- 1Faculty of Biosciences, Fisheries and Economics, UiT - The Arctic University of Norway, Tromsø, Norway
- 2The Arctic University Museum of Norway, UiT – the Arctic University of Norway, Tromsø, Norway
Marine invertebrate habitats are experiencing warming, and oceanic carbon dioxide levels are on the rise. These changes result in shifts in species distributions. Monitoring and understanding these shifts provides vital information because each species plays a unique ecological role, and the human utilization of marine species is intrinsically linked to their geographic locations. Here, we examine distribution shifts of marine invertebrates in the Barents Sea since pre-1900. Using data from the Global Biodiversity Information Facility, we analyzed species occurrences across warm, cold and mixed temperature zones, distinguishing between benthic and pelagic taxa. Our findings indicate community shifts in each of five separated time periods, with the most pronounced shifts occurring after 1980 in the cold and mixed zones, and earlier in the warm zone. The significant biogeographical changes at the community scale occurred both in benthic and pelagic realms, yet with differing trajectories in the period past 2000, and largely coincided with increased Atlantic Water inflow and reduced ice cover. Several invertebrate taxa exhibited a northward movement, falling into two categories: species migrating into the Barents Sea from the Norwegian mainland shelf, and those relocating from the southern Barents Sea to areas with mixed and colder temperatures. Some of these species may serve as indicator species for monitoring ecosystem and community change. The study highlights the importance of long-term datasets in quantifying community distribution shifts and understanding their ecological impacts.
1 Introduction
Marine invertebrates inhabit an environment that is being affected by global warming and increased ocean acidification (Byrne and Przeslawski, 2013). The Arctic is warming at an alarming rate and has warmed four times faster than the rest of the globe between 1979 and 2021 (Rantanen et al., 2022; Zhou et al., 2024). Within it, the Barents Sea, located in the Atlantic Arctic region in Norway’s and Russia’s waters, is a productive ecosystem that is disproportionally affected by the changing climate (Isaksen et al., 2022). Warming oceans are often characterized by changes in the distribution of marine species (Hastings et al., 2020). Although invertebrates can adapt to some extent to changes in their environment, their ability to acclimate is not necessarily sufficient to respond to the rapid shift in climate that has occurred since the last century (Hastings et al., 2020). Documenting shifts in species distributions is critical because different species provide different functions and ecosystem services (Sutton et al., 2020), and the human uses of marine species globally are inherently tied to their geographic distributions (Reygondeau, 2019). Detecting and interpreting shifts in species distribution requires extended time series data on species distributions. Yet observational time series are often sparse in high latitude ecosystems limiting our ability to detect changing distributions (Al-Habahbeh et al., 2020; Dalpadado et al., 2020).
The Barents Sea is a marginal sea of the Arctic Ocean. It is a shallow inflow shelf separated into two domains (Loeng, 1991): the north-eastern region is influence by colder Arctic waters, the water column is seasonally more stratified and seasonally ice-covered. The southern Barents Sea is influenced by the warmer, more saline Atlantic waters and has no ice cover (Lind et al., 2018). This water also reaches the north-western part of the Barents Sea. Both domains meet at the Polar Front and mix to some degree, creating a unique environment and productive zone (Loeng, 1997; Lind et al., 2018). Increasing transport of warm Atlantic water from the south in recent years has a strong influence on the temperature of the Barents Sea (Koenigstein, 2020; Pongrácz et al., 2020; Lundesgaard et al., 2022) with the most noticeable effect of reducing the ice cover in the Barents Sea (Docquier et al., 2020). Arctic sea-ice extent has decreased here by 10% per decade since the 1970s (Årthun et al., 2021). If the warming continues, a near-complete shift of the Barents Sea to conditions typical of the boreal northern North Atlantic is possible (Asplin et al., 2001; Lind et al., 2018). In addition to increasing water temperature, an increase in CO2 uptake in the Barents Sea has been reported, the main factor driving ocean acidification (AMAP, 2018; Csapó et al., 2021; Smedsrud et al., 2022). Temperature fluctuations in both the atmosphere and ocean of the region have been significant over decades and centuries, long preceding the recent warming period (Thomsen and Vorren, 1986; Drinkwater, 2006).
Marine organisms respond to such environmental changes (Jørgensen et al., 2021). Based on trend analysis from the 1850s to 2020 the poleward movement of marine species in the high latitudes is six times faster than that of terrestrial species (Lenoir et al., 2020). Such responses are, for example, mediated through warming waters changing or disrupting physiological processes and reproductive cycles of invertebrates, potentially leading to population-level changes (Richardson, 2008). Also, sea ice is essential for many Arctic marine organisms as it can modulate food supply, its quantity, composition, and quality (Comiso, 2002; Comiso et al., 2008; Cautain et al., 2022) and, hence, sea ice changes can affect shifts in distributions of associated biota. Also, changes in pH can create challenges for calcification of calcified organisms (Venn et al., 2013) such as molluscs and echinoderms.
Many invertebrates have a planktonic larval life stage between fecundation and metamorphosis. During this stage they disperse with ocean currents (Berge et al., 2005; Meyer-Kaiser et al., 2022). Their chances of survival as adults in the water column (for planktonic organisms) or in areas of the seafloor where the larvae settle (in the case of benthic adults) is largely determined by physical factors of the environment (Allmon and Hendricks, 2021). Due to their planktonic stage, some marine invertebrates may, in general, respond quickly to changes in environmental conditions and are useful indicators of change (Zakharov et al., 2020; Allmon and Hendricks, 2021). Holoplanktonic species, i.e. those with a completely pelagic life cycle, are assumed to respond to shorter-term shifts than benthic species given the former spent their entire life in the water column drifting with the current. In general, however, high latitude invertebrates might respond more slowly to environmental changes due to slower growth, longer generation times, and longer lifespans compared to their warm water relatives (Munch and Salinas, 2009). In the Barents Sea, species distributions are well documented in comparison to other Arctic regions although taxonomic and spatial gaps remain (Bluhm et al., 2011; Renaud et al., 2015; Johannesen et al., 2017). Several studies have indeed documented distributional changes in this region for particular taxa or taxon groups such as species in the phyla Mollusca and Echinodermata (Simkanin et al., 2005; Kantor et al., 2008; Snigirov et al., 2013; Berge et al., 2015; Zakharov and Jørgensen, 2017). Very few studies, however, have investigated distributional changes of marine invertebrates in the Barents Sea beyond two to four decades, or of larger groups of taxa. This larger perspective is needed, for example because a substantial number of marine invertebrates in that area is either harvested directly (Haug et al., 2017; Hvingel et al., 2021), or are prey to commercial species, and hence of broad interest.
Herein, we ask if and how the distribution of marine invertebrates in the Barents Sea has changed during the past 150 years. Such changes have potential consequences for the wider ecosystem, including its food webs, nutrient cycling, and carbon sequestration (Brierley and Kingsford, 2009). To address this question, we used data available through the Global Biodiversity Information Facility (GBIF), the largest global, online, open access taxon occurrence data portal (Callaghan et al., 2023; Lajeunesse and Fourcade, 2023). We then discuss if those changes can be related to a warming climate. We consider a period of roughly 150 years and use the GBIF-mediated data to test two hypotheses. Firstly, the distribution patterns of marine invertebrates in the Barents Sea have changed over the past 150 years. Secondly, Holo-planktonic organisms show different distributional responses to changing environmental conditions than benthic species due to potential faster dispersal through ocean currents.
2 Materials and methods
2.1 Study area and time periods
Our study area delineation corresponds to the Protection of the Arctic Marine Environment’s (PAME) Large Marine Ecosystems (LMEs) boundaries of the Barents Sea (Figure 1A, See Supplementary material for coordinates) (Protection of the Arctic Marine Environment PAME, 2023). By this definition, the Barents Sea Large Marine Ecosystem (LME) extends from the Norwegian and Russian coasts, including the Pechora Sea (roughly 65-70°N) in the south, to north of the Svalbard and Franz Josef Land archipelagos, including the continental slope and southern edge of the Nansen Basin in the north (roughly 81-82°N). In the west, the area is broadly delineated by the continental slope to the Norwegian Sea (roughly 6-15°E), while to the east, the Barents Sea borders the Kara Sea in a line following Novaya Zemlya to the eastern side of Franz Josef Land and northward (roughly 50-70°E). Whilst the polygon includes land, we only consider marine invertebrate species. Given the well-established thermal regimes of the Barents Sea we follow the literature for delineation of three thermal zones. The study area was separated into three zones based on the mean near-bottom water temperatures in the period 2004-2018 given in (Skagseth et al., 2020) in three unique polygons. Polygon “cold”, contains Arctic Water with a mean temperature between -2°C and 0°C, and comparatively low in salinity; polygon “mixed” has mean water temperatures ranging between 0°C to 2°C and is a result of mixing of Arctic and Atlantic waters; polygon “warm” consists of Atlantic Water that is high in salinity and temperature, between 2°C to 4°C.
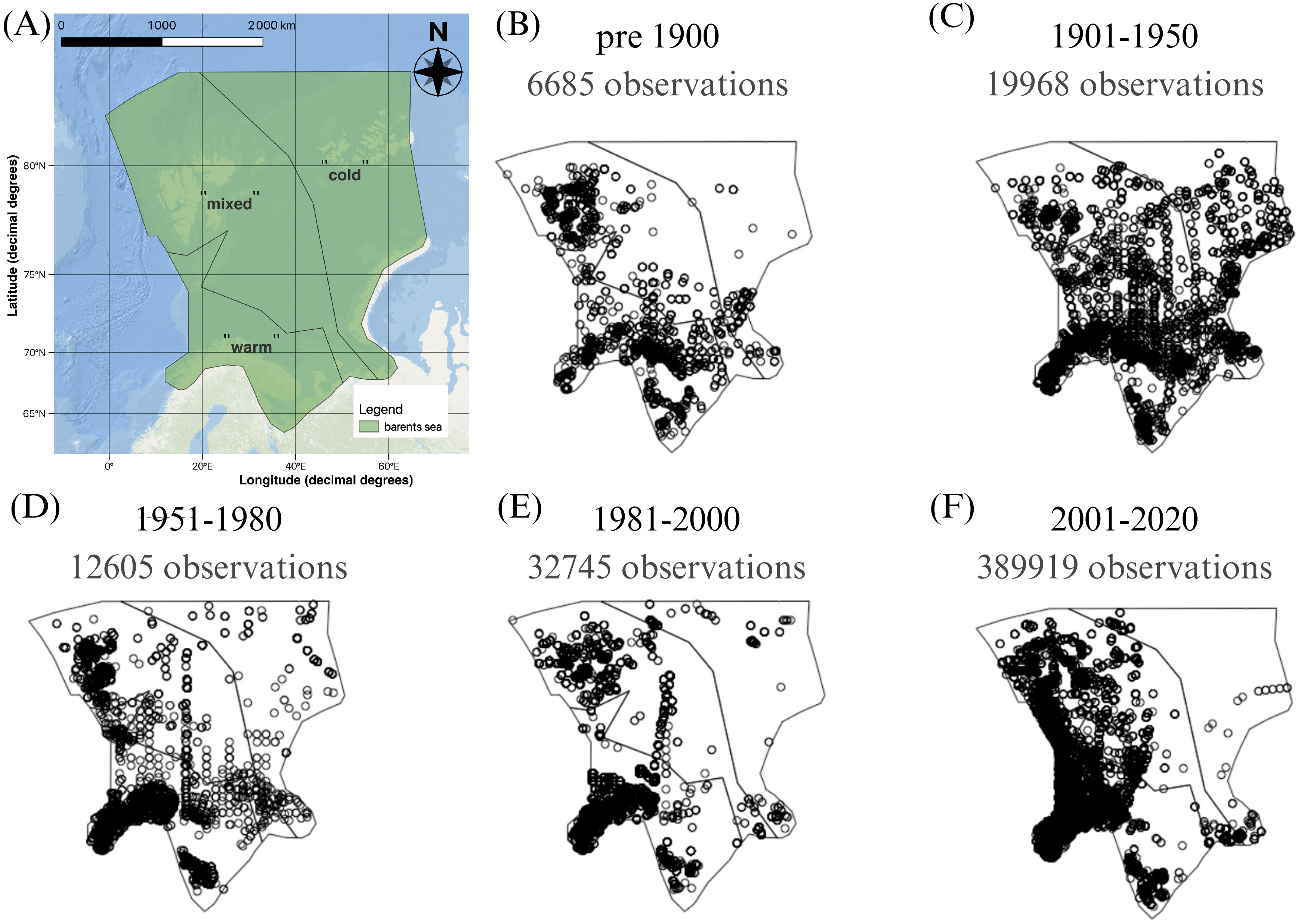
Figure 1. Map of the Barents Sea showing (A) the study area after the Protection of the Arctic Marine Environment’s Large Marine Ecosystems borders (Protection of the Arctic Marine Environment PAME, 2023) and its division in three zones based on average near-bottom water temperature in 2004-2018 in Skagseth et al. (2020) (shapefile “barents sea.shp” in Supplementary Material), and (B–F) the distribution of marine invertebrate occurrence record density by time period as well as the number of observations per period.
