- 1Marine Mammal Research, Department of Ecoscience, Aarhus University, Roskilde, Denmark
- 2Atmospheric Emissions & Modelling, Department of Environmental Science, Aarhus University, Roskilde, Denmark
Introduction: Drones, or Unmanned Aerial Vehicles (UAVs), have emerged as valuable tools for wildlife monitoring, offering potentially non-intrusive observations in challenging terrains like marine ecosystems. Despite their potential, widespread use is impeded by regulatory constraints, especially in protected areas.
Methods: This study aims to assess the impact of varying flight altitudes and flight approaches of two commercial drones on the behaviour of harbour seals (Phoca vitulina) and grey seals (Halichoerus grypus) and compare to existing findings for marine mammals. We conducted a comparative analysis of the response to flight altitude from 70 m gradually descending to 10 m and two different flight approaches between the DJI Phantom 4Pro and the Autel EVO II RTK drones in the Danish part of the Wadden Sea.
Results: We found varying responses, where species-specific sensitivity and environmental conditions appeared to influence the behavioural reactions of the seals. Our results reveal that seal reactions to drones are complex and depend on several factors, such as flight altitude, drone model, received noise levels, approach, weather conditions, the animals’ annual cycle, and geographic location.
Discussion: The outcomes hold significance for refining regulatory policies governing drone flights in protected marine environments, balancing conservation efforts with technological advancements in wildlife monitoring and ensuring undisturbed observations of seals.
Introduction
Unmanned Aerial Vehicles (UAVs), commonly known as drones, have become increasingly prevalent in marine wildlife monitoring and management (Gonzalez et al., 2016). These relatively inexpensive devices may enable regular surveillance of marine mammal populations, providing valuable insights into distribution, density, behaviour, growth, and seasonal variations. However, the widespread adoption of drones in these contexts presents multifaceted challenges, particularly in navigating regulatory frameworks aimed at protecting sensitive habitats and species. Regulatory policies governing drone flights in protected marine environments must navigate this delicate terrain. Studies on the impact of drones on different species vary significantly, making it unwise to assume uniform effects across species and drone models (Mustafa et al., 2018; Álvarez-González et al., 2023). In our investigation, we delve into these complexities and propose strategies to mitigate potential disturbances caused by drones. Specifically, we aim to assess the impact of varying flight heights and flight approaches of two commercial drones on the behaviour of harbour seals (Phoca vitulina) and grey seals (Halichoerus grypus) in the Danish part of the Wadden Sea.
The perception of risk by wildlife is influenced by various factors, including the characteristics of the drone itself, the way it is deployed, its size, noise emissions, flight patterns and speed (Stankowich and Blumstein, 2005; Tablado and Jenni, 2015). The transmission of drone noise into the underwater environment is limited due to reflections, reducing bioacoustic impacts compared to traditional observation platforms like motorized boats (e.g (Slabbekoorn et al., 2010; Erbe et al., 2012)). For scientific use of drones, in addition to conservation issues, it is also important not to impact the behaviour of the animals to avoid biased data. Additionally, it minimises the likelihood that animals underwater will respond to the observation platform, thereby avoiding data bias. However, animals resting on land may be affected by drone noise and the noise in the air should therefore be considered carefully.
While noise levels and visual stimuli from drones can elicit responses from both cetaceans and pinnipeds, the degree of reaction varies among species and individuals according to their hearing sensitivity (Fettermann et al., 2019; Duporge et al., 2021). Previous studies have documented diverse behavioural responses, ranging from no reactions for larger cetacean species (Domínguez-Sánchez et al., 2018; Torres et al., 2018; Christiansen et al., 2020; Fiori et al., 2020; Castro et al., 2021; Álvarez-González et al., 2023) and common bottlenose dolphins (Tursiops truncatus) (Castro et al., 2021), to pronounced changes in behaviour such as tail slaps, reorientation, changes in speed or surfacing patterns in beluga whales (Delphinapterus leucas) (Palomino-González et al., 2021), bottlenose dolphins (Ramos et al., 2018; Fettermann et al., 2019; Giles et al., 2021), common dolphins (Delphinus delphis) (Castro et al., 2021), and Antillean manatees (Trichechus manatus) (Ramos et al., 2018; Landeo-Yauri et al., 2022), depending on factors such as drone altitude, species sensitivity, and environmental conditions (Erbe et al., 2017; Ramos et al., 2018; Castro et al., 2021; Palomino-González et al., 2021).
Pinnipeds that rest on land exhibit distinct responses to drone presence, with behaviours such as increased vigilance and flush responses, where the animals rush into the water, have been observed at lower flight altitudes (Pomeroy et al., 2015; Sweeney et al., 2015; McIntosh et al., 2018; Laborie et al., 2021; Palomino-González et al., 2021; Tadeo et al., 2023). Previous studies on drone observations of pinniped species have indicated substantial variability in the minimum flight altitude that does not disrupt seal behaviour, ranging from 120 m over harbour seals (Phoca vitulina) (Palomino-González et al., 2021) to 15 m over California sea lions (Zalophus californianus) (Adame et al., 2017) across different drone models (Table 1). Unfortunately, the methods for determining whether the animals were disturbed or not, also vary from visual on-site ground observations (Goebel et al., 2015; Sweeney et al., 2015; Adame et al., 2017; McIntosh et al., 2018; Laborie et al., 2021), visual observations during overflight (Perryman et al., 2010; Goebel et al., 2015; Pomeroy et al., 2015; Laborie et al., 2021; Seganfreddo et al., 2023), stationary recordings from the ground collected 120 m away from the pinnipeds (Krause et al., 2021; Palomino-González et al., 2021), to post-analysis of photos (Pomeroy et al., 2015; Krause et al., 2021; Laborie et al., 2021) or continuous recordings (Tadeo et al., 2023) collected from the drone. Evaluating the details of disturbance on-site or from a horizontal perspective introduces some bias regarding coverage of all individuals and behavioural reactions depending on visibility. Adame et al. (2017) showed that the seal counting accuracy was higher using a drone compared to boat based observations, yet still used the latter to determine the level of disturbance of California sea lions for drone altitudes ranging from 40-10 m. The drone operations primarily focused on capturing images for seal counting rather than observing behavioural cues indicative of disturbance, hence the use of boat-based observations for disturbance assessment. Still images from the drone provide a better overview of the seals’ body position, but only at the specific time when the photo was taken, whereas continuous recordings allow for behavioural determination during the entire exposure. It is crucial to note that studies primarily concentrating on photogrammetry or individual counting may inadvertently overlook significant behavioural cues, particularly at higher altitudes than concluded based on on-site observations, potentially introducing bias in disturbance assessments.
