- 1State Key Laboratory of Estuarine and Coastal Research, East China Normal University, Shanghai, China
- 2Laboratory for Marine Geology, Qingdao Marine Science and Technology Center, Qingdao, China
The sedimentary total organic carbon (TOC) in mangrove ecosystems plays an essential role in the global carbon storage. Nevertheless, little information is available about the pattern of TOC in sediments varying from bare and flat to those beneath mangroves. To find out the roles of new-developing mangroves in sedimentary TOC accumulation, a serials of sediment samples were collected from the creek mudflat zone (CMZ) through the fringe mangrove zone (FMZ) to the interior mangrove zone (IMZ) in young mangrove system of Nanliu River Delta in China. Sediment compositions, TOC, total nitrogen (TN), molar C/N ratios, and carbon stable isotopes (δ13C) were analyzed to examine the accumulation processes. The results revealed the distinct differences in the sedimentary TOC values, with an obvious increasing trend from the CMZ and FMZ to the IMZ. We quantified that terrestrials, marine-derived and mangrove-derived sources contributed 39.2-74.1%, 24.7-63.1% and 0.9-6.9%, respectively, to the sedimentary TOC in the mangrove ecosystems. The organic carbon accumulation rates ranged from 2.59 to 269.60 g•m-2•a-1, with values of 8.77 ± 19.87, 24.78 ± 12.53, 167.19 ± 57.79 g•m-2•a-1 for CMZ, FMZ and IMZ. Our work highlights information showing that young mangrove forests of the tropical delta have important potential for carbon storage.
1 Introduction
Mangroves are increasingly seen as carbon-rich (i.e., blue carbon) ecosystems, because of the capacity to deposit and store carbon at a rather higher rate than those in terrestrial forests by 1-2 orders of magnitude (Wang et al., 2019; Matos et al., 2020). Although mangroves represent less than1% of the global coastal area, their productivity is high, contributing 10-15% of the total carbon accumulation in coastal sediments (Bergamaschi et al., 2012; Alongi, 2014). Therefore, mangrove ecosystem plays a disproportionate role in global and local carbon budgets.
Most studies indicate that mangroves have been declining at an accelerating rate mostly because of deforestation and land usage change (Zhong and Xu, 2011; Duarte et al., 2013; Serrano et al., 2019). These losses led to the degradation of tree biomass, ecological habitats and carbon deposit. In particular, degradation intensifies the decomposition of stored carbon, emits quantities of greenhouse gases into the ambient environment (Alongi, 2014; Zhu and Yan, 2022). To compensate for the loss of mangrove degradation, restoration projects have been implemented to increase mangrove areas and the carbon sequestration capacity. These projects are important for alleviating local and global climate change (Alongi, 2014; Feng et al., 2019; Zhu and Yan, 2022).
Due to rapid sediment accretion in mangroves, high autochthonous and allochthonous inputs, and low decomposition rates of organic matter under anoxic conditions, mangroves sedimentary carbon pools are much more effective than aboveground and belowground plant biomass (Donato et al., 2011; Breithaupt et al., 2014). The global average carbon accumulation rate of mangrove sediments was around 220 g•m-2•a-1 (Twilley et al., 2018). While the rates for Americas and Oceania were slightly lower than the global average, Africa was at minimum and Asia was higher than others (Jennerjahn, 2021). However, considering the large differences between the environmental conditions of mangrove ecosystems, there are large uncertainties in their sedimentary carbon storage (Kusumaningtyas et al., 2019; Fest et al., 2022). The within system variability of mangroves in the tropical delta can be very high due to the large variability in features on small spatial scales (Hinrichs et al., 2009; Woodroffe et al., 2016). Especially, the organic carbon accumulation rates can be distinguished by different sources and decomposition rates for different zones in mangrove ecosystem, since sedimentary TOC sources is different between interior mangrove area and bare zone (Tue et al., 2011). To better understand the spatial distribution of sedimentary carbon storage in mangrove ecosystem, a representative set of samples covering the within system scale variability of tropical delta is urgently needed.
Furthermore, the sedimentary total organic carbon (TOC) in mangrove can originate from autochthonous and allochthonous sources (Alongi, 2014). Generally, the major source of sedimentary organic carbon in mangroves are associated with mangrove materials (Adame and Fry, 2016; Medina-Contreras et al., 2023). The contribution of allochthonous sources (terrestrial or marine) may be higher in river- or tide-dominated mangrove forests, mainly from riverine sediment and marine plankton (Bouillon et al., 2003; Kristensen et al., 2008; Zakaria et al., 2021). Identifying different organic sources is of vital importance to evaluate the significance of the “blue carbon” sink in mangroves, because each source contributes differently to the carbon sequestration. TOC to total nitrogen (TN) ratios (C/N) both with isotopic signatures (δ13C) have been widely used as effective geochemical tracers to identify organic matter sources in estuarine and coastal environment (Ranjan et al., 2011; Manju et al., 2016; Zhao et al., 2019; Sánchez and Gómez-León, 2024). The δ13C of terrestrial organic matter usually shows more depletion values (< -28‰), meanwhile marine-derived values typically vary from -22‰ to -19‰ (Ke et al., 2017). The values of C/N for terrestrial and marine-derived sources are 5-8 and > 15, respectively (Meyers, 1997). In this research, application of the stable isotope technique distinguished the contributions of different organic sources to sedimentary TOC in mangrove ecosystems.
The mangrove forest areas in the Beibu Gulf are the second largest mangrove forest in China (Long et al., 2022). To date, little is known about the variations in sedimentary TOC of new-developing mangrove ecosystem along this area. Therefore, the aims of this research were (i) to investigate the spatial distribution of sedimentary TOC within the new-developing mangrove ecosystems and (ii) to analyze different sources of sedimentary TOC in the mangrove ecosystems. By identifying the spatial variation and sources of organic carbon in different zones of young mangrove ecosystem, it will enhance the understanding of carbon storage in this region. This work thereof can have significant implications for restoring TOC in mangrove ecosystems of global tropical deltas.
2 Materials and methods
2.1 Study area
The Nanliu River is the largest south subtropical river in Southwest China, with a total length of 287 km. Covering 550 km2, the Nanliu River Delta is the largest tropical delta in the Beibu Gulf (Figure 1). The annual displacement and sediment discharge were 5.13×109 m3 and 1.18×106 t, respectively (Li et al., 2017). The delta is a tidal-dominated, and the hydrodynamics of the study area are mainly controlled by waves (Long et al., 2022). The selected mangrove area is located on Qixing Island, which is the largest fluvial island of the Nanliu estuary (Figure 1). Mangrove species such as Aegiceras corniculatum (L) Blano and Kandelia candel (Linn.) are widely distributed, mostly within the five-year growth period in this region.
