- 1Conservation Decisions Lab, Department of Forest and Conservation Sciences, University of British Columbia, Vancouver, BC, Canada
- 2Comox Valley Project Watershed, Courtenay, BC, Canada
- 3University of Washington, Friday Harbor Labs, Friday Harbor, WA, United States
- 4The Loon Foundation, Madeirda Park, BC, Canada
- 5Peninsula Streams Society, North Saanich, BC, Canada
- 6SeaChange Marine Conservation Society, Victoria, BC, Canada
- 7Department of Biological Sciences, University of Manitoba, Winnipeg, MB, Canada
- 8Washington Department of Fish and Wildlife, Olympia, WA, United States
- 9Environment and Climate Change Canada, Wildlife Research Division, Delta, BC, Canada
- 10Department of Geography, University of Victoria, Victoria, BC, Canada
- 11Canadian Wildlife Service, Environment and Climate Change Canada, Sackville, NB, Canada
- 12National Oceanic and Atmospheric Administration, National Marine Fisheries Service, West Coast Region, Protected Resources Division, Lacey, WA, United States
- 13Department of Bioscience, University of Oslo, Oslo, Norway
- 14Tsleil-Waututh Nation/tə səlilwətaɬ xʷəlməxʷ, North Vancouver, BC, Canada
- 15Fisheries and Oceans Canada, Pacific Biological Station, Nanaimo, BC, Canada
- 16Fisheries, Marine Ecology and Conservation Lab, Department of Biology, University of Victoria, Victoria, BC, Canada
- 17Marine Science Program, Pacific Salmon Foundation, Vancouver, BC, Canada
- 18Fisheries and Oceans Canada, Institute of Ocean Sciences, Sidney, BC, Canada
- 19Sunshine Coast Friends of Forage Fish, Sechelt, BC, Canada
- 20Natural Resources Consultants, Seattle, WA, United States
- 21Coastal Watershed Institute, Port Angeles, WA, United States
- 22Pacific Rim National Park Reserve, Ucluelet, BC, Canada
Like many forage fish species, Pacific sand lance (Ammodytes personatus) play a key role in nearshore marine ecosystems as an important prey source for a diverse array of predators in the northeastern Pacific. However, the primary threats to Pacific sand lance and their habitat are poorly defined due to a lack of systematic data. Crucial information needed to assess their population status is also lacking including basic knowledge of their local and regional abundance and distribution. Sand lance are currently listed as ‘not evaluated’ under the IUCN red list and they have not been assessed by US and Canadian agencies. This hampers management and policy efforts focused on their conservation. To address this knowledge gap, we conducted a three-part, structured expert elicitation to assess the vulnerability of Salish Sea sand lance populations. Experts were asked to list and rank key threats to Salish Sea sand lance and/or their habitat, to further quantify the vulnerability of sand lance to identified threats using a vulnerability matrix, and to predict the population trajectory in 25 years from today. Impacts associated with climate change (e.g. sea level rise, sea temperature rise, ocean acidification, and extreme weather) consistently ranked high as threats of concern in the ranking exercise and quantified vulnerability scores. Nearly every expert predicted the population will have declined from current levels in 25 years. These results suggest sand lance face numerous threats and may be in decline under current conditions. This research provides vital information about which threats pose the greatest risk to the long-term health of sand lance populations and their habitat. Managers can use this information to prioritize which threats to address. Future research to reliably quantify population size, better understand the roles of natural and anthropogenic impacts, and to identify the most cost-effective actions to mitigate multiple threats, is recommended.
Introduction
Pacific sand lance, Ammodytes personatus (hereafter, sand lance), are small forage fish that play a significant role in the nearshore ecosystem of the northeast Pacific Ocean. Sand lance are known to comprise part of the diet for at least 100 predators (Robards and Piatt, 1999; Penttila, 2007; Harvey et al., 2010; Alheit and Peck, 2019; Staudinger, 2020; Scordino et al., 2022; Shaffer et al., 2023). Examples include seabirds, especially Alcids (Robards and Piatt, 1999; Zamon, 2000; Pastran et al., 2021); fish such as Chinook (Oncorhynchus tshawytscha) and coho salmon (Oncorhynchus kisutch; Duguid, 2020), Pacific cod (Gadus macrocephalus; Gunther et al., 2023), and lingcod (Ophiodon elongatus; Beaudreau and Essington, 2007); and larger mammals like Steller sea lions (Eumetopias jubatus; McKenzie and Wynne, 2008), harbor seals (Phoca vitulina richardii; Lance et al., 2012), and baleen whales such as humpback (Megaptera novaeangliae; Wright et al., 2016), minke (Balaenoptera acutorostrata; Okamura et al., 2009; Towers et al., 2019) and fin (Balaenoptera physalus; Moore et al., 2019). They play a particularly important role during the breeding of seabirds because of the high energy content, and slender bodies that are easily transported to, and consumed by, chicks (Willson et al., 1999; Bertram et al., 2001; Hedd et al., 2006; Beaubier and Hipfner, 2013). Sand lance range from California to Alaska and are one species within the Ammodytes genus, the only species that occurs in the Salish Sea, and one of two in the eastern Pacific (Robards and Piatt, 1999; Orr et al., 2015). They are dependant on specific spawning and burying habitats that must have coarse, silt-free, well oxygenated sandy substrates (hereafter “habitat”) (Baker et al., 2024). Declines in sand lance abundance could have serious rippling impacts on coastal ecosystems given their important role in the food web (Bertram et al., 2001; Robards et al., 2002; Piatt et al., 2020).
Piecemeal observations by researchers, including some of the authors, Indigenous groups, and anecdotal reports from recreational anglers in the northeastern Pacific over the last decade have resulted in a growing concern that forage fish, including sand lance populations, face numerous threats (Dethier et al., 2016; Frick et al., 2022; Robinson et al., 2023). While some research has been undertaken on their biology (Penttila, 2007; Haynes et al., 2008; Haynes and Robinson, 2011; Hipfner and Galbraith, 2013; Matta and Baker, 2020; Zhukova and Baker, 2022; Robinson et al., 2023), distribution and seasonal abundance (Selleck et al., 2015), and habitat distribution (Robinson, 2013; Baker et al., 2019; Robinson et al., 2021; Huard et al., 2022; Baker et al., 2023; Gunther et al., 2023), there is a lack of comprehensive empirically derived information to contextualize their population status at a scale as wide as the Salish Sea.
Sand lance have an uneven distribution throughout their range, often restricted to small, specific, and patchy habitats that are not well mapped (Haynes et al., 2008; Robinson et al., 2013; Speed and Baker, 2016; Greene et al., 2020; Robinson et al., 2021; Huard et al., 2022). Their occurrence is temporally variable (Selleck et al., 2015) and they are dormant much of the winter (van Deurs et al., 2010; Haynes and Robinson, 2011; Robinson et al., 2013; Greene et al., 2015; Baker et al., 2019, 2023). Accurate estimates employed in the North Sea require use of both acoustics and nets at great cost with imperfect results (Greenstreet et al., 2006, 2010). There has been limited species-specific population assessments at the Salish Sea scale, and one projecting forward in the US side of the Salish Sea. In Washington State, field surveys targeting the San Juan Channel sand wavefield (approximately 0.6 km2) have occurred over the past 15 years estimating that there is an average of 81 million sand lance (Blaine, 2006; Baker et al., 2019; Baker et al., 2024); however, these surveys are limited to one area and do not speculate on population trends (Speed and Baker, 2016; Greene et al., 2020, Greene et al., 2021). The National Oceanic and Atmospheric Administration (NOAA) has conducted research on abundance trend estimates for several forage fish within Puget Sound and found sand lance populations increased in some basins over the study time (1971-1985; 2002-2003) (Greene et al., 2015). A handful of additional studies relating to Pacific sand lance in the Salish Sea exist, however they lack specific temporal, spatial, or species-specific assessments to be useful to understanding the sand lance population trends (Penttila, 1995; Selleck et al., 2015) (Tomlin et al., 2021) (Bertram and Kaiser, 1993; Hedd et al., 2006; Thayer et al., 2008) (Gunther et al., 2023), (Duguid, 2020; Baker et al., 2021) (Einoder, 2009). The historical lack of research conducted on sand lance may have contributed to the lack of knowledge and research into population status as it can be difficult to acquire research funding if there is no/little demonstratable risk to the species or ecosystem.
There are multiple, potentially interacting threats on sand lance in the Salish Sea (Krueger et al., 2010; Hipfner et al., 2018; Baker et al., 2019; Buchanan et al., 2019; Liedtke and Conn, 2021; Selden and Baker, 2023). The Salish Sea is a densely populated area on the Northeastern Pacific coast where rapid anthropogenic growth and development is exerting increasing pressure on regional biodiversity (Gaydos and Pearson, 2011; Gaydos et al., 2015; Singh et al., 2017). Anthropogenic activities in the Salish Sea have the potential to negatively impact sand lance populations and habitat, which could result in cascading effects (Staudinger, 2020). As a species with an obligate association to very specific, rare habitats, sand lance are particularly exposed to impacts from habitat loss and degradation (Pearson et al., 1984; Quinn, 1999; Robinson et al., 2013; Bizzarro et al., 2016; Huard et al., 2022; Smith and Liedtke, 2022).
While many threats have been documented individually, including the vulnerability of sand lance to climate change (Hare et al., 2016; Rovellini et al., 2024), there is no comprehensive list of threats acting on sand lance. These data deficiencies make it challenging to understand pressures on sand lance, and to prioritize management actions to abate threats. Evaluating the key threats would help to understand drivers of decline and inform decisions to conserve and manage sand lance.
In this study, we sought to overcome these challenges through the use of expert judgement to identify a comprehensive list of threats to their persistence, including a ranking of the vulnerability of sand lance to those threats, and to develop a hypothesis for Salish Sea sand lance population trends. Structured expert elicitation is routinely applied in conservation and decision-making contexts, when data are incomplete or unavailable, and time and resources to collect such data are limited (Wolfson et al., 1996; Harwood, 2000; Wilson et al., 2005; De Lange et al., 2010; Martin et al., 2012; Burgman et al., 2015; The Salish Sea Pacific Herring Assessment and Management Strategy Team, 2018). Structured approaches are designed to facilitate the elicitation of expert judgement in such a way that common biases are mitigated, and the resulting data conform to the same level of empirical control and transparency afforded to other forms of empirical data and have been shown to improve the accuracy and calibration of results (Hemming et al., 2018; Camaclang et al., 2021; Hanea et al., 2022).
Ranking the potential impact of threats requires a clear definition of each and connecting such impacts with putative individual or population-level effects across a range of exposure levels (O’Hagan, 2019). Direct ranking methods are often expedient, but can over-rely on the opinions of experts, and may result in availability biases, with threats that are more easily recalled, or more familiar to experts, being listed higher than other threats (e.g., Donlan et al., 2010). Indirect methods, by contrast, identify explicit criteria for assessing the magnitude of any threat, and can be more time consuming, but may guard against availability bias. In ecology, vulnerability is often categorized into exposure, sensitivity, and resilience. However; there are a number of different approaches (Van Straalen, 1993; Turner et al., 2003; Wilson et al., 2005; De Lange et al., 2010; Speirs-Bridge et al., 2010; Beroya-Eitner, 2016; Berrouet et al., 2018; Hou et al., 2022) requiring a choice to be made about the best criteria to represent vulnerability for the species and ecosystems in question. We used both indirect and direct ranking methods to help order the list of threats, choosing to adapt the methods and vulnerability scores developed by Halpern et al. (2007) and Teck et al. (2010); and repeated by Grech et al. (2011) and Kappel et al. (2012) as applied in the marine realm to the top ranked threats of concern. The focus of our study was to expand the knowledge of sand lance and their habitats to support ecosystem conservation. Specifically, we aimed to:
1. Create a list of threats to sand lance habitats and populations;
2. Rank the threats through a vulnerability assessment to identify those with the highest potential to reduce populations and/or their critically required habitat; and
3. Generate a hypothesis on the population trajectory of sand lance.
Methods
We applied the IDEA protocol for structured expert elicitation (Investigate, Discuss, Estimate, and Aggregate); (Hanea et al., 2018; Hemming et al., 2018). We began our assessment of threats to sand lance with a literature review to identify a list of threats. We then supplemented this list through an expert elicitation. This aimed to overcome biases in the literature due to publication biases, such as demographic biases, as well as lags in the literature whereby emerging threats may not have been documented or sufficiently studied for publication (Baum and Martin, 2018).
This research was undertaken under the University of British Columbia Human Ethics H19-01635 for the Salish Sea Cumulative Threats project, led by Dr. Tara Martin. The expert elicitation took place from January – April 2021, using remote elicitation. To address our questions, we administered three sequential surveys (Surveys were delivered through Qualtrics [https://www.qualtrics.com/]), through following the workflow of:
● Recruitment of experts.
● Survey 1: Initial review of threats and definitions.
● Videoconference: Provide feedback on Survey 1 and introduce process for Surveys 2 and 3.
● Survey 2: Initial estimate of population size and vulnerability assessment of threats.
● Survey 3: Review of Survey 2 results and finalize estimates.
Recruitment of experts
Species and/or habitat experts were identified based on literature searches (including grey literature), word-of-mouth, and personal experience of the authors. A snowball technique was also used where invited participants were asked to forward the participation request to other potential knowledge holders. Anyone who self-identified as being knowledgeable on sand lance or on habitats in the Salish Sea was invited to participate. Over 80 invitations were extended, invitations (via e-mail) can be found in the Supplementary Material.
