- 1School of Biosciences, Cardiff University, Cardiff, United Kingdom
- 2Cardigan Bay Marine Wildlife Centre, The Wildlife Trust of South and West Wales, New Quay, United Kingdom
Global marine biodiversity declines require bold and ambitious plans to safeguard ecosystem services. Overfishing, habitat loss and projected climate impacts have yielded deleterious effects on marine predators, in particular, driving increasing threat of extinction for many shark species with implications for ecosystem health. Identifying and protecting critical habitat areas for sharks is fundamental to their protection, and may allow for species recovery. Here we use baited remote underwater video stations to investigate spatial and temporal patterns of habitat use by sharks in a Special Area of Conservation (SAC) that is centrally important to the regional blue economy in the UK, the Cardigan Bay SAC. Our results show heterogeneous spatial habitat use and temporal trends in habitat sharing between smallspotted catsharks Scyliorhinus canicula and nursehounds S. stellaris. Nearshore reef habitats are substantially more important than non-reef habitats for both species. The relative abundance of each species, however, is roughly inversely seasonally proportional, with S. canicula and S. stellaris relative abundance highest in March and September, respectively. Temporal niche partitioning may be an important mechanism in marine systems, but has not been widely investigated in sympatric shark communities. These findings are directly relevant for ongoing development of the Welsh Marine Evidence Strategy (2019-2025) and the Welsh National Marine Plan, particularly to inform spatial planning to strengthen the role of SAC management design in protecting important shark areas.
Introduction
Marine predatory fish biomass has decreased dramatically since the onset of industrialised fishing (Myers and Worm, 2003, 2005) and global elasmobranch (shark and ray) populations are in long-term decline (Myers and Worm, 2003, 2005; Dulvy et al., 2014; MacNeil et al., 2020; Dulvy et al., 2021; Pacoureau et al., 2021; Simpfendorfer et al., 2023). The role of sharks in structuring marine communities (Ferretti et al., 2010; Heupel et al., 2014), and thus the potential for wider ecosystem impacts linked to ongoing depletion (Heithaus et al., 2008; McCauley et al., 2012), have prompted calls for conservation action (Chapman and Frisk, 2013). Popular approaches include implementing marine reserves or shark sanctuaries (Worm et al., 2013; Chapman et al., 2021), whereby spatial protection may facilitate population recovery (Speed et al., 2018). Successful outcomes, however, are linked both to anthropogenic influence (Kaplan et al., 2015; Juhel et al., 2018; Letessier et al., 2019; MacNeil et al., 2020) and ecological factors including species residency and movement ecology (Dwyer et al., 2020). A panacea for management would extend benefits to multiple species, but this highlights a prohibitive knowledge gap: the long-term mechanisms of shark coexistence (Heupel et al., 2019). Niche partitioning can facilitate species coexistence through reduced competition for resources (Chesson, 2000; Albrecht and Gotelli, 2001; Bethea et al., 2004; Heupel et al., 2018). There is evidence for this in sharks, particularly in the context of trophic (Curnick et al., 2019; Galindo et al., 2021; Chandelier et al., 2023; Weideli et al., 2023), and spatial partitioning (Gallagher et al., 2017; Matich et al., 2017; Afonso et al., 2022). Temporal niche partitioning, on the other hand, may be an important ecosystem process but has received little research attention (Lear et al., 2021) - it is unclear even how widespread it is amongst marine apex and mesopredators (Bass et al., 2021). Sharks are a diverse taxonomic group, in which ecological interactions between the same sympatric species can differ between locations (Shiffman et al., 2019). Hence, elucidating processes that structure shark communities should be a priority, to both better predict and mitigate wider effects of ecosystem decline, and address specific knowledge gaps facing regional management.
Shark research priorities include population status, movement ecology and critical habitat areas (Jorgensen et al., 2022). Attention is skewed towards large, charismatic, high conservation value and/or heavily depleted species (Shiffman et al., 2020), while temperate species are relatively under-studied (Jorgensen et al., 2022). The smallspotted catshark Scyliorhinus canicula is a widespread Northeast Atlantic mesopredator (trophic level 3.8). It is listed as ‘Least Concern’ by the International Union for Conservation of Nature (IUCN) Red List, with a stable population (Finucci et al., 2021a). Nevertheless, it should be considered for species-level management attention given food web centrality (Wieczorek et al., 2018), genetically distinct populations within the UK (Gubili et al., 2014), and mesopredator linkage between lower and upper trophic levels (Vaudo and Heithaus, 2011). Furthermore, given widespread depletion of marine predatory biomass (Myers and Worm, 2003, 2005), the relative trophic position of mesopredators in exploited systems is likely higher than in unexploited systems (Prugh et al., 2009; Ritchie and Johnson, 2009). This could elevate the importance of species such as S. canicula in structuring marine communities. In contrast with S. canicula, the nursehound S. stellaris is listed as ‘Vulnerable’ by the IUCN Red List (Finucci et al., 2021b), yet relatively under-researched (Supplementary Table S1). Studies have identified refuging behaviour in both sheltered and exposed coastal environments, with some philopatry (Sims et al., 2005), and it is prominent bycatch in Celtic Sea, Irish Sea, and Bristol Channel lobster and crab pot fisheries (Moore et al., 2023). Nevertheless, ecological evidence paucity is concerning given trophic level (4.0), sports fishing blue economy importance (Tim Harrison, personal comms.), and widespread availability in fishmongers and food takeaway establishments (Hobbs et al., 2019).
International programmes such as the Decade of Ocean Science for Sustainable Development (Ryabinin et al., 2019), the European Union (EU) Biodiversity Strategy 2030 (European Commission: Directorate-General for Environment, (2021)), and the EU Green Deal (European Commission: Directorate-General for Research and Innovation, (2021)) present opportunities for ambitious marine spatial planning targets. These should seek to strengthen species and habitat protection, and incorporate restoration and recovery objectives (Santos et al., 2019; Harris et al., 2022; Holness et al., 2022; Manea et al., 2023). In Wales, UK, a network of Special Areas of Conservation (SAC) has general objectives to safeguard species and habitats under the Habitats Directive (SACs; Habitats Directive, 1992). Additional regional biodiversity action plans include the Welsh Marine Evidence Strategy (2019-2025) and the Welsh National Marine Plan. Given that the most vulnerable species to extinction in continental shelf seas are long-lived and occupy upper trophic levels (Coulon et al., 2023), meeting these targets requires regional level species ecology knowledge. As such, there is currently an important and timely opportunity to contribute research-driven locally relevant ecological evidence to further strengthen national SAC management objectives in line with international programmes.
Baited remote underwater video stations (BRUVS) are commonly used to study elasmobranch ecology including distribution (White et al., 2013) and population trends (Flowers et al., 2022), behaviour (Sherman et al., 2020; Lester et al., 2022) and trophic interactions (Bond et al., 2019), anthropogenic influence (Clementi et al., 2021; Letessier et al., 2024), and diversity (Simpfendorfer et al., 2023) and abundance patterns (Bond et al., 2012; MacNeil et al., 2020; Cook et al., 2024). Here we undertook, to our knowledge, the first standardised BRUVS sampling of the shark community assemblage in Cardigan Bay SAC, Wales, UK. Specific objectives were to: 1) establish a baseline of shark species present; 2) elucidate spatio-temporal variations in relative abundance of shark species observed; and, 3) investigate mechanisms of species co-existence in the context of regional spatiotemporal habitat use by sharks.
Materials and methods
The Cardigan Bay SAC, west Wales, covers approximately 1000 km2, extending from Ceibwr Bay in Pembrokeshire to Aberarth in Ceredigion (Figure 1). The SAC status is based on seven qualifying features under Annex I and II of the Habitats Directive, including river lamprey Lampetra fluviatilis, semi-resident bottlenose dolphins Tursiops truncatus, and submerged or partially submerged sea caves. The SAC is important for the regional blue economy, particularly wildlife watching tours. Sports fishing charters seasonally catch and release sharks including Scyliorhinus stellaris and tope Galeorhinus galeus, while S. canicula can be bycatch in small scale local fisheries (Tim Harrison, personal comms.).
