- 1Shenzhen Key Laboratory of Nanozymes and Translational Cancer Research, Department of Otolaryngology, Shenzhen Institute of Translational Medicine, Shenzhen Second People’s Hospital, The First Affiliated Hospital of Shenzhen University, Guangdong Key Laboratory for Biomedical Measurements and Ultrasound Imaging, National-Regional Key Technology Engineering Laboratory for Medical Ultrasound, School of Biomedical Engineering, Shenzhen University Medical School, Shenzhen, China
- 2Center for Protein and Cell-Based Drugs, Institute of Biomedicine and Biotechnology, Shenzhen Institutes of Advanced Technology, Chinese Academy of Sciences, Shenzhen, China
- 3State Key Laboratory of Chemical Oncogenomics, Guangdong Provincial Key Laboratory of Chemical Genomics, Peking University Shenzhen Graduate School, Shenzhen, China
Lsm14b (LSM family member 14b) is a messenger ribonucleoprotein (mRNP) and a widely present component in eukaryotes. Lsm14b participates in oocyte development by regulating mRNA translation, however, the specific translational regulatory mechanisms remain unclear. Here, we explore the function of Lsm14b during early oocyte development and identify specific translational regulatory mechanisms. We established female-infertile lsm14b mutant zebrafish using CRISPR/Cas9. Histological examination showed that the oocyte development in the mutant zebrafish was arrested at the primary growth (PG) stage. The gene set enrichment analysis (GSEA) analysis of the transcriptome revealed that signaling pathways associated with mRNA translation suppression and mRNA poly(A) tail shortening were significantly downregulation in the mutant. The poly(A) tail length (PAT) assay confirmed the lengthen of mRNA poly(A) tail of the oocyte development-related genes zar1 and figla in the mutant. Further studies have suggested that the loss of Lsm14b triggers the unfolded protein response (UPR), which is related to abnormal translation inhibition. Our results demonstrate that Lsm14b assists in maintaining the translation-inhibited state of mRNA by regulating the length of the mRNA poly(A) tail in zebrafish early oocytes, which ensures that the mRNA synthesized and stored during the growth stage of oocytes, is necessary for the normal growth and development of oocytes.
1 Introduction
Mature and healthy oocytes are essential for reproduction in females (Keefe et al., 2015; Robker et al., 2018). The growth and development of oocytes are precisely regulated (Das and Arur, 2017; Conti and Franciosi, 2018; Alam and Miyano, 2020). During oogenesis, growing oocytes synthesize large amounts of mRNA, some of which, such as GDF9 and BMP15, are translated into proteins that participate in oocyte growth and follicle formation (Ma et al., 2013; De Castro et al., 2016; Sha et al., 2018; Dai et al., 2022). However, most mRNAs remain in a translationally inhibited state and are stored in a granular structure known as messenger ribonucleoprotein (mRNP) (Richter and Lasko, 2011; Winata and Korzh, 2018; Dai et al., 2019). The timely activation of these translation-inhibiting mRNAs at later stages is necessary for oocyte maturation and subsequent development (Zheng et al., 2020; Cheng et al., 2022; Jiang et al., 2023).
Abnormalities in translational inhibition of maternal mRNA can lead to developmental and maturation defects in oocytes, resulting in female infertility (Zheng et al., 2020; Cheng et al., 2022). The translational regulatory mechanism that controls mRNA poly(A)-tail length during oocyte meiosis resumption, maturation, egg fertilization, and early embryonic development is widely understood (Richter, 2007; Liu et al., 2019; Sha et al., 2019; Poetz et al., 2022). However, the regulation of mRNA translation inhibition/activation by the poly(A) tail during early oocytes development remains unclear (Dai et al., 2022). Illustrating the mechanism of mRNA translation repression during early oocyte development will provide a better understanding of oocyte development.
RNA-binding protein Lsm14 (LSM family member 14, also known as RAP55) is a ubiquitous mRNA-protein complex component in eukaryotes (Albrecht and Lengauer, 2004). There are two Lsm14 homologous genes, Lsm14A and Lsm14B (Mura et al., 2003; Albrecht and Lengauer, 2004). As a key component of the P-body (a typical structures without membrane compartments), LSM14A primarily binds to mRNA silencing factors and inhibits mRNA translation (Tanaka et al., 2006; Mili et al., 2015). However, the function of LSM14B remains unclear. LSM14B interacts with numerous RNA-binding proteins involved in almost every aspect of RNA metabolism, indicating its role in mRNA metabolism by forming versatile ribonucleoprotein (RNP) complexes, which is an important section of translational regulation (Li et al., 2023). Xenopus LSM14B has been reported to be specifically expressed in oocytes (Ladomery and Sommerville, 2015). Most mRNA bound by Xenopus LSM14B is undergoing translation, whereas a small portion is in a translational inhibition state (Ladomery and Sommerville, 2015). It remains unclear whether LSM14B induces mRNA translation activation or assists in maintaining the translational inhibition of mRNAs. Mouse LSM14B is highly expressed in the cytoplasm of oocytes and is localized to the mitochondria-associated ribonucleoprotein domain (MARDO) (Cheng et al., 2022). The knockdown of mouse Lsm14b misregulated the expression of Cyclin B1, and Cdc20, leading to the development of oocytes blocked in the first meiotic metaphase (Zhang et al., 2017). Recent studies have shown that Lsm14b knockout leads to infertility in female mice (Li et al., 2023; Shan et al., 2023; Wan et al., 2023; Zhang et al., 2024). However, these studies were focused on the role of LSM14B in oocyte maturation, meiosis, and early embryonic development (Zhang et al., 2017; Li et al., 2023; Shan et al., 2023; Wan et al., 2023; Zhang et al., 2024). Its role in early oocyte growth and specific translational regulatory mechanisms remain unclear.
In this study, our goals were to explore the function of Lsm14b during early oocyte development and identify specific translational regulatory mechanisms. We used zebrafish as a model and created an lsm14b mutant using CRISPR/Cas9 technology and found that the lack of Lsm14b in zebrafish led to the oocyte developmental arrest at PG stage, resulting in female sterility. The translation-related signaling pathway was active, whereas the deadenylation pathway was downregulated in the lsm14b mutant. Furthermore, the poly(A) tail lengths of zar1 and figla mRNA were significant increased in the lsm14b mutant. Our results suggest that Lsm14b may control the poly(A) tail length of certain mRNA through the adenylation pathway and thus help maintain mRNA in a translationally inhibition state during early oocyte growth.
2 Result
2.1 Lsm14b expresses in early zebrafish oocytes specifically
As reported previously, Lsm14b is specifically expressed in early stages oocytes (Zhao et al., 2010). To confirm this, we used recently released single-cell transcriptome data from juvenile zebrafish ovaries (Liu et al., 2022). Uniform manifold approximation and projection (UMAP) analysis showed that lsm14b was expressed specifically in early oocytes of the juvenile ovary (Figure 1A) and was co-expressed with zar1, org, and gdf9 (Figure 1B).
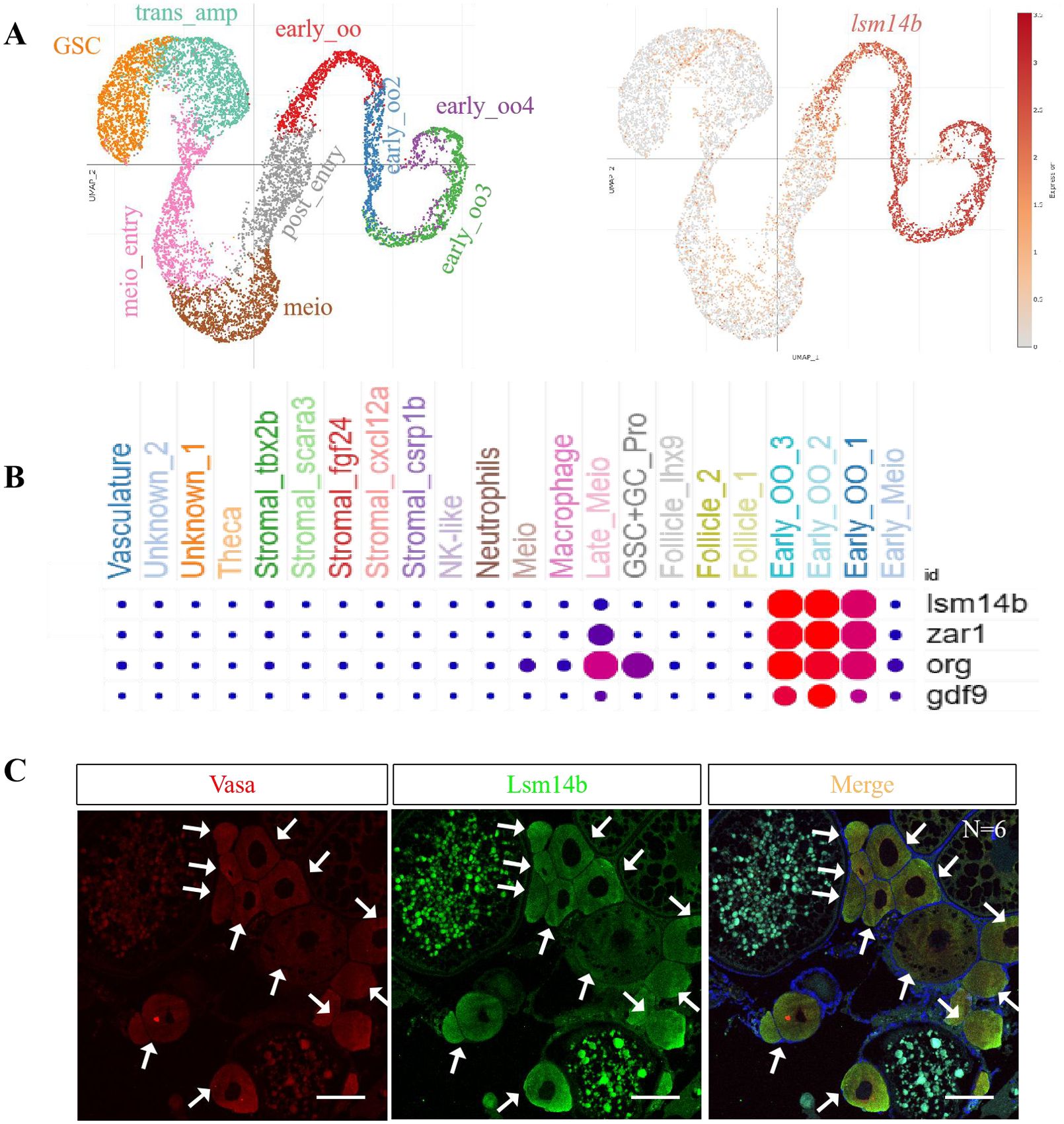
Figure 1. Expression pattern of lsm14b in zebrafish ovary. (A) Cells expressing lsm14b were analyzed by UMAP using a previously published juvenile zebrafish ovarian single-cell transcriptome database. Left (a1), ovary cell types at 40 dpf. Right (a2), distribution of lsm14b-expressed cells. (B) The co-expression of lsm14b with the oocyte marker genes zar1, org, and gdf9 in early oocytes was analyzed using an ovarian single-cell transcriptome database. (C) Coexpression of Lsm14b (green) with the germ cell marker Vasa (red) by double IF staining (N = 6). The arrowhead to indicates the signal. Scale bar, 100 µm. N represents analyzed zebrafish number.
