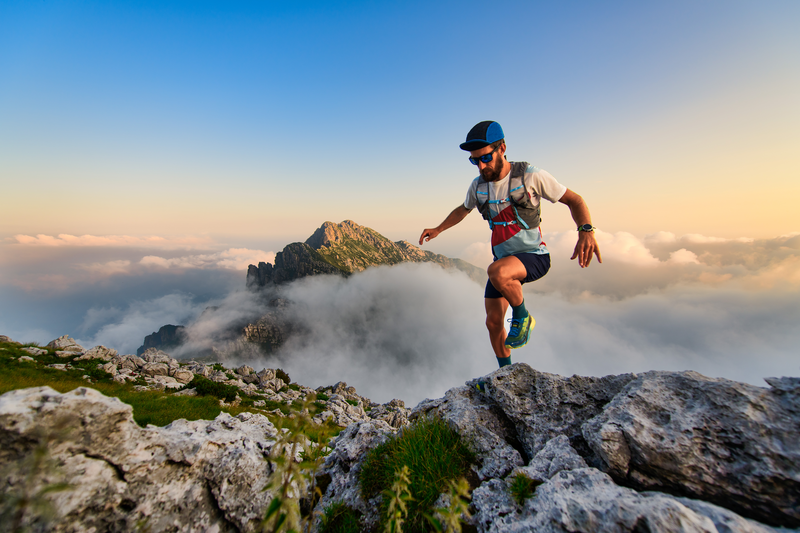
95% of researchers rate our articles as excellent or good
Learn more about the work of our research integrity team to safeguard the quality of each article we publish.
Find out more
ORIGINAL RESEARCH article
Front. Mar. Sci. , 30 September 2024
Sec. Ocean Solutions
Volume 11 - 2024 | https://doi.org/10.3389/fmars.2024.1420095
The intentional combination of two or more marine activities with the purpose of sharing space, infrastructure, resources and/or operations, referred to as multi-use, is gaining attention as a means to reduce the spatial footprint of human activities but possibly also its ecological footprint. In this study, the Spatial Cumulative Assessment of Impact Risk for Management (SCAIRM) method was adapted and applied to assess whether multi-use can reduce the ecological footprint in terms of the cumulative impacts on the marine ecosystem, by integrating multiple offshore activities in different configurations as compared to these activities separated in space, referred to as single-use. These configurations combine renewable energy, aquaculture, nature restoration and tourism activities, in different combinations. For the sake of this multi-use assessment these activities were subdivided into actions, their allocation in space and time represented in scenarios (e.g. single-use versus multi-use) which were then evaluated in terms of their ecological footprint (i.e. Impact Risk). The main finding is that the calculated Impact Risk in multi-use is often lower than that in single-use and in any case never higher. This study also shows that there is still much to be gained in terms of further reduction in Impact Risk through an optimization of the multi-use design by comparing the scenario based on actual pilots deemed more realistic (i.e. co-existence with limited synergies) with a hypothetical optimal scenario (i.e. multi-functional).
As demand for resources continues to grow and land-based sources decline, the use of marine resources is increasing, resulting in a race among diverse and often competing interests for ocean food, material, and space (Jouffray et al., 2020). Increased pressure on the marine environment has led to two important types of conflict: 1) competition between users for ocean space or having adverse effects on each other (user vs user conflicts) and 2) the cumulative impact of all these activities on the marine environment (user–environment conflicts) (Douvere and Ehler, 2009). The latter being more important as human-dominated marine ecosystems are experiencing accelerating loss of populations and species (Worm et al., 2006).
Within Europe, Multi-Use (MU) has been promoted, amongst others via the Blue Growth Strategy (European Commission, 2017) and the Maritime Spatial Planning (MSP) Directive (European Commission, 2014a), as a concept to reduce the spatial footprint of pressures on European Seas and create new opportunities for socio-economic development, along with potential environmental benefits (Schultz-Zehden et al., 2018). Marine, or ocean, MU is defined as the intentional combination of two or more marine activities, with the purpose of sharing space, infrastructure, resources and/or operations (Przedrzymirska et al., 2021). Other terms to describe MU are multiple-use, co-use, coexistence, interdependencies and co-location, which are sometimes used interchangeably (Przedrzymirska et al., 2021). As MU can relate to spatial proximity, overlap or concurrence, or economic interaction it is considered an umbrella term that covers a multitude of use combinations in the marine realm (Schupp et al., 2019). Schupp et al. (2019) identified four dimensions in which connections in MU occur: spatial, temporal, provisional, and functional. Based on these dimensions different types of MU can be defined (Figure 1) that range from the lowest level of connectivity (spatial proximity/coexistence) to the highest level of connectivity (multi-functionality). Multi-functionality is characterized by its need for a joint development processes (e.g. inclusive planning and joint licensing procedure, from start to finish), in order to account for every individual users needs, rights, and finally responsibilities in regard to any joint assets. For example, offshore aquaculture devices could be structurally integrated in offshore wind farms (Buck et al., 2017; Danovaro et al., 2024).
Figure 1. Schematic presentation of four different scenarios and use combinations (after Schupp et al. (2019)) of the sectoral activities considered in this study. Sharing is possible in the four dimensions: space, time (together represented by the green symbol), servicing (yellow symbol) and infrastructure/main functions (blue symbol). Scenario Single-use (SU) is represented by scheme I and assumes no connectivity between activities. Scenario MU 1 is represented by scheme II and III and assumes co-existence with a limited symbiotic use (some sharing of servicing). Scenario MU 2 is represented by scheme IV (sharing all dimensions) and assumes the highest level of connectivity between the involved activities, including sharing of servicing and structures/functions).