To assess changes in the distribution of species, we analyzed the number of occurrences within the “warm,” “mixed,” and “cold” zones over time. We divided the occurrence records into five distinct time periods, guided by the available temperature records and acknowledging interannual variability. These periods are defined as follows: “pre-industrial age” until 1900, 1901 to 1950, 1951 to 1980, 1981 to 2000, and 2001 to 2020.
The selection of these periods was influenced by the earliest available data in the GBIF for this region, which dates back to 1837. Post 1900, temperatures increased. For the period from 1901 to around 1950, the mean annual temperature index (Figure 2) generally ranged from approximately -0.5 to +1°C. Notably, a significant warming phase occurred from around 1930 to 1950, primarily due to increased northward transport of warm water via the Gulf Stream (Karsakov, 2009 as cited in Trofimov et al., 2019). Following this, a cooling phase ensued post-1950 (Carton et al., 2011), succeeded by several years of relative temperature stability yet with inherent variability (Figure 2). Starting around 1980, there has been a rise in water temperatures in the Barents Sea, with particularly pronounced and anomalously high temperatures since around 2000 (Boitsov et al., 2012; Trofimov et al., 2019). This warming is mainly driven by anomalous pulses of northward flowing Atlantic Water entering the Barents Sea with a particularly massive warming event beginning around 1999 (Carton et al., 2011) and also being increasingly strong in the area north of Svalbard (Lundesgaard et al., 2022). From a seasonal perspective, the warm temperatures have also lasted increasingly more days of the year during the ongoing century (Mohamed et al., 2022a). Coincidentally, air temperatures near the ocean surface of the Barents Sea showed a steep increase around the year 2003 (Cai et al., 2022). Consequently, we chose the most recent temperature period in our study to begin in 2000.
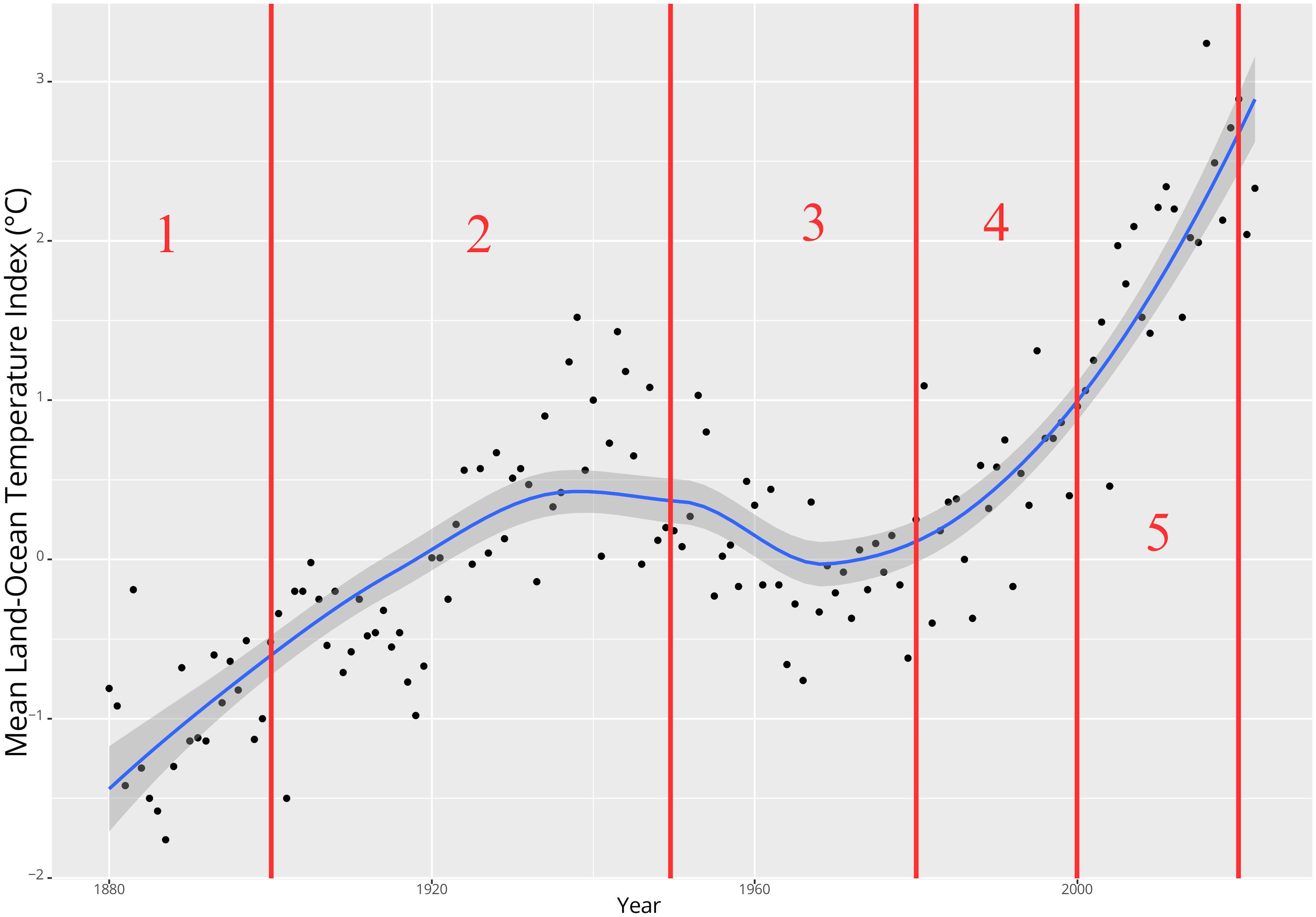
Figure 2. Timeline of annual mean land-ocean temperature index (°C) for the region extending from 64-90°N, including the division into 5 time periods (red lines and numbers) used in this study (GISS Surface Temperature Analysis GISTEMP v4 https://data.giss.nasa.gov/gistemp/) (Lenssen et al., 2019; GISTEMP Team, 2023).
2.2 Data acquisition and cleaning
We downloaded occurrence data of invertebrates in the Barents Sea for the period 1837 until 2020 from the Global Biodiversity Information Facility (GBIF). GBIF is a global biodiversity data infrastructure that provides access to georeferenced taxon occurrence information coming from a combination of sources ranging from historic museum records, to newer physical material collected during short-term and long-term research projects, and molecular sequence data, using a standardized vocabulary, and curated taxonomy through the World Register of Marine Species (Ahyong et al., 2024). Large past and ongoing research programs and museum collections housing material from the Barents Sea contribute to GBIF or linked databases such as the Ocean Biogeographic Information Service (OBIS) including, for example, the decade-long international Census of Marine Life program (2000-2010 and including compilation of earlier occurrence records), the Norwegian MAREANO program (2005-present) and the Arctic University Museum of Norway (records since 1877). However, other substantial data sets are not fully included such as those from the Ecosystem Survey conducted jointly by the Institute of Marine Research and the Russian Federal Institute of Fisheries and Oceanography PINRO. As a result, occurrence records are being incompletely captured, potentially leading to spatial, temporal or taxonomic under-representations. Using the software R (R Core Team, 2023) and the package rgbif (Chamberlain and Boettiger, 2017; Chamberlain et al., 2024) all occurrences of metazoans (excluding Craniata and Cephalochordata) in the Barents Sea were downloaded, separately for each time period (The Global Biodiversity Information Facility, GBIF.org, 2023a; The Global Biodiversity Information Facility, GBIF.org, 2023b; The Global Biodiversity Information Facility, GBIF.org, 2023c; The Global Biodiversity Information Facility, GBIF.org, 2023d; The Global Biodiversity Information Facility, GBIF.org, 2023e). Craniata includes all vertebrates, which were excluded from our analysis because they do not meet the criteria of being invertebrates. Similarly, while Cephalochordata are indeed invertebrates, they were not included due to their limited representation in the datasets.
We cleaned the datasets as follows: occurrences not identified to species level (e.g. Buccinum sp.), and species for which there were less than 10 observations during a given period in our study zone were removed. Non-marine taxa, including fossil, terrestrial, and freshwater species were excluded by running the list of species through the World Register of Marine Species (WoRMS) taxon match tool (https://marinespecies.org/aphia.php?p=match). Additionally, we defined each species as benthic or pelagic using our knowledge of Arctic benthic species and additional reference material for zooplankton (Kosobokova et al., 2011; Wold et al., 2023). Pelagic species were assigned as such when they were holoplanktonic.
2.3 Data analysis
All analyses were done using R (R Core Team, 2023). To analyze the temporal development of similarity patterns of species occurrences grouped by geographic zones, we used Correspondence Analysis (CA) (Greenacre and Primicerio, 2014; Thioulouse et al., 2018). Using the R packages ade4 (Thioulouse et al., 2018) and vegan (Oksanen et al., 2022) we ran a CA on all marine invertebrate species combined first, and then on benthic and pelagic species separately. In order to be able to run a CA the missing values due to the removal of species with less than 10 occurrences in some of the time periods were turned into “0”.
The CA for pelagic species strongly separated “1981-2000 ‘cold’” from all other groups. This was driven by the species Sarsicytheridea punctillata, Rhizocaulus verticillatus, Themisto compressa, Sagitta bipunctata, Hymenodora glacialis, Ptychogena crocea and Staurostoma mertensii. Sensitivity to a small number of data points is a well-known issue with CA (Greenacre, 2013). To illustrate additional patterns present in the data but obscured in the CA, we ran a second CA on the pelagic occurrence records in which we removed the above listed species. Here, we present this rerun, including 92 of the 99 species. To assess if functional groups (benthic vs pelagic) differed in terms of spatio-temporal distributions, we used the permutation tests provided in vegan, using ‘species groups’ as a predictor in a Canonical Correspondence Analysis (CCA).
Examples of species associated with particularly strong temporal shifts in geographic distribution over time were identified from the CA of the total occurrence record data set. Using the package explor (Barnier, 2023) we visualized species driving these shifts in the CA. The explor package opened an interactive shiny window where we isolated the driving species and tabulated them. For illustration, eight examples of species with high occurrence density that extended northwards were mapped using Quantum Geographic Information System (QGIS).
3 Results
3.1 Number of species
The complete download of data from GBIF of Animalia in the study area in June 2023 contained 14,544 species and 2,875,221 occurrence records. After removal of taxa identified to genus level the amount of occurrence records was 2,658,467. After removal of Insecta, Craniata and Cephalochordata, 5,293 species and 534,606 occurrence records remained. After removal of species with less than 10 occurrences per time period 2,227 species and 512,551 occurrence records remained. Of these, additional species were removed for multiple reasons: 307 because they could not be matched by the WoRMS taxon match tool (because they were not marine, unaccepted, or otherwise not matchable), 36 because they were “ambiguous” (variation in writing of species names) and 223 species because they were either fossil, freshwater or terrestrial species yet still in WoRMS. We also removed the diatom Actinocyclus octonarius because it was incorrectly registered as a mollusc in GBIF. After all cleaning steps, 1,660 species and 461,229 occurrence records remained for analysis (Supplementary Figure 1).
3.2 Distribution of occurrence records
The 461,229 occurrence records were distributed unequally across phyla, and in space and time (Figures 1B–F; Supplementary Figure 1). Most occurrence records where in the phylum Annelida (45%), followed by the phyla Arthropoda (22%), Mollusca (22%) and Echinodermata (5%). The remaining 21,261 occurrences were distributed across 13 phyla that each had <1.5% of the occurrence records. Most observations were of benthic species, namely 417,157 occurrences across 1,561 species, while 44,072 occurrences were distributed across 99 pelagic species.
