- 1Biological Sciences Department, Clemson University, Clemson, SC, United States
- 2Engineering and Science Education Department, Clemson University, Clemson, SC, United States
- 3Forestry and Environment Conservation Department, Clemson University, Clemson, SC, United States
The Florida Keys reef tract has rapidly shifted from a structurally complex, hard coral-dominated reef to a less rugose, soft coral-dominated reef. This transition has been facilitated by persistent anthropogenic stressors including recreational and commercial fishing and increased anthropogenic marine debris. During the summers of 2020–2022, benthic censuses were conducted to identify substrate and marine debris composition for 30 reefs in the middle Florida Keys. Inshore reefs contained higher rugosity, coral cover, and marine debris abundance primarily comprised of monofilament and rope from fishing traps. Plastic items (e.g., ropes and monofilament) overall had the highest species diversity. Additionally, marine debris appears to promote turf algae growth on inshore reefs. While is it not yet possible to determine if this pattern of high debris nearshore is due to proximity to onshore debris sources, accumulation due to higher rugosity snagging debris, or increased debris removal efforts offshore, these differences in marine debris types and abundances suggest variability in potential impacts of debris on marine biota on inshore versus offshore reefs. Therefore, the differential use of marine debris by associated biota should be considered in marine debris management practices.
1 Introduction
The Florida reef tract is the largest coral reef system in the continental United States (Finkl and Andrews, 2008; Toth et al., 2018). This archipelago extends approximately 350 km from Key Biscayne to the Dry Tortugas and is comprised of numerous shelf and patch reefs (Toth et al., 2018, Toth et al., 2022). These reefs are habitats for a large variety of reef fishes and invertebrates (McCarthy et al., 2020). Unfortunately, the Florida reef track has undergone severe loss of hard corals due to anthropogenic stressors and climate change (Office of National Marine Sanctuaries, 2011). This decline began in the 1970s with a severe outbreak of white band disease followed by physical disturbance from hurricanes and algal blooms exacerbated by increased human impact (Dustan, 1977; Precht et al., 2016). Subsequent waves of coral disease, cold snaps, extreme heat, nutrient eutrophication, and local disturbance from boating and commercial fishing have severely limited hard coral recovery (Aronson and Precht, 2001; Manzello, 2015). In general, the Caribbean alone has experienced a loss of percent hard coral cover from nearly 50% in 1970 to less than 5% today (Gardner et al., 2003; Jackson et al., 2014). This loss of hard corals has resulted in the shifting of reef habitats from structurally complex hard coral thickets to structurally simple soft coral and macroalgal fields (Maliao et al., 2008; Donovan et al., 2020). The complex, three-dimensional reefs represent more than 75% of the volume of shelters available to protect benthic fishes and invertebrates (Takada et al., 2007). Thus, it is now uncertain how this decrease in this complexity will affect both macro- and microfauna that rely on the structural intricacies of the reef.
In addition to the decreases in reef structural complexity, there is also an increasing volume of human-made debris on Florida Keys reefs (Miller et al., 2008). The Florida reef tract has accumulated anthropogenic marine debris (henceforth referred to as marine debris) through severe storms, tourism, and accidental and intentional abandonment indicating that much of the marine debris on Florida Keys reefs are locally sourced (Diersing, 2013). One of the most influential influxes of marine debris into the Florida Keys has resulted from lost and abandoned fishing gear. It is estimated that upwards of 100,000 lobster traps are lost each year Keys-wide (Renchen et al., 2021). In the lower Florida Keys alone, 90% of all debris consists of monofilament, wood from lobster pots, rope from lobster traps, fishing weights, leaders, and hooks (Chiappone et al., 2002; Renchen et al., 2021). This marine debris is known to accumulate along ledges and reefs due to winds, currents, and boating activity (Bauer et al., 2008; Uhrin et al., 2014; Renchen et al., 2021). Such loss of fishing gear causes damage to reef communities from breakage, entanglement, laceration, spread of disease, and restriction of sunlight (Lewis et al., 2009; Lamb et al., 2018).
Marine debris accumulation has been well documented to have detrimental and deleterious effects on marine taxa (Moore, 2008; Rochman et al., 2016); however, few studies indicate alternative uses of marine debris by cryptic reef taxa (Katsanevakis et al., 2007; De Carvalho-Souza et al., 2018; Teng et al., 2023). As of 2020, over 900 marine species were documented to interact with marine debris (Kühn and van Franeker, 2020). Of that, 701 species were documented to have ingested marine debris, and 354 species experienced entanglement (Kühn and van Franeker, 2020). It is estimated that at least 17% of species documented to have interactions with marine debris also appear on the International Union for Conservation of Nature Red List (Gall and Thompson, 2015). However, the full scope to which marine species are interacting with the debris is unclear. Oftentimes, marine debris uses such as refuge, homing dens, and substrate by marine taxa go unreported.
Human-made structures have long been used to promote biodiversity for fishing success and recruitment of spiny lobster Panulirus argus for Caribbean fishermen (Lee et al., 2018; Ross et al., 2022). Casitas, for example, are illegally placed low-lying structures that vary in material composition and structure. Ross et al. (2022) found that casitas, while they may be artificial, increase the biodiversity of fishes and mobile invertebrates and mimic the effects of naturally occurring coral heads. While some uses of marine debris may be seen as having positive influences on marine biota as habitat, currently, there is a lack of “positive” and net neutral interaction data to fully assess long-term effects and management. Furthermore, Katsanevakis et al. (2007) suggest that “positive” interactions with marine debris may be short-lived as artificial structures may promote predation and decreased survival rates. While studies of predation and survival rates of organisms on marine debris are not yet prominent, Komyakova et al. (2021) note that poorly designed artificial reefs could serve as ecological traps and increase mortality rates of inhabiting species as Katsanevakis et al. (2007) suggest. Such conclusions may directly contradict the use of man-made materials as artificial reefs and the non-removal of marine debris overgrown by biota (Donohue et al., 2001; Smith and Edgar, 2014).
Here, we conducted a 3-year survey of benthic marine debris on reefs in the middle Florida Keys. This study provides an analysis of the influence of structural complexity and community composition on marine debris. First, we hypothesize that marine debris abundance is related to reef rugosity and predict that debris concentration would increase with an increase in reef structural complexity (rugosity) and that this difference would be most noticeable between reefs inshore versus those farther from shore. Second, we hypothesize that most marine debris is from recreational and commercial fishing sources and predict that derelict fishing gear comprised of lobster traps and trap debris would be uniformly distributed regardless of distance from shore. Third, given that most marine debris come from similar sources, we expect the distribution of marine debris material types to be similar inshore to offshore. Fourth, we hypothesize that the taxa community associated with marine debris differs by material type independent of distance from shore. By understanding the patterns of marine debris distribution, material types, and associations with marine fauna, we hope to expand our understanding of the costs and benefits of marine debris removal in the middle Florida Keys.
2 Methods
2.1 Study sites
Permitted research was conducted on 15 paired reef sites in the Florida Keys National Marine Sanctuary (FKNMS; 24.7°N, 80.8°W) from Islamorada to Marathon (Figure 1). Each of the paired reef sites is approximately 500 m from each other and over 1,000 m from the nearest adjacent site of paired reefs. Previous research was conducted on these sites examining reef substrate, rugosity, and reef fish behavior over the last 12 years (Smith et al., 2018, Smith et al., 2019; Noonan and Childress, 2020). None of the 15 locations occurred in Special Preservation Areas. Seven of the paired reefs were considered inshore (3–8 m depth, within 4 km from shore) and eight of the paired reefs were considered offshore (5–10 m depth, >8 km from shore). All reefs were monitored once each year in the summer (May–July) of 2020, 2021, and 2022.
