- 1Master Program of Marine Conservation, Padjadjaran University, Jatinangor, West Java, Indonesia
- 2Department of Fisheries, Faculty of Fisheries and Marine Science, Padjadjaran University, Jatinangor, West Java, Indonesia
- 3Biogeochemical Laboratory, Faculty of Fisheries and Marine Science, Padjadjaran University, Jatinangor, West Java, Indonesia
- 4Department of Marine Science, Faculty of Fisheries and Marine Science, Padjadjaran University, West Java, Jatinangor, Indonesia
- 5Hydro-Oceanographic Centre of The Indonesian Navy, Jakarta, Indonesia
- 6Research Center for Radioisotope, Radiopharmaceutical, and Biodosimetry Technology National Research and Innovation Agency (BRIN), Tangerang, Indonesia
- 7Komitmen Research Group, Padjadjaran University, Jatinangor, West Java, Indonesia
Despite growing global concerns, there has been limited research on the characterization and distribution of microplastics in the Indonesian Throughflow (ITF) pathways, such as the Flores Sea. The Flores Sea is a component of the Indian–Pacific Current, a significant ocean current system that links the Pacific and Indian oceans and has the capacity to carry pollution over large marine ecosystems, making this research extremely important. Understanding the microplastic pollution in this area informs local environmental management. It provides insights into how these currents may distribute microplastics (MPs) across regional and global scales, impacting marine life and human health far beyond the immediate area. To respond to these concerns, this research aims to determine the characterization and distribution of MPs at six research sites in the Flores Sea that are precisely located within the ITF. This work exhibits an extensive dataset focusing on the occurrence, attributes, and dispersion of microplastics in the Flores Sea. The water sampling was carried out during a Jala Citra 3 by the Indonesian Navy from April to May 2023. Sea surface water samples were collected using a Neuston net, while sediment samples were taken from three stations at the shallowest depth using the Ekman Grab sampler. Additionally, abundance, size, shape, and color analyses were conducted using a light microscope, and microplastic types were identified through Raman spectroscopy. The results indicated that the Flores Sea waters and sediment are polluted with microplastics, with relative abundances ranging from 0.75 ± 0.49 to 2.13 ± 0.25 items/l samples. The most dominant shapes identified were filament (77.45%) and fragment (13.40%), with sizes varying between surface water 4.70 to 3799.25 μm and seabed from 67.20 mm to 2176.87 mm, while black (30.07%) and blue (24.51%) were reported as the common MPs colors. The identified polymers include PET and PE. This study confirms visual evidence of microplastics in the open waters of eastern Indonesia. While it may not fully capture the wide range of temporal variations, it establishes initial microplastic presence and dispersion levels. Given that the ITF influences both the Pacific and Indian Oceans, this research contributes to the global understanding of microplastic distribution across ocean basins, underscoring the need for coordinated international efforts to address marine pollution.
1 Introduction
Marine debris or marine litter is a term used to refer to materials or substances discarded by humans that are introduced into marine environments, whether intentionally or unintentionally (Browne et al., 2011; Iñiguez et al., 2016; Thushari and Senevirathna, 2020). This problem contributes to a decline in ocean health and has far-reaching impacts on human life (Chiba et al., 2018; Smith et al., 2018). Among the various types of debris typically observed in the marine environment, plastics and foams stand out as significant contributors (Cózar et al., 2014; Chiba et al., 2018; Faizal et al., 2022a). Macroplastics deteriorate into smaller fragments referred to microplastics (MPs), through a stressor (oxidation, photodegradation, and hydrolysis) in the ocean (Kameda et al., 2021). Microplastic is categorized into small particles with a size of < 5.00 mm that have been found in various marine environments and transported through external conditions (Lebreton et al., 2012; Kameda et al., 2021; Faizal et al., 2022a). Microplastics can move vertically to deep layers and accumulate in the seabed. This movement is influenced by various factors, including the size and buoyancy of microplastics, ocean currents, and interactions with marine organisms. As microplastics settle onto the seabed, they may integrate into sediment layers. The presence of microplastics on the sea surface and sediment can impact the biogeochemical processes of the ocean and impact the sustainability of marine biodiversity (Kershaw et al., 2019; Purba et al., 2020; Sevwandi Dharmadasa et al., 2021; Zhang et al., 2023). However, a critical knowledge gap remains regarding the specific characterization and distribution of microplastics in under-studied regions like the Indonesian Throughflow, particularly in the Flores Sea. This study addresses that gap by providing baseline data that is essential for understanding how microplastic pollution is distributed in these globally significant waters. This global issue is particularly relevant to Indonesia, where the pervasive problem of plastic pollution is a growing concern. As part of the Indonesian Throughflow, the Flores Sea has a unique oceanographic setting that makes it important to investigate the presence of microplastics in great detail. This will help with both regional efforts to protect the environment and world efforts to control pollution.
Indonesia has become one of the world’s leading contributors to plastic debris pollution (Jambeck et al., 2015) and this raised the attention of the government which developed a national action plan on plastic debris (Vriend et al., 2021). Despite these efforts, research on microplastics in the water column remains notably limited in Indonesia. A study by Cordova and Wahyudi (2016) observed several types of microplastics in the seabed near Sumatera Island. Fragments were the most common type, followed by fibers and films that were identified in the east Java Island seabed (Yona et al., 2019). Furthermore, the waters in the eastern Indonesia region are the main sources for the production of fisheries in Indonesia (Khan et al., 2023). The presence of microplastics might impact the sustainability of fisheries, as the potential health risks of microplastics might pose potential health risks for consumers (Rist et al., 2018). Microplastics were detected on the sea surface as fragments, fiber, and films in the Savu Sea, East Tenggara (Hiwari et al., 2019). A similar study has found fibers (45.45%), granules (36.36%), and other plastic forms (18.18%) in the water column in Sumba waters (Cordova and Hernawan, 2018). While microplastics have been detected in the surface waters in the eastern Indonesian region, less is known about their presence in deeper layers and on the seabed, especially in strategic locations such as the Flores Sea, which is directly influenced by the Indonesian Throughflow (ITF). Thus, further investigation is needed into their potential upstream distribution and characterization due to the strategic location of the eastern Indonesian waters within the complex circulation pattern of the ITF. The ITF, which transports water mass and heat from the Pacific Ocean to the Indonesian Ocean, results in an increase in sea surface temperature and turbulent mixing, affecting carbon, oxygen, and nutrient levels (Taufiqurrahman et al., 2020). The elevated temperature and changes in ocean chemistry might potentially influence the physical breakdown of macroplastics into microplastics and lead to the accumulation of microplastics in the ocean (Wang et al., 2020).
To assess the microplastic characterization and distribution in the unique circulation pattern of the ITF, the Flores Sea was investigated. The Flores Sea is located in the transition region of ocean currents from the Makassar Strait, Java Seas, and the Indian Ocean (Putriani et al., 2019). Given its distinct location, this zone is crucial to comprehending the distribution of contaminants, such as microplastics, throughout extensive oceanic systems. Research in this field offers important insights into the widespread effects of pollution in an area that is crucial for both the environment and the local economy. Furthermore, this region is a fishing ground, particularly for small pelagic, large pelagic, demersal, and reef fish, as well as various species of crustaceans, mollusks, other aquatic organisms, and aquatic plants such as seaweed. In terms of management, the Flores Sea falls within the Fisheries Management Area 713 (WPPNRI 713). The Directorate General of Capture Fisheries (2016) reported that in 2014, WPPNRI 713 contributed to the second-largest fish production in Indonesia, accounting for 12.43% of the national total production (6,037,654 tons) (Koeshendrajana et al., 2016). Despite its significant potential, the Flores Sea faces global challenges related to marine pollution, particularly from microplastics that accumulate in the waters and pose threats to marine life and human health. These microplastics originate from various sources, including poorly managed plastic waste and degraded consumer products. Regarding the impact of microplastic transport, it has been observed that ocean circulation and wind conditions have a strong impact on the spread of microplastics (Rahmania et al., 2021). It has been observed that debris in Indonesian seas originates not only from the terrestrial environment but also from the surrounding seas (Attamimi et al., 2015; Purba et al., 2021). Previous research reported that the spread of marine debris in the eastern seas of Indonesia is dominantly caused by ocean circulations, local tides, waves, and wind patterns (Hiwari et al., 2019; Faizal et al., 2022b). Microplastic distribution and characterization in the Flores Sea, located in the ITF, has never been investigated.
In this study, we systematically investigated the types and abundances of microplastics present in surface waters and seabed. The Indonesian Navy hosted the Jala Citra 3 expedition (2023), with one of its primary objectives being to identify microplastics in both seawater and the seabed. This study represents a microplastic research investigation in both surface water and seabed sediments in the Flores Sea. This comprehensive study will provide information for future environmental assessments and policy development related to mitigating marine debris in Indonesian seas, especially in the Flores Sea and its surroundings.
2 Materials and methods
2.1 Geographic location
Geographically, the region of the Flores Sea is located between Flores Island and South Sulawesi Province. The depth of the Flores Sea ranges between 300 and 5,500 m, with the maximum depth at the southern site (Site 5) (Figure 1). On its western boundary, it connects to the Java Sea and the eastern side of the Banda Sea.
