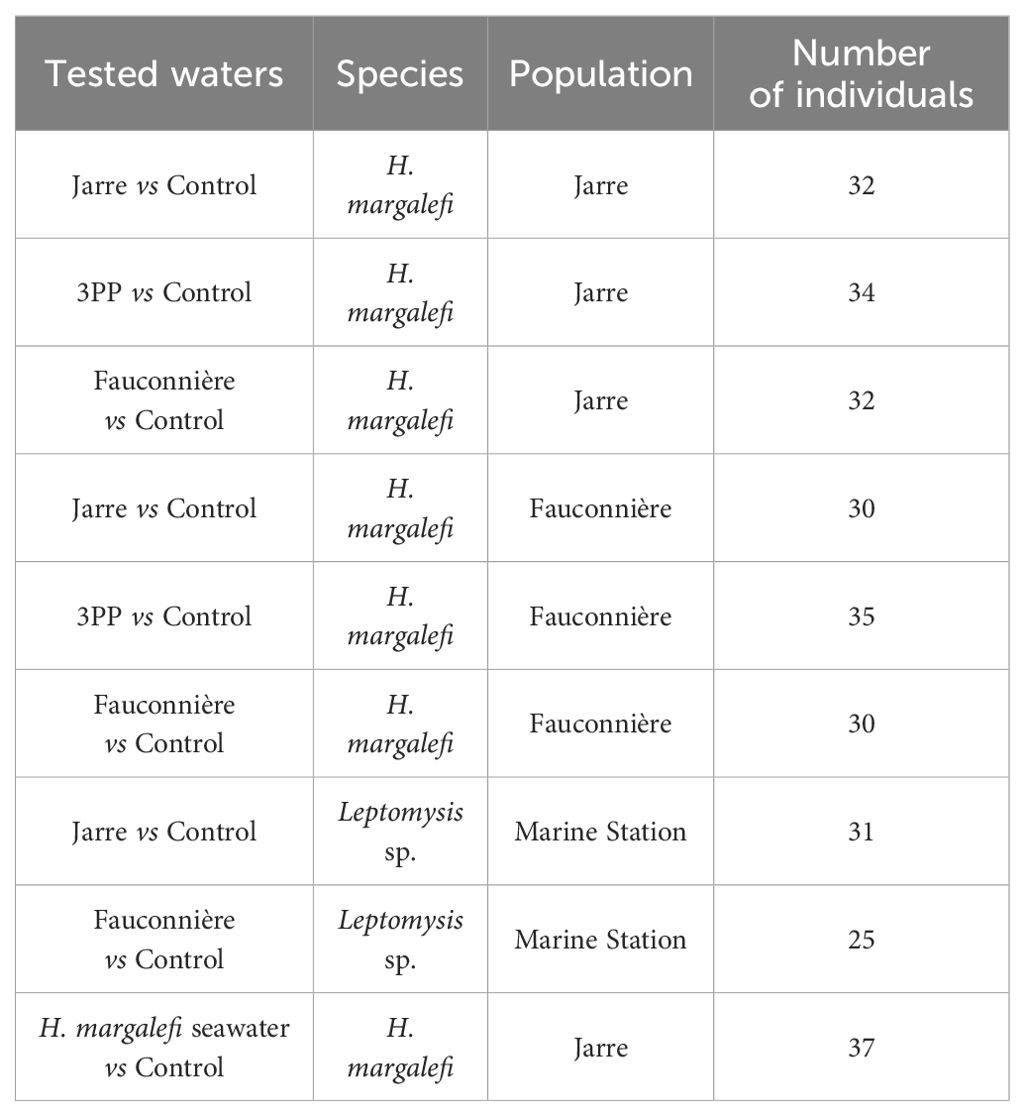
Table 1. Details of the 9 behavioral experiments conducted with different combinations of seawaters, mysid species and populations. Numbers of tested individuals per experiment are also given.
2.3 Metabolomic analyses
Prior to each chemosensory experiment, a volume of 500 mL of seawater was collected from the aquarium tanks for the metabolomic analyses. These water samples were passed successively through 0.45 µm pore-sized filters (polyamide 47 mm, Sartorius®) and octadecyl-bonded silica extraction discs (Empore™ C18 47 mm SPE discs, Sigma-Aldrich®) to obtain their particulate and dissolved phases. The filters were extracted for particulate phase (or eluted for dissolved phase) with 10 mL of methanol (anhydrous grade, Carlo Erba®). The extracts were then concentrated to dryness and frozen at -20 °C for 24 hours to precipitate salts. Supernatant of each extract was dissolved in 1 mL methanol (MS grade, Carlo Erba®) and filtered on 0.2 µm polytetrafluoroethylene (PTFE) luer-lock filters (13 mm, Restek®) (Supplementary S1.1 for further details). A total of 67 samples were prepared for LC-MS analysis (Table 2).
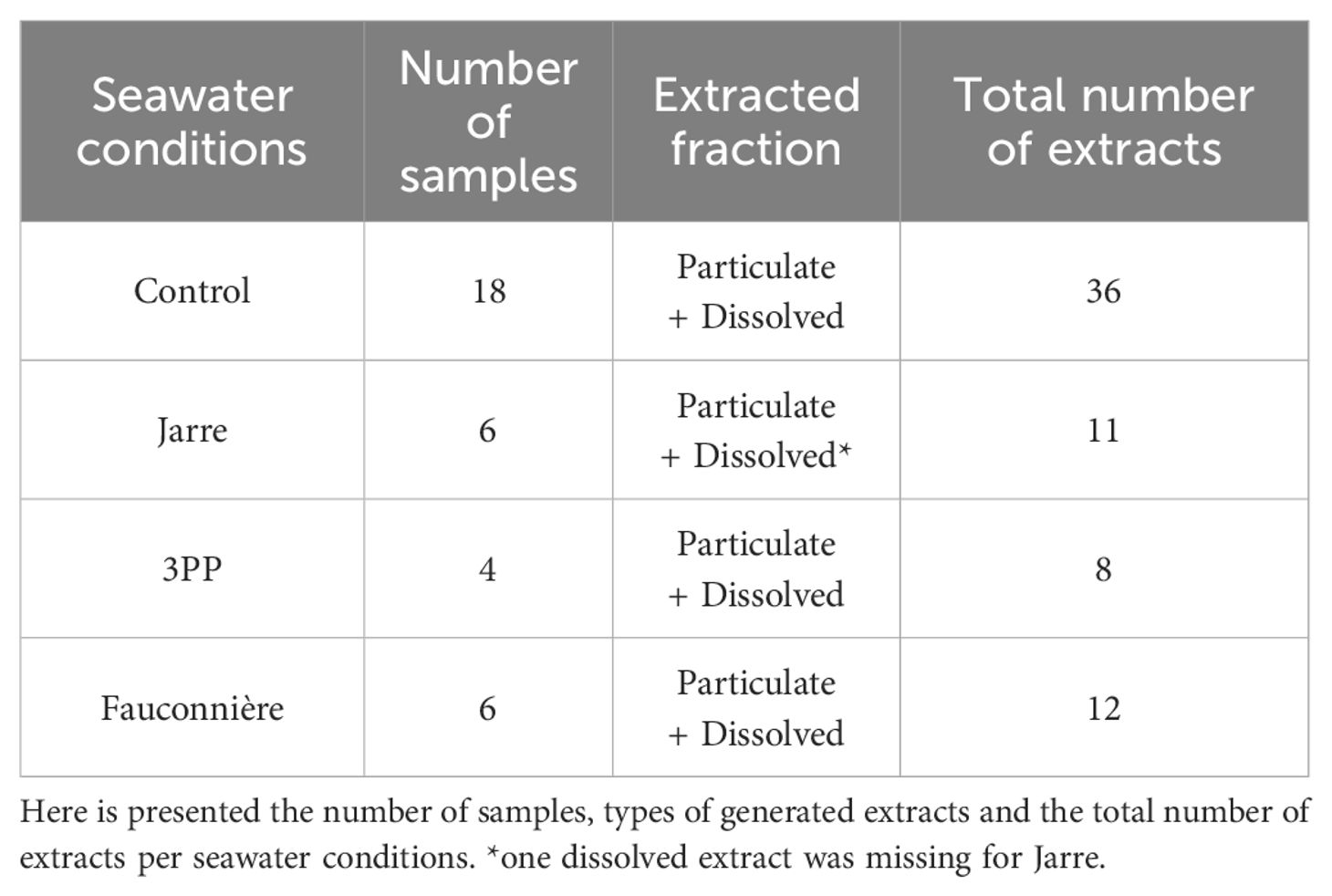
Table 2. Details of cave and control seawater extracts per condition used in the metabolomic analyses.
Ultra-High-Performance Liquid Chromatography coupled to High-Resolution Mass Spectrometer (UHPLC-HR-MS) analyses were performed using a Dionex Ultimate 3000 system equipped with an autosampler and connected to a quadrupole Time of Flight (qToF) mass spectrometer equipped with an electrospray ionization interface (Bruker Impact II) to record mass spectra in the positive and negative modes (see Supplementary S1.2 for detailed methods). Chromatographic separation was achieved using Kinetec® Phenylhexyl column (150 × 2 mm, 1.7 µm, Thermo Scientific) at 0.5 mL.min-1 flow rate using a linear elution gradient of water:acetonitrile (Carlo Erba®, LC/MS grade) with 0.1% formic acid (Carlo Erba®, LC/MS grade) from 95:5 (v:v, isocratic from 0 to 2 min) to 0:100 in 8 min (v:v, isocratic from 10 to 13 min) with a return to initial conditions from 13.01 to 17 min. The injected volume was set to 1 µL. The mass spectrometer parameters were set as follows: nebulizer sheath gas, N2 (3.5 bar); dry gas, N2 (12 L.min-1); capillary temperature, 200 °C; capillary voltage, 2500 V in positive mode and 3000 V in negative mode; end plate offset, 500 V; collision gas, N2; collision energy, 4 eV. Data were acquired at 2 Hz in the 50 to 1200 m/z range in full scan mode. For MS/MS acquisitions the collision energy was set at 20 eV. Two independent analytical sequences were injected: the first one aimed at highlighting metabolic differences between the control and the cave seawaters (N extracts = 67, 2 groups were compared) without trying to annotate chemical signals and a second sequence included only the three cave seawater samples (N extracts = 31, 3 groups were compared) in order to evaluate difference in their chemical composition and annotate cave-specific and reproducibly-detected chemical features.