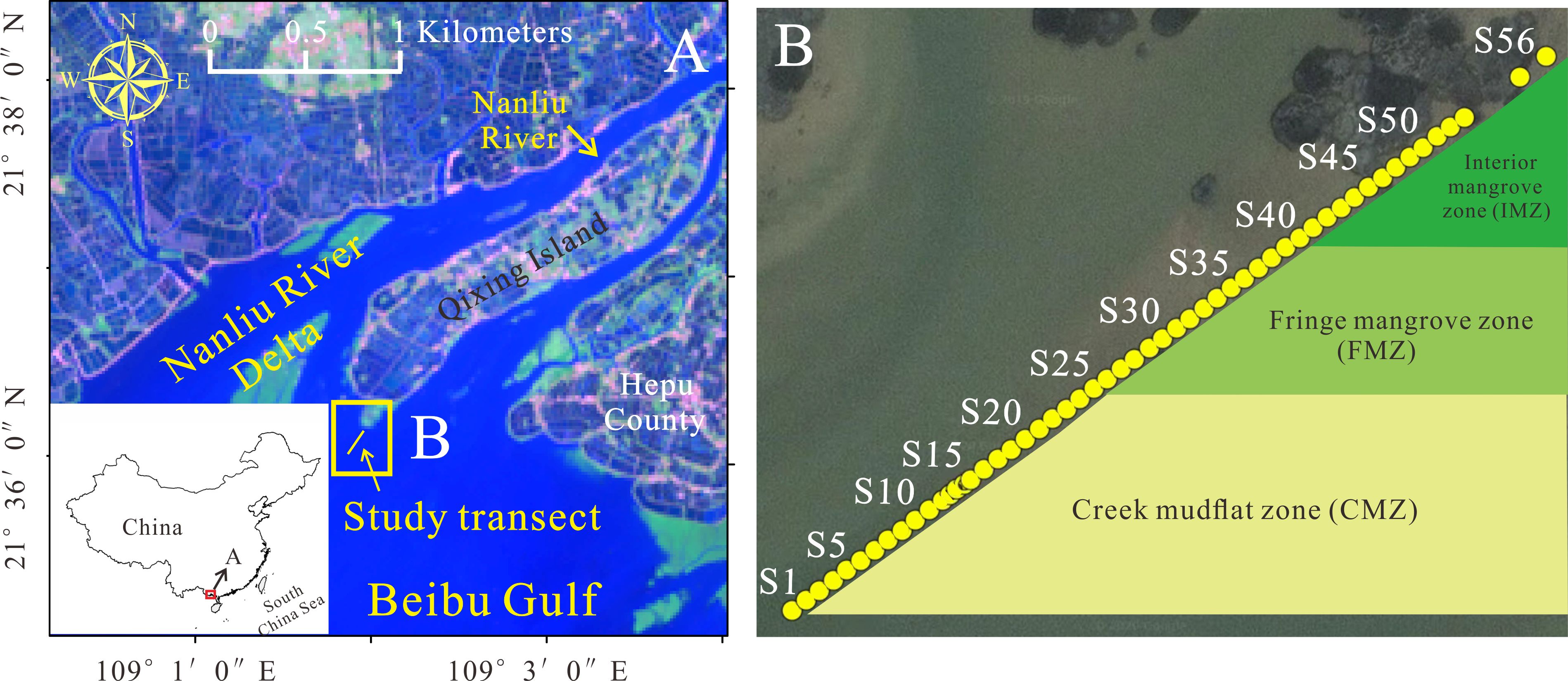
Figure 1 Study area and sampling sites; (A) Beibu Gulf; (B) Nanliu River Delta and locations of sampling sites.
2.2 Field sampling
Samples were collected in July 2020, and transect was set as the shortest distance between the intertidal creek and land. This transect was about 600 m long and consisted of a creek mudflat zone (CMZ, S1-S25), a fringe mangrove zone (FMZ, S26-S40), and an interior mangrove zone (IMZ, S41-S56) to better understand the spatial variations in TOC in mangrove sediments. Sample collection was conducted at the lowest tide level on 22 July. Fifty-six sediment samples (0-3 cm) were collected with approximately 10 m intervals, with approximately 20 m collected at several sites. The locations are shown in Figure 1. Surface sediments were packaged in the labeled polyethylene sealed bags, transported to the lab and then stored at -20°C before analysis. Meanwhile, samples from different zones were collected to analyze the bulk density. Elevation data were collected monthly to calculate the sediment deposition rate.
2.3 Sample processes and analyses
Frozen surface samples were first thawed at room temperature and completely dried with electric oven at 45°C.
2.3.1 Sediment grain size
Each sediment sample was pretreated with 5 mL H2O2 (10%) and 5 mL HCl (10%) sequentially to remove the organic matter and carbonate and then washed with deionized water, followed by the addition of 5 ml 5% (NaPO3)6 to prevent flocculation. After 15 min of ultrasonic vibration, the grain size of the surface sediment sample could be determined by a Beckman laser particle size analyzer (LS13320, Coulter). Glass beads particle size standard material (Chinese Standard Material GBW(E) 120009d) was selected to determine the accuracy. The precision was lower than 0.3%.
2.3.2 Elemental and stable isotope analysis
Samples were ground to sieve an 80-mesh nylon screen for elemental and stable isotope analysis. The TOC and δ13C were analyzed after removing carbonate carbon by acidification with HCl (0.1 M), washed in deionized water, and subsequently dried at 65°C. Total nitrogen (TN) was analyzed in non-acidified subsamples. The TN and TOC concentrations were analyzed by an elemental analyzer (TOC-V, Shimadzu). Standard materials (TMQC0177 and RMH-A073) were used to measure the accuracy of TOC and TN, with the precision of 0.3% and 0.3%, respectively.
The organic carbon accumulation rates (OCAR) were calculated by multiplying TOC content, bulk density with annual deposition rate of the sediment.
The stable isotope δ13C was measured by stable isotope mass spectrometry (Delta Plus XP, Thermo). The accuracy of δ13C was determined using a variety of standard materials including Caffeine (IAEA-600), Cellulose (IAEA-CH-3), Ammonium Sulfate (IAEA N2) and Black Carbon (the Chinese Standard Material GBW04407 and GBW04408), with a precision of 0.1‰. δ13C isotopes deviated from the standard value by the following:
Where Rsample was the isotope ratio (13C/12C) of the sample, and Rstandard was the isotope ratio (13C/12C) of the international VPDB standard.
2.3.3 Stable isotope mixing model
Bayesian isotopic modeling package Stable Isotope Mixing Model in R (SIMMR) based on the C/N and δ13C features were performed to quantitatively calculate the proportional contributions of potential organic carbon sources to deposited TOC (Parnell et al., 2010; Watanabe and Kuwae, 2015). Given that early degradation of the sedimentary organic carbon did not significantly alter the composition of stable isotope, we assumed isotopic fractionation and concentration dependency of 0. We ran the model 1×104 iterations, with the results performed by a box plot describing the 50% confidence interval.
2.4 Statistical analysis
Different sources of organic carbon were identified by utilizing a combination of atomic C/N ratios and the stable isotope δ13C. All statistical analyses were performed with SPSS software (Ver 23.0), Origin software (Ver 9.1) and R programming (Ver 4.2.0). One-way ANOVA was employed to test the significance differences of sediment composition, elemental and stable isotopic composition in different zones. Principal component analysis (PCA) was used to investigate the relationships between geochemical parameters.
3 Results
3.1 Sediment composition
A plot of the percentages of sediment composition on a ternary diagram is shown in Supplementary Figure S1. Mangrove surface sediments were composed of sand, ranging from 46.66% to 98.65% (Figure 2). Meanwhile, the silt fraction ranged from 1.25% to 43.29%, with a clay fraction of less than 13.75%. The sand fraction of CMZ (91.18 ± 6.2%) was highest but markedly dropped to 64.37 ± 11.28% in IMZ (p<0.01), as shown in Table 1. The median grain size of the sediment varied similarly to this variation pattern (p<0.01). To contrast, the fine fraction (<63 μm) displayed an inverse trend, increasing as follows: CMZ, FMZ and IMZ. The sediment grain sizes of the different zones varied widely, with respective values of 265.62 ± 149.58, 172.93 ± 44.58 and 110.24 ± 56.13 μm for the median size in CMZ, FMZ and IMZ.
