- 1School of Ocean, Yantai University, Yantai, China
- 2National Biopesticide Engineering Research Centre, Hubei Biopesticide Engineering Research Centre, Hubei Academy of Agricultural Sciences, Wuhan, China
Due to anthropogenic input of nutrients and emissions of greenhouse gases, macroalgae inhabiting coastal areas often experience drastic fluctuations in nutrients and seawater warming. In this work, we investigated the photosynthetic performance and antioxidant response of the commercially important red macroalgae Gracilariopsis lemaneiformis under four different nutrient conditions at 20°C and 23°C. Our results showed that the enrichment of NO3- and PO43- (high concentrations of nitrogen (N) and phosphorus (P), denoted as HNHP) significantly enhanced photosynthesis and growth by up to 42% and 66% for net photosynthesis rate and 83% and 134% for relative growth rate (RGR) under 20°C and 23°C, respectively, compared with natural seawater (low concentrations of N and P, denoted as LNLP). However, enriching only with PO43- (low concentration of N and high concentration of P, denoted as LNHP) or NO3- (high concentration of N and low concentration of P, denoted as HNLP) brought no significant change in RGR. A two-way ANOVA analysis revealed an interaction between nutrient variations and temperature, with elevated temperature intensifying the inhibition observed under HNLP conditions. To further elucidate this interaction, we assessed the damage and recovery processes of the photosynthetic apparatus, along with the antioxidant activities. The increased damage (k) and reduced recovery (r) rates of photosystem II (PSII) in both LNLP and HNLP conditions indicated a heightened susceptibility to photoinhibition in G. lemaneiformis, leading to reactive oxygen species (ROS) accumulation and exacerbated oxidative stress, culminating in decreased photosynthesis and growth rates. At higher temperatures, these phosphorus deficiency-induced inhibitions were amplified, as evidenced by increases in k values and ROS contents, coupled with a decrease in r values. In summary, our data suggest that the photosynthetic performance and growth of G. lemaneiformis are vulnerable to phosphorus deficiency, particularly in the context of future ocean warming. Consequently, phosphorus fertilization during cultivation warrants more attention.
Introduction
The growth of macroalgae is highly sensitive to variable environmental changes, such as light fluctuations, temperature, and nutrients (Zhang et al., 2020a, b; Cohen et al., 2022; Jiang et al., 2022; Li et al., 2022). In coastal ecosystems, the levels of key nutrients, primarily nitrogen (N) and phosphorus (P), undergo dramatic shifts due to human activities. As an essential component, N is involved in the formation of proteins, chlorophyll, enzymes, nucleic acids, etc., and its availability significantly influences the physiological performance of algae (Roleda and Hurd, 2019). A number of studies have shown that a high nitrogen concentration could significantly prompt photosynthesis and growth of macroalgae, including Gracilariopsis lemaneiformis (Chen et al., 2018; Jiang et al., 2020), Ulva sp (Gao et al., 2018; Traugott et al., 2020). Similarly, phosphorus, another vital macronutrient, also influences photosynthetic productivity and biomass in the ocean (Karl, 2000; Kipp and Stüeken, 2017), with its enrichment shown to enhance photosynthesis in species such as G. lemaneiformis (Xu et al., 2010), Sargassum muticum (Xu et al., 2017) and Pyropia yezoensis (Kim et al., 2019). Conversely, nutrient limitations often decrease primary production by affecting carbon flux redirection and cellular energy (Falkowski and Raven, 2007; Lin et al., 2016; Brembu et al., 2017). In coastal areas, a previous investigation showed that N concentrations ranged from 10 to 17 μmol L-1 and the P concentration ranged from 0.2 to 1 μmol L-1 (Li et al., 2022). Another report also demonstrated that the lowest P concentration in core areas of large-scale macroalgae cultivation was only 0.08 μmol L-1 (Zhou et al., 2022). Such a dramatic fluctuation result in the N:P ratio, from 17:1 to 50:1, which exceeds the Redfield ratio of 16:1, suggests that P availability may be a limiting factor controlling algal photosynthesis and growth.
Temperature is considered to be another crucial factor that affects the photosynthesis and growth of macroalgae (Ji and Gao, 2021). Anthropogenic activities have increased atmospheric carbon dioxide (CO2) from roughly 280 ppm in pre-industrial times to over 410 ppm today. Under the SSP5-8.5 emissions scenario, the greenhouse gas is expected to cause an increase in global mean temperature of 4.3°C by the end of this century (Masson-Delmotte et al., 2021), with ocean surface temperature potentially rising by 2.34-2.82°C (Pörtner et al., 2019). Previous studies have shown that ocean warming can have varied effects on algae—positive, negative, or neutral—likely due to species-specific optimal growth temperatures (Liu et al., 2020; Ji and Gao, 2021). For example, a ~3°C rise in eastern Tasmania led to a >90% decline in Macrocystis pyrifera forests (Johnson et al., 2011). In Japan, a ~1°C temperature increase favored warm-temperate species such as Ecklonia cava, Ecklonia stolonifera, and Undaria peterseniana, while reducing cold-temperate species such as Laminaria japonica, Kjellmaniella crassifolia, and Costaria costata (Serisawa et al., 2004; Kirihara et al., 2006). Another example in northern Spain, the decrease of Fucus serratus and Himanthalia elongata was linked to a 1.5°C–2°C rise in coastal seawaters (Duarte et al., 2013). These findings imply that macroalgae are highly sensitive to changes in temperature associated with global climate change despite being adapted to natural variations in temperature.
Gracilariopsis lemaneiformis (Gracilariaceae, Rhodophyta), an economically important marine crop, is the second largest cultivated macroalga after Saccharina japonica in China (Zhou et al., 2024). By using floating longlines and vegetative propagation methods, G. lemaneiformis has been seasonally cultivated from northern to southern China (Pang et al., 2017; Xue et al., 2022). As reported, the cultivation area of G. lemaneiformis in China is 13,924 hm2 and its annual output reached 610,824 t (dry weight) in 2022 (Compiled by Fisheries Bureau of Ministry of Agriculture, 2023). Such high production of G. lemaneiformis not only provides food or industry resources but also contributes to mitigating climate change through the assimilation of inorganic carbon. Photosynthesis, the most general and sensitive physiological response, is pivotal for understanding how macroalgae adapt to varying temperatures and nutrient conditions (Ye et al., 2013). Currently, a number of studies have investigated the effect of nutrients or warming on photosynthesis and growth of G. lemaneiformis (Yang et al., 2021; Li et al, 2022; Zhou et al., 2024), while interaction between these factors has received less attention. Moreover, those published papers tend to focus on the reduction in photosynthetic efficiency, leaving the mechanisms of photoinhibition and their impact on cellular activities less explored. In our present study, changes in both photosynthesis performance and antioxidant enzyme activity were measured, aiming to characterize the different physiological responses of G. lemaneiformis subjected to nutrient variations and ocean warming under natural sunlight.
Materials and methods
Experimental treatments
Thalli of Gracilariopsis lemaneiformis were collected from farmed rafts offshore of Ningde, Fujian province of China (119.31°E, 26.39°N), in December 2023, and transferred to the laboratory in a cooled Styrofoam box. Following rinsing, weighted thalli of ~0.5 g fresh weight (FW) were grown for 10 days in 1.5 L open-ended tubes filled with artificial seawater, which was continuously aerated and renewed every 2 days. According to previous studies (Jiang et al., 2022; Zhou et al., 2022, 2024), as well as in-situ measurement of seawater temperature (19.6°C), the ambient temperature was set as 20°C. The warming treatment (23°C) was set following the prediction of SSP5-8.5 (Pörtner et al., 2019; Masson-Delmotte et al., 2021), where the ocean surface temperature would increase by ~3°C. Under each temperature, four nutrient levels were set as low concentrations of N and P (LNLP) (N: 8 μmol L-1, P: 0.5 μmol L-1), low concentration of N and high concentration of P (LNHP) (N: 8 μmol L-1, P: 10 μmol L-1), high concentration of N and low concentration of P (HNLP) (N: 160 μmol L-1, P: 0.5 μmol L-1), and high concentrations of N and P (HNHP) (N: 160 μmol L-1, P: 10 μmol L-1). The artificial seawater used in this study was prepared according to Berges et al. (2001) without the addition of major nutrients and elements. The tubes were partly immersed in two water baths, where the ambient water temperature, 20°C and 23°C, were controlled by two heaters (SunSun, AR-450, SunSun Group Co., Ltd, China). Four nutrient levels were adjusted by adding NO3- and PO43- into artificial seawater. Three independent replicate cultures were used for each treatment (n=3). The experiment set-up graphic is shown in Figure 1.
Determination of relative growth rate and contents of Chl a
Relative growth rate (RGR) was determined by measuring the changes in FW of the thalli after 10 days and was calculated by using the following equation RGR (% d-1) = 100×(lnN10-lnN0)/10, where N10 and N0 represented fresh weights of the thalli at day 10 and 0, respectively.