Following the two UHPLC-HR-MS data acquisitions of seawaters, two different feature detection methods were performed (see detailed methods in Supplementary S1.3).
Files for the cave seawater comparisons were converted into the open format *.mzXML using MSConvert (Proteowizard version 3.0.23332) (Chambers et al., 2012). Positive and negative modes were separately processed on MZmine 3.9 (Schmid et al., 2023) for data matrix generation.
2.4 Multivariate data analyses
Multivariate analyses were performed with the combined feature lists resulting from both acquisition modes. Dissolved and particulate fractions of extracts were combined for analysis keeping the sample origin for each feature. The combined list was imported on MetaboAnalyst 5.0 (Pang et al., 2022). Data were log-transformed and Pareto-scaled (i.e. mean-centered and divided by the square root of the standard deviation of each variable). Partial-Least Square Discriminant Analyses (PLS-DA) were conducted to preview the disparity of the chemical composition of samples for each group of seawater sample. Associated to the PLS-DA, permutation tests (1E4 permutations, PLS-DA models, based on a double cross-validation, outer loop = 30 or 7-fold cross-validation, and inner loop = 21 or 6-fold cross-validation) was performed and followed by post hoc permutational pairwise test (Hervé, 2021). The generated Classification Error Rate (CER, 0 – 100%) was calculated to estimate the strength of group differentiation: a CER close to zero suggests a low error rate, thus testifying of a high group separation. The CER associated p-value gives an information on the level of confidence obtained on the CER value.
2.5 Molecular networking and analysis of chemical diversity
The two feature lists acquired from positive and negative acquisitions, the feature quantification tables (csv file format) and the corresponding lists of MS2 spectra (mgf file format) were exported from MZmine 3.9 for Feature Based Molecular Networking on the GNPS platform (https://gnps.ucsd.edu) (Nothias et al., 2020). Positive and negative networks were then gathered on the same platform using the “merge network polarity” workflow (ppm tolerance: 10 and RT tolerance: 0.18 min).
The resulting network was visualized and interpreted using Cytoscape 3.8.2 (Shannon et al., 2003), where each chemical feature corresponding to a MS2 spectrum is represented by a node (Supplementary S5). By using the Cytoscape filter tab, we determined the number of features detected in each cave seawater (e.g. column filter: cave, number of nodes containing “3PP”) to have a first overview of the chemical diversity.
To delineate the chemical fingerprint of each cave seawater, we counted the features reproducibly detected in all samples of each cave seawater. SIRIUS software was used to blindly annotate the features using standard parameters (Dührkop et al., 2019). Then, a manual check of these annotations based on MS1/MS2 spectra was performed using the Bruker DataAnalysis 5.0 software to verify and correct the first automatic SIRIUS annotations. Annotations received a Confidence Level (CL) according to Schymanski et al. (2014): When a putative structural identification could be proposed for the selected features after manual inspection, feature received a confidence level 2b or 3 (several possible structural candidates). When only a chemical formula was provided, a confidence level 4 was given. If several features referred to the same molecule, these features were counted as a single compound. The INCHI of each proposed putative structure was used for the assignment of structural class based on natural biosynthesis pathway predictions from NPClassifier web interface (Kim et al., 2021). However, NP Classifier was not employed for inorganic salts (see SI S6.4) and substances suspected to be pollutants (e.g. Dialkyl phosphate).
Chemical class of each annotated molecule were given and bar plots were built to represent the chemical diversity of reproducible molecules specific to each cave.
2.6 Statistical analyses of chemosensory results
For each of the nine experiments (Table 1), paired t-tests (or paired Wilcoxon tests when normality and homoscedasticity conditions were not met) were performed to compare the time spent in each of the two upstream channels (control vs cave seawater) in order to assess the differences between the selected seawaters. The time spent in the downstream compartment was removed from the data because it symbolized the mixing chamber of the two upper stream channels and not a choice made by the mysids.
Two-sample t-tests (or Mann-Whitney when normality and homoscedasticity conditions were not met) were performed to compare the time spent in the channels between morning and afternoon, in order to evaluate whether mysid residence duration was affected by the time of day.
3 Results
3.1 Behavioral experiments
In all conducted experiments in the Y channels, the time spent by all tested mysid populations was not affected by the time of the day (morning vs afternoon, Supplementary S2.1; S2.2; S2.3). Thus, the following results present all individual replicates of a given experiment without considering any specific time of the day exposure.
3.1.1 Residence times of H. margalefi and Leptomysis sp. subjected to cave seawaters
Hemimysis margalefi from Jarre cave significantly spent more time in the cave seawater collected in its own cave, staying 16 times longer in this channel, than in the control channel (Figure 1A). In contrast, H. margalefi from Jarre cave demonstrated no significant preference for cave seawaters relative to the control when the channel was filled with Fauconnière or 3PP cave seawaters (Figures 1B, C).
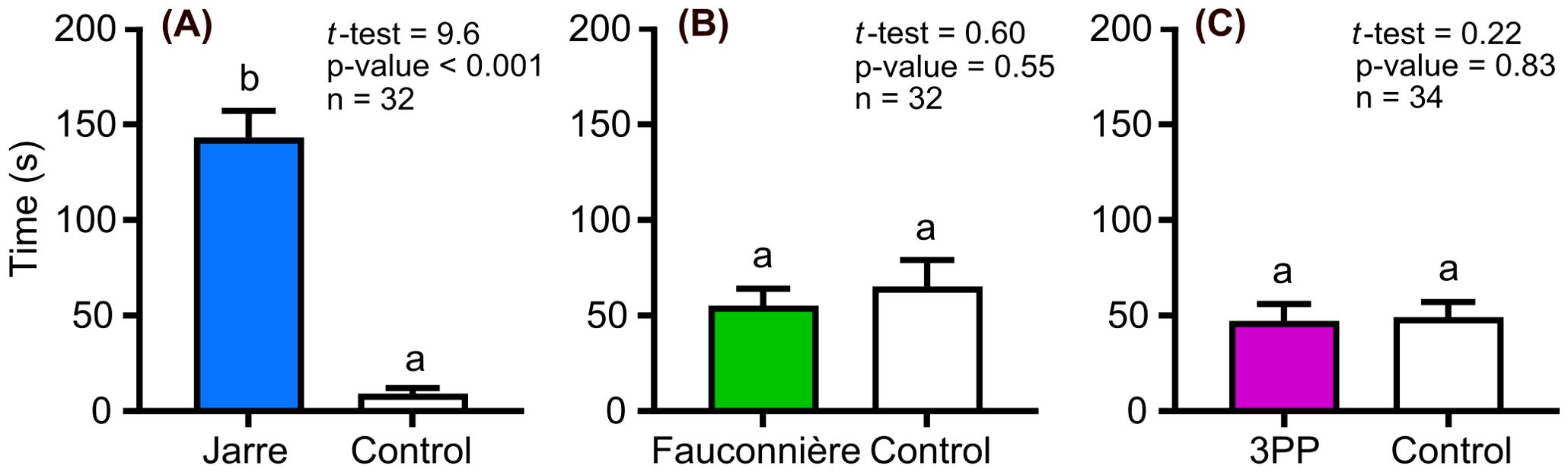
Figure 1. Residence time of Hemimysis margalefi from Jarre cave subjected to: (A) Jarre cave seawater vs Control, (B) Fauconnière cave seawater vs Control and (C) 3PP cave seawater vs Control. Results are mean time ± Standard Error (SE) in seconds spent by each mysid individual in the channel filled with cave seawater (colored bar) and the control channel (white bar). Different letters indicate significant difference between treatments.
Hemimysis margalefi from Fauconnière cave also stayed longer in the cave seawater collected in its own cave, but this behavioral response was less pronounced as the individuals stayed only 3 times longer in this channel than in the control channel (Figure 2A). Finally, H. margalefi from Fauconnière cave were non-responsive to the two other cave seawaters compared to the controls (Figures 2A, C).