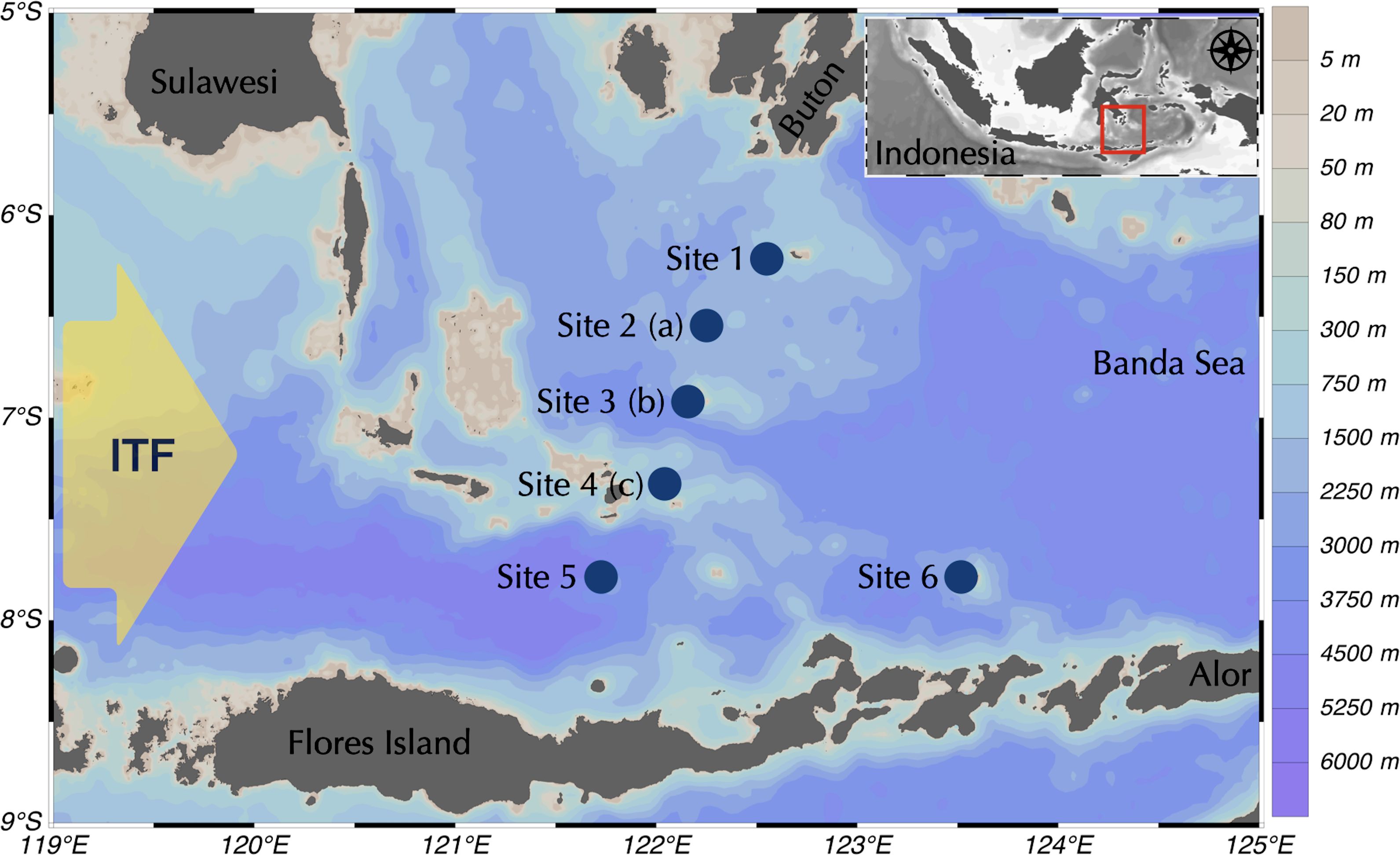
Figure 1. Geographical regions of Flores Sea overlayed with bathymetry and station sampling. Microplastic sampling sites on the surface (1-6) and seabed (a-c) are shown. Seabed samples were taken from three sites, including site 2 with a depth of 1,145 m, site 3 with a depth of 850 m, and site 4 with a depth of 500 m.
The western part of the Flores Sea is the area of intersection of the main ocean currents originating from the Makassar Strait. Surface wind primarily influences water circulation in the upper layer, whereas, in the thermocline layer, sea level differences play a significant role. The primary axis of the ITF consistently shifted along the southern region of the Flores Sea, predominantly characterized by its zonal component (Putriani et al., 2019). Furthermore, this region is affected by monsoon situations that occur periodically, with eddies and upwelling in the Flores Sea and surroundings (Nuzula et al., 2016).
2.2 Sampling method
Sampling was carried out in the Indonesian Flores Sea from April to May 2023 as a part of the Jala Citra 3 expedition. In 2023, microplastic sampling was carried out at Stations 1 and 2 on Wednesday, 26 April; Station 3, Thursday, 27 April; Station 4, Friday, 28 April; Station 5, Saturday, 29 April; and Station 6, Wednesday, 3 May. There are six datasets from the surface water and only three from the seabed due to the ocean conditions during the expedition. Seawater sampling was carried out by lowering a bucket from the vessel and collecting approximately 20-30 L of water. The collected water was subsequently filtered on the ship using Neuston nets with a mesh size of 0.3 mm and 30 cm diameter in order to capture the minute particles and organisms in the seawater. The plankton net was rinsed using distilled water, then the collected distilled water was stored in a sample bottle for later observation in the laboratory. This filtration process guaranteed that the samples were free of pollutants and ready for further testing for water quality and biological composition. The Neuston nets were rinsed externally with decontaminated water to prevent any contamination and stacked along the net during the sampling. Samples were collected using a Neuston net with a 0.3 mm mesh size and then deposited into washed glass bottles (Cutroneo et al., 2020; Kieu-Le et al., 2023). Following the sampling process, all the observers used latex gloves during sampling activities to avoid contamination. Each sample was placed into covered glass bottles. Once cleaned, the net was available for subsequent sampling sessions (Cutroneo et al., 2020). For sediment from the seabed, the samples were collected using a grab sampler tool. The sampler utilized has a diameter of 107 cm and a radius of 53.5 cm, making it a half-circle. Approximately 100-500 g of sediment was collected at each site and kept in a zipped plastic bag. All the samples were stored in a ship cooler at -17°C before being transported to the laboratory for analysis. This low temperature helped preserve the original condition of the samples, especially when dealing with organic matter and microplastics. Freezing the samples minimized the risk of contamination, ensuring that any volatile compounds were retained, and stabilized the samples for accurate laboratory testing later on. All components were thoroughly washed with distilled water, and procedures were performed in the sterilized laboratory to prevent contamination.
2.3 Sample preparation
The extraction of the surface water samples followed the method of the National Oceanographic and Atmospheric Administration (Masura et al., 2015). Sea surface water samples were dissolved in a 10% potassium hydroxide (KOH) solution, which was prepared by dissolving KOH flakes in aquadest. The samples were then treated with a 10% potassium hydroxide (KOH) solution at a ratio of 1:3. The mixture was stirred for 10 min at room temperature and left to sit for 24 h or until the organic matter dissolved. This was done once for each sample to destroy the organic particles and leave the microplastic particles. The extraction of sediment samples was adopted from several publications (Zobkov et al., 2020), and sediment samples were dried at 60°C for 8 h. Samples were then subjected to 300 ml of 46% NaCl solution for density separation. Density separation utilizes the buoyancy of plastic particles in solutions with densities higher than those of the plastics (ρ = 0.9–1.6 g/cm³), allowing soil minerals such as silica (ρ > 2.0 g/cm³) to remain at the bottom. To prepare such a solution, weigh 20 g of NaCl and dissolve it in water until the total volume reaches 100 mL. A saturated NaCl solution (ρ = 1.2 g/cm³) is particularly effective for separating low-density polymers such as PE, PP, and PS from soil mineral matrices due to its affordability, availability, and environmental safety. This method is also suitable for separating polymers such as PET (ρ = 1.3–1.6 g/cm³) and PVC (ρ = 1.1–1.6 g/cm³). Furthermore, the degradation of organic matter for sediment samples was carried out using an oxidative degradation method with 30% H2O2 until all-natural organic matter was digested. Then, a 3% HCl solution was added to dissolve the remaining chitin fractions and other mineralized solids.
The samples were then agitated using a magnetic stirrer for 30 min until the supernatants were created. Preserved sea surface water and sediment samples were then poured through a vacuum filter using cellulose filter paper within a Buncher funnel. Both sets of samples were then filtered through a Whatman Grade 42 filter paper, a high-quality cotton linters-based filter material, which has a diameter of 42.5 mm and a thickness of 200 μm. The filter offers a pore size of 2.5 μm, suitable for fine particle retention, with a basis weight of 100 g/m². It has a wet burst pressure of 0.40 psi. The ash content is minimal at ≤0.007%, ensuring low contamination for precise filtration applications. The filter was then transferred to a rinsed petri-slide for drying. The filter paper was then removed, dried, and ready for further analysis. The filtered microplastics were observed by moving the particles, utilizing tweezers without counting the biological structure in the filter paper as described previously (Li et al., 2019). Due to the microplastics not being uniform, only 80% of the most abundant microplastic shapes were analyzed.
2.4 Microplastic quantification and identification
The filtered microplastic particles were visualized under a microscope (Carl Zeiss Microscopy GmbH Königsalle 9-21 37081 Göttingen, Germany) connected to a camera (Zeiss/Axiocam 105 Colour) at a magnification of 40× and the particle images were counted for abundance analysis. The rules of misidentification of microplastics and contamination identification were subject to the method described previously (Curren and Leong, 2019). Microplastic abundance was presented in item L-1 for the seawater samples and item g-1 for the sea bed samples. The filtered seawater was observed under 40x magnification, ensuring no organisms were present (Curren and Leong, 2019). Microplastic morphology was categorized into seven sizes based on their length. Microplastics were identified based on color and shape and then measured for size on the longest dimension as described in the previous protocol (Kershaw et al., 2019). The microplastics were visually observed through microscopy to categorize their size and color including the absence of visible cellular or organic structures, thick fibers, and particles that demonstrated a consistent color (Kershaw et al., 2019). In the smallest size range of 1-40 µm, particle sizes vary between 4.704 µm and 37.247 µm, with fragment-shaped particles, which are typically small, irregularly shaped pieces broken off from larger objects, ranging in size from a minimum of 4,704 µm to a maximum of 207,628 µm. In the 40-60 µm size range, the sizes range from 40.739 µm to 55.339 µm, while in the 60-80 µm range, the sizes range from 61.886 µm to 76.289 µm. Filament-shaped particles, which are long, thin fibers like those found in textiles or fishing nets, span a size range from 18,562 µm to 3,799,246 µm.