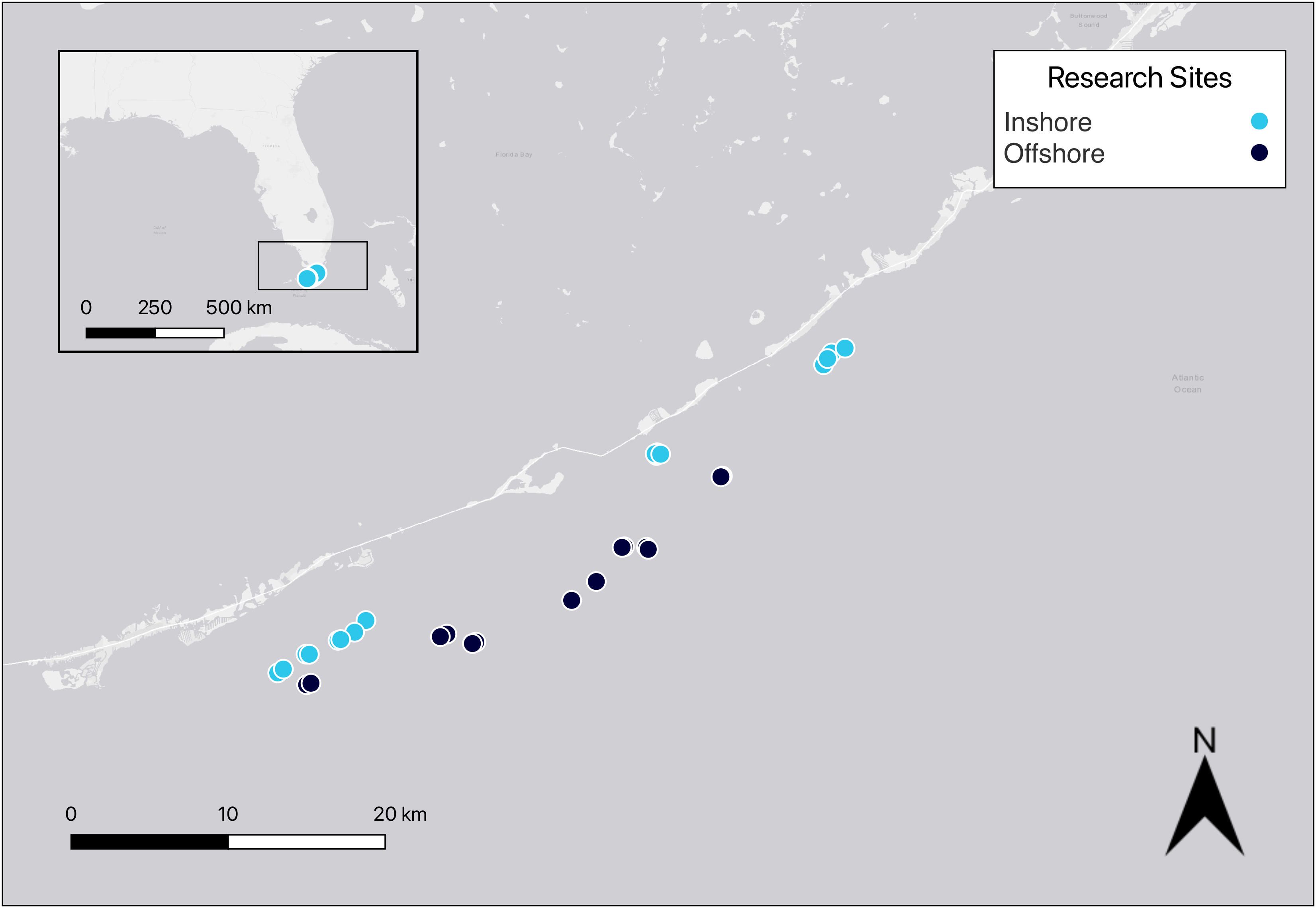
Figure 1 Map of 30 reef locations in the middle Florida Keys. Marine debris, substrate cover, and rugosity were measured at each of the inshore and offshore locations. Map generated using QGIS version 3.32.3-Lima (QGIS.org, 2023).
2.2 Reef benthic community and rugosity census
Reefs were censused on SCUBA using a 50 m transect line that ran parallel to the primary axis of the reef and four 30 m transects that laid perpendicular crossing at distances of 10, 20, 30, and 40 m, creating a 50 m × 30 m grid (Noonan and Childress, 2020; Noonan et al., 2021). Rugosity was measured using a chain and tape method (Risk, 1972; Luckhurst and Luckhurst, 1978; Noonan et al., 2021) taking three measurements on each of the four perpendicular transects. Rugosity measurements (n = 12) were averaged for the overall rugosity of each reef. To estimate the benthic community composition, divers took digital photographs of 50 cm × 50 cm areas of substrate on each side of the 50 m transect for the 0, 10, 20, 30, 40, and 50 m (n = 12 per reef). Photographs were analyzed for percent cover using 25 randomly placed points per picture using Coral Point Count with Excel extension V4.1 (Kohler and Gill, 2006).
2.3 Reef debris census
Using the 50 m × 30 m transect grid, a U-shaped diver search pattern began starting at the zero end of the first 30 m creating a 30 m × 30 m census area. All anthropogenic marine debris found within the 900 m2 area was recorded and mapped using XY-coordinate notation. Marine debris observed were grouped into nine material types including plastic, mixed, wood, metal, concrete, glass, cloth, paper, and other. Materials grouped into “other” included items that were hard to identify (i.e., due to biofouling). Mapping included identifying the material of debris and taxa–debris interactions using predetermined codes adapted from De Carvalho-Souza et al. (2018) (Figure 2). Specifically, interactions were categorized as catches in derelict fishing gear (CDFG), use of debris as a mobile home (MH), debris covering or smothering an organism (COV/SMO), entrapment or entanglement within debris (ENT), use of debris as refuge (RF), and use of debris as substrate (S). Catches in fishing gear and use of debris as a mobile home were not observed and therefore were not included in the current study. In the current study, entanglement (ENT) also included instances of rubbing and abrasion. Spatially calibrated photos of all debris were used to further identify organisms associated with debris. Mapping included little to no disturbance of debris; therefore, only marine taxa interacting with exposed parts of debris were identified. Taxa associations were counted based on the presence/absence of each marine debris item found.
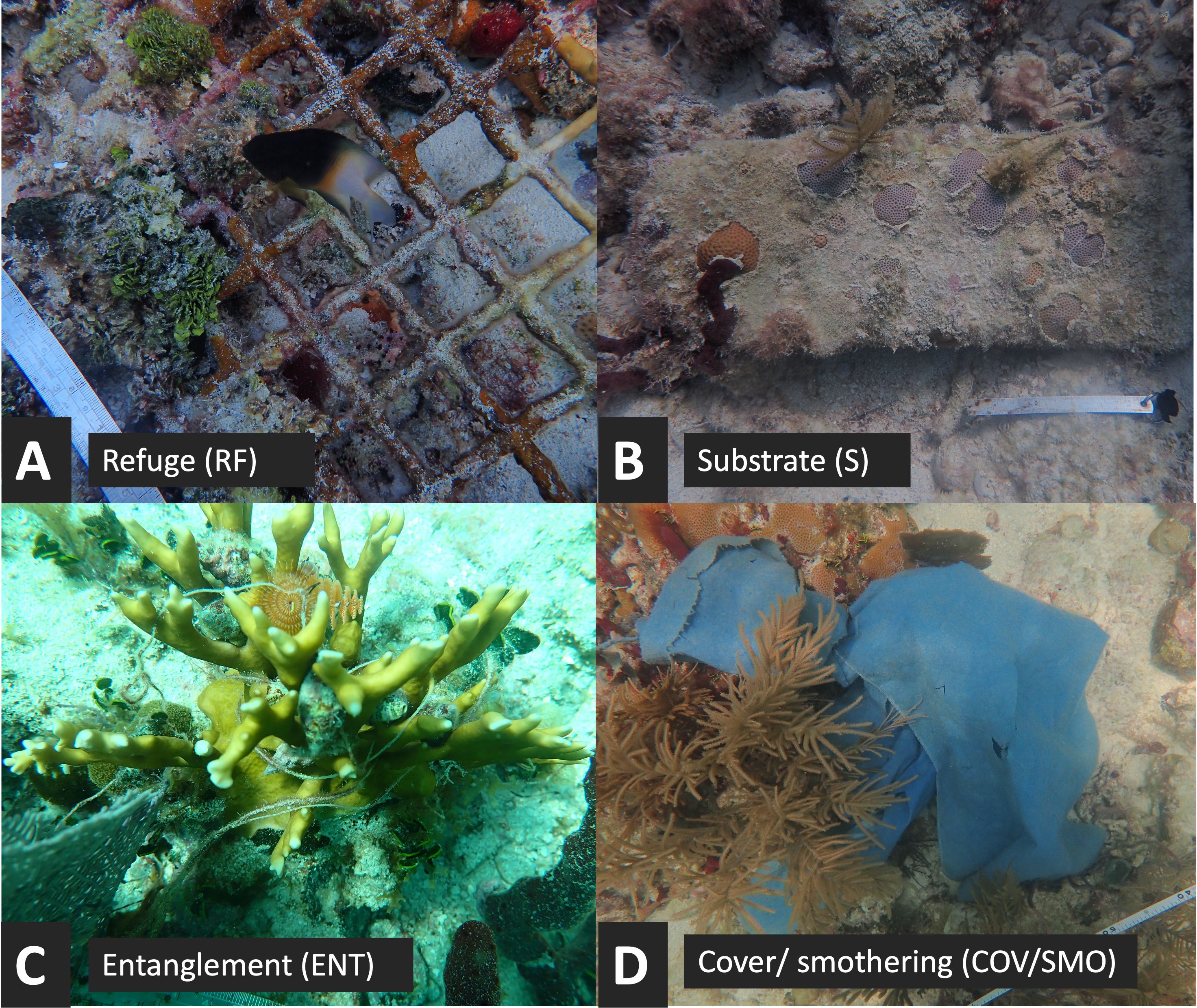
Figure 2 Examples of marine taxa interactions with anthropogenic marine debris modified from De Carvalho-Souza et al. (2018). Image (A) (top left) indicates the use of marine debris as a refuge. In this case, the bicolor damselfish is using lobster trap debris as a home. Image (B) (top right) indicates the use of marine debris as substrate such as the use of concrete slabs for coral and sponge growth. Image (C) (bottom left) indicates marine debris (fishing line) being entangled in fire coral. Image (D) (bottom right) shows marine debris (cloth) covering marine organisms such as soft coral.