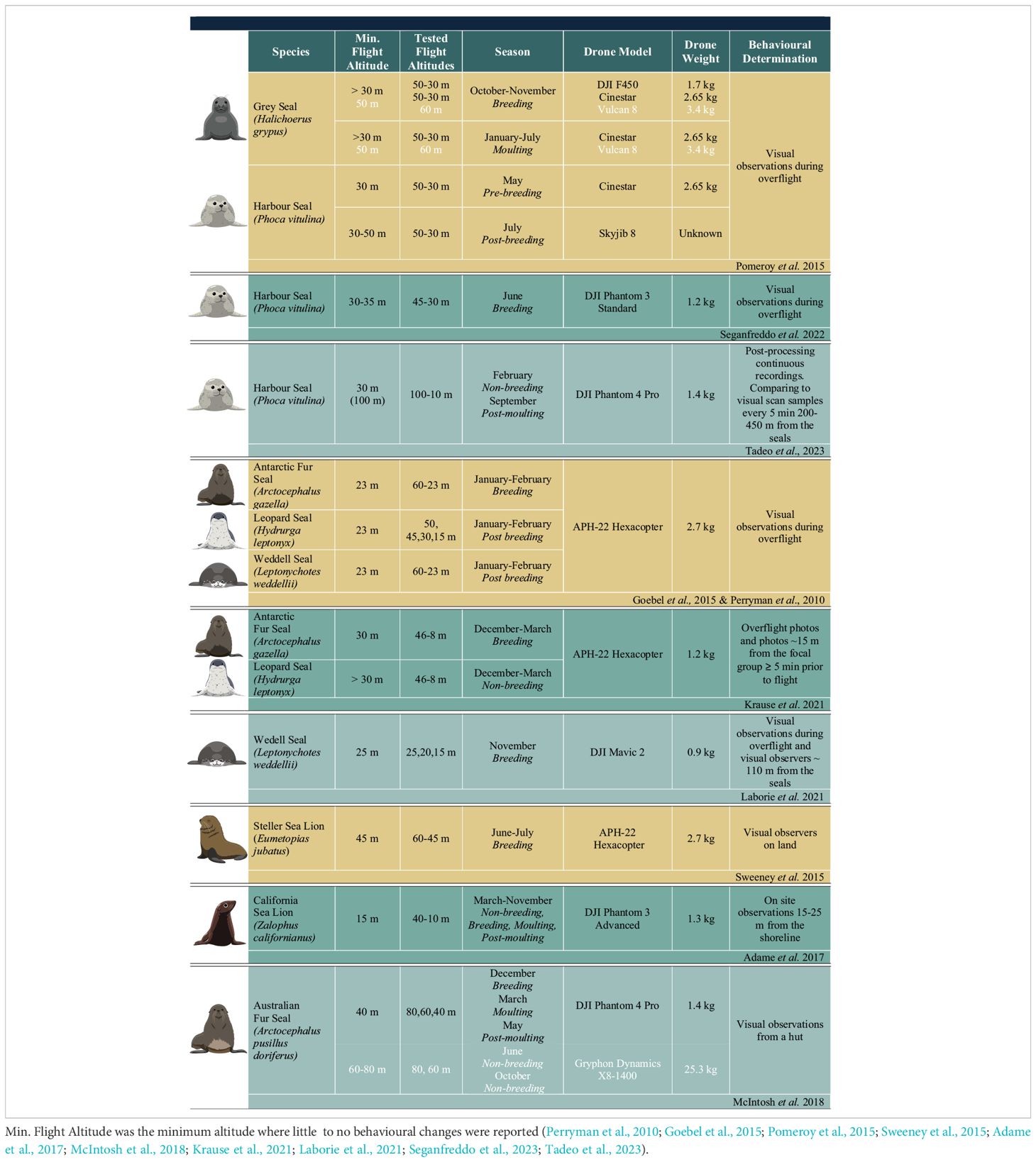
Table 1. Minimum flight altitude (m) with no disturbance observed for various rotor drone models over pinniped species.
Determining the optimal flight altitude that minimises disturbance while maximising data collection efficiency remains a challenge, given the variability in species, seasons, geography, drone characteristics (weight, propulsion system, shape, lights, and size) and flight pattern (speed, altitude, and approach angle) (Goebel et al., 2015; Pomeroy et al., 2015; Smith et al., 2016; Adame et al., 2017; Krause et al., 2017; Mulero-Pázmány et al., 2017; Krause et al., 2021; Laborie et al., 2021; Álvarez-González et al., 2023). This study aims to contribute valuable insights by systematically evaluating the behavioural responses of grey and harbour seals in the Danish part of the Wadden Sea to different flight altitudes and approaches from two commercial drones: a DJI Phantom 4 Pro and an Autel Evo II RTK. While acknowledging the variability across species, sites, and research aim, our goal is to provide a methodological framework that can serve as a foundation for best practices in drone-based wildlife monitoring, recognising that local conditions and specific research objectives may necessitate adjustments.
Methods
The study was conducted at two distinct haul-out sites within the Danish Wadden Sea (Figure 1), spanning from April 23rd to May 31st, 2022, with data collection occurring between 8 am and 11 pm, contingent upon tidal conditions for Jørgens Lo, which was only accessible for the seals to haul out on during low tide, as it is otherwise submerged. A DJI Phantom 4 Pro (1.4 kg) and an Autel EVO II RTK drone (1.2 kg) were utilised in conjunction with an iPad Air 2 running the DJI APP and a Samsung Galaxy Tab Active 3 (Enterprise Edition) operating the Autel Explorer App for navigation and real-time behavioural assessments on-site. The Phantom 4 Pro was equipped with low-noise propellers with an aerodynamic design that is supposed to reduce the noise of the drone according to the manufacturer.
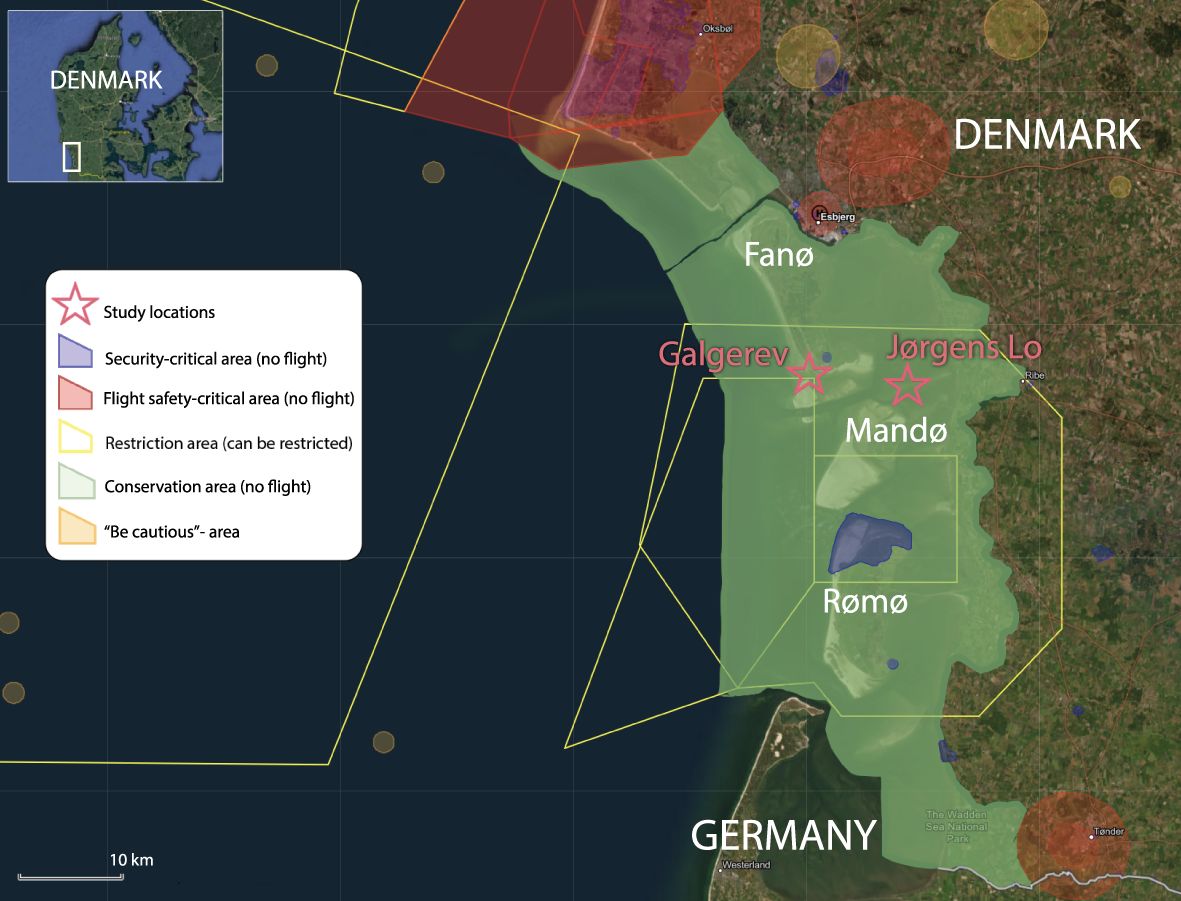
Figure 1. Map of study sites Galgerev and Jørgens Lo (stars), including the restriction areas for drone flight in the Danish Wadden Sea (www.dronezoner.dk). Flight in these areas requires a permit from The Danish Civil Aviation and Railway Authority (blue, green, and red areas), The Danish Defence Command (blue), The Danish Environmental Protection Agency (green) or Notice To AirMen (red).