Furthermore, in the 80-100 µm size range, particle sizes range between 80.342 µm and 99.253 µm, while the 100-500 µm size range shows variation between 102.854 µm and 493.4 µm. Film-shaped particles, which are flat, flexible sheets with smooth or angular edges like plastic wraps, range in size from 26,387 µm to 858,405 µm. In the 500-1000 µm range, particle sizes vary from 502.589 µm to 992.411 µm, and pellet-shaped particles, which are small, rounded granules with a smooth or granular surface often used as raw materials in plastic production, have sizes ranging from 4,984 µm to 81,488 µm. In the largest size range of 1000-5000 µm, particle sizes vary between 1,027.724 µm and 3,799.246 µm. These size ranges reflect the significant variation in the sizes and shapes of microplastic particles, each with unique physical characteristics. We analyzed the polymer identification of approximately 30% of the particles and selected a different morphology of particles (Hendrickson et al., 2018). The microplastic polymers were identified using the Micro-Raman method. Microplastics were placed into the sample chamber and read using a Micro-Raman machine. (Horiba Jobin Yvon-LabRam HR Evolution, Longjumeau, France) with a 785 nm excitation laser and integration times of 7 s to several minutes. Areas representing ND filter and an accumulation of 25% of the total surface were conducted to determine the polymer type (Horiba Jobin Yvon-LabRam HR Evolution, Longjumeau, France).
2.5 Quality control
To ensure the precision of the results, the Neuston nets and Ekman Grab samplers were calibrated before sampling, using recognized reference materials to validate their effectiveness in identifying microplastics. Furthermore, the light microscope and Raman spectroscopy equipment underwent calibration using established standards prior to analysis in order to verify the accuracy of size, shape, and polymer identification. Furthermore, to prevent contamination in laboratory analysis, glass and metal labware were used following previous protocols (Curren and Leong, 2019). The lab bench was cleaned with ethanol before each process to remove any present particles from the workspace. During the analysis, the glass and metal labware were used and thoroughly washed with deionized water to prevent contamination or cross-contamination of samples. The sample containers were covered with glass lids to prevent airborne contamination. Furthermore, in the laboratory setting, during the analysis of microplastics, it was imperative for the individuals involved to wear appropriate attire. This included using cotton lab coats, which were preferable to minimize the risk of static charge and potential contamination, and latex gloves to maintain a sterile and controlled environment (Cutroneo et al., 2020).
2.6 Statistical analysis
Using SPSS v24.0, we performed a one-way ANOVA with Tukey’s Honestly Significant Difference test (p = 0.05) to evaluate the influence of research station location on different MP properties in water and sediment samples. Significant variations in the abundance of films, pellets, and filaments between stations were found by the analysis, with F-values above the crucial F-value (Ftab). The MP abundance in the water and sediment samples was analyzed using a one-way ANOVA with Turkey’s Honest Significant Difference test (p=0.05) using the SPSS v24.0 software (Advanced Concurrent User Edition, SPSS Inc, Chicago, IL, USA).
3 Results
3.1 Qualitative of abundance and distribution of Microplastics Resource Identification
Microplastics were observed in each replicate from the samples collected at six sampling sites from the Flores Sea (Figure 1). The abundance of microplastic ranged from 0.84 items L-1 to 2.24 items L-1 in the surface waters and 0.84 items g-1 to 2.75 items g-1 in the seabed (Figure 2; Supplementary Information 1). As shown in Figure 2, the abundance of microplastic was highest in the surface water of Site 2 (2.23 ± 0.25 items L-1), followed by Site 4 (1.43 ± 0.1 items L-1) whereas the lowest abundance was found at Site 5 (0.75 ± 0.49 items L-1) in the Flores Sea. Among the seabed sampling sites, Site 4 had the lowest abundance with 0.84 items g-1, and Site 3 had the highest abundance of 2.75 items g-1. Our result demonstrated the presence and widespread distribution of microplastics in the Flores Sea.
3.2 Characterization of microplastics from the surface water and seabed of the Flores Sea
The color variations were generally slightly different between surface water and seabed. In the surface water, microplastics of eight colors were observed in the sea: blue, brown, black, red, yellow, gray, green, and transparent. Microplastics in the seabed were observed in blue, brown, black, red, and yellow. Blue, brown, black, red, and gray microplastics were found across all research sites, while yellow, green, and transparent microplastics were specific to certain locations. Yellow was identified at Sites 1, 2, 3, and 4; green at Sites 2, 5, and 6; and transparent at Sites 1, 2, and 4 (Figure 3; Figure 4; Appendix 2). The ANOVA statistics showed a significance level of 0.05 indicating that the calculated F-value (Fcalc) was greater than the critical F-value (Ftab). This suggests that the research station location significantly affected the quantity of microplastics in filament, pellet, and film forms. However, for microplastics in fragment form, the Fcalc value was less than the critical F-value, indicating that the research station location did not significantly impact the quantity of fragment microplastics. Additionally, when examining the colored microplastics (blue, brown, red, yellow, grey, green, and transparent) from Stations 1 to 6, the Fcalc values were smaller than the Ftab. Thus, the research station location had no significant influence on the quantity of these colored microplastics. However, for black-colored microplastics, the Fcalc value was greater than the critical F-value, indicating that the research station location significantly affected the quantity of black-colored microplastics. For microplastic size categories 1-40 μm and 80-100 μm from Stations 1 to 6, the Fcalc values were greater than the critical F-value, suggesting a significant impact of the research station location on the number of microplastics in these size ranges. Conversely, for microplastics sized between 40-1,000 μm and 1,000-5,000 μm, the Fcalc values were smaller than the Ftab, indicating no significant difference in microplastic size across these six stations. Finally, the analysis of microplastic abundance from Stations 1 to 6 resulted in Fcalc values smaller than the critical F-value, implying that the research station location did not significantly affect the quantity of microplastics based on abundance. Microplastics found in the seabed were not subjected to statistical analysis because the samples only came from three stations (Stations 2,3, and 4, as denoted by a, b, and c in Figure 1). Thus, the number of samples was limited and no repetitions were carried out.
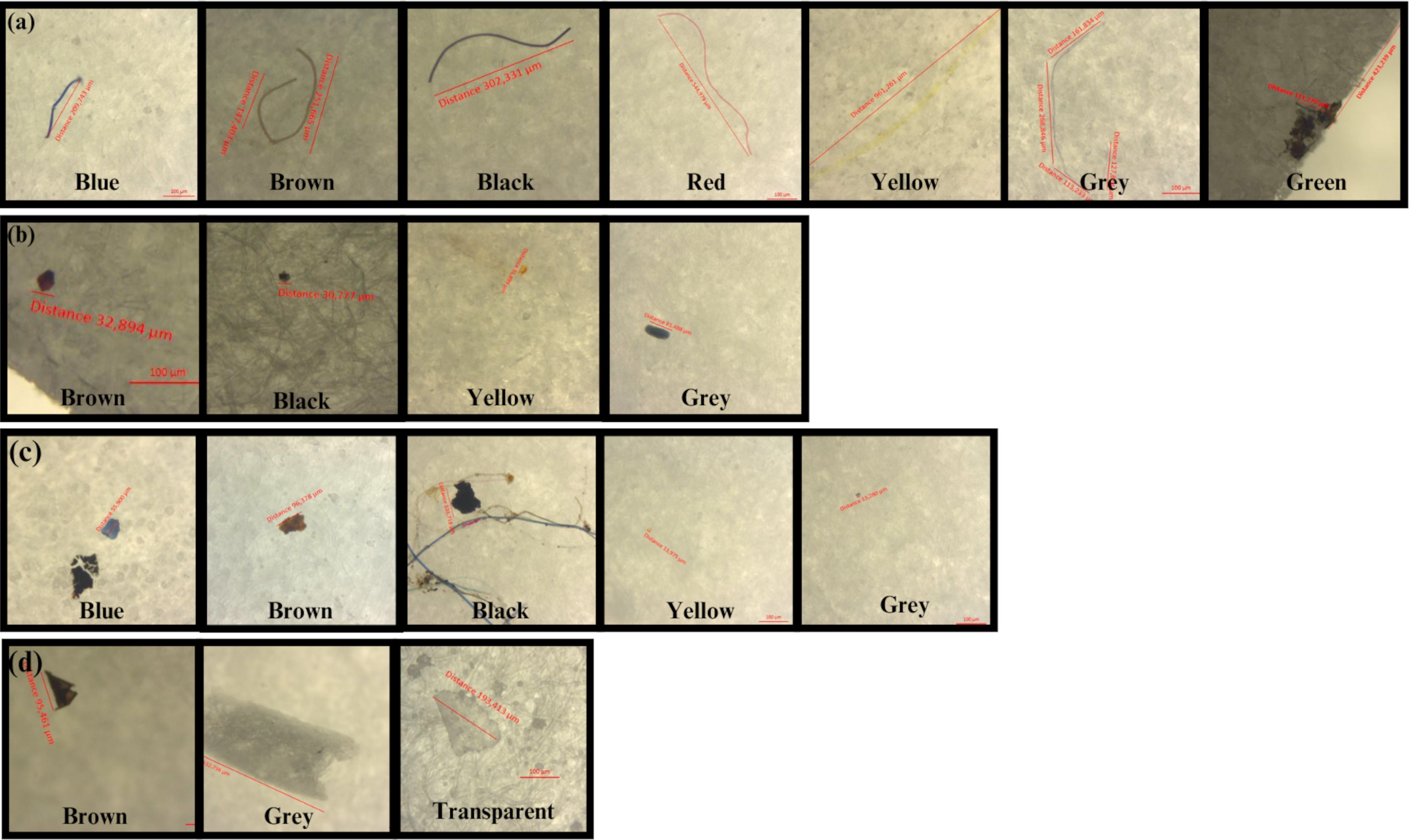
Figure 3. Microplastic found in the Flores Sea (A) filament, (B) pellet, (C) fragment, and (D) film.