To confirm the expression of Lsm14b in the ovary, we generated a rabbit polyclonal antibody against Lsm14b. As shown by immunofluorescence (IF) against anti-Lsm14b and anti-Vasa antibodies, Lsm14b was enriched in early oocytes, specifically in PG and pre-vitellogenic (PV) stage oocytes (Figure 1C), which is consistent with the UMAP analysis. These results confirm that Lsm14b is specifically expressed in early zebrafish oocytes.
2.2 Lack of Lsm14b leads to female infertility
To investigate the functions of Lsm14b, we generated an lsm14b mutant using the CRISPR/Cas9 system. We obtained two mutated alleles, one with a deletion of 2 base pairs (lsm14b-2/-2) and the other with an insertion of 11 base pairs (lsm14b+11/+11) (Figures 2A–D). The open reading frame of Lsm14b was shifted as a result of both mutations. Since these two homozygous mutant alleles showed no phenotypic differences, we used the lsm14b+11/+11 allele for subsequent analyses (marked as lsm14b-/-). The deficiency of lsm14b in lsm14b-/- was confirmed by RT-qPCR and IF (Figures 2E–G), suggesting that nonsense mediated mRNA decay (NMD) promoted the degradation of lsm14b mRNA.
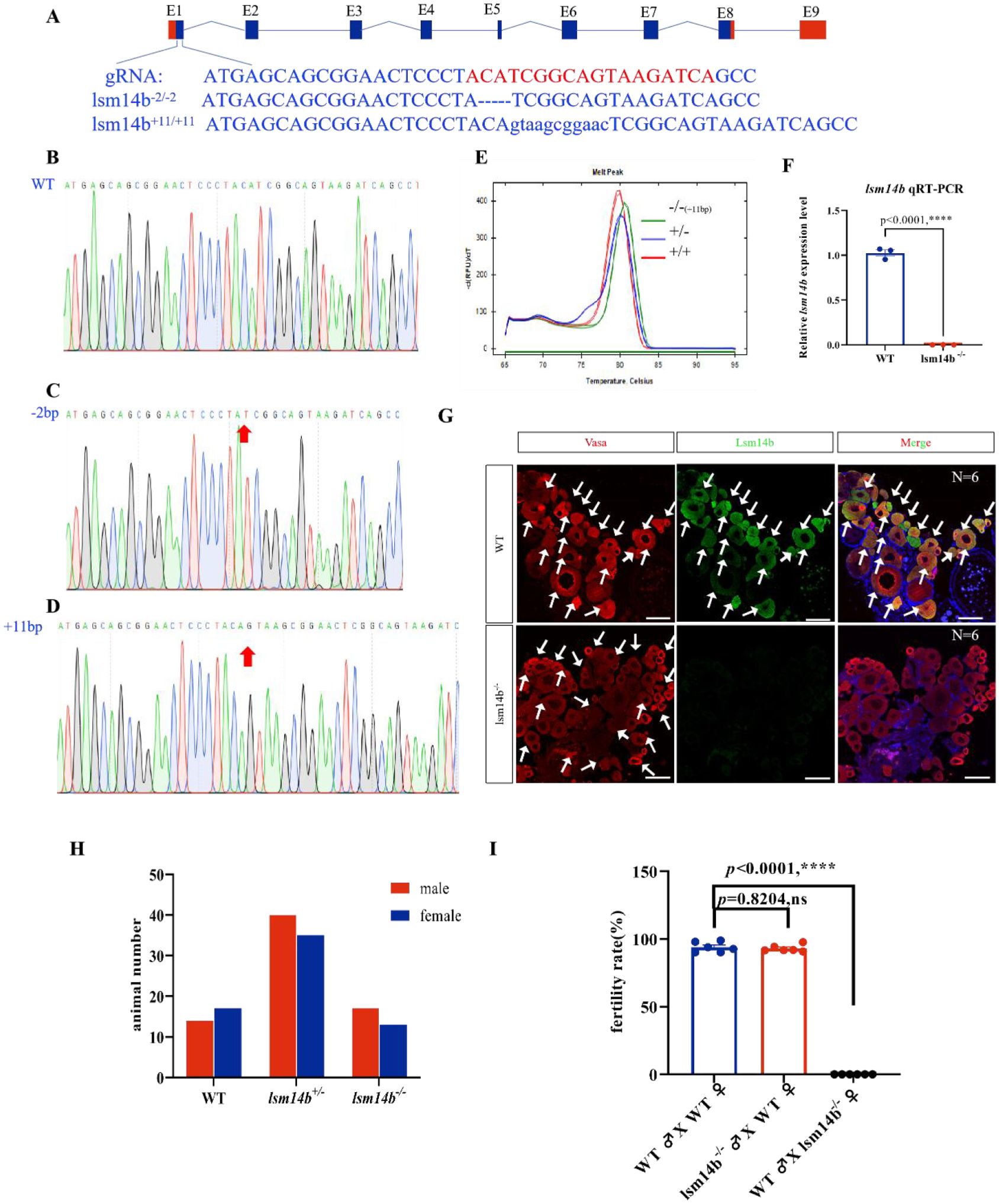
Figure 2. Deletion of Lsm14b leads to female infertility. (A) Schematic diagram of the zebrafish lsm14b gene structure and location of gRNA target sites. The gRNA target sites are highlighted in red. (B-D) The sequencing results of the WT (B), strain with a 2-bp deletion (C), and strain with an 11-bp insertion (D), the indels of DNA are highlighted in red arrow. (E) High-resolution melting curves showing the melting curves of WT (red), heterozygous (purple), and 11-bp insertion homozygous mutations (green). (F) RT-qPCR results showing the expression of lsm14b in the ovaries of lsm14b-/- and WT adults. Data are presented as the mean ± SEM. ****P ≤0.0001; ns, no significance. (G) The expression of Lsm14b in the ovaries of lsm14b-/- and WT zebrafishes was analyzed by double IF with Lsm14b (green) and germ cell marker Vasa (red) (N = 6). The arrowhead indicates the signal. Scale bar, 100 µm. (H) Number of male and female zebrafish in each genotype of lsm14b heterozygous incross offspring. (I) Fertilization rates of lsm14b-/- males and female (N = 6). Data are presented as the mean ± SEM, ****P ≤0.0001.
Zebrafish with defects in oocyte development have often been reported to induce female-to-male sex reversal and develop exclusively into males (Dranow et al., 2016; Lau et al., 2016; Miao et al., 2017; Yin et al., 2017). To confirm the function of Lsm14b in zebrafish oocyte development, we mated heterozygotes and counted the sex ratio of the offspring by observing the secondary sex characteristics of zebrafish, including the genital papilla and the color of the anal fin (Kossack and Draper, 2019; Song et al., 2021). Among the adult offspring, 31 were wild-type (WT), 75 were heterozygous, and 30 were homozygous (Figure 2H), showing an expected genotype ratio of 1:2:1, indicating that no abnormal death occurred. And the sex ratio also showed a normal 1:1 ratio (Figure 2H). These results suggested that the absence of Lsm14b does not affect the sexual differentiation in zebrafish.
We then evaluated the fertility of lsm14b-/- female mutants by mating with WT males, and lsm14b-/- male mutants by mating with WT females. The lsm14b-/- male mutants exhibited normal fertility at 4-months post-fertilization (mpf), similar to the WT. However, lsm14b-/- female mutants were unable to spawn (Figure 2I). These results indicate that deletion of Lsm14b does not affect sexual differentiation or the reproductive capacity of males, but leads to female infertility, suggesting that Lsm14b plays a specific role in zebrafish oocyte development or ovulation.