Drone flights were exclusively conducted during dry weather conditions with wind speeds of ≤ 10 m/s, launching from a position approximately 500 m horizontally distant from the haul-out sites, outside the range of disturbance.
This study was conducted pre-breeding for harbour seals and post-moulting for grey seals, so we deliberately initiated flights at an altitude sufficiently elevated to mitigate any potential influence on seal behaviour. The drone ascended vertically to an altitude of 70 m immediately after take-off and then proceeded to approach the haul-out site horizontally, maintaining a maximum speed of 9 m/s. Upon reaching approximately 100 m from the seals, video recordings commenced, following a speed reduction to a maximum of 4 m/s. As the drone closed in to within approximately 20 m of the hauled-out seals, the speed was further reduced to a maximum of 2.5 m/s until the group of seals was within vertical view (90°) of the camera.
Two flight protocols were tested (Figure 2):
1) The LawnMower approach, the drone flew directly over all the seals with an average flight speed ranging from 0.5–2.5 m/s, resembling the flight protocol for photogrammetry where overlapping photos are collected (Goebel et al., 2015; Adame et al., 2017; Mulero-Pázmány et al., 2017). Once all the seals had been recorded, the drone would continue past them, descend vertically, and follow the same protocol. This was repeated from 70 to 15 m, with 5-10 m intervals, depending on the number of seals and the weather conditions that both influenced the flight time of the drones. DJI Phantom 4Pro did not have enough battery capacity to include 15 m, so the flight had to be abandoned and therefore this altitude was only tested for Autel Evo II RTK. With large haul-out groups and/or windy weather, it was not possible to get sufficient flight time to do 5 m interval, and therefore 10 m intervals were performed instead.
2) Direct approach, the drone hovered at 70 m above a group of seals (all seals or a randomly selected part of the group) that would fit in the field of view of the camera and recorded for 30-60 seconds, before descending 5-10 m depending on the battery flight time of the drone and the weather conditions. Here, the number of seals was not influencing the flight time, as the drone stayed with the same group of seals throughout the trial and did not fly over the entire haul-out site. This was done down to 15 m altitude with an average decent speed was 0.5 m/s.
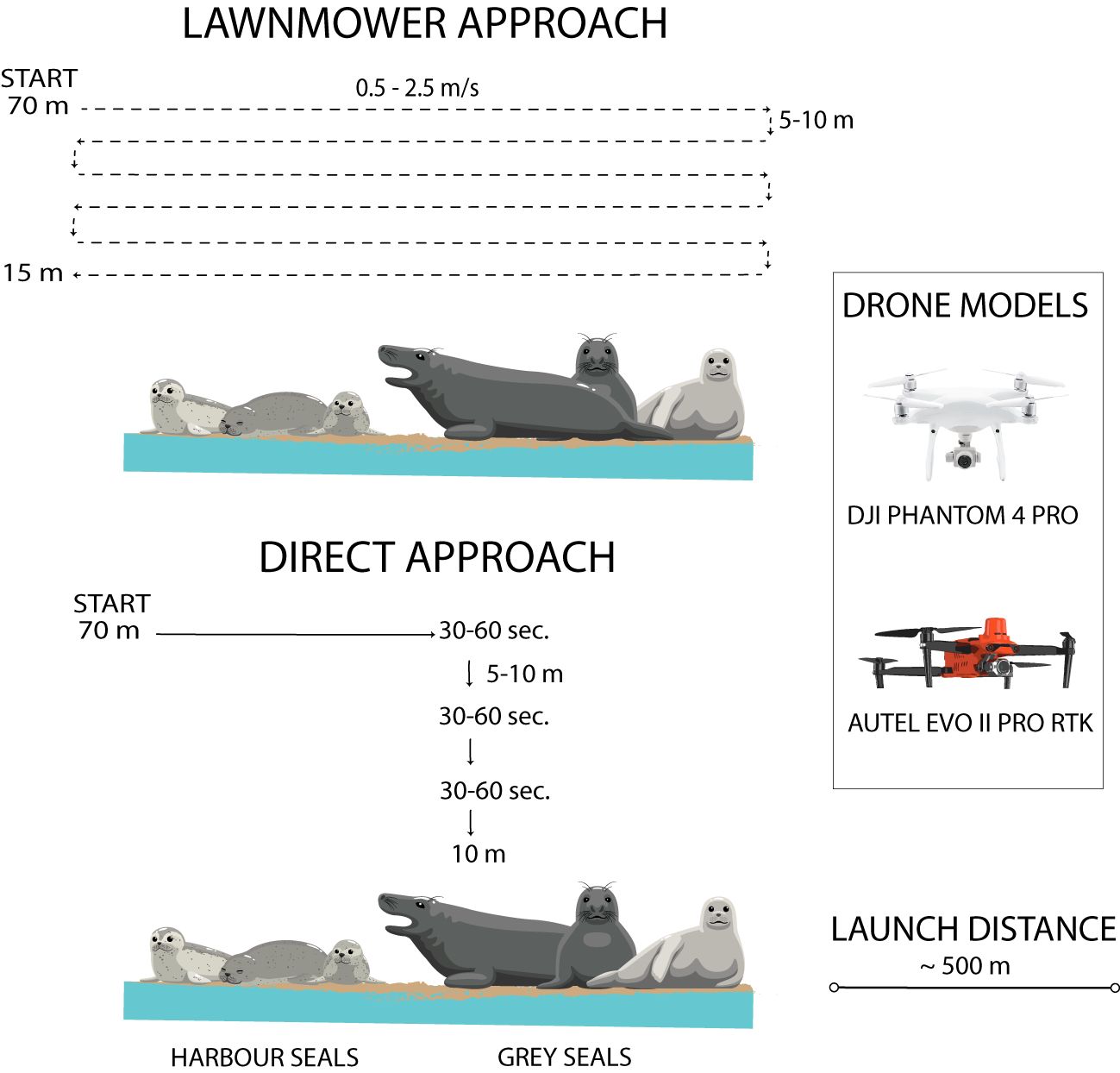
Figure 2. Overview of the two flight protocols starting at 70 m altitude; Top LawnMower approach, speed of 0.5-2.5 m/s, descent of 5-10 m (depending on conditions) and descent to 15 m. Bottom Direct approach, hovering for 30-60 sec. at each altitude, descent of 5-10 m (depending on conditions) and down to 10 m altitude with descent speed ≤ 0.5 m/s. Two drone models were tested, with identical methods, launched ~ 500 m from the haul-out site.
To reduce the risk of affecting any non-target species near the ground such as resting birds, the drones ascended to 70 m before returning to the take–off location after each trial (see Supplementary Material for flight altitudes over birds).
As the study was conducted outside the moulting and breeding seasons of both species, the negative impacts of the experiment were deemed to be modest. It was prioritised to obtain complete data sets including observations from all heights, and flights were not interrupted after seals started to flush into the water.