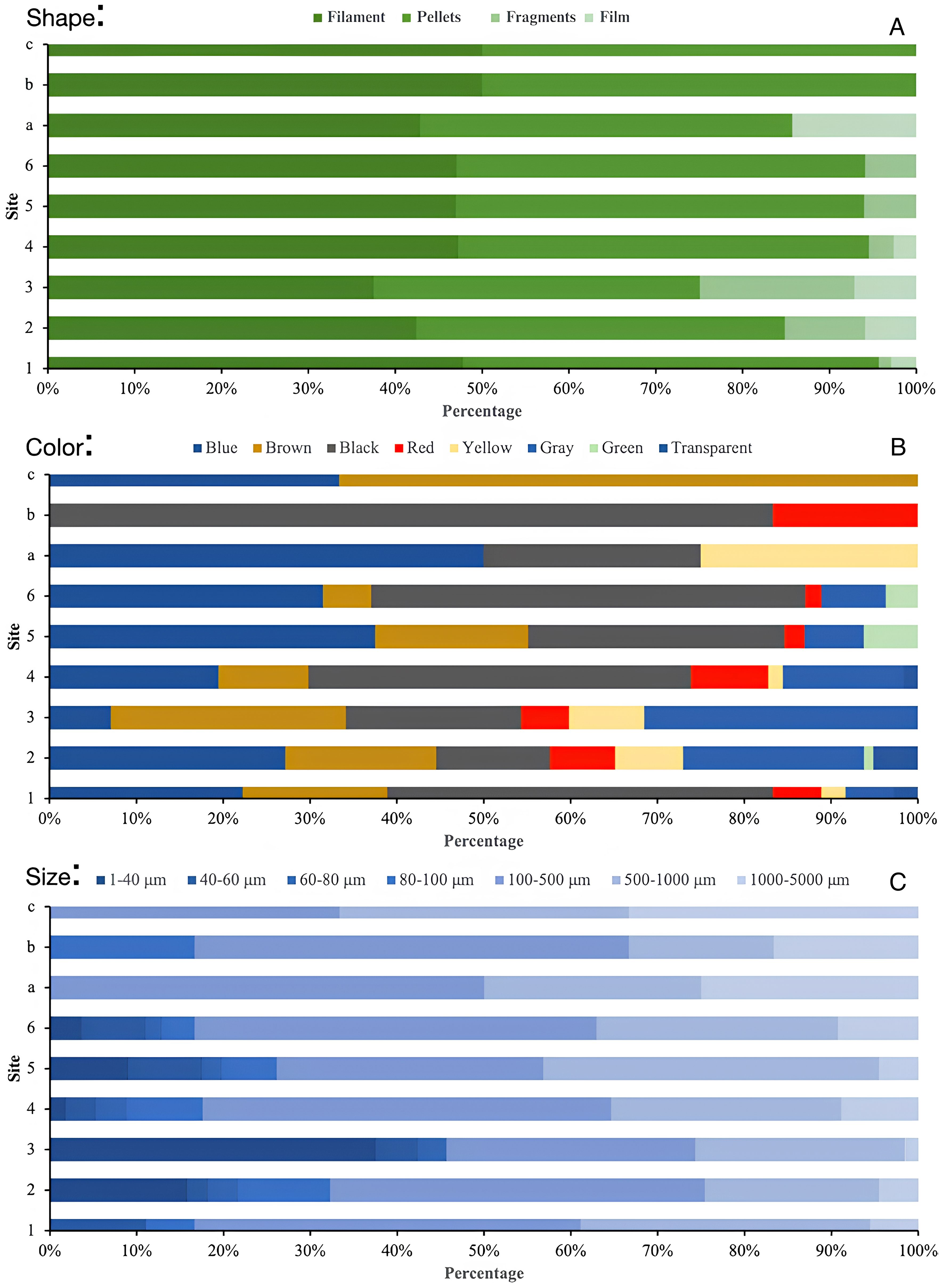
Figure 4. Percentage of MPs by shape, color, and size at the six surface sites (1-6) and three seabed sites (A-C).
The microplastics identified in the surface water were filaments, pellets, fragments, and films (Figures 3, 4). Filaments and fragments were found at all sites. Film was found at four sites, and pellets were only found at two sites in the central region (Sites 2 and 3). Filament-shaped microplastics were significantly more abundant compared with fragments, films, and pellets. The microplastics identified in the seabed were in the form of filaments and fragments.
The microplastics identified at each site on the water surface varied in size and were categorized into seven size classes, measuring between 1-40 μm to 1000-5000 μm. The microplastics found at depths of 500 to 1,145 meters were larger than the microplastics on the water’s surface. The microplastics found at a depth of 500 meters (Site 4) were filament-shaped and were blue, black, and yellow, with sizes ranging from 375.143 μm to 1,005.008 μm. At a depth of 850 meters (Site 3), they were filament-shaped, appearing in blue, black, and yellow, with sizes ranging from 67.197 μm to 2,176.87 μm. Finally, at a depth of 1,145 meters (Site 2), they were filament-shaped, with colors of blue, black, and yellow, and sizes ranging from 110.439 μm to 1,562.58 μm. Microplastics on the seabed of the Flores Sea were relatively more abundant and larger than on the surface, requiring a long time to degrade into smaller sizes. Microplastics dispersed on the surface of the Flores Sea, especially those of tiny sizes, are a concern as they may be consumed by zooplankton. The abundance of MPs did not exhibit any significant change. The F-values were smaller than Ftab, which shows that the abundance of most of the colored microplastics (blue, brown, red, yellow, grey, green, and transparent) did not differ significantly across stations. Nonetheless, the station placement had a considerable impact on black-colored microplastics, with F-values larger than Ftab. Strong differences were seen in microplastic diameters of 80-100 μm and 1-40 μm, with F-values larger than Ftab, indicating a strong influence of station location. Nevertheless, microplastics with F-values smaller than Ftab and sizes between 40-1000 μm and 1000-5000 μm did not exhibit any significant change. Overall, since the F-values were smaller than the Ftab, there was no discernible variation in the amount of microplastics across stations. Seabed microplastics were not included in the statistical analysis since there were not enough repetitions in the small number of sediment samples (from only three locations).
The distribution of black microplastics remained consistent at Stations 1, 4, and 6, but diverged at Stations 2, 3, and 5. Moreover, the proportion of blue microplastics ranged from 7.07% to 37.50%, with the lowest found at Site 3 and the highest at Site 5. Brown microplastics varied from 5.56% at Site 6 to 27.06% at Site 3, while red microplastics ranged from 1.85% at Site 6 to 7.50% at Site 2. Gray microplastics ranged from 5.56% at Site 1 to 31.52% at Site 3, while black microplastics ranged from 13.13% at Site 2 to 50.00% at Site 6. Yellow microplastics ranged from 1.67% at Site 4 to 7.30% at Site 2, and green microplastics ranged from 1.09% at Site 2 to 6.29% at Site 5. Transparent microplastics ranged from 1.67% at Site 4 to 5.13% at Site 2. In the seabed, microplastics in five colors—blue, brown, black, red, and yellow—were observed. At Site 2, blue, black, and yellow microplastics were found; at Site 3, black and red microplastics; and at Site 4, blue and brown microplastics.
3.3 Polymer Identification of the Microplastics
MPs found in the Flores Sea have different types of polymers depending on the chemical structure. Chemical bands and chemical environments where the MPs are located have different vibration energies and produce different bands on the Raman spectrum. The identification result shows the types of plastic that are distributed in the surface water and seabed Flores Sea were polyethylene terephthalate (PET/PETE) and polyester (PE) film, and another material identified was cellulose. The color material used in polyethylene terephthalate is Terre Verte and the chemical structure and spectrum are shown in Figure 5.