Approximately 0.05 g (FW) thalli was ground and extracted in 5 mL absolute methanol at 4°C in darkness for 12 h. After centrifugation at 4°C, 5000g for 15 min, the absorbance of the supernatant was measured from 400 nm – 700 nm using a scanning spectrophotometer (752N, INESA Co. Ltd., Shanghai, China). The contents of chlorophyll a (Chl a, mg/g FW) were calculated according to Porra (2002),
where Ax is the absorbance under the x-wavelength, VE is the volume of the methanol extraction, and m is the weight of the algae.
Measurement and analysis of chlorophyll fluorescence
A portable fluorimeter (AquaPen AP110, Photon Systems Instruments, Brno, Czech Republic) was employed to measure the photosynthetic performance of photosystem II (PSII). During the measurements, a blue LED emitter with excitation light at 455 nm was used to eliminate the effect of phycobiliproteins on chlorophyll fluorescence. The minimal fluorescence (Fo) for 30 min dark-adapted thalli was induced by a low irradiance (~0.15 μmol photons m-2 s-1), and the maximum fluorescence (Fm) was obtained during a saturating flash (4000 μmol photons m-2 s-1). Following that, an actinic light with an intensity of 400 μmol photons m-2 s-1 was employed to induce a steady state of photosynthesis. The stable fluorescence (F) and the corresponding maximum steady fluorescence (Fm') during the saturating flash were monitored. The maximum photochemical quantum yield of PSII (Fv/Fm), the non-photochemical quenching (NPQ), and the effective photochemical quantum yield of PSII (YII) were calculated as Fv/Fm = (Fm – Fo)/Fm; NPQ = (Fm – Fm')/Fm'; and YII = (Fm' – F)/Fm', respectively.
According to Miao et al. (2018) and Heraud and Beardall (2000), the damage and recovery processes of the photosynthetic apparatus were obtained by periodically measuring the YII during photoinhibitory exposure (~1000 μmol photons m-2 s-1). The damage (k, min-1) and repair (r, min-1) rates were estimated using the Kok model and calculated with the following equation:
where Yn and Yo are YII at time tn and to, respectively.
Measurement of net photosynthesis and respiration rates
Net photosynthesis and dark respiration rates were measured with optical dissolved oxygen (DO) sensors (ProODO-BOD, YSI, USA). Approximately 0.2 g FW of G. lemaneiformis from each treatment was placed in a 100 mL BOD bottle containing cultivation artificial seawater, which was stirred continuously during the measurement. Temperature was maintained at either 20°C or 23°C, corresponding to the cultivation temperatures. The net photosynthesis and dark respiration rates (μmol O2 h-1 g-1 FW) were determined as the variations of DO content during light (400 μmol photons m-2 s-1) and dark conditions, respectively.
Measurement of reactive oxygen species content and antioxidant enzyme activity
Fresh samples were ground with liquid nitrogen and the tissue homogenates were used to analyze the ROS (mainly referred to as hydrogen peroxide, H2O2) content and enzyme (mainly referred to as superoxide dismutase (SOD) and catalase (CAT)) activity of G. lemaneiformis with a commercial assay kit (Jiancheng, Nanjing, China) following the manufacturer's protocols.
Statistical analyses
Statistical analyses were performed using SPSS 19.0 (SPSS Inc., Chicago, USA). The homogeneity of variance was examined using Levene's test before all statistical analyses. One-way ANOVA and t-test were used to establish differences among treatments. A two-way ANOVA was used to identify the effects of warming, nutrients, and their interactions. As shown in Figure 1, warming treatments were achieved by heating the water in the tank, therefore, all ANOVA analyses regarding warming in this study should be temperature and tank effects. Differences were considered to be statistically significant at p<0.05.
Results
Relative growth rate and Chl a content
As shown in Figure 2A, the RGR of Gracilariopsis lemaneiformis displayed a significant difference among the treatments. Compared with LNLP treatment (natural seawater), the enrichment with NO3- and PO43- (HNHP) significantly increased RGR by up to 83% and 134% in 20°C and 23°C, respectively, reaching 3.2% and 3.5% per day. However, enrichment with only PO43- concentration (LNHP), and enrichment with only NO3- concentration (HNLP) showed no significant effects on RGR. In terms of temperature variations, i.e., the temperature and tank effects, the higher temperature significantly increased the RGR in the HNHP condition but decreased in HNLP. A two-way ANOVA showed that both temperature, nutrient variations, and their interaction significantly affected RGR (Table 1). Considering the fact that both NO3- and PO43- are essential for pigment formation, a significant increase of Chl a content was observed in HNHP treatment, but not for LNHP and HNLP treatments (Figure 2B). In contrast to higher values of RGR under 23°C, the elevated temperature did not enhance the contents of Chl a (Figure 2B).
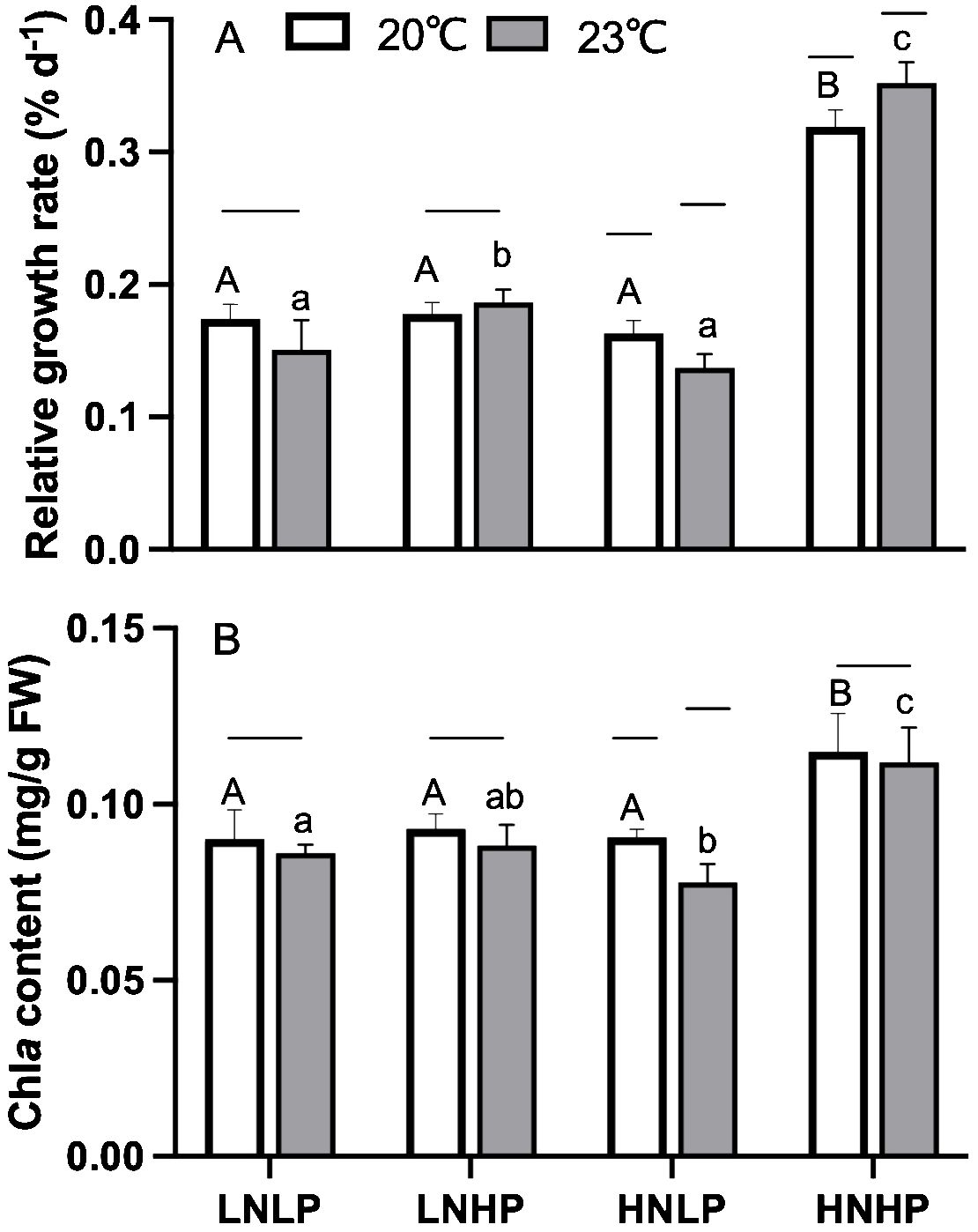
Figure 2. Relative growth rate[%d-1, panel (A)] and Chl a content [mg/g FW, panel (B)] of Gracilariopsis lemaneiformis after growing for 10 days at four nutrients levels (LNLP, LNHP, HNLP and HNHP) and two temperature (20°C, open bars, 23°C, grey bars). Each data point is means ± SD (n=3). Different upper and lower letters above the bars indicate significant differences between nutrients levels under 20°C and 23°C, respectively (p<0.05, One-way ANOVA). Unconnected line above the bars indicate significant differences between temperature under each nutrients level (p<0.05, One-way ANOVA). LNLP, low nitrogen and low phosphorus concentrations (N: 8μmol/L, P: 0.5 μmol/L); LNHP, low nitrogen and high phosphorus concentrations (N: 8μmol/L, P: 10 μmol/L); HNLP, high nitrogen and low phosphorus concentrations (N: 160μmol/L, P: 0.5 μmol/L); HNHP, high nitrogen and high phosphorus concentrations (N: 160μmol/L, P: 10 μmol/L); FW, fresh weight; SD, standard deviation.