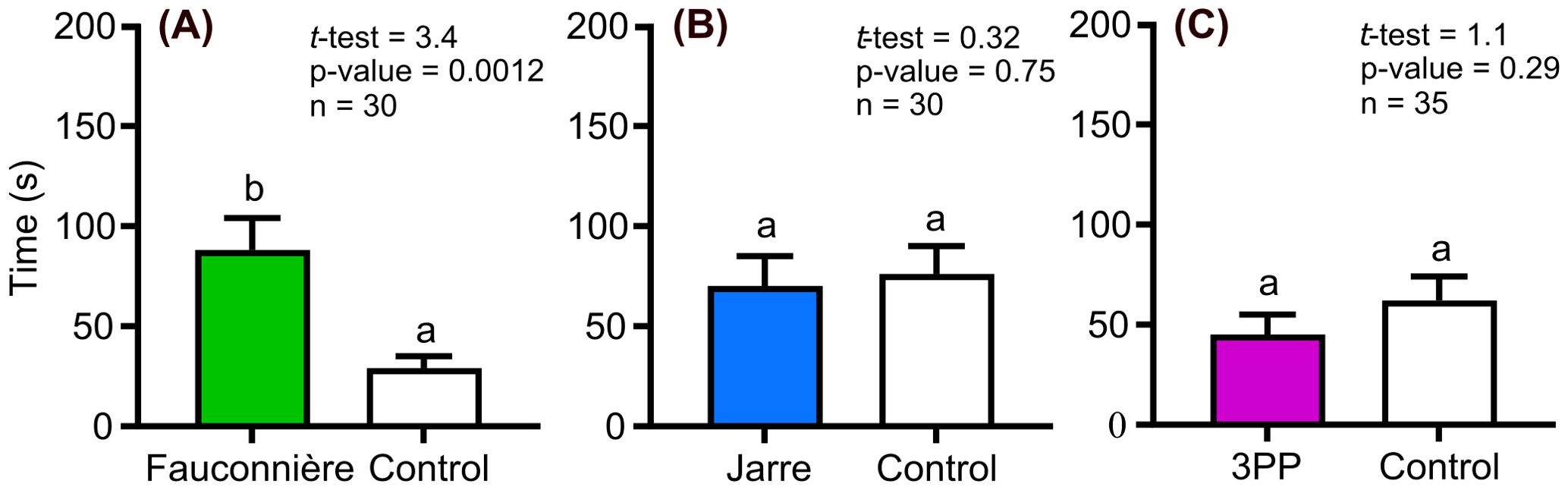
Figure 2. Residence time of Hemimysis margalefi from Fauconnière cave subjected to: (A) Fauconnière cave seawater vs Control, (B) Jarre cave seawater vs Control and (C) 3PP cave seawater vs Control. Results are mean time (± SE) in seconds spent by each mysid individual in the channel filled with cave seawater (colored bars) and the control channel (white bar). Different letters indicate significant difference between treatments.
In contrast, the non-cave mysid Leptomysis sp. significantly spent more time in the control channel, staying respectively 4 and 7 times longer in this channel than in the channels filled with Jarre or Fauconnière cave seawaters (Figures 3A, B).
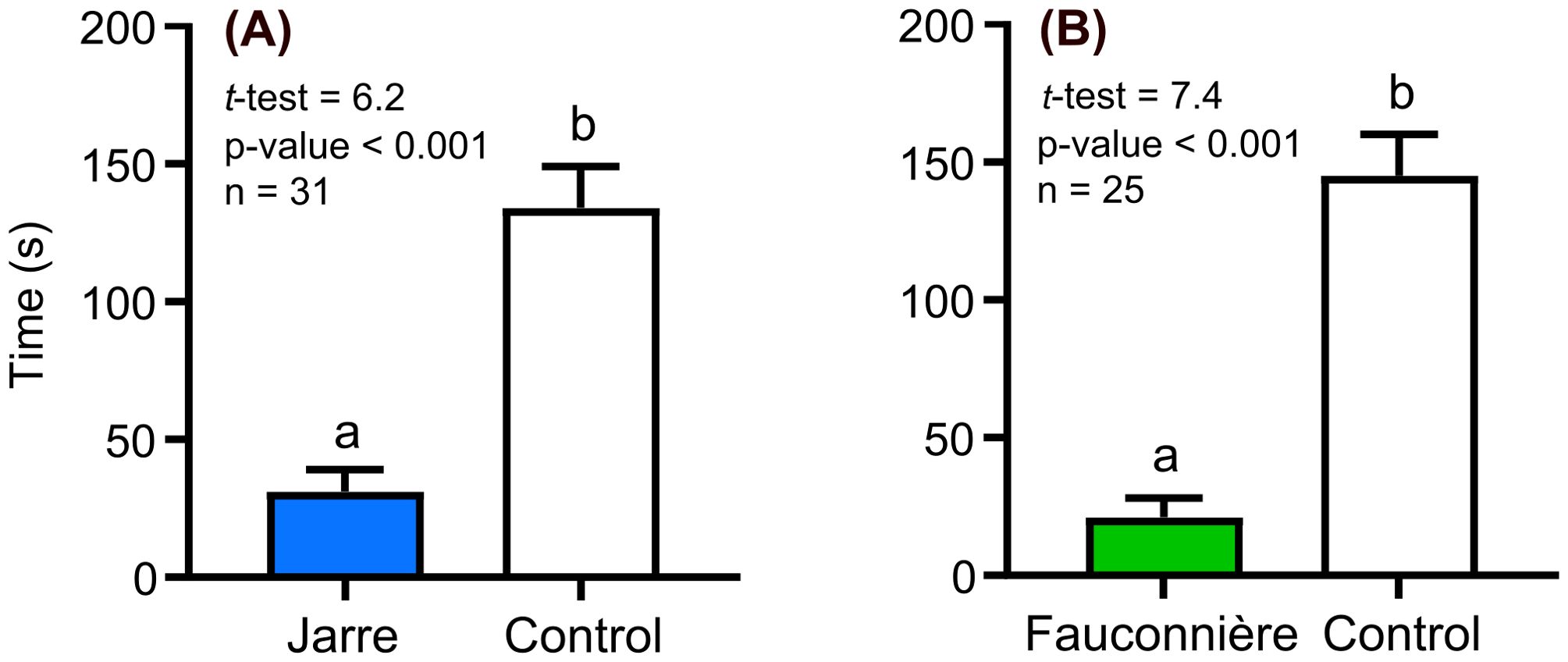
Figure 3. Residence time of Leptomysis sp. subjected to (A) Jarre cave seawater vs Control, and (B) Fauconnière cave vs Control. Results are mean time (± SE) in seconds spent by each mysid individual in the channel filled with cave seawater (colored bars) and the control channel (white bar). Different letters indicate significant difference between treatments.
3.1.2 Residence times of H. margalefi from Jarre subjected to conspecific-conditioned seawater
Hemimysis margalefi from Jarre did not show significant preference for seawater supposed to contain its conspecific exometabolites (Figure 4).
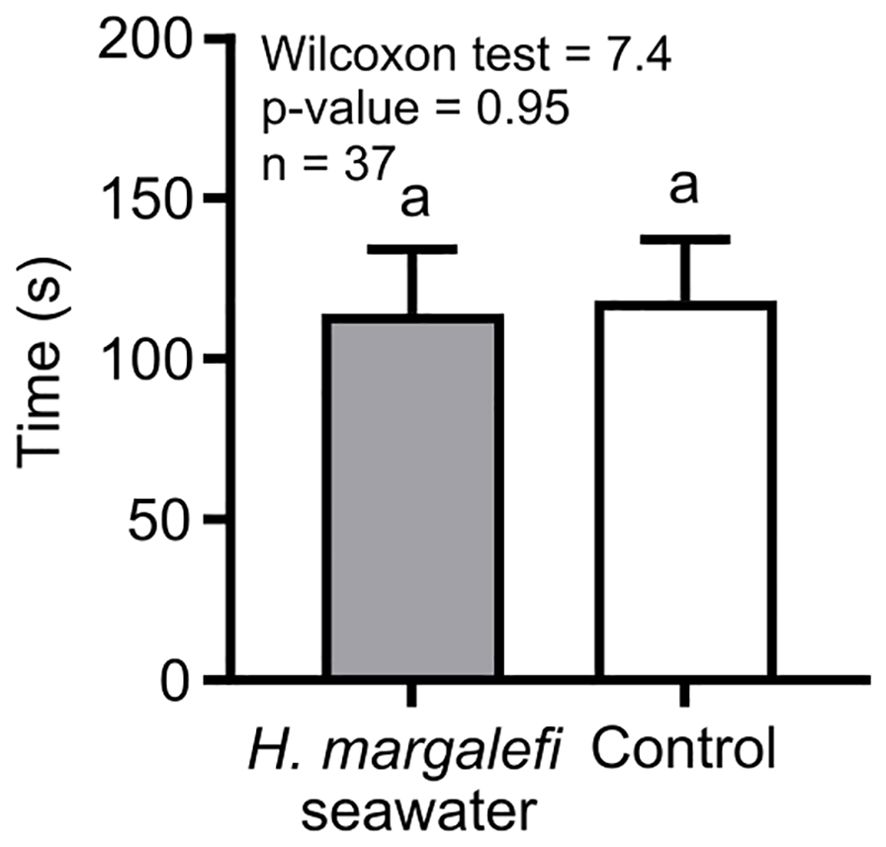
Figure 4. Residence times of Hemimysis margalefi from Jarre cave to their own conditioned seawater vs Control. Results are mean time (± SE) in seconds spent by each crustacean individual in the channel filled with H. margalefi conditioned seawater channel (grey bar) and the control channel (white bar). Same letters indicate no significant difference between treatments.
3.2 Seawater chemical compositions
Untargeted MS-based metabolomic analyses were performed upon noticing a significant time spent in a cave seawater in order to compare the chemical composition of seawater conditions and possibly, identify molecules that could be involved in the observed behavior. As no difference in residence time was observed when H. margalefi were faced to the control vs their own seawater, metabolomic analyses were not performed on these samples.
PLS-DA results (Supplementary S3) comparing the chemical composition of the control seawater to those of the three cave seawaters showed that cave seawaters significantly displayed a different chemical fingerprint compared to control [Classification Error Rate (CER) = 2%, p-value < 0.001]. For each experiment, the tested seawater conditions were chemically different, suggesting that the recorded residence durations could be triggered by chemical signals from the selected seawater medium. As the behavioral response of Leptomysis sp. was used as control, we did not further characterize the chemical diversity of the control water. Thus, the following part focuses on the metabolomic comparison of the three cave seawaters.