2.4 Data analysis
Principal component analyses (PCAs) were used to characterize the differences between inshore and offshore reef substrate composition and concentration of marine debris by material type. Welch two-sample t-tests were used to determine differences in the prevalence of marine debris material type by inshore and offshore reefs. Reef substrate composition, depth, distance, and total marine debris abundance were characterized using a Spearman rank correlation analysis. The Spearman rank correlation analysis and principal coordinate analysis (PCoA) were constructed using Bray–Curtis dissimilarity indices to assess relationships between percent substrate cover, distance from shore, reef depth, rugosity, and total marine debris abundance. Richness, Pielou’s J′ evenness, and Shannon and inverse Simpson’s alpha diversity indices for debris-associated taxa were compared by region using Kruskal–Wallis and post-hoc Dunn’s multiple comparison test with a Holm–Bonferroni multiple comparisons correction to identify areas of significance. Debris-related taxa were identified and assessed based on overall influence (positive, negative, neutral) on the reef community inshore and offshore. Influences were determined based on systematic literature searches for each taxonomic group. Positive associations were defined by having an overall net-positive influence on reef health such as reef builders or herbivores to assist in mitigating macroalgal overgrowth. Net-negative influences included taxa groups that are currently recognized as decreasing the overall health of positive organisms, such as parasites, macroalgae, corallivores, and invasives, whereas neutral influences were determined either by a group having both positive and negative influences that canceled out or having a lack of evidence for either positive or negative influences. Percent occurrence rates were estimated using abundances from the presence/absence of taxa found in marine debris in the current study. A 10% occurrence cutoff was used to assess which organisms may have the biggest impact on the surrounding reef community. All analyses and figures were performed using RStudio© 2022.07.1 Build 554 (Oksanen et al., 2022; R Core Team, 2023).
3 Results
3.1 Increased rugosity is associated with higher rates of marine debris
Inshore and offshore reefs in the middle Florida Keys differ in substrate composition as evidenced by distinct clustering using PCA including distance from shore, depth, rugosity, and benthic substrate cover (Figure 3). Inshore reefs are shallow with high Scleractinia (hard coral) cover and low Alcyonacea (soft coral) cover leading to higher rugosity. Offshore reefs are deeper with low hard coral cover and high soft coral cover leading to lower rugosity.
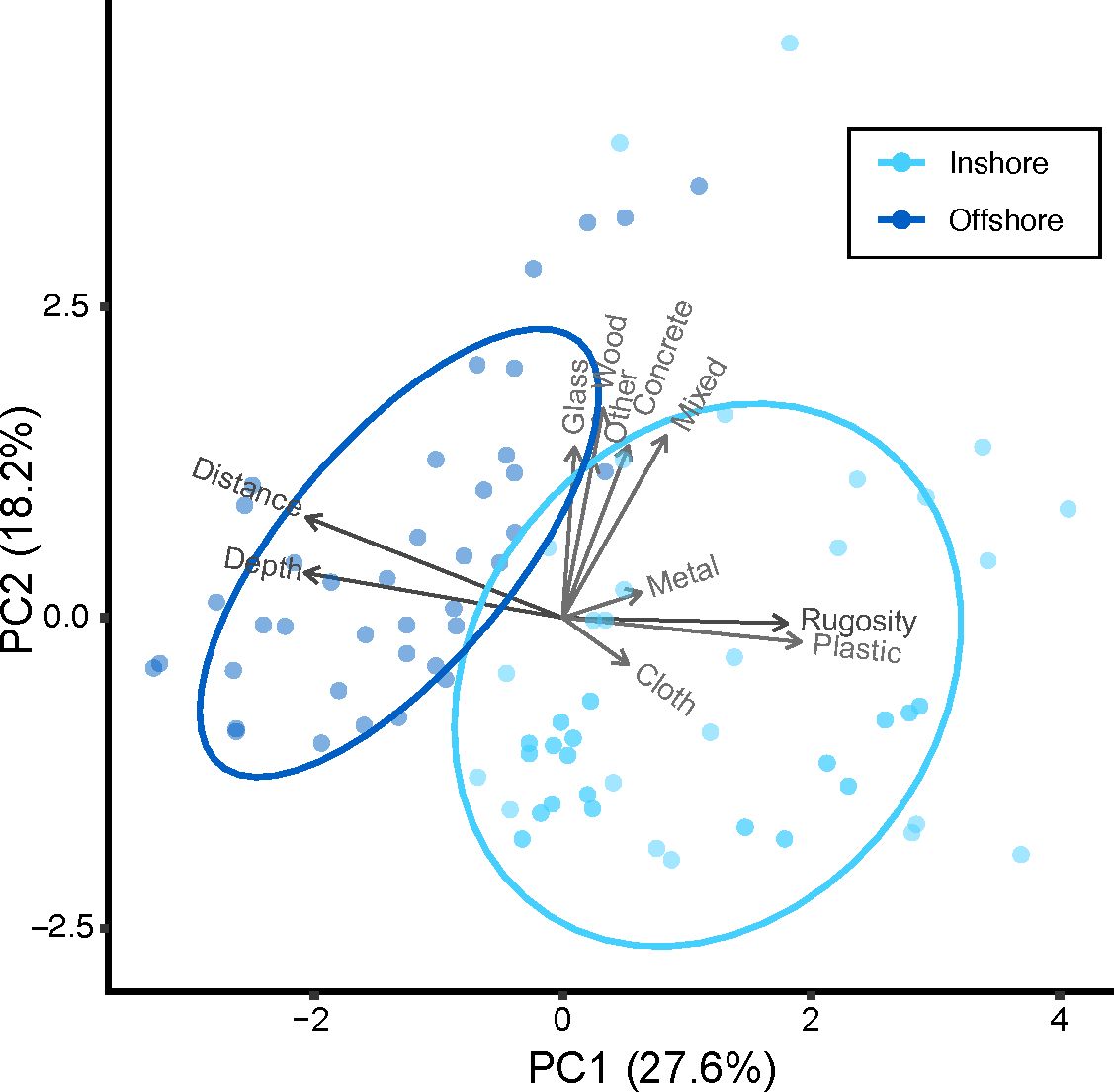
Figure 3 PCA plot loaded with percent substrate cover, rugosity, depth, and distance from shore demonstrates differences in offshore versus inshore reefs. PC1 is driven by relationships in offshore, deeper reefs comprised of turf and inshore, and shallower reefs with high rugosity and higher coral cover. PC2 is driven by differences in soft coral, sponge, macroalgae, and sand cover.