Several European projects have explicitly focused on MU, such as EU-FP7 projects TROPOS (Modular Multi-use Deep Water Offshore Platform Harnessing and Servicing Mediterranean, Subtropical and Tropical Marine and Maritime Resources), MERMAID (Innovative Multi-purpose off-shore platforms: planning, Design and operation) and H2OCEAN (Development of a wind-wave power open-sea platform equipped for hydrogen generation with support for multiple users of energy) and the EU-H2020 projects MARIBE (Marine Investment for the Blue Economy), MUSES (Multi-Use of European Seas Project), Space@Sea (Multi-use affordable standardized floating Space at Sea) and UNITED (Multi-Use offshore platforms demonstrators for boosting cost-effective and eco-friendly production in sustainable marine activities). To date, the main focus of MU projects has been on technical, social, economic and environmental aspects of MU which provided a good basis for the MU concept development (Airoldi et al., 2016; El Serafy, 2020; European Commission, 2018; Flikkema and Waals, 2019; van Hoof et al., 2020; Papandroulakis et al., 2017; van den Burg et al., 2020). For example, the EU projects TROPOS and MERMAID provided specific information regarding environmental impacts of floating islands and their applications (Airoldi et al., 2016; TROPOS, 2014), which has been used together with new insights within the Space@Sea project to develop an environmental impact assessment for floating island applications (Tamis et al., 2021). Another example is the MUSES project, that increased the knowledge base on environmental interactions with tidal energy (Sangiuliano, 2018). However, implementation of MU is still in its infancy (Bocci et al., 2019; Stancheva et al., 2022). A recent review of support tools to assess ocean multi sectoral interactions identified a need for development of approaches that can integrate across different sectors (Turschwell et al., 2022). Within UNITED, MU of marine areas is encouraged by developing guidelines, tools and experience through five pilots in three European seas (Baltic Sea, North Sea and Mediterranean Sea). These pilots combine renewable energy, aquaculture, nature restoration and tourism activities in different combinations, with the aim to provide evidence for the viability of marine MU (Kerkhove et al., 2021; Van Gerven et al, in prep.). More specifically, the pilots aim to: demonstrate in practice the benefits of the MU of offshore wind farms and aquaculture activities; investigate the possibilities of floating solar panels and seaweed aquaculture offshore; improve the design and deployment methods for offshore aquaculture (shellfish and seaweed) activities combined with restoration (flat oyster) at offshore wind farms; investigate possibilities to expand tourism activities at offshore wind farms and tourism activities at aquaculture sites (Brouwers et al., 2020). The EIA (Environmental Impact Assessment) Directive (2011/92/EU as amended by 2014/52/EU) requires Member States to ensure that projects likely to have significant effects on the environment, such as the installation of offshore infrastructure, are subject to an assessment of their environmental effects, which should take place before development consent is given by the authorities. EIA should focus on ensuring ‘no-net-loss’ of biodiversity and avoiding effects from the start, before considering mitigation, with compensation being used as a last resort (European Commission, 2013). As such, MU can be regarded as a concept to advance towards this goal. In addition to the existing EIA guidance and tools, the Ocean Multi-Use Assessment Framework (OMUAF) was developed (Van Gerven et al., 2024)1, The OMUAF is designed to allow for comparisons between single-use (SU, i.e. activities are taking place simultaneously but in different exclusive areas) and MU approaches in an integrated manner based on three pillars of sustainable development: an environmental, societal and an economic assessment. In addition to these three pillars, two categories of conditions must be met: technological feasibility and regulatory appropriateness (legal, policy and governance) (Van Gerven et al., 2024)1. This study addresses the development of the third pilar for the OMUAF; the environmental assessment.
Because MU involves at least two marine sectors or activities being together, an assessment of the potential impacts of all involved activities and their pressures is essential. Cumulative effect assessments (CEAs) and/or cumulative impact assessments (CIAs), are both defined as: “a systematic procedure for identifying and evaluating the significance of effects from multiple pressures and/or activities on single or multiple receptors” (Judd et al., 2015). As such, they are therefore considered the main tools to fulfill that role (Halpern and Fujita, 2013; Piet et al., 2023; Stelzenmüller et al., 2018; Tamis et al., 2016). CIAs are also recommended to inform an Ecosystem Approach to MSP (European Commission, 2014b; European Commission et al., 2021). The Spatial Cumulative Assessment of Impact Risk for Management (SCAIRM) method (Piet et al., 2023) is a novel CIA approach for the marine ecosystem and is here applied to assess the Impact Risk of multiple offshore activities in different configurations. These configurations are based on actual pilots but to avoid confounding location-specific effects, the configurations are assumed to occur in a hypothetical area in the southern North Sea. Hence, this study aims to assess whether MU as applied in the UNITED pilots (Brouwers et al., 2020) can reduce the ecological footprint in terms of the cumulative impacts on the marine ecosystem.