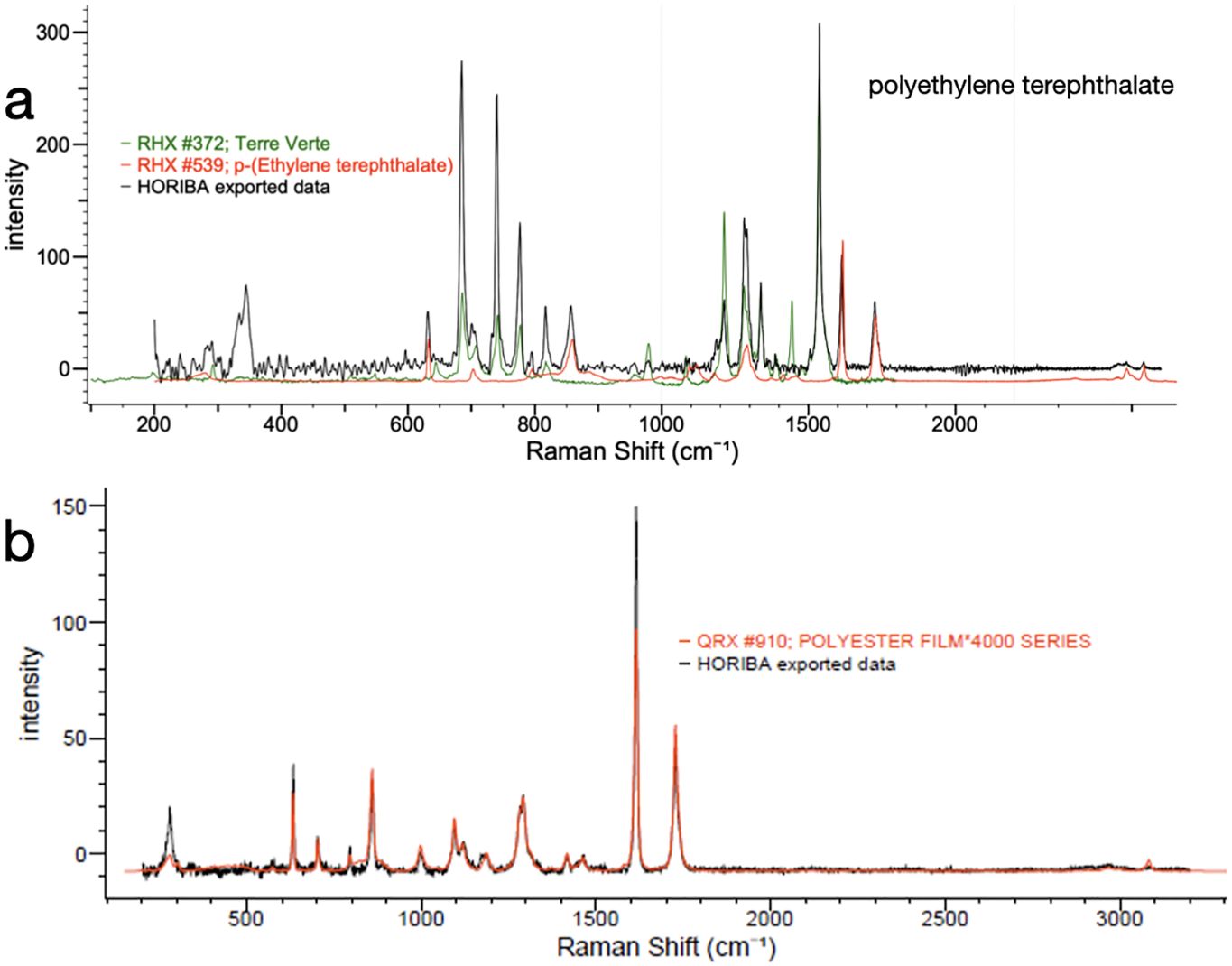
Figure 5. Type of polymers identified in the Flores Sea. (A) Spectrum of PET. (B) Spectrum of polyester film.
The most predominant polymer found at the study site was PET/PETE, which is a thermoplastic resin belonging to the polyester group. It is produced within the chemical industry and finds applications in synthetic fibers, beverage bottles, and food containers. Polyester film is a plastic film derived from the polymer PET. PE is a synthetic fiber material that was discovered in 1941 by John Rex Whinfield. This material boasts high strength, resistance to chemicals, elasticity, excellent flexibility, durability, and recyclability. It can be utilized in various industries, including packaging, printing, and electronics.
4 Discussion
This study represents the first report on microplastics in the layers of the ocean in the Flores Sea. Results from this study revealed that elevated levels of microplastics were observed in surface water compared to the seabed across six different sites, including three seabed sites, within the West Nusa Tenggara Province and South Sulawesi Province. The site with the highest abundance of microplastics in all surface water samples (2.13 ± 0.25 items L-1) was identified in the central region (Site 2), located northwest of Batuata Island. Notably, Kupang Bay exhibited a substantial abundance as well, with 0.1033 items L-1 during high tide and 0.0725 items L-1 during low tide (Kapo et al., 2020). Furthermore, an abundance of 1.03 items L-1 was found on the water surface at Bala-Bakang islands, Mamuju, West Sulawesi (Putra, 2019). In sediment at Site 3, 2.75 items g–1 at a depth of 850 m was recorded, compared to 0.110 item g–1 at Bala-Bakang islands, which was the highest record in the deep sea. Previous research showed that near Kupang (Station 6), the abundance of microplastic was found to be 0.018 ± 0.175 item L-1 in the surface water (Hiwari et al., 2019). Thus, the high abundance of MPs in surface water and sediment in the Flores Sea was derived from human activities. To the northwest of the Flores Sea is the city of Makassar, where previous research has found that this area produces microplastics from river inputs (Faizal et al., 2021; Wicaksono et al., 2021). Recent studies indicated that fibers, fragments, pellets, and foams are the types of microplastics in the waters of Indonesia (see Table 1). Our findings are consistent with observed trends of microplastics in Indonesia, highlighting gaps in the identification of small particles in the water column.
The local hydrodynamic conditions, including ocean currents, wind, tides, and eddies, can have a substantial impact on the dispersion and deposition of microplastics at individual study sites. The ITF is a significant driver for the dispersion of marine debris in the Flores Sea. This extensive hydrological system affects the dispersion of marine debris over very long distances and can result in fluctuating levels of microplastics at different sites. Microplastic distribution and spreading due to complex oceanographic characteristics correspond with wind-driven currents (Nuzula et al., 2016). The Flores Sea, located in Southeast Asia, is part of the larger complex of seas in the western Pacific Ocean. It is bordered by various islands, including Sulawesi, Flores, and Sumbawa, and it serves as a passage for water exchange between the Pacific and Indian Oceans. The water flows from the Java Sea and Makassar Strait through the Flores Sea to the Banda Sea (Putriani et al., 2019). Meanwhile, there are surface eddies that influence circulation in this region. In this case, the pathways of MPs on the surface might be transported to the eastern regions (Banda Sea), and then stranded on the northern island due to the circulation of eddies (Nuzula et al., 2016) (Figure 6). This circulation and turbulence might influence the distribution of microplastics in the water column, with each polymer such as PVC, PE, and PET, having distinct molecular weights and densities (Lusher et al., 2015; Barrows et al., 2018; Pan et al., 2019). It has been reported that microplastics were transported from the surface to the sub-surface over a period of time due to the higher velocities occurring in surface currents compared to sub-surface currents in the Bohai Sea (Dai et al., 2018). Taken together, the physical density and shape of microplastic particles might determine their ability to be distributed from the surface to the seabed, depending on the oceanographic characteristics present.
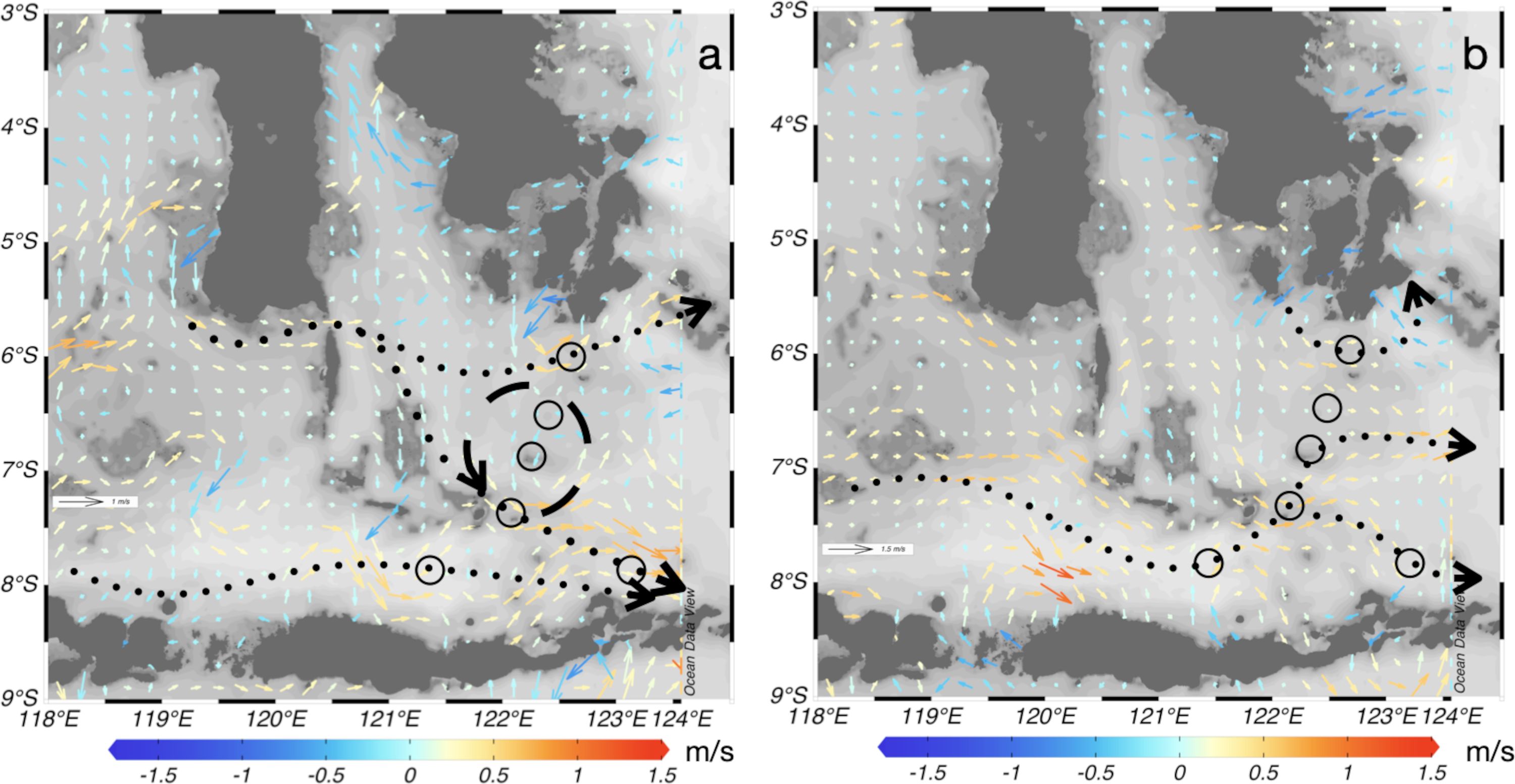
Figure 6. Surface ocean currents during sampling activities overlaid with station samplings in (A) the Northwest Monsoon and (B) the Southeast Monsoon. Dot-arrows indicate the general direction of ocean surface currents intersecting with the station samplings.