In space, available occurrence records were most numerous in the southern, “warm” zone across all time periods (Figures 1B–F). Occurrence records in the “mixed” zone had a particularly high concentration around Svalbard in some periods. The distribution maps show a deficiency of available occurrences in the “cold” zone before 1900 and past 1981 (Figures 1B, D–F).
3.3 Change of invertebrate occurrence records over time
3.3.1 Benthic taxa
In the Correspondence Analysis (CA) of the occurrences of the 1,561 benthic species, the first three axes captured significant shifts in species occurrence patterns over the study period (Figures 3A, C). Specifically, CA axis 1 explained 21.8% of the total variance, CA axis 2 accounted for 15.1%, and CA axis 3 for 13.1%, cumulatively explaining 50.0% of the total variance in species occurrence patterns. This result confirms our first hypothesis that the distribution patterns of marine invertebrates in the Barents Sea have changed over the past 150 years.
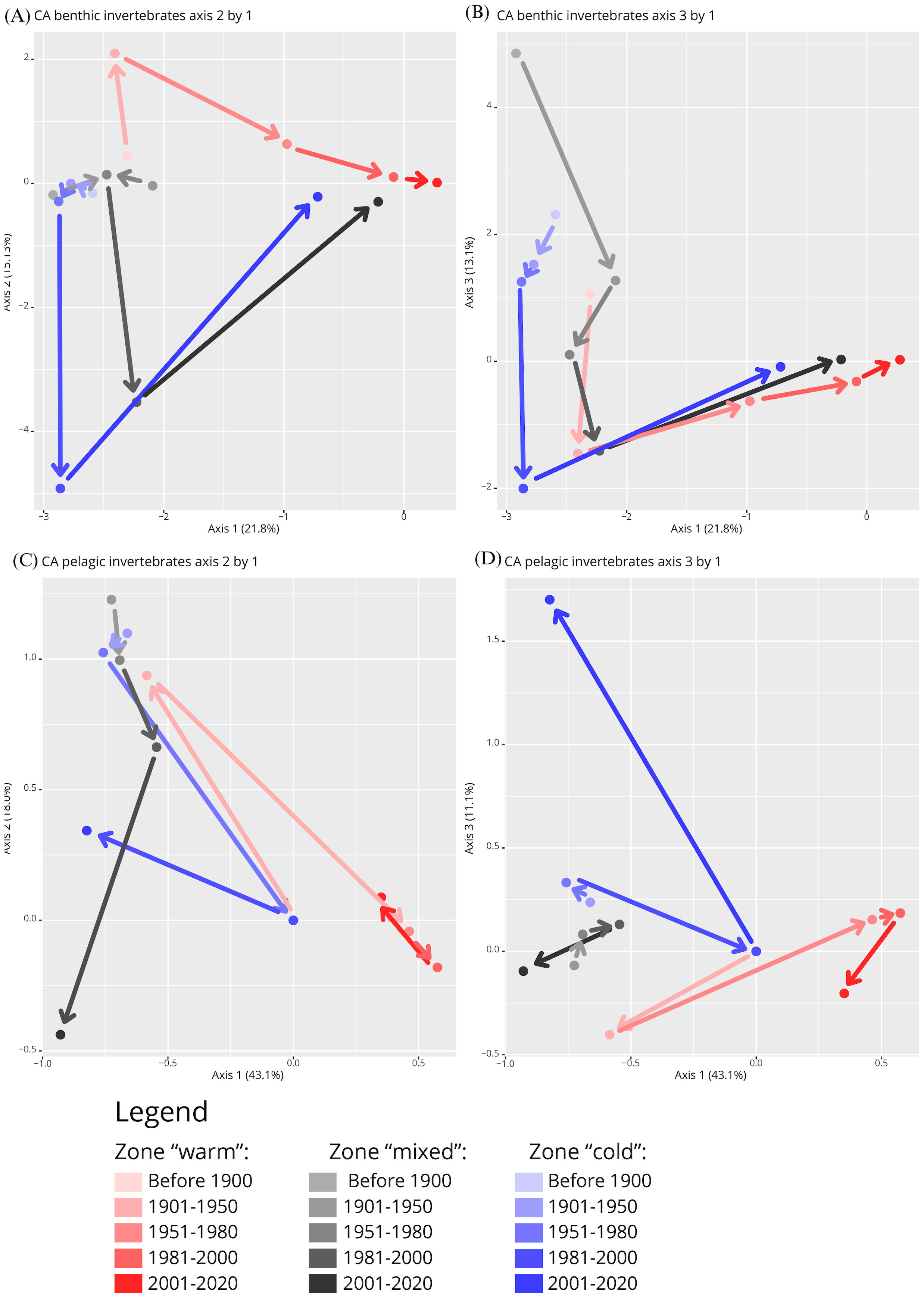
Figure 3. Shifts of marine invertebrate occurrences in the Barents Sea in three geographic zones with different temperature regimes over time (<1900 to 2020), shown as a correlation analysis (CA) of (A, B) benthic fauna, and (C, D) pelagic fauna. (A, C) show CA axis 2 by axis 1 and (B, D) show CA axis 3 by axis 1. Percent values indicate the amount of variation in the data set explained by the given axis. Arrows between zone-period occurrence groups were added to visualise the trajectories of community shifts with colours matching the respective period of a given arrow.
The observed shifts in species occurrence patterns across different temperature zones over time reflect potential ecological changes. Specifically, in the ‘cold’ and ‘mixed’ zones, the shifts in species occurrences were relatively minor until 1980, suggesting a period of relative ecological stability or slower ecological changes during this time. Post-1980, these zones experienced more pronounced shifts, indicating a period of rapid ecological change, possibly driven by environmental factors such as temperature changes. This is visually represented by the movement from the top left quadrant to the bottom left, and then to the top right quadrant of the CA plot from 1981 to 2020 (Figure 3A). In contrast, the ‘warm’ zone showed an earlier onset of change, starting after 1950, with less extreme shifts post-1980, suggesting an earlier response to environmental changes, which then stabilized. The trajectory of these shifts was consistently in the same direction, indicating a sustained directional change in species occurrences within this zone.
At the end points in 2020, occurrence records in all three zones had shifted into the same direction suggesting more taxa were shared among zones than in the two preceding periods. While endpoints were less similar to each other than at the starting point in <1900, they were much more similar than in 1981-2000. At the end point, occurrence records in the “mixed” zone were more similar to the other two zones than “warm” and “cold” zones were to each other. The CA displaying axis 3 against axis 1 points out a substantial contribution of axis 3 to an occurrence shift in the “warm” and “mixed” zones from <1900 to 1901-1950 (Figure 3B).
3.3.2 Differences in distribution shifts between pelagic and benthic taxa
Both the number of occurrence records and the number of taxa were drastically lower for pelagic than benthic species. The CAs of the two groups showed both similarities and differences in how occurrence patterns shifted over the last century in the Barents Sea (Figures 3A–D), partially confirming our second hypothesis. The occurrence patterns were statistically significantly different between pelagic and benthic data sets according to the CCA permutation test (F1,1658 = 115.9, P<0.001).
The CA pattern of pelagic occurrences was characterized by a pronounced dissimilarity after the 1981-2000 period in the “cold” and “mixed” zones, and past 1951-1980 in the “warm” zone (Figures 3C, D). In the “warm” zone there were two large shifts in pelagic occurrences in opposite direction occurring after 1900 and 1950, in agreement with the benthic occurrence shifts. The “mixed” zone, in contrast, was rather indistinct in its pattern of pelagic occurrences when axis 3 was plotted against axis 2 (Figure 3D). The CA with axis 3 plotted against axis 1 (Figure 3C), however, showed a continuous directional shift from 1901 to 2000, in contrast to the benthic trajectory in this zone. When comparing the same time periods across all zones, it becomes evident that taxa from before 1950 were similar whereas post 2000 they were positioned in opposite quadrants of the CA plot (Figure 3D). Occurrences before 1900 were available only in the “warm” zone for pelagic taxa.
3.3.3 Example taxa shifting distributions northwards
The periods of strongest shifts in species occurrence records occurred in 1901-1950 in the “warm” zone, and in 1981-2000 in the “mixed” and “cold” zones. Associated with these shifts were a total of 188 species, 64 corresponded to the 1901-1950 period in the “warm” zone, and 87 and 37 species, respectively, to 1981-2000 period in the “cold” and “mixed” zones (Supplementary Table 1). Individual verification of changes in spatial occurrence patterns for these species on GBIF revealed varied outcomes: some species showed no change in their distribution, others had decreasing occurrence record densities suggesting declines in abundance or distribution range in the study area, and a number of species expanded or shifted their range northward over time.
In Figures 4A–H examples of species are shown where occurrence records were present much further north in recent decades, especially in the most recent study period (2001-2020), than in the earlier periods. In examples shown in Figures 4A–E these species were commonly found along the Norwegian shelf south of the study region in the periods before 1981 according to GBIF (not shown) and appeared in the “warm” zone by the 1951-1980 or 1981-2000 periods. After the year 2000 the species in Figures 4B–E had spread northward inside the “warm” zone or – as can be seen especially for Tryphosella horingi (Figure 4F) - had crossed into the “mixed” zone yet remained present in the southwestern area of that same zone as well. Before 1981, these species had been largely absent from the offshore regions of the Barents Sea. The distribution maps indicate that while these species have expanded northwards, they have not penetrated into the “cold” zone, though we caution that studies conducted in the last decade may not yet be completely represented in GBIF perhaps biasing this result.
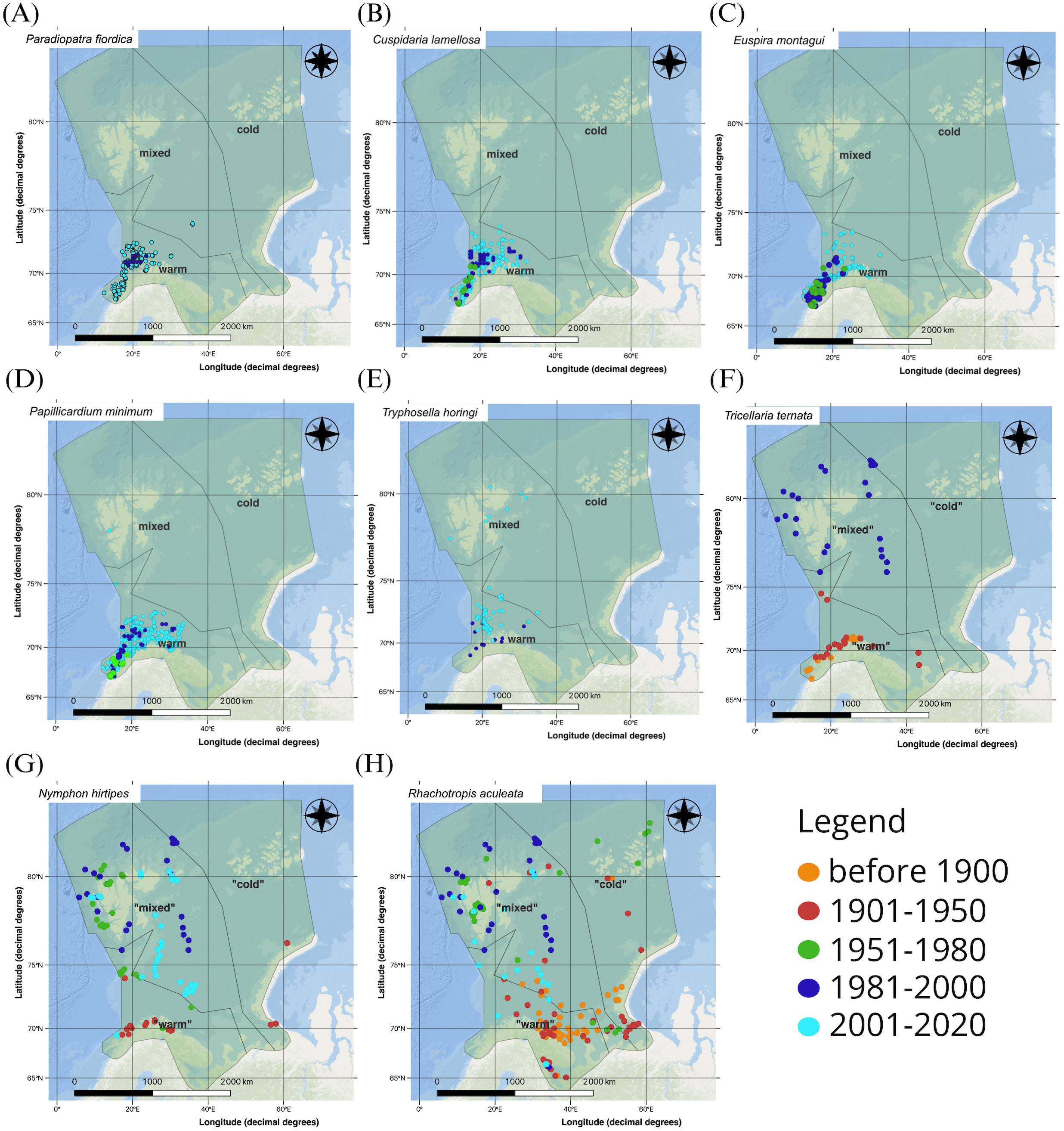
Figure 4. Examples of benthic marine invertebrate showing northward expansion from before 1900 to 2020, based on occurrence records from GBIF: (A) the polychaete Paradiopatra fiordica, (B) the bivalve Cuspidaria lamellosa, (C) the gastropod Euspira montagui, (D) the bivalve Papillicardium minimum, (E) the amphipod Tryphosella horingi, (F) the bryozoan Tricellaria ternata, (G) the pycnogonid Nymphon hirtipes (H) the amphipod Rhachotropis aculeata. GBIF records show that the species (A–F) were all common south of the study area along the Norwegian shelf in earlier periods.