As part of the UNITED project, this study is subjected to MU applied in five pilots in the Baltic Sea, North Sea and Mediterranean Sea, with different environmental and ecological conditions (e.g. depth, salinity. seabed substrates, species). These differences in ecosystems may affect the environmental impact of human activities. Because this study is aimed to compare the Impact Risk of MU versus SU and not necessarily to assess the local Impact Risk of the pilots, there is no need to address differences related to location. Therefore, to avoid unnecessary complexity, all pilots are assumed to be applied in one European Sea. The study area is defined as a hypothetical area of 22 km2 (comprising area for the activity and area for the potential dispersal of pressures) in the southern North Sea consisting of two habitats, i.e. sublittoral sediment and pelagic water column, and three species groups, i.e. fish, birds, and marine mammals. The size of the study area is based on the pilot with the largest spatial footprint, the Offshore Windfarm (OWF) Belwind (i.e. 17 km2, Table 1) buffered with a zone surrounding the area to account for maximum potential dispersal of the pressures. Belwind is an existing OWF in Belgium and is included in one of the five pilots of the UNITED project (Brouwers et al., 2020).
Table 1. Activities and extent of their areas (km2) for the five configurations under Single-Use (SU) and Multi-Use (MU) scenarios.
The different combinations of ten activities divided over four sectors are represented by configuration A to E (Table 1). These activities and configurations are based on the UNITED practical pilots (Brouwers et al., 2020), with two exceptions: 1) each configuration is assumed to occur in a hypothetical area (as defined above) and 2) the OWF of configuration A (based on the pilot with OWF Belwind) is representative for OWF in all configurations. These adaptations are for simplicity reasoning and enable a better comparison between the environmental impact of MU versus SU. In addition to the selection of activities and their combinations, also the required areas (Table 1) are based on the practical pilots of UNITED.
Scenarios then describe how the configurations of these activities are arranged in space and time. Three scenarios are applied to all configurations A to E: The first scenario represents SU (Figure 1, scheme I), for the configurations (assuming no sharing). This scenario is used for comparison to the MU scenarios to assess the change in environmental impact between SU and MU configurations. The second scenario represents MU for the configurations operating in a MU context. Since translating the concept of MU into practice has proven to be challenging (Guyot-Téphany et al., 2024), a low level of sharing is assumed for current MU (Figure 1, scheme II and III). However, this scenario does not reflect the full potential of MU as reflected by a multifunctional design with the highest level of connectivity between the involved activities (Figure 1, scheme IV). Therefore, an additional MU scenario is used that represents MU for the configurations operating with the highest possible level of connectivity. The two distinct MU scenarios thus follow the different types of MU as defined by Schupp et al. (2019) with 1) current practice represented by co-existence, characterized by a moderate to low degree of connectivity between the involved activities and a limited symbiotic use (sharing is possible for only some actions related to servicing) and 2) optimal represented by multi-functionality, characterized by the highest level of connectivity between the involved activities, including sharing of structures and/or installations.
Following the four MU dimensions by Schupp et al. (2019) (spatial, temporal, provisional, and functional, Figure 1), the combinations of activities may involve sharing space (here reflected in the reduction of area extent and hence spatial footprint, Table 1), time (here reflected in duration, Supplementary Material SM1), provisions and/or functions (here reflected by the actions, Supplementary Material SM2).
These scenarios are subsequently evaluated in terms of the resulting Impact Risk for each relevant Ecosystem Component (i.e. species and habitats) to test if MU can potentially reduce environmental impact and to which extent.
In addition to the scenarios applied to the configurations, a baseline scenario is included. The baseline consists of North Sea activities but without any of the activities used in the various configurations. It is used to describe the average environmental impact in the study area prior to application of the configurations (Table 1). A set of common (“business as usual”) activities at average intensities on the North Sea was used from Piet et al. (2023) to represent the baseline scenario: Angling; Boating/Yachting/Watersports (with and without engine); Commercial fishing (Benthic trawls and dredges; Nets (fixed/set/gillnets/other nets/lines); Pelagic trawls); and Shipping. These baseline activities are not part of the configurations (Table 1) and it is assumed that these will be excluded from the area.
The surface area required for each activity in the configuration is indicated for SU and MU scenario (Table 1) where SU assumes no spatiotemporal connectivity between activities and MU assumes connectivity between activities (Figure 1). The boundary conditions for the scenarios are specified such that they have the same yield of the desired commercial products from the activities. Three project phases are distinguished for the configurations: installation phase (e.g. turbine installation and laying of cables), operational phase (e.g. wind energy production and presence of cables) and decommissioning phase (e.g. removal of turbines and cables).
In the configurations A to D, wind farms are considered to be the basic activity designated to occupy that specific area and in configuration E (the only configuration without a wind farm) the basic activity is aquaculture (see Table 1). Other activities may be combined with the basic activity and will thus be situated within the area designated for wind farms or aquaculture, as additional activities. As such, of these other activities do not require additional space, and their spatial extent is maximally set to zero if entirely confined within the wind farm or aquaculture area. For example, when combining solar energy with offshore wind energy (configuration D), bundling of electricity cables reduces the spatial footprint. However, some actions are exclusive to a specific activity, or are not expected to be shared with other activities. For example, aerial structures are only applicable for wind farms in the form of wind turbines therefore spatial sharing with other activities (hence extent reduction) is not applicable. Another example is foundations and installations which can only be shared spatially under a multi-purpose/functional MU scenario (Figure 1). Servicing may be shared resulting in a reduced duration of the actions (Supplementary Material SM1). For example, activities in MU can share shipping time, thereby reducing costs and disturbances. For each of the actions in a specific configuration it is indicated if sharing is expected in the two MU scenarios (Supplementary Material SM2). In case sharing is expected, the extent and duration expected in MU apply for that specific action (see Table 1 and Supplementary Material SM1, respectively). In case no sharing is expected the extent and duration as in SU apply for that specific action. For configuration A (wind energy, aquaculture (macro-algae and shellfish) and nature development) 44 actions are required of which 10 are expected to change in scenario MU 1 (limited sharing) and 26 are expected to change in scenario MU 2 (highest level of sharing). The same is expected for configuration B (wind energy and aquaculture (macro-algae and shellfish)). Less actions (38) are required for configuration C (wind energy and tourism (diving, day trips and touristic fishing) of which 4 are expected to change in scenario MU 1, and 8 are expected to change in scenario MU 2. For configuration D (wind energy, solar energy and aquaculture (macro-algae) most actions are required (46) of which 14 are expected to change in scenario MU 1 and 27 are expected to change in scenario MU 2. Configuration E (aquaculture (finfish) and tourism (diving)) requires 36 actions of which 3 are expected to change in scenarios MU 1 and MU 2.