3.2.1 Chemical diversity of the three cave seawaters
Multivariate analyses were performed and combined with annotations of recorded MS2 spectra in order to describe and compare the chemical diversity of each cave water. A second PLS-DA (Figure 5A) was performed with data originating from a newly generated Mzmine feature containing 499 chemical signals detected in both positive (283) and negative (216) ionization modes. In this case, the chemical composition of only Jarre seawater was statistically different from the seawater of Fauconnière cave (Pairwise permutation statistical analyses, p-value = 0.0003, Supplementary Table S4), while the composition of 3PP seawater was not statistically different from the two other cave waters (Supplementary Table S4). For each cave, the composition of the particulate phases of extracts appeared distinct from the dissolved phases.
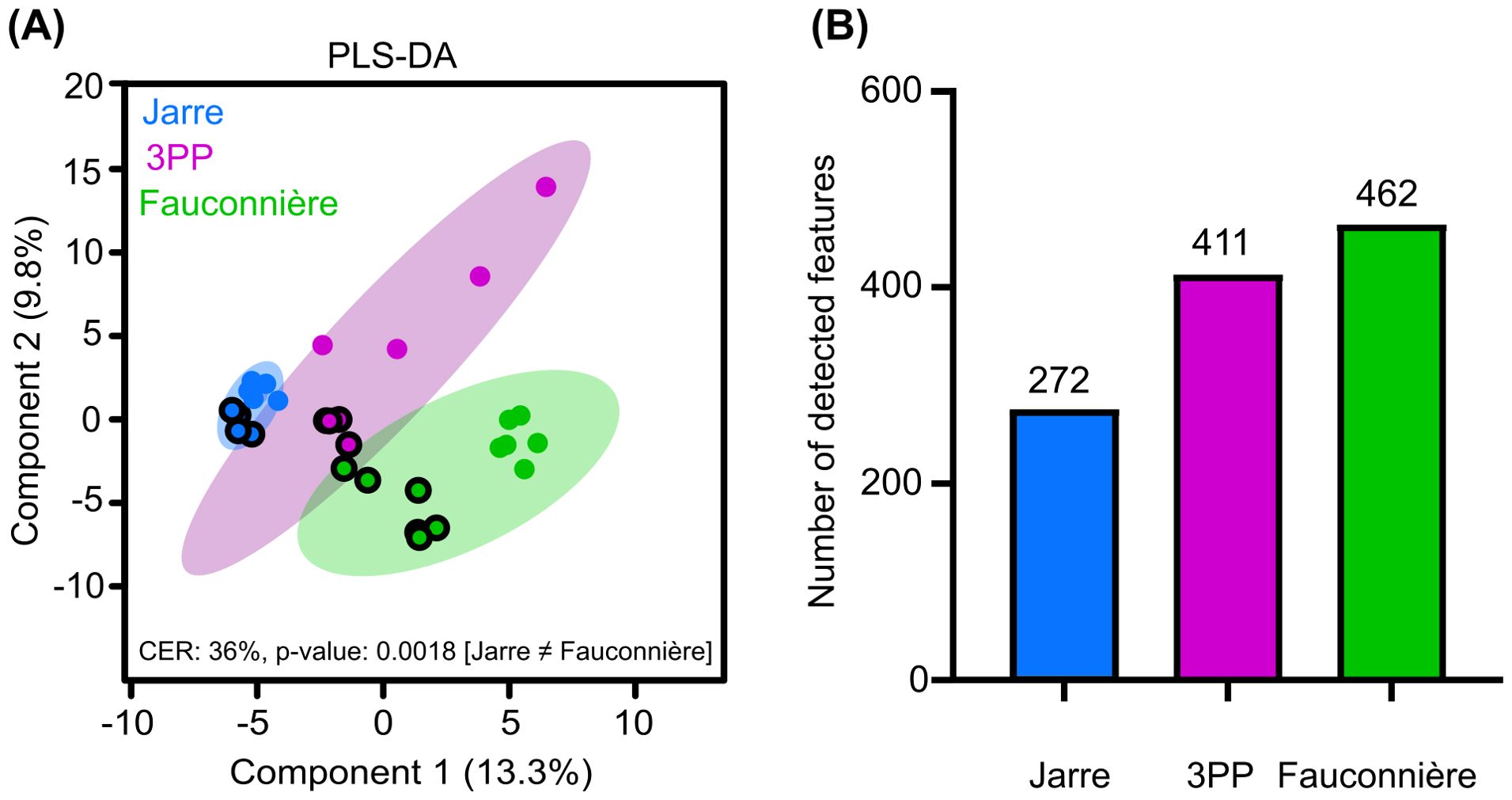
Figure 5. Chemical diversity of the cave seawaters. (A) Partial Least Squares Discriminant Analysis (PLS-DA) based on the chemical features detected in the different cave seawater samples analyzed by UHPLC-HRMS/MS. Differentiation between chemical fingerprints was tested using a permanova test (PPLS-DA model, 1E4 permutations with double-cross validation) followed by a post-hoc permutation pairwise test given in Supplementary Table S4. The strength of group differentiation was evaluated using the classification error rate (CER, 0 – 100%). Dots circled represent the fraction of the sample associated with the particulate extracts when remaining dots correspond to the dissolved fraction. (B) Number of detected features per cave.
The total number of detected features was calculated for each cave seawater to initiate a comparative analysis of chemical diversity. Jarre seawater harbored the lowest number with 272 features while Fauconnière and 3PP seawaters had higher numbers with 462 and 411 respectively (Figure 5B).
In order to establish the specific chemical fingerprint of these three caves, we counted the number of reproducible features specific to each cave. Forty chemical signals were obtained and MS spectra of each of these signals were further annotated to putatively identify 20 underlying compounds, collectively organized by structural class (Figure 6).
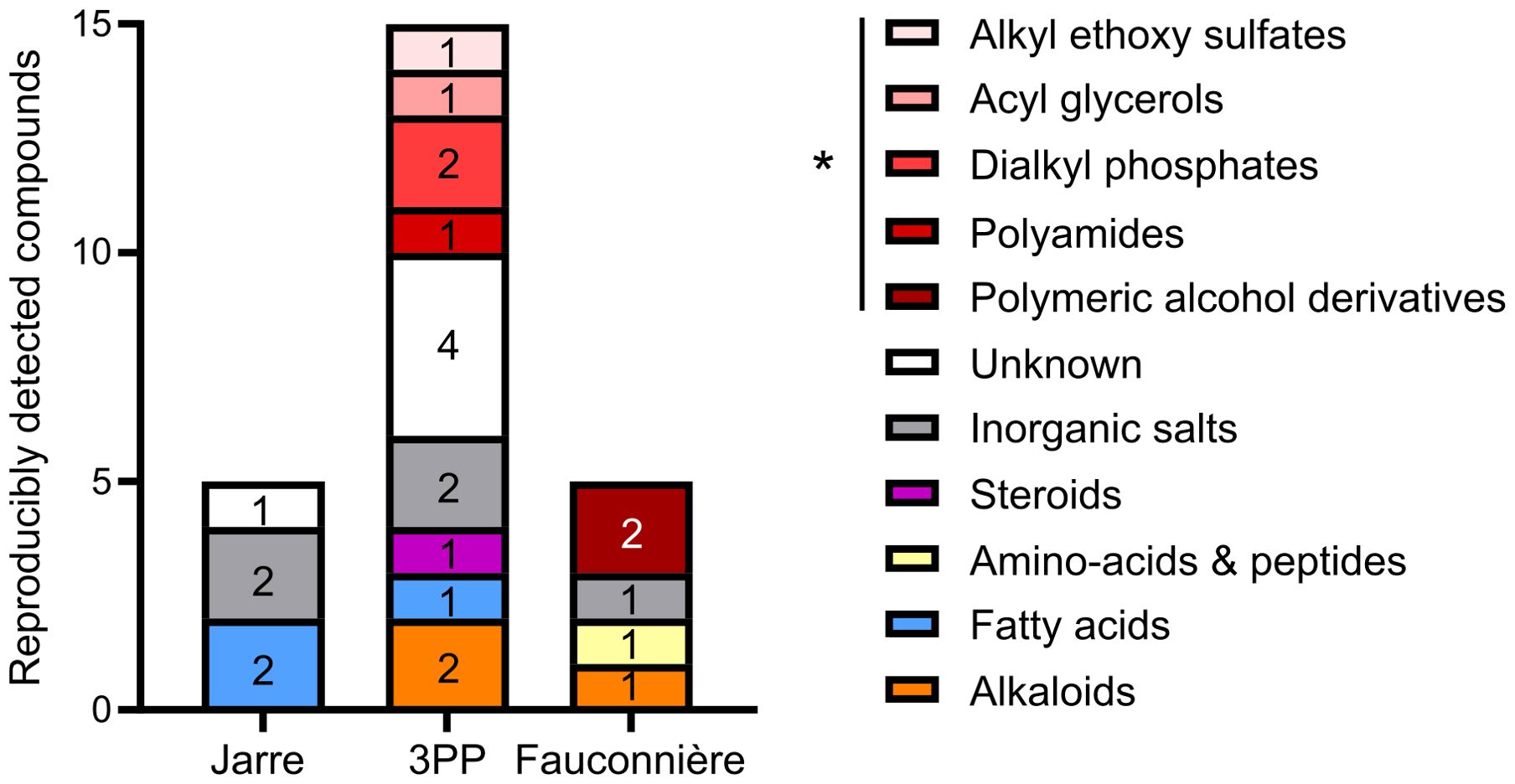
Figure 6. The barplots represent the chemical diversity of reproducibly detected compounds in each cave seawater. Structural classes were determined using outcomes from NP Classifier web interface, except for inorganic salts and substances suspected to be pollutants. *Compounds with a dubious biosynthetic origin possibly related to organic pollutants (e.g. surfactants, flame retardants).