The three example species illustrated in Figures 4F–H all occurred in the “warm” zone in the first two time periods, with a few scattered occurrence records further north at that time. By the 1980s, these species were found far to the north, around Svalbard and in the central Barents Sea inside the “mixed” zone where they have since remained in cases of Nymphon hirtipes and Rhachotropis aculeata (Figures 4G, H). In case of the bryozoan it is curious that no records occurred in GBIF in the most recent period since it is still present past 2000 (Evseeva and Dvoretsky, 2023). In contrast to the examples shown in Figures 4A–E, the last three species were almost absent from the southern, “warm” zone past the 1980s, suggesting their possible retreat out of that region.
4 Discussion
Our analysis of publicly accessible invertebrate occurrence records from the Barents Sea revealed significant variability in species occurrence patterns from before 1900 to the present. Benthic invertebrate species in the Arctic as a whole and also in the Barents Sea are more diverse (species rich) compared to pelagic species (Bluhm et al., 2011). This difference is attributed to several factors, including the more complex and stable habitats provided by benthic environments, which support a greater diversity of life forms (Sebens, 1991). In contrast, the pelagic zone in the Arctic is less diverse, partly due to the extreme and less stable conditions encountered in open water environments (Angel, 2003). We observed that the pattern of temporal shifts in species occurrences varied between the southern, warmer part of the Barents Sea and the northeastern, colder regions. In the southern Barents Sea, the most pronounced shifts in benthic faunal occurrences were after 1950, whereas in the central and northeastern areas, the largest shifts were noted after 1980. By the year 2020, the benthic occurrence patterns in the central and northern regions had begun to align with those in the warmer southern region, suggesting an increasing homogenization of invertebrate communities across the Barents Sea in recent years. In the pelagic, containing fewer records and species, the largest shift in the south was again before 1980 while it was after 1980 in the northern region. In contrast to the benthic occurrences, however, the communities of the current century are rather dissimilar in the three zones. Below, these observed patterns are examined in the context of the climatic conditions that prevailed in the region during the respective time periods.
4.1 Biological community shifts over time in relation to climate variability
4.1.1 Period pre-1900
Climate variability in the Arctic has led to significant faunal shifts over geological timescales. Approximately 10,000-year B.P. the Barents Sea experienced a major faunal transition after the end of the glaciation: Arctic fauna was replaced by boreal fauna and then shifted into the modern fauna (Thomsen and Vorren, 1986). This shift has been primarily attributed to increased temperature, salinity, and nutrient levels, delivered by the North Atlantic Current (Thomsen and Vorren, 1986). However, just prior to 1900, air temperatures in the northern North Atlantic were relatively cool compared to the subsequent warming trend that followed, as also reflected in reconstructed sea ice cover records (Shapiro et al., 2003; Drinkwater, 2006).
In the decades leading up to 1900, the GBIF benthic invertebrate occurrence data indicate that each geographic zone of the Barents Sea had a slightly different species composition; pelagic occurrences were partly lacking. Overall, however, the occurrence patterns of benthic invertebrates were more similar across the entire Barents Sea area during this time than in any later period except post-2000. While it is tempting to attribute this uniformity to consistent (and lower than today’s) bottom water temperatures, it is more plausible that the scarcity of occurrence records from the northern part of the “cold” zone introduces a bias, potentially exaggerating the perceived similarity in species composition pre-1900. Furthermore, the total number of species recorded during this early period was lower than in later periods, suggesting that researchers at that time may have focused primarily on documenting the easy-to-catch, larger fauna, possibly overlooking smaller species. This is consistent with the rate of marine species discovery and description globally being particularly high just after this time period (Appeltans et al., 2012). Yet, comparison with species records along the Norwegian mainland shelf suggests that it is indeed likely that a suite of boreal taxa now found in the Barents Sea was absent from, or at least very sparse in, the entire Barents Sea region at that time.
4.1.2 Period 1901-1950
The northern North Atlantic experienced a warming trend from the relatively cool temperatures at the end of the 19th century, continuing through to around the mid-20th century (Drinkwater, 2006). During this period, temperatures in Svalbard and the Barents Sea exhibited some fluctuations, with notably cooler years around 1920, and warmer years in the 1940s-1950s, varying by location (Levitus et al., 2009). The Kola Peninsula transect crossing the southern Barents Sea also confirms the 1930s being a warm period (Matishov et al., 2012).
During this period, the warming in the northern North Atlantic coincides with moderate shifts in benthic invertebrate occurrences in the “warm” and “mixed” zones (and a more substantial shift for pelagic species in the “warm” zone), while the “cold” zone remained largely unchanged from the preceding period. This suggests that increasing atmospheric and bottom water temperatures impacted the boreal taxa in the southern and central Barents Sea more than the Arctic species to the north. This observation aligns with the northward expansion of boreal Atlantic cod (Gadus morhua), which spread further northwards to around Bear Island and as far as western Svalbard, temporarily creating new opportunities for fishing in these regions (Blacker, 1957, 1965; Cushing, 1982). Plankton data suggests that these shifts in cod distribution and behavior were driven by bottom-up ecological processes (Drinkwater, 2009), consistent with the more substantial shift in pelagic rather than benthic invertebrates in the warm zone in our analysis.
4.1.3 Period 1951-1980
Interannual fluctuations and regional differences characterize this period in the study region and northern North Atlantic, but overall, a cooling trend occurred over multiple years (Drinkwater, 2006). Near the Krendel Observatory in Svalbard, annual mean atmospheric temperatures dropped by 2°C over 10 years (1950 to 1960), and then remained stable around -14°C until the 1980s (Ivanov et al., 2021). Negative temperature anomalies were also seen in upper ocean temperature along the long-term hydrographic transect off the Kola Peninsula in the late 1960s and late 1970s (Matishov et al., 2012). In fact, the depth-averaged annual mean water temperatures along the Kola Peninsula transect decreased by as much as 2°C during some years (Yashayaev and Seidov, 2015). Overall, the temperature anomalies relative to the 1950-2015 period were mostly in the negative between 1951 and 1980 (Yashayaev and Seidov, 2015). Despite a brief warming period in the mid-1970s, the overall cooling trend persisted into the 1980s in that region (Supplementary Table 2).
This climate period is matched by differing patterns of invertebrate occurrences between 1951 and 1980 across the three geographic zones (Yashayaev and Seidov, 2015). Our analysis suggests that the “warm” zone in the southwest of the Barents Sea underwent a more substantial transformation in species composition during the observed period than the other two zones. Benthic biomass in this region declined compared to surveys in the 1920-30s, which some have interpreted as an effect of the cooling, while others have explained it as a time-delayed response of Arctic-boreal species to the warming in the preceding period (Kiyko and Pogrebov, 1997). In contrast, species occurrences in the “cold” and “mixed” zones remained similar to those in the previous period and to each other, consistent with (Dyer et al., 1984).
4.1.4 Period 1981-2000
A warm period began in the study region in the 1980s and continues to the present day, particularly affecting northern regions above ca. 60⁰N and reaching to 30°N in its southernmost extent (Drinkwater, 2009). From 1981 to 2000, the warmest phase started in 1987 and reached a peak in the early 1990s in the Barents Sea opening and along the southern Kola Peninsula transects, with positive anomalies of 0.3 to 1°C in the upper ocean layer [relative to the 1950-2015 mean; (Yashayaev and Seidov, 2015)].
Coinciding with the climate pattern, large shifts in the distribution of both benthic and pelagic invertebrates in the Barents Sea occurred after 1980, especially in the “cold” and “mixed” zones. The reported occurrence density of certain Arctic species, for example the brittle star Ophiocten sericeum, decreased markedly during this period. Conversely, occurrence records of other species, both Arctic-boreal and boreal, increased, e.g., the snail Euspira montagui and the bivalve Cuspidaria lamellosa (details in section 4.2). These changes in occurrences indicate a northward distribution shift (discussed in section 4.2), likely linked to the warming waters during that time. In more localized areas, such as Kongsfjorden in western Svalbard, the composition of coastal hard-bottom benthic communities varied in response to the strength of North Atlantic climate indices (Beuchel et al., 2006), with a pronounced shift in 1994 and 1996, coinciding with the North Atlantic Oscillation Index’s transition from a positive to a negative mode (Beuchel et al., 2006). In this interval and subsequent years overall biodiversity increased while a decline in certain taxa such as actiniarians occurred, and a dense carpet of brown algae emerged at Svalbard coastal sites (Beuchel et al., 2006). These new algal habitats, in turn, facilitated the settlement of additional species (Kortsch et al., 2012), exemplifying how climate-induced changes can create new ecological niches that then attract a suite of previously absent species.
4.1.5 Period past 2000: strong Atlantification
In the mid-2000s, a rapid climate shift took place in the Barents Sea (Lind et al., 2018). This was due to several factors: a warmer surface layer, reduced stratification of the water column resulting from a decrease in freshwater input due to reduced ice melt and increased vertical mixing. These changes caused the warming waters to extend increasingly to the seafloor (Lind et al., 2018). Sea surface temperatures progressively warmed at a rate of 0.25°C per decade from the 1980s – 2020 (Mohamed et al., 2022b), while air temperatures increased by 2.7 – 4°C per decade (Isaksen et al., 2022). Water temperatures across the top 200 m were particularly high between 2001 and 2006 (Matishov et al., 2012), associated with increasing Atlantic water inflow (Ingvaldsen et al., 2021). The decline in sea ice cover by approximately 15 – 10% per decade led to an extended open water period by more than three months in the ice-covered shelf areas (Isaksen et al., 2022). This change has slightly increased pelagic primary production in the northern Barents Sea, as observed in a time series from 1998-2012 (Dalpadado et al., 2014). Additionally, the peak period of the phytoplankton bloom has advanced by over a month and has extended in duration, depending on the location. The reduction in sea-ice cover, along with the presumed decline in sea-ice algal production, is expected to have negative effects on ice-dependent and sympagic species (Dalpadado et al., 2020). Jointly, the strengthening influence of Atlantic Water in the Barents Sea region on hydrography and biota has been termed ‘Atlantification’ (Ingvaldsen et al., 2021), or more broadly ‘borealization’, a process where sub-Arctic Atlantic (and Pacific) waters, and their biota, are brought into the polar region (Polyakov et al., 2020).