2.3 Lsm14b controls PG-PV growth transition during zebrafish folliculogenesis
Female infertility results from the arrest of oocyte development or problems with ovulation (Chu et al., 2014; Sun et al., 2018; Shang et al., 2019). To identify the cause of infertility induced by Lsm14b deficiency, we analyzed the oocyte development in lsm14b-/- female mutants in detail. We found that lsm14b-/- females appeared comparable to WT females, but their ovary morphology differed significantly (Figure 3A). The gonadosomatic index (GSI) data showed that the ovary of lsm14b-/- females were significantly lower than those of the WT females (Figure 3B). The ovary of WT female contained with all stages of follicles, including the PG, PV, early vitellogenic (EV), mid-vitellogenic (MV), late vitellogenic (LV), and full-grown (FG) pre-ovulatory stages. However, oocytes in the lsm14b-/- were arrested at the PG stage, only a few PV and no EV, MV or FG stage oocytes were found (Figures 3C–E). These results show that Lsm14b plays an important role in the oocyte PG - PV transition in zebrafish.
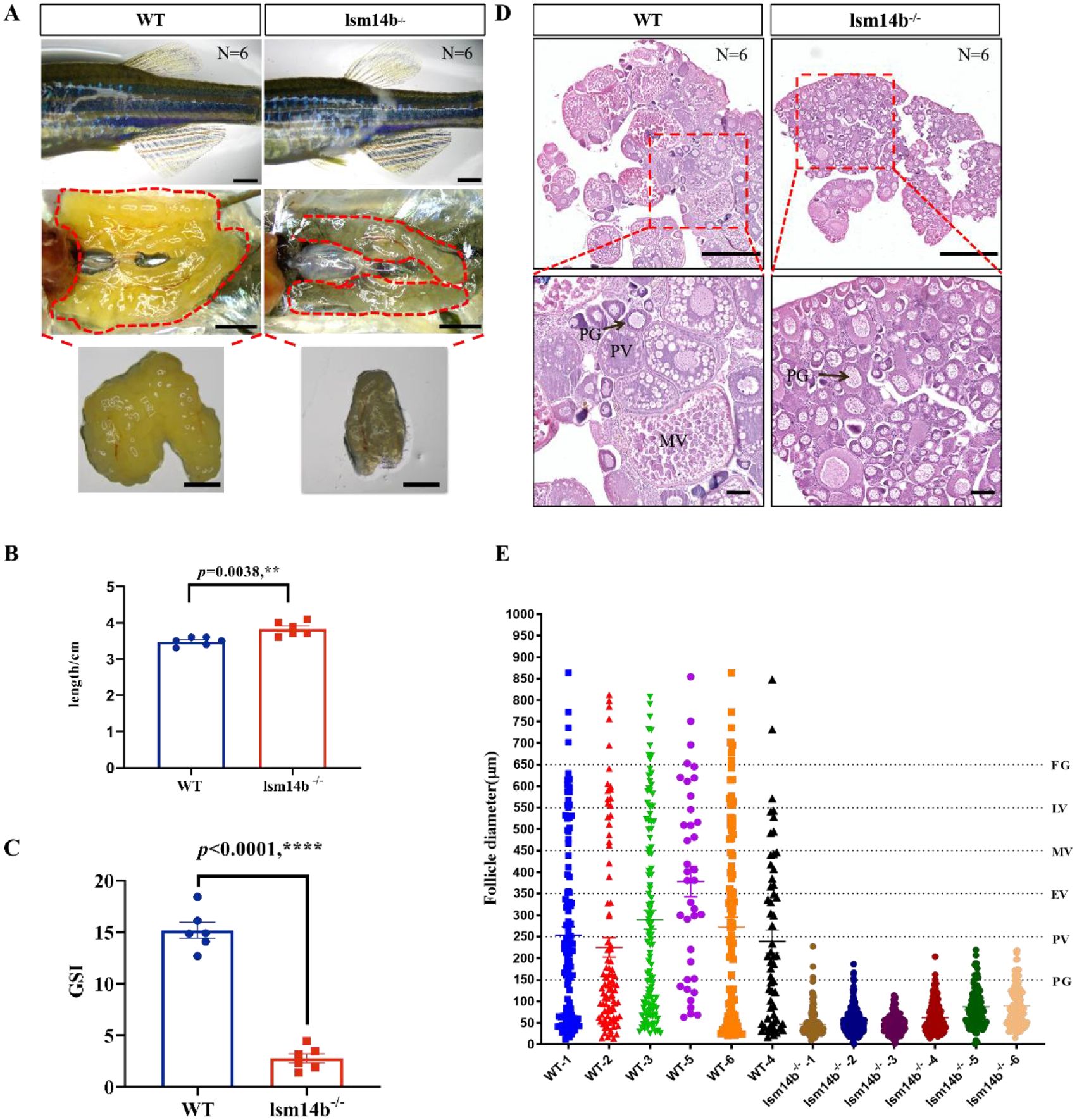
Figure 3. The oocyte development of lsm14b-/- female zebrafish is blocked in PG-PV growth transition. (A) Secondary sex characteristics and gonadal morphology of lsm14b-/- and WT female zebrafish. (N = 6). Scale bar, 2 mm. (B) Body length of lsm14b-/- zebrafish (N = 6). Data are presented as the mean ± SEM. ****P ≤0.0001. (C) GSI of lsm14b-/- zebrafish (N = 6). Data are presented as the mean ± SEM. ****P ≤0.0001. (D) HE staining of lsm14b-/- and WT ovaries (N = 6). The regions of red box are magnified in the below. Scale bar, 500 µm. (E) Quantitative assessment of follicle diameter of lsm14b-/- and WT zebrafish (N = 6). ** p≤0.01.
2.4 Lsm14b regulates the expression of genes related to oocyte development
To investigate the mechanism by which Lsm14b regulates early oocyte development, we isolated the 4 mpf ovaries from WT females and lsm14b-/- and performed RNA-seq analysis. RNA-seq analysis showed that 5574 genes were differentially expressed in lsm14b-/-ovaries compared to WT ovaries. Among them, 3158 genes were significantly downregulated, and 2416 genes were upregulated (padj ≤ 0.05) (Figure 4A). According to GO annotation, the differentially expressed genes were enriched in the GO terms of cellular process, metabolic process, biological regulation, regulation of biological process, and response to stimulus (Figure 4B). KEGG pathway analysis showed that FoxO signaling pathway, apoptosis, P53 signaling pathway, and progesterone-mediated oocyte maturation pathway were significantly disrupted in the lsm14b-/- oocytes (Figure 4C).
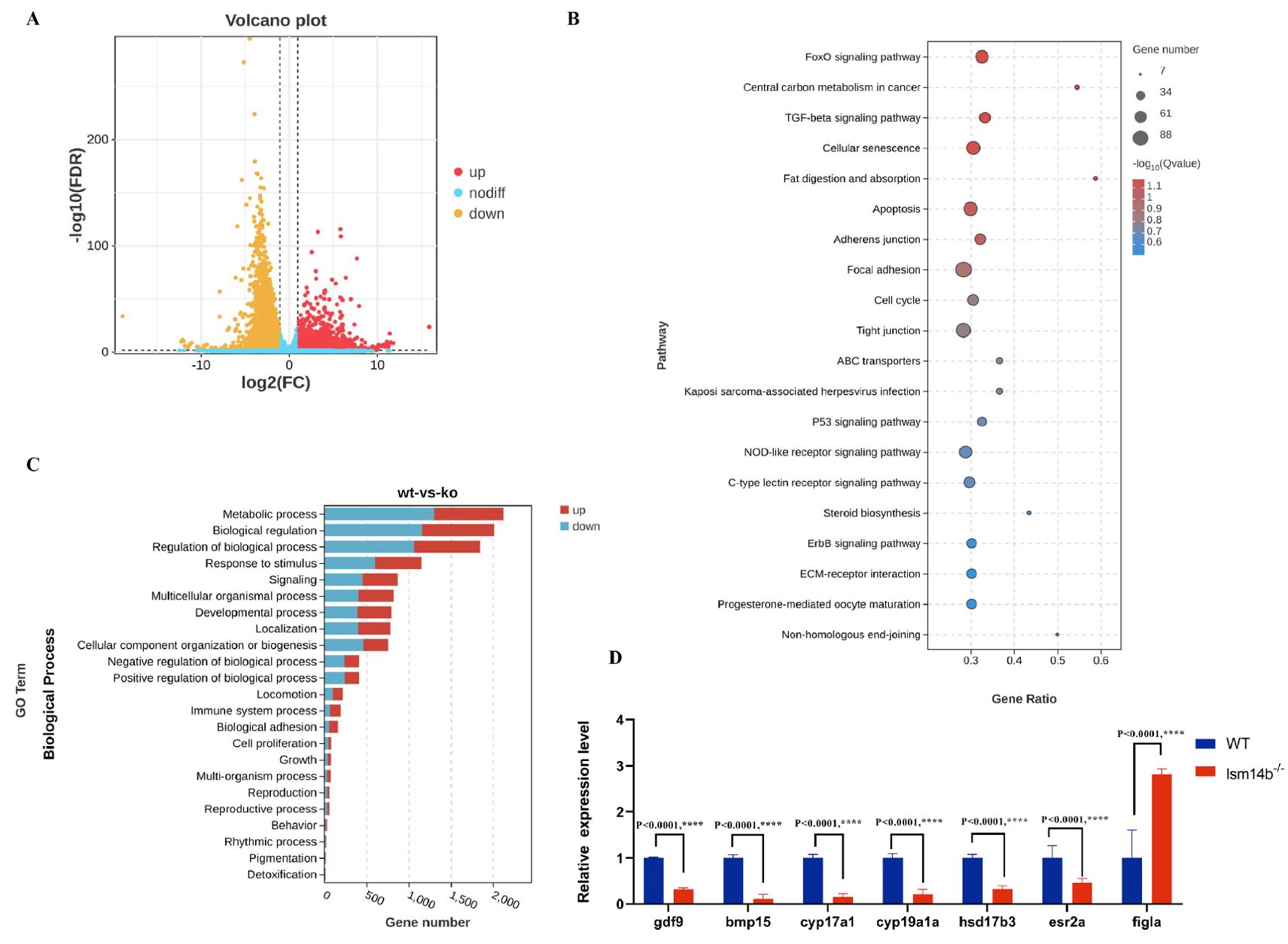
Figure 4. Expression of genes associated with oocyte development and reproductive processes are downregulated in lsm14b-/- zebrafish. (A) Volcano plot showing the differentially expressed genes in lsm14b-/- and WT zebrafish. (B) Top 20 significantly enriched KEGG pathways in lsm14b-/- zebrafish oocyte. (C) Top enriched biological processes in lsm14b-/- zebrafish oocyte. (D) RT-qPCR analysis of oocyte development-related genes in lsm14b-/-oocytes. Data are presented as the mean ± SEM. ****P ≤0.0001.