Spearman rank correlation strongly indicated that reef rugosity is positively influenced by percent Scleractinia (hard coral) and percent Corallinales (calcareous algae) (Spearman’s r = 0.8, p-value < 0.001, Figure 4). Spearman rank correlations greater than 0.5 or less than −0.5 indicated significant positive or negative correlations, respectively. Percent Alcyonacea and turf alga also exhibited an inverse relationship with rugosity (Spearman’s r = −0.6, p-value = 0.044 and Spearman’s r = −0.8, p-value < 0.001, respectively). Rugosity also shows a strong correlation with marine debris abundance (Spearman’s r = 0.8, p-value < 0.001). No relationship was observed with macroalgal cover and marine debris abundance (Spearman’s r = 0, p-value = 0.476). The Spearman rank correlation also indicated a highly inverse relationship with rugosity and depth and distance (Spearman’s r = −0.8, p-value < 0.001). Therefore, both depth and distance are highly negatively correlated with marine debris abundance (Spearman’s r = −0.8, p-value < 0.001). PCoA indicated distinct clustering of inshore and offshore reefs, and additional clustering occurred within inshore reef sites of adjacent sites (see Supplementary Figure S1).
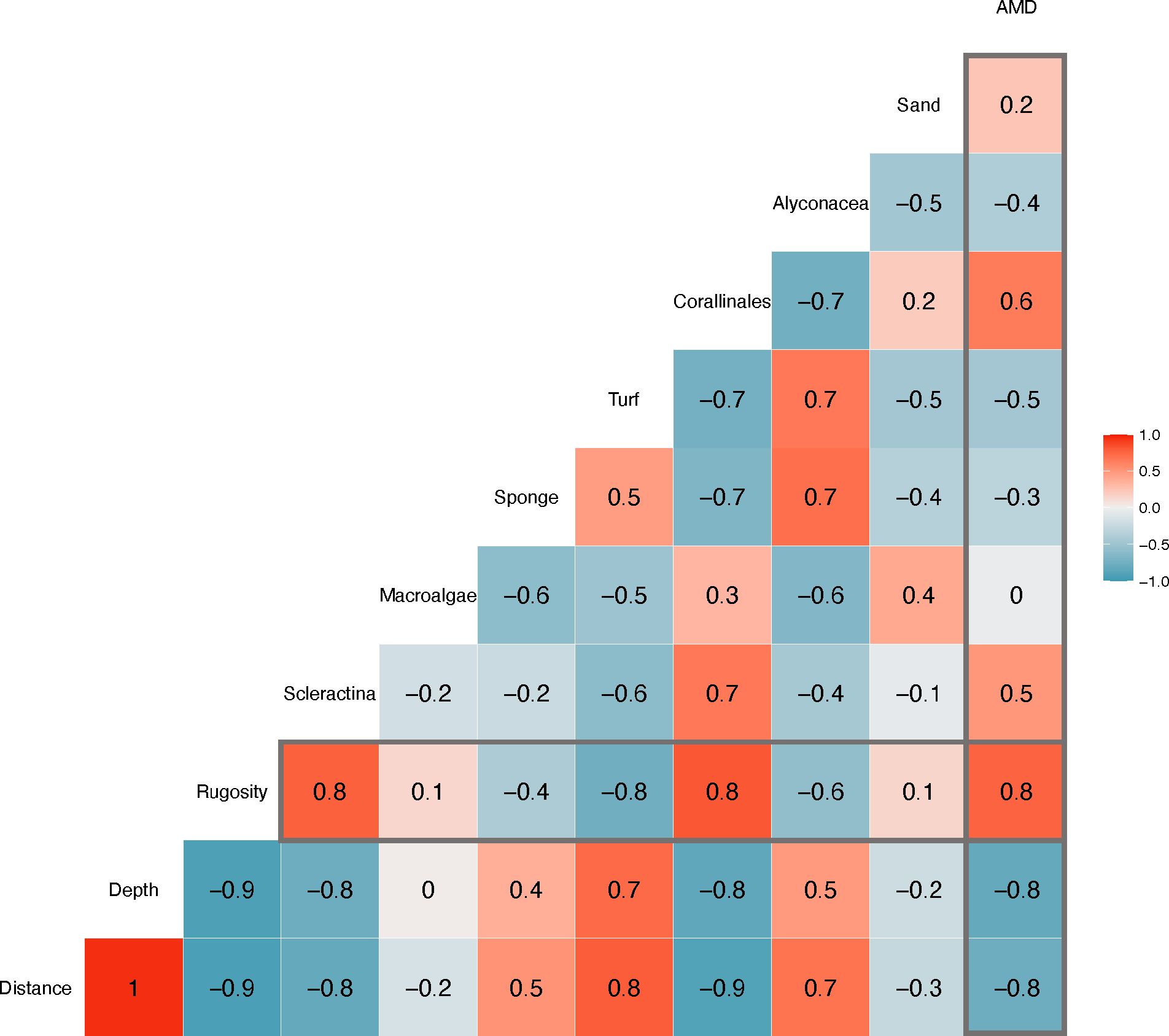
Figure 4 Spearman rank correlation. Spearman rank correlations >0.5 or <−0.5 indicated significant p-values. Associations with anthropogenic marine debris (AMD) with all measured community components as well as associations with rugosity are outlined in gray.
3.2 Plastic and metal concentrate on rugose inshore reefs
Total marine debris densities for inshore reefs were greater than for offshore sites (t = 2.95, p-value = 0.004). Average marine debris densities were approximately 14.5 (SE ± 1.2) pieces per 900 m2 area for inshore reefs and 10.4 (SE ± 1.0) pieces for offshore reefs per 900 m2 area. Marine debris differed by material type by region for plastic (t = 6.07, p-value < 0.0001) with approximately 8.1 (SE ± 0.7) pieces of plastic for inshore reefs and 3.3 (SE ± 0.3) pieces for offshore reefs per 900 m2 area. Plastic materials primarily represented rope from lobster and stone crab traps and monofilament. Metal materials consisting primarily of leader lines and trolling wire were significantly higher on inshore reefs than offshore sites (averages = 2 and 1, respectively; p-value = 0.01; Supplementary Figure S2). Such plastic and metal materials were grouped as fishing-related debris averaging 6.7 (SE ± 0.7) pieces inshore and 5.7 (SE ± 0.9) pieces offshore per 900 m2. All other material types did not significantly differ by region indicating a potentially even distribution of concrete, glass, mixed material, wood, and other across both inshore and offshore reefs (Supplementary Figure S3). Non-fishing-related debris density averaged 2.1 (SE ± 0.4) per site inshore and 1 (SE ± 0.4) piece per site offshore. Non-fishing-related debris made up approximately 13.8% of all debris inshore and 8.0% of all debris found on offshore sites. All ranges for debris items by region are listed in Supplementary Table S1.
3.3 Taxa interactions with marine debris differ based on region and debris type
Debris-associated taxa were comprised primarily of what was considered positive non-excavating sponges and hard corals, negative turf algae, and green and brown macroalgae (Supplementary Table S2). Regardless of marine debris, material type-associated taxa differed in J′ evenness by region, inshore and offshore (χ2 = 4.149, p-value < 0.05; Figure 5). However, species richness and alpha diversity indices did not differ significantly (richness: χ2 = 0.130, p-value = 0.717; Shannon: χ2 = 0.027, p-value = 0.867; inverse Simpson: χ2 = 0.292, p-value = 0.588; Figure 5). Taxa richness and alpha diversity indices significantly differed based on marine debris material type (richness: χ2 = 69.497, p-value < 0.001; Shannon: χ2 = 62.708, p-value <0.001; inverse Simpson: χ2 = 56.716, p-value < 0.001; J′: χ2 = 28.156, p-value <0.001; Supplementary Table S3). Furthermore, richness and alpha diversity also differed inshore compared to those of offshore. All diversity indices were significant for inshore taxa driven by taxa associated with plastics (Supplementary Table S4). Plastics overall contained the highest species richness above all other material types (Supplementary Figure S4). For offshore, the evenness of taxa found on wood was significantly different compared to the taxa found on glass (p-value < 0.01). No other offshore diversity association was significant. While the percent cover of turf algae occurring on the inshore reef community was not significant, significantly more turf algae were associated with debris inshore compared to offshore (χ2 = 72.627, p-value < 0.001; Figure 6A). Scleractina hard corals and calcareous Corallinales taxa occurring on debris were also significantly abundant on inshore reefs (χ2 = 6.130, p-value < 0.05; χ2 = 4.445, p-value < 0.05; Figure 6A).