Participants were from federal government agencies (28.5%, 11), not-for-profit organizations or non-governmental organizations (23%, 7), academic institutions (20.5%, 2), consulting firms (7.8%, 1), First Nation governments (staff not necessarily Indigenous identifying individuals) (2.6%, 1), First Nation non-governmental organizations (staff not necessarily Indigenous identifying individuals) (2.6%, 1), and Other (5.1%, 2). Respondents identified as men (39.5%, 15), women (44.7%, 17), and prefer not to answer (15.8%, 7). Participants ranged in age from 25 to 75 years old, with a majority falling into the 25 to 34, and 35 to 44 years old bands. Four participants [10.3%] chose not to respond to the age demographic question. Respondents identified as White (70.5%), Hispanic or Latino (2.6%), and prefer not to answer (26.9%). When asked about their type of expertise, 71.8% of respondents identified having knowledge of sand lance; 10% identified having knowledge of habitats; 5.4% selected both of the previous two categories; and 12.8% identified having related but other knowledge (such as knowledge of forage fishes in general in the Salish Sea, and/or knowledge of species that rely on sand lance). When asked about geographical area of expertise, 64.1% identified as being most familiar with the Canadian Salish Sea (i.e., British Columbia), 25.6% identified as being most familiar with the American Salish Sea (i.e., Washington State), 7.7% identified as being familiar with the entire Salish Sea, and 2.6% identified as not having familiarity with the Salish Sea but feeling they were able to provide insight to threats regardless of location.
Survey 1 – threat identification
An initial list of 20 potential threats to sand lance and/or their habitats was developed from a literature review (see Supplementary Table S1 for initial threat list). Each threat was accompanied by a definition of the threat. This list was reviewed by a small group of five experts to further reduce linguistic ambiguity and compiled into Survey 1 for review by 39 experts. In Survey 1 experts were first asked to review the initial list of threats and, if desired, provide and describe up to five additional threats. They were then asked to categorize the threats into one of three broad tiers of impact to sand lance: Of Concern, Least Concern, and Uncertain. Within each of the three tiers, experts were asked to directly rank threats in order of greatest to least risk, with no ties permitted, to the persistence of sand lance and/or to the persistence of their habitats in the Salish Sea. This was used to help generate a Cumulative Rank Score (CRS) across experts and threats (See Supplementary Material for calculation methods).
A 1-hour video conference was held on January 23, 2022. During the video conference, participants were formally introduced to the project and reviewed and discussed the 20 threats of concern. They were then informed of the process for completing Surveys 2 and 3 and invited to raise any questions or concerns. Following the video call, the list of threats to include in Survey 2 was reorganized, reworded, and in some cases expanded or collapsed based on the discussions.
Survey 2: vulnerability assessment and expert population trajectory assessment
Survey 2 was split into two parts: i) an assessment of the population trajectory; and ii) the relative quantification of vulnerability scores for the top threats.
Vulnerability assessment
For the vulnerability assessment (Survey 2), we sought to reduce the number of threats provided from Survey 1 to avoid expert fatigue. To determine the list of threats to be assessed, we ranked the 42 threats that received at least one vote as being a threat Of Concern by the CRS score (See Supplementary Table S3). Following the survey, the top 20 threats categorized as being Of Concern were utilized (see Supplementary Material for specific methods used Supplementary Table S2). The final 20 threats under evaluation were divided into two groups (A and B) each with 10 threats. Participants were then assigned to either group based on the threats they indicated had the most familiarity with as indicated in Survey 1. Group A had 22 participants assigned to it and Group B had 21. A copy of Survey 2 can be found in the Supplementary Material S2.
Experts were also asked to characterize the level of certainty for their responses to a given threat and allowed space to provide caveats and qualifiers to contextualize their responses. The specific questions asked in the survey are shown in Table 1.
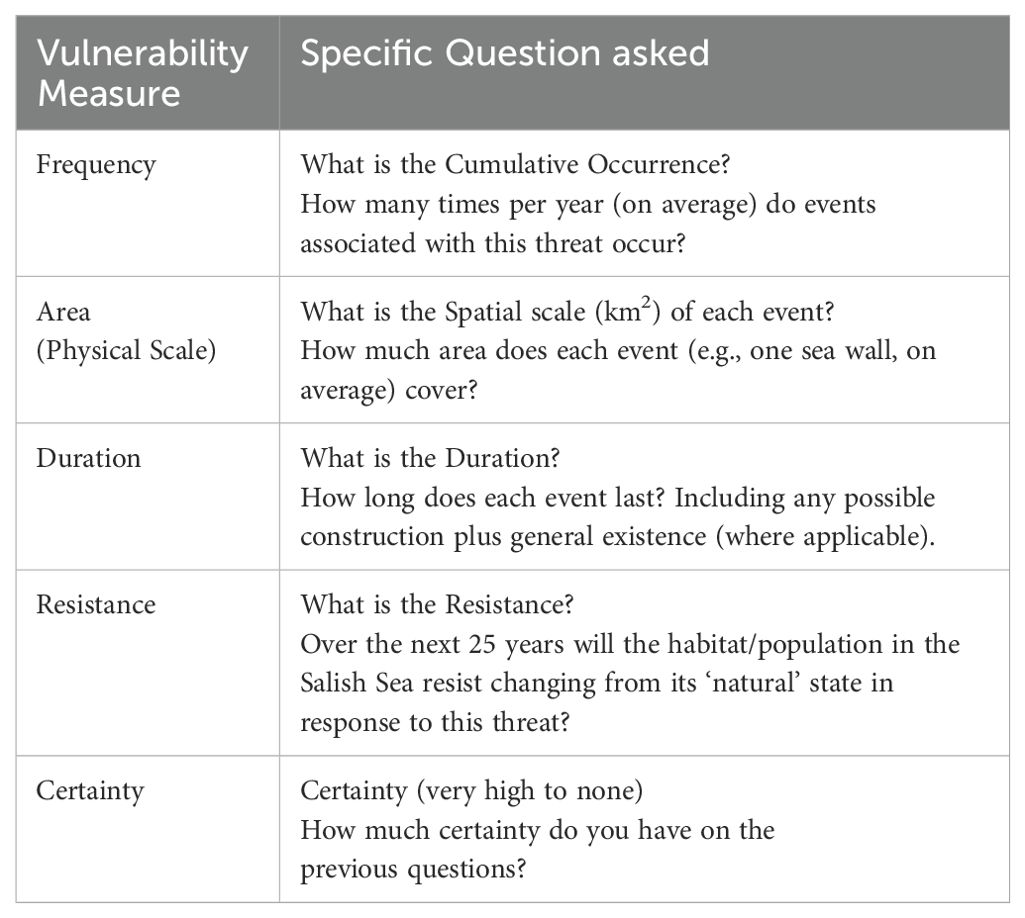
Table 1. Vulnerability measures assessed in Survey 2 in the expert elicitation of threats to sand lance and marine sand habitats in the Salish Sea.
As per Halpern et al. (2007) and Teck et al. (2010), experts were asked to consider the vulnerability criteria for each threat on an annual basis, and assign a relative value (0-4 or 0-6) using a predefined drop-down list (Table 2). Data from expert responses were used to develop a weighted average vulnerability score to represent a relative measure of how vulnerable sand lance and their habitats are to each threat. This approach to calculating relative vulnerability of sand lance is mathematically represented as
where Si,k is the value of threat i and a vulnerability measure k, and W is the weight assigned to vulnerability measure k, such that Wk≥0, ∑k=1,…,4Wk=1. The weights are normalized so that they sum to one and were empirically derived using a multicriteria decision model. Resistance was estimated to explain 66.5% of the vulnerability (Teck et al., 2010), when experts were assessing the vulnerability of various scenarios with pre-determined values and, therefore, we used 0.665 for the weighting of resistance. For the weighting of the three scale-related criteria, we assumed that frequency, area, and duration were of equal weights and used 1 – 0.665 = 0.334 divided by 3 to give each a weight of 0.112.
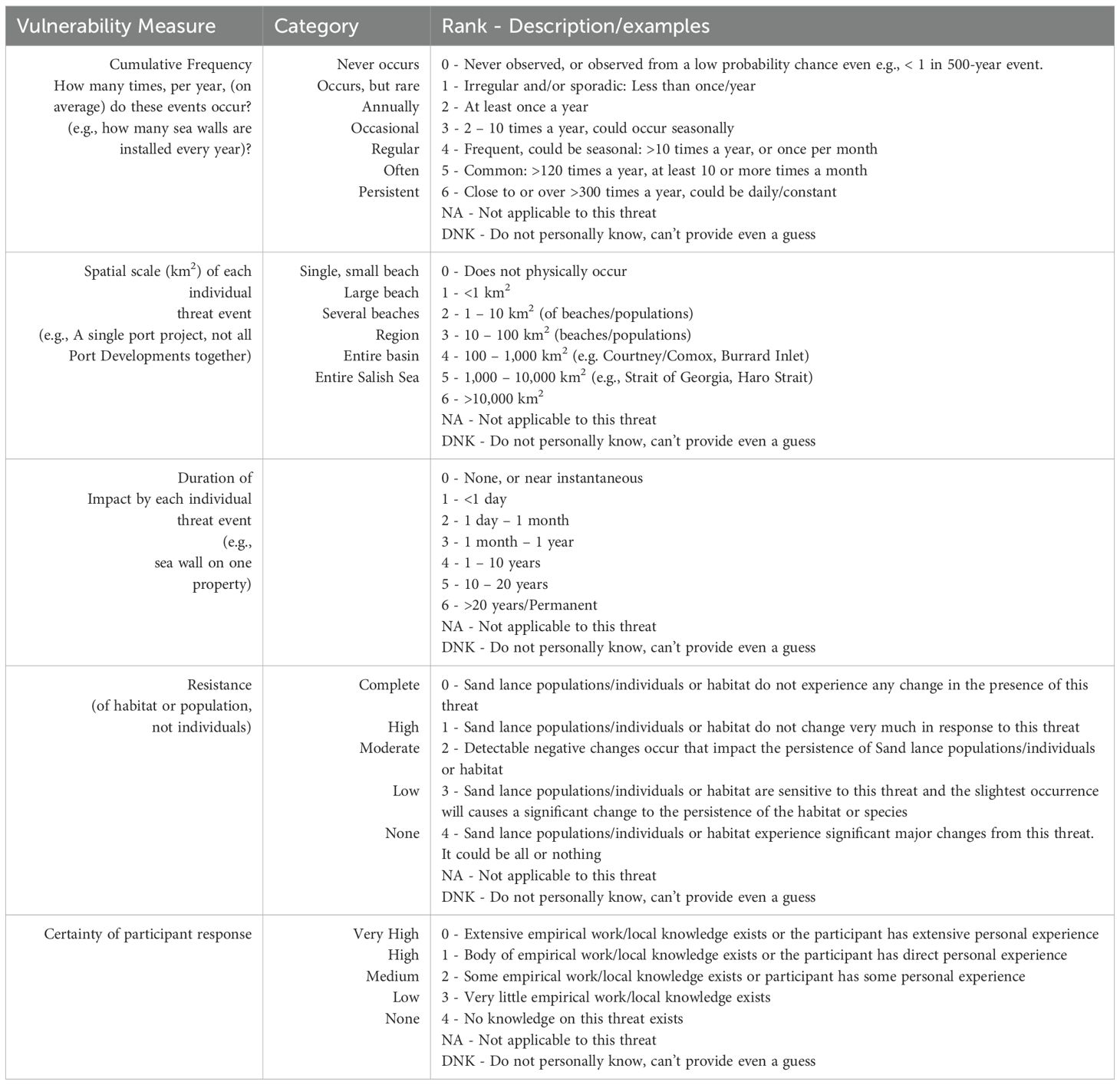
Table 2. Ranking system for vulnerability measures to assess how threats affect sand lance and/or coarse, silt- free sand, habitats.
Frequency, area, and duration vulnerability measure results were standardized to have the same 0 to 4 scale (by multiplying the vulnerability value by 4/6) so they are comparable and were all given equal weight to resistance. Then, for each expert’s vulnerability score, the values were divided by the highest possible score (4) to give a value between 0 and 1. Values closer to 1 represent higher estimated vulnerability to that threat and values closer to 0, lower vulnerability. For each threat, the mean and confidence intervals across the experts were calculated to provide a single weighted vulnerability score. The mean and confidence intervals were calculated using the DescTools package in R, ‘meanCI’ function using bootstrapping methods. The confidence intervals are the 2.5th and 97.5th centiles, or a 95% confidence interval of an equi-tailed, two-sided, nonparametric interval using a basic bootstrap interval.
Population trajectory assessment
There were concerns that quantifying the trajectory of sand lance may be too onerous for the experts to accurately assess given the species’ cryptic nature, boom-and-bust episodic reproductive cycles, and the lack of a stock assessment. To overcome these issues, the team elicited population trajectory predictions using questions of increasing resolution. Experts were asked to consider a hypothetical survey program that had averaged 100 fish per survey each year over the previous five years. Experts were then asked to consider if the same population in the Salish Sea was evaluated 25 years from present (year 2047) would there be an increase, decrease, or no change relative to the current five-year average. The timeframe of 25 years was used because it encompasses multiple generations of the sand lance life cycle and is within the realm of experience that can be reasonably predicted by expert participants. This question was accompanied by a visual image of potential changes in sand lance (Figure 1). The purpose of this framing was to convey population changes of sand lance in a hypothetically observable and meaningful quantity for experts (a key criterion for structured expert elicitation questions [Hemming et al., 2018]).