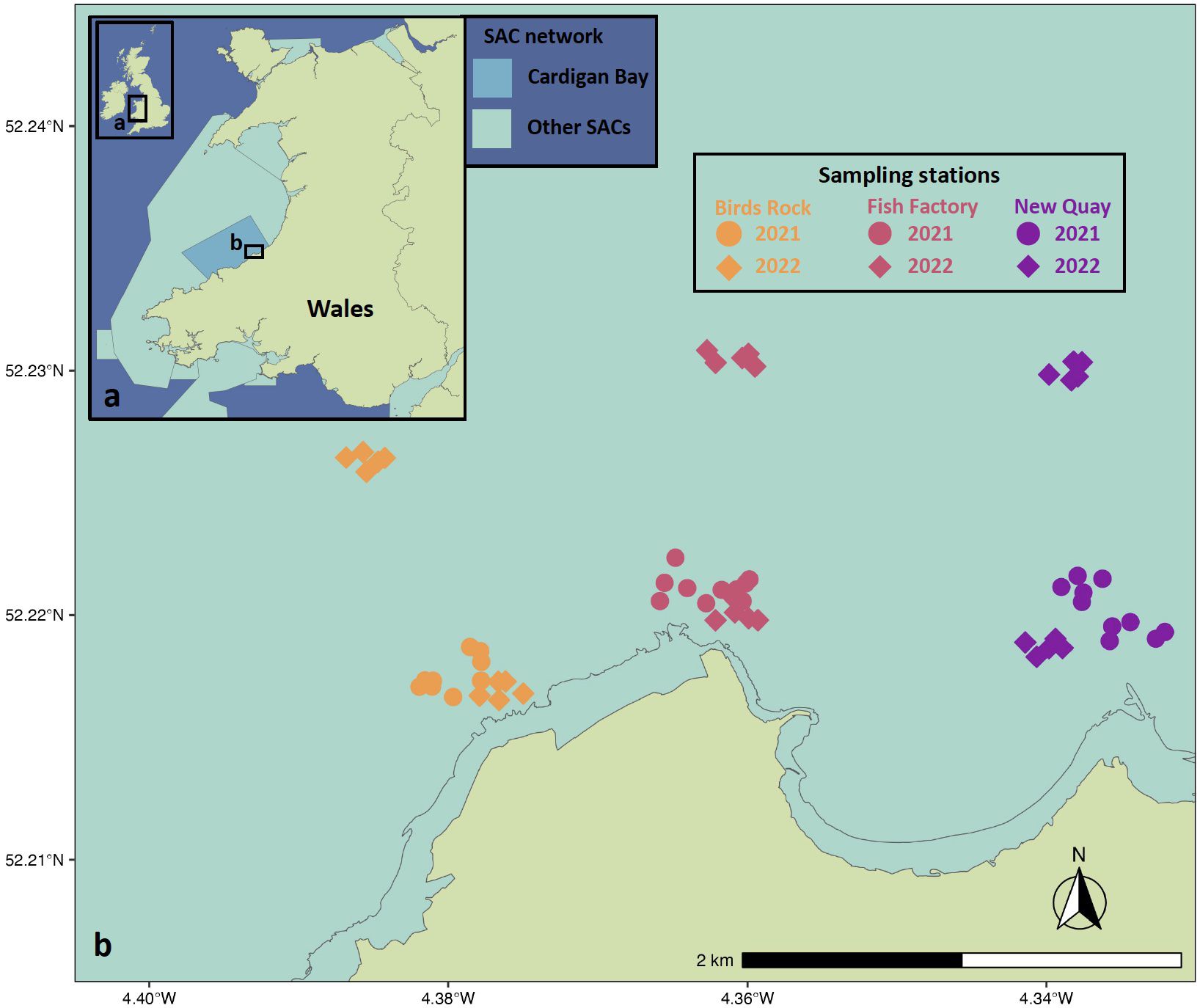
Figure 1. Wales, UK (A) location of Special Areas of Conservation (SAC), including Cardigan Bay SAC. (B) Cardigan Bay SAC baited remote underwater video stations sampling sites (Birds Rock, Fish Factory, New Quay) with stations therein, including spatially discrete offshore and nearshore stations sites sampled in May, July and September 2021, and March, May, July and September 2022.
Three sampling sites were identified to represent different habitat types at comparable distances from the closest human population centre (New Quay; 52.21515, -4.35887), all frequently visited by wildlife watching boat trips (Sarah Perry, personal comms.). All sampling areas were approximately 1 km2, within which GPS coordinates for sampling points (hereafter, stations) were randomly generated using the Cruise tool in Google Earth. Ten stations were generated per sampling site for 2021. For 2022, an additional three offshore sampling sites were added, both to increase spatial coverage of the study and to explore spatial connectivity between nearshore sites and wider Cardigan Bay. Five stations were randomly generated per sampling site for 2022 (Figure 1; Supplementary Table S2). Thus, each repeat sampling effort aimed to cover a total of 30 stations across 5 days, although this was not always possible due to weather conditions. Sampling was conducted in May (12th – 16th), July (5th – 9th) and September (22nd – 25th) 2021, and March (28th – 29th), May (23rd), July (11th – 16th), and September (12th – 13th) 2022.
The BRUVS setup comprised an aluminium frame (approximately 1 m x 0.6 m x 0.6 m) with a 1 m bait arm in front of the camera position and supporting a wire mesh cage in the middle of the field of view. A GoPro Hero7 Black camera set to 1440 video format at 30 frames per second was mounted in a GoPro SuperSuit housing (60 m depth rated), approximately 40 cm from the base. The mesh cage was baited with approximately 1 kg of chopped oily fish, scad Trachurus trachurus or herring Clupea harengus, to encourage bait plume dispersal (Dorman et al., 2012; Whitmarsh et al., 2017). A rope with sufficient scope to avoid toppling the BRUVS was attached to a surface buoy to mark location (Murray et al., 2019).
BRUVS were deployed between 09h00 and 17h00 to ensure sufficient light for video recording (Jones et al., 2021) and were left to record for a minimum of 60 minutes before retrieval. This both aligns with recommended protocol (Langlois et al., 2020) and is the minimum time required to sample 66% of fish species including several shark species in nearshore North Atlantic waters (Unsworth et al., 2014), and therefore conducive to meeting our research objectives. Up to 11 stations were sampled per day, and BRUVS deployed simultaneously were separated by at least 1 km to ensure independent sampling (Harvey et al., 2019). A Garmin GPSMAP 86s handheld GPS was used to navigate to each station. Depth was either estimated using a rope marked at 50 cm intervals and weighted with a dive weight (2021), or measured using a Platimo Echotest II handheld depth sounder (2022). Additional data were recorded at both drop and haul time: Beaufort wind force scale as a measure of sea state and percentage cloud cover.
Data recording began when the BRUVS settled on the substrate. Video quality was assessed using standardised criteria (Jones et al., 2021), with four rankings from excellent to unusable (Supplementary Figure S1). This excluded a similar proportion of datasets from each site from analysis (Supplementary Figure S1), yielding a total of 121 video datasets for full annotation (Table 1; Supplementary Table S2). For those retained for full annotation, sampling effort was standardised with two independent observers watching each video file to 60 minutes using QuickTime Player software. One observer watched real-time playback to identify which stations yielded a shark observation. These observations were cross-referenced by a senior annotator watching at double playback speed, who also recorded species and the time of first arrival for each species of shark observed for each BRUVS. Next, we recorded MaxN, defined as the maximum number of individuals per species in the field of view at any given point in time throughout a BRUVS set. This prevents double counting individuals (Willis et al., 2000).
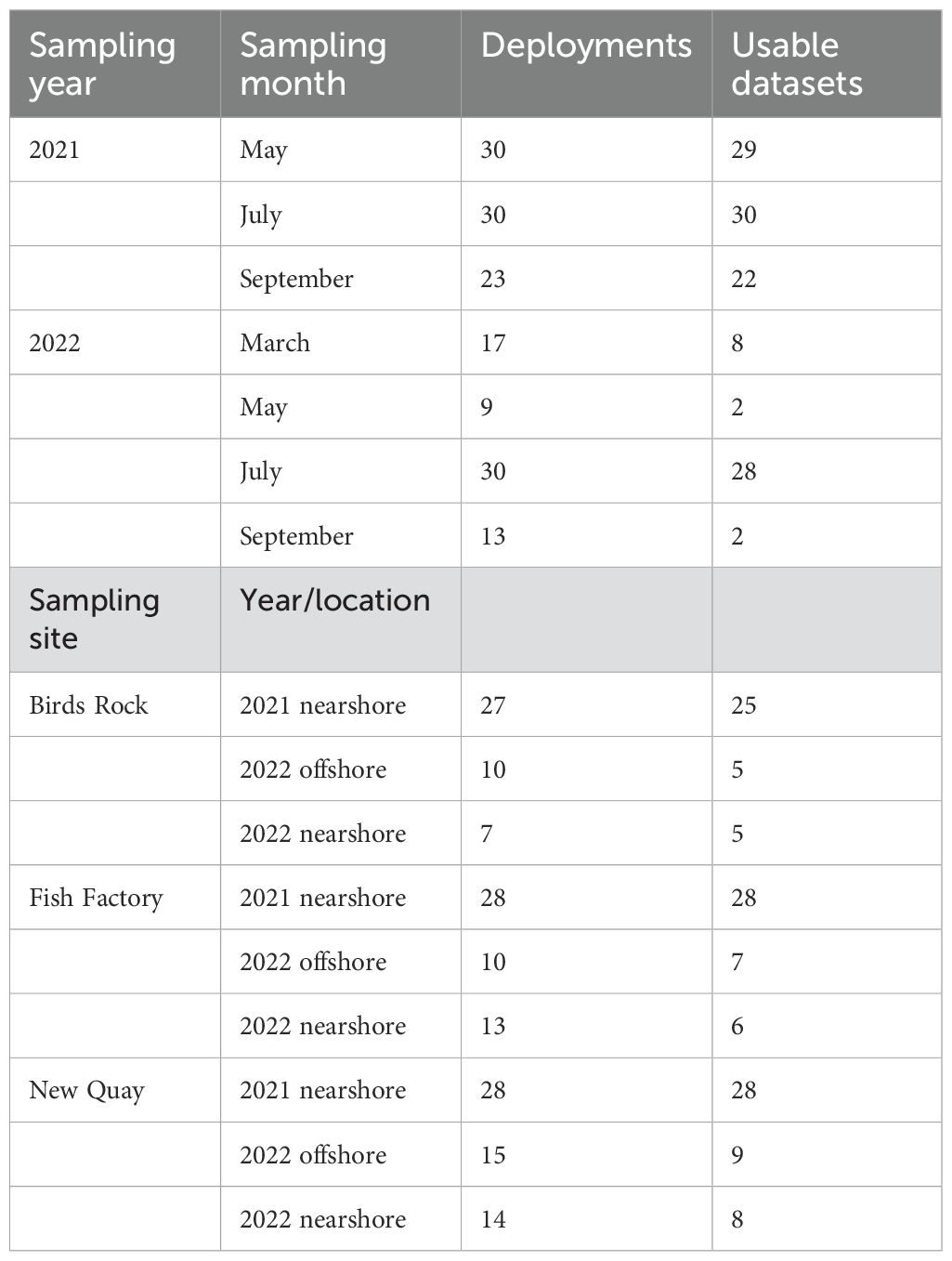
Table 1. Baited remote underwater video stations set in the Cardigan Bay Special Area of Conservation per year, site and habitat, and the number of usable sets according to standardised criteria (Jones et al., 2021).