To assess the potential impacts on ecosystem components use is made of the SCAIRM method (Piet et al., 2023). At the basis of SCAIRM is a linkage framework consisting of impact chains that link causes from stressors to impacts on receptors via the three main elements, i.e. activities, pressures and ecosystem components. Human activities are sectoral at their basic level (e.g. fishing, renewable energy) but can be further sub-divided into operations. Pressures (e.g. abrasion, noise) represent the mechanism through which human activities interact with the ecosystem. The ecosystem components include (at the most basic level) pelagic habitats, benthic habitats and species groups (birds, mammals, reptiles, fish, cephalopods).
The framework includes a total of 42,333 impact chains covering all North Sea activities, pressures and ecosystem components (Piet et al., 2023). These impact chains cover the baseline (2,487 impact chains related to fisheries, shipping and tourism) and activities selected for the configurations (1,245 impact chains related to offshore wind, aquaculture, solar, oyster reef restoration and tourism). To explore the potential combinations in MU, the activities defined in SCAIRM (sector activities and their operations) are split up into actions to provide a higher level of detail, e.g. on the installation of foundations, manual labor etc. See supplemental material (Supplementary Material SM3) for a description of all actions. Because of this introduced level of detail, the number of impact chains increased from 1,245 to 6,791 (Supplementary Material SM4). This level of detail is required to describe how functions and provisioning are shared between sectors, and therewith distinguish between the different scenarios.
The key concept of SCAIRM is Impact Risk, reflecting the expected pressure-induced loss of a specific ecosystem component relative to an undisturbed situation for that specific assessment period (Piet et al., 2023). Impact Risk is estimated per impact chain as a function of Exposure and Effect Potential (Piet et al., 2023) after one year of exposure (Supplementary Material SM5).
Exposure reflects the chance of co-occurrence of the ecosystem component (receptor) and the pressure from an activity (stressor), representing the proportion of an ecosystem component (percentage or fraction of its distribution area or population) that may be perturbed by the pressure (Piet et al., 2023). For the configurations, the areas as presented in Table 1 are used, in proportion to the study area (activity extent) complemented with pressure-specific dispersal scores (Piet et al., 2023). Ecosystem components were assumed to be evenly distributed within the study area. For the temporal overlap the applied extent and dispersal and hence estimated Exposure were adjusted to account for the duration of the activities (i.e. multiplied by the fraction of the year that activities occur).
In case of Exposure, the Effect Potential then reflects the degree of impact expressed as the expected change in abundance (numbers, biomass) of the ecosystem component assuming that each spatial unit is a closed system with no exchange with its surroundings. The Effect Potential is assessed using data available in SCAIRM for the North Sea (Piet et al., 2023), see Supplementary Material SM5.
The SCAIRM output is basically an aggregation of Impact Risk across impact chains and thus cumulative pressures (Piet et al., 2023):
Where IR = Impact Risk, EC = Ecosystem Component, P = Pressure, A = Activity
This means that for the baseline and for each configuration, the total Impact Risk is estimated for each Ecosystem Component by summation of the Impact Risk of all impact chains related to the involved activities.
The cumulative impacts from a set of common activities being part of the baseline on the North Sea shows that fish suffer the biggest threat followed by benthic habitats (Figure 2, left). Benthic fisheries contribute most to the Impact Risk for all ecosystem components. Comparing the Impact Risk between all activities, including activities operated in SU configuration (Figure 2, right), shows that for all ecosystem components except birds, maximum Impact Risk is posed by benthic fisheries in the baseline. From the operation of SU activities, birds endure the biggest threat. Wind farms contribute most to the Impact Risk (birds), followed by finfish culture (fish and marine mammals).
Figure 2. Impact Risk from activities on ecosystem components in the Baseline (North Sea average, left) and SU configurations (right). Activities included in this baseline are taken from Piet et al. (2023) and include: Angling; Boat_eng (boating/yachting, with engine); Boat_no (boating/yachting/water sports, without engine); Fish_Bent (benthic trawls and dredges); Fish_Net (nets, fixed/set/gillnets/other nets/lines); Fish_Pel (pelagic trawls); Ship (shipping). The Impact Risk of SU activities that are included in more than one configuration (wind farms, shellfish, macro-algae, Table 1) represents a worst case, i.e. the maximum Impact Risk is used.