The Flores Sea exhibits a complex and multifaceted circulation pattern. In the Northwest Monsoon (NWM) (Figure 6A), ocean currents predominantly flow towards the eastern part (Banda Sea), accompanied by the circulation of eddies, adding to the intricate dynamics of the region. The spreading of microplastics is influenced by ocean currents originating from the Makassar Strait and Java Sea, contributing to the dispersion patterns observed in the area. In the Southeast Monsoon (SEM) (Figure 6B), the ocean currents also move towards the eastern side; however, the velocity is lower than that observed in the NWM, further shaping the transport characteristics of microplastics in the Flores Sea. This ocean current system has the potential to transport marine debris, including plastics and other pollutants, from Indonesia to other countries in the region and beyond (Purba et al., 2021). The fast-moving waters of the Indonesian Throughflow can carry debris over long distances, increasing the likelihood that it will reach coastal areas in neighboring countries and even more distant regions. This process can contribute to the transboundary issue of marine pollution, affecting Indonesia, the surrounding nations, and the broader marine ecosystem. Indonesia’s seas are connected to the Pacific and Indian Oceans; therefore, regional joint observations are needed.
All the surface water and seabed microplastics were collected from six different surface water sites and 3 seabed sites (Figure 4). The heterogeneity of microplastic characteristics was measured in size (Figure 4). The size of the microplastics was examined in this study to determine whether the microplastics might pose a possible biological risk due to microplastic pollution (Kobayashi et al., 2021). We found that microplastics presented in four types, namely, filaments, pellets, fragments, and film. Our result shows that filaments represented the largest proportion of microplastics observed in the surface waters and seabed, accounting for approximately 40%, whereas the size of the filaments was larger in the seabed at different depths (67.197 μm - 1,005.008 μm) (Figure 4). Our result is consistent with previous findings where the abundance of filaments/fibers was found to be widespread in the open ocean (Abayomi et al., 2017). Furthermore, our findings of the prevalence of filament is consistent with the study by Desforges et al. (2014), which found that filaments are the most-identified microplastic type in the Pacific Ocean. The filaments identified in this study are most likely derived from fishery and shipping activities due to tuna fishing. Interestingly, the size of the microplastics varied at different depths. We found that the size of microplastics present in the seabed were larger than those in the surface water in the Flores Sea. This is similar to previous findings that microplastics lose their fouling, causing them to sink into the water column or accumulate on the seabed (Dai et al., 2018; Fazey & Ryan, 2016). The plastic was transported from the land into the open ocean and ended up in the seabed. The plastic degrades into tiny particles through photo-oxidation, biological processes, and abrasion (Andrady, 2017; Cole et al., 2011). Thus, ocean currents divert small particles or microplastics such as fibers, filaments, film, and fragments from the shallow water to the deep water. Therefore, the seabed topography of the ocean features and ocean dynamics may explain the existence of MPs in the deep sea (Kane et al., 2020). This is the first report to successfully demonstrate the distribution and morphology of microplastics in the Flores Sea by comparing sea surface and seabed sites.
Plastic polymer components are commonly found in the sea surface water and deep sea (Kane et al., 2020; Wang et al., 2022). Analyzing microplastic chemical composition is a necessary approach to elucidate the source of the microplastics identified in the ocean. Our results demonstrate that PET was a major polymer in the study area (Figure 5). Our findings are consistent with the previous studies globally, which found that fiber/filament compositions consist of PET, PE, PP, PVA, and PE (Browne et al., 2011; Wang et al., 2022). Other studies in the waters of Sumba found a similar composition to the findings in this research (Cordova and Hernawan, 2018). It has further been reported that PET is used in clothes and the components of ropes, nets, and fishing lines (Wang et al., 2020). The filament sources in this study are likely fishing nets since the Flores Sea is one of Indonesia’s main fishing grounds. PET degrades into particles with higher densities that are likely to sink to the bottom of the ocean (Wang et al., 2022). The vertical transport MPs is influenced by oceanographic phenomena, including wind, storm, currents, and tides (Lusher et al., 2015; Reisser et al., 2015). In this study, we demonstrated that PET and PE were the types of polymers present in the Flores Sea water columns and seabed. This indicates that the concern regarding the region being polluted by microplastics is valid. MPs have become ubiquitous in marine benthic environments within open waters. It has been well-documented that microplastic particles, such as biofilms, have been found in benthic organisms such as shrimp and mussels (Li et al., 2019; Fernández Severini et al., 2020). The bacteria Photobacterium rosenbergii have been observed colonizing the surface of microplastics found in the Singapore Strait (Curren and Leong, 2019). Microplastics have also been found in the gastrointestinal tracts of fish and zooplankton (Collard et al., 2017; Ory et al., 2017). Moreover, it would be interesting to inspect the impact of microplastics on the mega and microfauna on the seabed within this region.
The presence of microplastics in the Flores Sea highlights the urgent need for effective strategies to mitigate their effects. Establishing long-term monitoring programs will provide continuous data on the presence and distribution of microplastics, essential for formulating policy grounded in robust evidence. Community engagement and education are important in this undertaking. By implementing public awareness campaigns and coordinating community cleanup efforts, it is possible to successfully reduce plastic waste and develop a proactive culture of environmental responsibility. The need for international cooperation is essential. Given that microplastic pollution often surpasses geographic borders, developing and strengthening regional and worldwide partnerships, participating in international agreements, and supporting advanced solutions and technology for the eradication and recycling of microplastics are essential actions. In addition to the discussed observations and findings, it is crucial to underscore the significance of the ITF within the framework of microplastic contamination. The ITF is a substantial circulation that arises from the Pacific Ocean, known for the existence of the Pacific Garbage Patch. The oceanographic feature in question serves as a significant conduit for marine garbage, particularly microplastics, throughout the Indo-Pacific region. Positioned inside this flow, the Flores Sea is a crucial location for monitoring and comprehending the spread and consequences of microplastics. By considering its location along the ITF routes, this region offers a crucial understanding of the transportation and accumulation of microplastics from larger marine currents. Hence, comprehensive evaluation and observation along these routes are crucial for thoroughly addressing and reducing marine debris contamination. The present study not only explained the present condition of microplastics in the Flores Sea but also emphasized the necessity for ongoing monitoring and future actions to alleviate the impacts of MPs in the eastern Indonesian Seas.
5 Conclusions
We identified MPs in the Flores Sea, revealing their presence in both surface waters and the seabed, with variations in abundance, shape, color, and size. The spatial trends suggest that MP abundances are higher in the surface water. The microplastics in the Flores Sea mainly originate from PET and PE polymers, the dominant components being films, filaments, and fragments. The study also highlighted the complex circulation patterns and oceanographic characteristics of the Flores Sea, which play a significant role in the distribution of microplastics. This study contributes to our understanding of the distribution and characteristics of microplastics in the Flores Sea. It is a vital reference point for future environmental assessments and policy development to mitigate marine debris in Indonesian seas, particularly in the Flores Sea and surrounding areas. To identify patterns and comprehend the long-term effects on marine ecosystems, regular monitoring of microplastic pollution in the Flores Sea and other ITF-affected areas will be crucial. Research findings can also be used to guide national and international policy actions, including stricter regulation of the sources of plastic pollution and the preservation of crucial marine habitats such as the Flores Sea. Because the ITF affects both the Pacific and Indian Oceans, the results of this study expand to our knowledge of how microplastics are dispersed between ocean basins, highlighting the necessity of concerted worldwide efforts to combat marine pollution.
Data availability statement
The original contributions presented in the study are included in the article/Supplementary Material. Further inquiries can be directed to the corresponding author.
Author contributions
TH: Conceptualization, Formal Analysis, Funding acquisition, Project administration, Writing – original draft, Writing – review & editing. WD: Methodology, Writing – original draft, Writing – review & editing. GY: Data curation, Formal Analysis, Methodology, Software, Writing – review & editing. YI: Conceptualization, Resources, Validation, Writing – review & editing. BP: Formal Analysis, Investigation, Writing – original draft. GH: Writing – review & editing. MM: Writing – review & editing. HS: Writing – review & editing. RM: Data curation, Writing – review & editing. FK: Formal Analysis, Resources, Writing – review & editing. CF: Formal Analysis, Software, Writing – review & editing. NP: Conceptualization, Formal Analysis, Supervision, Visualization, Writing – original draft, Writing – review & editing.
Funding
The author(s) declare that financial support was received for the research, authorship, and/or publication of this article. This research was support and funded by the Indonesian Navy’s Hydro-Oceanographic Centre (Pushidrosal)-Jakarta and Universitas Padjadjaran-Bandung, Indonesia. The water and seabed sampling conducted in this study was funded by the Navy, and laboratory analysis was done in the Biogeochemical Laboratory Faculty of Fisheries and Marine Sciences-Universitas Padjadjaran.
Acknowledgments
The authors thank Komandan Pushidrosal for the data use permission and the conference funding. We also thank Kapten RV Rigel for their support during the Jala Citra 3-2023 “Flores” Expedition.
Conflict of interest
The authors declare that the research was conducted in the absence of any commercial or financial relationships that could be construed as a potential conflict of interest.
Publisher’s note
All claims expressed in this article are solely those of the authors and do not necessarily represent those of their affiliated organizations, or those of the publisher, the editors and the reviewers. Any product that may be evaluated in this article, or claim that may be made by its manufacturer, is not guaranteed or endorsed by the publisher.