Furthermore, the expression of genes associated with oocyte development were significantly downregulated in lsm14b-/-. Among these, the decreased expression of genes, including growth differentiation factor 9(gdf9), bone morphogenetic protein 15 (bmp15), cytochrome P450, family 17, subfamily A, polypeptide 1 (cyp17a1), cytochrome P450, family 19, subfamily A, polypeptide 1a (cyp19a1a), and hydroxysteroid (17-beta) dehydrogenase 3 (hsd17b3), were confirmed further by RT–qPCR (Figure 4D). The exception was folliculogenesis specific bHLH (figla), which was expressed both in the PG and PV stages of oocyte and was significantly upregulated (Figure 4D). This was consistent with our previous result showing only the PG stage oocytes exist in the ovary of lsm14b-/-. These results demonstrate that Lsm14b is essential for oocyte development, particularly for maintaining the correct expression of genes related to oocyte development.
2.5 Lsm14b maintains translational inhibition state of mRNA by regulating the poly(A) tail length
Previous studies suggested that Lsm14b is involved in mRNA translation (Ladomery and Sommerville, 2015; Li et al., 2023). However, it is still inconclusive whether it induces translational activation or helps maintains the translational inhibition state (Winata and Korzh, 2018; Li et al., 2023). We conducted the overall gene set enrichment analysis (GSEA) of the RNA-seq data and found that genes associated with negative regulation of translation were downregulated significantly (Figure 5A). The same result was further confirmed by RT-qPCR (Figure 5D). This suggests that Lsm14b acts as a translational repressor in zebrafish oocytes.
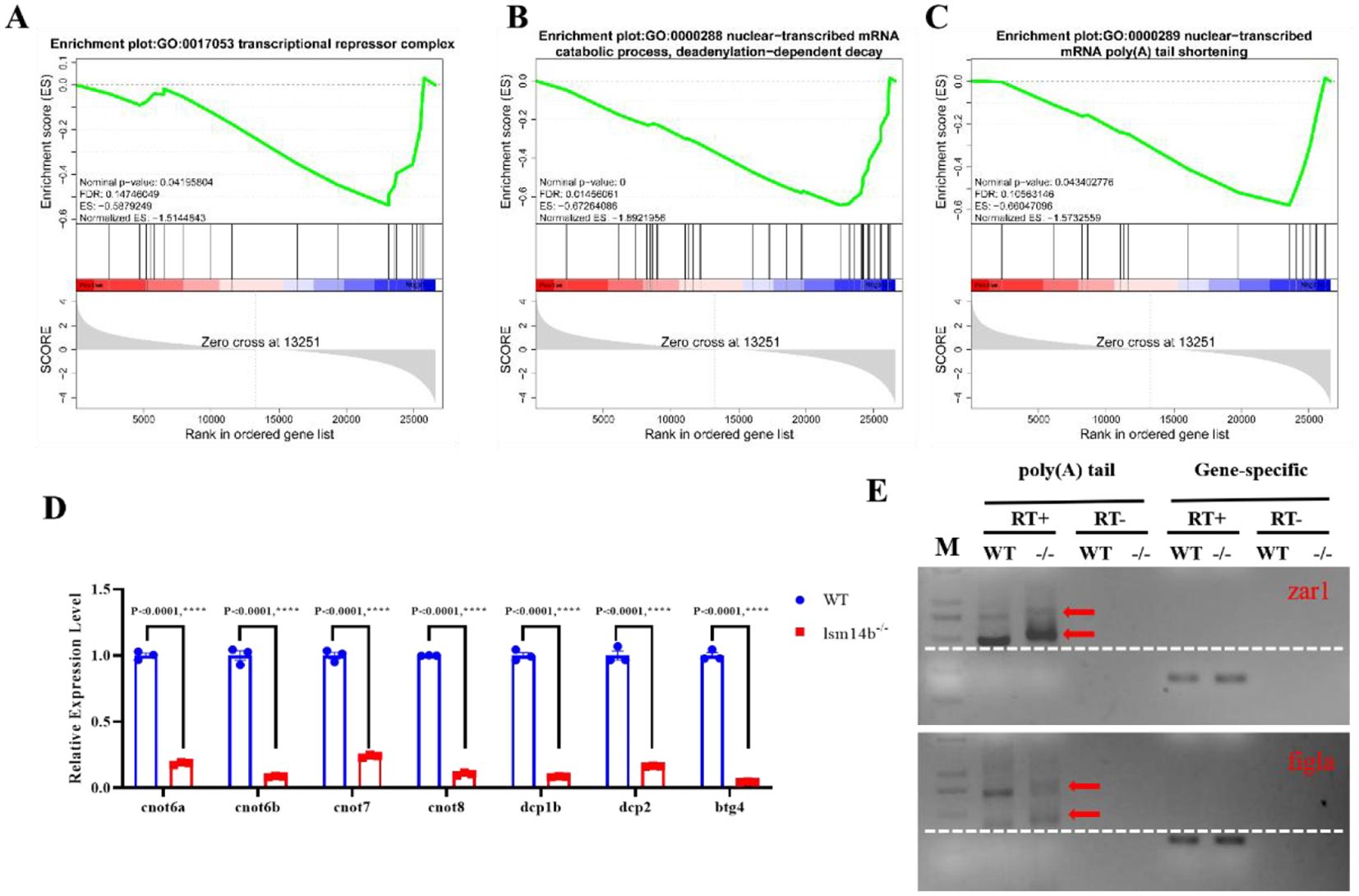
Figure 5. The translation is aberrant in lsm14b-/- zebrafish oocytes. (A-C) GSEA results revealing that the downregulated genes are enriched in negative regulation of translation (A), deadenylation-dependent decay (B) and mRNA poly(A) tail shortening (C). (D) RT-qPCR analysis of the expression of mRNA deadenylation-related genes in lsm14b-/- oocytes. Data are presented as the mean ± SEM. ****P ≤0.0001. (E) Poly(A) tail length analysis of zar1 and figla mRNA in lsm14b-/- oocytes from the PAT experiment. The increase in poly(A) tail length corresponds to a higher molecular mass of the sequence, which manifests as larger molecular mass of PCR products. Poly(A) PCR products are indicated by red arrow. Horizontal lines are represented by white dashed lines. M, Marker; RT+, reverse transcription; RT−, no reverse transcription.
The length of the mRNA poly(A) tail is a crucial mechanism regulating mRNA translation under normal conditions. This regulation is a part of the natural process that maintains mRNA stability and translation efficiency, which ensures cellular homeostasis (Richter, 2007; Liu et al., 2019; Sha et al., 2019; Poetz et al., 2022). The poly(A) polymerase is responsible for the poly(A) lengthening, whereas the CCR4-NOT complex mediates poly(A) shortening (Passmore and Coller, 2022). GSEA revealed that the expression of genes associated with deadenylation-dependent decay and mRNA poly(A) tail shortening were significantly downregulated (Figures 5B, C). The RT-qPCR results revealed that the subunits of CCR4-NOT complex, including cnot6a/b, cnot7, cnot8, dcp1b, dcp2, and btg4, were significantly downregulated (Figure 5D). The PAT assay accurately recapitulates changes in poly(A) tail lengths, allowing precise measurement and analysis of mRNA stability and translation efficiency (Sha et al., 2018). The PAT assay showed that the poly(A) length of zar1 and figla mRNA were significantly increased in lsm14b-/- ovary (Figure 5E). According to previous studies, both Zar1 and Figla are associated with oocyte development. These results suggest that Lsm14b is involved in maintaining the translational repression state of mRNAs related to oocyte development by regulating the length of the mRNA poly(A) tail.
2.6 Lsm14b deletion triggers ER stress and unfolded protein response
Over-activation of mRNA translation consistently induces ER stress and the UPR in cells (Hetz et al., 2020; Bhatter et al., 2024). Consistently, the RNA-seq data revealed that ER stress and UPR-related genes, including atf3, plvapb, ddit4, and ddit3 were upregulated significantly in lsm14b-/- (Figures 6A, B). Furthermore, the transcription of xbp1 has underwent obvious splicing in the fourth exon in lsm14b-/-(Figure 6C). Xbp1 is a transcription factor that induces the expression of ER molecular chaperones and the ER-associated protein degradation (ERAD) to decrease ER stress during mRNA splicing (Shen et al., 2001; Yoshida et al., 2001; Luo et al., 2022). To verify these data, we examined xbp1 mRNA transcripts in lsm14b-/- oocytes using RT-PCR (Figures 6D, E). The results showed that the splicing ratio of xbp1 was increased in lsm14b-/- ovary, further confirming the occurrence of ER stress.