The Impact Risk on Ecosystem Components (ECs) from activities in SU, MU 1 (Co-existence with limited symbiotic use) and MU 2 (Multi-functional) for each configuration distinguishing an installation, operational, and decommissioning phase (Figure 3) reveals that for all ECs except sublittoral sediment the highest Impact Risk is expected for the operational phase. For sublittoral sediment the highest Impact Risk is expected for the installation phase. In most configurations, wind farms cause highest Impact Risk for sublittoral sediment, fish, mammals and birds (Figure 4). Fish culture causes highest Impact Risk for the pelagic water column, fish and mammals. The Impact Risk in MU is often lower and in any case never higher than the Impact Risk in SU. Differences between Impact Risk of SU and Impact Risk of MU are most pronounced for the installation phase of the configurations that combine oyster reefs (configuration A) and solar platforms (configuration D) with wind farms. The Impact Risk in MU 2 is never higher than the Impact Risk in MU 1 and is in some cases lower. The highest difference between Impact Risk in MU 1 and MU 2 is shown for the sublittoral sediment in configuration D, which is mainly related to the combination of solar platforms with wind farms.
Figure 3. Impact Risk on five ecosystem components in configuration (A–E) in Single Use and Multi Use aggregated per phase. Multi Use: 1 = Co-existence with limited symbiotic use and; 2 = Multi-functional.
Figure 4. Impact Risk on five ecosystem components in configuration (A–E) in Single Use and Multi Use aggregated per activity. Multi Use: 1 = Co-existence with limited symbiotic use and; 2 = Multi-functional.
This study shows that the ecological footprint (i.e. Impact Risk) posed by the common activities in the baseline situation (fishing, shipping, tourism) exceeds that from activities operated in SU configuration, except for birds which are strongly impacted by wind farms. Therefore, if existing common activities are excluded within the area of a configuration (and hence assumed removed from the ecosystem, not reallocated), it can be expected that for all ecosystem components, except birds, the total Impact Risk in the operational phase of the configuration area will be reduced compared to the situation prior to the configuration development (i.e. baseline situation).
The Impact Risk of a suite of activities, captured in a specific configuration, shows that in many cases the estimated Impact Risk in MU is lower than the Impact Risk in SU and it is never higher. This is expected as only reductions in the spatial extent and/or the duration of activities are foreseen, which are the two variables in the assessment of Impact Risk for SU compared to MU. Differences between Impact Risk of SU and Impact Risk of MU are most pronounced for the installation phase of the configurations that combine oyster reefs and solar platforms with wind farms. Installation activities affect benthic habitats by e.g. abrasion/damage for which the Impact Risk of the combined installation is much less than individual installations. Minor reductions of Impact Risk are expected in the operational phase. However, since the (reduction of) Impact Risk is based on a yearly exposure this could ultimately result in a significant reduction considering the full operational lifespan of the initiative (an anticipated 15 years or more). The highest difference between Impact Risk in MU 1 (co-existence with limited symbiotic use) and MU 2 (multi-functional) is shown for the sublittoral sediment in configuration D, which is mainly related to the combination of solar platforms with wind farms. These are both renewable energy activities and show the greatest reduction of Impact Risk in a multi-functional type of MU where structures such as electricity cables (infield and export) are shared. Assuming co-existence with limited symbiotic use is currently a more realistic scenario, and therefore there is still much to be gained through a (further) optimization of the MU design. For example, offshore aquaculture devices could be structurally embedded in the offshore wind turbine infrastructure (Buck and Langan, 2017). Multi-functionality requires a joint development process (inclusive planning and joint licensing procedure, from start to finish) in order to account for every individual user’s needs, rights, and finally responsibilities in regard to any joint asset (Schupp et al., 2019). Obstacles during this process, in the form of legal aspects, insurance, risk and uncertainty, and the overall governance of multi-use, determine whether multi-use can be implemented successfully and be financially viable (Ciravegna et al., 2024).
In this study, the SCAIRM methodology (Piet et al., 2023) has been adapted and applied to assess the environmental impact of MU. While the method was initially intended for studies on ecosystem scale (i.e. regional seas), this is the first application of SCAIRM at the local scale. Additional detail deemed necessary for these assessments has been obtained by dividing the activities into actions. Also, this is the first time that the SCAIRM method has been used to assess the change in cumulative impacts that can be achieved by integrating multiple activities and functions (i.e. Multi-Use). For this latter application, the method has been further developed and extended to include the combinations of actions using the four dimensions as defined by (Schupp et al., 2019): space, time, provisions and/or functions. The methodology has been applied in a representative hypothetical area in the southern North Sea comprising the two most common habitats, i.e. sublittoral sediment and pelagic water column, and three species groups, i.e. fish, birds, and marine mammals. This assumed identical environment simplified the assessment and allowed the comparison of Impact Risk between configurations to focus on differences between SU and MU and not on differences between local conditions. Application of a representative hypothetical area for such assessments proved useful where application in other areas and/or regions than the North Sea might involve other habitats such as littoral sediment or rocks and other hard substrates, and other species groups such as reptiles.
Regarding this type of CIA, the spatial extent relates to the proportion surface area of the study area in which both the pressure and ecosystem component co-occur. The Impact Risk is assessed assuming linear additivity. Within CIA approaches, the assumption of additivity is generally accepted (Judd et al., 2015; Stelzenmüller et al., 2018; Piet et al., 2023) and is currently the best available technique. This implies that the Impact Risk of all impact chains affecting an ecosystem component are summed and possible synergistic and antagonistic effects (Crain et al., 2008) are ignored.