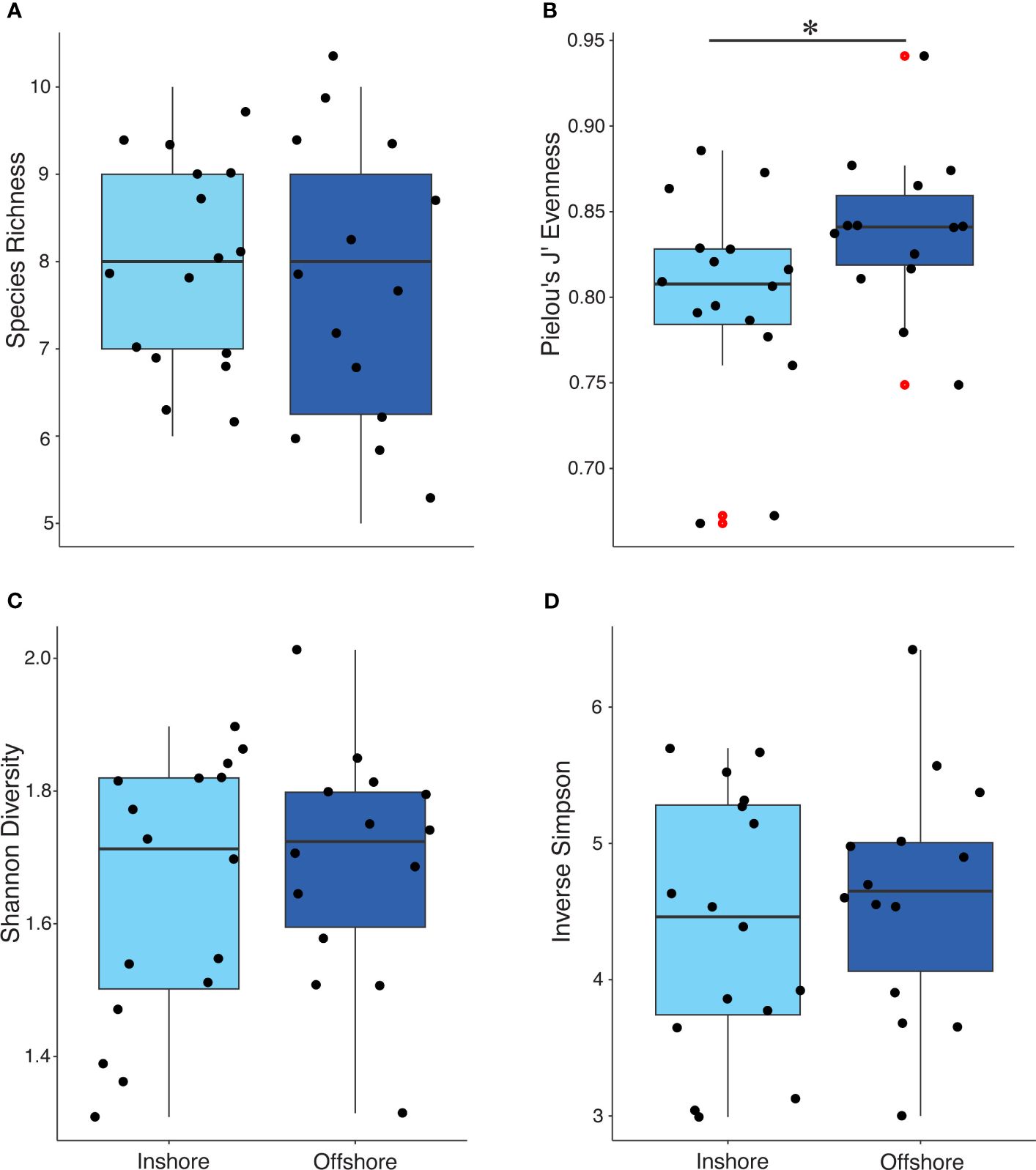
Figure 5 Jittered box plots of taxa richness (A), Pielou’s J′ evenness (B), and alpha diversities; Shannon (C) and inverse Simpson (D) for inshore versus offshore reef locations. Red dots indicate outliers in the data. Only evenness was significant (p < 0.05) indicated by the bar and asterisk (B).
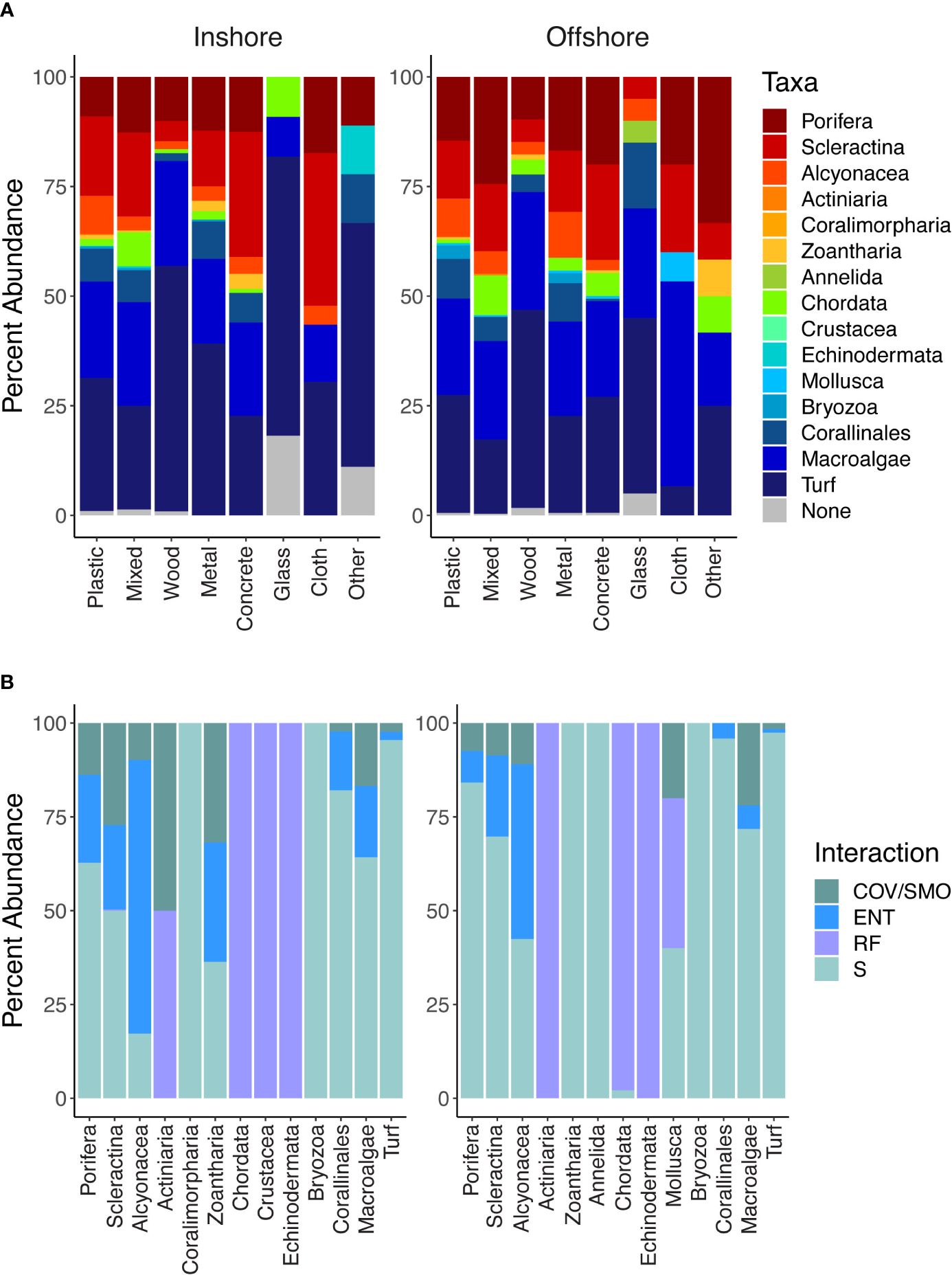
Figure 6 (A) Stacked bar graph demonstrating the percent abundance of taxa associated with anthropogenic marine debris by material type for inshore and offshore reefs. Taxa were identified based on the presence/absence for each piece of marine debris observed in situ. (B) Stacked bar graph demonstrating the percent interaction type for each identified taxon for inshore and offshore locations. Interactions included cover and smothering (COV/SMO), entanglement and abrasion (ENT), refuge (RF), and substrate (S).
Taxa interactions with marine debris were categorized as covering and/or smothering (COV/SMO), refuge (RF), substrate (S), and entanglements or entrapments (ENT). Mobile organisms such as chordates used all materials as a form of shelter or refuge except for cloth and paper (Figure 6B). However, sessile zoanthids preferred concrete and our “other” material category. Taxa also significantly differed by their interaction with debris (χ2 =3054.7, p-value < 0.001). Alcyonacea (soft corals) while noted to interact with most all marine debris material types observed, most of the interactions involved entanglements.