Data were analysed using R software (v4.2.2; R Core Team, 2022). We first calculated mean MaxN ± standard error (SE) as the metric for relative abundance to standardise sampling effort among study sites (Goetze et al., 2018). We then excluded Mustelus sp. from further analyses given only a single observation of this taxon. Given non-normal data distribution, we used generalised linear models to investigate the association between sampling year and month (temporal explanatory variables), site and nearshore/offshore location (spatial explanatory variables), depth and image quality (environmental explanatory variables), MaxN of other shark species observed (proxy for partitioning), and the relative abundance of each species (MaxN; response variable). We examined error structure using the R package ‘DHARMa’ (Hartig, 2020) to check residual diagnostics for all response variables, selecting a negative binomial structure for both S. canicula and S. stellaris (Supplementary Table S3). We then used the ‘dredge’ function in the R package ‘MuMIn’ (Barton, 2020) to identify all possible variable combinations, followed by an information theoretic approach (Akaike’s information criteria, AIC; Akaike, 1998) to identify the best predictive model (ΔAIC = 0; Supplementary Table S4).
Results
We recorded sharks on 43 of 152 of BRUVS deployed in the Cardigan Bay SAC, Wales (28%; Supplementary Figure S2). Total species richness was three: smallspotted catshark Scyliorhinus canicula (Figure 2), nursehound S. stellaris (Figure 2), and an individual smooth hound shark Mustelus sp. of unknown species. The most frequently sighted species, S. canicula, was recorded on 19% of BRUVS, compared with 16% for S. stellaris (Supplementary Figure S2). Furthermore, of those BRUVS that yielded sightings, 75% recorded both S. canicula and S. stellaris within the first 40 minutes of recording (Supplementary Figure S3), justifying our standardised 60 minutes sampling effort.
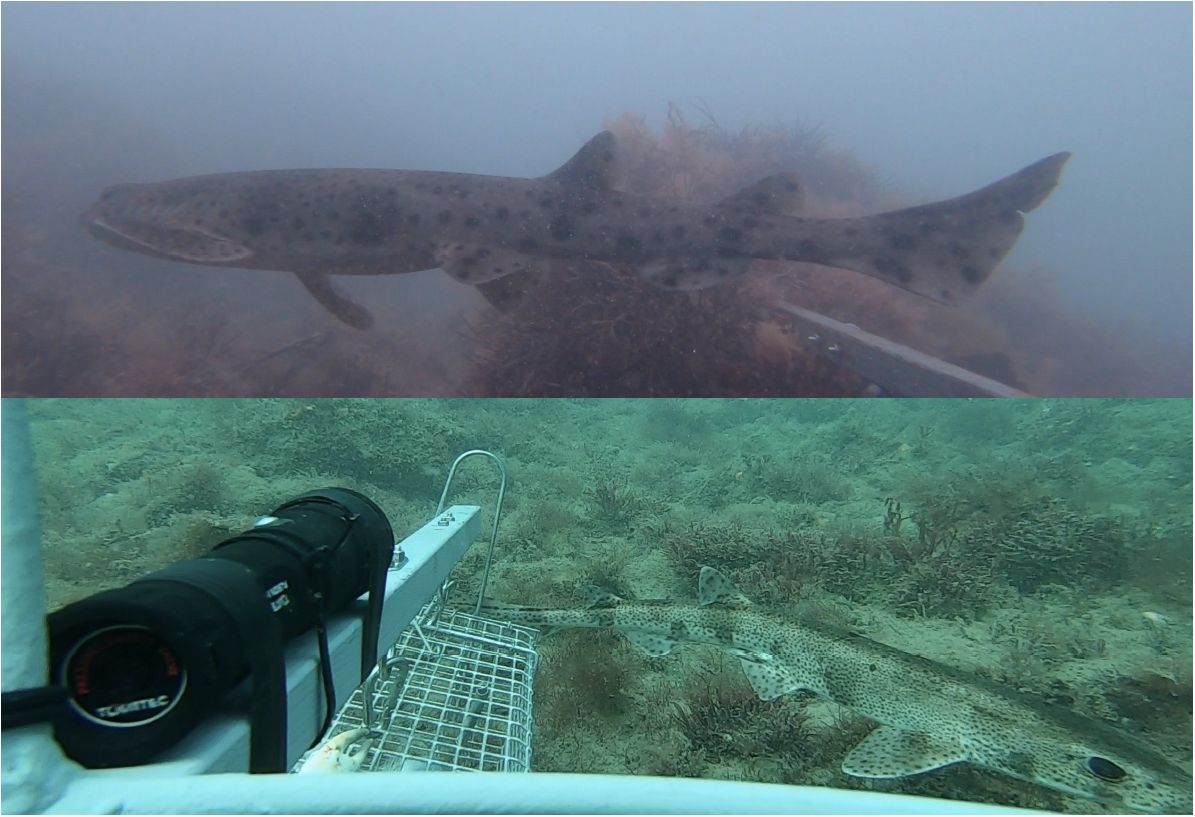
Figure 2. A smallspotted catshark Scyliorhinus canicula (below), and a nursehound S. stellaris (above), observed during baited remote underwater video stations sampling in the Cardigan Bay Special Area of Conservation, UK. Note S. stellaris second dorsal fin origin well ahead of anal fin insertion, anal fin base equal or greater than interdorsal space, and longer than anal-caudal fin space, blotches on flank.
Mean MaxN of S. canicula varied between 0.78 ± 0.17 at the offshore New Quay sites, and 0 ± 0 at the nearshore Fish Factory sites (Figure 3), while mean MaxN of S. stellaris varied between 0.97 ± 0.16 at the nearshore New Quay sites and 0 ± 0 at both the Birds Rock and Fish Factory offshore sites (Figure 3). The results of our GLM analysis (p < 0.0001; Supplementary Table S5) support this finding that the New Quay sites are more important than either of the other sites for both species, although particularly for S. stellaris and at the nearshore rather than offshore New Quay sites (Table 2).
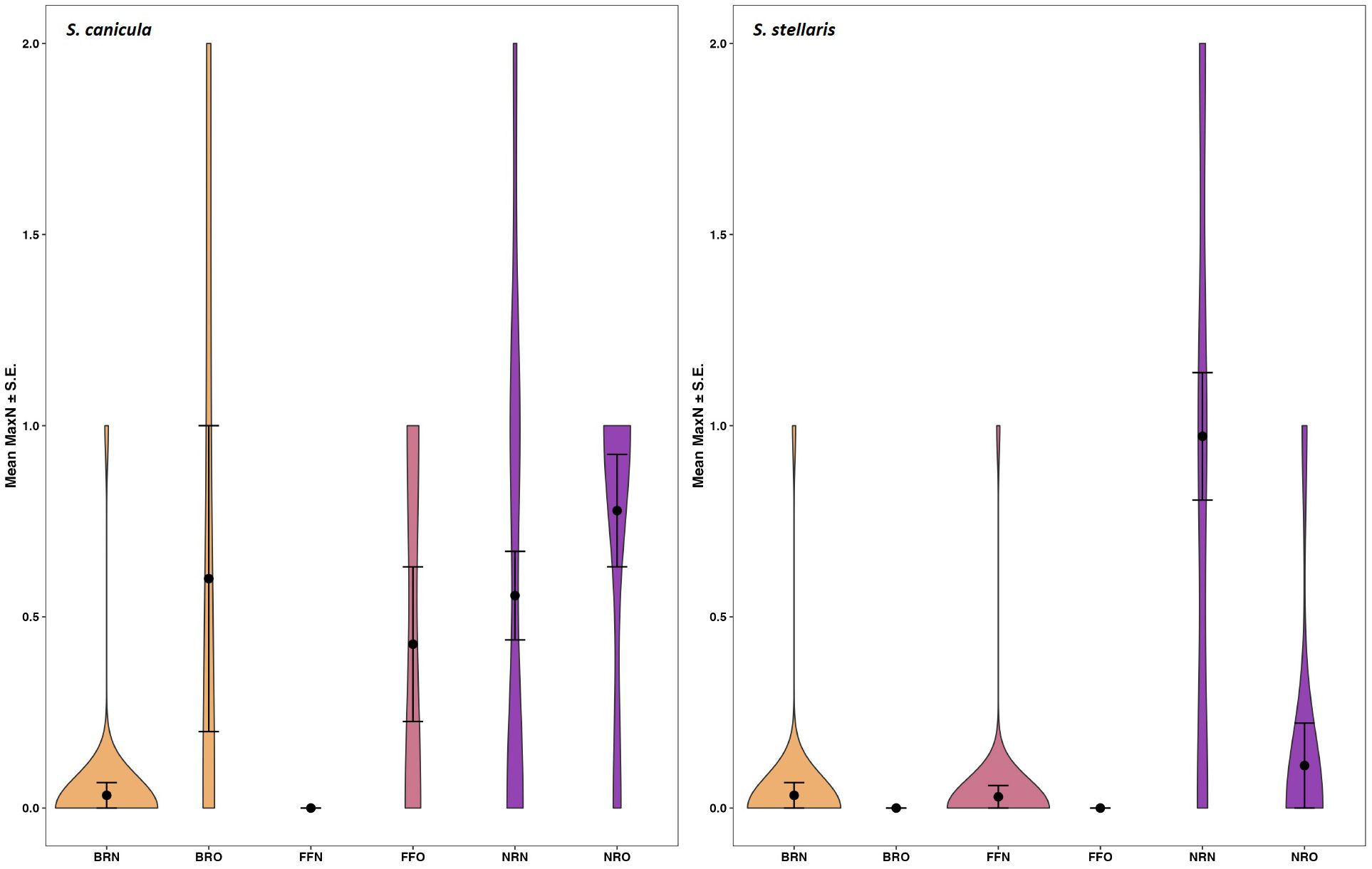
Figure 3. Mean MaxN (± S.E.) of smallspotted catsharks, Scyliorhinus canicula (left), and nursehounds, Scyliorhinus stellaris (right) recorded at baited remote underwater video stations sampling sites in the Cardigan Bay Special Area of Conservation, UK. Site codes: BR, Birds Rock; FF, Fish Factory; NR, New Quay; “N” suffix, Nearshore; “O” suffix, Offshore.