The CIA applied here with SCAIRM only addresses potential negative impacts on ecosystem components. However, it has been suggested that MU might generate positive environmental or even social impacts as well, including: more diverse species assemblages thanks to an artificial reef effect, an increase in commercial fish species abundance, changes in nutrient cycling leading to less eutrophication, carbon sequestration from organic matter accumulating on the seafloor if the seafloor remains undisturbed, sustainable food production, increased renewable energy production per amount of space dedicated for renewable energy production thanks to the combination of wind turbines and floating solar panels, more space for conservation elsewhere thanks to the combination of space-demanding activities, a reduction of conflicts over space use and an increased social acceptance of offshore wind farms and aquaculture (Van Gerven et al., 2023). Potential positive environmental impacts are thus relevant in the evaluation and prediction of the impact of ocean multi-use and hence included in the UOMAF (Van Gerven et al., 2024)1. Besides (positive and negative) environmental impacts, the UOMAF also includes other key pillars of assessment, i.e. economic and social, and addresses technological and regulatory conditions therewith providing a holistic view of the impacts and benefits associated with MU scenarios. This holistic view is especially important in the context of MSP, which is a political and social management process informed by both the natural and social sciences for the distribution of human activities in space and time to achieve ecological, economic and social objectives and outcomes (Ehler et al., 2019).
Information to properly evaluate any hypothetical optimal MU scenarios is currently limited or even lacking but during commercial rollout more information is expected to become available, for example specific structural designs, details of proposed activities, but also regarding specific locations including geographical and environmental information. The SCAIRM method allows harmonization of qualitative and quantitative approaches into a single method, which has the advantage that it is comprehensive and can be used in data-poor situations while allowing the use of available quantitative information in more data-rich situations (Piet et al., 2023). The SCAIRM method as applied in this study can thus be used throughout the commercial rollout process. Moreover, it has been considered an advantage to conduct the (cumulative) environmental impact assessment at a high strategic level even at an early stage when data are still limited and apply it consistently throughout the sequential levels of decision making (Partidário, 2000; Tamis et al., 2016). Since MU may cover many combinations of activities involving spatial, temporal, provisional, and functional dimensions (Schupp et al., 2019) the SCAIRM method as applied here can be used as a tool for communication between users and to help identify possible beneficial connections.
This study contributes to the MU knowledge base and may help implementation of MU in spatial planning aimed at reducing environmental impacts. The presented methodology can easily be adopted in future studies to explore other MU scenarios, as well as be applied to other regions. The differences in environmental impact caused by MU and how this is determined by various assumptions regarding spatial and temporal aspects of MU indicates that it is important to consider these assumptions in the commercial rollout and to discuss with sector representatives or researchers the possibilities for optimizing the design of activities and their actions in practice.
The datasets presented in this study can be found in online repositories. The names of the repository/repositories and accession number(s) can be found in the article/Supplementary Material.
JT: Conceptualization, Methodology, Visualization, Writing – original draft, Writing – review & editing. RHJ: Conceptualization, Methodology, Writing – original draft, Writing – review & editing. MR: Conceptualization, Writing – review & editing. AG: Methodology, Visualization, Writing – review & editing. PV: Conceptualization, Data curation, Methodology, Visualization, Writing – original draft, Writing – review & editing. AVG: Writing – review & editing. RGJ: Conceptualization, Writing – review & editing. GP: Conceptualization, Methodology, Supervision, Writing – review & editing.
The author(s) declare financial support was received for the research, authorship, and/or publication of this article. We acknowledge any financial support or funding received for this research. The European Union’s Horizon 2020 Research and Innovation Programme under Grant Agreement n° 862915 provided financial support for this study. The funders had no role in the study design, data collection, analysis, decision to publish, or preparation of the manuscript.
This study is part of the UNITED project and has received funding from the European Union’s Horizon 2020 Research and Innovation Programme under Grant Agreement n° 862915. All authors actively contributed to the conception, design, and execution of this article.
The authors declare that the research was conducted in the absence of any commercial or financial relationships that could be construed as a potential conflict of interest.
All claims expressed in this article are solely those of the authors and do not necessarily represent those of their affiliated organizations, or those of the publisher, the editors and the reviewers. Any product that may be evaluated in this article, or claim that may be made by its manufacturer, is not guaranteed or endorsed by the publisher.
The Supplementary Material for this article can be found online at: https://www.frontiersin.org/articles/10.3389/fmars.2024.1420095/full#supplementary-material
Airoldi L., Møhlenberg F., Evriviadou M., Jimenez C., Hansen B., Dávila O. G., et al. (2016). “EIA Manual for MUOP (Multi Use Offshore Platforms),” in Mermaid Project Report ID 3.5 to the European Commission, (Belgium: European Commission).
Bocci M., Sangiuliano S. J., Sarretta A., Ansong J. O., Buchanan B., Kafas A., et al. (2019). Multi-use of the sea: A wide array of opportunities from site-specific cases across Europe. PLoS One 14, e0215010. doi: 10.1371/journal.pone.0215010
Brouwers E., Meijer N., Strothotte E., Jaeger M., Declercq A. M., Drigkopoulou I., et al. (2020). Review of pilot TRL, legal aspects, technical solutions and risks. Available online at: https://www.h2020united.eu/images/PDF_Reports/D71_Review_of_pilot_TRL_legal_aspects_technical_solutions_and_risks_revised_after_ECReview_Dec2021_220207.pdf (Accessed February 20, 2024).