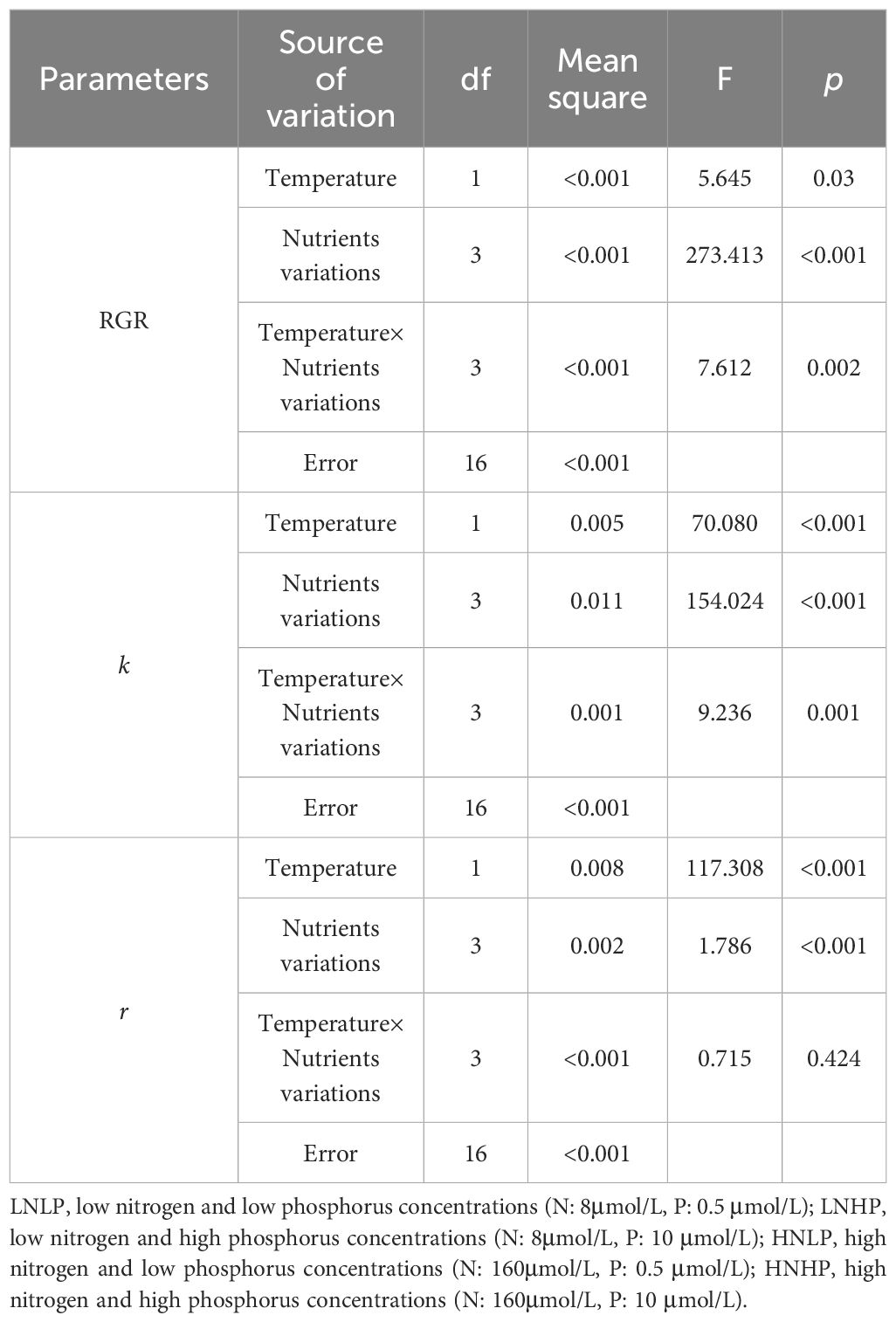
Table 1. Two-way ANOVA for the effects of temperature (20°C and 23°C) and nutrients variations (LNLP, LNHP, HNLP, HNHP) on the relative growth rate (RGR), the damage (k) and repair (r) rate.
Chlorophyll fluorescence, photosynthesis, and respiration
The maximum quantum yield of PSII (Fv/Fm) showed the highest values in the HNHP treatment, with an average value of ~0.54 at both 20°C and 23°C, and the lowest values in the HNLP treatment (Figure 3A). In terms of temperature, the values of Fv/Fm in 23°C in the LNHP and HNLP treatments were significantly higher than that in 20°C (t-test, p<0.05, p<0.05), while in the LNLP and HNHP treatments, the values of Fv/Fm showed no significant difference between 20°C and 23°C (Figure 3A, t-test, p=0.243, p=0.197). In contrast, the NPQ was significantly upregulated in the LNHP and HNLP treatment, with an average value of 0.39 and 0.42 at 20°C and 23°C, respectively. The elevated temperature did not affect the NPQ, except for the HNLP treatment (Figure 3B).
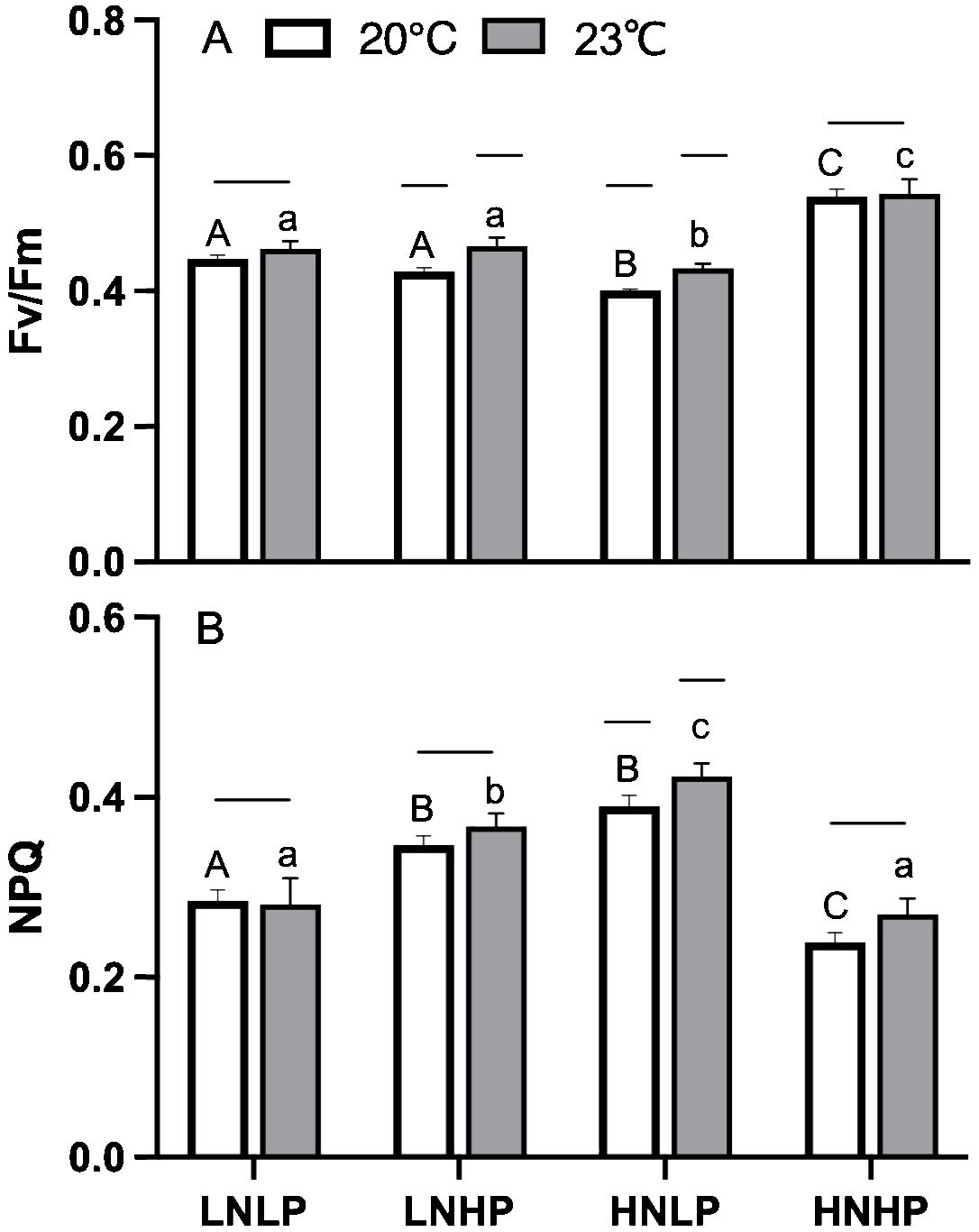
Figure 3. The maximum photochemical quantum yield of photosystem II [Fv/Fm, panel (A)] and non-photochemical quenching [NPQ, panel (B)] of Gracilariopsis lemaneiformis after growing for 10 days at four nutrients levels (LNLP, LNHP, HNLP and HNHP) and two temperature (20°C, open bars, 23°C, grey bars). Each data point is means ± SD (n=3). Different upper and lower letters above the bars indicate significant differences between nutrients levels under 20°C and 23°C, respectively (p<0.05, One-way ANOVA). Unconnected line above the bars indicate significant differences between temperature under each nutrients level (p<0.05, One-way ANOVA). LNLP, low nitrogen and low phosphorus concentrations (N: 8μmol/L, P: 0.5 μmol/L); LNHP, low nitrogen and high phosphorus concentrations (N: 8μmol/L, P: 10 μmol/L); HNLP, high nitrogen and low phosphorus concentrations (N: 160μmol/L, P: 0.5 μmol/L); HNHP, high nitrogen and high phosphorus concentrations (N: 160μmol/L, P: 10 μmol/L); SD, standard deviation.