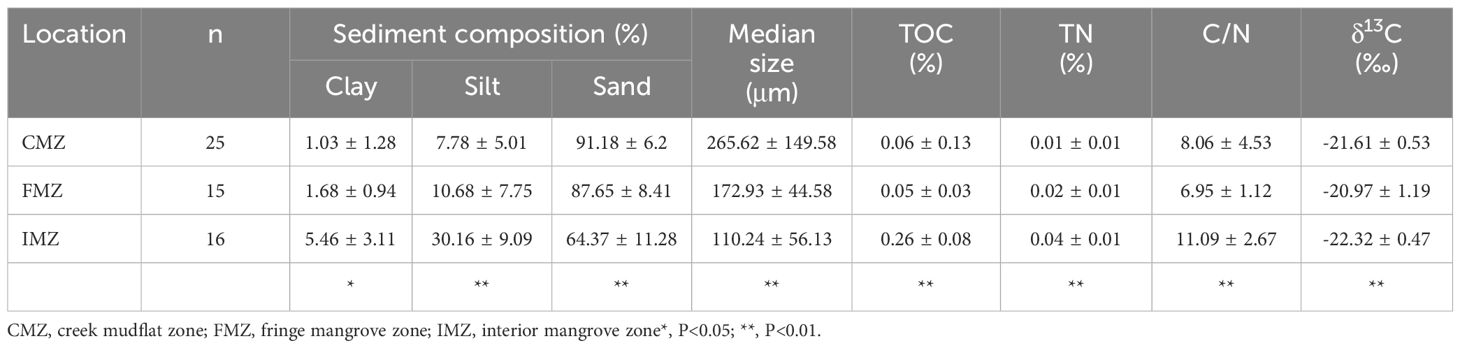
Table 1 Sediment composition, median size, elemental TOC, TN, atomic C/N, and δ13C of the sediments from different zones in the Nanliu Delta mangrove ecosystem.
3.2 Elemental and stable isotope composition
The contents and within system spatial variations in elemental and stable isotope compositions in the mangrove sediments are displayed in Table 1; Figure 3. Overall, the TOC values ranged from 0.02 to 0.66%. The mean TOC value of the IMZ was significantly higher than those in the CMZ and FMZ, with values of 0.26 ± 0.08%, 0.06 ± 0.13% and 0.05 ± 0.03%, respectively (p<0.01). There was no significant difference between the CMZ and FMZ. The TN contents ranged from 0.006 to 0.065%, which slightly increased from 0.01 ± 0.01% to 0.02 ± 0.01% for CMZ and FMZ, but the values in IMZ (0.04 ± 0.01%) were significantly higher (p<0,01). The within-system δ13C values varied from -23.66‰ to -19.95‰, with significant differences between different zones. The organic carbon accumulation rates ranged from 2.59 to 269.60 g•m-2•a-1, with values of 8.77 ± 19.87, 24.78 ± 12.53, 167.19 ± 57.79 g•m-2•a-1 for CMZ, FMZ and IMZ.
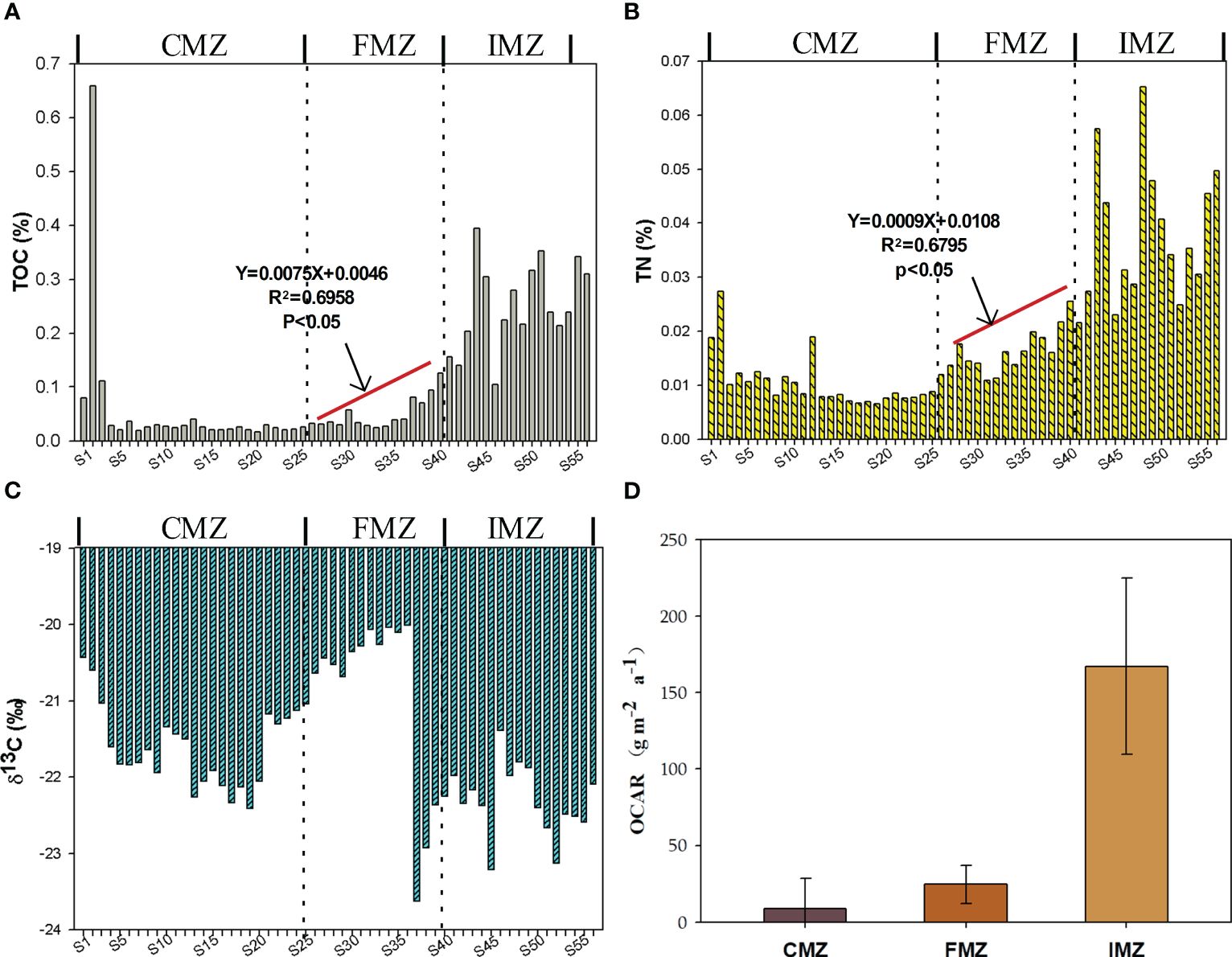
Figure 3 The within-system spatial variations in elemental and isotopic composition for sediments: (A) TOC (%); (B) TN (%); (C) δ13C (‰); (D) The organic carbon accumulation rates (g•m-2•a-1).
The significant component loading matrix of principal component analysis (PCA) is listed in Supplementary Table S1. The first two components accounted for 83.82% of the data variation (Figure 4). The first component (PC1) illustrated 71.03%, which showed a significant positive loading for clay, silt, TN and TOC, with a negative loading for sand. These results also supplied strong evidence for the increase of TOC and TN with the increase of fine fractions. The second component (PC2) accounted for12.59%, showing significant positive load for δ13C.