No more than one flight was performed per day at each of the two locations. However, it should be noted that within a single flight session, flight altitudes were systematically reduced from 70 m down to 15-10 m at 5-10 m intervals, resulting in multiple exposures at varying altitudes during the course of the flight. This approach resulted in cumulative exposures rather than entirely independent exposures at each altitude. We prioritised reducing the number of independent flight exposures over decoupling the altitude from flight time because this would result in many more flight exposures. Furthermore, previous studies indicate that harbour seals are less disturbed by vehicles approaching gradually from the sea in a parallel angle (Allen et al., 1984; Suryan and Harvey., 1999; Johnson and Gutiérrez, 2007) rather than directly (Henry and Hammill, 2001). We assumed that approaching gradually at higher altitudes would result in less disturbance and allow us to achieve a lower flight altitude compared to starting the approach at lower altitudes. Given this methodology, it is important to acknowledge that the altitude variable is confounded by total flight time, with each flight comprising continuous exposures across different altitudes (as illustrated in Figure 2).
Flight logs were uploaded to www.airdata.com and retrieved as .csv files for flight altitudes, speed and GPS coordinates correlated to the timing of the recordings. Weather data were collected from The Danish Meteorological Institute (www.dmi.dk) specifically for Fanø and Mandø, the UAV Forecast App and the lunar phase from www.timeanddate.com.
Recordings were analysed in Wondershare Filmora 13 for sufficient zoom function at altitudes exceeding 30 m. The behaviour of each seal was determined using the ethogram in Table 2, and the level of disturbance was determined from 0-5 with 0 being no disturbance effect, and 5 being the highest level of disturbance with flush response. The behaviour was analysed using all the frames during the overflight for the LawnMower approach, and 1 second after descending to a certain altitude in the Direct approach.
Legal compliance
The drone pilot (ES) was registered at the European Aviation Safety Agency in category A1/A3 and A2 by The Danish Civil Aviation and Railway Authority. The drones were flown in compliance with Commission Implementing Regulation (EU) 2019/947 of 24 May 2019 on the rules and procedures for the operation of unmanned aircraft, with a dispensation given for paragraph 9 regarding nature conservation areas. The Danish Civil Aviation and Railway Authority approved the content of this study to be qualified as public surveillance and monitoring animals, partly funded by The Danish Environmental Protection Agency. The drones were flown according to The Commission Implementing Regulation (EU) of 24 May 2019 on the rules and procedures for the operation of unmanned aircraft: the flight height must not exceed 120 m and must always be flown within sight. The local military station was informed about every flight. In addition, the drones were included in Aarhus University’s insurance policy, providing public liability cover.
Noise level measurements
To compare the potential noise difference between the drone models, we performed an experiment to estimate noise exposure levels (equivalent sound pressure; peak hold) at the applied flight altitudes for the two drones used in this study, DJI Phantom 4 Pro, Autel Evo II Pro RTK, but also two additional drones, a DJI Mavic 2 and a Mavic 3 at an experimental site at Aarhus University, Roskilde, Denmark. We included the two DJI Mavic models to compare with other commonly used drone models. Wind conditions during the experiment were 2-6 m/s with -1°C. Each drone hovered at each altitude for 30 seconds while we recorded the noise level using a portable sound level meter (MK3) with Class 1 accuracy (sampling frequency: per second). The sound recorder was calibrated using a standard sound calibrator at 90 dBA. The recorder was placed 0.5 m above ground on a tripod and the drones were hovering above the microphone at 10 to 70 m in 5 m intervals. A full frequency spectrum (20 – 162500 Hz) at 1/3 octave band frequencies was used to record the peak-hold equivalent sound pressure levels (Leq) in dB.
The recorded sound levels were retrieved from the sound recorder and subsequently processed in R Studio (2022.07.2) (Team R, 2022). The recorded data were averaged for each frequency centre band and altitude. To assess the perceived loudness, we quantified the noise produced by the drones using an audiogram-weighted received sound level, measured in dB re 20μPa, with a specific focus on the impact of this noise on phocid carnivores in an airborne environment [as discussed by (Southall et al., 2007)].
Data analysis
We employed ordinal logistic regression to analyse the factors influencing disturbance levels. This model handles ordinal response variables, such as the severity levels of disturbance categorized from 0 to 5, while simultaneously considering multiple predictor variables. This allows us to examine how various factors contribute to changes in the severity of disturbances. The model formula included the disturbance level as the ordinal response variable and several predictor variables, namely species, gestation status, drone model, drone altitude (in meters), approach type, number of drone exposures (the number of individual flights at that location), location, wind speed (in meters per second), cloud cover (in percentage), tidal state, and lunar phase. Gestation status was evaluated by the large circumference on the lower body half of the harbour seals. Wind speed was incorporated into the model because stronger winds have the potential to increase or decrease the noise level emitted by the drones’ motors while maintaining their position, or it could mask or carry away drone noise. This increase or decrease in noise could contribute to altered disturbance levels. Cloud cover was included as a predictor variable due to its impact on lighting conditions, altering the visibility of the drones. Lunar phase was selected based on previous findings of tendencies to venture into the sea more frequently following full moon phases (Cronin et al., 2009) and potentially lower levels of alertness after new moon phases (Speakman et al., 2019).
The analyses were conducted in the R Studio (2022.07.2) programming environment (Team R, 2022) utilizing the MASS package (Venables and Ripley, 2002) for fitting the ordinal logistic regression model. Specifically, the polr function from the MASS package was used to fit the proportional odds logistic regression model to the data. Model summaries and diagnostics were obtained using built-in functions available in R.
Model diagnostics including the likelihood ratio tests, Wald tests, and examination of the residual deviance were performed to assess the overall goodness-of-fit and the significance of individual predictor variables. To ensure the robustness of the model estimates and to account for potential issues such as non-convergence, the model was refitted using the update function applied to the original model object. This step helped in obtaining reliable estimates of coefficients and associated statistics. The statistical significance of the coefficient estimates was assessed using Wald tests, and corresponding p-values were computed. A significance level of 0.05 was used to determine the statistical significance of the predictor variables.
Results
The study comprised a total of 39 flights, with 20 flights conducted at Jørgens Lo and 19 at Galgerev, spanning 23 days. On average, the seals had an exposure time of 13.8 min. (range = 6-25 min.), which varied depending on the drone battery capacity and/or the duration of data collection for each of the altitudes (70-10 m, see Figures 3, 4). The Autel Evo II RTK drone had the longest exposure time during both approaches, with 18.8 min. for LawnMower compared to 15.8 min. for the DJI Phantom 4 Pro, and 11.9 min. for Direct compared to 9.3 min. The mean group size was 60 individuals (range = 4-104) at Galgerev and 170 at Jørgens Lo (range = 8-296), and the cumulative video analysis duration was 9.14 hours.