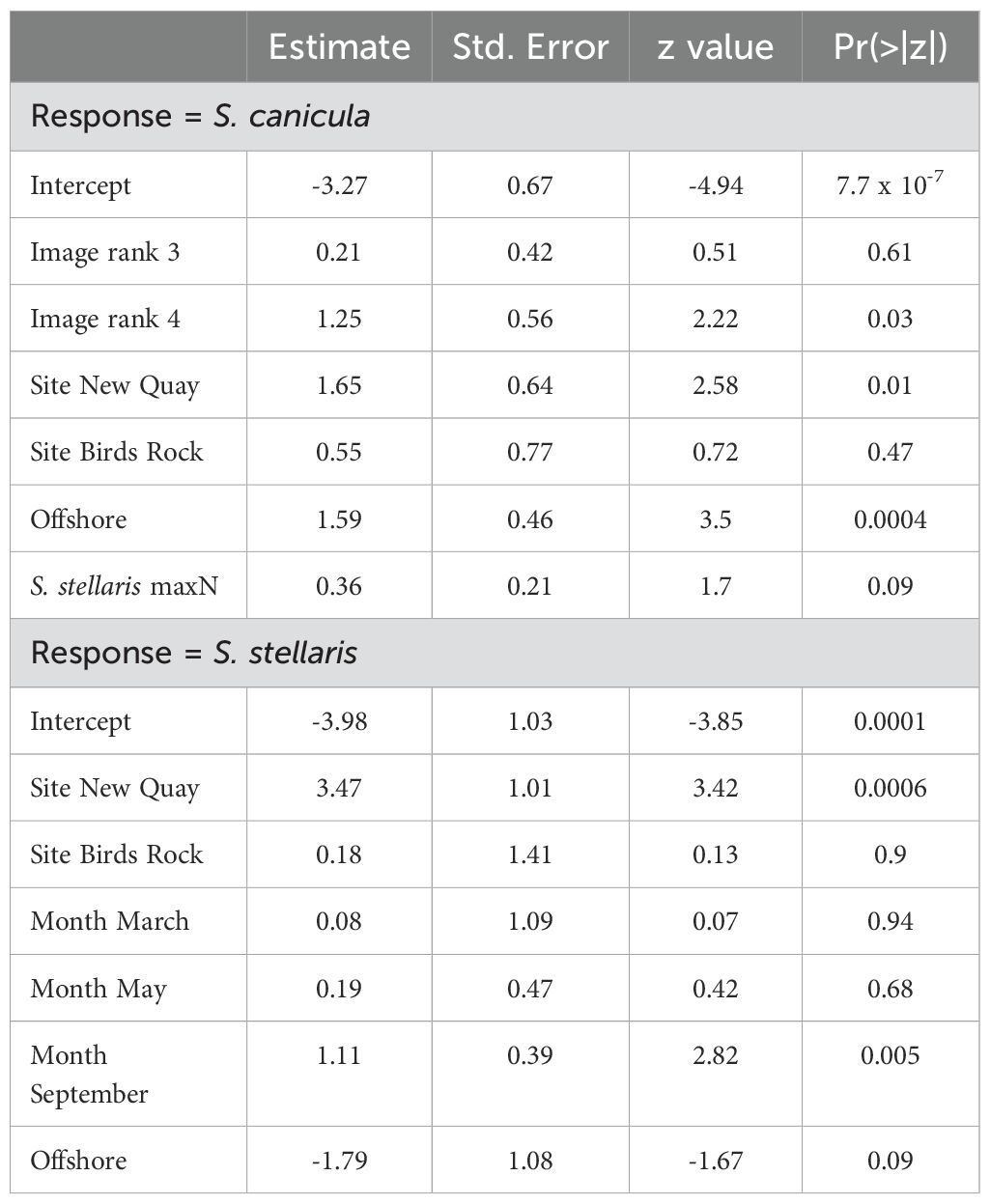
Table 2. Model-averaged coefficients from top models predicting the association between environmental variables and MaxN observations of smallspotted catsharks Scyliorhinus canicula and nursehounds S. stellaris recorded at baited remote underwater video stations sampling sites in the Cardigan Bay Special Area of Conservation, UK.
Regarding temporal variation, sampling month was a factor in the second top and the top model predicting MaxN of S. canicula and S. stellaris, respectively (Supplementary Table S4), with GLM analysis revealing an association between sampling month and S. stellaris MaxN (p < 0.01; Table 2; Supplementary Table S5). Temporal patterns of mean MaxN throughout the year at the New Quay sites were approximately inversely proportional for each species (Figure 4); relative abundance of S. canicula was highest in March (1.0 ± 0) and lowest in July (0.37 ± 0.14), while for S. stellaris this was highest in September (1.7 ± 0.3) and lowest in March (0.25 ± 0.25).
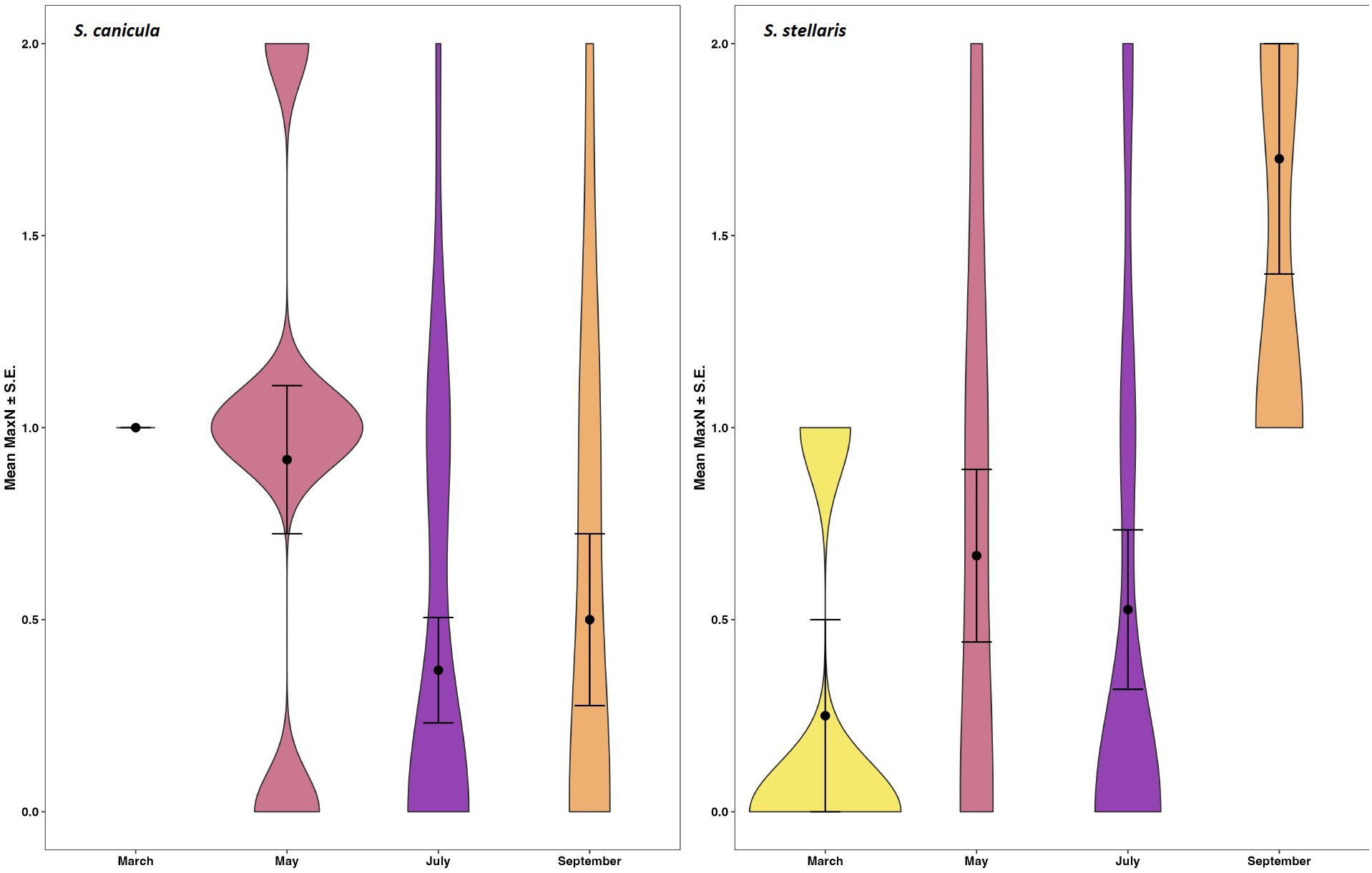
Figure 4. Temporal variation in mean MaxN (± S.E.) of smallspotted catsharks Scyliorhinus canicula (left) and nursehounds S. stellaris (right) recorded at baited remote underwater video stations within the New Quay sampling sites in the Cardigan Bay Special Area of Conservation, UK.