3.3 Contributions of sedimentary organic carbon sources
Sedimentary δ13C values varied narrowly from -23.66‰ to -19.95‰, with C/N values ranging from 5.43 to 28.01 (Figure 5). Three sources (terrestrial organic, marine derived organic and mangrove derived organic) were selected as endmembers for the mixing model. The signatures of endmembers were obtained from region-relevant literature. The terrestrial organic and marine derived organic sources were demonstrated by riverine sediments flowing into the Beibu Gulf (C/N = 12.6 ± 1.9 andδ13C = (-24.3 ± 0.6) ‰) and phytoplankton in the South China Sea (C/N = 6.5 ± 0.7 andδ13C = (-16.1 ± 0.8) ‰) (Xue et al., 2009; Zhang et al., 2021).The contents of C/N andδ13Cwere usually consistent between mangrove litter leaves and dead roots (Kusumaningtyas et al., 2019; Sasmito et al., 2020). Thus, the mangrove endmember was regarded by the leaves of different mangrove species nearby the coastal areas (C/N = 36.4 ± 7.3 andδ13C = (-28.7 ± 0.8) ‰) (Xia et al., 2015; Meng et al., 2017).
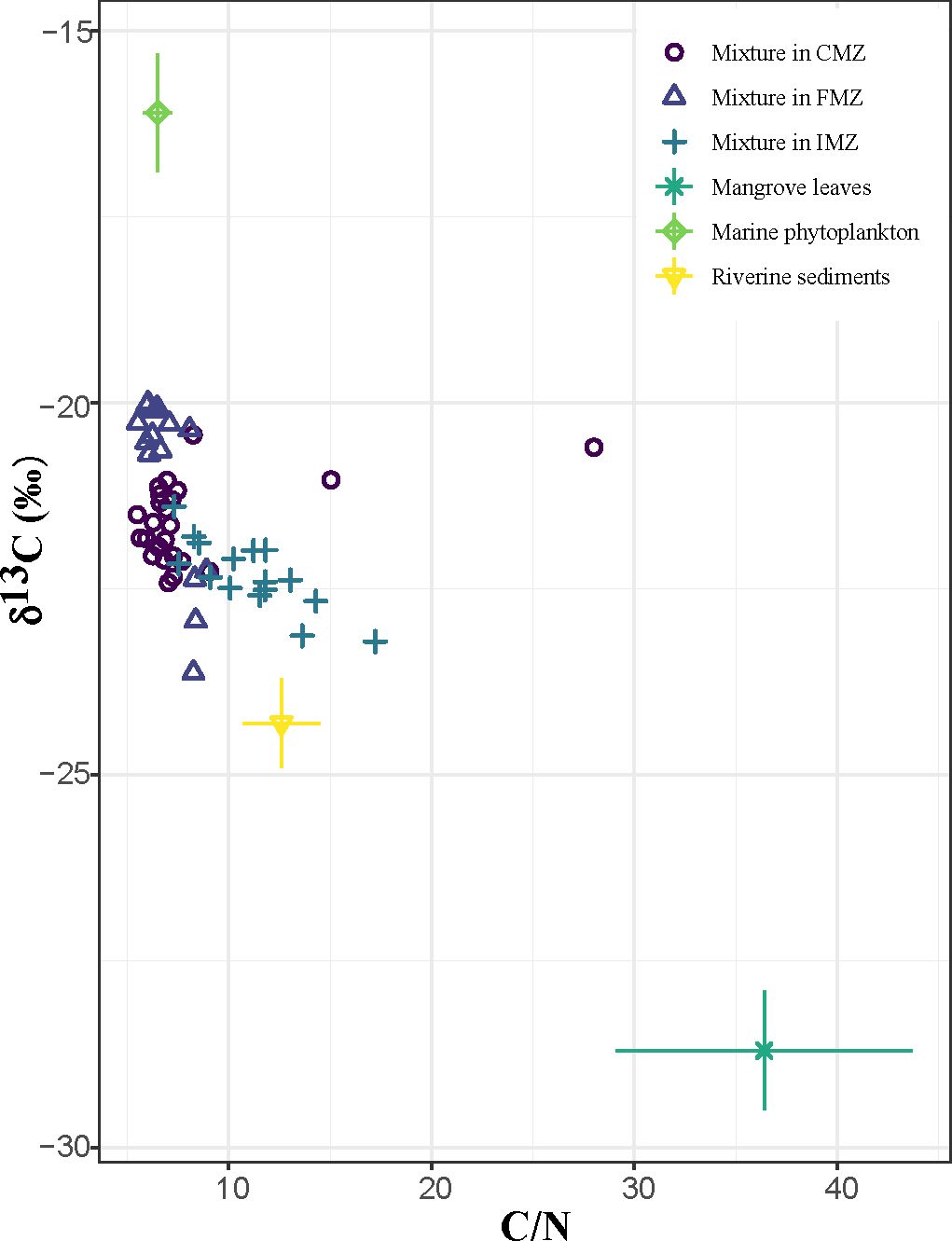
Figure 5 Tracer plot of δ13C and C/N (molar ratio) of sediments from the Nanliu River Delta and potential endmembers.
Most sediment samples were located in the area connecting marine phytoplankton and riverine sediments, indicating most mixtures of these two endmembers. Variations inδ13C and TOC were observed among the three zones (Table 1). Therefore, the contributions of different endmembers in the CMZ, FMZ and IMZ were estimated, as shown in Figure 6. The contribution proportion of riverine sediments ranged from 39.2% to 74.1%, with values of 61.3 ± 3.8%, 43.7 ± 16.1% and 68.9 ± 4.1% for the CMZ, FMZ and IMZ, respectively. The values of marine phytoplankton were 35.1 ± 1.8%, 53.5 ± 16.3% and 26.8 ± 2.1% (ranging from 24.7% to 63.1%). The contribution of TOC from mangrove leaves was quite small, with values from 0.9% to 6.9%. TOC from riverine sediments accounts for many more fractions of TOC in both the CMZ and IMZ. However, the fractions of marine phytoplankton were quite equal to the part of riverine sediments in the FMZ.