4 Discussion
Anthropogenic marine debris has been documented worldwide, and the Florida Keys National Marine Sanctuary is no exception (Raimondo et al., 2024). This anthropogenic disturbance is in juxtaposition with changes in climate and ecosystem shifts (Ford et al., 2022; Thirukanthan et al., 2023). Over the last decades, we have seen a melting of reef structures from diverse structural complexities to flatter less diverse reefs (Hughes, 1994; Donovan et al., 2020). This altered coral reef ecosystem, in conjunction with increased marine debris, introduces shifts from natural to artificial structures. In addition, the use of coral reefs by humans for nutrition, medicines, safe shores, and recreation and changes in climate add a significant influx of debris (Ford et al., 2022; Thirukanthan et al., 2023). For example, recreational and commercial fishing is responsible for creating the highest types of debris on the adjacent reef environment in the Florida Keys (Uhrin et al., 2014; Renchen et al., 2021). What is not known in full is the distribution and use of marine debris by marine organisms. Our study has identified patterns of marine debris distribution regarding the naturally occurring benthic community on 30 reef locations in the middle Florida Keys. The highest abundance of debris occurred on shallow, inshore reefs associated with higher rugosity, coral cover, and calcareous algae (Corallinales). These inshore reefs contain massive Siderastrea siderea and Orbicella spp. boulder corals that provide habitat for recreational game fish and become a sink for recreational fishing and trap debris that is moved by wind and current (Lewis et al., 2009).
We observed that the primary marine debris material types found on inshore reefs were plastics and metals from fishing gear suggesting that these are locally derived and may indicate a difference in recreational use of inshore reefs versus offshore reefs. This observation warrants further investigation as metal was represented by leader line and wire and much of the plastic materials consisted of monofilament fishing line and nylon and polypropylene rope. Increased monofilament and leaders could suggest that inshore reefs experience higher recreational hook and line fishing pressure or that the increased reef complexity results in more entangled or caught lines than the less rugose offshore reefs. The same could be said for rope from fishing traps as cut lines may find their way entangled in a more complex structure. An alternative explanation for this increased abundance of marine debris inshore could be related to marine debris removal activities by local dive shops. Based on interviews with commercial dive shops in the Florida Keys, many focus on offshore reefs due to clearer visibility and the belief that more debris is found deeper and along the continuous reef track (not published). This does not appear to be the case for our middle Keys reefs for which very few marine debris removal activities, either inshore or offshore, have been conducted based on the PADI Aware Foundation Dive Against Debris database (PADI AWARE Foundation, 2022).
Total marine debris abundance was positively correlated with calcareous red algae which appeared on all debris types and were known to facilitate hard coral settlement (Tebben et al., 2015; Gómez-Lemos et al., 2018; Deinhart et al., 2022). We note that structures such as plastic and concrete allow for the facilitation of hard coral recruitment; however, the duration of sustained growth is currently unknown and warrants further investigation. No correlation of total debris with naturally occurring fleshy macroalgae cover was noted, and thus, this study indicates that while increases in macroalgae have coincided with the shifting of reefs to less structurally complex communities (Maliao et al., 2008; Donovan et al., 2020), marine debris does not seem to be facilitating fleshy macroalgal growth.
The significant presence of calcareous algae, hard corals, and turf on marine debris inshore heavily influenced the significantly lower evenness of taxa inshore versus offshore. However, the evenness of taxa on offshore debris was higher with only notable differences in communities on wood and glass, with glass containing the least difference in taxa. Due to higher turf cover on offshore reefs compared to inshore reefs, we would expect that marine debris offshore would have more turf present. However, we found that marine debris inshore contained 122% more turf than debris offshore. Turf competes with hard corals for space and accumulates sediments further preventing coral growth (Tebbet and Bellwood, 2019; Brown et al., 2020). Turf algae, if introduced by marine debris at higher than naturally occurring levels, could have cascading effects on coral reef habitats. Such interactions have occurred in the Yellow Sea with the carnivorous anemone Metridium senile fimbriatum (Teng et al., 2023).
With decreases in three-dimensional reef structure, studies are showing that various marine organisms are finding habitat among anthropogenic marine debris (De Carvalho-Souza et al., 2018). While deleterious effects are heavily documented (Moore, 2008; Rochman et al., 2016), this study also notes additional taxa–debris interactions as a future opportunity to better understand how marine debris is affecting coral reef communities. For instance, here, we showed that species composition can differ significantly based on the material of the marine debris. This information may inform debris removal efforts for retrieval efficiency and reef health by focusing on materials causing the most risk. We can assume that interactions such as covering/smothering (COV/SMO) and entanglement (ENT) are detrimental to marine life while refuge (RF) and substrate (S) may be beneficial depending on what is utilizing the space. However, the extent to which these interactions are positive, negative, or net-neutral is still unknown, and we encourage future marine debris research to incorporate these elements moving forward. It is estimated that two-thirds of coral reefs are made of hidden nooks and crevices creating homes for many cryptic benthic fishes and invertebrates (Takada et al., 2007; Glynn and Enochs, 2011). Thus, the question remains as to how marine debris is being used by these cryptic species in situ and in the long term. While only visually exposed organisms were accounted for in our study, we acknowledge that more cryptic or hidden biota could be utilizing and/or affected by debris that cannot be identified until the debris is disturbed or removed. Therefore, our proportions of organisms covered (COV/SMO) or marine debris used as refuge (RF) may be underestimates and will be further investigated. For instance, the oviposition of fishes on marine debris was not noted in this study but has been raised as an area needing further research by Shruti et al. (2023). Additionally, we note that non-indigenous species such as sun corals, Tubastrea spp., which have been previously documented on marine debris in the Florida Keys (Parsons et al., 2023) were not identified in this study and require further investigation to determine the cost and benefits of debris materials as substrate or refuge.
There is strong evidence that high natural structure from massive reef-building corals contributes to reef biodiversity (Alvarez-Filip et al., 2011; Graham and Nash, 2013). Therefore, the concern is that the loss of reef-building corals will lead to a flattening of the reef and a corresponding loss of biodiversity (Alvarez-Filip et al., 2009; van Woesik et al., 2022). This may also elevate the importance of structure from marine debris which may serve as unintentional artificial reefs attracting the settlement of reef species (Spanier and Almog-Shtayer, 1992; Kiessling et al., 2015). This raises an important question when considering the net benefits of the removal of debris heavily covered in sessile species with a shrinking natural area for settlement. This study serves as a framework to continue the study of marine debris on Florida Keys reefs. Future studies will work to better understand debris patterns on Florida reefs and the potential costs and benefits of debris on cryptic marine biota. Such research will aid in natural resource management and assist in safe marine debris removal for cleaner, healthier reefs.
Data availability statement
The raw data supporting the conclusions of this article will be made available by the authors, without undue reservation.
Author contributions
TP: Conceptualization, Data curation, Formal analysis, Funding acquisition, Investigation, Methodology, Project administration, Resources, Software, Supervision, Validation, Visualization, Writing – original draft, Writing – review & editing. RS: Data curation, Funding acquisition, Writing – review & editing. MC: Data curation, Funding acquisition, Resources, Supervision, Writing – review & editing.
Funding
The author(s) declare financial support was received for the research, authorship and/or publication of this article. Research funding was provided by Clemson University, Clemson University Graduate Student Government, Clemson University Department of Biological Sciences Graduate Student Association, and Clemson University Creative Inquiry Initiative. Authorship and Publication costs were supported by South Carolina Space Grant and South Carolina Sea Grant Kathryn Sullivan Earth and Marine Science Fellowship Department of Commerce/ NOAA Federal Award No. NA22OAR4170114 (CFDA #11.417).
Acknowledgments
The authors would like to thank Dr. Kylie Smith, Kara Titus, Dr. Disha Bhattacharjee, Clara Flores, and the Clemson Conservation of Marine Resources and Marine Ecology Research Creative Inquiry Research teams. Specifically, we would like to thank Leann Jackson, Maddison Parker, Alexis Smith, Ean Tucker, and Amanda Turner. All fieldwork for this research was conducted under permit no. FKNMS-2020–187.
Conflict of interest
The authors declare that the research was conducted in the absence of any commercial or financial relationships that could be construed as a potential conflict of interest.