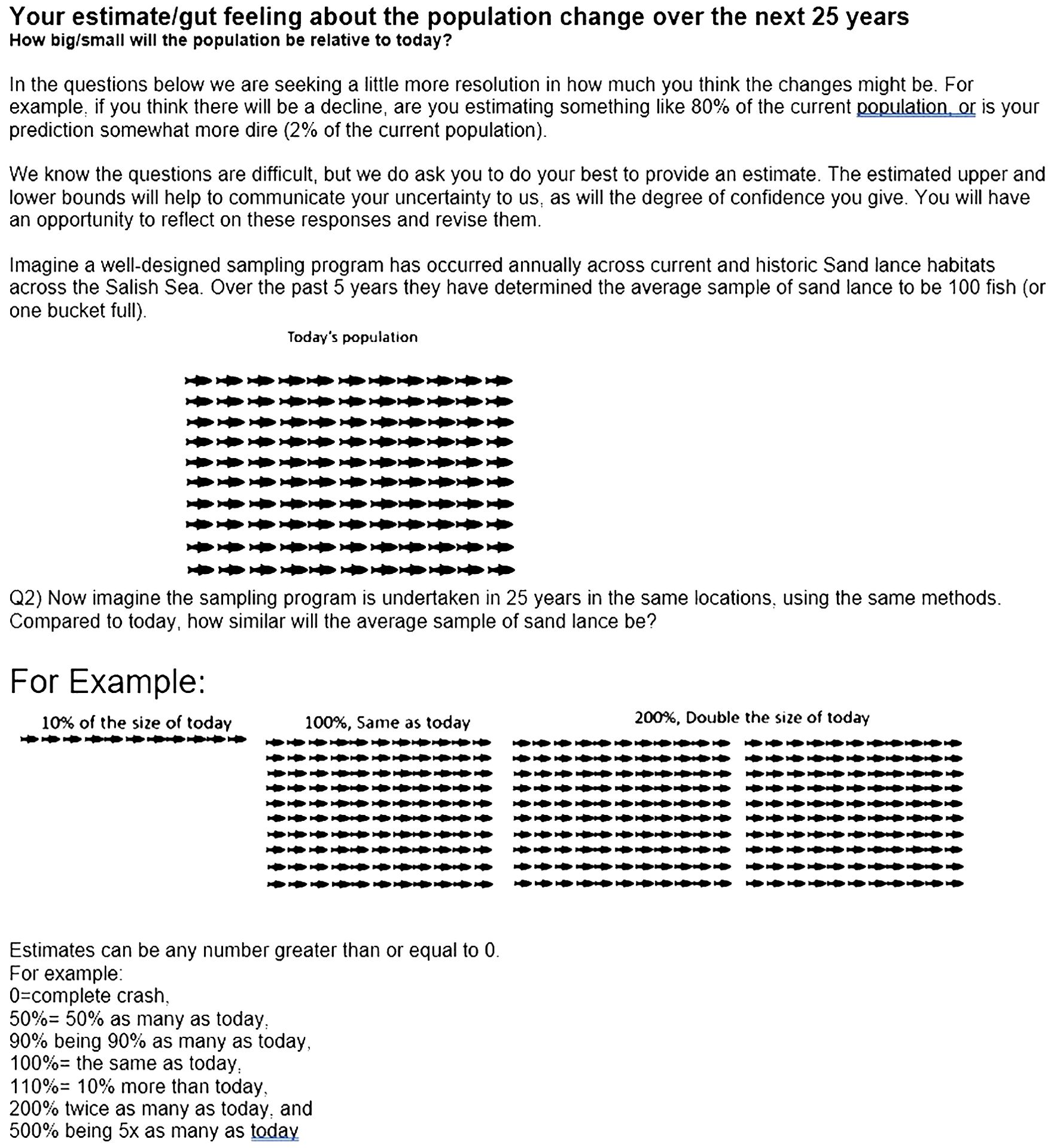
Figure 1. Information given to participants to help estimate the relative change in sand lance population trajectory provided to experts in Survey 2.
Finally, experts were asked to quantify their estimates by providing their: (1) high estimate; (2) low estimate; and (3) best estimate of the relative catch of sand lance from a monitoring program in 25 years, as compared to today (Figure 1). Experts were also asked to provide an estimate between 50% and 100% for the confidence level associated with their intervals (Speirs-Bridge et al., 2010). To compare between experts, the lower and upper bounds were standardized to 90% credible intervals (Hemming et al., 2018).
Survey 3: review
Survey 3 provided an opportunity to review the results of the 2nd Survey, including the comments and allow experts to finalize their estimates, adjusting if needed. Twenty-one experts completed Survey 3. We used the results of Survey 3 to calculate the final relative vulnerability assessments and as the final expert estimate of the population trajectory.
Results
Survey 1 – threat identification
Experts recommended 0 to 5 additional threats each. After accounting for duplicates, 34 additional threats were provided by the experts (Supplementary Table S2). When asked to broadly categorize the list of threats, participants placed as few as one and up to 23 in the Of Concern category (average of 10), zero to nine in the Least Concern category (average of three), and zero to 11 in the Uncertain category (average of seven). Climate change, followed by shoreline armoring were the most voted-for threats in the Of Concern category, with 31 and 30 total votes, respectively (Table 3). Recreational boating (14), commercial fishing (12), and recreational sites (11) were listed the most for threats of Least Concern. Under the Uncertain category, geoduck harvesting received the most votes (21), followed closely by renewable energy and construction (20), and commercial ship anchorages (19). Other threats with considerable uncertainty votes included major shipping and port developments (15), aquaculture (15), and freshwater dams (15). There were 19 threats that only received one vote, and most were additional threats provided by an expert and not seen by the remaining experts. The only way such a potential threat could have received more than one vote would be for two experts to identify the same threat independently.
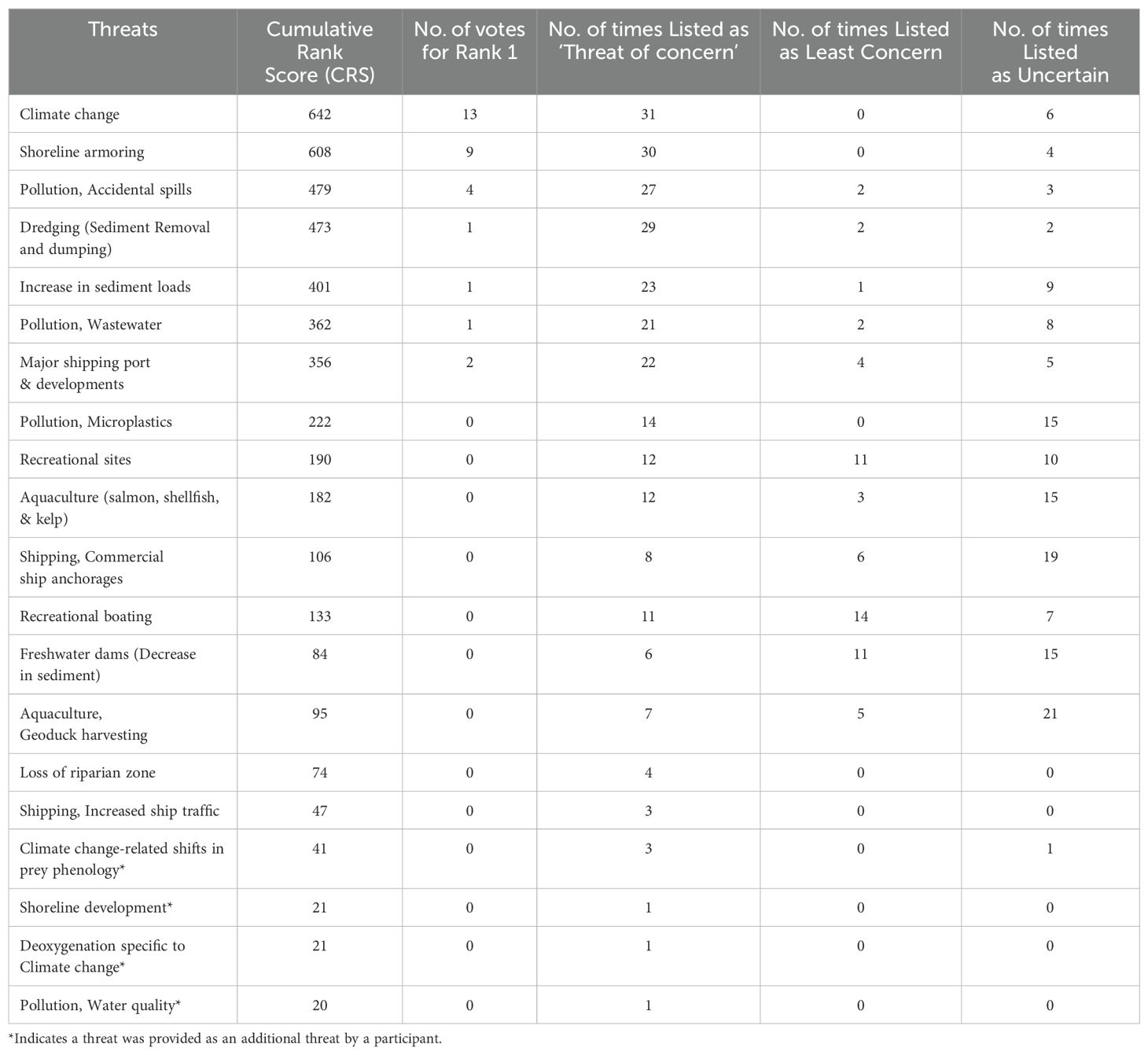
Table 3. Collated results of Survey 1 threat ranking showing top 20 threats identified by experts as of concern including cumulative rank score, total votes given (number of times listed under ‘threat of concern’ category), number of times listed as Least concern, and number of times listed as Uncertain.
The Cumulative Rank Scores (CRS) typically led to the same ranking as the number of votes a given threat received (Table 3). For example, climate change, followed by shoreline armoring, again scored the highest in the total CRS, with scores of 642 and 608, respectively. Some exceptions were: accidental spills (received 27 votes with a CRS of 479), and dredging (received 29 votes with a CRS of 473). However, there were only three threats with a lower CRS rank compared to the direct rank.
In the comments section, many participants addressed their lack of certainty with the threats and difficulty in ranking them. Several commented on how the spatiotemporal nature of a particular threat impacted their ranking choices, while others noted how their familiarity with a particular threat may have driven their rankings. Others commented they were not able to rank the threats without additional information given differences in scale among threats. Several participants with geology backgrounds pointed out that natural impacts such as earthquakes or shifts in geophysical processes may also have impacts on sand lance habitats. Several participants commented on confusion or specific concerns they had with certain threats, or how the threats were categorized. These comments were used to refine the final threats list and description of threats for Survey 2 (Tables 3, 4).
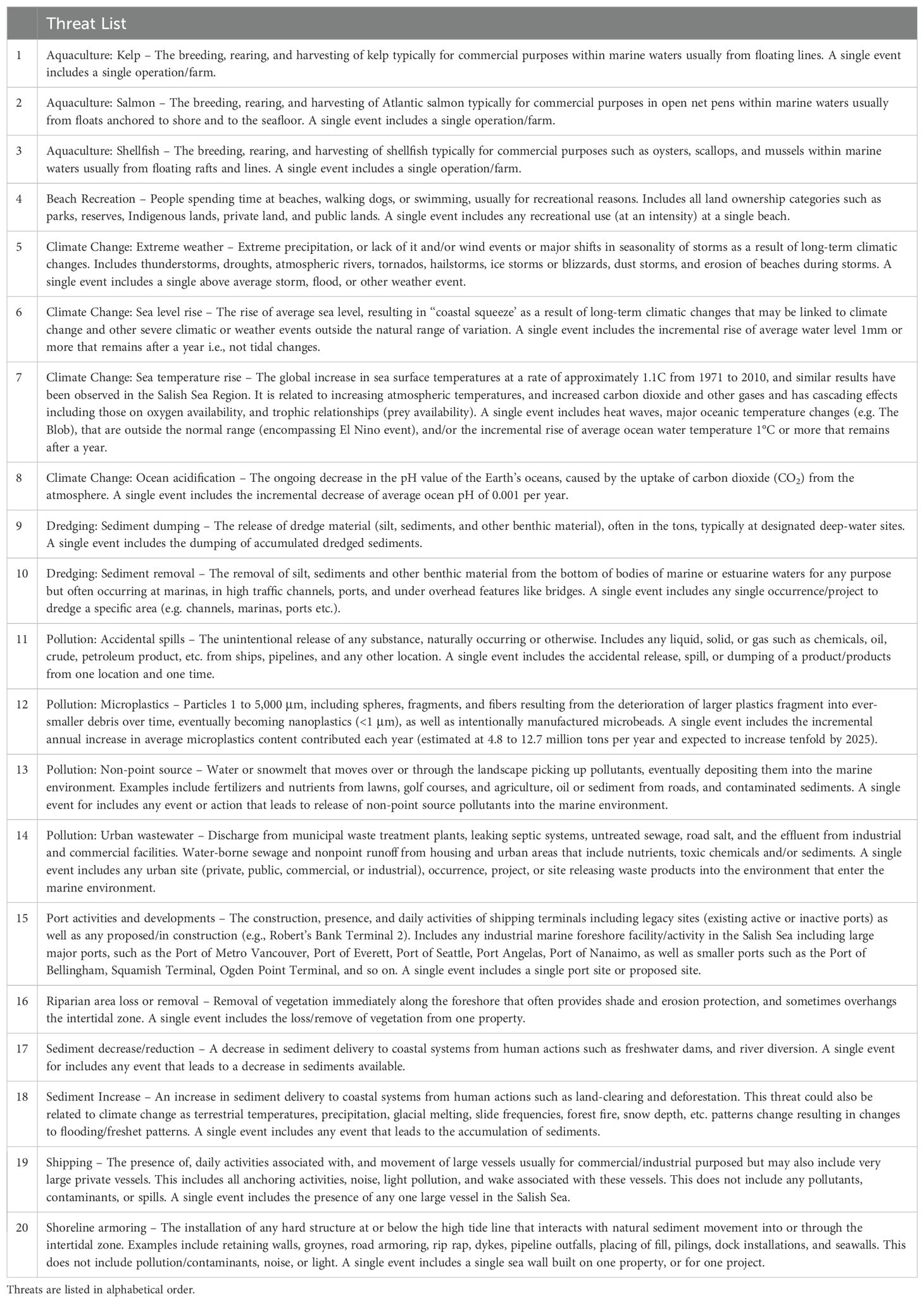
Table 4. Threat list developed through results of Survey 1 and the definitions provided to participants for Survey 2.