Since the year 2000, all three temperature zones have maintained distinct benthic species occurrence patterns; however, they have shifted in the same direction in the CA. This suggests increasing similarity among the three zones compared to previous decades, except before 1900 when the communities were also similar to each other but differed from today’s. Recent studies have confirmed the trend of borealisation, showing that boreal megabenthic species have increased in number and extended their range further north and north-eastward, while the spatial range of Arctic species has retracted north-eastward and diminished (Jørgensen et al., 2019; Zakharov et al., 2020). The extended ice-free periods have led to community composition and related functional shifts in some cases, for example evidenced through a decrease in the proportion of benthic polychaetes and an increase in both the proportion and diversity of bivalves in the Makarov Strait, located within our “cold” zone (Pavlova et al., 2023). Increased vertical carbon flux in this area is thought to have led to a higher abundance of infaunal species, specifically subsurface deposit feeders, and mobile suspension feeders (Pavlova et al., 2023). In the pelagic, communities have been variable, yet with a notable increase in biomass in krill post 2000 relative to earlier decades, especially in the southwestern and northwestern Barents Sea (Zhukova et al., 2009, Johannesen et al. 2012). Krill composition varied interannually, with warmer water species tending to appear in or slightly following warm pulses (Zhukova et al., 2009).
Reported range extensions of boreal species cover substantial distances: The boreal amphipod Gammarus oceanicus, for example, became dominant in Svalbard’s shore zone from 2008 onwards, whereas from 1980 to 1994, it was only sporadically observed along the west and north coast of Spitsbergen; the total expansion of the distribution range was over 1300 km (Węsławski et al., 2018). Other examples include the cephalopod species Teuthowenia magalops and Todaropsis eblanae which have been found in the Arctic part of the Barents Sea, more than 2500 km from their previously known ranges (Golikov et al., 2013). The gastropod Aporrhais pespelecani was also recorded nearly 1000 km beyond its known range on the Murman coast in the Eastern part of the Barents Sea (Kantor et al., 2008). Such range shifts are also seen in other Nordic areas, for example research on the Canadian Atlantic shelf has shown that the commercial decapod crustacean species Pandalus borealis and Chionoecetes opilio are likely to respond significantly to climate change. This includes a northward distribution shift for the northern shrimp and a decrease in the abundance of both the snow crab and shrimp, which is associated with rising temperatures (Zabihi-Seissan et al., 2024).
Overall, all invertebrate phyla considered in this study were affected by borealisation. Within the Barents Sea and at a whole-community level this movement was quantified to be 4.4 km north-eastward per year in its center of gravity from 2004 to 2013 in a model approach (Nascimento et al., 2023). Whole community shifts imply function changes in the system as have been documented for food web structure of the Barents Sea (Kortsch et al., 2015). Feeding guild compositional shifts could also be anticipated with regional increases in primary production, for example regular recruitment events of filter-feeding species from boreal environments might start to alter functional biogeography and enhance borealisation at the polar front, similar to changes observed in Arctic fish communities (Reed et al., 2021). However, successful recruitment depends on favorable conditions for fertilization, larval development, and settlement, aspects of benthic invertebrate biology (Reed et al., 2021).
Like invertebrates, boreal fish communities have been expanding northward in response to climate shifts, while the Arctic fish communities retract north-eastward (Fossheim et al., 2015). Again, functional shifts are associated: true Arctic fishes, typically small bottom-dwelling benthivores, are being replaced by larger, longer lived, generalist and piscivorous boreal species (Frainer et al., 2017) whose presence increases food web connectivity (Kortsch et al., 2015). Consistent with these assessments, the Arctic is expected to experience the highest species turnover globally due to invasions and local extinctions, with invasion intensity projected to be five times the global average (Cheung et al., 2009). Another study estimated a climate velocity of 0.96°N/year, and a distribution shift velocity of 0.90°N/year for 325 taxa, using the bias and relative bias (in °N per year) between taxon-specific velocities and observation (Pinsky et al., 2013).
4.2 Temporal development of distribution ranges of example species
This and previous studies have documented a shift in species and community distributions in the Barents Sea region. Collectively, such changes reflect changing environmental conditions across large spatial scales (Worm and Lotze, 2009; Pinsky et al., 2013). Knowledge of individual species’ ranges and their shifts can aid in identifying useful indicator species for long-term monitoring. In the study area, taxa typical of either Arctic or Atlantic water masses are targeted for monitoring in some cases (Mańko et al., 2015). Such species should be reasonably common and easy to identify reliably – a factor recently highlighted when the very common pelagic indicator species for Atlantic and Arctic waters, the copepods Calanus finmarchicus and C. glacialis, respectively, were shown to be often misidentified using traditional morphological characteristics (Gabrielsen et al., 2012). While we identified some taxa that could serve as useful indicators of boreal Atlantic or Arctic Ocean climates, given the distribution patterns and shifts we have identified, it is important to consider that benthic species, especially those with high longevity and lacking pelagic larvae, such as amphipods and pycnogonids, or brooding species, may exhibit a delayed response to changing conditions compared to pelagic species [estimated at 1-5 years by Kiyko and Pogrebov (1997)]. Additionally, specialized species with small niches are thought to be less adaptable to climate warming than less specialized species with larger niches (Sewell and Hofmann, 2011).
We provide examples for both species with retreating distribution ranges away from the southern Barents Sea, as well as boreal species coming from the Norwegian mainland coast and expanding distribution ranges northwards. Pycnogonids, benthic arthropods without pelagic larval stages and not as well studied as some other arthropod groups, are regularly found, albeit typically not in very high abundances, in the Barents Sea, including Nymphon hirtipes (Vassilenko and Petryashov, 2009; Jørgensen et al., 2014; Ringvold et al., 2014). As a shelf and upper continental slope species, N. hirtipes is widely distributed in the Barents, Kara and Laptev seas (Vassilenko and Petryashov, 2009), but also in NE Greenland (Fredriksen et al., 2020) and in the Beaufort Sea (BB, pers. obs.). Bamber and Thurston (2008) characterized the biogeography of the species as Arctic, and the spatio-temporal distribution pattern that we derived from GBIF records supports this conclusion. This species’ occurrence in the southern, warmer part of the Barents Sea before 1950 may indicate that its thermal tolerance window was exceeded after the 1980s when bottom waters began to warm substantially. Albeit few, early occurrence records in the “cold” region demonstrate the species’ occurrence there throughout the whole time period considered. N. hirtipes may be a useful benthic climate indicator species for the Barents Sea region, and it is advisable to search for and add additional historic records to GBIF. Similarly, the amphipod Rhachotropic aculeata, a widespread species across much of the Arctic and north Atlantic, is a cold-adapted species with an optimum around 3°C (Lörz et al., 2022). Bottom water temperatures exceeded 4°C in large parts of the southern Barents Sea in the period 2000-2009 (and perhaps beyond) and even 6°C in a substantial area in the southwestern part (Boitsov et al., 2012). We suspect this warming was substantial enough to shrink this amphipod’s distribution range while it was frequently found further north in colder water.
In contrast, the burrowing predatory bivalve Cuspidaria lamellosa (Pearson et al., 1996) and the carnivorous gastropod Euspira montagui (Durieux et al., 2010) are species with distributions in temperate and boreal areas that, as we have shown, contributed to the borealisation of the Barents Sea. For C. lamellosa, GBIF had fewer than 20 occurrences recorded before 1980 in our study zone. By 1996, 301 occurrences were recorded in the Northern North Sea (Pearson et al., 1996), while the majority of occurrences were registered after the year 2000, and virtually all of those were located along the coast of Norway and in the southern Barents Sea (Figure 4B). This suggests an extension of the species’ distribution area northward, though enhanced research effort in the north may bias this inference to some degree. The snail E. montagui (described as Natica montagui) is also widespread in the Northeast Atlantic from Iceland to the UK, into the Baltic Sea, and north to Norway where it has long been known to occur from the Skagerrak coast to Lofoten and north to Hammerfest (Høisæter, 2009), while records offshore in the Barents Sea are from the latest decades, past 2000 (Figure 4C). Planktonic trochophore larvae and later juvenile veliger stages may have supported their northward spread from 1950 to 2020 (Figure 4C). While we here focus on range extensions northwards, the implication is at the same habitat loss in areas where temperatures exceed thermal adaptations. The response of the arctic bivalve Mya truncata to marine heatwaves, for example, suggests that M. truncata may struggle to adapt to warmer conditions (Beaudreau et al., 2024). The patterning of marine heatwaves in the Northwest Atlantic could be a significant indicator of this and other species’ population viability and potential range changes. Given that polar regions are warming at a rate faster than the global average, M. truncata could be at a competitive disadvantage as the warm adapted species M. arenaria encroaches on M. truncata’s native territory (Beaudreau et al., 2024).
The phenomenon of borealisation is not only present in the Barents Sea, but can also be observed in the Pacific Arctic region, namely the Bering and Chukchi seas. In these regions, borealisation, or Pacification, is related to an increased influx of warm Pacific waters into the Arctic Ocean through the Bering Strait, associated with increases in heat (and freshwater) fluxes (Grebmeier et al., 2018; Polyakov et al., 2020). Linked to this phenomenon is the retreating sea ice; jointly, these changes promote borealisation on the Pacific Arctic inflow shelf (Mueter and Litzow, 2008; von Biela et al., 2023).
4.3 Dealing with uncertainties in working with GBIF data
Analyses such as those in the present study suffer from biases inherently associated with datasets compiled over long time periods, large geographic areas, and originating from many data providers with different cultures of data archival and levels of taxonomic expertise. Factors such as war can also affect regional marine research, such as during World War II, when research in the Barents Sea region was suspended. As a result of all the possible combined biases, effort should be dedicated to quality-controlling and cleaning the data present in public databases such as GBIF and WoRMS (Ahyong et al., 2024). The dataset we used, spanning a century with thousands of observations, contains inherent uncertainties due to changes in sampling methods and effort, as well as advances of taxonomic knowledge, which may lead to species misrepresentations (Nekhaev, 2014; Wessels et al., 2014; Lenoir et al., 2020). In this regard, we used additional sources (e.g., Zakharov et al., 2020 for megabenthos species; Kosobokova et al., 2011 and Wold et al., 2023 for zooplankton species; various primary literature for determining which species were freshwater, terrestrial and fossil taxa, WoRMS 2023 (Ahyong et al., 2024) for the most current nomenclature) to improve the correctness of the taxon list that forms the basis for the presented analysis. Taxonomic name changes over time are addressed in WoRMs through built-in updates to the most current accepted taxonomy. Removing likely artefacts yielded a more robust dataset, though the possibility of hidden artefacts in our dataset remains.
Sampling effort has increased over time, particularly in areas relevant to the human harvest of marine resources, and is likely related to more advanced knowledge-based management regimes (Rocha-Ortega et al., 2021). In the 1901-1950 period, previously undetected species appeared in the study area. Given the increasing number of occurrences in this period, however, part of the occurrence changes seen in the correspondence analysis was likely due to the increase in research effort, and perhaps also to taxonomic knowledge and the diversity of sampling tools used during sampling campaigns. In the last 40 years (1981-2020), more samples were reported than in the entire previous century, demonstrating the inequality of sampling effort throughout history (Rocha-Ortega et al., 2021), or reflecting the fact that many of early observation records are not FAIR (findable, accessible, interoperable, reusable). It remains likely, however, that studies conducted in the recent decades are not yet fully represented in GBIF. In addition, Figure 1 illustrates the uneven sampling effort among regions, with less intense sampling in the “cold” zone of the Arctic than the “warm” zone, a difference likely largely related to the seasonal ice cover in the former, which requires specialized vessels. Geographic inaccuracy – especially in older records – found in occurrence mapping may also affect diversity assessments, and some suggest perhaps even more so (Maldonado et al., 2015). Mitigating this problem to some degree, GBIF has geographic quality control built in by comparing coordinates with the country referenced in the metadata and the latitude/longitude data given in the data files, flagging possible mismatches. While we could not address the unequal geographic distribution of records, our use of credible taxon distribution information (Dalpadado et al., 2020; Zakharov et al., 2020) enhanced our confidence in the correctness of the georeferencing of distribution records.
Finally, the availability of taxon occurrence data, encouraged through open access policies and their enforcement, has been unequal over time and across nations and their respective data-regulating bodies (Wessels et al., 2014; Yu et al., 2022). Differences in funding and data-sharing cultures among data providers can result in data availability biases in GBIF (Beck et al., 2014). While the consequences of this caveat could not be addressed in the present study, national governments are increasingly moving towards data-sharing protocols and enforcement (Michener, 2015), such that analyses like those attempted in this study will become even more complete and, hence, more robust in the future.