Supplementary material
The Supplementary Material for this article can be found online at: https://www.frontiersin.org/articles/10.3389/fmars.2024.1440587/full#supplementary-material
References
Abayomi O. A., Range P., Al-Ghouti M. A., Obbard J. P., Almeer S. H., Ben-Hamadou R. (2017). Microplastics in coastal environments of the Arabian Gulf. Mar. pollut. Bull. 124, 181–188. doi: 10.1016/j.marpolbul.2017.07.011
Afdal M., Werorilangi S., Faizal A., Tahir A. (2019). Studies on microplastics morphology characteristics in the coastal water of makassar city, south sulawesi, indonesia. Int. J. Environment Agric. Biotechnol. 4, 1028–1033. doi: 10.22161/ijeab.4421
Andrady A. L. (2017). The plastic in microplastics: A review. Mar. pollut. Bull. 119, 12–22. doi: 10.1016/j.marpolbul.2017.01.082
Attamimi A., Purba N. P., Anggraini S. R., Harahap S. A. (2015). Investigation of marine debris in Kuta Beach, Bali. Proc. Environ. Eng. Water Technol. Integrated Water System Governance. 1–17.
Azizi A., Setyowati W. N., Fairus S., Puspito D. A., Irawan D. S. (2021). Microplastic pollution in the sediment of Jakarta Bay, Indonesia., in IOP Conference Series: Earth and Environmental Science, (IOP Publishing Ltd), 1–7. doi: 10.1088/1755-
Bancin L. J., Walther B. A., Lee Y. C., Kunz A. (2019). Two-dimensional distribution and abundance of micro- and mesoplastic pollution in the surface sediment of Xialiao Beach, New Taipei City, Taiwan. Mar. pollut. Bull. 140, 75–85. doi: 10.1016/j.marpolbul.2019.01.028
Barrows A. P. W., Cathey S. E., Petersen C. W. (2018). Marine environment microfiber contamination: Global patterns and the diversity of microparticle origins. Environ. pollut. 237, 275–284. doi: 10.1016/j.envpol.2018.02.062
Browne M. A., Crump P., Niven S. J., Teuten E., Tonkin A., Galloway T., et al. (2011). Accumulation of microplastic on shorelines woldwide: Sources and sinks. Environ. Sci. Technol. 45, 9175–9179. doi: 10.1021/es201811s
Chiba S., Saito H., Fletcher R., Yogi T., Kayo M., Miyagi S., et al. (2018). Human footprint in the abyss: 30 year records of deep-sea plastic debris. Mar. Policy 96, 204–212. doi: 10.1016/j.marpol.2018.03.022
Cole M., Lindeque P., Halsband C., Galloway T. S. (2011). Microplastics as contaminants in the marine environment: A review. Mar. pollut. Bull. 62, 2588–2597. doi: 10.1016/j.marpolbul.2011.09.025
Collard F., Gilbert B., Compère P., Eppe G., Das K., Jauniaux T., et al. (2017). Microplastics in livers of European anchovies (Engraulis encrasicolus, L.). Environ. pollut. 229, 1000–1005. doi: 10.1016/j.envpol.2017.07.089
Cordova M. R., Hernawan U. E. (2018). Microplastics in Sumba waters, East Nusa Tenggara. IOP Conf Ser. Earth Environ. Sci. 162, 1–8. doi: 10.1088/1755-1315/162/1/012023
Cordova M. R., Wahyudi A. J. (2016). Microplastic in the deep-sea sediment of Southwestern Sumatran waters. Mar. Res. Indonesia 41, 27–35. doi: 10.14203/mri.v41i1.99
Cózar A., Echevarría F., González-Gordillo J. I., Irigoien X., Úbeda B., Hernández-León S., et al. (2014). Plastic debris in the open ocean. Proc. Natl. Acad. Sci. U.S.A. 111, 10239–10244. doi: 10.1073/pnas.1314705111
Curren E., Leong S. C. Y. (2019). Profiles of bacterial assemblages from microplastics of tropical coastal environments. Sci. Total Environ. 655, 313–320. doi: 10.1016/j.scitotenv.2018.11.250
Cutroneo L., Reboa A., Besio G., Borgogno F., Canesi L., Canuto S., et al. (2020). Correction to: Microplastics in seawater: sampling strategies, laboratory methodologies, and identification techniques applied to port environment (Environmental Science and Pollution Research, (2020), 27, 9, (8938-8952), 10.1007/s11356-020-07783-8). Environ. Sci. pollut. Res. 27, 20571. doi: 10.1007/s11356-020-08704-5
Dai Z., Zhang H., Zhou Q., Tian Y., Chen T., Tu C., et al. (2018). Occurrence of microplastics in the water column and sediment in an inland sea affected by intensive anthropogenic activities. Environ. pollut. 242, 1557–1565. doi: 10.1016/j.envpol.2018.07.131
Desforges J. P. W., Galbraith M., Dangerfield N., Ross P. S. (2014). Widespread distribution of microplastics in subsurface seawater in the NE Pacific Ocean. Mar. pollut. Bull. 79, 94–99. doi: 10.1016/j.marpolbul.2013.12.035
Faizal I., Anna Z., Utami S. T., Mulyani P. G., Purba N. P. (2022a). Baseline data of marine debris in the Indonesia beaches. Data Brief 41, 107871. doi: 10.1016/j.dib.2022.107871
Faizal I., Purba N. P., MartasUganda M. K., Abimanyu A., Akbar M. R., Sugianto E. (2022b). Physical control on marine debris spreading around Muara Gembong, Jakarta Bay. J. Ecol. Eng. 23, 12–20. doi: 10.12911/22998993/150718
Faizal A., Werorilangi S., Samad W., Lanuru M., Dalimunte W. S., Yahya A. (2021). Abundance and spatial distribution of marine debris on the beach of Takalar Regency, South Sulawesi. IOP Conf Ser. Earth Environ. Sci. 763, 1–12. doi: 10.1088/1755-1315/763/1/012060
Falahudin D., Cordova M. R., Sun X., Yogaswara D., Wulandari I., Hindarti D., et al. (2020). The first occurrence, spatial distribution and characteristics of microplastic particles in sediments from banten bay, indonesia. Sci. Total Environ. 705, 1–10. doi: 10.1016/j.scitotenv.2019.135304
Fauziah S. H., Liyana I. A., Agamuthu P. (2015). Plastic debris in the coastal environment: The invincible threat? Abundance of buried plastic debris on Malaysian beaches. Waste Manage. Res. 33, 812–821. doi: 10.1177/0734242X15588587
Fazey F. M. C., Ryan P. G. (2016). Biofouling on buoyant marine plastics: An experimental study into the effect of size on surface longevity. Environ. pollut. 210, 354–360. doi: 10.1016/j.envpol.2016.01.026
Fernández Severini M. D., Buzzi N. S., Forero López A. D., Colombo C. V., Chatelain Sartor G. L., Rimondino G. N., et al. (2020). Chemical composition and abundance of microplastics in the muscle of commercial shrimp Pleoticus muelleri at an impacted coastal environment (Southwestern Atlantic). Mar. pollut. Bull. 161, 111700. doi: 10.1016/j.marpolbul.2020.111700
Fok L., Cheung P. K. (2015). Hong Kong at the Pearl River Estuary: A hotspot of microplastic pollution. Mar. pollut. Bull. 99, 112–118. doi: 10.1016/j.marpolbul.2015.07.050
Hendrickson E., Minor E. C., Schreiner K. (2018). Microplastic abundance and composition in Western Lake superior as determined via microscopy, pyr-GC/MS, and FTIR. Environ. Sci. Technol. 52, 1787–1796. doi: 10.1021/acs.est.7b05829
Hiwari H., Purba N. P., Ihsan Y. N., Yuliadi L. P. S., Mulyani P. G. (2019). Kondisi sampah mikroplastik di permukaan air laut sekitar Kupang dan Rote, Provinsi Nusa Tenggara Timur Condition of microplastic garbage in sea surface water at around Kupang and Rote, East Nusa Tenggara Province. Purwokerto, Indonesia: Masyarakat Biodiversitas Indonesia Vol. 5, 165–171. doi: 10.13057/psnmbi/m050204
Iñiguez M. E., Conesa J. A., Fullana A. (2016). Marine debris occurrence and treatment: A review. Renewable Sustain. Energy Rev. 64, 394–402. doi: 10.1016/j.rser.2016.06.031
Jambeck J. R., Roland G., Wilcox C., Siegler T. R., Perryman M., Andrady A., et al. (2015). Marine pollution. Plastic waste inputs from land intothe ocean. Sci. (1979) 347, 764–768. doi: 10.1017/CBO9781107415386.010
Kameda Y., Yamada N., Fujita E. (2021). Source- and polymer-specific size distributions of fine microplastics in surface water in an urban river. Environ. pollut. 284, 117516. doi: 10.1016/j.envpol.2021.117516
Kane I. A., Clare M. A., Miramontes E., Wogelius R., Rothwell J. J., Garreau P., et al. (2020). Seafloor microplastic hotspots controlle by deep-sea circulation. Sci. (1979) 368, 1140–1145. doi: 10.1126/science.aba5899
Kapo F. A., Toruan L. N. L., Paulus C. A. (2020). Jenis dan Kelimpahan Mikroplastik pada Kolom Permukaan Air di Perairan Teluk Kupang. Jurnal Bahari Papadak 1, 10–21.
Kershaw P., Turra A., Galgani F. (2019). Guidelines for the monitoring and assessment of plastic litter in the ocean: GESAMP Joint Group of Experts on the Scientific Aspects of Marine Environmental Protection. Rep. Stud. GESAMP 99, 138.