Buck B. H., Krause G., Pogoda B., Grote B., Wever L., Goseberg N., et al. (2017). “The german case study: pioneer projects of aquaculture-wind farm multi-uses,” in Aquaculture Perspective of Multi-Use Sites in the Open Ocean (Cham: Springer International Publishing), 253–354. doi: 10.1007/978-3-319-51159-7_11
Buck B. H., Langan R. (Eds.) (2017). Aquaculture Perspective of Multi-Use Sites in the Open Ocean: The Untapped Potential for Marine Resources in the Anthropocene (Cham: Springer Nature), 413. doi: 10.1007/978-3-319-51159-7_7
Ciravegna E., Hoof L., Frier C., Maes F., Rasmussen H. B., Soete A., et al. (2024). The hidden costs of multi-use at sea. Mar. Policy 161. doi: 10.1016/j.marpol.2024.106017
Crain C. M., Kroeker K., Halpern B. S. (2008). Interactive and cumulative effects of multiple human stressors in marine systems. Ecol. Lett. 11, 1304–1315. doi: 10.1111/j.1461-0248.2008.01253.x
Danovaro R., Bianchelli S., Brambilla P., Brussa G., Corinaldesi C., Borghi A. D., et al. (2024). Making eco-sustainable floating offshore wind farms: Siting, mitigations, and compensations. Renewable Sustain. Energy Rev. 197, 114386. doi: 10.1016/j.rser.2024.114386
Douvere F., Ehler C. N. (2009). New perspectives on sea use management: Initial findings from European experience with marine spatial planning. J. Environ. Manage. 90, 77–88. doi: 10.1016/j.jenvman.2008.07.004
Ehler C., Zaucha J., Gee K. (2019). “Maritime/Marine Spatial Planning at the Interface of Research and Practice” in: Maritime Spatial Planning Zaucha J., Gee K. (eds). (Cham: Palgrave Macmillan).
El Serafy G. (2020). The UNITED Project and Multi-Use. Available online at: https://www.h2020united.eu/images/Webinar_Reports/UNITED-2020-06-03-Webinar_Introduction_El-Serafy.pdf (Accessed January 2, 2024).
European Commission (2013). Guidance on integrating climate change and biodiversity into environmental impact assessment. OJ L 257, 28.8.2014 (Publications Office), p. 135–145.
European Commission (2014). Directive 2014/89/EU of the European Parliament and of the Council of the 23 July 2014 establishing a framework for maritime spatial planning. (Belgium: European Commission).
European Commission (2017). Blue Growth Strategy (SWD, (2017) 128 final)—Report on the Blue Growth Strategy Towards More Sustainable Growth and Jobs in the Blue Economy. (Belgium: European Commission).
European Commission (2018). Development and demonstration of an automated, modular and environmentally friendly multi-functional platform for open sea farm installations of the Blue Growth Industry. Available online at: https://cordis.europa.eu/project/id/774426/fr (Accessed January 2, 2024).
European Commission, European Climate, I. and E. E. A., Ruskule A., Oulès L., Zamparutti T., et al. (2021). Guidelines for implementing an ecosystem-based approach in maritime spatial planning – Including a method for the evaluation, monitoring and review of EBA in MSP (Belgium: European Commission). doi: 10.2926/84261
Flikkema M., Waals O. (2019). Space@Sea the floating solution. Front. Mar. Sci. 6. doi: 10.3389/fmars.2019.00553
Guyot-Téphany J., Trouillet B., Diederichsen S., Juell-Skielse E., Thomas J.-B. E., McCann J., et al. (2024). Two decades of research on ocean multi-use: Achievements, challenges and the need for transdisciplinarity. NPJ Ocean Sustainability 3, 8. doi: 10.1038/s44183-024-00043-z
Halpern B. S., Fujita R. (2013). Assumptions, challenges, and future directions in cumulative impact analysis. EcoSphere 4, 1–11. doi: 10.1890/ES13-00181.1
Jouffray J.-B., Blasiak R., Norström A. V., Österblom H., Nyström M. (2020). The blue acceleration: the trajectory of human expansion into the ocean. One Earth 2, 43–54. doi: 10.1016/j.oneear.2019.12.016
Judd A. D., Backhaus T., Goodsir F. (2015). An effective set of principles for practical implementation of marine cumulative effects assessment. Environ. Sci. Policy 54, 254–262. doi: 10.1016/j.envsci.2015.07.008
Kerkhove T., Degraer S., Rozemeijer M., Jak R., Poelman M., Declercq A., et al. (2021). Assessment framework to determine ecological feasibility of Multi-Use platforms. Available online at: https://ec.europa.eu/research/participants/documents/downloadPublic?documentIds=080166e5e0647aea&appId=PPGMS (Accessed February 15, 2024).