Discussion
Elucidating mechanisms that structure marine communities is fundamental for management, not least to predict and mitigate negative global change impacts. Sharks are imperiled predators, and long-term research bias resulting in ecological evidence paucity for less charismatic species is a concern given increased mesopredator importance in depleted systems (Prugh et al., 2009; Ritchie and Johnson, 2009). Niche partitioning is an ecological process that may facilitate sympatry, yet remains a relative knowledge gap. Moreover, temporal niche partitioning may be an important driver but is less studied than either trophic or spatial partitioning. In this context, we use standardised BRUVS sampling to obtain novel insights into temporal niche partitioning amongst two sympatric mesopredator sharks. We identify approximately inversely proportional seasonal nearshore habitat use in a spatially discrete area within our study site, adding to an evidence base of ecologically similar marine predators that share space. These behaviours may facilitate long-term reduction of direct interactions and competition for resources, and our findings both suggest important habitat areas for multiple species and highlight opportunities for further research.
BRUVS are widely applied to investigate elasmobranch ecology but rely on individuals approaching sufficiently close to the camera to be identified, with inherent potential for species present to not be recorded. This may be the case with our dataset; species including tope Galeorhinus galeus and smoothhound Mustelus spp. are caught by recreational fishers within our study sites, but we observed only a single smoothhound. This is a shortcoming in our protocol and precludes establishing a baseline of species present in Cardigan Bay SAC, but also highlights an opportunity for alternative approaches to address this remaining knowledge gap, such as environmental DNA. Nevertheless, we have obtained evidence for spatial use by S. canicula and S. stellaris, two important mesopredators in nearshore Northeast Atlantic marine environments, with comparable trophic levels (3.8 ± 0.3 SE and 4.0 ± 0.3 SE, respectively; Froese and Pauly, 2000). This is notable given that S. stellaris distribution estimates have, to date, predominantly relied on invasive trawl or fisheries-dependent sampling (Martin et al., 2010; Moore et al., 2023) rather than in situ sampling. S. stellaris have also been under-reported compared to S. canicula for BRUVS set in the UK (Jones et al., 2021), and may be at risk of local extinction in parts of their distribution (Ragonese et al., 2013).
Our results suggest that the New Quay nearshore and offshore sites are substantially more important for both study species and should therefore be a priority for regional management, given both a higher observation frequency and higher relative abundance than the other study sites. Additionally, both S. stellaris and S. canicula were recorded during every sampling month and both sampling years at these sites. Furthermore, spatial partitioning and temporal abundance variations amongst S. canicula and S. stellaris may constitute temporal niche partitioning in these sites as distinct from the other study sites, although the influence of factors such as prey availability, reproduction or parturition remains unclear. For example, differential S. canicula relative abundance between March and May could reflect dispersal behaviours that drive ontogenetic and sexual segregation patterns (Cook, 2023). Notably, however, the highest seasonal relative abundance recorded for S. stellaris (September) correlates with bycatch peaks in Welsh lobster and crab pot fisheries (Moore et al., 2023). Resource partitioning, whether for space, time, or diet, facilitates the co-existence of sympatric species (Bethea et al., 2004; Page et al., 2005; Dale et al., 2011; Fossette et al., 2017; Weideli et al., 2023), and may be a key mechanistic driver of marine community composition (Kinney et al., 2011; Gavrilchuk et al., 2014). Trophic partitioning in sharks has been extensively studied through dietary analyses (Papastamatiou et al., 2006; Sommerville et al., 2011; Vaudo and Heithaus, 2011; Heithaus et al., 2013; Tilley et al., 2013) and, despite similar trophic levels, has been observed between S. canicula and S. stellaris (see Yemisken et al., 2019). Temporal niche partitioning is less well documented, although distinct diel activity patterns within coastal shark assemblages may facilitate temporal partitioning of foraging times by driving less dominant predators to suboptimal foraging times (Lear et al., 2021). Importantly, our BRUVS sampling was only conducted during daylight hours, therefore it was not possible to investigate temporal niche partitioning in the context of either diel or nocturnal behaviours. Although weather conditions prevented sampling from October 2021 to March 2022, we would expect continued correlation between abundance patterns and regional pot fisheries bycatch (see Moore et al., 2023). Should it remain infeasible to test this in the future using BRUVS (with either or both seasonal and nocturnal sampling using lights), acoustic telemetry could elucidate the period of partitioning behaviour, whether on a short cycle (days) or a long cycle (months/seasons).
Spatial distribution away from core habitat areas (here, New Quay nearshore) is important to determine spatial connectivity and migration corridors. Here, S. stellaris was only detected in the New Quay sites, while S. canicula was detected in both the New Quay sites as well as the offshore sites of both Birds Rock and Fish Factory. The sample size is small but suggests differential use of areas around the core habitat area that may reflect differential movement ecology, and should be further investigated. S. canicula show a strong tendency to repeatedly use the same entry and exit areas between a marine protected area and surrounding waters in Northwest Spain (Papadopoulo et al., 2023). Regional management requires the identification of such priority areas for protection.
Our results suggest habitats under Cardigan Bay SAC designation are potentially important areas for both S. canicula and S. stellaris. These findings are a step towards the ecological evidence base needed for regional management to confer conservation benefits on local shark populations. Standardised BRUVS sampling yields comparable datasets and conservation insights on unprecedented scales (MacNeil et al., 2020; Simpfendorfer et al., 2023). Therefore we strongly recommend engagement with other researchers to align continued BRUVS monitoring of Cardigan Bay with refined objectives and standardised protocols. For example, better speciation of visually similar species (such as S. canicula and S. stellaris, and G. galeus and M. mustelus) arising from small camera advancement encourages the inclusion of reference images in BRUVS studies. Individual identification of sharks enables novel insights into social network structure (Mourier et al., 2012; Jacoby et al., 2021; Mourier and Planes, 2021), and has been achieved with S. canicula in a laboratory setting (Hook et al., 2019). Verifying in situ individual identification of S. canicula and S. stellaris on BRUVS could strengthen population estimates and present a more affordable and non-invasive alternative to traditional tagging studies, yet yield similar insights (Flowers et al., 2017; Cerutti-Pereyra et al., 2018). Stereo-BRUVS analysis that yields size estimates could be used to determine age classes and possible ecological drivers of habitat use, such as reproduction or predator avoidance. For example, nursery areas are used by several shark species (Zanella et al., 2019; TinHan et al., 2020; LaFreniere et al., 2023), including S. canicula (see Cau et al., 2013, 2017). These are areas where neonate or young-of-year sharks are more commonly encountered than in other areas, have a tendency to remain or return for extended periods, and use the area repeatedly across years (Heupel et al., 2007), and are thus candidates for protection to safeguard critical life processes such as parturition and maturation.
A paucity of mesopredator ecological evidence is prohibitive for effective management design, and should not be disregarded given the ecological importance of these species in depleted systems. Sharks including S. stellaris and S. canicula should be more prominent within SAC research and management programmes, particularly in the context of identifying regionally important shark areas such as nursery habitats and migration corridors. These objectives are all relevant for conservation planning, not least the Welsh Marine Evidence Strategy (2019-2025) and Welsh National Marine Plan, but also towards the objectives of the international Decade of Ocean Science for Sustainable Development (Ryabinin et al., 2019), and could thus strengthen the evidence base for protection measures to promote regional shark recovery.
Data availability statement
The raw data supporting the conclusions of this article will be made available by the authors, without undue reservation.
Ethics statement
Ethical approval was not required for the study involving animals in accordance with the local legislation and institutional requirements because sampling was conducted using non-invasive camera technology.
Author contributions
NC: Conceptualization, Data curation, Formal analysis, Investigation, Methodology, Project administration, Writing – original draft, Writing – review & editing. AJ: Data curation, Investigation, Writing – review & editing. SLP: Data curation, Investigation, Supervision, Writing – review & editing. SEP: Conceptualization, Supervision, Writing – review & editing. JC: Conceptualization, Supervision, Writing – review & editing.
Funding
The author(s) declare financial support was received for the research, authorship, and/or publication of this article. NC was supported by a Knowledge Economy Skills Scholarship 2 (KESS 2) PhD programme. KESS 2 is a pan-Wales higher level skills initiative led by Bangor University on behalf of the HE sector in Wales. It is part funded by the Welsh Government’s European Social Fund (ESF).
Acknowledgments
We thank collaborators at the Cardigan Bay Marine Wildlife Centre, part of the Wildlife Trust of South and West Wales, for support. A special thank you to Steve Hartley of Dolphin Survey Boat Trips for providing the boats, crew and operational support, and to boat skippers Simon Rigby, and Hefin Davies. We also thank New Quay residents for their interest in and support of our work, including Winston Evans, Tim Harrison and Jamie Briddon for sourcing BRUVS bait. Lastly, we thank Isa Pais, Sven Hoehn, Chessie Mason and Tyler Cuddy for fieldwork assistance, James Cave for video annotation assistance, and Jonny Greatrex for designing and building BRUVS base frames.
Conflict of interest
The authors declare that the research was conducted in the absence of any commercial or financial relationships that could be construed as a potential conflict of interest.
Publisher’s note
All claims expressed in this article are solely those of the authors and do not necessarily represent those of their affiliated organizations, or those of the publisher, the editors and the reviewers. Any product that may be evaluated in this article, or claim that may be made by its manufacturer, is not guaranteed or endorsed by the publisher.