Survey 2 and 3: vulnerability assessment and expert population trajectory
Vulnerability assessment
The vulnerability assessment was completed by 30 experts; however, 5 abstained from providing estimates. Group A had 18 experts, and Group B had 12. In Survey 3, one expert altered just one of their responses to the vulnerability matrix table. This expert changed their response regarding the duration of shoreline armoring from (4) 1-10 years, to a higher value of (5) 10-20 years, changing the overall average from 5.78 to 5.8. The results presented are the final judgements by participants. The average of expert’s vulnerability score, the order of the relative vulnerability, and the most imminent threats scores were estimated to be (in order of highest to least): sea level rise, sea temperature rise, extreme weather, ocean acidification, decreased sediment loads from freshwater sources, and shoreline armoring (Figure 2). Aquaculture of kelp, salmon, and shellfish had the lowest scores.
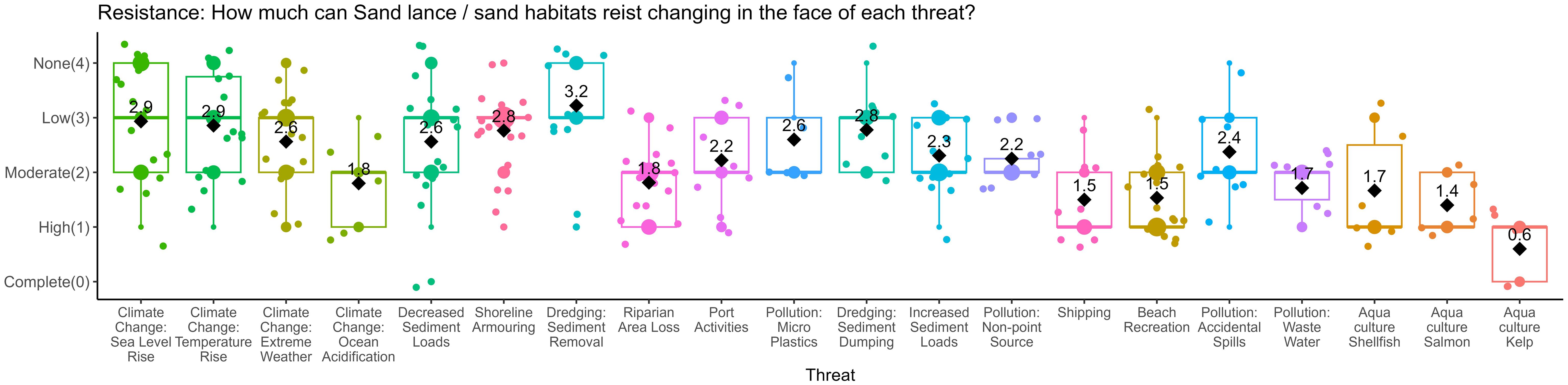
Figure 2. Box plots of vulnerability matrix estimates for sand lance and sand lance habitat resistance to evaluated threats. The median is represented by a line, the mean is displayed as a black diamond and the value of the mean is given. The color of each threat is consistent throughout, and the order of the x-axis is based on the overall vulnerability score.
The vulnerability scores were then disaggregated into area, duration, and occurrence (Figure 3); resistance (Figure 2); and certainty (Figure 4) and ordered according to the vulnerability scores, from highest to least (Figure 5). The resistance values roughly approximate the vulnerability scores, likely because of the higher weight this criterion was given relative to other criteria. However, duration, area, and frequency contribute to variability in these rankings. For example, climate change threats made up four of the top five threats in the overall relative vulnerability scores (Figure 5), but they were not always the top scoring resistance values (Figure 2). On average, experts thought sand lance are betterfrmoval) had the lowest median resistance value (3.2, Figure 2). Dredging had relatively low occurrence values, at least once per year (1.78, Figure 3A), meaning experts think sand lance and sand habitat are poorly able to cope with the impacts of dredging; however, they also thought this threat does not occur as often as other threats. At the other end of the threats assessed, kelp aquaculture had the lowest relative vulnerability score, and the lowest resistance values (0.6, Figure 2), but the highest occurrence value (6, Figure 3A), with moderate duration and area values. Certainty values varied little among threats, approximately 2, and made very little difference to the rankings of threats.
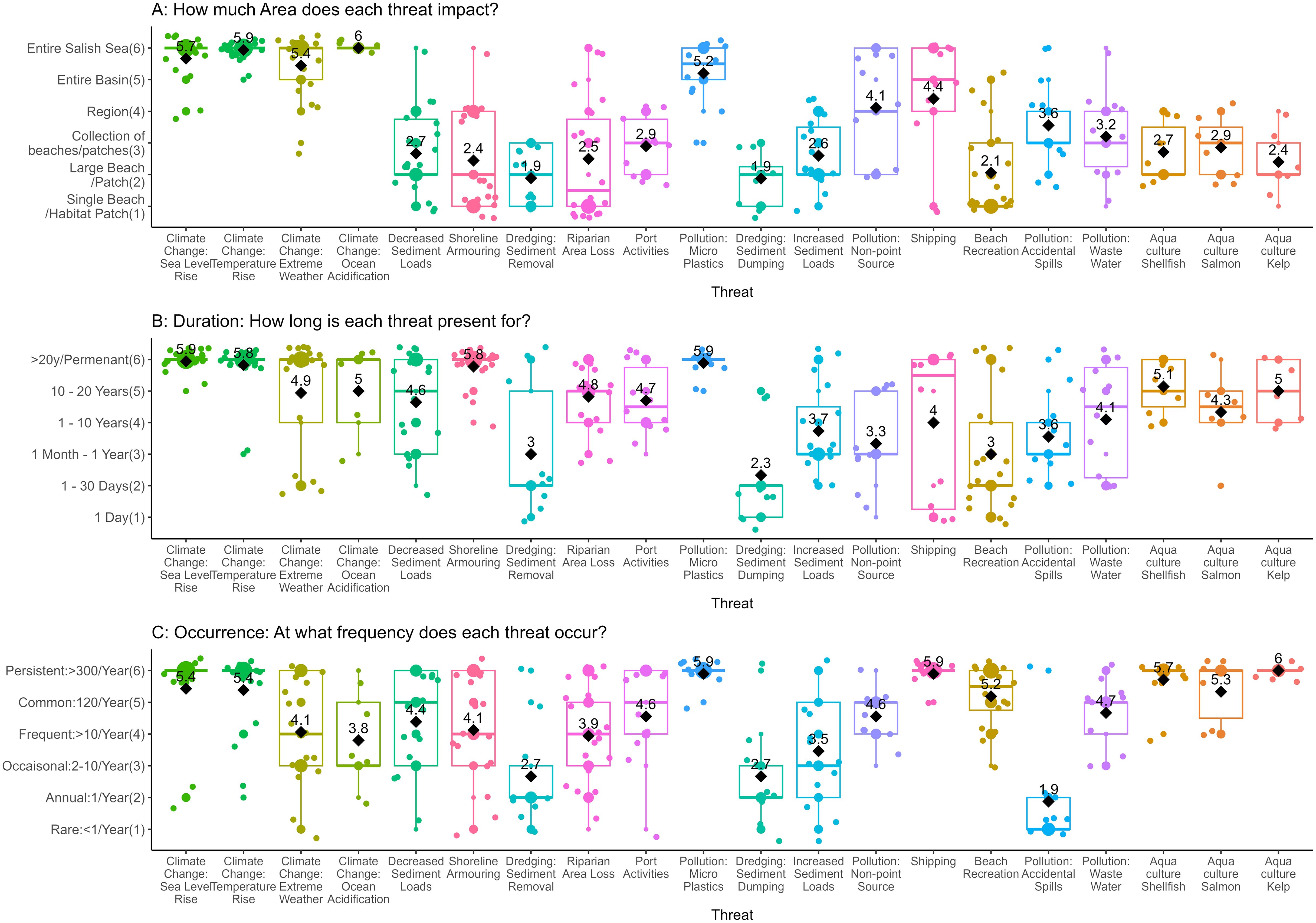
Figure 3. Box plots of vulnerability matrix estimates for (A) area, (B) duration, and (C) occurrence frequency. The median is represented by a line, the mean is displayed as a black diamond and the value of the mean is given. The color of each threat is consistent throughout, and the order of the x-axis is based on the overall vulnerability score.
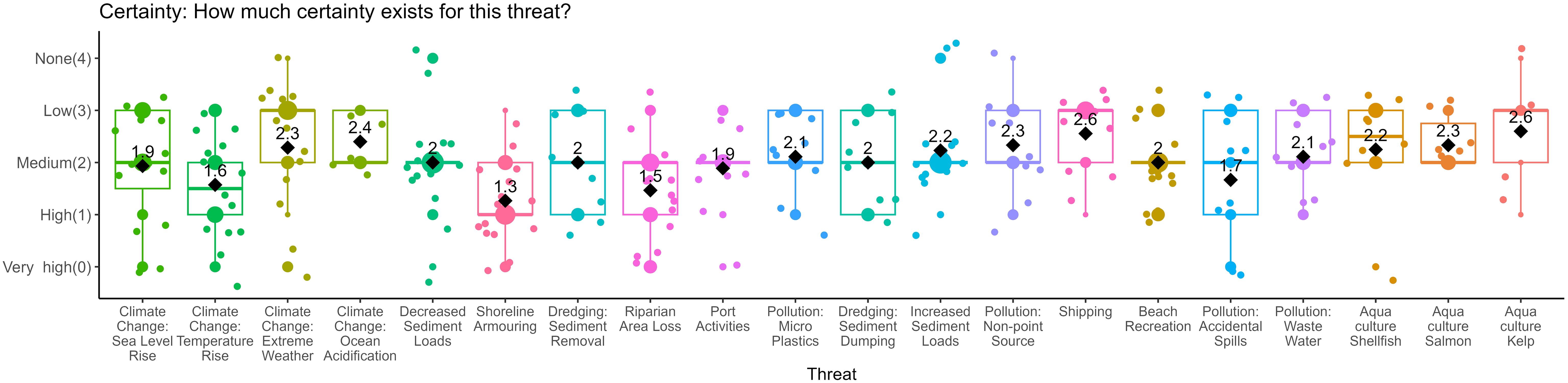
Figure 4. Results of vulnerability matrix estimates for certainty. The median is represented by a line, the mean is displayed as a black diamond and the value of the mean is given. The color of each threat is consistent throughout, and the order of the x-axis is based on the overall vulnerability score.
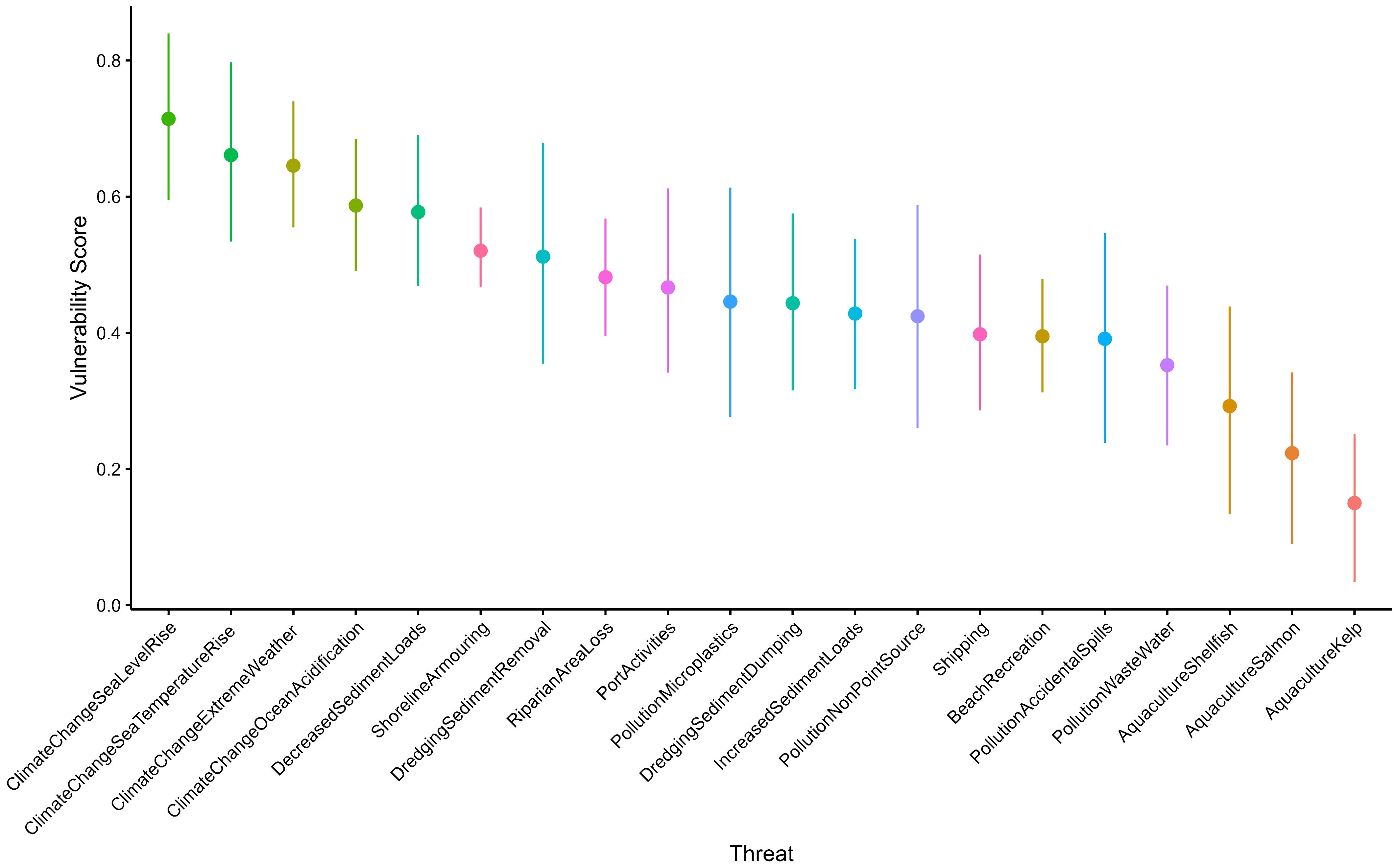
Figure 5. Weighted relative vulnerability mean scores of threats assessed to sand lance and their habitats in the Salish Sea, and their associated bootstrapped 95 percent confidence intervals.