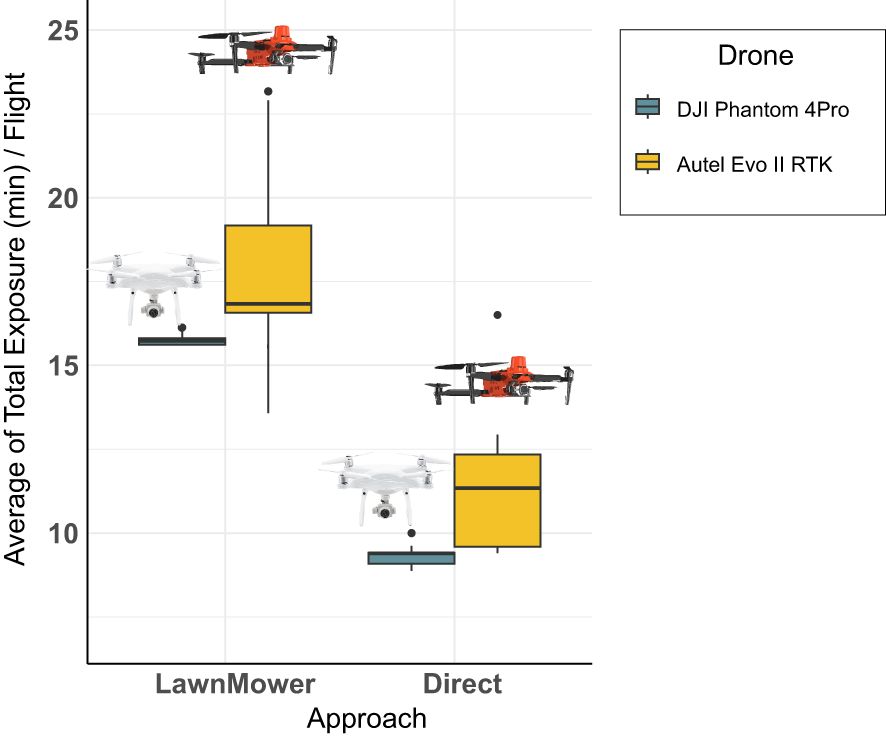
Figure 3. Average of total exposure time (min) per flight, during the LawnMower (left) and Direct (right) approach for DJI Phantom 4 Pro (green) and Autel Evo II RTK (yellow).
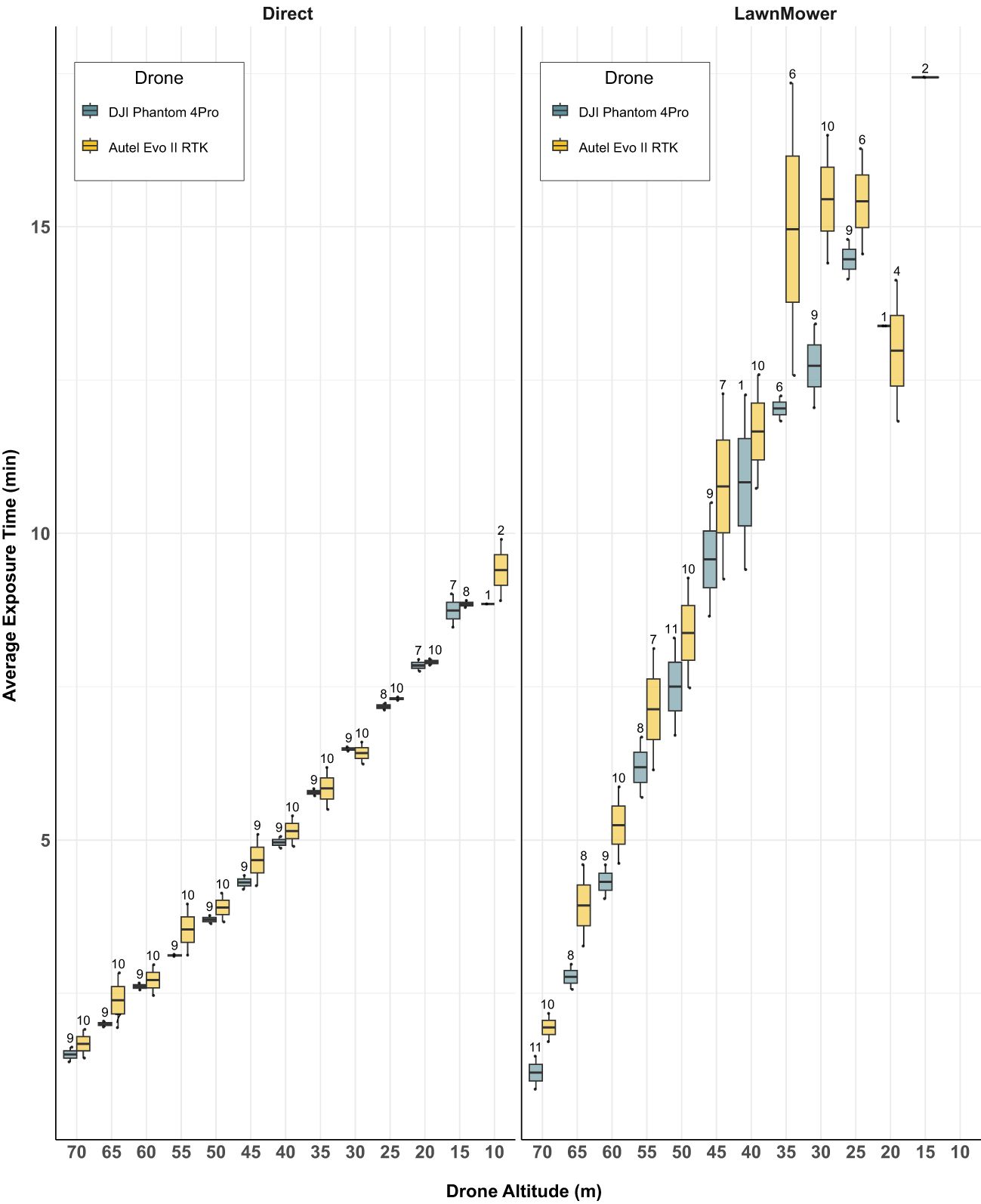
Figure 4. Average exposure time (min) per flight at each flown drone altitude (m), during the LawnMower (left) and Direct (right) approach for DJI Phantom 4 Pro (green) and Autel Evo II RTK (yellow). The altitudes are arranged in reverse order to reflect the exposure method, from high to low altitude. The number of individual flights is included above each box.
Grey seal behaviour
LawnMower approach
With DJI Phantom 4 Pro, a decline in grey seals’ resting behaviour was observed from 59-65% at 70-55 m altitude, to 31-38% from 50-30 m, and 24-0% from 25-20 m. The observant behaviour remained relatively stable from 10-17% from 70-20 m, whereas the vigilance increased from 3-6% at 70-50 m, to 16-25% from 45-25 m, to 50% at 20 m. Displacement increased from 0-4% at 40 m, to 11-24% at 20-25 m. At 50 m 14% of the grey seals displayed flush behaviour and at 20 m it was up to 17% (Figure 5). There was not enough battery to reach 15 m for the DJI Phantom 4Pro during the LawnMower approach.