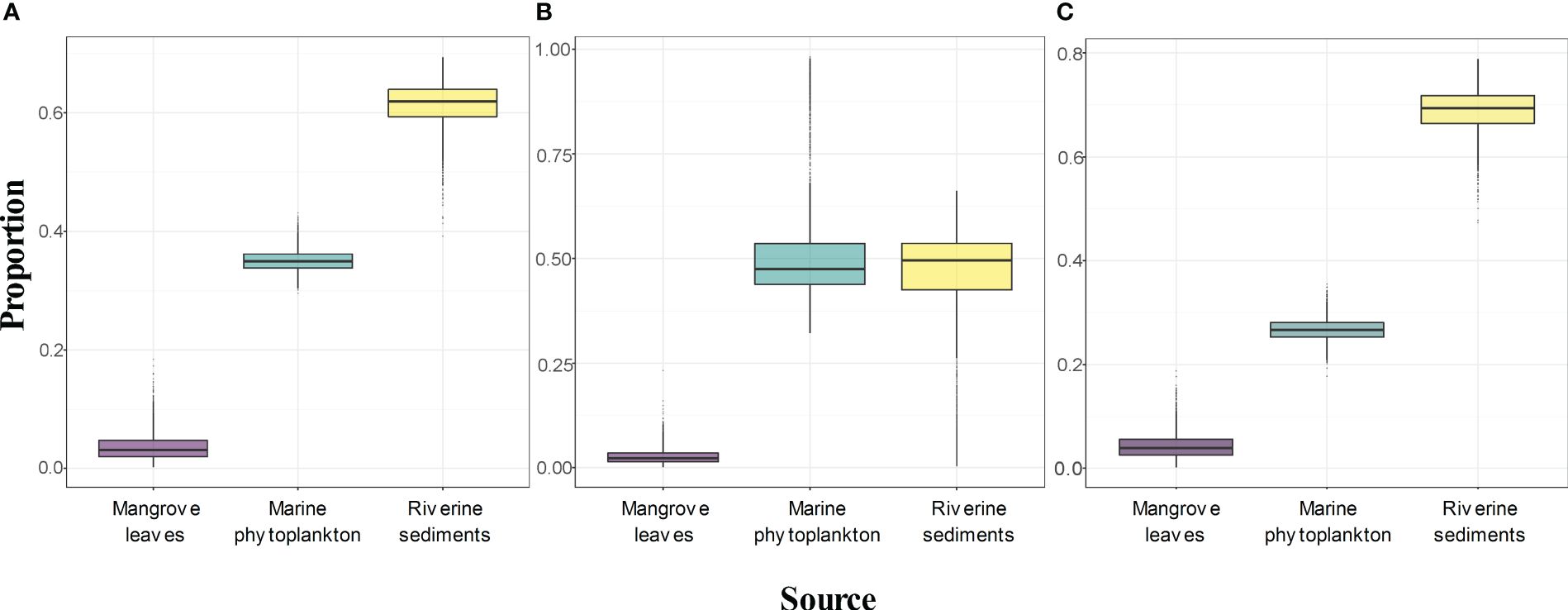
Figure 6 Variations in the contributions of different endmembers to sedimentary TOC by SIMMR: (A) CMZ; (B) FMZ; (C) IMZ. (Boxes enclose the 50% credibility interval; lines within boxes represent median values).
4 Discussion
4.1 Effects of mangrove on sediment grain size
The mangroves sediment composition can provide useful information to understand the effects of sedimentation processes of fluvial and marine (Yang et al., 2014; Tue et al., 2018). The mangroves sediment was mainly composed of sand and silt. It is consistent with the finding from the Marapanim River estuary, where sediments consist of sand (12-64%) and silt (34-80%) (Matos et al., 2020). Additionally, the results were similar to the observations from the Tien Yen Bay mangrove forests (Tue et al., 2018). The pattern of sediment composition does not fit with the reports of mangroves from the Gulf of Papua, the Red River Delta, Vietnam and Jiulongjiang Estuary, China (Walsh and Nittrouer, 2004; Alongi et al., 2005; Tue et al., 2012). The higher fractions of sand and silt in this study reflected a high energetic environment influenced by wave and fluvial processes (Long et al., 2022).
Mangrove ecosystems play a crucial role in sedimentary composition in coastal environments (Furukawa and Wolanski, 1996). The dense mangrove trees can effectively reduce wave energy and tidal flows and capture silt and clay. Low hydrodynamics in mangrove creeks may cause a higher fraction of fine sediments. Research has described that tidal currents decrease from bare flats through pioneer vegetation to interior mangroves (Van Santen et al., 2007). Because of the hydrodynamic attenuation and the ability of vegetation roots to combine sediments, fine fractions can accumulate in the interior mangrove. The fine fractions increased from the CMZ through the FMZ and to the IMZ, which indicated that mangrove ecosystems enhanced the accumulation of fine sediment grains. However, the ages of the native mangrove species of Aegiceras corniculatum in this study were less than 5 years, less able to capture more fine particles. This may be one reason why the fine fraction in the IMZ was lower than those in other researches (Xue et al., 2009; Zakaria et al., 2021; Zhang et al., 2021).
4.2 Impacts of ecosystem on sedimentary organic carbon storage
The sedimentary TOC and TN contents in this study were lower than those found in adjacent coastal sediments (Kauffman et al., 2018; Vilhena et al., 2018; Jiang et al., 2020).The highest value was surprisingly found in S2 from CMZ, which was significantly different from the values of ambient sites. The CZ is affected by the tidal scouring process, and phytoplankton may be washed into the area at high tide and remained at low tide. This may cause the extremely high TOC content in S2. Considering the strong hydrodynamic effects and high rate of sediment deposition in this mangrove ecosystem, the carbon storage capacity cannot be underestimated (Long et al., 2022). The organic carbon accumulation rates of IMZ (167.19 ± 57.79 g•m-2•a-1) were 3-5 times higher than the freshwater lake, while they were slightly below the global mangrove average rate (Jennerjahn, 2021; Mei et al., 2024). The carbon accumulation rates were related to continent, biogeographic region and coastal settings. The accumulation rates was estimated at 38 ± 13.5 g•m-2•a-1 in a macro-tidal mangrove forest on the Amazon coast (Passos et al., 2023). Liu et al. (2020) reported that the accumulation rate of burial organic carbon was estimated at 130 g•m-2•a-1 in the lower layer of mangrove with 12 years in Nanliu River estuary. Thereafter, even though the mangrove ecosystem is new-developing in this study, the carbon accumulation rate cannot be ignored.
The TOC and TN concentrations increased from the CMZ and FMZ to the IMZ (Figure 3), which may be directly influenced by fine fractions (clay and silt). This result suggested the capture ability for carbon and nutrient nitrogen together with the fine fractions of the mangrove ecosystem. The relationship among sediment composition, elemental and stable isotope composition is shown in Supplementary Figure S2. The TOC and TN contents increased as the fine fractions increased. This positive correlation pattern may be caused by the large surface areas and high adsorption capacity of the fine fractions (Loring and Rantala, 1992; Kumar et al., 2016). Obviously, there was a strong negative correlation between these contents and the sand fraction, indicating poor accumulation of this matter in the coarser fraction. The significant positive correlation between TOC and TN (p<0.001) suggested the probable same origin of these matter.
There was an inverse relationship between sedimentary TOC and δ13C, same with findings of other sediment cores in mangroves and other organic carbon-rich sediments, which presented quietly different from sediments of the oxygen minimum zone of the northeastern Mexican Pacific (Bouillon and Boschker, 2006; Sánchez et al., 2013; Zhang et al., 2021). The shift in relationship between sedimentary TOC and δ13C may be caused by the microbial mechanisms and the different organic carbon sources. Due to the very early development stage of mangroves and most creek mudflat areas, the degradation of organic carbon could be neglected. The TOC and δ13C relationship mostly resulted from different mixtures of organic carbon sources.
4.3 Sources of sedimentary organic carbon
Sedimentary organic carbon from different sources usually demonstrate different elemental and stable isotope characteristics, providing clues on the origins of the organic carbon pool (Bouillon et al., 2008; Sasmito et al., 2020). The δ13C versus C/N values indicated mixed sources of the sedimentary organic carbon. As seen in Figure 6, sedimentary TOC mostly originated from marine phytoplankton and riverine sediments. Furthermore, the contribution of riverine sediments is higher than that of marine-derived organic carbon at most sites, which is 2.5-fold higher in the IMZ. The hydrogeomorphic process could influence the differences in sedimentary TOC (Saintilan et al., 2013). When the tide transports riverine sediment to the estuary, the air-exposed roots attenuate tide velocity by increasing friction. Thus, the velocity of ebb tide carrying riverine sediments is slowed, and then the sediment is deposited and stored, known as the “tidal pumping” effect (Woodroffe et al., 2016). This may be responsible for the large contribution of riverine sediments, which could be regarded as the terrigenous organic carbon source.