Population assessment
Only five participants (of 30) declined to estimate the population trajectory (declining to answer each of three population questions). The results of the remaining 25 experts suggest that, on average, sand lance are expected to decline to 63% [95%CI: 20.1 – 185.5] of today’s population under a ‘business as usual’ scenario (Figure 6). While the upper and lower bound on this estimate exhibited high uncertainty, we note that only one participant (3%) estimated the population to increase, and three (9%) estimated it would remain the same. Many experts (40%) commented that they based their population projection estimate on assumptions that are supported in the literature, including anecdotal observations that suitable shoreline habitats are increasingly impacted or lost, threat intensity and occurrence frequencies appear to be increasing, extirpation and extinctions of other species in the Salish Sea are occurring at a rapid rate, and that ecosystem processes are decaying (Pimm et al., 2014; Díaz et al., 2019; Chase et al., 2020; Arimitsu et al., 2021; Laubenstein et al., 2023). The single expert that estimated a population increase cited that sand lance are a resistant species despite the habitat damage and loss, and changing climate conditions.
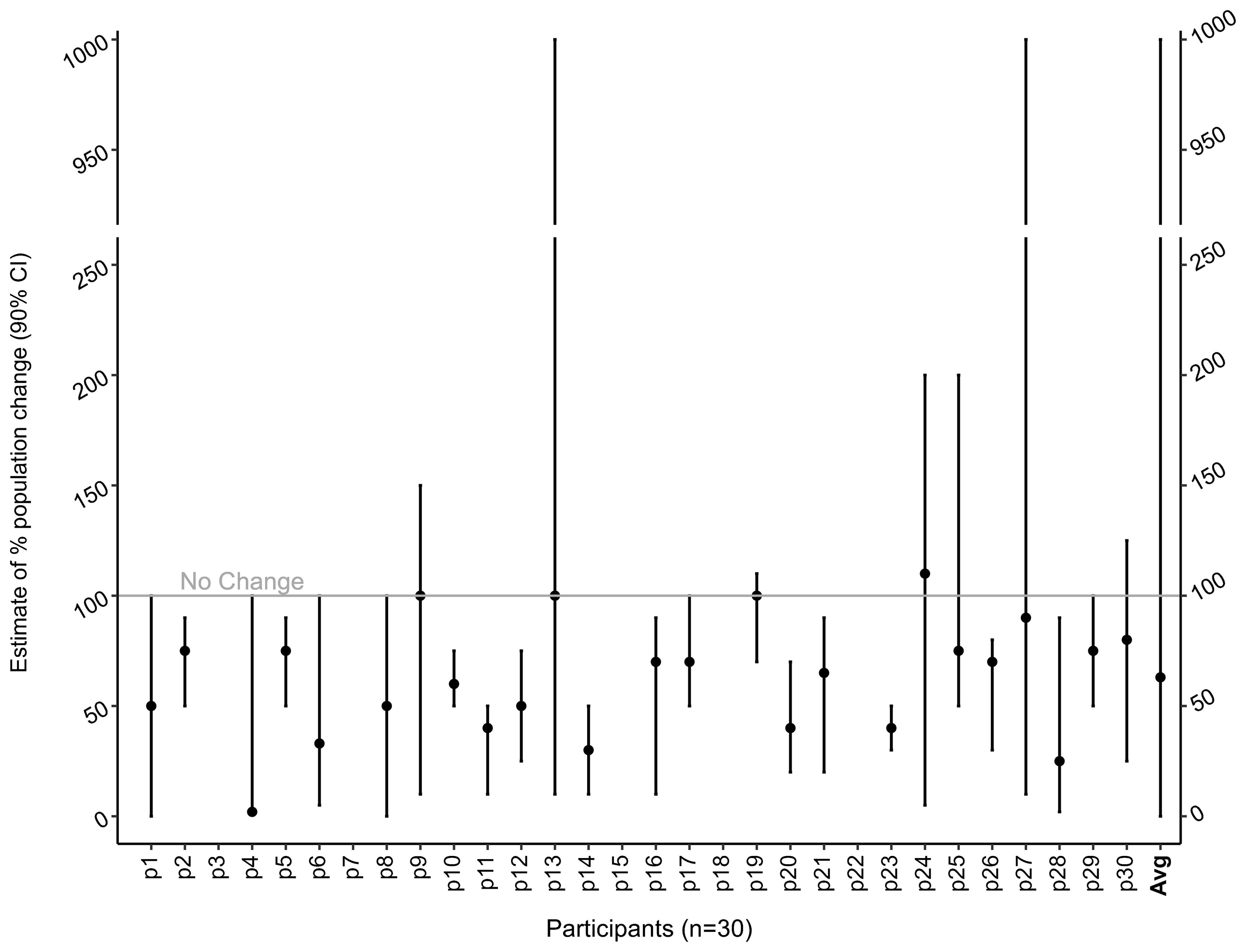
Figure 6. Population status estimate results from participants in Survey 2 (N=30, including edits in Survey 2 follow up). The upper and lower bounds were extrapolated to represent 90% credible intervals. 0%=complete crash, 50%= 50% as many as today, 90% = 90% as many as today, 100%= the same as today, 110%= 10% more than today, 200%=twice as many as today, and 500%=five times as many as today. The average estimate is 63% [95%CI: 20.1 – 185.5].
Three participants made changes in the follow-up survey after discussing initial results with other experts. There were two participants that changed their population trajectory assessment and widened their bounds (decreased their certainty), one also lowered their best guess. These changes made the overall average slightly lower and the bounds slightly wider.
Discussion
We present the first Salish Sea-wide evaluation of expert-based sand lance population trajectory, as well as the most comprehensive threat and vulnerability assessment conducted for the species to date.
Threats
The vulnerability assessment gathered experts from a wide range for backgrounds to carefully garner (1) a comprehensive list of threats to Salish Sea sand lance (Supplementary Material S1), (2) a list of the most concerning threats, and (3) prioritization of these top threats (Figures 2–6). The results indicate that sand lance are considered most vulnerable to climate change, which was thought to be a major threat to sandy beach habitats since the early 2000s (Brown and McLachlan, 2002). There is some existing research that specifically supports the notion that climate change poses a significant threat to sand lance populations. For example, a number of studies assessing sand lance mean body weight, size, condition, and fat content all negatively correlate with periods of increased temperatures (e.g., marine heat waves, Robards et al., 2002; Hipfner et al., 2018; von Biela et al., 2019; Arimitsu et al., 2021; Robinson et al., 2023). In addition, lab research on ocean acidification impacts to Ammodytes dubius, a species within the same genus occurring in the Northwest Atlantic, showed A. dubius eggs are highly sensitive to changes in CO2 at levels within the range of expected climate change values (Murray et al., 2019; Baumann et al., 2022). Finally, sea level rise is also projected to influence tidal currents, which may lead to the erosion and loss of sand, endangering rare shoreline and subtidal sand lance habitats (Healy, 1996; Greene et al., 2017; Greene et al., 2021).
While mitigation measures for climate change require national and global scale efforts, other threats (e.g., sediment loads, shoreline armoring and pollution) can be abated through local regulatory and/pr policy decisions of the Salish Sea (e.g., those approving permits for development or dredging). Shoreline armoring was among the top-ranked threats in the initial survey and has been of high concern amongst conservation groups since the early 2000s (de Graaf, 2010; Hart, 2010; de Graaf, 2014, 2017). Of the top threats, changes to sediment loads (e.g. such as that from dams or shoreline development) and shoreline armoring may be easier to address relative to the multifaceted, global nature of climate change (Hornsey and Fielding, 2019; Habel et al., 2020; Toft et al., 2021). Addressing local impacts, such as damage or loss of crucial spawning habitat, would contribute to offsetting complex threats such as sea level rise, sea temperature rise, pollution, and marine riparian area loss. There is mounting evidence of the benefits of dam removal which returns natural sediment regimes (Frick et al., 2022; Shaffer et al., 2023), avoiding shoreline armoring in favor of restoring and maintaining the natural capacity of nature to buffer adverse impacts using natural shoreline designs (Brown and McLachlan, 2002; Gittman et al., 2016; Martin and Watson, 2016), and avoiding dredging (Wenger et al., 2017).
While our assessment focused on threats to sand lance, we note that many of the top-ranked threats have been previously highlighted as key issues for other species and across ecosystems (Halpern et al., 2007; Crain et al., 2009; Defeo et al., 2009; Teck et al., 2010; Gaydos et al., 2015). They are also likely to be applicable to species that use the same habitats as sand lance, and for the predators that depend on them (Beaudreau and Essington, 2007; Defeo et al., 2009; Page et al., 2011; The Salish Sea Pacific Herring Assessment and Management Strategy Team, 2018; Smith and Liedtke, 2022). Therefore, applying a threat-based approach to management of sand lance habitats, such as a priority threat management plan (Martin et al., 2018), could provide an efficient means of safeguarding multiple species facing the same threats.
The threat assessment revealed that there is still much uncertainty about many threats, with 28 threats grouped as uncertain, and differences among experts in how they ranked threats. This is not surprising given the lack of research on this species and habitat. The uncertainty in the vulnerability matrix and comments provided by experts identifies collective gaps for future research (Supplementary Materials, Survey 2 Results Summary). While the threat assessment revealed many potential threats acting on sand lance, the average resistance value was ‘moderate’, suggesting sand lance may be resilient in the face of many threats. This result was echoed by several expert comments. This may explain the more optimistic predictions for population trajectory from some experts. Perhaps the secret to sand lance resilience is buried in their life history. Sand lance are more dormant throughout the later fall and winter months buried in sandy substrates, and, during this period, they regrow gonads in preparation for annual spawning (van Deurs et al., 2010; Zhukova and Baker, 2022). Mature sand eels in the North Sea are known to balance the increased metabolic costs of warm years with reduced gonad mass, which may buffer the effects of poor environmental conditions (Wright et al., 2017). Throughout marine heat wave years, when sea temperatures were above average for extended periods and zooplankton communities (prey) were altered, sand lance in Alaska (A. personatus) responded by burying more (Arimitsu et al., 2021). Researchers suggested that these sand lance may have adjusted daily and seasonal dormancy periods to reduce metabolic costs associated with warmer water temperatures, and subsequently thrived (Arimitsu et al., 2021). While sand lance may display resistance, their body condition, and thus nutritional value for predators, significantly declines during periods of warm ocean conditions, and/or when prey communities are altered (Litzow, 2000; Robards et al., 2002; von Biela et al., 2019; Robinson et al., 2023). This double impact of reduced abundance and nutritional value of sand lance and other forage fishes (e.g., capelin, Pacific herring, Pacific sardine, and northern anchovy) brought on by the 2014-2016 marine heat wave led to cascading impacts, shifting distributions, and resulting in large-scale mortality events of marine predators such as seabirds, marine mammals, and groundfish (Piatt et al., 2020; Arimitsu et al., 2021). Although sand lance populations may be resilient to climate change, it is not without any impact to the wider community.
Population
The experts interviewed in this study collectively suggest that sand lance populations in the Salish Sea could be 63% of what they are today (a 37% decline) in 25 years under current management scenarios. We acknowledge that there is much uncertainty around this population’s future status, which can be attributed to inherent biological attributes of forage fishes, a lack of wide scale, regularly occurring population surveys for sand lance, and a dearth of information about the direct impacts of specific threats on sand lance at both the individual and population levels. The expert projection contradicts the positive trend seen in the forage fish study across subbasins of Puget Sound using integrated trawl data (Greene et al., 2015). The authors of that study suggest that the differences in subbasin forage fish populations may be attributed to anthropogenic influences.
Our results highlight the need to better understand sand lance populations. A decline of the magnitude predicted by experts here would have ecosystem-wide implications particularly to predators that depend on sand lance during critical times in their life history (i.e., sea bird breeding) (Bertram and Kaiser, 1993; Bertram et al., 2001; Beaudreau and Essington, 2007; Gutowsky et al., 2009; Hipfner et al., 2018; Duguid, 2020). Given this species importance to the ecosystem and our collective interest in the economic and cultural values provided by top predators (e.g. salmon, orcas, lingcod, sea birds, etc.), we recommend the development and initiation of long-term, cross-border coordinated field-based population monitoring programs to address the hypothesis developed here that sand lance populations are in decline.
Method review
An additional benefit from this study is the progressive application of a method for assessing populations and threats together, and that these assessments may be applied to other cryptic species. The process applied here was collaborative, transboundary, and enabled information to be gathered from experts at a regional scale. The elicitation was relatively expedient, with results for 20 threats being obtained in three online surveys within 10 months. Experts provided 34 new threats that were not initially identified in the literature. While we did not quantify all threats with a vulnerability score, our list contains information on these threats. The framing of the elicitation question for the population status seemed to enable most experts (25 out of 30) to provide a best estimate of the expected trajectory of sand lance, and accompanying credible intervals, a response rate that surprised even the authors.