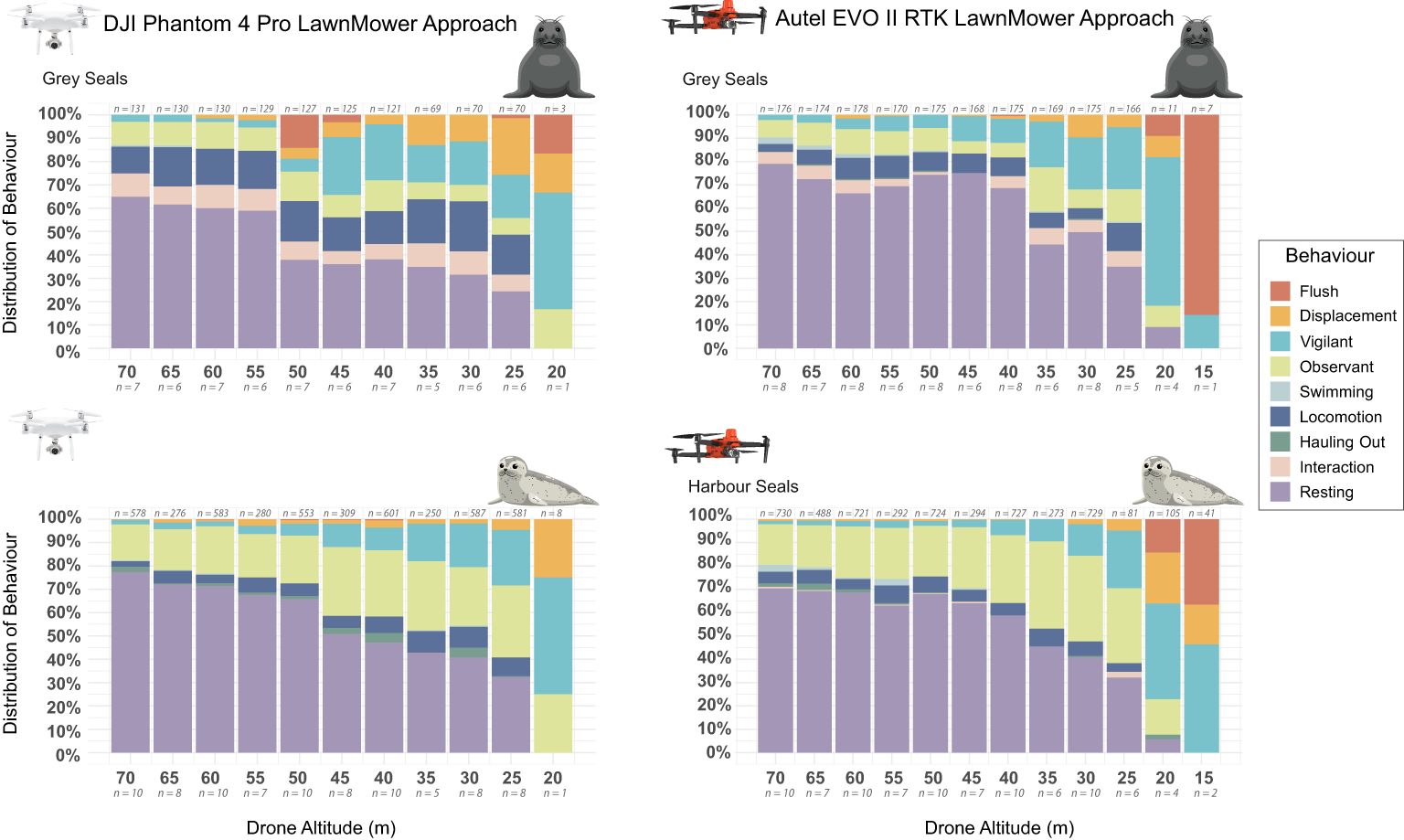
Figure 5. Distribution of behaviours (in percentage) during the LawnMower approach from 70-20 m altitude for DJI Phantom 4 Pro (left) and 70-15 m for the Autel Evo II RTK (right) over grey seals (top) and harbour seals (bottom). The altitudes are arranged in reverse order to reflect the exposure method, from high to low altitude. The cumulative number of seals during all flights is noted on top of the stacked bar chart, and the number of flights is noted on the bottom on the x-axis.
For the Autel EVO II RTK drone, the grey seals showed a decline in resting behaviour from 66-79% at 70-40 m, which decreased to 50-44% at 35-30 m, 35% at 25 m, down to 9-0% at 20-15 m. The observance varied from 5-14% down to 20 m. Vigilance increased from 5-11% at 70-40 m to 20-27% from 35-25 m, up to 64% at 20 m, and 14% at 15 m. The low vigilance rate at 15 m was due to 86% of the grey seals already having flushed into the water. At 20 m, 9% of the animals showed flush behaviour. Displacement accounted for 0-3% of the behaviours down to 35 m, and from 30-20 m it increased to 5-10% (Figure 5).
Direct approach
During the Direct approach with the DJI Phantom 4 Pro we observed 68-73% resting behaviour from 70-50 m, which decreased to 41-55% at 40-20 m, 27% at 15 m, and 0% at 10 m. Observant behaviour was relatively stable at 5-14% throughout 70-15 m. The vigilant behaviour increased from 6-10% at 70-50 m, to 17-28% at 45-20 m, and 39% at 15 m. The displacement behaviour increased from 1-4% from 70-20 m, to 11.2% at 15 m, and 100% at 10 m (Figure 6).
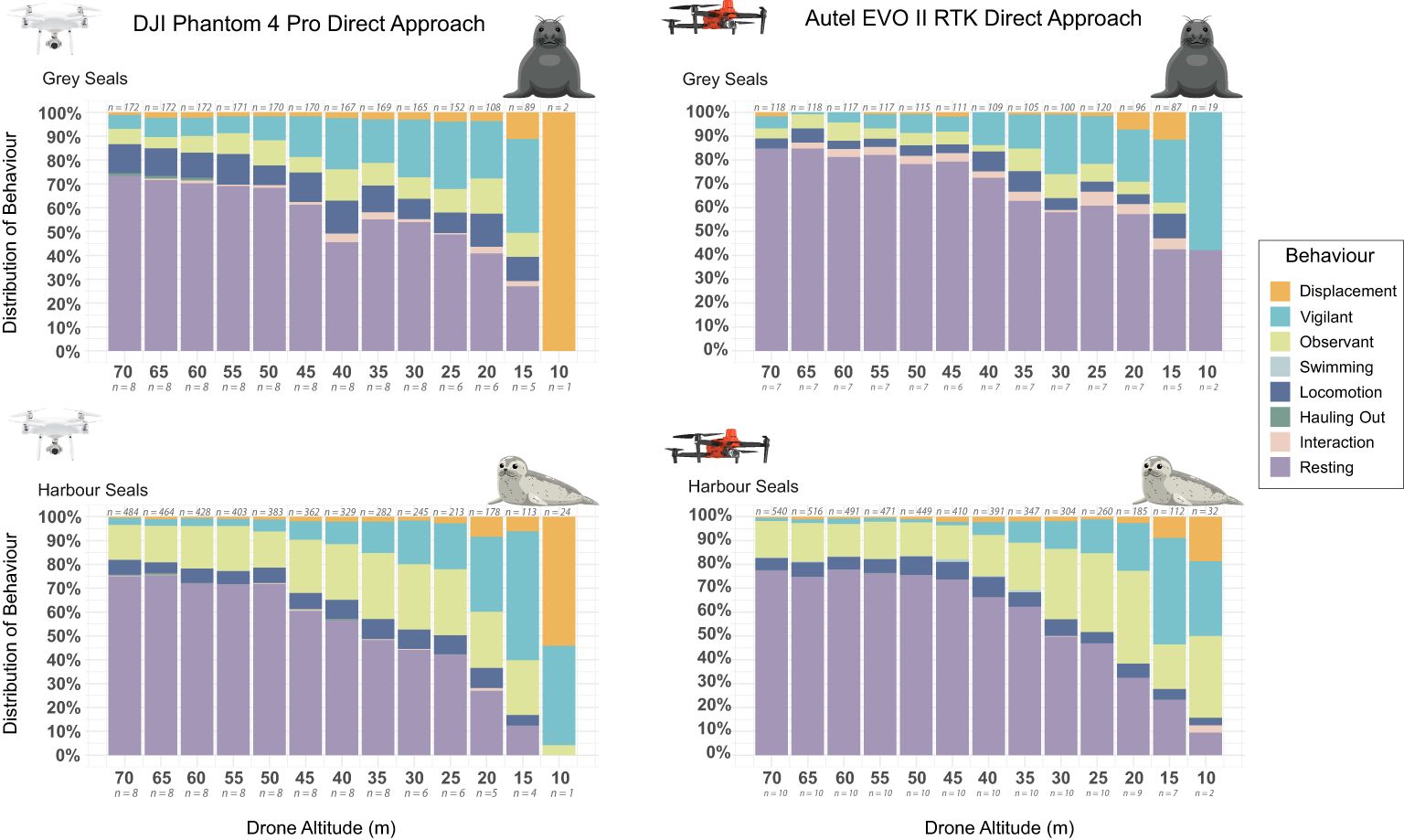
Figure 6. Distribution of behaviours (in percentage) during the Direct approach from 70-10 m altitude for DJI Phantom 4 Pro (left) and Autel Evo II RTK (right) over grey seals (top) and harbour seals (bottom). The altitudes are arranged in reverse order to reflect the exposure method, from high to low altitude. The cumulative number of seals is noted on top of the stacked bar chart, and the total number of flights is noted on the bottom on the x-axis.