Publisher’s note
All claims expressed in this article are solely those of the authors and do not necessarily represent those of their affiliated organizations, or those of the publisher, the editors and the reviewers. Any product that may be evaluated in this article, or claim that may be made by its manufacturer, is not guaranteed or endorsed by the publisher.
Supplementary material
The Supplementary Material for this article can be found online at: https://www.frontiersin.org/articles/10.3389/fmars.2024.1412858/full#supplementary-material
References
Alvarez-Filip L., Dulvy N. K., Gill J. A., Côté I. M., Watkinson A. R. (2009). Flattening of Caribbean coral reefs: region-wide declines in architectural complexity. Proc. R. Soc. B: Biol. Sci. 276, 3019–3025. doi: 10.1098/rspb.2009.0339
Alvarez-Filip L., Gill J. A., Dulvy N. K. (2011). Complex reef architecture supports more small-bodied fishes and longer food chains on Caribbean reefs. Ecosphere 2, art118. doi: 10.1890/ES11-00185.1
Aronson R. B., Precht W. F. (2001). White-band disease and the changing face of Caribbean coral reefs. Hydrobiologia 460, 25–38. doi: 10.1023/A:1013103928980
Bauer L. J., Kendall M. S., Jeffrey C. F. G. (2008). ). Incidence of marine debris and its relationships with benthic features in Gray’s Reef National Marine Sanctuary, Southeast USA. Mar. pollut. Bull. 56, 402–413. doi: 10.1016/j.marpolbul.2007.11.001
Brown K. T., Bender-Champ D., Hoegh-Guldberg O., Dove S. (2020). Seasonal shiftsin the competitive ability of macroalgae influencethe outcomes of coral–algal competition. R. Soc.Open Sci. 7, 201797. doi: 10.1098/rsos.201797
Chiappone M., White A., Swanson D. W., Miller S. L. (2002). Occurrence and biological impacts of fishing gear and other marine debris in the Florida Keys. Mar. pollut. Bulletin. 44, 597–604. doi: 10.1016/S0025-326X(01)00290-9
De Carvalho-Souza G. F., Llope M., Tinôco M. S., Medeiros D. V., Maia-Nogueira R., Sampaio C. L. S. (2018). Marine litter disrupts ecological processes in reef systems. Mar. pollut. Bull. 133, 464–471. doi: 10.1016/j.marpolbul.2018.05.049
Deinhart M., Mills M., Schils T. (2022). Community assessment of crustose calcifying red algae as coral recruitment substrates. PLoS One 17, e0271438. doi: 10.1371/journal.pone.0271438
Diersing N. (2013). Marine debris in reef habitats (Florida Keys National Marine Sanctuary). Available at: https://nmsfloridakeys.blob.core.windows.net/floridakeys-prod/media/archive/scisummaries/marinedebris2013.pdf.
Donohue M. J., Boland R. C., Sramek C. M., Antonelis G. A. (2001). Derelict fishing gear in the northwestern hawaiian islands: diving surveys and debris removal in 1999 confirm threat to coral reef ecosystems. Mar. Pollut. Bull. 42 (12), 1301–1312. doi: 10.1016/S0025-326X(01)00139-4
Donovan C., Towle E. K., Blondeau J., Eakin M., Edwards K., Edwards P., et al. (2020). Coral reef conditions: a status report for US coral reefs. NOAA Coral Reef Conservation Program and University of Maryland Center for Environmental Science. Available at: https://repository.library.noaa.gov/view/noaa/27295.
Dustan P. (1977). Vitality of reef coral populations off Key Largo, Florida: Recruitment and mortality. Geo 2, 51–58. doi: 10.1007/BF02430665
Finkl C. W., Andrews J. L. (2008). Shelf geomorphology along the southeast Florida Atlantic continental platform: Barrier coral reefs, nearshore bedrock, and morphosedimentary features. J. Coast. Res. 24, 823–849. doi: 10.2112/08A-0001.1
Ford H. V., Jones N. H., Davies A. J., Godley B. J., Jambeck J. R., Napper I. ,. N., et al. (2022). The fundamental links between climate change and marine plastic pollution. Sci. Total Environ. 806 (1), 150392. doi: 10.1016/j.scitotenv.2021.150392
Gall S. C., Thompson R. C. (2015). The impact of debris on marine life. Mar. pollut. Bulletin. 92, 170–179. doi: 10.1016/j.marpolbul.2014.12.041
Gardner T. A., Cote I. M., Gill J. A., Grant A., Watkinson A. R. (2003). Long-term region-wide declines in Caribbean corals. Science 301, 958–960. doi: 10.1126/science.1086050
Glynn P. W., Enochs I. C. (2011). “Invertebrates and their roles in coral reef ecosystems,” in Coral Reefs: An Ecosystem in Transition (Springer, Netherlands), 273–325. doi: 10.1007/978–94-007–0114-4_18
Gómez-Lemos L. A., Doropoulos C., Bayraktarov E., Diaz-Pulido G. (2018). Coralline algal metabolites induce settlement and mediate the inductive effect of epiphytic microbes on coral larvae. Sci. Rep. 8, 17557. doi: 10.1038/s41598–018-35206–9
Graham N. A. J., Nash K. L. (2013). The importance of structural complexity in coral reef ecosystems. Coral Reefs 32, 315–326. doi: 10.1007/s00338-012-0984-y
Hughes T. P. (1994). Catastrophes, phase shifts, and large-scale degradation of a Caribbean coral reef. Science. 265), 1578–1551. doi: 10.1126/science.265.5178.1547
Jackson J., Donovan M., Cramer K., Lam. V. (2014). “Status and trends of caribbean coral reefs: 1970 2012,” (Gland, Switzerland: Global Coral Reef Monitoring Network, IUCN). Available at: https://www.iucn.org/sites/default/files/import/downloads/caribbean_coral_reefs:_status_report_1970_2012.pdf.
Kühn S., van Franeker J. A. (2020). Quantitative overview of marine debris ingested by marine megafauna. Mar. pollut. Bull. 151, 110858. doi: 10.1016/j.marpolbul.2019.110858
Katsanevakis S., Verriopoulos G., Nicolaidou A., Thessalou-Legaki M. (2007). Effect of marine litter on the benthic megafauna of coastal soft bottoms: A manipulative field experiment. Mar. pollut. Bull. 54, 771–778. doi: 10.1016/j.marpolbul.2006.12.016
Kiessling T., Gutow L., Thiel M. (2015). “Marine litter as habitat and dispersal vector,” in Marine Anthropogenic Litter (AG Switzerland: Springer International Publishing), 141–181. doi: 10.1007/978–3-319–16510-3_6
Kohler K. E., Gill S. M. (2006). Coral Point Count with Excel extensions (CPCe): A Visual Basic program for the determination of coral and substrate coverage using random point count methodology. Comput. Geosciences 32, 1259–1269. doi: 10.1016/j.cageo.2005.11.009
Komyakova V., Chamberlain D., Swearer S. E. (2021). A multi-species assessment of artificial reefs as ecological traps. Ecol. Eng. 171, 106394. doi: 10.1016/j.ecoleng.2021.106394
Lamb J. B., Willis B. L., Fiorenza E. A., Couch C. S., Howard R., Rader D. N., et al. (2018). Plastic waste associated with disease on coral reefs. Science 359, 460–462. doi: 10.1126/science.aar3320
Lee M. O., Otake S., Kim J. K. (2018). Transition of artificial reefs (Ars) research and its prospects. Ocean Coast. Management. 154, 55–65. doi: 10.1016/j.ocecoaman.2018.01.010
Lewis C. F., Slade S. L., Maxwell K. E., Matthews T. R. (2009). Lobster trap impact on coral reefs: Effects of wind-driven trap movement. New Z. J. Mar. Freshw. Res. 43, 271–282. doi: 10.1080/00288330909510000
Luckhurst B. E., Luckhurst K. (1978). Analysis of the influence of substrate variables on coral reef fish communities. Mar. Biol. 49, 317–323. doi: 10.1007/BF00455026
Maliao R. J., Turingan R. G., Lin J. (2008). Phase-shifts in coral reef communities in the Florida Keys National Marine Sanctuary (FKNMS), USA. Mar. Biol. 154, 841–853. doi: 10.1007/s00227-008-0977-0
Manzello D. P. (2015). Rapid recent warming of coral reefs in the Florida keys. Sci. Rep. 5, 16762. doi: 10.1038/srep16762
McCarthy D. A., Lindeman K. C., Snyder D. B., Holloway-Adkins K. G. (2020). Ecology of nearshore hardbottom reefs along the East Florida Coast. Islands Sand: Ecol. Manage. Nearshore Hardbottom Reefs East Florida, 299–356. doi: 10.1007/978-3-030-40357-7
Miller S. L., Chiappone M., Rutten L. M. (2008). Large-scale assessment of marine debris and benthic coral reef organisms in the florida keys national marine sanctuary (Key Largo, FL: Quick look report and data summary. Center for Marine Science, University of North Carolina-Wilmington), 271.