3PP seawater harbored 3 times more reproducibly detected compounds (15) than Jarre and Fauconnière seawaters (5). The chemical composition of 3PP seawater appeared to be the most diversified, its reproducibly detected compounds belonging to 9 different structural classes. For Fauconnière and Jarre seawaters, their reproducibly detected compounds were distributed in only 4 and 2 different structural classes, respectively. For each cave seawater, more than a half of these molecules were related to either inorganic compounds or to organic pollutants (see Supplementary S6.1; S6.2; S6.3 for details). Among them, some pollutants were possibly related to surfactants (Acyl glycerols, Alkyl ethoxy sulfate), flame retardants (Dialkyl phosphates) and organic polymers such as nylon (Polyamides) and polyethylene glycol (Polymeric organic alcohol). Among the reported organic compounds that could derive from a biosynthetic origin, fatty acids were reproducibly found in the chemical signature of Jarre and 3PP. Alkaloids were detected in 3PP and Fauconnière seawaters. One peptide was reproducibly detected in Fauconnière seawater and putatively identified as cyclo(leucylleucyl). One steroid was reproducibly reported in 3PP seawater and annotated as sulfated cholesterol. MS data of these molecules and further details are available in Supplementary S6.1; S6.2; S6.3.
4 Discussion
Previous studies on Mediterranean caves led to the proposal of a first functioning model based on trophic exchanges (Russo and Bianchi, 2003; Rastorgueff et al., 2011, 2015). In this model, crustaceans living in dense swarms, such as mysids, are a keystone component driving organic matter transfers from the open sea to the back of the caves, thus mitigating the usual oligotrophy of the ecosystem. These horizontal migrations are similar to the vertical migrations of zooplankton (Hutchinson, 1967; Hays, 2003) and some pelagic mysid species (Viherluoto and Viitasalo, 2001) occurring at night. They navigate between the upper water column and deeper zones, a behavioral strategy to limit predation pressure while accessing to food resources (Lampert, 1993). Through their circadian migrations, whether vertical or horizontal, these small crustaceans are also vectors of organic matter supplying lower and upper levels of the trophic network (Rastorgueff et al., 2011; Kelly et al., 2019), thus playing an important role in the ecosystem functioning.
Few studies investigated the mechanisms behind the swimming behavior of cave-dwelling mysids. Previous laboratory observations (Macquart-Moulin, 1979; Bourdillon et al., 1980) showed that the movements of H. speluncola (a species closely related to H. margalefi, also found at 3PP cave) responded to light intensity. After in-situ experiments, Passelaigue and Bourdillon (1986) and then Riera et al. (1991) confirmed that light was the initial factor triggering the migration of H. speluncola. Other environmental factors could also influence the orientation (geotaxis) of migratory mysids such as temperature variation (Bourdillon and Castelbon, 1983) and current velocity (Crouau, 1986). Some studies also reported that chemical cues could influence mysid swimming behavior (Mauchline, 1980; Hamrén and Hansson, 1999). As chemical cues are among the main detection mechanisms involved in marine habitat recognition, notably in crustaceans (Boudreau et al., 1993; Castañeda-Fernández De Lara et al., 2005; Arvedlund and Kavanagh, 2009; Lecchini et al., 2010), it could be assumed that chemical mediation could also be involved in the habitat recognition of H. margalefi.
Santonja et al. (2018) tested this hypothesis in a previous study and showed that H. margalefi could significantly recognize a cave seawater or a seawater conditioned by syntopic cave-dwelling sponge species compared to a control seawater. This study suggested the possible involvement of recognized chemical signals from the mysid’s habitat. The present study intended to further investigate whether this recognition could be considered as a homing behavior and whether each cave displayed a singular chemical seascape that could play a role.
These new series of experiments showed that a given mysid population could recognize its home cave seawater. Furthermore, they showed no preference when exposed to a seawater from a different cave and a control seawater. These results suggest that the circadian migrations of H. margalefi, although most likely triggered by light, could use chemical recognition to ensure that mysids return to their home cave. Such homing behavior consequently constrains genetic exchanges with individuals from other caves. The consequences of homing behavior on the genetic structure of populations have already been demonstrated for salmons (Neville et al., 2006) and reef fishes (Gerlach et al., 2007; Salles et al., 2016) and could also contribute, in addition to parental brooding and natural habitat fragmentation, to explain the strong structuring of H. margalefi populations across Mediterranean caves (Lejeusne and Chevaldonné, 2006; Rastorgueff et al., 2014). To further explore the interactions between habitat recognition and genetic structuring of populations, it would be interesting to compare the behavioral responses of mysid populations with different degrees of genetic similarity (e.g. belonging to different caves within a single metapopulation or across genetic boundaries).
In the present study, we did not observe differences in behavioral responses between morning and afternoon, thereby suggesting that although H. margalefi homing must occur before daybreak, habitat chemical recognition might be independent of the time of the day. This result contrasts with those of Santonja et al. (2018) who recorded a greater capacity for habitat recognition during the morning experiments. Reproducibility of behavioral responses through time is a recurring problem in chemosensory experiments (Stamps et al., 2012; O’Neill et al., 2018; Hardege et al., 2024). Variations in time are anticipated among wild populations over the years separating our experiments. For example, microevolution in wild salmons can occur rapidly in response to global warming (Kovach, 2012; Piou and Prevost, 2013). In this sense, Kovach et al. (2012) demonstrated significant changes in genetic diversity associated to late-migration timing after only 17 generations. For H. margalefi, 4 cohorts are produced per year (Lejeusne and Chevaldonné, 2005), resulting in frequent turnover of the local population, which is increasingly exposed to environmental changes. This turnover may lead to small changes in the behavior of mysids, although the main migratory pattern is conserved over time.
Conspecific exometabolites in the seawater do not seem to influence the behavior of H. margalefi during its migrations. This result is in line with those from Lecchini et al. (2010), who showed that out of 9 studied crustaceans, 6 performed an active habitat selection for settlement without considering the presence or absence of conspecifics. They also found that in the case of conspecific attraction, visual cues are rather mobilized. These results strengthen the hypothesis of a homing behavior that could result from the detection of specific signals in the chemical seascape of their habitat.
Each of the three caves studied here harbors a specific chemical seascape. 3PP seawater is the most diverse, but also the one with the largest number of organic pollutants such as the alkyl ethoxy sulfate compound (Supplementary S6.3). This compound is a surfactant notably used as detergent and has been detected in river sediments after being released into the environment via wastewater (Sanderson et al., 2006). Its putative presence in the 3PP seawater samples could be explained by the proximity of the sewage outlet of La Ciotat city. Conversely, no organic pollutant was reproducibly found in Jarre, potentially because this cave, located on an island, has its entrance towards the offshore and is therefore less exposed to pollution. A few reproducibly detected compounds could derive from biological origin belonging to four chemical class: peptide, fatty acids, steroids and alkaloids. In crustaceans, peptides are reported to have signaling functions to coordinate homing and settlement (Rittschof and Cohen, 2004). Here, the peptide annotated as cyclo(leucylleucyl) has been reported in several bacterial taxa, including microbes associated with macroalgae (Anjali Das et al., 2023) but no ecological role has been associated with it so far. Lipids (Sakata et al., 1988) and steroids (Dulka et al., 1987) could also act as marine chemical cues. The sulfated fatty acid (molecular formula: C32H65NO6S) detected in Jarre may be a sphingolipid known from Cytophaga johnsona (Abbanat et al., 1988), bacteria commonly found in marine environments (Reichardt et al., 1983). In 3PP seawater, the reproducibly found steroid identified as a sulfated cholesterol (molecular formula C27H46O4S), represents a family of compound known from various benthic macroorganisms such as sea cucumbers, brittle stars (Levina et al., 1991; Makarieva et al., 1993) and sponges (Dai et al., 2010; Mioso et al., 2017). For instance, the amaroxocane B is a sulfated sterol produced by the coral reef sponge Phorbas amaranthus and interestingly, the Mediterranean sponge Phorbas tenacior is a common inhabitant of cave entrances (Grenier et al., 2018).
Santonja et al. (2018) hypothesized that 4 peptides and 4 oxylipin derivatives, potentially released by sponge holobionts, could play a major role in the habitat recognition for H. margalefi. In our dataset, we found 5 of these compounds, but they were neither reproducibly found (i.e. not present in all cave seawater replicates) nor specific to a particular cave (Supplementary S7), and thus did not appear in Figure 6. For instance, the peptide C24H44N4O4 was detected in Jarre (1/6 samples) and Fauconnière (1/6 samples) but never in 3PP seawater. Two oxylipins, C18H34O5 and C14H26O3, were found in 3PP (1/4 samples) and Fauconnière (1/6 samples and 3/6 samples) but never in Jarre seawater. Moreover, 2 compounds were detected in more than a half of the replicates of each of the 3 cave seawaters. The putative peptide C42H77N7O7 was reproducibly found in all seawater replicates of the 3 caves. The oxylipin C18H30O3 has been detected in 3PP (1/4 samples), Fauconnière (1/6 samples) and in Jarre (4/6 samples) but below the detection intensity limit (1E2, whereas the noise level for MS1 detection was fixed at 5E3). These last two compounds could thus be considered as cave chemical markers. The difficulty to reproducibly find the same dissolved compound, from one sample to another, has already been reported by Mauduit et al. (2023). In their study, they suggest that possible biotransformations could take place in the seawater for some structural families. Indeed, oxylipins are polyunsaturated fatty acids, which once dissolved in the seawater, may be subject to oxidation or hydroxylation of their carbon chain.