Similar to RGR, the net photosynthesis rate of G. lemaneiformis showed the highest values in the HNHP treatment, with an average value of 40.38 μmol O2 h-1 g-1 FW and 45.81 μmol O2 h-1 g-1 FW at 20°C and 23°C, respectively (Figure 4A). The LNHP treatment brought no significant change to net photosynthesis rate (t-test, p=0.189), and the HNLP treatment significantly decreased the net photosynthesis rate (t-test, p<0.05). The elevated temperature significantly increased the net photosynthesis rate in the HNHP treatment (t-test, p<0.05), but this decreased in the HNLP treatment (Figure 4A, t-test, p<0.05). Changes in respiration rate are shown in Figure 4B; the highest values were observed in the HNHP treatment and the lowest values were observed in the HNLP treatment (Figure 4B). The elevated temperature showed no significant effect on respiration rate, except for the HNHP treatment (Figure 4B, t-test, p=0.176, p=0.237, p=0.467 for LNLP, LNHP, and HNLP, respectively, and p<0.05 for HNHP).
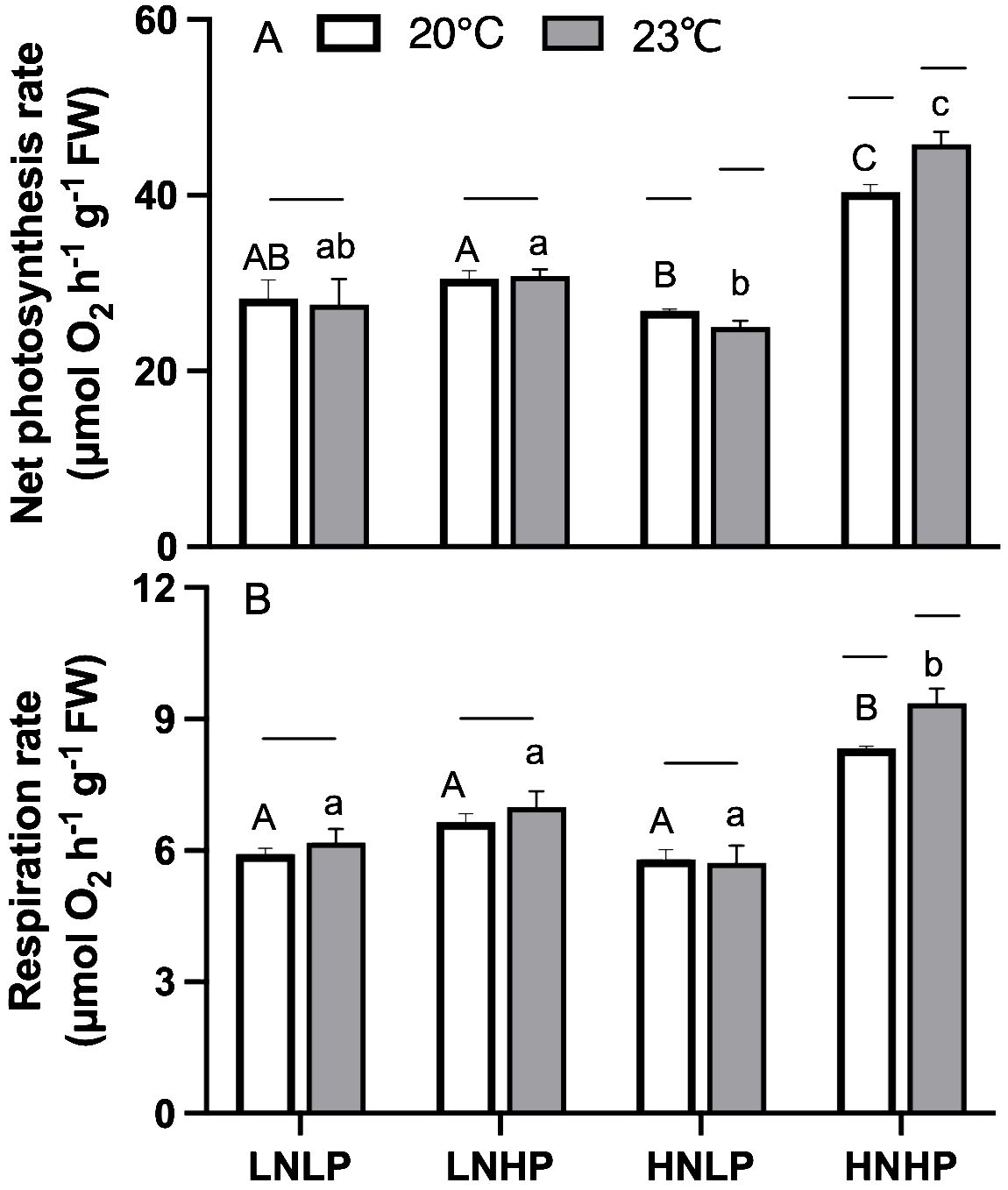
Figure 4. Net photosynthesis rate [μmol O2 h-1 g-1 FW, panel (A)] and respiration rate 9μmol O2 h-1 g-1 FW, panel (B)] of Gracilariopsis lemaneiformis after growing for 10 days at four nutrients levels (LNLP, LNHP, HNLP and HNHP) and two temperature (20°C, open bars, 23°C, grey bars). Each data point is means ± SD (n=3). Different upper and lower letters above the bars indicate significant differences between nutrients levels under 20°C and 23°C, respectively (p<0.05, One-way ANOVA). Unconnected line above the bars indicate significant differences between temperature under each nutrients level (p<0.05, One-way ANOVA). LNLP, low nitrogen and low phosphorus concentrations (N: 8μmol/L, P: 0.5 μmol/L); LNHP, low nitrogen and high phosphorus concentrations (N: 8μmol/L, P: 10 μmol/L); HNLP, high nitrogen and low phosphorus concentrations (N: 160μmol/L, P: 0.5 μmol/L); HNHP, high nitrogen and high phosphorus concentrations (N: 160μmol/L, P: 10 μmol/L); FW, fresh weight; SD, standard deviation.
Damage and repair rates of photosystem II
The rates of damage and repair of PSII during photoinhibitory exposure were estimated from the changes in the effective photochemical quantum yield of PSII (YII). The damage rate showed significantly higher values in low PO43- concentrations, i.e., the LNLP and HNLP treatments, especially under the elevated temperature (Figure 5A). The elevated temperature showed no significant effects on the values of k in both the LNHP and HNHP treatments (Figure 5A, t-test, p=0.105, p=0.217 for LNHP and HNHP, respectively). By contrast, the repair rate showed significantly higher values in high PO43- concentration, i.e., the LNHP and HNHP treatments (Figure 5A). The elevated temperature significantly decreased the repair rate by up to 8.5%, 3.4%, 16.1%, and 5.5% for LNLP, LNHP, HNLP, and HNHP, respectively. Accordingly, the ratio between r and k also showed significantly higher values in LNHP and HNHP treatments, and the elevated temperature significantly decreased the r/k (Figure 5C). A two-way ANOVA showed that temperature, nutrient variations, and their interaction, significantly affected the value of k (t-test, p<0.05, p<0.05, p<0.05) and r (Table 1, t-test, p<0.05, p<0.05), except for the interaction with r (t-test, p=0.424).