Supplementary material
The Supplementary Material for this article can be found online at: https://www.frontiersin.org/articles/10.3389/fmars.2024.1443357/full#supplementary-material
References
Afonso A. S., Macena B. C. L., Mourato B., Bezerra N. P. A., Mendonça S., de Queiroz J. D. G. R., et al. (2022). Trophic-mediated pelagic habitat structuring and partitioning by sympatric elasmobranchs. Front. Mar. Sci. 9. doi: 10.3389/fmars.2022.779047
Akaike H. (1998). “Information theory and an extension of the maximum likelihood principle,” in Selected papers of Hirotugu Akaike. Eds. Parzen E., Tanabe K., Kitagawa G. (Springer, New York, NY), 199–213.
Albrecht M., Gotelli N. J. (2001). Spatial and temporal niche partitioning in grassland ants. Oecologia 126, 134–141. doi: 10.1007/s004420000494
Barton K. (2020). MuMIn: Multi-Model Inference. Available online at: https://CRAN.R-project.org/package=MuMIn (accessed June 3, 2024).
Bass N. C., Day J., Guttridge T. L., Knott N. A., Brown C. (2021). Intraspecific variation in diel patterns of rocky reef use suggests temporal partitioning in Port Jackson sharks. Mar. Freshw. Res. 72, 1445–1456. doi: 10.1071/MF20204
Bethea D. M., Buckel J. A., Carlson J. K. (2004). Foraging ecology of the early life stages of four sympatric shark species. Mar. Ecol. Prog. Ser. 268, 245–264. doi: 10.3354/meps268245
Bond M. E., Babcock E. A., Pikitch E. K., Abercrombie D. L., Lamb N. F., Chapman D. D. (2012). Reef sharks exhibit site-fidelity and higher relative abundance in marine reserves on the mesoamerican barrier reef. PloS One 7, e32983. doi: 10.1371/journal.pone.0032983
Bond M. E., Valentin-Albanese J., Babcock E. A., Heithaus M. R., Grubbs R. D., Cerrato R., et al. (2019). Top predators induce habitat shifts in prey within marine protected areas. Oecologia 190, 375–385. doi: 10.1007/s00442-019-04421-0
Cau A., Follesa M. C., Bo M., Canese S., Bellodi A., Cannas R., et al. (2013). Leiopathes glaberrima forest from South West Sardinia: a thousand years old nursery area for the small spotted catshark Scyliorinus canicula. Rapp. Commun. Int. Mer Médit 40, 717. doi: 10.1002/aqc.2717
Cau A., Follesa M. C., Moccia D., Bellodi A., Mulas A., Bo M., et al. (2017). Leiopathes glaberrima millennial forest from SW Sardinia as nursery ground for the small spotted catshark Scyliorhinus canicula. Aquat. Conservation: Mar. Freshw. Ecosyst. 27, 731–735.
Cerutti-Pereyra F., Bassos-Hull K., Arvizu-Torres X., Wilkinson K., García-Carrillo I., Perez-Jimenez J., et al. (2018). Observations of spotted eagle rays (Aetobatus narinari) in the Mexican Caribbean using photo-ID. Environ. Biol. Fishes 101, 237–244. doi: 10.1007/s10641-017-0694-y
Chandelier G., Kiszka J. J., Dulau-Drouot V., Jean C., Poirout T., Estrade V., et al. (2023). Isotopic niche partitioning of co-occurring large marine vertebrates around an Indian Ocean tropical oceanic island. Mar. Environ. Res. 183, 105835. doi: 10.1016/j.marenvres.2022.105835
Chapman D. D., Ali K., MacNeil M. A., Heupel M. R., Meekan M., Harvey E. S., et al. (2021). Long-term investment in shark sanctuaries. Science 372, 473–473. doi: 10.1126/science.abj0147
Chapman D. D., Frisk M. G. (2013). Give shark sanctuaries a chance. Science 339, 757. doi: 10.1126/science.339.6121.757-a
Chesson P. (2000). Mechanisms of maintenance of species diversity. Annu. Rev. Ecol. Systematics 31, 343–366. doi: 10.1146/annurev.ecolsys.31.1.343
Clementi G. M., Babcock E. A., Valentin-Albanese J., Bond M. E., Flowers K. I., Heithaus M. R., et al. (2021). Anthropogenic pressures on reef-associated sharks in jurisdictions with and without directed shark fishing. Mar. Ecol. Prog. Ser. 661, 175–186. doi: 10.3354/meps13607
Cook N. (2023). Casting A Wide Net: Ecological insights to strengthen marine spatial planning for coastal shark conservation. Cardiff, UK: Cardiff University, UK.
Cook N. D., Clementi G. M., Flowers K. I., Fanovich L., Cable J., Perkins S. E., et al. (2024). Elasmobranch diversity around the southern Caribbean island of Tobago: opportunities for conservation in a regional trade hub. J. Mar. Biol. Assoc. United Kingdom 104, 1–10. doi: 10.1017/S0025315423000917
Coulon N., Lindegren M., Goberville E., Toussaint A., Receveur A., Auber A. (2023). Threatened fish species in the Northeast Atlantic are functionally rare. Global Ecol. Biogeography 32, 1827–1845. doi: 10.1111/geb.v32.10
Curnick D. J., Carlisle A. B., Gollock M. J., Schallert R. J., Hussey N. E. (2019). Evidence for dynamic resource partitioning between two sympatric reef shark species within the British Indian Ocean Territory. J. Fish Biol. 94, 680–685. doi: 10.1111/jfb.13938
Dale J. J., Wallsgrove N. J., Popp B. N., Holland K. N. (2011). Nursery habitat use and foraging ecology of the brown stingray Dasyatis lata determined from stomach contents, bulk and amino acid stable isotopes. Mar. Ecol. Prog. Ser. 433, 221–236. doi: 10.3354/meps09171
Dorman S. R., Harvey E. S., Newman S. J. (2012). Bait effects in sampling coral reef fish assemblages with stereo-BRUVs. PloS One 7, e41538. doi: 10.1371/journal.pone.0041538
Dulvy N. K., Fowler S. L., Musick J. A., Cavanagh R. D., Kyne P. M., Harrison L. R., et al. (2014). Extinction risk and conservation of the world's sharks and rays. Elife 3. doi: 10.7554/eLife.00590
Dulvy N. K., Pacoureau N., Rigby C. L., Pollom R. A., Jabado R. W., Ebert D. A., et al. (2021). Overfishing drives over one-third of all sharks and rays toward a global extinction crisis. Curr. Biol. 31, 4773–4787. doi: 10.1016/j.cub.2021.08.062
Dwyer R. G., Krueck N. C., Udyawer V., Heupel M. R., Chapman D., Pratt H. L., et al. (2020). Individual and population benefits of marine reserves for reef sharks. Curr. Biol. 30, 480–489. doi: 10.1016/j.cub.2019.12.005
European Commission: Directorate-General for Environment. (2021). EU biodiversity strategy for 2030. Bringing nature back into our lives. Publications Office of the European Union. Available online at: https://data.europa.eu/doi/10.2779/677548
European Commission: Directorate-General for Research and Innovation. (2021). European Green Deal – Research & innovation call. Publications Office of the European Union. Available online at: https://data.europa.eu/doi/10.2777/33415
Ferretti F., Worm B., Britten G. L., Heithaus M. R., Lotze H. K. (2010). Patterns and ecosystem consequences of shark declines in the ocean. Ecol. Lett. 13, 1055–1071. doi: 10.1111/j.1461-0248.2010.01489.x
Finucci B., Derrick D., Neat F., Pacoureau N., Serena F., VanderWright W. (2021a). Scyliorhinus Canicula (The IUCN Red list of Threatened Species). doi: 10.2305/IUCN.UK.2021-2.RLTS.T161307554A124478351.en (accessed June 03, 2024).
Finucci B., Derrick D., Pacoureau N. (2021b). Scyliorhinus Stellaris (The IUCN Red list of Threatened Species), e.T161484A124493465. doi: 10.2305/IUCN.UK.2021-2.RLTS.T161484A124493465.en
Flowers K. I., Babcock E. A., Papastamatiou Y. P., Bond M. E., Lamb N., Miranda A., et al. (2022). Varying reef shark abundance trends inside a marine reserve: evidence of a Caribbean reef shark decline. Mar. Ecol. Prog. Ser. 683, 97–107. doi: 10.3354/meps13954
Flowers K., Henderson A., Lupton J., Chapman D. (2017). Site affinity of whitespotted eagle rays Aetobatus narinari assessed using photographic identification. J. Fish Biol. 91, 1337–1349. doi: 10.1111/jfb.2017.91.issue-5
Fossette S., Abrahms B., Hazen E. L., Bograd S. J., Zilliacus K. M., Calambokidis J., et al. (2017). Resource partitioning facilitates coexistence in sympatric cetaceans in the California Current. Ecol. Evol. 7, 9085–9097. doi: 10.1002/ece3.2017.7.issue-21
Froese R., Pauly D. (2000). FishBase 2000: Concepts, design and data sources (Los Baños, Laguna, Philippines: International Center for Living Aquatic Resources Management (ICLARM).