In conclusion, the present study provides new evidence that the migratory behavior of H. margalefi might mostly refer to daily-based homing rather than to an occasional habitat recognition. We suggest that this behavior could be related to a specific chemical recognition which is not due to the involvement of H. margalefi’s exudates, but rather to the presence of a singular combination of compounds from its home habitat. Each unique chemical seascape is composed of a complex mixture of compounds belonging to different structural classes, of possible natural origin. A more precise characterization of these chemical seascapes is difficult to achieve because a majority of compounds are present in trace amounts and the fate of the majority of biosynthesized metabolites in seawater is yet unknown. The referencing of marine structural diversity is widely documented in various molecular databases (e.g. Marinlit, Lotus). Accurate identification of already known molecule requires access to raw spectral data both MS and NMR. The paucity of freely available spectral data of marine natural products slow down the dereplication process (syn. Confirming the identify of already known compounds). Such identification is further complicated by seawater biotransformation that a waterborne metabolite may undergo. Freely available marine spectral databases are urgently needed to help decipher further the exometabolite composition of seawater.
Determining the origin of biosynthesized molecules and their contribution to chemical seascapes is truly crucial. The availability of new devices enabling to concentrate diluted molecules and to trace fingerprints of keystone species represent a new hope to show how chemical mediation plays a central role in marine ecosystems (Mauduit et al., 2023). In the current context of global change and major threats on marine biodiversity, any change in species composition and/or abundance (e.g. sponges or corals frequently subject to mortality events) is likely to impact the composition of the chemical seascape and thus marine ecosystem functioning.
Supporting information
The Supplementary Material contains further details on the experimental details for MS-based Metabolomic analyses, responses of mysids according to the time of the day (morning vs. afternoon) with detailed statistical results, Partial Least Squares Discriminant Analysis (PLS-DA) comparing chemical features detected in the control and cave seawater samples, detailed statistical results of PLSDA analysis of Figure 5A, the merged feature-based molecular network combining data acquired in both positive and negative modes with publicly accessible job ID, MS data of reproducible features with proposed structural classes and MS data of the compounds found in seawater from both our previous (Santonja et al., 2018) and current studies.
Data availability statement
The original contributions presented in the study are included in the article/Supplementary Material. Further inquiries can be directed to the corresponding author.
Ethics statement
The manuscript presents research on animals that do not require ethical approval for their study.
Author contributions
MD: Writing – original draft, Writing – review & editing. MS: Writing – original draft, Writing – review & editing. SG: Writing – original draft, Writing – review & editing. SF: Writing – original draft. CS: Writing – original draft, Writing – review & editing. PC: Writing – original draft, Writing – review & editing. TP: Writing – original draft, Writing – review & editing.
Funding
The author(s) declare that financial support was received for the research, authorship, and/or publication of this article. TOTAL Foundation ANR (ANR-707 20-CE43-0003) AMIDEX Foundation (AMX-22-IN1-46).
Acknowledgments
The authors are grateful to the TOTAL Foundation, the ANR (ANR-707 20-CE43-0003) and AMIDEX Foundation (AMX-22-IN1-46) for funding our research program. We warmly thank Laurent Vanbostal for his help in collecting samples. We thank J-C. Martin (BIOMET/CRIBIOM) for home-made filter scripts used to clean metabolomic data matrix and F. Mercier for the home-made Y-shaped glass deployed in our experiment.
Conflict of interest
The authors declare that the research was conducted in the absence of any commercial or financial relationships that could be construed as a potential conflict of interest.
Publisher’s note
All claims expressed in this article are solely those of the authors and do not necessarily represent those of their affiliated organizations, or those of the publisher, the editors and the reviewers. Any product that may be evaluated in this article, or claim that may be made by its manufacturer, is not guaranteed or endorsed by the publisher.
Supplementary material
The Supplementary Material for this article can be found online at: https://www.frontiersin.org/articles/10.3389/fmars.2024.1448616/full#supplementary-material
References
Abbanat D. R., Godchaux W., Leadbetter E. R. (1988). Surface-induced synthesis of new sulfonolipids in the gliding bacterium Cytophaga johnsonae. Arch. Microbiol. 149, 358–364. doi: 10.1007/BF00411656
Anjali Das C. G., Ganesh Kumar V., Dharani G., Stalin Dhas T., Karthick V., Vineeth Kumar C. M., et al. (2023). Macroalgae-associated halotolerant marine bacteria Exiguobacterium aestuarii ADCG SIST3 synthesized gold nanoparticles and its anticancer activity in breast cancer cell line (MCF-7). J. Mol. Liq. 383, 122061. doi: 10.1016/j.molliq.2023.122061
Arvedlund M., Kavanagh K. (2009). “The Senses and Environmental Cues Used by Marine Larvae of Fish and Decapod Crustaceans to Find Tropical Coastal Ecosystems,” in Ecological Connectivity among Tropical Coastal Ecosystems. Ed. Nagelkerken I. (Springer Netherlands, Dordrecht), 135–184. doi: 10.1007/978-90-481-2406-0_5
Atema J., Kingsford M., Gerlach G. (2002). Larval reef fish could use odour for detection, retention and orientation to reefs. Mar. Ecol. Prog. Ser. 241, 151–160. doi: 10.3354/meps241151
Boudreau B., Bourget E., Simard Y. (1993). Behavioural responses of competent lobster postlarvae to odor plumes. Mar. Biol. 117, 63–69. doi: 10.1007/BF00346426
Bourdillon A., Castelbon C. (1983). Influence des variations de température sur la géotaxie de deux espèces de mysidacés. J. Exp. Mar. Bio. Ecol. 71, 105–117. doi: 10.1016/0022-0981(93)90067-X
Bourdillon A., Castelbon C., Macquart-Moulin C. (1980). Écophysiologie comparée des mysidacés Hemimysis speluncola ledoyer (Cavernicole) et Leptomysis lingvura G.O. Sars (non-cavernicole). L’orientation à la lumière: Tests de longue durée. Étude expérimentale des mouvements nycthéméraux. J. Exp. Mar. Bio. Ecol. 43, 61–86. doi: 10.1016/0022-0981(80)90147-1
Brönmark C., Hansson L.-A. (2000). Chemical communication in aquatic systems: an introduction. Oikos 88, 103–109. doi: 10.1034/j.1600-0706.2000.880112.x
Burks R. L., Lodge D. M. (2002). Cued in: Advances and opportunities in freshwater chemical ecology. J. Chem. Ecol. 28, 1901–1917. doi: 10.1023/A:1020785525081
Carroll A. R., Copp B. R., Davis R. A., Keyzers R. A., Prinsep M. R. (2022). Marine natural products. Nat. Prod. Rep. 39, 1122–1171. doi: 10.1039/D1NP00076D
Cassier P., Descoins C., Bohatier J. (2000). Communication chimique et environnement: les relations animales intraspécifiques et interspécifiques (Paris: Belin).
Castañeda-Fernández De Lara V., Butler M., Hernández-Vázquez S., Guzmán Del Próo S., Serviere-Zaragoza E. (2005). Determination of preferred habitats of early benthic juvenile California spiny lobster, Panulirus interruptus, on the Pacific coast of Baja California Sur, Mexico. Mar. Freshw. Res. 56, 1037. doi: 10.1071/MF04284
Chambers M. C., Maclean B., Burke R., Amodei D., Ruderman D. L., Neumann S., et al. (2012). A cross-platform toolkit for mass spectrometry and proteomics. Nat. Biotechnol. 30, 918–920. doi: 10.1038/nbt.2377
Chevaldonné P., Lejeusne C. (2003). Regional warming-induced species shift in north-west Mediterranean marine caves. Ecol. Lett. 6, 371–379. doi: 10.1046/j.1461-0248.2003.00439.x
Coma R., Carola M., Riera T., Zabala M. (1997). Horizontal transfer of matter by a cave-dwelling mysid. Mar. Ecol. 18, 211–226. doi: 10.1111/j.1439-0485.1997.tb00438.x
Coppock A. G., Gardiner N. M., Jones G. P. (2013). Olfactory discrimination in juvenile coral reef fishes: Response to conspecifics and corals. J. Exp. Mar. Bio. Ecol. 443, 21–26. doi: 10.1016/j.jembe.2013.02.026
Crouau Y. (1986). Étude du comportement rhéotaxique d’un Mysidacé Cavernicole. Crustac 50, 7–10. doi: 10.1163/156854085X00026
Dai J., Sorribas A., Yoshida W. Y., Kelly M., Williams P. G. (2010). Topsentinols, 24-isopropyl steroids from the marine sponge Topsentia sp. J. Nat. Prod. 73, 1597–1600. doi: 10.1021/np100374b
David P. M., Guerin-Ancey O., Van Cuyck J. P. (1999). Acoustic discrimination of two zooplankton species (mysid) at 38 and 120kHz. Deep Sea Res. 46, 319–333. doi: 10.1016/S0967-0637(98)00064-8
Dührkop K., Fleischauer M., Ludwig M., Aksenov A. A., Melnik A. V., Meusel M., et al. (2019). SIRIUS 4: A rapid tool for turning tandem mass spectra into metabolite structure information. Nat. Methods 16, 299–302. doi: 10.1038/s41592-019-0344-8
Dulka J. G., Stacey N. E., Sorensen P. W., Kraak G. J. V. D. (1987). A steroid sex pheromone synchronizes male–female spawning readiness in goldfish. Nature 325, 251–253. doi: 10.1038/325251a0
Dyer L. A., Philbin C. S., Ochsenrider K. M., Richards L. A., Massad T. J., Smilanich A. M., et al. (2018). Modern approaches to study plant–insect interactions in chemical ecology. Nat. Rev. Chem. 2, 50–64. doi: 10.1038/s41570-018-0009-7
Endres C. S., Putman N. F., Ernst D. A., Kurth J. A., Lohmann C. M. F., Lohmann K. J. (2016). Multi-modal homing in sea turtles: modeling dual use of geomagnetic and chemical cues in island-finding. Front. Behav. Neurosci. 10. doi: 10.3389/fnbeh.2016.00019
Gerlach G., Atema J., Kingsford M. J., Black K. P., Miller-Sims V. (2007). Smelling home can prevent dispersal of reef fish larvae. Proc. Natl. Acad. Sci. U.S.A. 104, 858–863. doi: 10.1073/pnas.0606777104
Gerovasileiou V., Bianchi C. N. (2021). “Mediterranean Marine Caves: A Synthesis of Current Knowledge,” in Oceanography and Marine Biology. Ed. Hawkins S. J., et al (CRC Press, Boca Raton, FL), 1–87. doi: 10.1201/9781003138846-1
Grenier M., Ruiz C., Fourt M., Santonja M., Dubois M., Klautau M., et al. (2018). Sponge inventory of the French Mediterranean waters, with an emphasis on cave-dwelling species. Zootaxa, 4466, 205–228. doi: 10.11646/zootaxa.4466.1.16
Hamrén U., Hansson S. (1999). A mysid shrimp (Mysis mixta) is able to detect the odour of its predator (Clupea harengus). Ophelia 51, 187–191. doi: 10.1080/00785326.1999.10409408
Hardege J. D., Fletcher N., Burnett J. W., Ohnstad H., Bartels-Hardege H. D. (2024). Bioassay complexities exploring challenges in aquatic chemosensory research. Front. Ecol. Evol. 11. doi: 10.3389/fevo.2023.1293585
Harmelin J.-G., Vacelet J., Vasseur P. (1985). Les grottes sous-marines obscures: un milieu extrême et un remarquable biotope refuge. Téthys 1, 214–229.