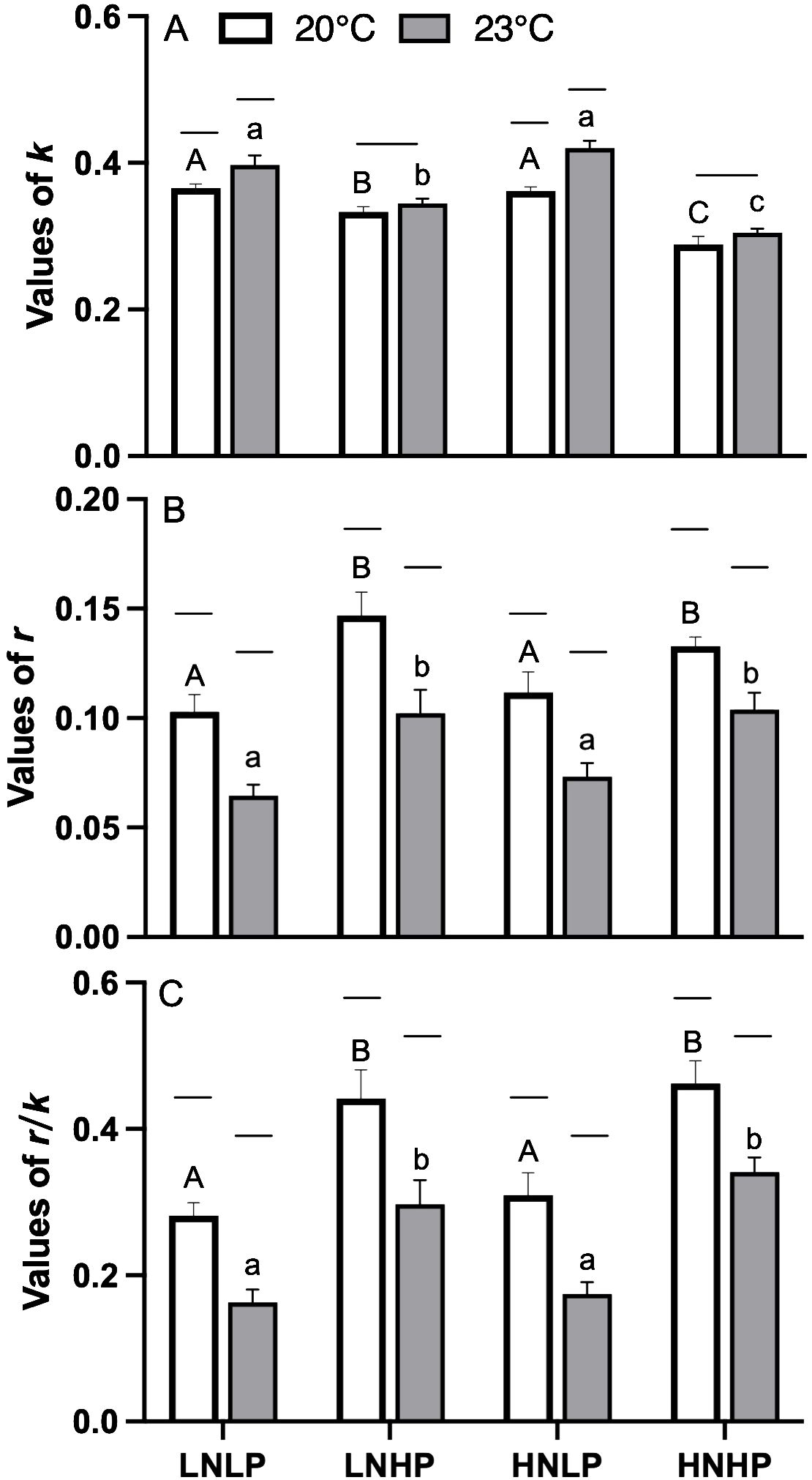
Figure 5. The damage [k, panel (A)], recovery [r, panel (B)] rates and the ratio between r and k [r/k, panel (C)] of Gracilariopsis lemaneiformis after growing for 10 days at four nutrients levels (LNLP, LNHP, HNLP and HNHP) and two temperature (20°C, open bars, 23°C, grey bars). Each data point is means ± SD (n=3). Different upper and lower letters above the bars indicate significant differences between nutrients levels under 20°C and 23°C, respectively (p<0.05, One-way ANOVA). Unconnected line above the bars indicate significant differences between temperature under each nutrients level (p<0.05, One-way ANOVA). LNLP, low nitrogen and low phosphorus concentrations (N: 8μmol/L, P: 0.5 μmol/L); LNHP, low nitrogen and high phosphorus concentrations (N: 8μmol/L, P: 10 μmol/L); HNLP, high nitrogen and low phosphorus concentrations (N: 160μmol/L, P: 0.5 μmol/L); HNHP, high nitrogen and high phosphorus concentrations (N: 160μmol/L, P: 10 μmol/L); SD, standard deviation.
Reactive oxygen species content and antioxidant enzyme activity
The ROS content was estimated by quantifying the production of H2O2 to assess the redox state of G. lemaneiformis under different treatments. As shown in Figure 6, the ROS content showed significantly higher values under the LNLP and HNLP treatments, while the enrichment of PO43- significantly alleviated the production of ROS. The elevated temperature increased the production of ROS in low PO43- concentrations (t-test, p<0.05 for both LNLP and HNLP treatments), but showed no significant effects on the LNHP and HNHP treatments (t-test, p=0.382, p=0.417 for LNHP and HNHP, respectively).
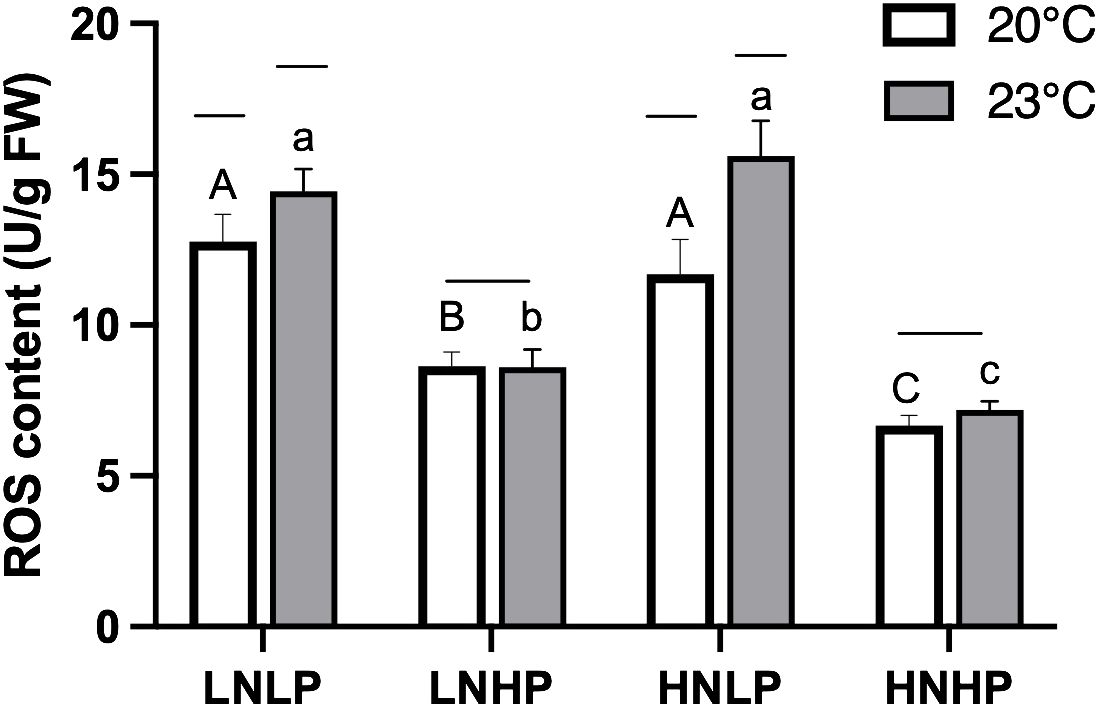
Figure 6. The reactive oxygen species content (U/g FW) of Gracilariopsis lemaneiformis after growing for 10 days at four nutrients levels (LNLP, LNHP, HNLP and HNHP) and two temperature (20°C, open bars, 23°C, grey bars). Each data point is means ± SD (n=3). Different upper and lower letters above the bars indicate significant differences between nutrients levels under 20°C and 23°C, respectively (p<0.05, One-way ANOVA). Unconnected line above the bars indicate significant differences between temperature under each nutrients level (p<0.05, One-way ANOVA). LNLP, low nitrogen and low phosphorus concentrations (N: 8μmol/L, P: 0.5 μmol/L); LNHP, low nitrogen and high phosphorus concentrations (N: 8μmol/L, P: 10 μmol/L); HNLP, high nitrogen and low phosphorus concentrations (N: 160μmol/L, P: 0.5 μmol/L); HNHP, high nitrogen and high phosphorus concentrations (N: 160μmol/L, P: 10 μmol/L); FW, fresh weight; SD, standard deviation.
In turn, changes in antioxidant enzyme activity showed similar patterns. As shown in Figure 7, both SOD and CAT showed significantly higher activities under both LNLP and HNLP treatments, while decreased by the enrichment of PO43-. The elevated temperature could further active the SOD and CAT activities in both LNLP and HNLP treatments, but not for LNHP and HNHP treatments.