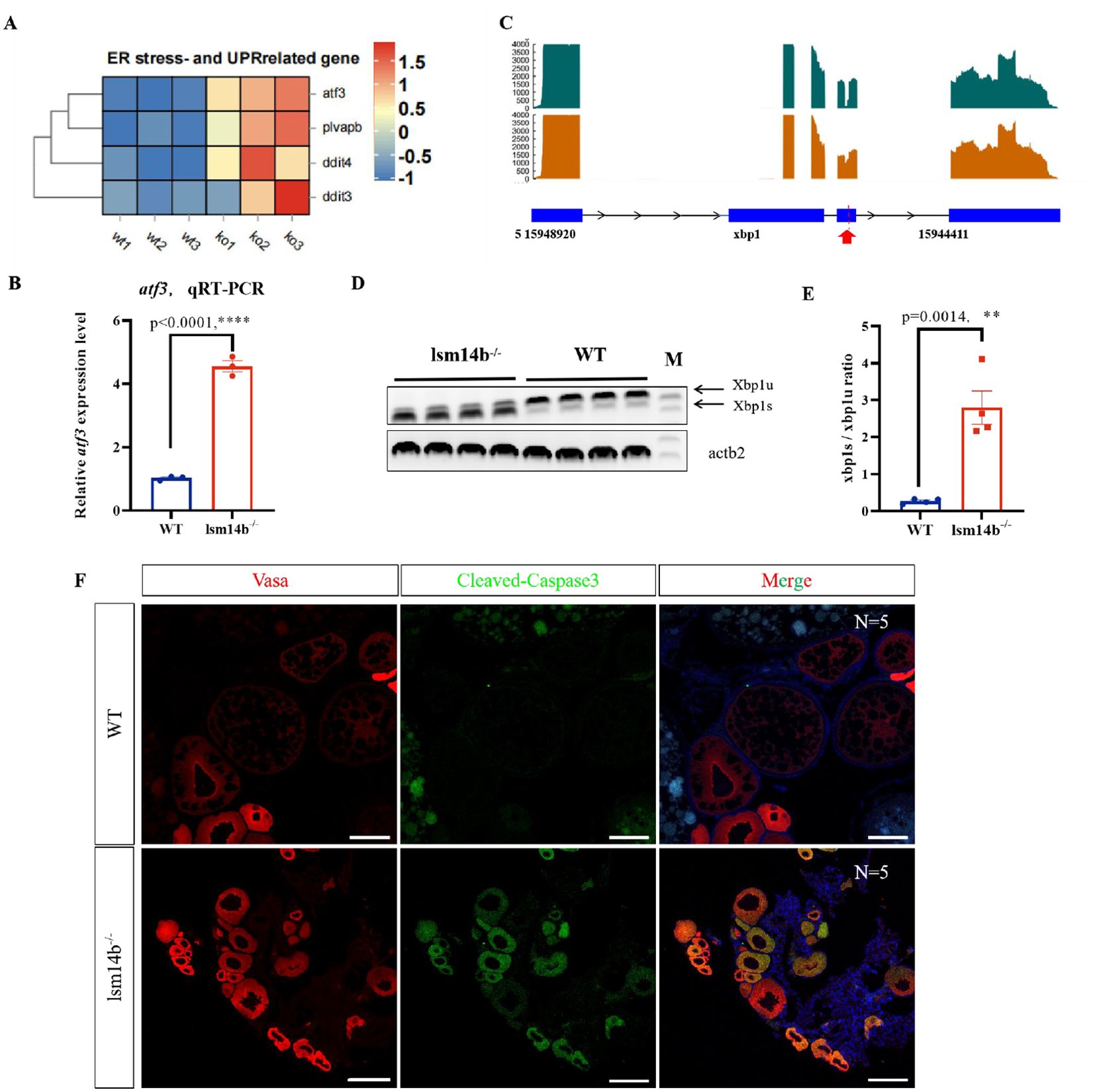
Figure 6. Lsm14b deletion triggers ER stress and UPR. (A) Heatmap showing the expression of genes related to ER stress and UPR. (B) The expression of atf3 in lsm14b-/- zebrafish oocytes determined by RT-qPCR. ****P ≤0.0001. (C) Selective splicing of Xbp1 in lsm14b-/- zebrafish oocytes revealed by transcriptomic analysis. The position of selective splicing is highlight in red arrow. (D) Xbp1 splicing confirmed by RT-PCR (N = 4), xbp1u, unspliced xbp1; xbp1s, spliced xbp1; β-actin (actb2) is the reference. (E) The xbp1s/xbp1u ratio in E represents the relative intensity ratio of corresponding PCR product bands in D. (F) Apoptosis in lsm14b-/- zebrafish oocytes analyzed by double immunofluorescence staining with cleaved-Caspase 3 (green) and germ cell marker Vasa (red) (N = 5). Scale bar, 100 µm. **p≤0.01.
Incorrect or untimely translation of mRNAs that should have been maintained in a translationally inhibited state can cause oocytes apoptosis (He et al., 2022). We also determined the level of apoptosis using a cleaved-Caspase 3 antibody in lsm14b-/- ovary and found apoptotic signals in the cytoplasm of early stage mutant oocytes but not in WT oocytes (Figure 6F). These findings indicate that lsm14b-/- oocytes trigger ER stress and excessive UPR, leading to oocytes apoptosis. These results further confirmed our conclusion that Lsm14b promotes early oocyte development in zebrafish by maintaining the timely translation of proteins related to oocyte development through regulating the length of the corresponding mRNA poly(A) tail.
3 Discussion
The function of Lsm14b in oocyte development has long remained unknown. Several recent studies have shown that LSM14B is essential for the mouse oocyte development (Li et al., 2023; Shan et al., 2023; Wan et al., 2023; Zhang et al., 2024). However, further investigation is required. In the present study, we evaluated the potential role and mechanism of Lsm14b in early oocyte development and found that Lsm14b can ensure a PG-PV transition by maintaining a state of translational repression oocyte development-related proteins by regulating the length of the mRNA poly(A) tail. Our results provide critical information regarding the functional roles of Lsm14b in controlling oocyte development, especially oocyte development during the PG-PV stage transition.
Folliculogenesis in zebrafish includes primary growth (PG, stage I), secondary growth stage (SG, stage II-III), maturation (stage IV), and ovulation (stage V) (Selman et al., 1993; Li and Ge, 2020). The SG stage can be divided into stage II, which can further be divided into pre-vitellogenic (PV), early vitellogenic (EV), mid-vitellogenic (MV) and late vitellogenic (LV), and stage III, which is the full-grown (FG) pre-ovulatory stage (Selman et al., 1993; Li and Ge, 2020). The PG-PV stage transition in zebrafish is similar to two comparable phases in mammals: primordial and early antral follicles (De Figueiredo et al., 2018). However, the PG-PV stage transition and its regulatory mechanisms in zebrafish remain poorly understood. Benefiting from advancements in reverse genetic technologies, some endocrine hormones and paracrine factors, such as fshb and fshr, have been shown to play key roles in the PG-PV stage transition in recent years (Chu et al., 2015; Zhang et al., 2015b). Deficiency of fshb in zebrafish causes PG-PV transition delay (Chu et al., 2015; Zhang et al., 2015b). The loss of fshr completely blocked oocyte development during the PG stage (Chu et al., 2015; Zhang et al., 2015a). However, the role of the RNA-binding proteins (RBP) in this process is still insufficient. Miao et al. reported that Zar1 is specifically expressed in early oocytes and is critical for the development of early stage follicles in zebrafish by binding to zona pellucida (ZP) mRNAs and repressing their translation (Miao et al., 2017). As also an RBP, the function of Lsm14b in oocyte development has long remained unknown. Since June 2023, several groups have reported that Lsm14b is critical for oocyte maturation, especially from MI to MII, mainly by regulating maternal mRNA metabolism and the assembly of P-body and MARDO t (Li et al., 2023; Shan et al., 2023; Wan et al., 2023; Zhang et al., 2024). However, the role of Lsm14b in earlier oocyte development has not yet been reported. Here, we analyzed previously reported single-cell transcriptome data of juvenile zebrafish ovaries and found that lsm14b was co-expressed with zar1, org, and gdf9 in juvenile zebrafish ovary, indicating a specific role of Lsm14b in early oocyte development. Our investigations indicated that the loss of Lsm14b in zebrafish caused oocytes development arrest at the PG stage (corresponding to the primordial stage of the mouse ovary), leading to female sterility. This is the first time that Lsm14b has been shown to promote the PG-PV stage transition during oocyte development.
Previous studies have shown that blocking zebrafish oocyte development during PG-PV generally leads to female-to-male sexual reversal and causes all offspring develop into males, such as in the mutants of bmp15, egfra, figla, and fshr (Chu et al., 2015; Zhang et al., 2015a; Dranow et al., 2016; Qin et al., 2018; Song et al., 2021). Notably, with Lsm14b mutation, oocytes also experienced PG-PV developmental arrest, however, the mutants still developed into females. To date, we have observed female lsm14b mutants at 12-mpf. We need to observe these female mutants over an extended period to determine whether they will undergo sexual reversal. Whether these mutants are fertile after the female-to-male sex reversal also requires further observation.