There are aspects of the approach applied here that might be improved for subsequent case studies. There were many decisions in the method development process, and we deliberated frequently over the best, or least-biased approach to acquire a truly relative comparison of the threats. On reflection, an additional initial survey to gather all the possible threats first, and then in a subsequent and separate survey to conduct a threat ranking activity would have allowed all experts to see the additional threats suggested by their peers. This would take additional time but may have reduced variability among experts.
Another improvement could be to hold a workshop to elicit which vulnerability criteria to use in the assessment, which would help better define criteria. This may lead to a more context-specific assessment process in which participants are more engaged (Stelzenmüller et al., 2018). Reassessing criteria weights used in the vulnerability calculation may also be warranted to have the best possible weighting specific to this context, area, and species and provide further expert engagement. Providing additional time for experts to spend thinking about the vulnerability criteria and the best threat weighting methodology may lead to better understanding of this complex concept and their application when accessing threats.
Defining terms was a difficult and time-demanding task. Even though attention was given to providing careful definitions, experts may have interpreted the threats differently, which may have contributed to some of the highly variable vulnerability scores. Linguistic uncertainty is a common issue in expert elicitation (Hemming et al., 2018) and underscores the importance of allowing experts to review and update their judgements, which can help to reveal and resolve uncertainties caused by ambiguity.
We faced a trade-off in mental load (how many threats we asked experts to evaluate) and the quality of their answers (e.g., was 10 threats too many or sufficient)?. One expert objected to leaving a particular threat out that they thought was important. Increasing collaboration and opportunities to review and change the list of threats may have addressed these concerns. To guard against a high non-response rate, we deliberately had large groups. Given the high response by experts, it may be possible to have assessed vulnerability for more threats, or to have split the experts into smaller groups of 5-8 experts to assess more of the threats.
Finally, many of the top threats identified (Table 4) occur over long time scales, and have both direct impacts on individuals as well as indirect impacts on sand lance habitat or food webs. Almost certainly, these threats interact, overlap, and amplify one another (Crain et al., 2008; Laubenstein et al., 2023). Although investigating the interactions was beyond the scope of this study, understanding if and how threats individually drive population responses and interact (e.g., acidification and warming; [Crain et al., 2008)] would help interpret and predict cumulative interactions.
Conclusion
Using expert elicitation, we gathered information on an understudied and difficult to study species, Pacific sand lance, in an efficient and low-cost manner. Expert opinion predict a decline in sand lance abundance in the Salish Sea under a business-as-usual scenario, highlighting the need for further investigation into Salish Sea sand lance populations and their persistence. A decline in sand lance abundance would have major cascading effects throughout the Pacific coastal ecosystem. The top threats identified include climate change, nearshore development, and pollution. Addressing knowledge gaps identified here to improve conservation decisions is one step toward a more sustainable, sand lance-abundant coast.
Data availability statement
The raw data supporting the conclusions of this article will be made available by the authors, without undue reservation.
Ethics statement
The studies involving humans were approved by University of British Columbia Human Ethics H19-01635 for the Salish Sea Cumulative Threats project. The studies were conducted in accordance with the local legislation and institutional requirements. The participants provided their written informed consent to participate in this study.
Author contributions
JRH: Conceptualization, Data curation, Formal analysis, Funding acquisition, Investigation, Methodology, Project administration, Resources, Software, Validation, Visualization, Writing – original draft, Writing – review & editing. VH: Conceptualization, Data curation, Methodology, Project administration, Supervision, Writing – original draft, Writing – review & editing. MB: Writing – review & editing. JB: Writing – review & editing. IB: Writing – review & editing. SC: Writing – review & editing. GD: Writing – review & editing. PD: Writing – review & editing. VE: Writing – review & editing. JMH: Writing – review & editing. NH: Writing – review & editing. BK: Writing – review & editing. DL: Writing – review & editing. RM: Writing – review & editing. GN: Writing – review & editing. BP: Writing – original draft, Writing – review & editing. MQ: Writing – review & editing. TQ: Writing – review & editing. CR: Conceptualization, Data curation, Investigation, Methodology, Supervision, Writing – original draft, Writing – review & editing. ER: Conceptualization, Writing – review & editing. DS: Writing – review & editing. JS: Writing – review & editing. AS: Writing – review & editing. NW: Writing – review & editing. JY: Writing – review & editing. TM: Conceptualization, Data curation, Formal analysis, Funding acquisition, Investigation, Methodology, Project administration, Resources, Supervision, Validation, Writing – original draft, Writing – review & editing.
Funding
The author(s) declare financial support was received for the research, authorship, and/or publication of this article. This research was supported by funding from a Mitacs Fellowship (JRH), NSERC Discovery Grant (TM), Liber Ero Chair in Conservation (TM) and Reid and Laura Carter.
Acknowledgments
This research is part of a Master’s thesis work by first author, JRH. The thesis can be found at https://open.library.ubc.ca/soa/cIRcle/collections/ubctheses/24/items/1.0434260 (DOI: 10.14288/1.0434260). We would like to thank all the experts interviewed which included the co-authors and in addition, Theresa Liedtke, Jenna Cragg, Alanna Vivani, Jennifer Boldt, William D.P. Duguid, Correigh M. Greene, Haley Tomlin, John Harper, Tanya Prinzing, Kirk Krueger, Jeremy Maynard, Karen Douglas and Tark Hamilton.
Conflict of interest
Author JS was employed by the company Natural Resources Consultants.
The remaining authors declare that the research was conducted in the absence of any commercial or financial relationships that could be construed as a potential conflict of interest.
Generative AI statement
The author(s) declare that no Generative AI was used in the creation of this manuscript.
Publisher’s note
All claims expressed in this article are solely those of the authors and do not necessarily represent those of their affiliated organizations, or those of the publisher, the editors and the reviewers. Any product that may be evaluated in this article, or claim that may be made by its manufacturer, is not guaranteed or endorsed by the publisher.
Supplementary material
The Supplementary Material for this article can be found online at: https://www.frontiersin.org/articles/10.3389/fmars.2024.1445215/full#supplementary-material
References
Alheit J., Peck M. (2019). Drivers of dynamics of small pelagic fish resources: biology, management and human factors. Mar. Ecol. Prog. Ser. 617–618, 1–6. doi: 10.3354/meps12985
Arimitsu M. L., Piatt J. F., Hatch S., Suryan R. M., Batten S., Bishop M. A., et al. (2021). Heatwave-induced synchrony within forage fish portfolio disrupts energy flow to top pelagic predators. Global Change Biol. 27, 1859–1878. doi: 10.1111/gcb.15556
Baker M. R., Greene H. G., Aschoff J., Aitoro E., Bates E., Hesselroth D., et al. (2024). Atlas of benthic habitat for sand lance – application of multibeam acoustics and directed sampling to identify viable subtidal substrates. Mar. Environ. Res. 202, 106778. doi: 10.2139/ssrn.4896945
Baker M. R., Williams K., Greene G. H., Aschoff J., Greufe C., Lopes H., Towler R. (2021). Use of manned submersible and autonomous stereo-camera array to assess forage fish and associated subtidal habitat. Fish. Res. 243, 106067. doi: 10.1016/j.fishres.2021.106067
Baker M., Matta M., Beaulieu M., Paris N., Huber S., Graham O., et al. (2019). Intra-seasonal and inter-annual patterns in the demographics of sand lance and response to environmental drivers in the North Pacific. Mar. Ecol. Prog. Ser. 617–618, 221–244. doi: 10.3354/meps12897
Baker M. R., Smeltz S., Williams K., Greufe C., Ewing M., Chapman J., et al. (2023). Diel vertical migration in a pelagic forage fish associated with benthic substrates. ICES J. Mar. Sci. 80, 1758–1772. doi: 10.1093/icesjms/fsad106
Baum J. K., Martin T. G. (2018). It is time to overcome unconscious bias in ecology. Nat. Ecol. Evol. 2, 201. doi: 10.1038/s41559-017-0441-y
Baumann H., Jones L. F., Murray C. S., Siedlecki S. A., Alexander M., Cross E. L. (2022). Impaired hatching exacerbates the high CO2 sensitivity of embryonic sand lance Ammodytes dubius. Mar. Ecol.-Prog. Ser. 687, 147–162. doi: 10.3354/meps14010
Beaubier J., Hipfner J. M. (2013). Proximate composition and energy density of forage fish delivered to rhinoceros auklet cerorhinca monocerata nestlings at Triangle Island, British Columbia. Mar. Ornithol. 41, 35–39. doi: 10.1111/jfb.12181
Beaudreau A. H., Essington T. E. (2007). Spatial, temporal, and ontogenetic patterns of predation on rockfishes by lingcod. Trans. Am. Fish. Soc. 136, 1438–1452. doi: 10.1577/T06-236.1
Beroya-Eitner M. A. (2016). Ecological vulnerability indicators. Ecol. Indic. 60, 329–334. doi: 10.1016/j.ecolind.2015.07.001
Berrouet L. M., MaChado J., Villegas-Palacio C. (2018). Vulnerability of socio—ecological systems: A conceptual Framework. Ecol. Indic. 84, 632–647. doi: 10.1016/j.ecolind.2017.07.051
Bertram D. F., Kaiser G. W. (1993). Rhinoceros Auklet (Cerorhinca monocerata) Nestling Diet May Gauge Pacific Sand Lance (Ammodytes hexapterus) Recruitment. Can. J. Fish. Aquat. Sci. 50, 1908–1915. doi: 10.1139/f93-213
Bertram D. F., Mackas D. L., McKinnell S. M. (2001). The seasonal cycle revisited: interannual variation and ecosystem consequences. Prog. Oceanogr. 49, 283–307. doi: 10.1016/S0079-6611(01)00027-1
Bizzarro J. J., Peterson A. N., Blaine J. M., Balaban J. P., Greene H. G., Summers A. P. (2016). Burrowing behavior, habitat, and functional morphology of the Pacific sand lance (Ammodytes personatus). Fishery Bulletin 114, 445–460. doi: 10.7755/FB.114.4.7
Blaine J. (2006). Pacific sand lance (Ammodytes hexapterus) present in the sandwave field of central San Juan Channel, WA: Abundance, density, maturity, and sediment association (Friday Harbor Laboratories, University of Washington), 23pp.
Brown A. C., McLachlan A. (2002). Sandy shore ecosystems and the threats facing them: some predictions for the year 2025. Envir. Conserv. 29, 62–77. doi: 10.1017/S037689290200005X
Buchanan M., Lesperance A., McArdle A. J., Sandborn C., Curran D. (2019). Saving Orcas by Protecting Fish Spawning Beaches (Victoria, British Columbia: World Wildlife Fund). Available online at: http://www.elc.uvic.ca/wordpress/wp-content/uploads/2019/11/2019-01-11-Saving-Orcas-by-Protecting-Fish-Spawning-Beaches.pdf (Accessed February 7 2020).
Burgman M., Jarrad F., Main E. (2015). Decreasing geographic bias in Conservation Biology. Conserv. Biol. 29, 1255–1256. doi: 10.1111/cobi.12589
Camaclang A. E., Currie J., Giles E., Forbes G. J., Edge C. B., Monk W. A., et al. (2021). Prioritizing threat management across terrestrial and freshwater realms for species conservation and recovery. Conserv. Sci. Pract. 3, e300. doi: 10.1111/csp2.300
Chase J. M., Blowes S. A., Knight T. M., Gerstner K., May F. (2020). Ecosystem decay exacerbates biodiversity loss with habitat loss. Nature 584, 238–243. doi: 10.1038/s41586-020-2531-2
Crain C. M., Halpern B. S., Beck M. W., Kappel C. V. (2009). Understanding and managing human threats to the coastal marine environment. Ann. New York Acad. Sci. 1162, 39–62. doi: 10.1111/j.1749-6632.2009.04496.x
Crain C. M., Kroeker K., Halpern B. S. (2008). Interactive and cumulative effects of multiple human stressors in marine systems. Ecol. Lett. 11, 1304–1315. doi: 10.1111/j.1461-0248.2008.01253.x
Defeo O., McLachlan A., Schoeman D. S., Schlacher T. A., Dugan J., Jones A., et al. (2009). Threats to sandy beach ecosystems: A review. Estuarine Coast. Shelf Sci. 81, 1–12. doi: 10.1016/j.ecss.2008.09.022
de Graaf R. (2010). Preliminary Habitat Assessment for suitability of intertidally spawning forage fish species, Pacific sand lance (Ammodytes hexapterus) and surf smelt (Hypomesus pretiosus) Esquimalt Lagoon, Colwood, British Columbia (Victoria, British Columbia: Capital Regional District). Available online at: https://www.crd.bc.ca/docs/default-source/es-harbours-pdf/esquimalt-lagoon/esquimaltlagoon-foragefishsurvey-degraffe-2010.pdf?sfvrsn=2 (Accessed May 22 2020).
de Graaf R. (2014). Bowen Island Surf smelt and Pacific sand lance Spawning Habitat Suitability Assessments (Bowen Island, BC: Bowen Island Municipality, Islands Trust, Bowen Island Local Trust Committee).
de Graaf R. (2017). Galiano Island Surf smelt and Pacific sand lance Beach Spawning Habitat Suitability Assessments (Islands Trust). Available online at: https://islandstrust.bc.ca/document/galiano-forage-fish-report/ (Accessed March 1 2023).
De Lange H. J., Sala S., Vighi M., Faber J. H. (2010). Ecological vulnerability in risk assessment — A review and perspectives. Sci. Total Environ. 408, 3871–3879. doi: 10.1016/j.scitotenv.2009.11.009
Dethier M. N., Raymond W. W., McBride A. N., Toft J. D., Cordell J. R., Ogston A. S., et al. (2016). Multiscale impacts of armoring on Salish Sea shorelines: Evidence for cumulative and threshold effects. Estuarine Coast. Shelf Sci. 175, 106–117. doi: 10.1016/j.ecss.2016.03.033
Díaz S., Settele J., Brondízio E. S., Ngo H. T., Agard J., Arneth A., et al. (2019). Pervasive human-driven decline of life on Earth points to the need for transformative change. Science 366, eaax3100. doi: 10.1126/science.aax3100
Donlan C. J., Wingfield D. K., Crowder L. B., Wilcox C. (2010). Using expert opinion surveys to rank threats to endangered species: a case study with sea turtles. Conserv. Biol. 24, 1586–1595. doi: 10.1111/j.1523-1739.2010.01541.x
Duguid W. (2020). British Columbia Adult Chinook and Coho Salmon Diet Program: 2019 Data Summary Report (Victoria, British Columbia: University of Victoria Fisheries Ecology and Marine Conservation Laboratory).