This result is similar to those of other studies, which showed a larger proportion of marine derived organic carbon source (Kennedy et al., 2004; Tue et al., 2012, Tue et al., 2018). Marchand et al (Marchand et al., 2005). found that algal material occupied a large part of sedimentary TOC, especially in the very early development stages of mangrove forests. Research has found that contribution of marine described organic carbon to the sedimentary TOC gradually decreased from the subtidal habitat to the bottoms and banks of creeks (Matos et al., 2020). This spatial pattern was not obvious in this study, perhaps caused by the high energetic environment and the short sampling distance.
Meanwhile, the growth period of the mangroves in this study was less than 5 years. The forest is not as dense and lush as other mature mangroves. This resulted in a low contribution (0.9%–6.9%) of mangrove leaves to sedimentary TOC. Conversely, research found that natural mature mangrove forests contributed more than 80% of sedimentary organic carbon in the mangrove ecosystems (Tue et al., 2012). Similarly, Zhang et al. found that the average contribution of mangrove-derived organic carbon was more than 68.5% (Zhang et al., 2021). The large discrepancy between previous studies and present results might be caused by several factors, including hydrodynamic conditions, geomorphological settings, mangrove density and growth stage (Chen et al., 2017). Furthermore, previous work also found that the short-term restoration of mangroves (less than two decades) will markedly increase the sediment carbon reserves (Feng et al., 2019). Therefore, the low contribution of mangrove forests to sedimentary TOC in this study confirms the potential of carbon storage of immature mangroves in the future. Even though the mangrove ecosystem in this study was new-developing, it also played an important role in carbon sequestration. Furtherly, while the amount of carbon sequestration of the mature mangroves is well known, the high carbon accumulation rate of young mangroves should not be ignored. It means that cultivation of young mangroves is very important to conserve and restore the mangrove ecosystem.
5 Conclusions
To better understand the spatial pattern of sedimentary organic carbon in mangrove ecosystems, a within system transect was selected to obtain a representative set of samples from developing immature mangroves in the tropical Nanliu River Delta. The majority fraction of mangrove sediment was sand and silt, reflecting a high energetic environment. There were significant differences in TOC, TN and δ13C between the different zones. The TOC contents increased as follows: CMZ<FMZ<IMZ, suggesting the capture ability of organic carbon for mangroves. The covariation between C/N and δ13C suggested a mixture of sources (riverine sediment and marine phytoplankton) contributing to the sedimentary organic carbon. Furthermore, the contribution of riverine sediments is higher than that of marine-derived organic carbon at most sites, which is 2.5-fold higher in the IMZ. The contribution of mangrove leave only occupies less than 7%. As mangroves grow, the proportion of mangroves to sedimentary TOC will also increase. In the future, mangrove restoration, which is one of the most important carbon sink routes, should be emphasized through policies.
Data availability statement
The original contributions presented in the study are included in the article/Supplementary Material. Further inquiries can be directed to the corresponding author.
Author contributions
YL: Conceptualization, Data curation, Methodology, Writing – original draft. CL: Formal analysis, Investigation, Writing – review & editing. ZD: Conceptualization, Writing – review & editing. XZ: Data curation, Formal analysis, Writing – review & editing.
Funding
The author(s) declare that financial support was received for the research, authorship, and/or publication of this article.
Acknowledgments
This study was supported by National Natural Science Key Foundation of China (NSFC) (41930537) and Shanghai International Science and Technology Cooperation Fund Project (23230713800). Thanks to Bingbin Feng, Jiejun Luo, and Lingxiao Wang for their field survey and laboratory processes.
Conflict of interest
The authors declare that the research was conducted in the absence of any commercial or financial relationships that could be construed as a potential conflict of interest.
Publisher’s note
All claims expressed in this article are solely those of the authors and do not necessarily represent those of their affiliated organizations, or those of the publisher, the editors and the reviewers. Any product that may be evaluated in this article, or claim that may be made by its manufacturer, is not guaranteed or endorsed by the publisher.
Supplementary material
The Supplementary Material for this article can be found online at: https://www.frontiersin.org/articles/10.3389/fmars.2024.1428229/full#supplementary-material
References
Adame M. F., Fry B. (2016). Source and stability of soil carbon in mangrove and freshwater wetlands of the Mexican Pacific coast. Wetl Ecol. Manage. 24, 129–137. doi: 10.1007/s11273-015-9475-6
Alongi D. M. (2014). Carbon cycling and storage in mangrove forests. Annu. Rev. Mar. Sci. 6, 195–219. doi: 10.1146/annurev-marine-010213-135020
Alongi D. M., Pfitzner J., Trott L. A., Tirendi F., Dixon P., Klumpp D. W. (2005). Rapid sediment accumulation and microbial mineralization in forests of the mangrove Kandelia candel in the Jiulongjiang Estuary, China. Estuar. Coast. Shelf S. 63, 605–618. doi: 10.1016/j.ecss.2005.01.004
Bergamaschi B. A., Krabbenhoft D. P., Aiken G. R., Patino E., Rumbold D. G., Orem W. H. (2012). Tidally driven export of dissolved organic carbon, total mercury, and methylmercury from a mangrove-dominated estuary. Environ. Sci. Technol. 46, 1371–1378. doi: 10.1021/es2029137
Bouillon S., Boschker H. T. S. (2006). Bacterial carbon sources in coastal sediments: a cross-system analysis based on stable isotope data of biomarkers. Biogeosciences. 3, 175–185. doi: 10.5194/bg-3-175-2006
Bouillon S., Connolly R. M., Lee S. Y. (2008). Organic matter exchange and cycling in mangrove ecosystems: Recent insights from stable isotope studies. J. Sea Res. 59, 44–58. doi: 10.1016/j.seares.2007.05.001
Bouillon S., Dahdouh-Guebas F., Rao A. V. V. S., Koedam N., Dehairs F. (2003). Sources of organic carbon in mangrove sediments: variability and possible ecological implications. Hydrobiologia. 495, 33–39. doi: 10.1023/A:1025411506526