Oocytes are transcriptionally active during early development and synthesize enormous amounts of mRNA, most of which is stored as translationally inhibited mRNP to provide an energy source for subsequent development (Richter and Lasko, 2011; Sha et al., 2018; Winata and Korzh, 2018; Dai et al., 2019; Sha et al., 2019). The translational regulation of mRNA plays an important role in this process. However, the mechanism underlying mRNA storage during oocyte genesis remains unclear. It is well known that mRNA translation is associated with mRNA adenylation (Richter, 2007; Liu et al., 2019; Sha et al., 2019; Poetz et al., 2022). In the early oocyte stage, most mRNAs undergo de-adenylation and from mRNPs with proteins. The mRNPs then aggregate into particles, such as Balbiani bodies and P-bodies, which specifically exist in early oocytes (A. Schisa, 2012; Zaucker et al., 2020; Cassani and Seydoux, 2024). Coincidentally, Lsm14b is expressed in the Balbiani body of zebrafish, indicating that it may be involved in the transcriptional regulation of mRNA in early oocytes (Jamieson-Lucy et al., 2022). As expected, in lsm14b mutants, mRNA translation was activated, suggesting that Lsm14b acts as a translational repressor during the early growth stage of oocyte development. Furthermore, our results showed that the deadenylation-related genes were significantly downregulated in the lsm14b mutant, and the poly(A) tail length of zar1 and figla mRNA significantly increased, suggesting that Lsm14b regulates mRNA storage by inhibiting the translation of certain “star factors” involved in oocyte development. Similarly, Xenopus LSM14B specifically interacts with the DDX6 helicase Xp54. Xp54 is detected in polysomes and blocks translation-initiation complexes by acting as a translation suppressor (Ladomery and Sommerville, 2015). However, Li et al. showed that the translation efficiency of LSM14b-bound mRNA in Lsm14b-defect oocytes was significantly reduced, indicating that the function of LSM14B in oocytes may not be translational inhibition, but rather selective promotion the translation of certain mRNA (Li et al., 2023). Different results have been obtained in various studies, and further exploration is required to understand the relationship between Lsm14b and mRNA translation.
ER stress and UPR are usually associated with abnormal translation. The ER stress response can be caused by excessive or misfolded protein accumulation, which triggers the UPR to restore homeostasis. Under ER stress, the endonuclease IRE1a induces Xbp1 mRNA splicing to produce mature mRNA that encoding the active transcription factor spliced XBP1 (XBP1s). The XBP1s-encoded proteins can be transported to the nucleus, where they induce the expression of ER molecular chaperones and ERAD to decrease ER stress (Shen et al., 2001; Yoshida et al., 2001; Luo et al., 2022). In our study, we found that the mRNA expression of ER stress and UPR-related genes (atf3, plvapb, ddit4, and ddit3) increased in the ovary of the lsm14b mutant. In addition, Xpb1 splicing occurred to form active Xpb1s. These results demonstrated that the loss of Lsm14b triggers ER stress and the UPR, leading to the failure of oocyte development. Although lsm14b-deleted zebrafish oocytes attempted to restore ER homeostasis, our results showed that oocyte apoptosis occurred. These results demonstrated that abnormal translational events occur in Lsm14b-deficient oocytes.
In conclusion, we created a lsm14b-deficient zebrafish line using CRISPR/Cas9 and found that the loss of Lsm14b caused oocytes developmental arrest at the PG stage, resulting in female sterility. In the absence of Lsm14b, the poly(A) tail length of mRNAs related to oocyte development was significantly increased, inducing ER stress and UPR, eventually, leading to oocyte apoptosis. Collectively, our results provide critical information regarding the functional role of Lsm14b in translational repression during early oocyte development in zebrafish. Our research is expected to reveal the regulatory role and mechanism of Lsm14b in the growth and development of oocyte and provide a theoretical basis and experimental evidence for assisted reproductive technology for female infertility caused by abnormal growth and development of oocytes.
4 Materials and methods
4.1 Zebrafish husbandry
Zebrafish husbandry and manipulation were approved by the Animal Care and Use Committee of The First Affiliated Hospital of Shenzhen University (Shenzhen Second People’s Hospital) (NO: IACUC-2023-8793-01). AB strain zebrafish was used as the wild-type (WT) fish to generate genetically modified lines. The zebrafish used in this study were maintained in a recirculating water system at 28°C with a 14 h light/10 h dark cycle.
4.2 Establishment of lsm14b mutant lines
The lsm14b mutants were established using CRISPR/Cas9, as described in our previous study (Jia et al., 2020). Briefly, the target sites of lsm14b were identified using ZiFiT tools (zifit.partners.org/ZiFiT/ChoiceMenu.aspx). The PCR primers used to amplify the gRNA template (sgDNA) are listed in Supplementary Table S1. The purified gRNA template was used to transcribe sgRNA using the MEGAshortscript™ T7 transcription kit (Invitrogen, AM1354) and purified with the mirVana miRNA Isolation Kit (Invitrogen, AM1560). A 2 nL mixture of TrueCut™ Cas9 Protein v2 (Invitrogen™, A36497) and sgRNA was co-injected into one-cell-stage zebrafish embryos. DNA sequencing was performed to confirm whether the F0 founders were mutated. Genomic DNA was extracted and amplified from 30 randomly injected embryos at 24 hpf. A DNA fragment containing the peripheral sequences of the target site was amplified using the two primers (listed in Supplementary Table S1).
4.3 High-resolution melt analysis
HRMA assay was used to identify mutants in F1 and F2 offspring. Briefly, genomic DNA was extracted using 100 μL NaOH (50 mM) at 95°C for 20 min. Afterward, 10 μL of Tris (1M, pH 8.0) was added to neutralize the solution. The primers used for HRMA are listed in Supplementary Table S1. HRMA was performed using EvaGreen Dye (Biotium, 31000) on the CFX96 Real-time PCR Detection System (Bio-Rad, USA). HRMA analysis was conducted using the Precision Melt Analysis software.
4.4 Fertility assay
Fertility tests were conducted by mating 4-mounth-old lsm14b-/- females and males with 4-mounth-old fertile WT males and females respectively. Individuals which were unable to spawn or produce fertilized embryos after at least 10 attempts were considered infertile.
4.5 Histological examination
The zebrafish at 4 months were anesthetized with MS-222. Body length and body weight were also measured. The fishes/ovaries were photographed before and after dissection for histological examination. The ovaries were fixed in Bouin’s solution at room temperature for 24 h. Then, fixed ovaries were dehydrated with a gradient of ethanol, hyalinized with xylene, and embedded in paraffin. The sections (7μm) were stained with standard hematoxylin and eosin.
4.6 Lsm14b antibody generation and immunofluorescence
The Lsm14b antibody was raised in rabbits commissioned by the AtaGenix Laboratories Co., Ltd. (Wuhan, PR China). The epitope of Lsm14b is NNENKPPTSRRKPGT. The ovaries were dissection for IF according to previously described (Song et al., 2015). Briefly, ovaries were fixed with 4% paraformaldehyde at room temperature for 2 h, and dehydrated in 30% sucrose at 4°C. Then, the gonads were embedded in OCT (optimal cutting temperature compound, Tissue-Tek) and frozen at -80°C. The frozen sections (10 μm) were cut by a cryosta (Lecia CM1950), and the sections were mounted on poly-L-lysine-coated coverslips. The sections were then air-dried and blocked with 10% bovine serum albumin for 1 h. After blocking, the sections were incubated with primary antibodies overnight at 4°C. After washing with PBS, the sections were incubated with secondary antibodies. Cell nuclei were stained with 4’,6-diamidino-2-phenylindole (DAPI) and imaged on a ZEISS confocal microscope. The primary antibodies are used in this study were Vasa (DIA-AN, 2008, 1:500), Lsm14b (our laboratory, 1:500) and cleaved-Caspase3 (CST, 9661S,1:1000). The secondary antibodies were Alexa Fluor 488-labeled donkey anti-rabbit antibody (Invitrogen, A21206, 1:1000) and Alexa Fluor 555-labeled donkey anti-mouse antibody (Invitrogen, A31570, 1:1000).
4.7 Real-time quantitative polymerase chain reaction
The relative mRNA levels of the genes in the control and lsm14b-/-oocytes were detected by using RT-qPCR. Quantitative primers used are listed in the Supplementary Table S1. Total RNA was extracted using TRIzol (Invitrogen, #15596-026), and reversed transcribed into cDNA using a reverse transcription kit (Toyobo, #TRT-101). RT-qPCR was performed on the CFX96 Real-Time PCR Systems using the SYBR Green Master Mix (Toyobo, #QPK-201). The expression levels of target genes were normalized to the housekeeping gene β-actin and then analyzed by 2−△△CT method.
4.8 Transcriptome analysis
To compare ovarian transcriptomes between WT and lsm14b-/-, the ovaries at 4-months were selected for RNA-seq analysis. Total RNA was extracted using the TRIzol reagent (Invitrogen, #15596-026). RNA-seq and data analyses were performed by Gene Denovo Biotechnology Co., Ltd (Guangzhou, China). RNA-seq libraries were prepared using the NEB Next Ultra RNA Library Prep Kit for Illumina (NEB, #7530) and sequenced on an Illumina Novaseq6000 platform. The raw data were deposited in SRA database (accession number: PRJNA1095659). The reads were mapped to the zebrafish genome by HISAT 2. Differential gene expression (DEGs) analysis was performed using DEseq2. Genes with p-values <= 0.05 and |log2FC|>1 were considered as DEGs. Gene Ontology (GO) terms of DEGs was conducted by Goatools. Kyoto Encyclopedia of Genes and Ge-nomes (KEGG) pathways enrichment analysis was performed using the KOBAS tools. Gene set enrichment analysis (GSEA) was performed using the software GSEA software (version 4.1.0). To determine significantly enriched functional annotations, we set the significance threshold at P value < 0.05 and |Normalized Enrichment Score (NES)| ≥ 1.
4.9 Poly(A) tail length assay
Total RNAs were extracted from the WT and lsm14b mutant at 4 months. Reverse transcription and poly(G/I) tailing were carried out by using a poly(A)-Tail Length Assay kit, following the manufacturer’s instructions (ThermoFisher, 764551KT). Briefly, RNA extracted from ovaries was underwent G/I tailing. Complementary DNA was synthesized via reverse transcription using the poly(G/I)-tailed RNA as a template. Targeted PCR products were generated using specific primers. The primers used for the poly(A) tail length assay are listed in Supplementary Table S1. The PCR products were separated on an agarose gel.