Galindo E., Giraldo A., Navia A. F. (2021). Feeding habits and trophic interactions of four sympatric hammerhead shark species reveal trophic niche partitioning. Mar. Ecol. Prog. Ser. 665, 159–175. doi: 10.3354/meps13681
Gallagher A. J., Shiffman D. S., Byrnes E. E., Hammerschlag-Peyer C. M., Hammerschlag N. (2017). Patterns of resource use and isotopic niche overlap among three species of sharks occurring within a protected subtropical estuary. Aquat. Ecol. 51, 435–448. doi: 10.1007/s10452-017-9627-2
Gavrilchuk K., Lesage V., Ramp C., Sears R., Berube M., Bearhop S., et al. (2014). Trophic niche partitioning among sympatric baleen whale species following the collapse of groundfish stocks in the Northwest Atlantic. Mar. Ecol. Prog. Ser. 497, 285–301. doi: 10.3354/meps10578
Goetze J. S., Langlois T. J., McCarter J., Simpfendorter C. A., Hughes A., Leve J. T., et al. (2018). Drivers of reef shark abundance and biomass in the Solomon Islands. PloS One 13, e0200960. doi: 10.1371/journal.pone.0200960
Gubili C., Sims D. W., Veríssimo A., Domenici P., Ellis J., Grigoriou P., et al. (2014). A tale of two seas: contrasting patterns of population structure in the small-spotted catshark across Europe. R. Soc. Open Sci. 1, 140175. doi: 10.1098/rsos.140175
Habitats Directive (1992). Council Directive 92/43/EEC of 21 May 1992 on the conservation of natural habitats and of wild fauna and flora. Off. J. Eur. Union 206, 50.
Harris L. R., Holness S. D., Kirkman S. P., Sink K. J., Majiedt P., Driver A. (2022). A robust, systematic approach for developing the biodiversity sector's input for multi-sector Marine Spatial Planning. Ocean Coast. Manage. 230, 106368. doi: 10.1016/j.ocecoaman.2022.106368
Hartig F. (2020). DHARMa: Residual Diagnostics for Hierarchical (Multi-Level / Mixed) Regression Models. Available online at: https://CRAN.R-project.org/package=DHARMa (accessed June 3, 2024).
Harvey E. S., Santana-Garcon J., Goetze J., Saunders B. J., Cappo M. (2019). The use of stationary underwater video for sampling sharks. In Carrier J. C., Heithaus M. R., Simpfendorfer C. A. (eds) Shark research: Emerging technologies and applications for the field and laboratory. Boca Raton, FL: Taylor & Francis, pp. 111–132.
Heithaus M. R., Vaudo J. J., Kreicker S., Layman C. A., Krutzen M., Burkholder D. A., et al. (2013). Apparent resource partitioning and trophic structure of large-bodied marine predators in a relatively pristine seagrass ecosystem. Mar. Ecol. Prog. Ser. 481, 225–237. doi: 10.3354/meps10235
Heithaus M. R., Frid A., Wirsing A. J., Worm B. (2008). Predicting ecological consequences of marine top predator declines. Trends Ecol. Evol. 23, 202–210. doi: 10.1016/j.tree.2008.01.003
Heupel M. R., Carlson J. K., Simpfendorfer C. A. (2007). Shark nursery areas: concepts, definition, characterization and assumptions. Mar. Ecol. Prog. Ser. 337, 287–297. doi: 10.3354/meps337287
Heupel M. R., Knip D. M., Simpfendorfer C. A., Dulvy N. K. (2014). Sizing up the ecological role of sharks as predators. Mar. Ecol. Prog. Ser. 495, 291–298. doi: 10.3354/meps10597
Heupel M. R., Lédée E. J. I., Simpfendorfer C. A. (2018). Telemetry reveals spatial separation of co-occurring reef sharks. Mar. Ecol. Prog. Ser. 589, 179–192. doi: 10.3354/meps12423
Heupel M. R., Munroe S. E. M., Lédée E. J. I., Chin A., Simpfendorfer C. A. (2019). Interspecific interactions, movement patterns and habitat use in a diverse coastal shark assemblage. Mar. Biol. 166, 68. doi: 10.1007/s00227-019-3511-7
Hobbs C. A. D., Potts R. W. A., Bjerregaard Walsh M., Usher J., Griffiths A. M. (2019). Using DNA barcoding to investigate patterns of species utilisation in UK shark products reveals threatened species on sale. Sci. Rep. 9, 1028. doi: 10.1038/s41598-018-38270-3
Holness S. D., Harris L. R., Chalmers R., De Vos D., Goodall V., Truter H., et al. (2022). Using systematic conservation planning to align priority areas for biodiversity and nature-based activities in marine spatial planning: A real-world application in contested marine space. Biol. Conserv. 271, 109574. doi: 10.1016/j.biocon.2022.109574
Hook S. A., McMurray C., Ripley D. M., Allen N., Moritz T., Grunow B., et al. (2019). Recognition software successfully aids the identification of individual small-spotted catsharks Scyliorhinus canicula during their first year of life. J. Fish Biol. 95, 1465–1470. doi: 10.1111/jfb.14166
Jacoby D. M., Fairbairn B. S., Frazier B. S., Gallagher A. J., Heithaus M. R., Cooke S. J., et al. (2021). Social network analysis reveals the subtle impacts of tourist provisioning on the social behavior of a generalist marine apex predator. Front. Mar. Sci. 8, 665726. doi: 10.3389/fmars.2021.665726
Jones R. E., Griffin R. A., Herbert R. J. H., Unsworth R. K. F. (2021). Consistency is critical for the effective use of baited remote video. Oceans 2, 215–232. doi: 10.3390/oceans2010013
Jorgensen S. J., Micheli F., White T. D., Van Houtan K. S., Alfaro-Shigueto J., Andrzejaczek S., et al. (2022). Emergent research and priorities for shark and ray conservation. Endangered Species Res. 47, 171–203. doi: 10.3354/esr01169
Juhel J. B., Vigliola L., Mouillot D., Kulbicki M., Letessier T. B., Meeuwig J. J., et al. (2018). Reef accessibility impairs the protection of sharks. J. Appl. Ecol. 55, 673–683. doi: 10.1111/1365-2664.13007
Kaplan K. A., Ahmadia G. N., Fox H., Glew L., Pomeranz E. F., Sullivan P. (2015). Linking ecological condition to enforcement of marine protected area regulations in the greater Caribbean region. Mar. Policy 62, 186–195. doi: 10.1016/j.marpol.2015.09.018
Kinney M. J., Hussey N. E., Fisk A. T., Tobin A. J., Simpfendorfer C. A. (2011). Communal or competitive? Stable isotope analysis provides evidence of resource partitioning within a communal shark nursery. Mar. Ecol. Prog. Ser. 439, 263–276. doi: 10.3354/meps09327
LaFreniere B. R., Sosa‐Nishizaki O., Herzka S. Z., Snodgrass O., Dewar H., Miller N., et al. (2023). Vertebral chemistry distinguishes nursery habitats of juvenile shortfin mako in the Eastern North Pacific Ocean. Mar. Coast. Fisheries 15, e10234. doi: 10.1002/mcf2.10234
Langlois T., Goetze J., Bond T., Monk J., Abesamis R. A., Asher J., et al. (2020). A field and video annotation guide for baited remote underwater stereo-video surveys of demersal fish assemblages. Methods Ecol. Evol. 11, 1401–1409. doi: 10.1111/2041-210x.13470
Lear K. O., Whitney N. M., Morris J. J., Gleiss A. C. (2021). Temporal niche partitioning as a novel mechanism promoting co-existence of sympatric predators in marine systems. Proc. R. Soc. B 288, 20210816. doi: 10.1098/rspb.2021.0816
Lester E., Langlois T., Lindgren I., Birt M., Bond T., McLean D., et al. (2022). Drivers of variation in occurrence, abundance, and behaviour of sharks on coral reefs. Sci. Rep. 12, 728. doi: 10.1038/s41598-021-04024-x
Letessier T. B., Mouillot D., Bouchet P. J., Vigliola L., Fernandes M. C., Thompson C., et al. (2019). Remote reefs and seamounts are the last refuges for marine predators across the Indo-Pacific. PloS Biol. 17, e3000366. doi: 10.1371/journal.pbio.3000366
Letessier T. B., Mouillot D., Mannocci L., Jabour Christ H., Elamin E. M., Elamin S. M., et al. (2024). Divergent responses of pelagic and benthic fish body-size structure to remoteness and protection from humans. Science 383, 976–982. doi: 10.1126/science.adi7562
MacNeil M. A., Chapman D. D., Heupel M., Simpfendorfer C. A., Heithaus M., Meekan M., et al. (2020). Global status and conservation potential of reef sharks. Nature 583, 801–806. doi: 10.1038/s41586-020-2692-z
Manea E., Agardy T., Bongiorni L. (2023). Link marine restoration to marine spatial planning through ecosystem-based management to maximize ocean regeneration. Aquat. Conservation: Mar. Freshw. Ecosyst. 3999, 1–13. doi: 10.1002/aqc.3999
Martin C., Vaz S., Ellis J., Coppin F., Le Roy D., Carpentier A. (2010). Spatio-temporal patterns in demersal elasmobranchs from trawl surveys in the Eastern English Channel, (1988–2008). Mar. Ecol. Prog. Ser. 417, 211–228. doi: 10.3354/meps08802
Matich P., Ault J. S., Boucek R. E., Bryan D. R., Gastrich K. R., Harvey C. L., et al. (2017). Ecological niche partitioning within a large predator guild in a nutrient-limited estuary. Limnology Oceanography 62, 934–953. doi: 10.1002/lno.10477
McCauley D. J., Hoffmann E., Young H. S., Micheli F. (2012). Night shift: expansion of temporal niche use following reductions in predator density. PloS One 7, e38871. doi: 10.1371/journal.pone.0038871
Moore A. B., Heney C., Lincoln H., Colvin C., Newell H., Turner R., et al. (2023). Bycatch in northeast Atlantic lobster and crab pot fisheries (Irish Sea, Celtic Sea and Bristol Channel). Fisheries Res. 265, 106745. doi: 10.1016/j.fishres.2023.106745
Mourier J., Planes S. (2021). Kinship does not predict the structure of a shark social network. Behav. Ecol. 32, 211–222. doi: 10.1093/beheco/araa119
Mourier J., Vercelloni J., Planes S. (2012). Evidence of social communities in a spatially structured network of a free-ranging shark species. Anim. Behav. 83, 389–401. doi: 10.1016/j.anbehav.2011.11.008
Murray R., Conales S., Araujo G., Labaja J., Snow S. J., Pierce S. J., et al. (2019). Tubbataha Reefs Natural Park: the first comprehensive elasmobranch assessment reveals global hotspot for reef sharks. J. Asia-Pac. Biodivers. 12 (1), 49–56. doi: 10.1016/j.japb.2018.09.009
Myers R. A., Worm B. (2003). Rapid worldwide depletion of predatory fish communities. Nature 423, 280–283. doi: 10.1038/nature01610
Myers R. A., Worm B. (2005). Extinction, survival or recovery of large predatory fishes. Philos. Trans. R. Soc. B 360, 13–20. doi: 10.1098/rstb.2004.1573
Pacoureau N., Rigby C. L., Kyne P. M., Sherley R. B., Winker H., Carlson J. K., et al. (2021). Half a century of global decline in oceanic sharks and rays. Nature 589, 567–571. doi: 10.1038/s41586-020-03173-9
Page B., McKenzie J., Goldsworthy S. D. (2005). Dietary resource partitioning among sympatric New Zealand and Australian fur seals. Mar. Ecol. Prog. Ser. 293, 283–302. doi: 10.3354/meps293283
Papadopoulo K., Villegas-Ríos D., Mucientes G., Hillinger A., Alonso-Fernández A. (2023). Drivers of behaviour and spatial ecology of the small spotted catshark (Scyliorhinus canicula). Aquat. Conservation: Mar. Freshw. Ecosyst. 33, 443–457. doi: 10.1002/aqc.3943
Papastamatiou Y. P., Wetherbee B. M., Lowe C. G., Crow G. L. (2006). Distribution and diet of four species of carcharhinid shark in the Hawaiian Islands: evidence for resource partitioning and competitive exclusion. Mar. Ecol. Prog. Ser. 320, 239–251. doi: 10.3354/meps320239
Prugh L. R., Stoner C. J., Epps C. W., Bean W. T., Ripple W. J., Laliberte A. S., et al. (2009). The rise of the mesopredator. Bioscience 59, 779–791. doi: 10.1525/bio.2009.59.9.9
Ragonese S., Vitale S., Dimech M., Mazzola S. (2013). Abundances of demersal sharks and chimaera from 1994-2009 scientific surveys in the central Mediterranean sea. PloS One 8, e74865. doi: 10.1371/journal.pone.0074865
R Core Team (2022). R: A Language and Environment for Statistical Computing (Vienna, Austria: R Foundation for Statistical Computing).