Einoder L. D. (2009). A review of the use of seabirds as indicators in fisheries and ecosystem management. Fish. Res. 95, 6–13. doi: 10.1016/j.fishres.2008.09.024
Frick K. E., Kagley A. N., Fresh K. L., Samhouri J. F., Ward L. S., Stapleton J. T., et al. (2022). Spatiotemporal variation in distribution, size, and relative abundance within a Salish sea nearshore forage fish community. Mar. Coast. Fish. 14, e10202. doi: 10.1002/mcf2.10202
Gaydos J. K., Pearson S. F. (2011). Birds and mammals that depend on the Salish sea: A compilation. Northwest. Nat. 92, 79–94. doi: 10.1898/10-04.1
Gaydos J. K., Thixton S., Donatuto J. (2015). Evaluating threats in multinational marine ecosystems: A coast Salish first nations and tribal perspective. PloS One 10, e0144861. doi: 10.1371/journal.pone.0144861
Gittman R. K., Scyphers S. B., Smith C. S., Neylan I. P., Grabowski J. H. (2016). Ecological consequences of shoreline hardening: A meta-analysis. BioScience 66, 763–773. doi: 10.1093/biosci/biw091
Grech A., Coles R., Marsh H. (2011). A broad-scale assessment of the risk to coastal seagrasses from cumulative threats. Mar. Policy 35, 560–567. doi: 10.1016/j.marpol.2011.03.003
Greene H. G., Baker M., Aschoff J. (2020). “Chapter 14 - A dynamic bedforms habitat for the forage fish Pacific sand lance, San Juan Islands, WA, United States,” in eafloor Geomorphology as Benthic Habitat, 2nd ed., vol. S . Eds. Harris P. T., Baker E. (Mount Vernon, WA: Elsevier), 267–279. doi: 10.1016/B978-0-12-814960-7.00014-2
Greene H. G., Cacchione D. A., Hampton M. A. (2017). Characteristics and dynamics of a large sub-tidal sand wave field—Habitat for pacific sand lance (Ammodytes personatus), Salish sea, Washington, USA. Geosciences 7, 107. doi: 10.3390/geosciences7040107
Greene C., Kuehne L., Rice C., Fresh K., Penttila D. (2015). Forty years of change in forage fish and jellyfish abundance across greater Puget Sound, Washington (USA): anthropogenic and climate associations. Mar. Ecol. Prog. Ser. 525, 153–170. doi: 10.3354/meps11251
Greene H. G., Baker M.R., Aschoff J., Pacunski R. (2021). Hazards evaluation of a valuable vulnerable sand wave field forage fish habitat in the marginal Central Salish Sea using a submersible. Oceanologia. 65, 1–9. doi: 10.1016/oceano.2121.06.002
Greenstreet S. P. R., Armstrong E., Mosegaard H., Jensen H., Gibb I. M., Fraser H. M., et al. (2006). Variation in the abundance of sandeels Ammodytes marinus off southeast Scotland: an evaluation of area-closure fisheries management and stock abundance assessment methods. ICES J. Mar. Sci. 63, 1530–1550. doi: 10.1016/j.icesjms.2006.05.009
Greenstreet S. P. R., Holland G. J., Guirey E. J., Armstrong E., Fraser H. M., Gibb I. M. (2010). Combining hydroacoustic seabed survey and grab sampling techniques to assess “local” sandeel population abundance. ICES J. Mar. Sci. 67, 971–984. doi: 10.1093/icesjms/fsp292
Gunther K. M., Baker M. R., Aydin K. Y. (2023). Using predator diets to infer forage fish distribution and assess responses to climate variability in the eastern Bering Sea. Mar. Ecol. Prog. Ser. SPF2. 741, 71-99. doi: 10.3354/meps14389
Gutowsky S., Janssen M., Arcese P., Kyser T., Ethier D., Wunder M., et al. (2009). Concurrent declines in nestling diet quality and reproductive success of a threatened seabird over 150 years. Endang. Species. Res. 9, 247–254. doi: 10.3354/esr00225
Habel M., Mechkin K., Podgorska K., Saunes M., Babinski Z., Chalov S., et al. (2020). Dam and reservoir removal projects: a mix of social-ecological trends and cost-cutting attitudes. Sci. Rep. 10, 19210. doi: 10.1038/s41598-020-76158-3
Halpern B. S., Selkoe K. A., Micheli F., Kappel C. V. (2007). Evaluating and ranking the vulnerability of global marine ecosystems to anthropogenic threats. Conserv. Biol. 21, 1301–1315. doi: 10.1111/j.1523-1739.2007.00752.x
Hanea A. M., Hemming V., Nane G. F. (2022). Uncertainty quantification with experts: present status and research needs. Risk Anal. 42, 254–263. doi: 10.1111/risa.13718
Hanea A. M., McBride M. F., Burgman M. A., Wintle B. C. (2018). The value of performance weights and discussion in aggregated expert judgments. Risk Anal. 38, 1781–1794. doi: 10.1111/risa.12992
Hare J. A., Morrison W. E., Nelson M. W., Stachura M. M., Teeters E. J., Griffis R. B., et al. (2016). A vulnerability assessment of fish and invertebrates to climate change on the northeast U.S. Continental shelf. PloS One 11, e0146756. doi: 10.1371/journal.pone.0146756
Hart C. (2010). Marine Shoreline Armoring and Puget Sound; Shorelands and Environmental Assistance Program. In: Frequently Asked Questions: 8 (Department of Ecology State of Washington). Available online at: https://apps.ecology.wa.gov/publications/documents/1006004.pdf (Accessed January 27 2024).
Harvey C. J., Bartz J., Davies T. B., Francis T. P., Good A. D., Guerry B., et al. (2010). A Mass-balance Model for Evaluating Food Web Structure and Community-scale Indicators in the Central Basin of Puget Sound (NOAA Technical Memorandum, NOAA). Available online at: https://repository.library.noaa.gov/view/noaa/3751 (Accessed January 27 2024).
Harwood J. (2000). Risk assessment and decision analysis in conservation. Biol. Conserv. 95, 219–226. doi: 10.1016/S0006-3207(00)00036-7
Haynes T., Robinson C. (2011). Re-use of shallow sediment patches by Pacific sand lance (Ammodytes hexapterus) in Barkley Sound, British Columbia, Canada. Environ. Biol. Fishes 92, 1–12. doi: 10.1007/s10641-011-9809-z
Haynes T. B., Robinson C. K. L., Dearden P. (2008). Modelling nearshore intertidal habitat use of young-of-the-year Pacific sand lance (Ammodytes hexapterus) in Barkley Sound, British Columbia, Canada. Environ. Biol. Fish 83, 473–484. doi: 10.1007/s10641-008-9374-2
Healy T. (1996). Sea level rise and impacts on nearshore sedimentation: an overview. Geologische Rundschau 85, 546–553. doi: 10.1007/BF02369009
Hedd A., Bertram D., Ryder J., Jones I. (2006). Effects of interdecadal climate variability on marine trophic interactions: rhinoceros auklets and their fish prey. Mar. Ecol. Prog. Ser. 309, 263–278. doi: 10.3354/meps309263
Hemming V., Burgman M. A., Hanea A. M., McBride M. F., Wintle B. C. (2018). A practical guide to structured expert elicitation using the IDEA protocol. Methods Ecol. Evol. 9, 169–180. doi: 10.1111/2041-210X.12857
Hipfner J. M., Galbraith M. (2013). Spatial and temporal variation in the diet of the Pacific sand lance Ammodytes hexapterus in waters off the coast of British Columbia, Canada. J. Fish Biol. 83, 1094–1111. doi: 10.1111/jfb.12181
Hipfner J. M., Galbraith M., Tucker S., Studholme K. R., Domalik A. D., Pearson S. F., et al. (2018). Two forage fishes as potential conduits for the vertical transfer of microfibres in Northeastern Pacific Ocean food webs. Environ. pollut. 239, 215–222. doi: 10.1016/j.envpol.2018.04.009
Hornsey M. J., Fielding K. S. (2019). Understanding (and Reducing) inaction on climate change. Soc. Issues Policy Rev. 14, 3–35. doi: 10.1111/sipr.12058
Hou K., Tao W., He D., Li X. (2022). A new perspective on ecological vulnerability and its transformation mechanisms. Ecosys. Health Sustainabil. 8, 2115403. doi: 10.1080/20964129.2022.2115403
Huard J. R., Proudfoot B., Rooper C. N., Martin T. G., Robinson C. L. K. (2022). Intertidal beach habitat suitability model for Pacific sand lance (Ammodytes personatus) in the Salish Sea, Canada. Can. J. Fish. Aquat. Sci. 79, 1681–1696. doi: 10.1139/cjfas-2021-0335
Kappel C. V., Halpern B. S., Selkoe K. A., Cooke R. M. (2012). “Eliciting Expert Knowledge of Ecosystem Vulnerability to Human Stressors to Support Comprehensive Ocean Management,” in Expert Knowledge and Its Application in Landscape Ecology. Eds. Perera A. H., Drew C. A., Johnson C. J. (Springer, New York, NY), 253–277. doi: 10.1007/978-1-4614-1034-8_13
Krueger K. L., Pierce K. B., Quinn T., Penttila D. E. (2010). “Anticipated Effects of Sea Level Rise in Puget Sound on Two Beach-Spawning Fishes,” in Washington Department of Fish & Wildlife, Puget Sound Shorelines and the Impacts of Armoring; “Proceedings of a State of the Science Workshop. Washington Department of Fish & Wildlife. Available at: https://wdfw.wa.gov/publications/01210.
Lance M. M., Chang W.-Y., Jefferies S. J., Pearson S., Acevedo-Gutiérrez A. (2012). Harbor seal diet in northern Puget Sound: implications for the recovery of depressed fish stocks. Mar. Ecol. Prog. Series. 464, 257–271. doi: 10.3354/meps09880
Laubenstein T., Smith T. F., Hobday A. J., Pecl G. T., Evans K., Fulton E. A., et al. (2023). Threats to Australia’s oceans and coasts: A systematic review. Ocean Coast. Manage. 231, 106331. doi: 10.1016/j.ocecoaman.2022.106331
Liedtke T. L., Conn K. E. (2021). Maternal transfer of polychlorinated biphenyls in Pacific sand lance (Ammodytes personatus), Puget Sound, Washington. Sci. Total Environ. 764, 142819. doi: 10.1016/j.scitotenv.2020.142819
Litzow M. (2000). Monitoring temporal and spatial variability in sandeel (Ammodytes hexapterus) abundance with pigeon guillemot (Cepphus columba) diets. ICES J. Mar. Sci. 57, 976–986. doi: 10.1006/jmsc.2000.0583
Martin T. G., Burgman M. A., Fidler F., Kuhnert P. M., Low-Choy S., Mcbride M., et al. (2012). Eliciting expert knowledge in conservation science: elicitation of expert knowledge. Conserv. Biol. 26, 29–38. doi: 10.1111/j.1523-1739.2011.01806.x
Martin T. G., Kehoe L., Mantyka-Pringle C., Chades I., Wilson S., Bloom R. G., et al. (2018). Prioritizing recovery funding to maximize conservation of endangered species. Conserv. Lett. 11, e12604. doi: 10.1111/conl.12604
Martin T. G., Watson J. E. M. (2016). Intact ecosystems provide best defense against climate change. Nat. Clim Change 6, 122–124. doi: 10.1038/nclimate2918
Matta M. E., Baker M. R. (2020). Age and growth of Pacific sand lance (Ammodytes personatus) at the latitudinal extremes of the Gulf of Alaska Large Marine Ecosystem. Soc. Northwest. Vertebr. Biol. 101, 34–49. doi: 10.1898/1051-1733-101.1.34
McKenzie J., Wynne K. M. (2008). Spatial and temporal variation in the diet of Steller sea lions in the Kodiak Archipelago 1999 to 2005. Mar. Ecol. Prog. Ser. 360, 265–283. doi: 10.3354/meps07383
Moore S. E., Haug T., Víkingsson G. A., Stenson G. B. (2019). Baleen whale ecology in arctic and subarctic seas in an era of rapid habitat alteration. Prog. Oceanogr. 176, 102118. doi: 10.1016/j.pocean.2019.05.010
Murray N. J., Phinn S. R., DeWitt M., Ferrari R., Johnston R., Lyons M. B., et al (2019). The global distribution and trajectory of tidal flats. Nature 565, 222–225. doi: 10.1038/s41586-018-0805-8
O’Hagan A. (2019). Expert knowledge elicitation: subjective but scientific. Am. Statistic. 73, 69–81. doi: 10.1080/00031305.2018.1518265
Okamura H., Nagashima H., Yonezaki S. (2009). Preliminary assessment of impacts on the sandlance population by consumption of minke whales off Sanriku region. Institute for Cetacean Research. Available online at: SC/JO9/JR14.
Orr J. W., Wildes S., Kai Y., Raring N., Nakabo T., Katugin O., et al. (2015). Systematics of North Pacific sand lances of the genus Ammodytes based on molecular and morphological evidence, with the description of a new species from Japan. Fish. Bull. 113, 129–156. doi: 10.7755/FB.113.2.3
Page N., Lilley P., Walker I. J., Vennesland R. G. (2011). Status report on coastal sand ecosystems in British Columbia. Coast. Sand Ecosyst. Recov. Team 90, 1–9. Available at: https://www.researchgate.net/publication/256373195_Status_Report_on_Coastal_Sand_Ecosystems_in_British_Columbia#fullTextFileContent.