Moore C. J. (2008). Synthetic polymers in the marine environment: A rapidly increasing, long-term threat. Environ. Res. 108, 131–139. doi: 10.1016/j.envres.2008.07.025
Noonan K. R., Childress M. J. (2020). Association of butterflyfishes and stony coral tissue loss disease in the Florida Keys. Coral Reefs 39, 1581–1590. doi: 10.1007/s00338-020-01986-8
Noonan K., Fair T., Matthee K., Sox K., Smith K., Childress M. (2021). Reef fish associations with natural and artificial structures in the Florida keys. Oceans 2, 634–647. doi: 10.3390/oceans2030036
Office of National Marine Sanctuaries. (2011). Florida keys national marine sanctuary condition report 2011 (Silver Spring, MD: U.S. Department of Commerce, National Oceanic and Atmospheric Administration, Office of National Marine Sanctuaries), 105. Available at: https://sanctuaries.noaa.gov/science/condition/fknms/.
Oksanen J., Simpson G., Blanchet F., Kindt R., Legendre P., Minchin P., et al. (2022) vegan: Community Ecology Package_. R package version 2.6–4. Available online at: https://CRAN.R-project.org/package=vegan.
PADI AWARE Foundation. (2022) Dive against debris ® map. Available at: https://www.diveagainstdebris.org.
Parsons K. T., Zimmerman R. A., William S. C. (2023). Colonization of marine debris in the Florida Keys coral reef ecosystem by nonindigenous Sun Corals (Tubastrea Spp.). Bull. Mar. Science. 99, 109–110(2). doi: 10.5343/bms.2022.0059
Precht W. F., Gintert B. E., Robbart M. L., Fura R., Van Woesik R. (2016). Unprecedented disease-related coral mortality in Southeastern Florida. Sci. Rep. 6, 31374. doi: 10.1038/srep31374
QGIS Development Team (2023). QGIS Geographic Information System (QGIS Association). Available at: http://www.qgis.org.
Raimondo G. M., Spinrad R. W., LaBoeuf N. R. (2024). 2023 accomplishments report (NOAA Office Response Restoration. Marine. Debris Program), P.2–P12. Available at: https://response.restoration.noaa.gov/marine-debris-program-releases-2023-accomplishments-report.
R Core Team (2023). R: A language and environment for statistical computing (Vienna, Austria: R Foundation for Statistical Computing). Available at: https://www.R-project.org/.
Renchen G. F., Butler C. B., Matthews T. R. (2021). Marine debris knows no boundaries: Characteristics of debris accumulation in marine protected areas of the Florida Keys. Mar. pollut. Bull. 173 (A), 112957. doi: 10.1016/j.marpolbul.2021.112957
Risk M. J. (1972). Fish diversity on a coral reef in the Virgin Islands. Atoll Res. Bull., 153. doi: 10.5479/si.00775630.153.1
Rochman C. M., Anthony Browne M., Underwood J., Van Franeker J. A., Thompson R. C., Amaral-Zettler L. A. (2016). The ecological impacts of marine debris: unraveling the demonstrated evidence from what is perceived. Ecology. 97, 302–312. doi: 10.1890/14-2070.1
Ross E. P., Butler J., Matthews T. R. (2022). ). Examining the ecological function of structure: species assemblages at casitas and coral heads in the Lower Florida Keys. Mar. Ecol. Prog. Ser. 681, 169–183. doi: 10.3354/meps13926
Shruti V. C., Kutralam-Muniasamy G., Pérez-Guevara F. (2023). Putting eggs on marine litter: Towards an understanding of a cause for concern. Mar. pollut. Bull. 190, 114900. doi: 10.1016/j.marpolbul.2023.114900
Smith S. D., Edgar R. J. (2014). Documenting the density of subtidal marine debris across multiple marine and coastal habitats. PLoS One 9, e94593. doi: 10.1371/journal.pone.0094593
Smith K. M., Payton T. G., Sims R. J., Stroud C. S., Jeanes R. C., Hyatt T. B., et al. (2019). Impacts of consecutive bleaching events on transplanted coral colonies in the Florida Keys. Coral Reefs. 38, 851–861. doi: 10.1007/s00338-019-01823-7
Smith K. M., Quirk-Royal B. E., Drake-Lavelle K., Childress M. J. (2018). Influences of ontogenetic phase and resource availability on parrotfish foraging preferences in the Florida Keys, FL USA. Mar. Ecol. Prog. Ser. 603, 175–187. doi: 10.3354/meps12718
Spanier E., Almog-Shtayer G. (1992). Shelter preferences in the Mediterranean slipper lobster: effects of physical properties. J. Exp. Mar. Biol. Ecol. 164, 103–116. doi: 10.1016/0022-0981(92)90139-2
Takada Y., Abe O., Shibuno T. (2007). Colonization patterns of mobile cryptic animals into interstices of coral rubble. Mar. Ecol. Prog. Ser. 343, 35–44. doi: 10.3354/meps06935
Tebben J., Motti C. A., Siboni N., Tapiolas D. M., Negri A. P., Schupp P. J., et al. (2015). Chemical mediation of coral larval settlement by crustose coralline algae. Sci. Rep. 5, 10803. doi: 10.1038/srep10803
Tebbet S. B., Bellwood D. R. (2019). Algal turf sediments on coral reefs: what’s known and what’s next. Mar. pollut. Bulletin. 149, (2019). doi: 10.1016/j.marpolbul.2019.110542
Teng G., Shan X., Jin X. (2023). Cascade effects of seafloor litter on benthic ecosystems in the northern Yellow Sea. Front. Mar. Sci. 9. doi: 10.3389/fmars.2022.1044232
Thirukanthan C. S., Azra M. N., Lananan F., Sara' G., Grinfelde I., Rudovica V., et al. (2023). The evolution of coral reef under changing climate: A scientometric review. Animals: an Open Access J. MDPI 13, 949. doi: 10.3390/ani13050949
Toth L. T., Courtney T. A., Colella M. A., Kupfner Johnson S. A., Ruzicka ,. R. R. (2022). The past, present, and future of coral reef growth in the Florida Keys. Global Change Biol. 28, 5294–5309. doi: 10.1111/gcb.16295
Toth L. T., Kuffner I. B., Stathakopoulos A., Shinn E. A. (2018). A 3,000-year lag between the geological and ecological shutdown of Florida's coral reefs. Global Change Biol. 24, 5471–5483. doi: 10.1111/gcb.14389
Uhrin A. V., Matthews T. R., Lewis C. (2014). Lobster trap debris in the Florida keys national marine sanctuary: Distribution, abundance, density, and patterns of accumulation. Mar. Coast. Fisheries 6, 20–32. doi: 10.1080/19425120.2013.852638
Keywords: marine debris, coral reefs, Florida Keys, biodiversity, taxa interaction
Citation: Payton TG, Sims RJ and Childress MJ (2024) Abundance, patterns, and taxa associations of anthropogenic marine debris on reefs in the middle Florida Keys. Front. Mar. Sci. 11:1412858. doi: 10.3389/fmars.2024.1412858
Received: 05 April 2024; Accepted: 10 May 2024;
Published: 05 June 2024.
Edited by:
Teresa Bottari, National Research Council (CNR), ItalyReviewed by:
Bilal Mghili, Abdelmalek Essaadi University, MoroccoGunasekaran Kannan, Chulalongkorn University, Thailand
Copyright © 2024 Payton, Sims and Childress. This is an open-access article distributed under the terms of the Creative Commons Attribution License (CC BY). The use, distribution or reproduction in other forums is permitted, provided the original author(s) and the copyright owner(s) are credited and that the original publication in this journal is cited, in accordance with accepted academic practice. No use, distribution or reproduction is permitted which does not comply with these terms.
*Correspondence: Tokea G. Payton, dHBheXRvbkBjbGVtc29uLmVkdQ==