Breithaupt J. L., Smoak J. M., Smith T. J., Sanders C. J. (2014). Temporal variability of carbon and nutrient burial, sediment accretion, and mass accumulation over the past century in a carbonate platform mangrove forest of the Florida Everglades. J. Geophys Res-Biogeo. 119, 2032–2048. doi: 10.1002/2014JG002715
Chen G. C., Azkab M. H., Chmura G. L., Chen S. Y., Sastrosuwondo P., Ma Z. Y., et al. (2017). Mangroves as a major source of soil carbon storage in adjacent seagrass meadows. Sci. Rep-Uk 7, 42406. doi: 10.1038/srep42406
Donato D. C., Kauffman J. B., Murdiyarso D., Kurnianto S., Stidham M., Kanninen M. (2011). Mangroves among the most carbon-rich forests in the tropics. Nat. Geosci. 4, 293–297. doi: 10.1038/ngeo1123
Duarte C. M., Losada I. J., Hendriks I. E., Mazarrasa I., Marba N. (2013). The role of coastal plant communities for climate change mitigation and adaptation. Nat. Clim Change. 3, 961–968. doi: 10.1038/nclimate1970
Feng J., Cui X., Zhou J., Wang L., Zhu X., Lin G. (2019). Effects of exotic and native mangrove forests plantation on soil organic carbon, nitrogen, and phosphorus contents and pools in Leizhou, China. CATENA 180, 1–7. doi: 10.1016/j.catena.2019.04.018
Fest B. J., Swearer S. E., Arndt S. K. (2022). A review of sediment carbon sampling methods in mangroves and their broader impacts on stock estimates for blue carbon ecosystems. Sci. Total Environment. 816, 151618. doi: 10.1016/j.scitotenv.2021.151618
Furukawa K., Wolanski E. (1996). Sedimentation in mangrove forests. Mangroves Salt Marshes. 1, 3–10. doi: 10.1023/A:1025973426404
Hinrichs S., Nordhaus I., Geist S. J. (2009). Status, diversity and distribution patterns of mangrove vegetation in the Segara Anakan lagoon, Java, Indonesia. Reg. Environ. Change. 9, 275–289. doi: 10.1007/s10113-008-0074-4
Jennerjahn T. C. (2021). Relevance and magnitude of 'Blue Carbon' storage in mangrove sediments: carbon accumulation rates vs. stocks, sources vs. sinks. Estuarine Coast. Shelf Science. 248, 107156. doi: 10.1016/j.ecss.2020.107027
Jiang R., Huang S., Wang W., Liu Y., Pan Z., Sun X., et al. (2020). Heavy metal pollution and ecological risk assessment in the Maowei sea mangrove, China. Mar. pollut. Bull. 161, 111816. doi: 10.1016/j.marpolbul.2020.111816
Kauffman J. B., Bernardino A. F., Ferreira T. O., Giovannoni L. R., Gomes L. E. D., Romero D. J., et al. (2018). Carbon stocks of mangroves and salt marshes of the Amazon region, Brazil. Biol. Lett., 14, 20180208. doi: 10.1098/rsbl.2018.0208
Ke Z. X., Tan Y. H., Huang L. M., Zhao C. Y., Jiang X. (2017). Spatial distributions of delta C-13, delta N-15 and C/N ratios in suspended particulate organic matter of a bay under serious anthropogenic influences: Daya Bay, China. Mar. pollut. Bull. 114, 183–191. doi: 10.1016/j.marpolbul.2016.08.078
Kennedy H., Gacia E., Kennedy D. P., Papadimitriou S., Duarte C. M. (2004). Organic carbon sources to SE Asian coastal sediments. Estuar. Coast. Shelf S. 60, 59–68. doi: 10.1016/j.ecss.2003.11.019
Kristensen E., Bouillon S., Dittmar T., Marchand C. (2008). Organic carbon dynamics in mangrove ecosystems: A review. Aquat. Botany. 89, 201–219. doi: 10.1016/j.aquabot.2007.12.005
Kumar A., Ramanathan A. L., Prasad M. B. K., Datta D., Kumar M., Sappal S. M. (2016). Distribution, enrichment, and potential toxicity of trace metals in the surface sediments of Sundarban mangrove ecosystem, Bangladesh: a baseline study before Sundarban oil spill of December 2014. Environ. Sci. pollut. Res. 23, 8985–8999. doi: 10.1007/s11356-016-6086-6
Kusumaningtyas M. A., Hutahaean A. A., Fischer H. W., Perez-Mayo M., Ransby D., Jennerjahn T. C. (2019). Variability in the organic carbon stocks, sources, and accumulation rates of Indonesian mangrove ecosystems. Estuar. Coast. Shelf S. 218, 310–323. doi: 10.1016/j.ecss.2018.12.007
Li S. S., Dai Z. J., Mei X. F., Huang H., Wei W., Gao J. J. (2017). Dramatic variations in water discharge and sediment load from Nanliu River (China) to the Beibu Gulf during 1960s-2013. Quatern Int. 440, 12–23. doi: 10.1016/j.quaint.2016.02.065
Liu T., Liu S., Wu B., Xu H., Zhang H. (2020). Increase of organic carbon burial response to mangrove expansion in the Nanliu River estuary, South China Sea. Prog. Earth Planet Sci. 7, 71. doi: 10.1186/s40645-020-00387-3
Long C. Q., Dai Z. J., Wang R. M., Lou Y. Y., Zhou X. Y., Li S. S., et al. (2022). Dynamic changes in mangroves of the largest delta in northern Beibu Gulf, China: Reasons and causes. For. Ecol. Manage. 504, 119855. doi: 10.1016/j.foreco.2021.119855
Loring D. H., Rantala R. T. T. (1992). Manual for the geochemical analyses of marine sediments and suspended particulate matter. Earth-Science Rev. 32, 235–283. doi: 10.1016/0012-8252(92)90001-A
Manju M. N., Resmi P., Kumar C. S. R., Gireeshkumar T. R., Chandramohanakumar N., Joseph M. M. (2016). Biochemical and stable carbon isotope records of mangrove derived organic matter in the sediment cores. Environ. Earth Sci. 75, 565. doi: 10.1007/s12665-016-5245-x
Marchand C., Disnar J. R., Lallier-Verg E., Lottier N. (2005). Early diagenesis of carbohydrates and lignin in mangrove sediments subject to variable redox conditions (French Guiana). Geochimica Et Cosmochimica Acta 69, 131–142. doi: 10.1016/j.gca.2004.06.016
Matos C. R. L., Berrêdo J. F., MaChado W., Sanders C. J., Metzger E., Cohen M. C. L. (2020). Carbon and nutrient accumulation in tropical mangrove creeks, Amazon region. Mar. Geol. 429, 106317. doi: 10.1016/j.margeo.2020.106317
Medina-Contreras D., Sánchez A., Arenas F. (2023). Macroinvertebrates food web and trophic relations of a peri urban mangrove system in a semi-arid region, Gulf of California, México. J. Mar. Systems. 240, 103864. doi: 10.1016/j.jmarsys.2023.103864
Mei X. F., Dai Z. J., Du J. Z., Cheng J. P. (2024). Three Gorges Dam enhanced organic carbon burial within the sediments of Poyang Lake, China. Catena 238, ARTN107859. doi: 10.1016/j.catena.2024.107859
Meng X. W., Xia P., Li Z., Meng D. Z. (2017). Mangrove Development and Its Response to Asian Monsoon in the Yingluo Bay (SW China) over the Last 2000 years. Estuaries And Coasts. 40, 540–552. doi: 10.1007/s12237-016-0156-3
Meyers P. A. (1997). Organic geochemical proxies of paleoceanographic, paleolimnologic, and paleoclimatic processes. Organic Geochemistry. 27, 213–250. doi: 10.1016/S0146-6380(97)00049-1
Parnell A. C., Inger R., Bearhop S., Jackson A. L. (2010). Source partitioning using stable isotopes: Coping with too much variation. PLoS One 5, e9672. doi: 10.1371/journal.pone.0009672
Passos T., Bernardino A. F., Penny D., Barcellos R., Passos F. U., Nobrega G. N., et al. (2023). Low carbon accumulation in a macro-tidal mangrove forest on the Amazon coast. Limnology Oceanography. 68, 1936–1948. doi: 10.1002/lno.12396