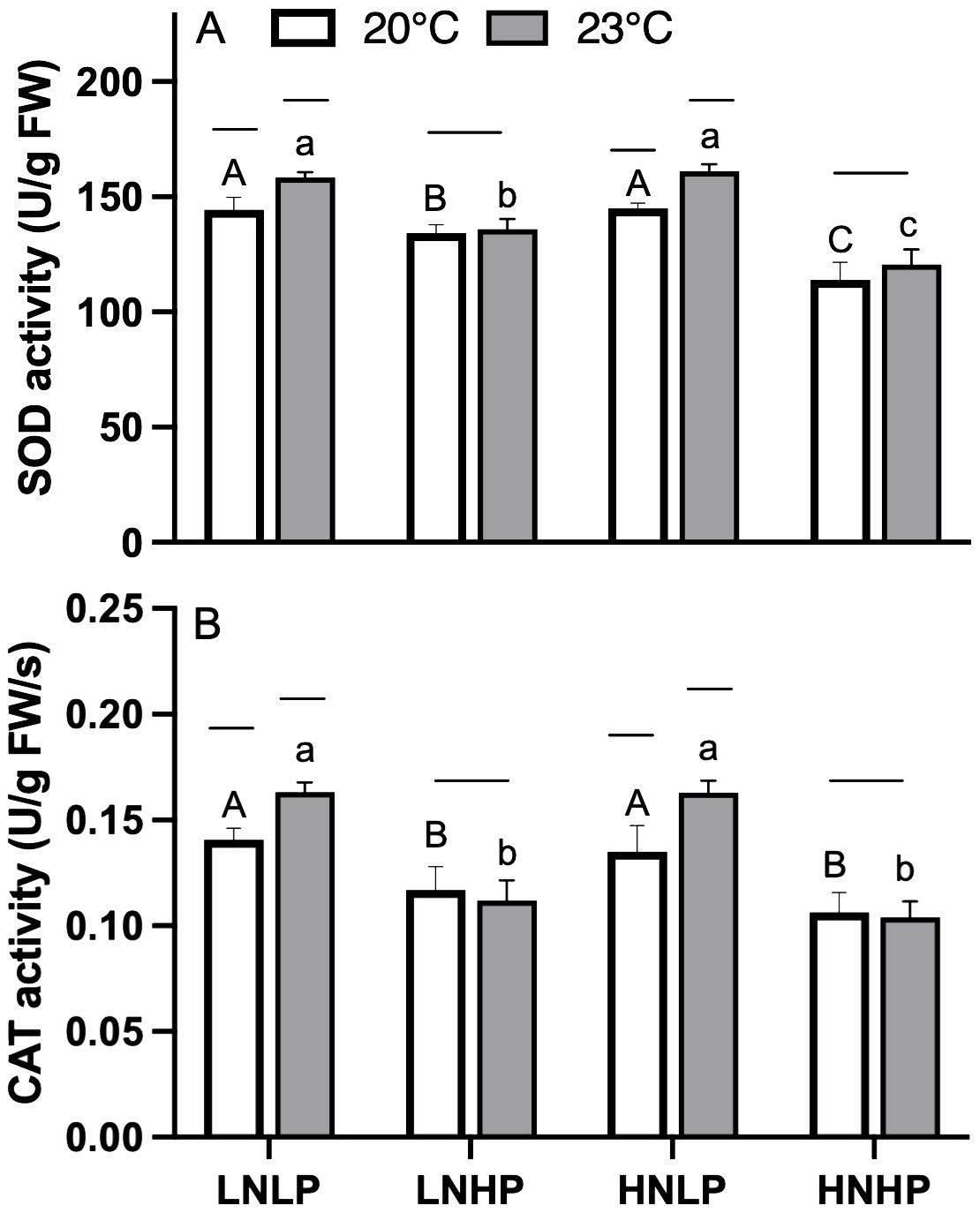
Figure 7. The SOD [U/g FW, panel (A)] and CAT [U/g FW, panel (B)] activities of Gracilariopsis lemaneiformis after growing for 10 days at four nutrients levels (LNLP, LNHP, HNLP and HNHP) and two temperature (20°C, open bars, 23°C, grey bars). Each data point is means ± SD (n=3). Different upper and lower letters above the bars indicate significant differences between nutrients levels under 20°C and 23°C, respectively (p<0.05, One-way ANOVA). Unconnected line above the bars indicate significant differences between temperature under each nutrients level (p<0.05, One-way ANOVA). SOD, superoxide dismutase; CAT, catalase; LNLP, low nitrogen and low phosphorus concentrations (N: 8μmol/L, P: 0.5 μmol/L); LNHP, low nitrogen and high phosphorus concentrations (N: 8μmol/L, P: 10 μmol/L); HNLP, high nitrogen and low phosphorus concentrations (N: 160μmol/L, P: 0.5 μmol/L); HNHP, high nitrogen and high phosphorus concentrations (N: 160μmol/L, P: 10 μmol/L); FW, fresh weight; SD, standard deviation.
Discussion
Effects of nutrients variations on Gracilariopsis lemaneiformis
Nitrogen and phosphorus are both involved the synthesis of amino acids and phycobilins, the transformation of enzymes, and the formation of germ cells in macroalgae, which are all necessary for growth (Zhou et al., 2024). In coastal areas, the drastic fluctuations of nutrients induced by human activities often expose macroalgae to an imbalance between nitrogen and phosphorus, which affects their growth and survival (Chu et al., 2019). In the present study, the enrichment of both PO43- and NO3- prompted the synthesis of Chl a and active photochemical efficiency, which led to an increase in the net photosynthesis rate of Gracilariopsis lemaneiformis and further resulted in a parallel increase in RGR. However, enrichment of either PO43- or NO3- alone did not enhance photosynthesis or growth. In the case of disturbed N:P, the lower phosphorus treatment (HNLP) exhibited a worse effect, showing lower values of RGR and net photosynthesis rate.
As essential nutrients, both nitrogen and phosphorus are not only involved in the formation of chloroplast DNA and RNA, but also highly necessary for the generation of ATP, the synthesis of phospholipids, and the phosphorylation of photosynthesis proteins (Scheerer et al., 2019). In our present study, the significant increase of Fv/Fm under the HNHP condition indicated efficient conversion of absorbed light into chemical energy, as shown by higher net photosynthesis rates. Conversely, the significant decline of Fv/Fm under the HNLP condition suggested an inhibition of photosynthesis, which could further lead to reduced production of ATP and NADPH. As two essential molecules that fuel the Calvin cycle, the diminished ability to fix carbon would naturally result in a lower net photosynthesis rate and RGR. Similar results were also reported in several other macroalgae (e.g. Sargassum muticum in Xu et al., 2017; Ulva linza in Gao et al., 2018; and Pyropia yezoensis in Kim et al., 2019). In addition to the limitation of carbon fixation, photoinhibition-induced lower generation of ATP would also inhibit the high turnover rate of D1 protein [the core protein of photosystem II (PSII)], which is the prerequisite for PSII to flexibly respond to environmental fluctuations (Powles, 1984; Long et al., 1994). To clarify the occurrence of P deficiency-induced photoinhibition, non-photochemical quenching, the most common and quickest photoprotection mechanism (Adams and Demmig-Adams, 1994; Ruban, 2016), and the rates of damage and repair of PSII, were measured. In G. lemaneiformis, a decrease in Fv/Fm was always accompanied by an increase in NPQ (Figure 3), indicating an increase in energy dissipation, especially under the HNLP condition. Specifically, the required light energy for G. lemaneiformis cultured in the HNLP condition should be much less than that of other treatments, which easily suffer from photoinhibition. In terms of the rates of damage and repair of PSII during photoinhibitory exposure, the higher value of k and the lower value of r suggested severe photoinhibition of PSII occurred in P deficiency treatments (Figure 5). These results confirmed that G. lemaneiformis was sensitive to P deficiency, which could induce significant photoinhibition by decreasing the P utilization in photophosphorylation (Scheerer et al., 2019; Zhou et al., 2024) and retarding the repair of D1 protein.
Once the thermal energy dissipation could not satisfy the energy balance between absorption and utilization, the excess excitation energy would result in the accumulation of ROS (Logan et al., 1998, 2006), which is known to induce oxidative stress and damage biomolecules such as pigments, proteins, and lipids in plants and algae (Miller et al., 2010; Suzuki et al., 2012; Nahar et al., 2015; Barati et al., 2019). In the present study, the production of ROS was highly consistent with the damage and repair rates of the PSII, with high content in P deficiency conditions (Figure 6). The antioxidant system that scavenges ROS has been previously reported as a second line of defense against photoinhibition-induced oxidative stress (Logan et al., 2006). Here, SOD and CAT, which are responsible for turning O2- into H2O2, and turning H2O2 to H2O, respectively, show similar trends to the production of ROS, with higher values in the P deficiency conditions. These results also confirmed that G. lemaneiformis was sensitive to P deficiency, which could induce severe oxidative stress by increasing photoinhibition risk.