Hay M. E. (2009). Marine chemical ecology: chemical signals and cues structure marine populations, communities, and ecosystems. Annu. Rev. Mar. Sci. 1, 193–212. doi: 10.1146/annurev.marine.010908.163708
Hays G. C. (2003). A review of the adaptive significance and ecosystem consequences of zooplankton diel vertical migrations. Hydrobiologia 503, 163–170. doi: 10.1023/B:HYDR.0000008476.23617.b0
Hentley W. T., Wade R. N. (2016). “Global Change, Herbivores and Their Natural Enemies,” in Global Climate Change and Terrestrial Invertebrates. Eds. Johnson S. N., Jones T. H. (Wiley, New York), 177–200. doi: 10.1002/9781119070894.ch10
Hervé M. (2021). Aide-mémoire de statistique appliquée à la biologie - Construire son étude et analyser les résultats à l’aide du logiciel R. Accessed December 13, 2021. Available online at: https://www.maximeherve.com/r-et-statistiques.
Horner A. J., Nickles S. P., Weissburg M. J., Derby C. D. (2006). Source and specificity of chemical cues mediating shelter preference of Caribbean spiny lobsters (Panulirus argus). Biol. Bull. 211, 128–139. doi: 10.2307/4134587
Hutchinson G. E. (1967). A treatise on limnology: Introduction to lake biology and the limnoplankton (New York: Wiley).
Kelly T. B., Davison P. C., Goericke R., Landry M. R., Ohman M. D., Stukel M. R. (2019). The importance of mesozooplankton diel vertical migration for sustaining a mesopelagic food web. Front. Mar. Sci. 6. doi: 10.3389/fmars.2019.00508
Kim H. W., Wang M., Leber C. A., Nothias L.-F., Reher R., Kang K. B., et al. (2021). NPClassifier: A deep neural network-based structural classification tool for natural products. J. Nat. Prod. 84, 2795–2807. doi: 10.1021/acs.jnatprod.1c00399
Kovach R. P. (2012). Salmonid phenology, microevolution, and genetic diversity in a warming Alaskan stream (University of Alaska: ProQuest Dissertations Publishing). Available at: https://go.exlibris.link/9x0ZhrpY.
Kovach R. P., Gharrett A. J., Tallmon D. A. (2012). Genetic change for earlier migration timing in a pink salmon population. Proc. Biol. Sci. 279, 3870–3878. doi: 10.1098/rspb.2012.1158
Lampert W. (1993).Ultimate causes of diel vertical migration of zooplankton: New evidence for the predator-avoidance hypothesis. Arch. Hydrobiol. Beih. Ergebn. Limnol. 39, 79–88. Available online at: https://pure.mpg.de/pubman/faces/ViewItemOverviewPage.jsp?itemId=item_1508686.
Lecchini D., Dixson D. L., Lecellier G., Roux N., Frédérich B., Besson M., et al. (2017). Habitat selection by marine larvae in changing chemical environments. Mar. pollut. Bull. 114, 210–217. doi: 10.1016/j.marpolbul.2016.08.083
Lecchini D., Mills S. C., Brié C., Maurin R., Banaigs B. (2010). Ecological determinants and sensory mechanisms in habitat selection of crustacean postlarvae. Behav. Ecol. 21, 599–607. doi: 10.1093/beheco/arq029
Lecchini D., Miura T., Lecellier G., Banaigs B., Nakamura Y. (2014). Transmission distance of chemical cues from coral habitats: implications for marine larval settlement in context of reef degradation. Mar. Biol. 161, 1677–1686. doi: 10.1007/s00227-014-2451-5
Lecchini D., Waqalevu V., Parmentier E., Radford C., Banaigs B. (2013). Fish larvae prefer coral over algal water cues: implications of coral reef degradation. Mar. Ecol. Prog. Ser. 475, 303–307. doi: 10.3354/meps10094
Lejeusne C., Chevaldonné P. (2005). Population structure and life history of Hemimysis margalefi (Crustacea: Mysidacea), a thermophilic cave-dwelling species benefiting from the warming of the NW Mediterranean. Mar. Ecol. Prog. Ser. 287, 189–199. doi: 10.3354/meps287189
Lejeusne C., Chevaldonné P. (2006). Brooding crustaceans in a highly fragmented habitat: the genetic structure of Mediterranean marine cave-dwelling mysid populations. Mol. Ecol. 15, 4123–4140. doi: 10.1111/j.1365-294X.2006.03101.x
Levina E. V., Fedorov S. N., Stonik V. A., Andriyashchenko P. V., Kalinovskii A. I., Isakov V. V. (1991). Steroid compounds from ophiuroids II. Sulfated steroids from Ophiura sarsi and Ophiura leptoctenia. Chem. Nat. Compd. 26, 408–411. doi: 10.1007/BF00598993
Macquart-Moulin C. (1979). Écophysiologie comparée des Mysidacés Hemimysis speluncola ledoyer (cavernicole) et Leptomysis lingvura G.O. Sars (non-cavernicole). L’orientation à la lumière: Tests ponctuels. J. Exp. Mar. Bio. Ecol. 38, 287–299. doi: 10.1016/0022-0981(79)90073-X
Macquart-Moulin C., Passelaigue F. (1982). Mouvements nychtéméraux d’Hemimysis speluncola Ledoyer, espèce cavernicole, et de Leptomysis lingvura GO Sars, espèce non-cavernicole (Crustacea, Mysidacea). Tethys 10, 221–228.
Makarieva T. N., Stonik V. A., Kapustina I. I., Boguslavsky V. M., Dmitrenoik A. S., Kalinin V. I., et al. (1993). Biosynthetic studies of marine lipids. 42. Biosynthesis of steroid and triterpenoid metabolites in the sea cucumber Eupentacta fraudatrix. Steroids 58, 508–517. doi: 10.1016/0039-128X(93)90026-J
Matsumura K., Nagano M., Fusetani N. (1998). Purification of a larval settlement-inducing protein complex (SIPC) of the barnacle, Balanus amphitrite. J. Exp. Zool. 281, 12–20. doi: 10.1002/(ISSN)1097-010X
Mauchline J. (1980). “The biology of mysids and euphausids,” in Advances in marine biology, ed. Blaxter J. H. S., Russell F. S., Yonge . (London: Academic Press) 3–369.
Mauduit M., Greff S., Herbette G., Naubron J.-V., Chentouf S., Huy Ngo T., et al. (2022). Diving into the Molecular Diversity of Aplysina cavernicola’s Exometabolites: Contribution of Bromo-Spiroisoxazoline Alkaloids. ACS Omega 7, 43068–43083. doi: 10.1021/acsomega.2c05415
Mauduit M., Derrien M., Grenier M., Greff S., Molinari S., Chevaldonné P., et al. (2023). In situ capture and real-time enrichment of marine chemical diversity. ACS Cent. Sci. 9, 2084–2095. doi: 10.1021/acscentsci.3c00661
Mioso R., Marante F., Bezerra R., Borges F., Santos B., Laguna I. (2017). Cytotoxic compounds derived from marine sponges. A reviereview, (2010–2012). Molecules 22, 208. doi: 10.3390/molecules22020208
Mollo E., Fontana A., Roussis V., Polese G., Amodeo P., Ghiselin M. T. (2014). Sensing marine biomolecules: smell, taste, and the evolutionary transition from aquatic to terrestrial life. Front. Chem. 2. doi: 10.3389/fchem.2014.00092
Mollo E., Garson M. J., Polese G., Amodeo P., Ghiselin M. T. (2017). Taste and smell in aquatic and terrestrial environments. Nat. Prod. Rep. 34, 496–513. doi: 10.1039/C7NP00008A
Munday P. L., Dixson D. L., Donelson J. M., Jones G. P., Pratchett M. S., Devitsina G. V., et al. (2009). Ocean acidification impairs olfactory discrimination and homing ability of a marine fish. Proc. Natl. Acad. Sci. U.S.A. 106, 1848–1852. doi: 10.1073/pnas.0809996106
Nelson C. E., Wegley Kelly L., Haas A. F. (2023). Microbial interactions with dissolved organic matter are central to coral reef ecosystem function and resilience. Annu. Rev. Mar. Sci. 15, 431–460. doi: 10.1146/annurev-marine-042121-080917
Neville H. M., Isaak D. J., Dunham J. B., Thurow R. F., Rieman B. E. (2006). Fine-scale natal homing and localized movement as shaped by sex and spawning habitat in Chinook salmon: insights from spatial autocorrelation analysis of individual genotypes. Mol. Ecol. 15, 4589–4602. doi: 10.1111/j.1365-294X.2006.03082.x
Nothias L.-F., Petras D., Schmid R., Dührkop K., Rainer J., Sarvepalli A. (2020). Feature-based molecular networking in the GNPS analysis environment. Nat Methods 17, 905–908. doi: 10.1038/s41592-020-0933-6
O’Neill S. J., Williamson J. E., Tosetto L., Brown C. (2018). Effects of acclimatisation on behavioural repeatability in two behaviour assays of the guppy Poecilia reticulata. Behav. Ecol. Sociobiol. 72, 166. doi: 10.1007/s00265-018-2582-7
Pang Z., Zhou G., Ewald J., Chang L., Hacariz O., Basu N., et al. (2022). Using MetaboAnalyst 5.0 for LC–HRMS spectra processing, multi-omics integration and covariate adjustment of global metabolomics data. Nat. Protoc. 17, 1735–1761. doi: 10.1038/s41596-022-00710-w
Parachnowitsch A. L., Manson J. S. (2015). The chemical ecology of plant-pollinator interactions: recent advances and future directions. Curr. Opin. Insect Sci. 8, 41–46. doi: 10.1016/j.cois.2015.02.005
Passelaigue F., Bourdillon A. (1986). Distribution and circadian migrations of the cavernicolous mysid Hemimysis speluncola Ledoyer. Stygologia 2, 112–118.