Pastran S. A., Drever M. C., Lank D. B. (2021). Marbled Murrelets prefer stratified waters close to freshwater inputs in Haida Gwaii, British Columbia, Canada. Ornithol. Appl. 123, duab043. doi: 10.1093/ornithapp/duab043
Pearson W. H., Woodruff D. L., Sugarman P. C., Olla B. L. (1984). The burrowing behavior of sand lance, Ammodytes hexapterus: Effects of oil-contaminated sediment. Mar. Environ. Res. 11, 17–32. doi: 10.1016/0141-1136(84)90008-4
Penttila D. (1995). Investigations of the spawning habitat of the pacific sand lance, Ammodytes hexapterus, in Puget Sound. Puget Sound Res. Proc. 2, 855–859.
Penttila D. (2007). Marine Forage Fishes in Puget Sound (Fort Belvoir, VA: Defense Technical Information Center). doi: 10.21236/ADA478081
Piatt J. F., Parrish J. K., Renner H. M., Schoen S. K., Jones T. T., Arimitsu M. L., et al. (2020). Extreme mortality and reproductive failure of common murres resulting from the northeast Pacific marine heatwave of 2014-2016. PloS One 15, e0226087. doi: 10.1371/journal.pone.0226087
Pimm S. L., Jenkins C. N., Abell R., Brooks T. M., Gittleman J. L., Joppa L. N., et al. (2014). The biodiversity of species and their rates of extinction, distribution, and protection. Science 344, 1246752. doi: 10.1126/science.1246752
Quinn T. (1999). Habitat Characteristics of an Intertidal aggregation of Pacific Sandlance (Ammodytes hexapterus) at a North Puget Sound Beach in Washington. Northw. Sci. 73, 44–50. Available at: http://rightsstatements.org/vocab/InC/1.0/.
Robards M. D., Piatt J. F. (1999). Sand lance: A review of biology and predator relations and annotated bibliography. In: Sand lance: A review of biology and predator relations and annotated bibliography (Portland, OR: Federal Government Series, USDA Forest Service, Pacific Northwest Research Station). Available online at: http://pubs.er.usgs.gov/publication/70187537 (Accessed March 12 2020).
Robards M. D., Rose G. A., Piatt J. F. (2002). Growth and Abundance of Pacific Sand Lance, Ammodytes hexapterus, under differing Oceanographic Regimes. Environ. Biol. Fishes 64, 429–441. doi: 10.1023/A:1016151224357
Robinson C. L. K. (2013). An evaluation of Pacific Sand lance (Ammodytes hexapterus) habitats in the Scott Islands National Wildlife Area (Nanaimo, BC: BC Conservation Foundation).
Robinson C., Bertram D., Shannon H., von Biela V., Greentree W., Duguid W., et al. (2023). Reduction in overwinter body condition and size of Pacific sand lance has implications for piscivorous predators during marine heatwaves. Mar. Ecol. Prog. Ser., 1-11. doi: 10.3354/meps14257
Robinson C. L. K., Hrynyk D., Barrie J. V., Schweigert J. (2013). Identifying subtidal burying habitat of Pacific sand lance (Ammodytes hexapterus) in the Strait of Georgia, British Columbia, Canada. Prog. Oceanogr. 115, 119–128. doi: 10.1016/j.pocean.2013.05.029
Robinson C. L. K., Proudfoot B., Rooper C. N., Bertram D. F. (2021). Comparison of spatial distribution models to predict subtidal burying habitat of the forage fish Ammodytes personatus in the Strait of Georgia, British Columbia, Canada. Aquat. Conserv.: Mar. Freshw. Ecosyst. 2855–2869. doi: 10.1002/aqc.3593
Rovellini A., Punt A. E., Bryan M. D., Kaplan I. C., Dorn M. W., Aydin K., et al. (2024). Linking climate stressors to ecological processes in ecosystem models, with a case study from the Gulf of Alaska. ICES J. Mar. Sci., fsae002. doi: 10.1093/icesjms/fsae002
Scordino J. J., Akmajian A. M., Edmondson S. L. (2022). Dietary niche overlap and prey consumption for the Steller sea lion (Eumetopias jubatus) and California sea lion (Zalophus californianus) in northwest Washington during 2010-2013. Fishery Bulletin 120, 39–54. doi: 10.7755/FB.120.1.4
Selden K. R., Baker M. R. (2023). Influence of marine habitat on microplastic prevalence in forage fish and salmon in the Salish Sea. Mar. pollut. Bull. 197, 115748. doi: 10.1016/j.marpolbul.2023.115748
Selleck J. R., Gibson C. F., Shull S., Gaydos J. K. (2015). Nearshore distributions of Pacific sand lance (Ammodytes personatus) in the inland waters of Washington State. Soc. Northwest. Vertebr. Biol. 96, 185–195. doi: 10.1898/1051-1733-96.3.185
Shaffer A., Gross J., Black M., Kalagher A., Juanes F. (2023). Dynamics of juvenile salmon and forage fishes in nearshore kelp forests. Aquat. Conserv.: Mar. Freshw. Ecosyst. 33, 822–832. doi: 10.1002/aqc.3957
Singh G. G., Sinner J., Ellis J., Kandlikar M., Halpern B. S., Satterfield T., et al. (2017). Mechanisms and risk of cumulative impacts to coastal ecosystem services: An expert elicitation approach. J. Environ. Manage. 199, 229–241. doi: 10.1016/j.jenvman.2017.05.032
Smith C. D., Liedtke T. L. (2022). “Potential effects of sea level rise on nearshore habitat availability for surf smelt (Hypomesus pretiosus) and eelgrass (Zostera marina), Puget Sound, Washington,” in Potential effects of sea level rise on nearshore habitat availability for surf smelt (Hypomesus pretiosus) and eelgrass (Zostera marina), Puget Sound, Washington (USGS Numbered Series, U.S. Geological Survey, Reston, VA). doi: 10.3133/ofr20221054
Speed L., Baker M. R. (2016). Abundance and Distribution of Pacific Sand Lance in San Juan Channel, WA. Poster 2016SSEC. Available online at: https://cedar.wwu.edu/ssec/2016ssec/species_food_webs/4/. (Accessed January 1, 2024).
Speirs-Bridge A., Fidler F., McBride M., Flander L., Cumming G., Burgman M. (2010). Reducing overconfidence in the interval judgments of experts. Risk analysis. Risk Analysis 30, 512–523. doi: 10.1111/j.1539-6924.2009.01337.x
Staudinger (2020). The role of sand lances (Ammodytes sp.) in the Northwest Atlantic Ecosystem: A synthesis of current knowledge with implications for conservation and management. Fish Fish. 616–633. doi: 10.1111/faf.12445
Stelzenmüller V., Coll M., Mazaris A. D., Giakoumi S., Katsanevakis S., Portman M. E., et al. (2018). A risk-based approach to cumulative effect assessments for marine management. Sci. Total Environ. 612, 1132–1140. doi: 10.1016/j.scitotenv.2017.08.289
Teck S. J., Halpern B. S., Kappel C. V., Micheli F., Selkoe K. A., Crain C. M., et al. (2010). Using expert judgment to estimate marine ecosystem vulnerability in the California Current. Ecol. Appl. 20, 1402–1416. doi: 10.1890/09-1173.1
Thayer J. A., Bertram D. F., Hatch S. A., Hipfner M. J., Slater L., Sydeman W. J., et al. (2008). Forage fish of the Pacific Rim as revealed by diet of a piscivorous seabird: synchrony and relationships with sea surface temperature. Can. J. Fish. Aquat. Sci. 65, 1610–1622. doi: 10.1139/F08-076
The Salish Sea Pacific Herring Assessment and Management Strategy Team. (2018). Assessment and management of Pacific herring in the Salish Sea: conserving and recovering a culturally significant and ecologically critical component of the food web. The SeaDoc Society, Orcas Island, WA. 74 pp. Available at: https://www.eopugetsound.org/sites/default/files/features/resources/Assessment%20and%20Management%20of%20Salish%20Sea%20Herring.pdf.
Toft J. D., Dethier M. N., Howe E. R., Buckner E. V., Cordell J. R. (2021). Effectiveness of living shorelines in the Salish Sea. Ecol. Eng. 167, 925–8574. doi: 10.1016/j.ecoleng.2021.106255
Tomlin H., Schellenberg C., Barrs J. B., Vivani A. J. S., Shaw P. (2021). Identifying and monitoring of forage fish spawning beaches in British Columbia’s Salish Sea for conservation of forage fish. FACETS 6, 1024–1043. doi: 10.1139/facets-2020-0038
Towers J. R., McMillan C. J., Piercey R. S. (2019). Sighting rates and prey of Minke Whales (Balaenoptera acutorostrata) and other cetaceans off Cormorant Island, British Columbia. Can. Field-Natural. 133, 144–150. doi: 10.22621/cfn.v133i2.2103
Turner B. L., Kasperson R. E., Matson P. A., McCarthy J. J., Corell R. W., Christensen L., et al. (2003). A framework for vulnerability analysis in sustainability science. Proc. Natl. Acad. Sci. 100, 8074–8079. doi: 10.1073/pnas.1231335100
van Deurs M., Christensen A., Frisk C., Mosegaard H. (2010). Overwintering strategy of sandeel ecotypes from an energy/predation trade-off perspective. Mar. Ecol. Prog. Ser. 416, 201–214. doi: 10.3354/meps08763
Van Straalen N. M. (1993). Biodiversity of ecotoxicological responses in animals. Neth J. Zool 44, 112–129. doi: 10.1163/156854294X00097
von Biela V., Arimitsu M., Piatt J., Heflin B., Schoen S. K., Trowbridge J., et al. (2019). Extreme reduction in nutritional value of a key forage fish during the Pacific marine heatwave of 2014-2016. Mar. Ecol. Prog. Ser. 613, 171–182. doi: 10.3354/meps12891
Wenger A. S., Harvey E., Wilson S., Rawson C., Newman S. J., Clarke D., et al. (2017). A critical analysis of the direct effects of dredging on fish. Fish Fish. 18, 967–985. doi: 10.1111/faf.12218
Willson M. F., Armstrong R. H., Robards M. D., Piatt J. F. (1999). Sand lance as cornerstone prey for predator populations. Environmental Management. 17–44, USDA Forest Service - Research Papers PNW-RP.
Wilson K., Pressey R. L., Newton A., Burgman M., Possingham H., Weston C. (2005). Measuring and incorporating vulnerability into conservation planning. Environ. Manage. 35, 527–543. doi: 10.1007/s00267-004-0095-9
Wolfson L. J., Kadane J. B., Small M. J. (1996). Bayesian environmental policy decisions: two case studies. Ecol. Appl. 6, 1056–1066. doi: 10.2307/2269590
Wright P. J., Orpwood J. E., Boulcott P. (2017). Warming delays ovarian development in a capital breeder. Mar. Biol. 164, 80. doi: 10.1007/s00227-017-3116-y
Wright D. L., Witteveen B., Wynne K., Horstmann-Dehn L. (2016). Fine-scale spatial differences in humpback whale diet composition near Kodiak, Alaska. Mar. Mammal Sci. 32, 1099–1114. doi: 10.1111/mms.12311
Zamon J. (2000). The influence of tidal currents on plankton densities and energy flow to seals, seabirds, and schooling fishes in the San Juan Islands, WA. thesis. University of California. Available at: https://uci.primo.exlibrisgroup.com/discovery/fulldisplay?docid=alma991035093417404701&context=L&vid=01CDL_IRV_INST:UCI&lang=en&search_scope=DN_Filtered&adaptor=Local%20Search%20Engine&isFrbr=true&tab=Everything&query=any,contains,zamon&sortby=rank&offset=0.
Keywords: expert elicitation, threats, conservation, marine food web, ecological risk assessment, forage fish, nearshore habitat
Citation: Huard JR, Hemming V, Baker MR, Blancard J, Bruce I, Cook S, Davoren GK, Dionne P, East V, Hipfner JM, Houtman NR, Koval BA, Lowry D, Monks R, Nicholas G, Proudfoot B, Quindazzi M, Quinn T, Robinson CLK, Rubidge EM, Sanford D, Selleck JR, Shaffer A, Wright N, Yakimishyn J and Martin TG (2024) Threat assessment for Pacific sand lance (Ammodytes personatus) in the Salish Sea. Front. Mar. Sci. 11:1445215. doi: 10.3389/fmars.2024.1445215
Received: 07 June 2024; Accepted: 30 September 2024;
Published: 30 October 2024.
Edited by:
Correigh Greene, National Oceanic and Atmospheric Administration (NOAA), United StatesReviewed by:
Anna Kagley, NOAA Fisheries, United StatesJoshua Chamberlin, National Marine Fisheries Service (NOAA), United States
Copyright © 2024 Huard, Hemming, Baker, Blancard, Bruce, Cook, Davoren, Dionne, East, Hipfner, Houtman, Koval, Lowry, Monks, Nicholas, Proudfoot, Quindazzi, Quinn, Robinson, Rubidge, Sanford, Selleck, Shaffer, Wright, Yakimishyn and Martin. This is an open-access article distributed under the terms of the Creative Commons Attribution License (CC BY). The use, distribution or reproduction in other forums is permitted, provided the original author(s) and the copyright owner(s) are credited and that the original publication in this journal is cited, in accordance with accepted academic practice. No use, distribution or reproduction is permitted which does not comply with these terms.
*Correspondence: Jacqueline R. Huard, amFjaHVhcmRAZ21haWwuY29t
†ORCID: Jacqueline R. Huard, orcid.org/0000-0003-4509-9422