Effects of warming on Gracilariopsis lemaneiformis
Generally, the elevated temperature could accelerate the growth of phytoplankton and macroalgae via upregulating the metabolic activity (Lund, 1949; Charan et al., 2017; Schaum et al., 2017; Wu et al., 2019). As mentioned above, the elevated temperature in this study was achieved by heating the water in the tank; therefore, the warming effect should be attributed to temperature and the tank. In G. lemaneiformis, both the net photosynthesis rate and growth under the HNHP treatment were increased by the elevated temperature. Similar results were also observed in Chaetomorpha linum and Gracilaria blodgettii, where their growth increased when the temperature rose from 20°C to 35°C within a phosphorus repletion condition (Zeng et al., 2020). In contrast, under P deficiency conditions, the higher temperature negatively affected both the net photosynthesis rate and growth in G. lemaneiformis. A plausible explanation for this is that the limited available P is prioritized for maintaining the basic functions of the cells and the high requirement of P (a high P uptake rate) induced by the high temperature could not be satisfied, resulting in a decrease in algal biomass (Talbot and De la Noüe, 1993; Mandal et al., 2015; Zhou et al., 2024). Additionally, the significant enhancement of k and decline of r at 23°C also implied that the elevated temperature increased the photoinhibition of G. lemaneiformis under P deficiency conditions. Together with the increase in ROS production (Figure 6) and antioxidant enzyme activity (Figure 7), our data demonstrated that future warming would exacerbate P deficiency-induced photoinhibition and oxidative stress.
Conclusion
Found in coastal areas, macroalgae are often exposed to drastic environmental fluctuations due to anthropogenic activities, including nutrient variations and seawater warming. The present study indicates that G. lemaneiformis is particularly susceptible to P deficiency, leading to significant photoinhibition and increased oxidative stress, ultimately reducing growth. Furthermore, projected seawater warming is likely to exacerbate the negative impacts of P deficiency, amplifying photoinhibition and oxidative stress.
Data availability statement
The raw data supporting the conclusions of this article will be made available by the authors, without undue reservation.
Author contributions
DZ: Conceptualization, Data curation, Formal analysis, Funding acquisition, Investigation, Methodology, Project administration, Writing – original draft, Writing – review & editing. J-ZS: Methodology, Writing – review & editing. M-HF: Investigation, Writing – review & editing. C-JL: Investigation, Writing – review & editing.
Funding
The author(s) declare financial support was received for the research, authorship, and/or publication of this article. This study was supported by the National Natural Science Youth Foundation (42306139) and the Natural Science Youth Foundation of Shandong Province (ZR2022QD134).
Conflict of interest
The authors declare that the research was conducted in the absence of any commercial or financial relationships that could be construed as a potential conflict of interest.
Publisher’s note
All claims expressed in this article are solely those of the authors and do not necessarily represent those of their affiliated organizations, or those of the publisher, the editors and the reviewers. Any product that may be evaluated in this article, or claim that may be made by its manufacturer, is not guaranteed or endorsed by the publisher.
References
Adams W. W. III, Demmig-Adams B. (1994). Carotenoid composition and down regulation of photosystem II in three conifer species during the winter. Physiol. Plant. 92, 451–458. doi: 10.1111/j.1399-3054.1994.tb08835.x
Barati B., Gan S. Y., Lim P. E., Beardall J., Phang S. M. (2019). Green algal molecular responses to temperature stress. Acta Physiol. Plant. 41, 1–19. doi: 10.1007/s11738-019-2813-1
Berges J. A., Franklin D. J., Harrison P. J. (2001). Evolution of an artificial seawater medium: improvements in enriched seawater, artificial water over the last two decades. J. phycol. 37, 1138–1145. doi: 10.1046/j.1529-8817.2001.01052.x
Brembu T., Mühlroth A., Alipanah L., Bones A. M. (2017). The effects of phosphorus limitation on carbon metabolism in diatoms. Philos. Trans. R. Soc. B: Biol. Sci. 372, 20160406.
Charan H., N’Yeurt A. D. R., Iese V., Chopin T. (2017). “The effect of temperature on the growth of two pest seaweeds in Fiji,” in 2nd International Conference on Energy, Environment and Climate-ICEECC, University of Mauritius, Jan 2017, Moka, Mauritius. hal-04581450
Chen B., Zou D., Du H., Ji Z. (2018). Carbon and nitrogen accumulation in the economic seaweed Gracilaria lemaneiformis affected by ocean acidification and increasing temperature. Aquaculture 482, 176–182. doi: 10.1016/j.aquaculture.2017.09.042
Chu Y. Y., Liu Y., Li J., Gong Q. (2019). Effects of elevated pCO2 and nutrient enrichment on the growth, photosynthesis, and biochemical compositions of the brown alga Saccharina japonica (Laminariaceae, Phaeophyta). PeerJ 7, e8040. doi: 10.7717/peerj.8040
Cohen F. P. A., Faria A. V. F., Braga E. S., Chiozzini V. G., Plastino E. M. (2022). Gracilarioid algae (Rhodophyta) cultured in eutrophic synthetic seawater: potential for growth and preliminary bioremediation assessment. J. Appl. Phycol. 34, 2783–2791. doi: 10.1007/s10811-022-02728-9
Compiled by Fisheries Bureau of Ministry of Agriculture. (2023). China Fishery Statistical Yearbook. China Agriculture, Beijing in Chinese.
Duarte L., Viejo R. M., Martínez B., deCastro M., Gómez-Gesteira M., Gallardo T. (2013). Recent and historical range shifts of two canopy-forming seaweeds in North Spain and the link with trends in sea surface temperature. Acta Oecol. 51, 1–10. doi: 10.1016/j.actao.2013.05.002
Falkowski P. G., Raven J. A. (2007). Aquatic Photosynthesis (Princeton, NJ: Princeton University Press).
Gao G., Beardall J., Bao M., Wang C., Ren W., Xu J. (2018). Ocean acidification and nutrient limitation synergistically reduce growth and photosynthetic performances of a green tide alga Ulva linza. Biogeosciences 15, 3409–3420. doi: 10.5194/bg-15-3409-2018
Heraud P., Beardall J. (2000). Changes in chlorophyll fluorescence during exposure of Dunaliella tertiolecta to UV radiation indicate a dynamic interaction between damage and repair processes. Photosynth. Res. 63, 123–134. doi: 10.1023/A:1006319802047
Ji Y., Gao K. (2021). Effects of climate change factors on marine macroalgae: A review. Adv. Mar. Biol. 88, 91–136. doi: 10.1016/bs.amb.2020.11.001
Jiang H., Liao X., Zou D., Huang B., Liu Z. (2020). The regulations of varied carbon-nitrogen supplies to physiology and amino acid contents in Gracilariopsis lemaneiformis (Gracilariales, Rhodophyta). Algal Res. 47, 101818. doi: 10.1016/j.algal.2020.101818
Jiang M., Gao L., Huang R., Lin X., Gao G. (2022). Differential responses of bloom-forming Ulva intestinalis and economically important Gracilariopsis lemaneiformis to marine heatwaves under changing nitrate conditions. Sci. Total Environ. 840, 156591. doi: 10.1016/j.scitotenv.2022.156591
Johnson C. R., Banks S. C., Barrett N. S., Cazassus F., Dunstan P. K., Edgar G. J., et al. (2011). Climate change cascades: Shifts in oceanography, species' ranges and subtidal marine community dynamics in eastern Tasmania. J. Exp. Mar. Biol. Ecol. 400, 17–32. doi: 10.1016/j.jembe.2011.02.032
Kim S., Yoon S. C., Yoo M. H., Park K. W., Park S. R., Youn S. H. (2019). Physiological responses of cultured seaweed Pyropia yezoensis to phosphorous limitation in the Nakdong river estuary, Korea. Ocean Sci. J. 54, 129–139. doi: 10.1007/s12601-018-0065-4
Kipp M. A., Stüeken E. E. (2017). Biomass recycling and Earth’s early phosphorus cycle. Sci. Adv. 3, eaao4795. doi: 10.1126/sciadv.aao4795
Kirihara S., Nakamura T., Kon N., Fujita D., Notoya M. (2006). Recent fluctuations in distribution and biomass of cold and warm temperature species of Laminarialean algae at Cape Ohma, northern Honshu, Japan. J. Appl. phycol. 18, 521–527. doi: 10.1007/s10811-006-9057-3
Li T., Wu J., Du H., Pei P., Yang C., Huang J., et al. (2022). Environmental nitrogen and phosphorus nutrient variability triggers intracellular resource reallocation in Gracilariopsis lemaneiformis (Rhodophyta). Algal Res. 66, 102778. doi: 10.1016/j.algal.2022.102778
Lin S., Litaker R. W., Sunda W. G. (2016). Phosphorus physiological ecology and molecular mechanisms in marine phytoplankton. J. Phycol. 52, 10–36. doi: 10.1111/jpy.12365
Liu C., Zou D., Liu Z., Ye C. (2020). Ocean warming alters the responses to eutrophication in a commercially farmed seaweed, Gracilariopsis lemaneiformis. Hydrobiologia 847, 879–893. doi: 10.1007/s10750-019-04148-2
Logan B. A., Demmig-Adams B., Adams W. W. III, Grace S. C. (1998). Antioxidants and xanthophyll cycle-dependent energy dissipation in Cucurbita pepo L. and Vinca major L. acclimated to four growth PPFDs in the field. J. Exp. Bot. 49, 1869–1879. doi: 10.1093/jxb/49.328.1869
Logan B. A., Kornyeyev D., Hardison J., Holaday A. S. (2006). The role of antioxidant enzymes in photoprotection. Photosynth. Res. 88, 119–132. doi: 10.1007/s11120-006-9043-2
Long S. P., Humphries S., Falkowski P. G. (1994). Photoinhibition of photosynthesis in nature. Annu. Rev. Plant Biol. 45, 633–662. doi: 10.1146/annurev.pp.45.060194.003221
Lund J. W. G. (1949). Studies on asterionella: I. @ the origin and nature of the cells producing seasonal maxima. J. Ecol. 37 (2), 389–419.