4.10 Statistical analysis
Data were analyzed using the GraphPad Prism9 and presented as the mean ± standard error of the mean (SEM). Two-tailed unpaired Student’s t-test or one-way analysis of variance (ANOVA) was used to determine significant differences between groups, with significance levels of *P ≤0.05, **P ≤ 0.01, ***P ≤0.001, and ****P ≤0.0001. “NS” indicates no significance difference.
Data availability statement
The datasets presented in this study can be found in online repositories. The names of the repository/repositories and accession number(s) can be found in the article/Supplementary Material.
Ethics statement
The animal study was approved by the Animal Care and Use Committee of The First Affiliated Hospital of Shenzhen University (Shenzhen Second People’s Hospital)(NO: IACUC-2023-8793-01). The study was conducted in accordance with the local legislation and institutional requirements.
Author contributions
XW: Data curation, Formal analysis, Investigation, Methodology, Funding acquisition, Writing – original draft, Writing – review & editing. XP: Investigation, Validation, Visualization, Writing – review & editing. TD: Investigation, Resources, Writing – review & editing. WP: Software, Methodology, Writing – review & editing. BH: Resources, Supervision, Visualization, Funding acquisition, Writing – review & editing. GN: Conceptualization, Funding acquisition, Project administration, Supervision, Writing – original draft, Writing – review & editing.
Funding
The author(s) declare financial support was received for the research, authorship, and/or publication of this article. This research was funded by National Key Research and Development Project (2023YFC2508002), Shenzhen Science and Technology Innovation Committee (JCYJ20230807115103007, JCYJ20190806163209126, JCYJ20220530150414031, and LCYSSQ20220823091403007), Medical-Engineering Interdisciplinary Research Foundation of Shenzhen University (Project no.2023YG003), National Natural Science Foundation of China (82192865 and 81970875), Natural Science Foundation of Guangdong Province (2019A1515011495), Guangdong Health Commission (A2022078), Sanming Project of Medicine in Shenzhen (SZSM202211022), and Shenzhen Portion of Shenzhen-Hong Kong Science and Technology Innovation Cooperation Zone (HTHZQSWS-KCCYB-2023060).
Conflict of interest
The authors declare that the research was conducted in the absence of any commercial or financial relationships that could be construed as a potential conflict of interest.
Publisher’s note
All claims expressed in this article are solely those of the authors and do not necessarily represent those of their affiliated organizations, or those of the publisher, the editors and the reviewers. Any product that may be evaluated in this article, or claim that may be made by its manufacturer, is not guaranteed or endorsed by the publisher.
Supplementary material
The Supplementary Material for this article can be found online at: https://www.frontiersin.org/articles/10.3389/fmars.2024.1440959/full#supplementary-material
References
Alam M. H., Miyano T. (2020). Interaction between growing oocytes and granulosa cells in vitro. Reprod. Med. Biol. 19, 13–23. doi: 10.1002/rmb2.12292
Albrecht M., Lengauer T. (2004). Novel Sm-like proteins with long C-terminal tails and associated methyltransferases. FEBS Lett. 569, 18–26. doi: 10.1016/j.febslet.2004.03.126
A. Schisa J. (2012). “Chapter seven - New Insights into the Regulation of RNP Granule Assembly in Oocytes,” in International Review of Cell and Molecular Biology. Ed. Jeon K. W. (Amsterdam: Academic Press), 233–289.
Bhatter N., Dmitriev S. E., Ivanov P. (2024). Cell death or survival: Insights into the role of mRNA translational control. Semin. Cell Dev. Biol. 154, 138–154. doi: 10.1016/j.semcdb.2023.06.006
Cassani M., Seydoux G. (2024). P-body-like condensates in the germline. Semin. Cell Dev. Biol. 157, 24–32. doi: 10.1016/j.semcdb.2023.06.010
Cheng S., Altmeppen G., So C., Welp L. M., Penir S., Ruhwedel T., et al. (2022). Mammalian oocytes store mRNAs in a mitochondria-associated membraneless compartment. Science 378, eabq4835. doi: 10.1126/science.abq4835
Chu L., Li J., Liu Y., Cheng C. H. K. (2015). Gonadotropin signaling in zebrafish ovary and testis development: insights from gene knockout study. Mol. Endocrinol. 29, 1743–1758. doi: 10.1210/me.2015-1126
Chu L., Li J., Liu Y., Hu W., Cheng C. H. K. (2014). Targeted gene disruption in zebrafish reveals noncanonical functions of LH signaling in reproduction. Mol. Endocrinol. 28, 1785–1795. doi: 10.1210/me.2014-1061
Conti M., Franciosi F. (2018). Acquisition of oocyte competence to develop as an embryo: integrated nuclear and cytoplasmic events. Hum. Reprod. Update 24, 245–266. doi: 10.1093/humupd/dmx040
Dai X. X., Jiang J. C., Sha Q. Q., Jiang Y., Ou X. H., Fan H. Y. (2019). A combinatorial code for mRNA 3’-UTR-mediated translational control in the mouse oocyte. Nucleic Acids Res. 47, 328–340. doi: 10.1093/nar/gky971
Dai X. X., Pi S. B., Zhao L. W., Wu Y. W., Shen J. L., Zhang S. Y., et al. (2022). PABPN1 functions as a hub in the assembly of nuclear poly(A) domains that are essential for mouse oocyte development. Sci. Adv. 8, eabn9016. doi: 10.1126/sciadv.abn9016
Das D., Arur S. (2017). Conserved insulin signaling in the regulation of oocyte growth, development, and maturation. Mol. Reprod. Dev. 84, 444–459. doi: 10.1002/mrd.v84.6
De Castro F. C., Cruz M. H., Leal C. L. (2016). Role of growth differentiation factor 9 and bone morphogenetic protein 15 in ovarian function and their importance in mammalian female fertility - A review. Asian Australas J. Anim. Sci. 29, 1065–1074. doi: 10.5713/ajas.15.0797
De Figueiredo J. R., De Lima L. F., Silva J. R. V., Santos R. R. (2018). Control of growth and development of preantral follicle: insights from in vitro culture. Anim. Reprod. 15, 648–659. doi: 10.21451/1984-3143-AR2018-0019
Dranow D. B., Hu K., Bird A. M., Lawry S. T., Adams M. T., Sanchez A., et al. (2016). Bmp15 is an oocyte-produced signal required for maintenance of the adult female sexual phenotype in zebrafish. PloS Genet. 12, e1006323. doi: 10.1371/journal.pgen.1006323
He M., Jiao S., Zhang R., Ye D., Wang H., Sun Y. (2022). Translational control by maternal Nanog promotes oogenesis and early embryonic development. Development 149 (24), dev201213. doi: 10.1242/dev.201213
Hetz C., Zhang K., Kaufman R. J. (2020). Mechanisms, regulation and functions of the unfolded protein response. Nat. Rev. Mol. Cell Biol. 21, 421–438. doi: 10.1038/s41580-020-0250-z
Jamieson-Lucy A. H., Kobayashi M., James Aykit Y., Elkouby Y. M., Escobar-Aguirre M., Vejnar C. E., et al. (2022). A proteomics approach identifies novel resident zebrafish Balbiani body proteins Cirbpa and Cirbpb. Dev. Biol. 484, 1–11. doi: 10.1016/j.ydbio.2022.01.006
Jia S., Wu X., Wu Y., Cui X., Tao B., Zhu Z., et al. (2020). Multiple developmental defects in sox11a mutant zebrafish with features of coffin-siris syndrome. Int. J. Biol. Sci. 16, 3039–3049. doi: 10.7150/ijbs.47510
Jiang Y., Adhikari D., Li C., Zhou X. (2023). Spatiotemporal regulation of maternal mRNAs during vertebrate oocyte meiotic maturation. Biol. Rev. 98, 900–930. doi: 10.1111/brv.12937
Keefe D., Kumar M., Kalmbach K. (2015). Oocyte competency is the key to embryo potential. Fertil. Steril. 103, 317–322. doi: 10.1016/j.fertnstert.2014.12.115
Kossack M. E., Draper B. W. (2019). Genetic regulation of sex determination and maintenance in zebrafish (Danio rerio). Curr. Top. Dev. Biol. 134, 119–149. doi: 10.1016/bs.ctdb.2019.02.004
Ladomery M., Sommerville J. (2015). The Scd6/Lsm14 protein xRAPB has properties different from RAP55 in selecting mRNA for early translation or intracellular distribution in Xenopus oocytes. Biochim. Biophys. Acta 1849, 1363–1373. doi: 10.1016/j.bbagrm.2015.10.002
Lau E. S.-W., Zhang Z., Qin M., Ge W. (2016). Knockout of zebrafish ovarian aromatase gene (cyp19a1a) by TALEN and CRISPR/Cas9 leads to all-male offspring due to failed ovarian differentiation. Sci. Rep. 6, 37357. doi: 10.1038/srep37357
Li J., Ge W. (2020). Zebrafish as a model for studying ovarian development: Recent advances from targeted gene knockout studies. Mol. Cell. Endocrinol. 507, 110778. doi: 10.1016/j.mce.2020.110778
Li H., Zhao H., Yang C., Su R., Long M., Liu J., et al. (2023). LSM14B is an oocyte-specific RNA-binding protein indispensable for maternal mRNA metabolism and oocyte development in mice. Adv. Sci. (Weinh) 10, e2300043. doi: 10.1002/advs.202300043