Ranjan R. K., Routh J., Ramanathan A. L., Klump J. V. (2011). Elemental and stable isotope records of organic matter input and its fate in the Pichavaram mangrove-estuarine sediments (Tamil Nadu, India). Mar. Chem. 126, 163–172. doi: 10.1016/j.marchem.2011.05.005
Saintilan N., Rogers K., Mazumder D., Woodroffe C. (2013). Allochthonous and autochthonous contributions to carbon accumulation and carbon store in southeastern Australian coastal wetlands. Estuar. Coast. Shelf S. 128, 84–92. doi: 10.1016/j.ecss.2013.05.010
Sánchez A., Gómez-León A. (2024). Azoic sediments and benthic foraminifera: Environmental quality in a subtropical coastal lagoon in the gulf of California. Environ. Res. 244, 117924. doi: 10.1016/j.envres.2023.117924
Sánchez A., López-Ortiz B. E., Aguíñiga-García S., Balart E. F. (2013). Distribution and composition of organic matter in sediments of the oxygen minimum zone of the Northeastern Mexican Pacific: paleoceanographic implications. J. Iberian Geology. 39, 111–120. doi: 10.5209/rev_JIGE.2013.v39.n1.41753
Sasmito S. D., Kuzyakov Y., Lubis A. A., Murdiyarso D., Hutley L. B., Bachri S., et al. (2020). Organic carbon burial and sources in soils of coastal mudflat and mangrove ecosystems. Catena. 187, 104414. doi: 10.1016/j.catena.2019.104414
Serrano O., Lovelock C. E., Atwood T. B., Macreadie P. I., Canto R., Phinn S., et al. (2019). Australian vegetated coastal ecosystems as global hotspots for climate change mitigation. Nat. Commun. 10, 4313. doi: 10.1038/s41467-019-12176-8
Tue N. T., Hamaoka H., Sogabe A., Quy T. D., Nhuan M. T., Omori K. (2011). The application of δ13C and C/N ratios as indicators of organic carbon sources and paleoenvironmental change of the mangrove ecosystem from Ba Lat Estuary, Red River, Vietnam. Environ. Earth Sci. 64, 1475–1486. doi: 10.1007/s12665-011-0970-7
Tue N. T., Ngoc N. T., Quy T. D., Hamaoka H., Nhuan M. T., Omori K. (2012). A cross-system analysis of sedimentary organic carbon in the mangrove ecosystems of Xuan Thuy National Park, Vietnam. J. Sea Res. 67, 69–76. doi: 10.1016/j.seares.2011.10.006
Tue N. T., Nguyen P. T., Quan D. M., Dung L. V., Quy T. D., Nhuan M. T., et al. (2018). Sedimentary composition and organic carbon sources in mangrove forests along the coast of northeast Vietnam. Regional Stud. Mar. Science. 17, 87–94. doi: 10.1016/j.rsma.2017.12.001
Twilley R. R., Rovai A. S., Riul P. (2018). Coastal morphology explains global blue carbon distributions. Front. Ecol. Environment. 16, 503–508. doi: 10.1002/fee.1937
Van Santen P., Augustinus P. G. E. F., Janssen-Stelder B. M., Quartel S., Tri N. H. (2007). Sedimentation in an estuarine mangrove system. J. Asian Earth Sci. 29, 566–575. doi: 10.1016/j.jseaes.2006.05.011
Vilhena M. P. S. P., Costa M. L., Berredo J. F., Paiva R. S., Moreira M. Z. (2018). The sources and accumulation of sedimentary organic matter in two estuaries in the Brazilian Northern coast. Regional Stud. Mar. Science. 18, 188–196. doi: 10.1016/j.rsma.2017.10.007
Walsh J. P., Nittrouer C. A. (2004). Mangrove-bank sedimentation in a mesotidal environment with large sediment supply, Gulf of Papua. Mar. Geol. 208, 225–248. doi: 10.1016/j.margeo.2004.04.010
Wang F., Lu X., Sanders C. J., Tang J. (2019). Tidal wetland resilience to sea level rise increases their carbon sequestration capacity in United States. Nat. Commun. 10, 5434. doi: 10.1038/s41467-019-13294-z
Watanabe K., Kuwae T. (2015). How organic carbon derived from multiple sources contributes to carbon sequestration processes in a shallow coastal system? Global Change Biol. 21, 2612–2623. doi: 10.1111/gcb.12924
Woodroffe C. D., Rogers K., McKee K. L., Lovelock C. E., Mendelssohn I. A., Saintilan N. (2016). Mangrove sedimentation and response to relative sea-level rise. Annu. Rev. Mar. Sci. 8, 243–266. doi: 10.1146/annurev-marine-122414-034025
Xia P., Meng X., Li Z., Feng A., Yin P., Zhang Y. (2015). Mangrove development and its response to environmental change in Yingluo Bay (SW China) during the last 150years: Stable carbon isotopes and mangrove pollen. Organic Geochemistry. 85, 32–41. doi: 10.1016/j.orggeochem.2015.04.003
Xue B., Yan C., Lu H., Bai Y. (2009). Mangrove-derived organic carbon in sediment from Zhangjiang Estuary (China) mangrove wetland. J. Coast. Res. 2009, 949–956. doi: 10.2112/08-1047.1
Yang J., Gao J., Liu B., Zhang W. (2014). Sediment deposits and organic carbon sequestration along mangrove coasts of the Leizhou Peninsula, southern China. Estuarine Coast. Shelf Science. 136, 3–10. doi: 10.1016/j.ecss.2013.11.020
Zakaria R., Chen G. C., Chew L., Sofawi A. B., Moh H. H., Chen S. Y., et al. (2021). Carbon stock of disturbed and undisturbed mangrove ecosystems in Klang Straits, Malaysia. J. Sea Res. 176, 102113. doi: 10.1016/j.seares.2021.102113
Zhang Y., Meng X., Xia P., Zhang J., Liu D., Li Z., et al. (2021). Spatiotemporal variations in the organic carbon accumulation rate in mangrove sediments from the Yingluo Bay, China, since 1900. Acta Oceanologica Sinica. 40, 65–77. doi: 10.1007/s13131-021-1864-5
Zhao C. Y., Jiang Z. J., Wu Y. C., Liu S. L., Cui L. J., Zhang J. P., et al. (2019). Origins of sediment organic matter and their contributions at three contrasting wetlands in a coastal semi-enclosed ecosystem. Mar. pollut. Bull. 139, 32–39. doi: 10.1016/j.marpolbul.2018.12.008
Zhong B. A., Xu Y. J. (2011). Risk of inundation to coastal wetlands and soil organic carbon and organic nitrogen accounting in Louisiana, USA. Environ. Sci. Technol. 45, 8241–8246. doi: 10.1021/es200909g
Keywords: total organic carbon, organic carbon accumulation rates, sources, sediments, mangrove ecosystem
Citation: Li Y, Long C, Dai Z and Zhou X (2024) Pattern of total organic carbon in sediments within the mangrove ecosystem. Front. Mar. Sci. 11:1428229. doi: 10.3389/fmars.2024.1428229
Received: 06 May 2024; Accepted: 04 July 2024;
Published: 25 July 2024.
Edited by:
Derrick Y. F. Lai, The Chinese University of Hong Kong, ChinaReviewed by:
Alberto Sánchez-González, National Polytechnic Institute (IPN), MexicoSonglin Liu, Chinese Academy of Sciences (CAS), China
Copyright © 2024 Li, Long, Dai and Zhou. This is an open-access article distributed under the terms of the Creative Commons Attribution License (CC BY). The use, distribution or reproduction in other forums is permitted, provided the original author(s) and the copyright owner(s) are credited and that the original publication in this journal is cited, in accordance with accepted academic practice. No use, distribution or reproduction is permitted which does not comply with these terms.
*Correspondence: Zhijun Dai, empkYWlAc2tsZWMuZWNudS5lZHUuY24=