5 Conclusion
We documented shifts in invertebrate occurrence records that likely reflect shifting distributions of invertebrates in the Barents Sea from before 1900 to 2020. These shifts varied between the warmer southern and colder northern regions in their pattern over time, yet for benthic species, they converged in the same direction after the year 2000, while they do less so for (the much fewer) pelagic occurrences. Recognizing that GBIF does not contain a complete record of taxon distributions, these shifts are consistent with previous studies on borealisation and were not specific to any particular phylum. While this study did not quantitatively link these distribution changes to temperature or other climatic factors, they broadly coincide with temperature patterns from time series in the atmosphere and ocean in the study region. This study contributes to the growing body of literature documenting that marine invertebrates respond to changing environmental conditions in community assemblage, anchored in part by their physiological tolerances (Logerwell et al., 2022), but also through processes such as facilitation and predator-prey relationships (Kortsch et al., 2012). Long-term monitoring programs and open-access databases, combined with stringent data-sharing principles, are important for tracking changes in the distribution of marine invertebrates, and we encourage efforts that help to make species distribution data available in a timely manner. One of the challenges in monitoring and selecting indicator species is that responses to shifting climates vary among taxa, and even closely related species may show variable responses (Lörz et al., 2022). In addition, factors other than temperature, such as depth, stratification, available sediments, and functional guilds of organisms, should be considered to comprehensively understand drivers of shifts in species distributions (Baker and Hollowed, 2014). Knowledge of species distribution shifts is essential for identifying potential conservation measures, adjusting stock assessments and region-specific harvest rates, and developing feasible future scenarios for adaptive management.
Data availability statement
The original contributions presented in the study are included in the article/Supplementary Material. Further inquiries can be directed to the corresponding author.
Author contributions
NC: Writing – review & editing, Writing – original draft, Visualization, Formal analysis, Data curation, Conceptualization. BB: Writing – review & editing, Supervision, Data curation, Conceptualization. NY: Writing – review & editing, Visualization, Formal analysis. AA: Writing – review & editing, Supervision, Software, Data curation, Conceptualization.
Funding
The author(s) declare financial support was received for the research, authorship, and/or publication of this article. BB and NC were supported by the Nansen Legacy project funded by the Norwegian Research Council and partner institutions including UiT The Arctic University of Norway (grant number 276730). AA was supported by the UArctic Thematic Network on Arctic Marine Biodiversity.
Acknowledgments
We thank Dag Endresen (GBIF Norway) and Arve Lynhammar (UiT) for fruitful discussions. The basis of the article is the master thesis by NC.
Conflict of interest
The authors declare that the research was conducted in the absence of any commercial or financial relationships that could be construed as a potential conflict of interest.
Publisher’s note
All claims expressed in this article are solely those of the authors and do not necessarily represent those of their affiliated organizations, or those of the publisher, the editors and the reviewers. Any product that may be evaluated in this article, or claim that may be made by its manufacturer, is not guaranteed or endorsed by the publisher.
Supplementary material
The Supplementary Material for this article can be found online at: https://www.frontiersin.org/articles/10.3389/fmars.2024.1421475/full#supplementary-material
References
Ahyong S., Boyko C. B., Bailly N., Bernot J., Bieler R., Brandão S. N., et al. (2024). World Register of Marine Species (WoRMS) (WoRMS Editorial Board). Available at: https://www.marinespecies.org.
Al-Habahbeh A. K., Kortsch S., Bluhm B. A., Beuchel F., Gulliksen B., Ballantine C., et al. (2020). Arctic coastal benthos long-term responses to perturbations under climate warming. Philos. Trans. R. Soc A 378, 20190355. doi: 10.1098/rsta.2019.0355
Angel M. V. (2003). “Ecosystems of the world,” in Ecosystems of the deep oceans, vol. 28 . Ed. Tyler P. A. (Elsevier, Amsterdam, The Netherlands), 39–79.
Appeltans W., Ahyong S., Anderson G., Angel M., Artois T., Bailly N., et al. (2012). The magnitude of global marine species diversity. Curr. Biol. 22, 2189–2202. doi: 10.1016/j.cub.2012.09.036
Årthun M., Onarheim I. H., Dörr J., Eldevik T. (2021). The Seasonal and Regional Transition to an Ice-Free Arctic. Geophys. Res. Lett. 48 (1), e2020GL090825. doi: 10.1029/2020GL090825
Asplin L., Ingvaldsen R., Loeng H., Sætre R. (2001). Transport of atlantic water in the western Barents Sea (International Council for the Exploration of the Sea (ICES)). doi: 10.17895/ices.pub.25636305.v1
Baker M. R., Hollowed A. B. (2014). Delineating ecological regions in marine systems: Integrating physical structure and community composition to inform spatial management in the eastern Bering Sea. Deep Sea Res. Part II: Topical Stud. Oceanography 109, 215–240. doi: 10.1016/j.dsr2.2014.03.001
Bamber R. N., Thurston M. H. (2008). The deep-water pycnogonids (Arthropoda: Pycnogonida) of the northeastern Atlantic Ocean. Zool. J. Linn. Soc 115, 117–162. doi: 10.1111/zoj.1995.115.issue-2
Barnier J. (2023). explor: interactive interfaces for results exploration: R package. R package version 0.3.10.9000. https://juba.github.io/explor/.
Beaudreau N., Page T. M., Drolet D., McKindsey C. W., Howland K. L., Calosi P. (2024). Using a metabolomics approach to investigate the sensitivity of a potential Arctic-invader and its Arctic sister-species to marine heatwaves and traditional harvesting disturbances. Sci. Total Environ. 917, 170167. doi: 10.1016/j.scitotenv.2024.170167
Beck J., Böller M., Erhardt A., Schwanghart W. (2014). Spatial bias in the GBIF database and its effect on modeling species' geographic distributions. Ecol. Inform. 19, 10–15. doi: 10.1016/j.ecoinf.2013.11.002
Berge J., Heggland K., Lønne O. J., Cottier F., Hop H., Gabrielsen G. W., et al. (2015). First records of Atlantic mackerel (Scomber scombrus) from the Svalbard archipelago, Norway, with possible explanations for the extensions of its distribution. Arctic 68 (1), 54–61. doi: 10.14430/arctic4455
Berge J., Johnsen G., Nilsen F., Gulliksen B., Slagstad D. (2005). Ocean temperature oscillations enable reappearance of blue mussels Mytilus edulis in Svalbard after a 1000 year absence. Mar. Ecol. Prog. Ser. 303, 167–175. doi: 10.3354/meps303167
Beuchel F., Gulliksen B., Carroll M. L. (2006). Long-term patterns of rocky bottom macrobenthic community structure in an Arctic fjord (Kongsfjorden, Svalbard) in relation to climate variability, (1980–2003). J. Mar. Syst. 63, 35–48. doi: 10.1016/j.jmarsys.2006.05.002
Blacker R. (1957). Benthic animals as indicators of hydrographic conditions and climatic change in Svalbard waters (London: Fisheries Investigations) Series 2. 20, 1–49.
Blacker R. (1965). Recent changes in the benthos of the West Spitsbergen fishing grounds Vol. 6 (Special Publication of the International Commission on the Northwest Atlantic Fisheries), 791–794.
Bluhm B. A., Gebruk A. V., Gradinger R., Hopcroft R. R., Huettmann F., Kosobokova K. N., et al. (2011). Arctic marine biodiversity an update of species richness and examples of biodiversity change. Oceanography 24, 232–248. doi: 10.5670/oceanog
Boitsov V. D., Karsakov A. L., Trofimov A. G. (2012). Atlantic water temperature and climate in the Barents Sea 2000–2009. ICES J. Mar. Sci. 69, 833–840. doi: 10.1093/icesjms/fss075
Brierley A. S., Kingsford M. J. (2009). Impacts of climate change on marine organisms and ecosystems. Curr. Biol. 19, R602–R614. doi: 10.1016/j.cub.2009.05.046
Byrne M., Przeslawski R. (2013). Multistressor impacts of warming and acidification of the ocean on marine invertebrates’ Life histories. Integr. Comp. Biol. 53, 582–596. doi: 10.1093/icb/ict049
Cai Z., You Q., Chen H. W., Zhang R., Chen D., Chen J., et al. (2022). Amplified wintertime Barents Sea warming linked to intensified Barents oscillation. Environ. Res. Lett. 17, 044068. doi: 10.1088/1748-9326/ac5bb3
Callaghan C. T., Borda-de-Água L., van Klink R., Rozzi R., Pereira H. M. (2023). Unveiling global species abundance distributions. Nat. Ecol. Evol. 7, 1600–1609. doi: 10.1038/s41559-023-02173-y
Carton J. A., Chepurin G. A., Reagan J., Häkkinen S. (2011). Interannual to decadal variability of Atlantic Water in the Nordic and adjacent seas. J. Geophysical Research: Oceans 116, C11035. doi: 10.1029/2011JC007102
Cautain I. J., Last K. S., McKee D., Bluhm B. A., Renaud P. E., Ziegler A. F., et al. (2022). Uptake of sympagic organic carbon by the Barents Sea benthos linked to sea ice seasonality. Front. Mar. Sci. 9. doi: 10.3389/fmars.2022.1009303
Chamberlain S., Barve V., Mcglinn D., Oldoni D., Desmet P., Geffert L., et al. (2024). rgbif: interface to the global biodiversity information facility API. R package version 3.8.0. https://CRAN.R-project.org/package=rgbif.
Chamberlain S. A., Boettiger C. (2017). R Python, and Ruby clients for GBIF species occurrence data. PeerJ Prepr 5, e3304v3301. doi: 10.7287/peerj.preprints.3304v1
Cheung W. W. L., Lam V. W. Y., Sarmiento J. L., Kearney K., Watson R., Pauly D. (2009). Projecting global marine biodiversity impacts under climate change scenarios. Fish Fish. 10, 235–251. doi: 10.1111/j.1467-2979.2008.00315.x
Comiso J. C. (2002). A rapidly declining perennial sea ice cover in the Arctic. Geophys. Res. Lett. 29 (20), 17-11–17-14. doi: 10.1029/2002GL015650
Comiso J. C., Parkinson C. L., Gersten R., Stock L. (2008). Accelerated decline in the Arctic sea ice cover. Geophys. Res. Lett. 35. doi: 10.1029/2007GL031972
Csapó H. K., Grabowski M., Węsławski J. M. (2021). Coming home - Boreal ecosystem claims Atlantic sector of the Arctic. Sci. Total Environ. 771, 144817. doi: 10.1016/j.scitotenv.2020.144817
Dalpadado P., Arrigo K. R., Hjøllo S. S., Rey F., Ingvaldsen R. B., Sperfeld E., et al. (2014). Productivity in the barents sea - response to recent climate variability. PloS One 9, e95273. doi: 10.1371/journal.pone.0095273
Dalpadado P., Arrigo K. R., van Dijken G. L., Skjoldal H. R., Bagøien E., Dolgov A. V., et al. (2020). Climate effects on temporal and spatial dynamics of phytoplankton and zooplankton in the Barents Sea. Prog. Oceanogr. 185, 102320. doi: 10.1016/j.pocean.2020.102320
Docquier D., Fuentes-Franco R., Koenigk T., Fichefet T. (2020). Sea ice—Ocean interactions in the barents sea modeled at different resolutions. Front. Earth Sci. 8, 172. doi: 10.3389/feart.2020.00172
Drinkwater K. F. (2006). The regime shift of the 1920s and 1930s in the North Atlantic. Prog. Oceanogr. 68, 134–151. doi: 10.1016/j.pocean.2006.02.011
Drinkwater K. (2009). Comparison of the response of Atlantic cod (Gadus morhua) in the high-latitude regions of the North Atlantic during the warm periods of the 1920s–1960s and the 1990s–2000s. Deep Sea Res. Part II: Topical Stud. Oceanography 56, 2087–2096. doi: 10.1016/j.dsr2.2008.12.001
Durieux E. D., Morin J., Alizier S., Dauvin J.-C., Brind’Amour A. (2010). Temporal changes in the spatial coupling between bentho-demersal fishes and their macrobenthic preys in the Seine estuary. ICES 17, 21.