Khan K., Zeb M., Younas M., Sharif H. M. A., Yaseen M., Al-Sehemi A. G., et al. (2023). Heavy metals in five commonly consumed fish species from River Swat, Pakistan, and their implications for human health using multiple risk assessment approaches. Mar. pollut. Bull. 195, 115460. doi: 10.1016/j.marpolbul.2023.115460
Kieu-Le T. C., Thuong Q. T., Truong T. N. S., Le T. M. T., Tran Q. V., Strady E. (2023). Baseline concentration of microplastics in surface water and sediment of the northern branches of the Mekong River Delta, Vietnam. Mar. pollut. Bull. 187, 114605. doi: 10.1016/j.marpolbul.2023.114605
Kisnarti E. A., Ningsih N. S., Putri M. R., Hendriati N., Box C. (2021). “Microplastic observations in the waters of labuan bajo-gili trawangan, indonesia,” in IOP conference series: Earth and environmental science, vol. 925. (IOP Publishing Ltd), 1–8. doi: 10.1088/1755-1315/925/1/012043
Kobayashi T., Yagi M., Kawaguchi T., Hata T., Shimizu K. (2021). Spatiotemporal variations of surface water microplastics near Kyushu, Japan: A quali-quantitative analysis. Mar. pollut. Bull. 169, 1–19. doi: 10.1016/j.marpolbul.2021.112563
Koeshendrajana S., Rusastra I. W., Martosubroto P. (2016). Potensi Sumber Daya Kelautan dan Perikanan. Jakarta, Indonesia: Ministry of Fishery and Marine Affairs.
Lebreton L. C. M., Greer S. D., Borrero J. C. (2012). Numerical modelling of floating debris in the world’s oceans. Mar. pollut. Bull. 64, 653–661. doi: 10.1016/j.marpolbul.2011.10.027
Li J., Lusher A. L., Rotchell J. M., Deudero S., Turra A., Bråte I. L. N., et al. (2019). Using mussel as a global bioindicator of coastal microplastic pollution. Environ. pollut. 244, 522–533. doi: 10.1016/j.envpol.2018.10.032
Lusher A. L., Tirelli V., O’Connor I., Officer R. (2015). Microplastics in Arctic polar waters: The first reported values of particles in surface and sub-surface samples. Sci. Rep. 5, 1–9. doi: 10.1038/srep14947
Masura J., Baker J., Foster G., Arthur C. (2015). Laboratory methods for the analysis of microplastics in the marine environment: Recommendations for quantifying synthetic particles in waters and sediments (NOAA Technical Memorandum NOS-OR&R-48), 1–39.
Nuzula F., Syamsudin M. L., Yuliadi L. P. S., Purba N. P. (2016). Preface: international conference on recent trends in physics (ICRTP 2016). J. Phys. Conf Ser. 755, 0–9. doi: 10.1088/1742-6596/755/1/011001
Ory N. C., Sobral P., Ferreira J. L., Thiel M. (2017). Amberstripe scad Decapterus muroadsi (Carangidae) fish ingest blue microplastics resembling their copepod prey along the coast of Rapa Nui (Easter Island) in the South Pacific subtropical gyre. Sci. Total Environ. 586, 430–437. doi: 10.1016/j.scitotenv.2017.01.175
Pan Z., Guo H., Chen H., Wang S., Sun X., Zou Q., et al. (2019). Microplastics in the Northwestern Pacific: Abundance, distribution, and characteristics. Sci. Total Environ. 650, 1913–1922. doi: 10.1016/j.scitotenv.2018.09.244
Purba N. P., Faizal I., Abimanyu A., Zenyda K. S., Jaelani A., Indriawan D., et al. (2020). Vulnerability of Java Sea marine protected areas affected by marine debris. IOP Conf Ser. Earth Environ. Sci. 584, 0–9. doi: 10.1088/1755-1315/584/1/012029
Purba N. P., Faizal I., Cordova M. R., Abimanyu A., Afandi N. K. A., Indriawan D., et al. (2021). Marine debris pathway across Indonesian boundary seas. J. Ecol. Eng. 22, 82–98. doi: 10.12911/22998993/132428
Putra T. P. (2019). Studi Pencemaran Mikroplastik pada Ikan, Air dan Sedimen di Kepulauan Bala-Balakang, Kabupaten Mamuju, Sulawesi Barat. Makassar, Indonesia: Universitas Hasanuddin, 1–48.
Putriani P. Y., Atmadipoera A. S., Nugroho D. (2019). Interannual variability of Indonesian throughflow in the Flores Sea. IOP Conf Ser. Earth Environ. Sci. 278, 1–13. doi: 10.1088/1755-1315/278/1/012064
Rahmania R., Setiawan A., Tussadiah A., Kusumaningrum P. D., Yulius, Prihantono J., et al. (2021). Mapping seasonal marine debris patterns and potential hotspots in Banten Bay, Indonesia. IOP Conf Ser. Earth Environ. Sci. 763, 0–10. doi: 10.1088/1755-1315/763/1/012056
Reisser J., Slat B., Noble K., Du Plessis K., Epp M., Proietti M., et al. (2015). The vertical distribution of buoyant plastics at sea: An observational study in the North Atlantic Gyre. Biogeosciences 12, 1249–1256. doi: 10.5194/bg-12-1249-2015
Rist S., Carney Almroth B., Hartmann N. B., Karlsson T. M. (2018). A critical perspective on early communications concerning human health aspects of microplastics. Sci. Total Environ. 626, 720–726. doi: 10.1016/j.scitotenv.2018.01.092
Sevwandi Dharmadasa W. L. S., Andrady A. L., Kumara P. B. T. P., Maes T., Gangabadage C. S. (2021). Microplastics pollution in marine protected areas of Southern Sri Lanka. Mar. pollut. Bull. 168, 112462. doi: 10.1016/j.marpolbul.2021.112462
Smith M., Love D. C., Rochman C. M., Neff R. A. (2018). Microplastics in seafood and the implications for human health. Curr. Environ. Health Rep. 5, 375–386. doi: 10.1007/s40572-018-0206-z
Taufiqurrahman E., Wahyudi A. J., Masumoto Y. (2020). The Indonesian throughflow and its impact on biogeochemistry in the Indonesian Seas. ASEAN J. Sci. Technol. Dev. 37, 29–35. doi: 10.29037/AJSTD.596
Thushari G. G. N., Senevirathna J. D. M. (2020). Plastic pollution in the marine environment. Heliyon 6, e04709. doi: 10.1016/j.heliyon.2020.e04709
Vriend P., Hidayat H., van Leeuwen J., Cordova M. R., Purba N. P., Löhr A. J., et al. (2021). Plastic pollution research in Indonesia: state of science and future research directions to reduce impacts. Front. Environ. Sci. 9. doi: 10.3389/fenvs.2021.692907
Wang C., Tang J., Yu H., Wang Y., Li H., Xu S., et al. (2022). Microplastic pollution in the soil environment: characteristics, influencing factors, and risks. Sustainability (Switzerland) 14, 1–14. doi: 10.3390/su142013405
Wang F., Zhang X., Zhang S., Zhang S., Sun Y. (2020). Interactions of microplastics and cadmium on plant growth and arbuscular mycorrhizal fungal communities in an agricultural soil. Chemosphere 254, 126791. doi: 10.1016/j.chemosphere.2020.126791
Wicaksono E. A., Werorilangi S., Galloway T. S., Tahir A. (2021). Distribution and seasonal variation of microplastics in tallo river, makassar, eastern Indonesia. Toxics 9, 1–7. doi: 10.3390/toxics9060129
Widiastuti I. M., Serdiati N., Tahya A. M. (2021). Study of microplastic sediment abundance in palu bay, central sulawesi, indonesia. AACL Bioflux 14, 2857–2865. Available online at: http://www.bioflux.com.ro/aacl.
Yona D., Sari S. H. J., Iranawati F., Bachri S., Ayuningtyas W. C. (2019). Microplastics in the surface sediments from the eastern waters of Java Sea, Indonesia. F1000Res 8, 1–8. doi: 10.12688/f1000research.17103.1
Zhang Q., Wang F., Xu S., Cui J., Li K., Shiwen X., et al. (2023). Polystyrene microplastics induce myocardial inflammation and cell death via the TLR4/NF-κB pathway in carp. Fish Shellfish Immunol. 135, 108690. doi: 10.1016/j.fsi.2023.108690
Keywords: Jalacitra Expedition, marine litter, anthropogenic pollution, polymer, ocean circulation, Indonesian seas, ocean health
Citation: Herawati T, Damayanti W, Yuda GA, Ihsan YN, Pasaribu B, Harsono G, Marlina M, Suseno H, Mustopa RA, Karya FSW, Febriani C and Purba NP (2024) Assessment of microplastic characterization and distribution from surface water and the seabed in the Flores Sea, Indonesia. Front. Mar. Sci. 11:1440587. doi: 10.3389/fmars.2024.1440587
Received: 29 May 2024; Accepted: 04 October 2024;
Published: 30 October 2024.
Edited by:
Muhammad Reza Cordova, The Indonesian National Research and Innovation Agency, IndonesiaReviewed by:
Meng Chuan Ong, University of Malaysia Terengganu, MalaysiaGideon Gywa, Case Western Reserve University, United States
Ajay Harit, Kalinga University Raipur Chhattisgarh, India
Copyright © 2024 Herawati, Damayanti, Yuda, Ihsan, Pasaribu, Harsono, Marlina, Suseno, Mustopa, Karya, Febriani and Purba. This is an open-access article distributed under the terms of the Creative Commons Attribution License (CC BY). The use, distribution or reproduction in other forums is permitted, provided the original author(s) and the copyright owner(s) are credited and that the original publication in this journal is cited, in accordance with accepted academic practice. No use, distribution or reproduction is permitted which does not comply with these terms.
*Correspondence: Titin Herawati, dGl0aW4uaGVyYXdhdGlAdW5wYWQuYWMuaWQ=