Ritchie E. G., Johnson C. N. (2009). Predator interactions, mesopredator release and biodiversity conservation. Ecol. Lett. 12, 982–998. doi: 10.1111/j.1461-0248.2009.01347.x
Ryabinin V., Barbière J., Haugan P., Kullenberg G., Smith N., McLean C., et al. (2019). The UN decade of ocean science for sustainable development. Front. Mar. Sci. 6. doi: 10.3389/fmars.2019.00470
Santos C. F., Ehler C. N., Agardy T., Andrade F., Orbach M. K., Crowder L. B. (2019). “Marine spatial planning,” in World Seas: An Environmental Evaluation, 2nd ed. (Kidlington, Oxford OX5 1GB, United Kingdom: Academic Press), 571–592.
Sherman C. S., Heupel M. R., Moore S. K., Chin A., Simpfendorfer C. A. (2020). When sharks are away, rays will play: effects of top predator removal in coral reef ecosystems. Mar. Ecol. Prog. Ser. 641, 145–157. doi: 10.3354/meps13307
Shiffman D. S., Ajemian M. J., Carrier J. C., Daly-Engel T. S., Davis M. M., Dulvy N. K., et al. (2020). Trends in chondrichthyan research: an analysis of three decades of conference abstracts. Copeia 108, 122–131. doi: 10.1643/OT-19-179R
Shiffman D. S., Kaufman L., Heithaus M., Hammerschlag N. (2019). Intraspecific differences in relative isotopic niche area and overlap of co-occurring sharks. Aquat. Ecol. 53, 233–250. doi: 10.1007/s10452-019-09685-5
Simpfendorfer C. A., Heithaus M. R., Heupel M. R., MacNeil M. A., Meekan M., Harvey E., et al. (2023). Widespread diversity deficits of coral reef sharks and rays. Science 380, 1155–1160. doi: 10.1126/science.ade4884
Sims D., Southall E., Wearmouth V., Hutchinson N., Budd G., Morritt D. (2005). Refuging behaviour in the nursehound Scyliorhinus stellaris (Chondrichthyes: Elasmobranchii): preliminary evidence from acoustic telemetry. J. Mar. Biol. Assoc. United Kingdom 85, 1137–1140. doi: 10.1017/S0025315405012191
Sommerville E., Platell M. E., White W. T., Jones A. A., Potter I. C. (2011). Partitioning of food resources by four abundant, co-occurring elasmobranch species: relationships between diet and both body size and season. Mar. Freshw. Res. 62, 54–65. doi: 10.1071/mf10164
Speed C. W., Cappo M., Meekan M. G. (2018). Evidence for rapid recovery of shark populations within a coral reef marine protected area. Biol. Conserv. 220, 308–319. doi: 10.1016/j.biocon.2018.01.010
Tilley A., López-Angarita J., Turner J. R. (2013). Diet reconstruction and resource partitioning of a Caribbean marine mesopredator using stable isotope bayesian modelling. PloS One 8, e79560. doi: 10.1371/journal.pone.0079560
TinHan T. C., O'Leary S. J., Portnoy D. S., Rooker J. R., Gelpi C. G., Wells R. D. (2020). Natural tags identify nursery origin of a coastal elasmobranch Carcharhinus leucas. J. Appl. Ecol. 57, 1222–1232. doi: 10.1111/1365-2664.13627
Unsworth R. K. F., Peters J. R., McCloskey R. M., Hinder S. L. (2014). Optimising stereo baited underwater video for sampling fish and invertebrates in temperate coastal habitats. Estuar. Coast. Shelf Sci. 150, 281–287. doi: 10.1016/j.ecss.2014.03.020
Vaudo J. J., Heithaus M. R. (2011). Dietary niche overlap in a nearshore elasmobranch mesopredator community. Mar. Ecol. Prog. Ser. 425, 247–260. doi: 10.3354/meps08988
Weideli O. C., Daly R., Peel L. R., Heithaus M. R., Shivji M. S., Planes S., et al. (2023). Elucidating the role of competition in driving spatial and trophic niche patterns in sympatric juvenile sharks. Oecologia 201, 673–688. doi: 10.1007/s00442-023-05355-4
White J., Simpfendorfer C. A., Tobin A. J., Heupel M. R. (2013). Application of baited remote underwater video surveys to quantify spatial distribution of elasmobranchs at an ecosystem scale. J. Exp. Mar. Biol. Ecol. 448, 281–288. doi: 10.1016/j.jembe.2013.08.004
Whitmarsh S. K., Fairweather P. G., Huveneers C. (2017). What is Big BRUVver up to? Methods and uses of baited underwater video. Rev. Fish Biol. Fisheries 27, 53–73. doi: 10.1007/s11160-016-9450-1
Wieczorek A. M., Power A. M., Browne P., Graham C. T. (2018). Stable-isotope analysis reveals the importance of soft-bodied prey in the diet of lesser spotted dogfish Scyliorhinus canicula. J. Fish Biol. 93, 685–693. doi: 10.1111/jfb.13770
Willis T. J., Millar R. B., Babcock R. C. (2000). Detection of spatial variability in relative density of fishes: comparison of visual census, angling, and baited underwater video. Mar. Ecol. Prog. Ser. 198, 249–260. doi: 10.3354/meps198249
Worm B., Davis B., Kettemer L., Ward-Paige C. A., Chapman D., Heithaus M. R., et al. (2013). Global catches, exploitation rates, and rebuilding options for sharks. Mar. Policy 40, 194–204. doi: 10.1016/j.marpol.2012.12.034
Yemisken E., Navarro J., Forero M., Megalofonou P., Eryilmaz L. (2019). Trophic partitioning between abundant demersal sharks coexisting in the North Aegean Sea. J. Mar. Biol. Assoc. United Kingdom 99, 1213–1219. doi: 10.1017/S0025315419000110
Keywords: Cardigan bay, elasmobranch, marine protected area, marine spatial planning, Northeast Atlantic, Scyliorhinidae
Citation: Cook ND, Jenkins A, Perry SL, Perkins SE and Cable J (2024) Temporal niche partitioning as a potential mechanism for coexistence in two sympatric mesopredator sharks. Front. Mar. Sci. 11:1443357. doi: 10.3389/fmars.2024.1443357
Received: 03 June 2024; Accepted: 30 September 2024;
Published: 21 October 2024.
Edited by:
Brendan Shea, Beneath the Waves, Inc., United StatesReviewed by:
Robert Bullock, Save Our Seas Foundation, SwitzerlandJeremy Jenrette, Virginia Tech, United States
Copyright © 2024 Cook, Jenkins, Perry, Perkins and Cable. This is an open-access article distributed under the terms of the Creative Commons Attribution License (CC BY). The use, distribution or reproduction in other forums is permitted, provided the original author(s) and the copyright owner(s) are credited and that the original publication in this journal is cited, in accordance with accepted academic practice. No use, distribution or reproduction is permitted which does not comply with these terms.
*Correspondence: Neil D. Cook, Y29va243QGNhcmRpZmYuYWMudWs=
†These authors share last authorship