Dyer M., Cranmer G., Fry P., Fry W. (1984). The distribution of benthic hydrographic indicator species in Svalbard waters 1978–1981. J. Mar. Biol. Assoc. U.K. 64, 667–677. doi: 10.1017/S0025315400030332
Evseeva O. Y., Dvoretsky A. G. (2023). Shallow-water bryozoan communities in a glacier fjord of west svalbard, Norway: species composition and effects of environmental factors. Biology 12, 185. doi: 10.3390/biology12020185
Fossheim M., Primicerio R., Johannesen E., Ingvaldsen R. B., Aschan M. M., Dolgov A. V. (2015). Recent warming leads to a rapid borealization of fish communities in the Arctic. Nat. Clim. Change. 5, 673–677. doi: 10.1038/nclimate2647
Frainer A., Primicerio R., Kortsch S., Aune M., Dolgov A. V., Fossheim M., et al. (2017). Climate-driven changes in functional biogeography of Arctic marine fish communities. Proc. Natl. Acad. Sci. U.S.A. 114, 12202–12207. doi: 10.1073/pnas.1706080114
Fredriksen R., Christiansen J. S., Bonsdorff E., Larsen L.-H., Nordström M. C., Zhulay I., et al. (2020). Epibenthic megafauna communities in Northeast Greenland vary across coastal, continental shelf and slope habitats. Polar Biol. 43, 1623–1642. doi: 10.1007/s00300-020-02733-z
Gabrielsen T. M., Merkel B., Søreide J. E., Johansson-Karlsson E., Bailey A., Vogedes D., et al. (2012). Potential misidentifications of two climate indicator species of the marine arctic ecosystem: Calanus glacialis and C. finmarchicus. Polar Biol. 35, 1621–1628. doi: 10.1007/s00300-012-1202-7
Golikov A. V., Sabirov R. M., Lubin P. A., Jørgensen L. L. (2013). Changes in distribution and range structure of Arctic cephalopods due to climatic changes of the last decades. Biodiversity 14, 28–35. doi: 10.1080/14888386.2012.702301
Grebmeier J. M., Frey K. E., Cooper L. W., Kędra M. (2018). Trends in benthic macrofaunal populations, seasonal sea ice persistence, and bottom water temperatures in the Bering Strait Region. Oceanography 31, 136–151. doi: 10.5670/oceanog
Greenacre M. (2013). The contributions of rare objects in correspondence analysis. Ecology 94, 241–249. doi: 10.1890/11-1730.1
Greenacre M., Primicerio R. (2014). Multivariate Analysis of Ecological Data. Fundación BBVA, Bilbao, Spain.
Hastings R. A., Rutterford L. A., Freer J. J., Collins R. A., Simpson S. D., Genner M. J. (2020). Climate change drives poleward increases and equatorward declines in marine species. Curr. Biol. 30, 1572–1577. e1572. doi: 10.1016/j.cub.2020.02.043
Haug T., Bogstad B., Chierici M., Gjøsæter H., Hallfredsson E. H., Høines Å .S., et al. (2017). Future harvest of living resources in the Arctic Ocean north of the Nordic and Barents Seas: A review of possibilities and constraints. Fish. Res. 188, 38–57. doi: 10.1016/j.fishres.2016.12.002
Hendricks J. R., Stigall A. L., Lieberman B. S. (2015). The Digital Atlas of Ancient Life: delivering information on paleontology and biogeography via the web. Palaeontologia Electronica 18.2.3E, 1–9. doi: 10.26879/153E
Høisæter T. (2009). Distribution of marine, benthic, shell bearing gastropods along the Norwegian coast. Fauna Norv. 28, 5–107. doi: 10.5324/fn.v28i0.563
Hvingel C., Sainte-Marie B., Kruse G. H. (2021). Cold-water shellfish as harvestable resources and important ecosystem players. ICES J. Mar. Sci. 78, 479–490. doi: 10.1093/icesjms/fsab005
Ingvaldsen R. B., Assmann K. M., Primicerio R., Fossheim M., Polyakov I. V., Dolgov A. V. (2021). Physical manifestations and ecological implications of Arctic Atlantification. Nat. Rev. Earth Environ. 2, 874–889. doi: 10.1038/s43017-021-00228-x
Isaksen K., Nordli Ø., Ivanov B., Køltzow M.A. Ø., Aaboe S., Gjelten H. M., et al. (2022). Exceptional warming over the Barents area. Sci. Rep. 12, 9371. doi: 10.1038/s41598-022-13568-5
Ivanov B., Karandasheva T., Demin V., Revina A., Sviashchennikov P., Isaksen K., et al. (2021). Assessment of long-term changes in the surface air temperature from the High Arctic archipelago Franz Joseph Land from 1929 to the present, (2017). Czech Polar Rep. 11, 114–133. doi: 10.5817/CPR2021-1-9
Johannesen E., Ingvaldsen R. B., Bogstad B., Dalpadado P., Eriksen E., Gjøsæter H., et al (2012). Changes in Barents Sea ecosystem state, 1970-2009: climate fluctuations, human impact, and trophic interactions. ICES J Mar Sci. 69 (5), 880–889. doi: 10.1093/icesjms/fss046
Johannesen E., Jørgensen L. L., Fossheim M., Primicerio R., Greenacre M., Ljubin P. A., et al. (2017). Large-scale patterns in community structure of benthos and fish in the Barents Sea. Polar Biol. 40, 237–246. doi: 10.1007/s00300-016-1946-6
Jørgensen L. L., Arvanitidis C., Birchenough S. N., Clark M. R., Silva Cruz I. C., Cunha M., et al. (2021). Marine invertebrates. In Second World Ocean Assessment. New York: The United Nations. pp. 141–159.
Jørgensen L. L., Ljubin P., Skjoldal H. R., Ingvaldsen R. B., Anisimova N., Manushin I. (2014). Distribution of benthic megafauna in the Barents Sea: baseline for an ecosystem approach to management. ICES J. Mar. Sci. 72 (2), 595–613. doi: 10.1093/icesjms/fsu106
Jørgensen L., Primicerio R., Ingvaldsen R., Fossheim M., Strelkova N., Thangstad T., et al. (2019). Impact of multiple stressors on sea bed fauna in a warming Arctic. Mar. Ecol. Prog. Ser. 608, 1–12. doi: 10.3354/meps12803
Kantor Y. I., Rusyaev S. M., Antokhina T. I. (2008). Going eastward—climate changes evident from gastropod distribution in the Barents Sea. Ruthenica 18, 51–54.
Karsakov A. L. (2009). Hydrographic studies on the Kola Section in the Barents Sea in 1900–2008 (Murmansk, (in Russian): PINRO Press). 139 pp.
Kiyko O. A., Pogrebov V. B. (1997). Long-term benthic population changes, (1920–1930s-present) in the Barents and Kara Seas. Mar. pollut. Bull. 35, 322–332. doi: 10.1016/S0025-326X(97)00113-6
Kortsch S., Primicerio R., Beuchel F., Renaud P. E., Rodrigues J., Lønne O. J., et al. (2012). Climate-driven regime shifts in Arctic marine benthos. Proc. Natl. Acad. Sci. U.S.A. 109, 14052–14057. doi: 10.1073/pnas.1207509109
Kortsch S., Primicerio R., Fossheim M., Dolgov A. V., Aschan M. (2015). Climate change alters the structure of arctic marine food webs due to poleward shifts of boreal generalists. Proc. R. Soc B 282, 20151546. doi: 10.1098/rspb.2015.1546
Kosobokova K. N., Hopcroft R. R., Hirche H.-J. (2011). Patterns of zooplankton diversity through the depths of the Arctic’s central basins. Mar. Biodivers 41, 29–50. doi: 10.1007/s12526-010-0057-9
Lajeunesse A., Fourcade Y. (2023). Temporal analysis of GBIF data reveals the restructuring of communities following climate change. J. Anim. Ecol. 92, 391–402. doi: 10.1111/1365-2656.13854
Lenoir J., Bertrand R., Comte L., Bourgeaud L., Hattab T., Murienne J., et al. (2020). Species better track climate warming in the oceans than on land. Nat. Ecol. Evol. 4, 1044–1059. doi: 10.1038/s41559-020-1198-2
Lenssen N. J. L., Schmidt G. A., Hansen J. E., Menne M. J., Persin A., Ruedy R., et al. (2019). Improvements in the GISTEMP uncertainty model. Geophys. Res. Atmos. 124, 6307–6326. doi: 10.1029/2018JD029522
Levitus S., Matishov G., Seidov D., Smolyar I. (2009). Barents Sea multidecadal variability. Geophys. Res. Lett. 36, L19604. doi: 10.1029/2009GL039847
Lind S., Ingvaldsen R. B., Furevik T. (2018). Arctic warming hotspot in the northern Barents Sea linked to declining sea-ice import. Nat. Clim. Change. 8, 634–639. doi: 10.1038/s41558-018-0205-y
Loeng H. (1991). Features of the physical oceanographic conditions of the Barents Sea. Polar Res. 10, 5–18. doi: 10.3402/polar.v10i1.6723
Loeng H. (1997). Water fluxes through the Barents Sea. ICES J. Mar. Sci. 54, 310–317. doi: 10.1006/jmsc.1996.0165
Logerwell E. A., Wang M., Jörgensen L. L., Rand K. (2022). Winners and losers in a warming Arctic: Potential habitat gain and loss for epibenthic invertebrates of the Chukchi and Bering Seas 2008–2100. Deep Sea Res. Part II: Topical Stud. Oceanography 206, 105210. doi: 10.1016/j.dsr2.2022.105210
Lörz A.-N., Oldeland J., Kaiser S. (2022). Niche breadth and biodiversity change derived from marine Amphipoda species off Iceland. Ecol. Evol. 12, e8802. doi: 10.1002/ece3.8802
Lundesgaard Ø., Sundfjord A., Lind S., Nilsen F., Renner A. H. H. (2022). Import of Atlantic Water and sea ice controls the ocean environment in the northern Barents Sea. Ocean Sci. 18, 1389–1418. doi: 10.5194/os-18-1389-2022
Maldonado C., Molina C. I., Zizka A., Persson C., Taylor C. M., Albán J., et al. (2015). Estimating species diversity and distribution in the era of Big Data: to what extent can we trust public databases? Global Ecol. Biogeogr. 24, 973–984. doi: 10.1111/geb.12326
Mańko M., Panasiuk A., Żmijewska M. (2015). Pelagic coelenterates in the Atlantic sector of the Arctic Ocean - Species diversity and distribution as water mass indicators. Oceanol. Hydrobiol. Stud. 44, 466–479. doi: 10.1515/ohs-2015-0044
Matishov G., Moiseev D., Lyubina O., Zhichkin A., Dzhenyuk S., Karamushko O., et al. (2012). Climate and cyclic hydrobiological changes of the Barents Sea from the twentieth to twenty-first centuries. Polar Biol. 35, 1773–1790. doi: 10.1007/s00300-012-1237-9
Meyer-Kaiser K. S., Schrage K. R., von Appen W.-J., Hoppmann M., Lochthofen N., Sundfjord A., et al. (2022). Larval dispersal and recruitment of benthic invertebrates in the Arctic Ocean. Prog. Oceanogr. 203, 102776. doi: 10.1016/j.pocean.2022.102776
Michener W. K. (2015). Ecological data sharing. Ecol. Inform. 29, 33–44. doi: 10.1016/j.ecoinf.2015.06.010
Mohamed B., Nilsen F., Skogseth R. (2022a). Interannual and decadal variability of sea surface temperature and sea ice concentration in the barents sea. Remote Sens. 14, 4413. doi: 10.3390/rs14174413
Mohamed B., Nilsen F., Skogseth R. (2022b). Marine heatwaves characteristics in the barents sea based on high resolution satellite data, (1982–2020). Front. Mar. Sci. 13, 4436. doi: 10.3389/fmars.2022.821646
Mueter F. J., Litzow M. A. (2008). Sea ice retreat alters the biogeography of the Bering Sea continental shelf. Ecol. Appl. 18, 309–320. doi: 10.1890/07-0564.1
Munch S. B., Salinas S. (2009). Latitudinal variation in lifespan within species is explained by the metabolic theory of ecology. Proc. Natl. Acad. Sci. U.S.A. 106, 13860–13864. doi: 10.1073/pnas.0900300106
Nascimento M. C., Husson B., Guillet L., Pedersen T. (2023). Modelling the spatial shifts of functional groups in the Barents Sea using a climate-driven spatial food web model. Ecol. Model. 481, 110358. doi: 10.1016/j.ecolmodel.2023.110358
Nekhaev I. (2014). Marine shell-bearing Gastropoda of Murman (Barents Sea): an annotated check-list. Ruthenica 24, 75–121.