For the Autel EVO II RTK the grey seals showed 73-85% resting behaviour from 70-40 m, which decreased to 57-63% from 35-20 m, and 42% at 15-10 m. Observant behaviour was relatively stable from 4-10% from 70-15 m. Vigilance increased from 1-8% at 70-45 m, to 20-26% from 30-15 m and 58% at 10 m. At 20-15 m the displacement behaviour increased to 7-12%, while only 1-2% was displaced at 70-25 m (Figure 6).
Harbour seal behaviour
LawnMower approach
DJI Phantom 4 Pro reduced the harbour seals’ resting behaviour from 66-77% from 70-50 m altitude, to 41-51% at 45-30 m, and 32-0% at 25-20 m. The observant behaviour was 15-20% from 70-50 m, after which it increased to 25-31% at 45-20 m. Vigilance increased from 2-5% at 70-50 m to 10-24% from 25-45 m, and at 20 m it accounted for 50% of the seals’ behaviour. The displacement behaviour increased from 0-5% for 70-25 m, to 25% at 20 m (Figure 5).
The Autel EVO II RTK showed a reduction in resting from 63-70% at 70-45 m, 59% at 40 m, 41-45% at 35-30 m, 32% at 25 m, and 6-0% from 20-15 m. Observance went up from 17-22% at 70 to 50 m, 26-29% from 45-40 m, 29-37% from 35-25 m, and then decreased to 15% at 20 m, when many seals had already flushed into the water. Vigilance went up from 2-3% from 70-45 m, to 7-13% from 40-30 m, up to 25% at 25 m, and 41-46% from 20-15 m. At 30-25 m, the harbour seals started to show displacement behaviour with 2-5%, which increased to 17-22% at 25-15 m. At 20 m they started flushing into the water with a flush response of 14% which increased to 37% at 15 m (Figure 5).
Direct approach
With the DJI Phantom 4 Pro, the harbour seals reduced resting behaviour from 72-75% at 70-50 m to 57-61% at 45-40 m, 42-48% at 35-25 m to 27% at 20 m, 12% at 15 m. The observant behaviour changed from 15-32% from 70-40 m to 23-27% from 45-15 m. Vigilance increased from 3-5% from 70-50 m, 8-13% from 45-35 m, 18-19% at 30-25 m, 32% at 20 m, 54% at 15 m. At 20 m the harbour seals started to increase their displacement behaviour from 1-3% to 8%. At 10 m 42% was vigilant and 54% show displacement behaviour (Figure 6).
With the Autel EVO II RTK drone, the harbour seals reduced their resting behaviour from 74-78% at 70-45 m, to 66-62% at 40-35 m, 50-47% at 30-25 m, 32.4% at 20 m, 23% at 15 m and 9% at 10 m. Their observant behaviour changed from 14-20% from 70-35 m, to 30-39% at 30-10 m. Vigilance accounted for 1-5% of the behaviour at 70-40 m, increased to 9-14% at 35-25 m, and from 20% at 20 m and 45-31% from 15-10 m. At 15 m displacement behaviour increased from 2% at 70-20 m, to 9% and at 10 m it increased to 19% (Figure 6).
No flushing was observed during the Direct approach for either drone.
The average disturbance level across various flight approaches is depicted in Figure 7, as outlined by the ethogram detailed in Table 2. Initially, both approaches exhibited a low disturbance level, ranging between 0.2 to 0.3, for encounters with both grey and harbour seals. However, with the DJI Phantom 4 Pro, the disturbance level escalated to 0.5 or higher when the drone reached 45 m altitude, except for instances of the LawnMower approach over grey seals, where it peaked at 1.1 at 50 m. Conversely, with the Autel Evo II RTK, the disturbance level surpassed 0.5 at 35 m altitude for the LawnMower approach across both seal species, and at 30 m for the Direct approach. Notably, for this drone model, the disturbance level exceeded 1 at 30 m for harbour seals and 20 m for grey seals during the LawnMower approach, and at 15 m during the Direct approach for both species. Subsequently, between 20 to 15 m altitude, the disturbance level spiked from 2.2 to 4.6 for the Autel drone during LawnMower approach over grey seals, and from 2.5 to 3.4 for harbour seals. No DJI Phantom 4 Pro flights sustained sufficient battery power to descend to lower than 20 m, however, at 20 m, the disturbance level reached 2.2 for harbour seals and 2.5 for grey seals. During the Direct approach, the DJI Phantom 4 Pro achieved a disturbance level of 3 for harbour seals and 3.5 for grey seals at 10 m altitude, while the Autel recorded levels of 1.6 and 1.2 for harbour and grey seals, respectively. Notably, it was a limited sample size of only three flights that informed the 10 m observations.
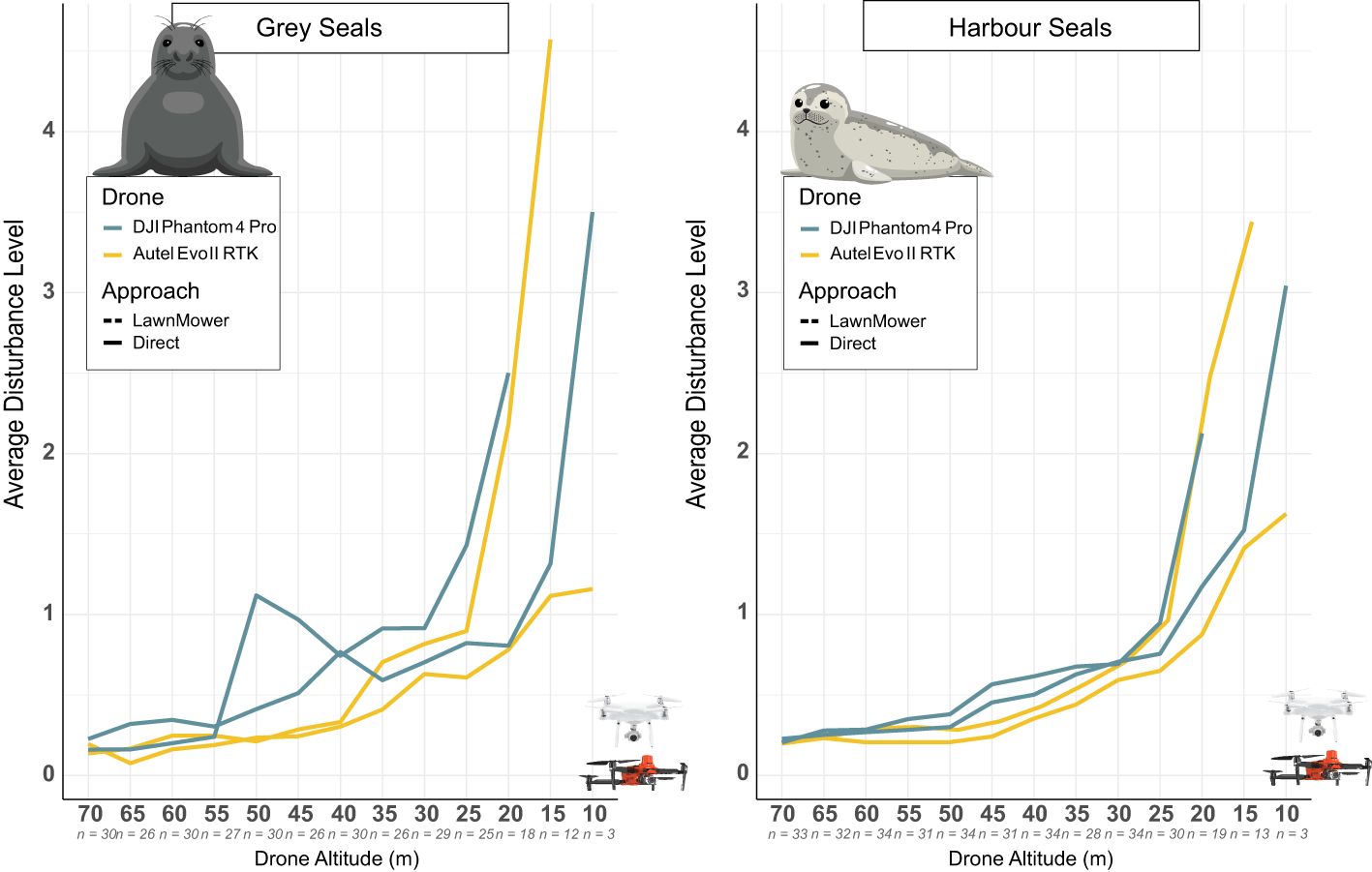
Figure 7. Average disturbance level, based on the behaviours in Table 2 for grey seals (left) and harbour seals (right), when exposed to LawnMower (dashed) or Direct (solid) approach of DJI Phantom 4 Pro (blue) and Autel Evo II RTK (yellow) from 70-10 m altitude. The altitudes are arranged in reverse order to reflect the exposure method, from high to low altitude. The total number of flights is noted on the bottom on the x-axis.