Mandal S. K., Ajay G., Monisha N., Malarvizhi J., Temkar G., Mantri V. A. (2015). Differential response of varying temperature and salinity regimes on nutrient uptake of drifting fragments of Kappaphycus alvarezii: implication on survival and growth. J. Appl. Phycol. 27, 1571–1581. doi: 10.1007/s10811-014-0469-1
Masson-Delmotte V., Zhai P., Pirani A., Connors S. L., Péan C., Chen Y., et al. (2021). IPCC 2021: climate change 2021: the physical science basis. contribution of working group I to the sixth assessment report of the intergovernmental panel on climate change (Cambridge: Cambridge University Press).
Miao H., Beardall J., Gao K. (2018). Calcification moderates the increased susceptibility to UV radiation of the coccolithophorid Gephryocapsa oceanica grown under elevated CO2 concentration: evidence based on calcified and non-calcified cells. Photochem. Photobiol. 94, 994–1002. doi: 10.1111/php.12928
Miller G. A. D., Suzuki N., Ciftci-Yilmaz S., Mittler R. O. N. (2010). Reactive oxygen species homeostasis and signalling during drought and salinity stresses. Plant Cell Environ. 33, 453–467. doi: 10.1111/j.1365-3040.2009.02041.x
Nahar K., Hasanuzzaman M., Alam M. M., Fujita M. (2015). Exogenous glutathione confers high temperature stress tolerance in mung bean (Vigna radiata L.) by modulating antioxidant defense and methylglyoxal detoxification system. Environ. Exp. Bot. 112, 44–54. doi: 10.1016/j.envexpbot.2014.12.001
Porra R. J. (2002). The chequered history of the development and use of simultaneous equations for the accurate determination of chlorophylls a and b. Photosynth. Res. 73, 149–156. doi: 10.1023/A:1020470224740
Pörtner H.-O., Roberts D. C., Masson-Delmotte V., Zhai P., Tignor M., Poloczanska E., et al. (2019). “IPCC special report on the ocean and cryosphere in a changing climate,” in IPCC intergovernmental panel on climate change, vol. 1. (Cambridge University Press, Cambridge, UK and New York, NY, USA).
Powles S. B. (1984). Photoinhibition of photosynthesis induced by visible light. Annu. Rev. Plant Physiol. 35, 15–44. doi: 10.1146/annurev.pp.35.060184.000311
Roleda M. Y., Hurd C. L. (2019). Seaweed nutrient physiology: application of concepts to aquaculture and bioremediation. Phycologia 58, 552–562. doi: 10.1080/00318884.2019.1622920
Ruban A. V. (2016). Nonphotochemical chlorophyll fluorescence quenching: mechanism and effectiveness in protecting plants from photodamage. Plant Physiol. 170, 1903–1916. doi: 10.1104/pp.15.01935
Schaum C. E., Barton S., Bestion E., Buckling A., Garcia-Carreras B., Lopez P., et al. (2017). Adaptation of phytoplankton to a decade of experimental warming linked to increased photosynthesis. Nat. Ecol. Evol. 1, 0094. doi: 10.1038/s41559-017-0094
Scheerer U., Trube N., Netzer F., Rennenberg H., Herschbach C. (2019). ATP as phosphorus and nitrogen source for nutrient uptake by Fagus sylvatica and Populus x canescens roots. Front. Plant Sci. 10, 416196. doi: 10.3389/fpls.2019.00378
Serisawa Y., Imoto Z., Ishikawa T., Ohno M. (2004). Decline of the Ecklonia cava population associated with increased seawater temperatures in Tosa Bay, southern Japan. Fish. Sci. 70, 189–191. doi: 10.1111/j.0919-9268.2004.00788.x
Suzuki N., Koussevitzky S. H. A. I., Mittler R. O. N., Miller G. A. D. (2012). ROS and redox signalling in the response of plants to abiotic stress. Plant Cell Environ. 35, 259–270. doi: 10.1111/j.1365-3040.2011.02336.x
Talbot P., De la Noüe J. (1993). Tertiary treatment of wastewater with Phormidium bohneri (Schmidle) under various light and temperature conditions. Water Res. 27, 153–159. doi: 10.1016/0043-1354(93)90206-W
Traugott H., Zollmann M., Cohen H., Chemodanov A., Liberzon A., Golberg A. (2020). Aeration and nitrogen modulated growth rate and chemical composition of green macroalgae Ulva sp. cultured in a photobioreactor. Algal Res. 47, 101808. doi: 10.1016/j.algal.2020.101808
Wu H., Feng J., Li X., Zhao C., Liu Y., Yu J., et al. (2019). Effects of increased CO2 and temperature on the physiological characteristics of the golden tide blooming macroalgae Sargassum horneri in the Yellow Sea, China. Mar. pollut. Bull. 146, 639–644. doi: 10.1016/j.marpolbul.2019.07.025
Xu Z., Gao G., Xu J., Wu H. (2017). Physiological response of a golden tide alga (Sargassum muticum) to the interaction of ocean acidification and phosphorus enrichment. Biogeosciences 14, 671–681. doi: 10.5194/bg-14-671-2017
Xu Z. G., Zou D. H., Gao K. S. (2010). Effects of elevated CO2 and phosphorus supply on growth, photosynthesis and nutrient uptake in the marine macroalga Gracilaria lemaneiformis (Rhodophyta). Botanica Marina 53, 123–129. doi: 10.1515/BOT.2010.012
Xue J., Pang T., Liu J. (2022). Changes in the temperature tolerance profile of Gracilariopsis lemaneiformis from the perspective of photosynthesis, respiration, and biochemical markers after many years of vegetative propagation. J. Appl. Phycol. 34, 1045–1058. doi: 10.1007/s10811-022-02687-1
Yang Y., Li W., Li Y., Xu N. (2021). Photophysiological responses of the marine macroalga Gracilariopsis lemaneiformis to ocean acidification and warming. Mar. Environ. Res. 163, 105204. doi: 10.1016/j.marenvres.2020.105204
Ye C. P., Zhang M. C., Zhao J. G., Yang Y. F., Zuo Y. (2013). Photosynthetic response of the macroalga, Gracilaria lemaneiformis (Rhodophyta), to various N and P levels at different temperatures. Int. Rev. hydrobiol. 98, 245–252. doi: 10.1002/iroh.201301435
Zeng J., Wu X., Liao X., Yang S., Huang M., Tang X. (2020). Effect of temperature on the uptake capacities of five large seaweeds for nitrogen and phosphorus. Chin. Fish Qual Standards 10, 31–37.
Zhang D., Beer S., Li H., Gao K. (2020a). Photosystems I and II in Ulva lactuca are well protected from high incident sunlight. Algal Res. 52, 102094. doi: 10.1016/j.algal.2020.102094
Zhang D., Xu J., Bao M., Yan D., Beer S., Beardall J., et al. (2020b). Elevated CO2 concentration alleviates UVR-induced inhibition of photosynthetic light reactions and growth in an intertidal red macroalga. J. Photochem. Photobiol. B: Biol. 213, 112074. doi: 10.1016/j.jphotobiol.2020.112074
Zhou W., Wu H., Huang J., Wang J., Zhen W., Wang J., et al. (2022). Elevated-CO2 and nutrient limitation synergistically reduce the growth and photosynthetic performances of a commercial macroalga Gracilariopsis lemaneiformis. Aquaculture 550, 737878. doi: 10.1016/j.aquaculture.2021.737878
Keywords: antioxidant enzymes, Gracilariopsis lemaneiformis, nutrient variations, ocean warming, photosynthesis
Citation: Zhang D, Sun J-Z, Fu M-H and Li C-J (2024) Photosynthetic performance and antioxidant activity of Gracilariopsis lemaneiformis are sensitive to phosphorus deficiency in elevated temperatures. Front. Mar. Sci. 11:1432937. doi: 10.3389/fmars.2024.1432937
Received: 15 May 2024; Accepted: 26 July 2024;
Published: 22 August 2024.
Edited by:
Christopher Edward Cornwall, Victoria University of Wellington, New ZealandReviewed by:
Wei Liu, Shanghai University, ChinaFangfang Yang, Chinese Academy of Sciences (CAS), China
Ralf Rautenberger, Norwegian Institute of Bioeconomy Research (NIBIO), Norway
Copyright © 2024 Zhang, Sun, Fu and Li. This is an open-access article distributed under the terms of the Creative Commons Attribution License (CC BY). The use, distribution or reproduction in other forums is permitted, provided the original author(s) and the copyright owner(s) are credited and that the original publication in this journal is cited, in accordance with accepted academic practice. No use, distribution or reproduction is permitted which does not comply with these terms.
*Correspondence: Di Zhang, zhangdi@ytu.edu.cn