Piou C., Prévost E. (2013). Contrasting effects of climate change in continental vs. oceanic environments on population persistence and microevolution of Atlantic salmon. Glob. Change Biol. 19, 711–723. doi: 10.1111/gcb.12085
Quinlan Z. A., Bennett M.-J., Arts M. G. I., Levenstein M., Flores D., Tholen H. M., et al. (2023). Coral larval settlement induction using tissue-associated and exuded coralline algae metabolites and the identification of putative chemical cues. Proc. R. Soc B. 290, 20231476. doi: 10.1098/rspb.2023.1476
Rastorgueff P.-A., Bellan-Santini D., Bianchi C. N., Bussotti S., Chevaldonné P., Guidetti P., et al. (2015). An ecosystem-based approach to evaluate the ecological quality of Mediterranean undersea caves. Ecol. Indic. 54, 137–152. doi: 10.1016/j.ecolind.2015.02.014
Rastorgueff P.-A., Chevaldonné P., Arslan D., Verna C., Lejeusne C. (2014). Cryptic habitats and cryptic diversity: unexpected patterns of connectivity and phylogeographical breaks in a Mediterranean endemic marine cave mysid. Mol. Ecol. 23, 2825–2843. doi: 10.1111/mec.12776
Rastorgueff P., Harmelin-Vivien M., Richard P., Chevaldonné P. (2011). Feeding strategies and resource partitioning mitigate the effects of oligotrophy for marine cave mysids. Mar. Ecol. Prog. Ser. 440, 163–176. doi: 10.3354/meps09347
Reichardt W., Gunn B., Colwell R. R. (1983). Ecology and taxonomy of chitinoclastic Cytophaga and related chitin-degrading bacteria isolated from an estuary. Microb. Ecol. 9, 273–294. doi: 10.1007/BF02097742
Riera T., Zabala i Limousin M., Peñuelas J. (1991). Mysids from a submarine cave emerge each night to feed. Sci. Mar. 55, 605–609.
Rittschof D., Cohen J. H. (2004). Crustacean peptide and peptide-like pheromones and kairomones. Peptides 25, 1503–1516. doi: 10.1016/j.peptides.2003.10.024
Roggatz C. C., Saha M., Blanchard S., Schirrmacher P., Fink P., Verheggen F., et al. (2022). Becoming nose-blind—Climate change impacts on chemical communication. Glob. Change Biol. 28, 4495–4505. doi: 10.1111/gcb.16209
Russo G. F., Bianchi C. N. (2003). “Organizzazione trofica,” in Grotte Marine: Cinquant’Anni di Ricerca in Italia. Ed. Cicogna F., et al (Ministero dell’Ambiente e della Tutela del Territorio, Rome), 313–320.
Saha M., Berdalet E., Carotenuto Y., Fink P., Harder T., John U., et al. (2019). Using chemical language to shape future marine health. Front. Ecol. Environ. 17, 530–537. doi: 10.1002/fee.2113
Sakata K., Sakura T., Ina K. (1988). Algal phagostimulants for marine herbivorous gastropods. J. Chem. Ecol. 14, 1405–1416. doi: 10.1007/BF01020144
Salles O. C., Pujol B., Maynard J. A., Almany G. R., Berumen M. L., Jones G. P., et al. (2016). First genealogy for a wild marine fish population reveals multigenerational philopatry. Proc. Natl. Acad. Sci. U.S.A. 113, 13245–13250. doi: 10.1073/pnas.1611797113
Sanderson H., Price B. B., Dyer S. D., De Carvalho A. J., Robaugh D., Waite S. W., et al. (2006). Occurrence and hazard screening of alkyl sulfates and alkyl ethoxysulfates in river sediments. Sci. Total Environ. 367, 312–323. doi: 10.1016/j.scitotenv.2005.11.021
Santonja M., Greff S., Le Croller M., Thomas O. P., Pérez T. (2018). Distance interaction between marine cave-dwelling sponges and crustaceans. Mar. Biol. 165, 121. doi: 10.1007/s00227-018-3377-0
Schmid R., Heuckeroth S., Korf A., Smirnov A., Myers O., Dyrlund T. S., et al. (2023). Integrative analysis of multimodal mass spectrometry data in MZmine 3. Nat. Biotechnol. 41, 447–449. doi: 10.1038/s41587-023-01690-2
Scholz A. T., Horrall R. M., Cooper J. C., Hasler A. D. (1976). Imprinting to chemical cues: the basis for home stream selection in salmon. Science 192, 1247–1249. doi: 10.1126/science.1273590
Schymanski E. L., Jeon J., Gulde R., Fenner K., Ruff M., Singer H. P., et al. (2014). Identifying small molecules via high resolution mass spectrometry: communicating confidence. Environ. Sci. Technol. 48, 2097–2098. doi: 10.1021/es5002105
Shannon P., Markiel A., Ozier O., Baliga N. S., Wang J. T., Ramage D., et al. (2003). Cytoscape: A software environment for integrated models of biomolecular interaction networks. Genome Res. 13, 2498–2504. doi: 10.1101/gr.1239303
Stabell O. B. (1992). “Olfactory control of homing behaviour in salmonids,” in Fish Chemoreception. Ed. Hara T. J. (Springer Netherlands, Dordrecht), 249–270. doi: 10.1007/978-94-011-2332-7_12
Stamps J. A., Briffa M., Biro P. A. (2012). Unpredictable animals: individual differences in intraindividual variability (IIV). Anim. Behav. 83, 1325–1334. doi: 10.1016/j.anbehav.2012.02.017
Ternon E., Zarate L., Chenesseau S., Croué J., Dumollard R., Suzuki M. T., et al. (2016). Spherulization as a process for the exudation of chemical cues by the encrusting sponge C. crambe. Sci. Rep. 6, 29474. doi: 10.1038/srep29474
Viherluoto M., Viitasalo M. (2001). Effect of light on the feeding rates of pelagic and littoral mysid shrimps: a trade-off between feeding success and predation avoidance. J. Exp. Mar. Bio. Ecol. 261, 237–244. doi: 10.1016/S0022-0981(01)00277-5
Wegley Kelly L., Nelson C. E., Petras D., Koester I., Quinlan Z. A., Arts M. G. I., et al. (2022). Distinguishing the molecular diversity, nutrient content, and energetic potential of exometabolomes produced by macroalgae and reef-building corals. Proc. Natl. Acad. Sci. U.S.A. 119, e2110283119. doi: 10.1073/pnas.2110283119
Wilke C. (2021). Climate change could alter undersea chemical communication. ACS Cent. Sci. 7, 1091–1094. doi: 10.1021/acscentsci.1c00819
Wyatt T. D. (2010). Pheromones and signature mixtures: defining species-wide signals and variable cues for identity in both invertebrates and vertebrates. J Comp Physiol A 196, 685–700. doi: 10.1007/s00359-010-0564-y
Keywords: marine ecology, chemical seascape, homing behavior, underwater cave, Mysida
Citation: Derrien M, Santonja M, Greff S, Figueres S, Simmler C, Chevaldonné P and Pérez T (2024) Circadian migrations of cave-dwelling crustaceans guided by their home chemical seascape. Front. Mar. Sci. 11:1448616. doi: 10.3389/fmars.2024.1448616
Received: 13 June 2024; Accepted: 02 August 2024;
Published: 17 September 2024.
Edited by:
Elva G. Escobar-Briones, National Autonomous University of Mexico, MexicoReviewed by:
Ernesto Maldonado, National Autonomous University of Mexico, MexicoMarcel Jaspars, University of Aberdeen, United Kingdom
Copyright © 2024 Derrien, Santonja, Greff, Figueres, Simmler, Chevaldonné and Pérez. This is an open-access article distributed under the terms of the Creative Commons Attribution License (CC BY). The use, distribution or reproduction in other forums is permitted, provided the original author(s) and the copyright owner(s) are credited and that the original publication in this journal is cited, in accordance with accepted academic practice. No use, distribution or reproduction is permitted which does not comply with these terms.
*Correspondence: Thierry Pérez, thierry.perez@imbe.fr
†These authors have contributed equally to this work and share first authorship