Oksanen J., Simpson G., Blanchet F. G., Kindt R., Legendre P., Minchin P., et al. (2022). vegan: Community Ecology Package. R package version 2.6-4. https://CRAN.R-project.org/package=vegan.
Pavlova L. V., Dvoretsky A. G., Frolov A. A., Zimina O. L., Evseeva O. Y., Dikaeva D. R., et al. (2023). The impact of sea ice loss on benthic communities of the makarov strait (Northeastern barents sea). Animals 13, 2320. doi: 10.3390/ani13142320
Pearson T. H., Mannvik H.-P., Evans R., Falk-Petersen S. (1996). The benthic communities of the Snorre field in the Northern North Sea (61 30′ N 2 10′ E): 1. The distribution and structure of communities in undisturbed sediments. J. Sea Res. 35, 301–314. doi: 10.1016/S1385-1101(96)90757-1
Pinsky M. L., Worm B., Fogarty M. J., Sarmiento J. L., Levin S. A. (2013). Marine taxa track local climate velocities. Science 341, 1239–1242. doi: 10.1126/science.1239352
Polyakov I. V., Alkire M. B., Bluhm B. A., Brown K. A., Carmack E. C., Chierici M., et al. (2020). Borealization of the arctic ocean in response to anomalous advection from sub-arctic seas. Front. Mar. Sci. 7. doi: 10.3389/fmars.2020.00491
Pongrácz E., Pavlov V., Hänninen N. (2020). Arctic Marine Sustainability Arctic Maritime Businesses and the Resilience of the Marine Environment: Arctic Maritime Businesses and the Resilience of the Marine Environment. Springer International Publishing, Cham. doi: 10.1007/978-3-030-28404-6
Protection of the Arctic Marine Environment PAME (2023). Large Marine Ecosystems (LME's) of the Arctic. Available online at: https://pame.is/projects/ecosystem-approach/arctic-large-marine-ecosystems-lme-s. August 30 2023.
Rantanen M., Karpechko A. Y., Lipponen A., Nordling K., Hyvärinen O., Ruosteenoja K., et al. (2022). The Arctic has warmed nearly four times faster than the globe since 1979. Commun. Earth Environ. 3, 168. doi: 10.1038/s43247-022-00498-3
Reed A. J., Godbold J. A., Solan M., Grange L. J. (2021). Reproductive traits and population dynamics of benthic invertebrates indicate episodic recruitment patterns across an Arctic polar front. Ecol. Evol. 11, 6900–6912. doi: 10.1002/ece3.7539
Renaud P. E., Sejr M. K., Bluhm B. A., Sirenko B., Ellingsen I. H. (2015). The future of Arctic benthos: Expansion, invasion, and biodiversity. Prog. Oceanogr. 139, 244–257. doi: 10.1016/j.pocean.2015.07.007
Reygondeau G. (2019). “Chapter 9 - Current and future biogeography of exploited marine groups under climate change,” in Predicting Future Oceans. Eds. Cisneros-Montemayor A. M., Cheung W. W. L., Ota Y. (Elsevier), 87–101. doi: 10.1016/B978-0-12-817945-1.00009-5
Richardson A. J. (2008). In hot water: zooplankton and climate change. ICES J. Mar. Sci. 65, 279–295. doi: 10.1093/icesjms/fsn028
Ringvold H., Hassel A., Bamber R., Buhl-Mortensen L. (2015). Distribution of sea spiders (Pycnogonida, Arthropoda) off northern Norway, collected by MAREANO. Mar. Biol. Res. 11 (1), 62–75. doi: 10.1080/17451000.2014.889308
Rocha-Ortega M., Rodriguez P., Córdoba-Aguilar A. (2021). Geographical, temporal and taxonomic biases in insect GBIF data on biodiversity and extinction. Ecol. Entomol. 46, 718–728. doi: 10.1111/een.13027
Sebens K. P. (1991). “Habitat structure and community dynamics in marine benthic systems,” in Habitat Structure: The physical arrangement of objects in space. Eds. Bell S. S., McCoy E. D., Mushinsky H. R. (Dordrecht: Springer Netherlands, Dordrecht), 211–234.
Sewell M. A., Hofmann G. E. (2011). Antarctic echinoids and climate change: a major impact on the brooding forms. Global Change Biol. 17, 734–744. doi: 10.1111/j.1365-2486.2010.02288.x
Shapiro I., Colony R., Vinje T. (2003). April sea ice extent in the Barents Sea 1850–2001. Polar Res. 22 (1), 5–10. doi: 10.3402/polar.v22i1.6437
Simkanin C., Power A. M., Myers A., McGrath D., Southward A., Mieszkowska N., et al. (2005). Using historical data to detect temporal changes in the abundances of intertidal species on Irish shores. J. Mar. Biol. Assoc. U.K. 85, 1329–1340. doi: 10.1017/S0025315405012506
Skagseth Ø., Eldevik T., Årthun M., Asbjørnsen H., Lien V. S., Smedsrud L. H. (2020). Reduced efficiency of the Barents Sea cooling machine. Nat. Clim. Change. 10, 661–666. doi: 10.1038/s41558-020-0772-6
Smedsrud L. H., Muilwijk M., Brakstad A., Madonna E., Lauvset S. K., Spensberger C., et al. (2022). Nordic Seas heat loss, Atlantic inflow, and Arctic sea ice cover over the last century. Rev. Geophys 60, e2020RG000725. doi: 10.1029/2020RG000725
Snigirov S., Sizo R., Sylantyev S. (2013). Lodgers or tramps? Aporrhais pespelecani and Turritella communis on the north-western Black Sea shelf. Mar. Biodivers. Rec. 6, e119. doi: 10.1017/S1755267213000900
Sutton R. T., Pincock D., Baumgart D. C., Sadowski D. C., Fedorak R. N., Kroeker K. I. (2020). An overview of clinical decision support systems: benefits, risks, and strategies for success. NPJ Digit. Med. 3, 17. doi: 10.1038/s41746-020-0221-y
The Global Biodiversity Information Facility GBIF.org (2023a). Occurrence Download 1900-1950. doi: 10.15468/dl.arcfsv
The Global Biodiversity Information Facility GBIF.org (2023b). Occurrence Download 1950-1980. doi: 10.15468/dl.87er2a
The Global Biodiversity Information Facility GBIF.org (2023c). Occurrence Download 1980-2000. doi: 10.15468/dl.r25sp2
The Global Biodiversity Information Facility GBIF.org (2023d). Occurrence Download 2000-2020. doi: 10.15468/dl.nbgka9
The Global Biodiversity Information Facility GBIF.org (2023e). Occurrence Download Before end of 1900. doi: 10.15468/dl.wmdd89
Thioulouse J., Dray S., Dufour A.-B., Siberchicot A., Jombart T., Pavoine S. (2018). Multivariate analysis of ecological data with ade4. New York, NY: Springer New York, 329 pp. doi: 10.1007/978-1-4939-8850-1
Thomsen E., Vorren T. O. (1986). Macrofaunal palaeoecology and stratigraphy in late quaternary shelf sediments off Northern Norway. Palaeogeogr. Palaeoclimatol. Palaeoecol. 56, 103–150. doi: 10.1016/0031-0182(86)90110-0
Trofimov A., Karsakov A., Ivshin V. (2019). “Climate changes in the Barents Sea over the last half century. In “Influence of Ecosystem Changes on Harvestable Resources at High Latitudes,” in The Proceedings of the 18th Russian-Norwegian Symposium, Murmansk, Russia, 5–7 June 2018. Ed. By Shamray E., Huse G., Trofimov A. IMR/PINRO Joint Report Series, No. 1-2019. Bergen/Murmansk.
Vassilenko S. V., Petryashov V. V. (2009). Illustrated Keys to Free-Living Invertebrates of Eurasian Arctic Seas and Adjacent Deep Waters Vol. 1 (AKU (Alaska Sea Grant): University of Alaska Fairbanks), 186 pp.
Venn A. A., Tambutté E., Holcomb M., Laurent J., Allemand D., Tambutté S. (2013). Impact of seawater acidification on pH at the tissue–skeleton interface and calcification in reef corals. Proc. Natl. Acad. Sci. U.S.A. 110, 1634–1639. doi: 10.1073/pnas.1216153110
von Biela V. R., Laske S. M., Stanek A. E., Brown R. J., Dunton K. H. (2023). Borealization of nearshore fishes on an interior Arctic shelf over multiple decades. Global Change Biol. 29, 1822–1838. doi: 10.1111/gcb.16576
Węsławski J. M., Dragańska-Deja K., Legeżyńska J., Walczowski W. (2018). Range extension of a boreal amphipod Gammarus oceanicus in the warming Arctic. Ecol. Evol. 8, 7624–7632. doi: 10.1002/ece3.4281
Wessels B., Finn R. L., Linde P., Mazzetti P., Nativi S., Riley S., et al. (2014). Issues in the development of open access to research data. Prometheus 32, 49–66. doi: 10.1080/08109028.2014.956505
Wold A., Søreide J. E., Svensen C., Halvorsen E., Hop H., Kwasniewski S., et al. (2022). Mesozooplankton biodiversity Nansen Legacy JC1 [Data set]. Norwegian Polar Institute. doi: 10.21334/npolar.2022.f8d4a1cb
Worm B., Lotze H. K. (2009). “Chapter 14 - changes in marine biodiversity as an indicator of climate change,” in Clim. Change. Ed. Letcher T. M. (Elsevier, Amsterdam), 263–279.
Yashayaev I., Seidov D. (2015). The role of the Atlantic Water in multidecadal ocean variability in the Nordic and Barents Seas. Prog. Oceanogr. 132, 68–127. doi: 10.1016/j.pocean.2014.11.009
Yu H., Wang T., Skidmore A., Heurich M., Bässler C. (2022). 50 years of cumulative open-source data confirm stable and robust biodiversity distribution patterns for macrofungi. J. Fungus 8, 981. doi: 10.3390/jof8090981
Zabihi-Seissan S., Baker K. D., Stanley R. R. E., Tunney T. D., Beauchamp B., Benoit H. P., et al. (2024). Interactive effects of predation and climate on the distributions of marine shellfish in the Northwest Atlantic. Oikos, e10524. doi: 10.1111/oik.10524
Zakharov D., Jørgensen (2017). New species of the gastropods in the Barents Sea and adjacent waters. Russ. J. Biol. Invasions 8, 226–231. doi: 10.1134/S2075111717030146
Zakharov D., Jørgensen, Manushin, Strelkova (2020). Barents Sea megabenthos: Spatial and temporal distribution and production. J. Mar. Biol. 5, 19–37. doi: 10.21072/mbj.2020.05.2.03
Zhou W., Leung L. R., Lu J. (2024). Steady threefold Arctic amplification of externally forced warming masked by natural variability. Nat. Geosci 17, 508–515. doi: 10.1038/s41561-024-01441-1
Keywords: Arctic, climate change, Barents Sea, invertebrates, species distribution, distribution shift
Citation: Calvet N, Bluhm BA, Yoccoz NG and Altenburger A (2024) Shifting invertebrate distributions in the Barents Sea since pre-1900. Front. Mar. Sci. 11:1421475. doi: 10.3389/fmars.2024.1421475
Received: 22 April 2024; Accepted: 29 July 2024;
Published: 23 August 2024.
Edited by:
Jan Marcin Weslawski, Polish Academy of Sciences, PolandReviewed by:
Matthew R. Baker, North Pacific Research Board, United StatesJinlin Liu, Tongji University, China
Copyright © 2024 Calvet, Bluhm, Yoccoz and Altenburger. This is an open-access article distributed under the terms of the Creative Commons Attribution License (CC BY). The use, distribution or reproduction in other forums is permitted, provided the original author(s) and the copyright owner(s) are credited and that the original publication in this journal is cited, in accordance with accepted academic practice. No use, distribution or reproduction is permitted which does not comply with these terms.
*Correspondence: Andreas Altenburger, andreas.altenburger@uit.no