Papandroulakis N., Thomsen C., Mintenbeck K., Mayorga P., Hernández-Brito J. J. (2017). “The EU-project “TROPOS.“,” in Aquaculture Perspective of Multi-Use Sites in the Open Ocean: The Untapped Potential for Marine Resources in the Anthropocene (Cham: Springer International Publishing), 355–374. doi: 10.1007/978-3-319-51159-7_12
Partidário M. R. (2000). Elements of an SEA framework—Improving the added-value of SEA. Environ. Impact Assess. Rev. 20, 647–663. doi: 10.1016/S0195-9255(00)00069-X
Piet G., Grundlehner A., Jongbloed R., Tamis J., de Vries P. (2023). SCAIRM: A spatial cumulative assessment of impact risk for management. Ecol. Indic. 157, 111157. doi: 10.1016/j.ecolind.2023.111157
Przedrzymirska J., Zaucha J., Calado H., Lukic I., Bocci M., Ramieri E., et al. (2021). Multi-use of the sea as a sustainable development instrument in five EU sea basins. Sustainability 13, 8159. doi: 10.3390/su13158159
Sangiuliano S. J. (2018). Analysing the potentials and effects of multi-use between tidal energy development and environmental protection and monitoring: A case study of the inner sound of the Pentland Firth. Mar. Policy 96, 120–132. doi: 10.1016/j.marpol.2018.08.017
Schultz-Zehden A., Lukic I., Ansong J. O., Altvater S., Bamlett R., Barbanti A., et al. (2018). Ocean Multi-Use Action Plan (Edinburgh: MUSES project). Available at: https://2020.submariner-network.eu/images/projects/MUSES/MUSES_Multi-Use_Action_Plan.pdf.
Schupp M. F., Bocci M., Depellegrin D., Kafas A., Kyriazi Z., Lukic I., et al. (2019). Toward a common understanding of ocean multi-use. Front. Mar. Sci. 6. doi: 10.3389/fmars.2019.00165
Stancheva M., Stanchev H., Zaucha J., Ramieri E., Roberts T. (2022). Supporting multi-use of the sea with maritime spatial planning.The case of a multi-use opportunity development—Bulgaria, Black Sea. Mar. Policy 136. doi: 10.1016/j.marpol.2021.104927
Stelzenmüller V., Coll M., Mazaris A. D., Giakoumi S., Katsanevakis S., Portman M. E., et al. (2018). A risk-based approach to cumulative effect assessments for marine management. Sci. Total Environ. 612, 1132–1140. doi: 10.1016/j.scitotenv.2017.08.289
Tamis J. E., Jongbloed R. H., Piet G. J., Jak R. G. (2021). Developing an environmental impact assessment for floating island applications. Front. Mar. Sci. 8. doi: 10.3389/fmars.2021.664055
Tamis J. E., Vries P., Jongbloed R. H., Lagerveld S., Jak R. G., Karman C. C., et al. (2016). Toward a harmonized approach for environmental assessment of human activities in the marine environment. Integrated Environ. Assess. Manage. 12, 632–642. doi: 10.1002/ieam.1736
TROPOS. (2014). Report on Environmental Impact Assessment and Mitigation Strategies. (Richland, WA: PNNL, University Bremen/MARUM). Available at: https://tethys.pnnl.gov/sites/default/files/publications/Tropos_D6.2_Report.pdf.
Turschwell M., Hayes M., Lacharité M., Abundo M., Adams J., Blanchard J., et al. (2022). A review of support tools to assess multi-sector interactions in the emerging offshore Blue Economy. Environ. Sci. Policy 133, 203–214. doi: 10.1016/j.envsci.2022.03.016
van den Burg S. W. K., Schupp M. F., Depellegrin D., Barbanti A., Kerr S. (2020). Development of multi-use platforms at sea: Barriers to realising Blue Growth. Ocean Eng. 217, 107983. doi: 10.1016/j.oceaneng.2020.107983
Van Gerven A., Rozemeijer M. J. C., Jongbloed R., Tamis J., Patel T., Kerkhove T., et al. (2023). “Environmental impact assessment models for the commercial rollout of Multi-Use Platforms.” in European Horizon 2020 UNITED project, Deliverable 4.4. (RBINS, Brussels).
van Hoof L., van den Burg S. W. K., Banach J. L., Röckmann C., Goossen M. (2020). Can multi-use of the sea be safe? A framework for risk assessment of multi-use at sea. Available online at: https://edepot.wur.nl/506748 (Accessed February 15, 2024).
Keywords: multi-use, offshore, cumulative impact assessment, cumulative effect assessment, footprint, human activities, marine spatial planning, environmental benefit
Citation: Tamis JE, Jongbloed RH, Rozemeijer MJC, Grundlehner A, de Vries P, Van Gerven A, Jak RG and Piet GJ (2024) Assessing the potential of multi-use to reduce cumulative impacts in the marine environment. Front. Mar. Sci. 11:1420095. doi: 10.3389/fmars.2024.1420095
Received: 19 April 2024; Accepted: 04 September 2024;
Published: 30 September 2024.
Edited by:
Ould El Moctar, University of Duisburg-Essen, GermanyReviewed by:
Di Jin, Woods Hole Oceanographic Institution, United StatesCopyright © 2024 Tamis, Jongbloed, Rozemeijer, Grundlehner, de Vries, Van Gerven, Jak and Piet. This is an open-access article distributed under the terms of the Creative Commons Attribution License (CC BY). The use, distribution or reproduction in other forums is permitted, provided the original author(s) and the copyright owner(s) are credited and that the original publication in this journal is cited, in accordance with accepted academic practice. No use, distribution or reproduction is permitted which does not comply with these terms.
*Correspondence: Jacqueline E. Tamis, SmFjcXVlbGluZS50YW1pc0B3dXIubmw=
Disclaimer: All claims expressed in this article are solely those of the authors and do not necessarily represent those of their affiliated organizations, or those of the publisher, the editors and the reviewers. Any product that may be evaluated in this article or claim that may be made by its manufacturer is not guaranteed or endorsed by the publisher.
Research integrity at Frontiers
Learn more about the work of our research integrity team to safeguard the quality of each article we publish.