Liu Y., Kossack M. E., Mcfaul M. E., Christensen L. N., Siebert S., Wyatt S. R., et al. (2022). Single-cell transcriptome reveals insights into the development and function of the zebrafish ovary. Elife 11, e76014. doi: 10.7554/eLife.76014
Liu Y., Nie H., Liu H., Lu F. (2019). Poly(A) inclusive RNA isoform sequencing (PAIso-seq) reveals wide-spread non-adenosine residues within RNA poly(A) tails. Nat. Commun. 10, 5292. doi: 10.1038/s41467-019-13228-9
Luo X., Alfason L., Wei M., Wu S., Kasim V. (2022). Spliced or unspliced, that is the question: the biological roles of XBP1 isoforms in pathophysiology. Int. J. Mol. Sci. 23, 2746. doi: 10.3390/ijms23052746
Ma J. Y., Li M., Luo Y. B., Song S., Tian D., Yang J., et al. (2013). Maternal factors required for oocyte developmental competence in mice: transcriptome analysis of non-surrounded nucleolus (NSN) and surrounded nucleolus (SN) oocytes. Cell Cycle 12, 1928–1938. doi: 10.4161/cc.24991
Miao L., Yuan Y., Cheng F., Fang J., Zhou F., Ma W., et al. (2017). Translation repression by maternal RNA binding protein Zar1 is essential for early oogenesis in zebrafish. Development 144, 128–138. doi: 10.1242/dev.144642
Mili D., Georgesse D., Kenani A. (2015). Localization and role of RAP55/LSM14 in HeLa cells: a new finding on the mitotic spindle assembly. Acta Biochim. Pol. 62, 613–619. doi: 10.18388/abp.2015_1107
Mura C., Phillips M., Kozhukhovsky A., Eisenberg D. (2003). Structure and assembly of an augmented Sm-like archaeal protein 14-mer. Proc. Natl. Acad. Sci. 100, 4539–4544. doi: 10.1073/pnas.0538042100
Passmore L. A., Coller J. (2022). Roles of mRNA poly(A) tails in regulation of eukaryotic gene expression. Nat. Rev. Mol. Cell Biol. 23, 93–106. doi: 10.1038/s41580-021-00417-y
Poetz F., Lebedeva S., Schott J., Lindner D., Ohler U., Stoecklin G. (2022). Control of immediate early gene expression by CPEB4-repressor complex-mediated mRNA degradation. Genome Biol. 23, 193. doi: 10.1186/s13059-022-02760-5
Qin M., Zhang Z., Song W., Wong Q. W.-L., Chen W., Shirgaonkar N., et al. (2018). Roles of figla/figla in juvenile ovary development and follicle formation during zebrafish gonadogenesis. Endocrinology 159, 3699–3722. doi: 10.1210/en.2018-00648
Richter J. D. (2007). CPEB: a life in translation. Trends Biochem. Sci. 32, 279–285. doi: 10.1016/j.tibs.2007.04.004
Richter J. D., Lasko P. (2011). Translational control in oocyte development. Cold Spring Harb. Perspect. Biol. 3, a002758. doi: 10.1101/cshperspect.a002758
Robker R. L., Hennebold J. D., Russell D. L. (2018). Coordination of ovulation and oocyte maturation: A good egg at the right time. Endocrinology 159, 3209–3218. doi: 10.1210/en.2018-00485
Selman K., Wallace R. A., Sarka A., Qi X. (1993). Stages of oocyte development in the zebrafish, Brachydanio rerio. J. Morphol. 218, 203–224. doi: 10.1002/jmor.1052180209
Sha Q. Q., Yu J. L., Guo J. X., Dai X. X., Jiang J. C., Zhang Y. L., et al. (2018). CNOT6L couples the selective degradation of maternal transcripts to meiotic cell cycle progression in mouse oocyte. EMBO J. 37 (24), e99333. doi: 10.15252/embj.201899333
Sha Q.-Q., Zhang J., Fan H.-Y. (2019). A story of birth and death: mRNA translation and clearance at the onset of maternal-to-zygotic transition in mammals†. Biol. Reprod. 101, 579–590. doi: 10.1093/biolre/ioz012
Shan L. Y., Tian Y., Liu W. X., Fan H. T., Li F. G., Liu W. J., et al. (2023). LSM14B controls oocyte mRNA storage and stability to ensure female fertility. Cell Mol. Life Sci. 80, 247. doi: 10.1007/s00018-023-04898-2
Shang G., Peng X., Ji C., Zhai G., Ruan Y., Lou Q., et al. (2019). Steroidogenic acute regulatory protein and luteinizing hormone are required for normal ovarian steroidogenesis and oocyte maturation in zebrafish†. Biol. Reprod. 101, 760–770. doi: 10.1093/biolre/ioz132
Shen X., Ellis R. E., Lee K., Liu C. Y., Yang K., Solomon A., et al. (2001). Complementary signaling pathways regulate the unfolded protein response and are required for C. elegans development. Cell 107, 893–903. doi: 10.1016/S0092-8674(01)00612-2
Song Y., Chen W., Zhu B., Ge W. (2021). Disruption of epidermal growth factor receptor but not EGF blocks follicle activation in zebrafish ovary. Front. Cell Dev. Biol. 9, 750888. doi: 10.3389/fcell.2021.750888
Song Y., Duan X., Chen J., Huang W., Zhu Z., Hu W. (2015). The distribution of kisspeptin (Kiss)1- and Kiss2-positive neurones and their connections with gonadotrophin-releasing hormone-3 neurones in the zebrafish brain. J. Neuroendocrinol. 27, 198–211. doi: 10.1111/jne.12251
Sun J., Yan L., Shen W., Meng A. (2018). Maternal Ybx1 safeguards zebrafish oocyte maturation and maternal-to-zygotic transition by repressing global translation. Development 145 (19), dev166587. doi: 10.1242/dev.166587
Tanaka K. J., Ogawa K., Takagi M., Imamoto N., Matsumoto K., Tsujimoto M. (2006). RAP55, a cytoplasmic mRNP component, represses translation in Xenopus oocytes. J. Biol. Chem. 281, 40096–40106. doi: 10.1074/jbc.M609059200
Wan Y., Yang S., Li T., Cai Y., Wu X., Zhang M., et al. (2023). LSM14B is essential for oocyte meiotic maturation by regulating maternal mRNA storage and clearance. Nucleic Acids Res. 51, 11652–11667. doi: 10.1093/nar/gkad919
Winata C. L., Korzh V. (2018). The translational regulation of maternal mRNAs in time and space. FEBS Lett. 592, 3007–3023. doi: 10.1002/1873-3468.13183
Yin Y., Tang H., Liu Y., Chen Y., Li G., Liu X., et al. (2017). Targeted disruption of aromatase reveals dual functions of cyp19a1a during sex differentiation in zebrafish. Endocrinology 158, 3030–3041. doi: 10.1210/en.2016-1865
Yoshida H., Matsui T., Yamamoto A., Okada T., Mori K. (2001). XBP1 mRNA is induced by ATF6 and spliced by IRE1 in response to ER stress to produce a highly active transcription factor. Cell 107, 881–891. doi: 10.1016/S0092-8674(01)00611-0
Zaucker A., Kumari P., Sampath K. (2020). Zebrafish embryogenesis – A framework to study regulatory RNA elements in development and disease. Dev. Biol. 457, 172–180. doi: 10.1016/j.ydbio.2019.01.008
Zhang Z., Lau S.-W., Zhang L., Ge W. (2015a). Disruption of zebrafish follicle-stimulating hormone receptor (fshr) but not luteinizing hormone receptor (lhcgr) gene by TALEN leads to failed follicle activation in females followed by sexual reversal to males. Endocrinology 156, 3747–3762. doi: 10.1210/en.2015-1039
Zhang T., Li Y., Li H., Ma X. S., Ouyang Y. C., Hou Y., et al. (2017). RNA-associated protein LSM family member 14 controls oocyte meiotic maturation through regulating mRNA pools. J. Reprod. Dev. 63, 383–388. doi: 10.1262/jrd.2017-018
Zhang H., Zhang T., Wan X., Chen C., Wang S., Qin D., et al. (2024). LSM14B coordinates protein component expression in the P-body and controls oocyte maturation. J. Genet. Genomics 51, 48–60. doi: 10.1016/j.jgg.2023.07.006
Zhang Z., Zhu B., Ge W. (2015b). Genetic analysis of zebrafish gonadotropin (FSH and LH) functions by TALEN-mediated gene disruption. Mol. Endocrinol. 29, 76–98. doi: 10.1210/me.2014-1256
Zhao C. L., Yang Q. W., Hu J. R., Ye D., Gong W. M., Lu H. Y., et al. (2010). Identification of zRAP55,a gene preponderantly expressed in Stages I and II oocytes of zebrafish. Dongwuxue Yanjiu 31, 469–475. doi: 10.3724/SP.J.1141.2010.05469
Keywords: Lsm14b, oocyte development, translational repressor, polyadenylation of mRNA poly(A) tail, zebrafish
Citation: Wu X, Peng X, Deng T, Peng W, Hu B and Nie G (2024) Lsm14b controls zebrafish oocyte growth by regulating polyadenylation of the mRNA poly(A) tail. Front. Mar. Sci. 11:1440959. doi: 10.3389/fmars.2024.1440959
Received: 30 May 2024; Accepted: 19 August 2024;
Published: 04 September 2024.
Edited by:
Ming Li, Ningbo University, ChinaReviewed by:
Suvra Roy, Central Inland Fisheries Research Institute (ICAR), IndiaDr. D. K. Meena, Central Inland Fisheries Research Institute (ICAR), India
Copyright © 2024 Wu, Peng, Deng, Peng, Hu and Nie. This is an open-access article distributed under the terms of the Creative Commons Attribution License (CC BY). The use, distribution or reproduction in other forums is permitted, provided the original author(s) and the copyright owner(s) are credited and that the original publication in this journal is cited, in accordance with accepted academic practice. No use, distribution or reproduction is permitted which does not comply with these terms.
*Correspondence: Guohui Nie, bmllZ3VvaHVpQGVtYWlsLnN6dS5lZHUuY24=