Factors influencing disturbance levels
An ordinal logistic regression model was employed to explore the factors influencing disturbance levels in the study area, considering a scale of seal disturbance ranging from 0 to 5, except for observance (level 1) (Table 3). Due to interspecies specific difference in the observance behaviour (level 1), where harbour seals in general have a higher level of observance than grey seals, we did not include this level in the analysis (see Figures 5, 6). The analysis revealed significant associations between several predictor variables and disturbance levels, as summarized in Table 3:
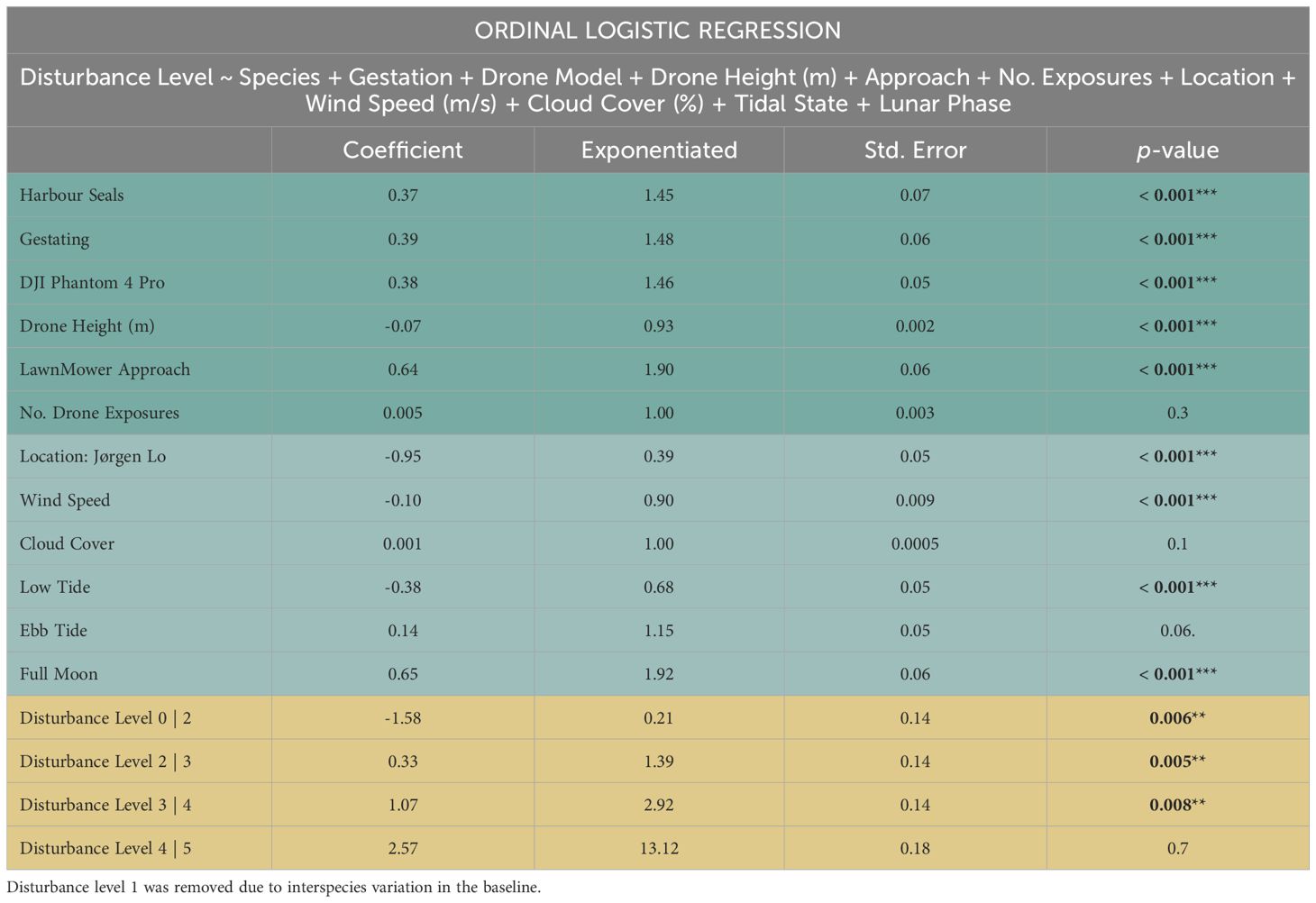
Table 3. Ordinal logistic regression analysis of factors influencing seal response to disturbance levels, including species, drone parameters, environmental conditions, and location.
Harbour seals were 54.1% more likely to increase in disturbance levels compared to grey seals (coefficient = 0.37, exp= 1.45, p < 0.001), and gestating harbour seals had 47.7% higher odds of disturbance compared to non-gestating or undefined individuals (coefficient = 0.39, p < 0.001).
The use of the DJI Phantom 4 Pro drone resulted in 46.5% higher odds of disturbance compared to the Autel EVO II RTK (coefficient = 0.38, p < 0.001). Additionally, for each 5 m increase in drone altitude, the odds of disturbance decreased by approximately 7.1% (coefficient = -0.07, p < 0.001).
The LawnMower approach increased the odds of disturbance by 90.2% compared to the Direct approach (coefficient = 0.64, p < 0.001). The number of exposures did not have a significant effect (coefficient = 0.005, p = 0.3).
The location of Jørgens Lo, with the highest average number of seals (170), was associated with a 61.4% lower disturbance odds ratio, compared to Galgerev, which had a lower average number of seals (60) (coefficient = -0.95, p < 0.001).
The weather conditions also influenced disturbance levels, with increasing wind speed from 1-10 m/s reducing disturbance levels by approximately 9.4% (coefficient = -0.10, p < 0.001). Increasing cloud cover in percent did not have a significant effect on disturbance levels (coefficient = 0.001, p = 0.1).
During low tide, there was a 31.4% decrease in the odds of disturbance (estimate = -0.38, p < 0.001), while ebb tide had 15% higher odds of disturbance though the effect was not significant (coefficient = 0.14, p = 0.06). At full moon there was 91.7% higher odds of disturbance compared to other lunar phases (coefficient = 0.65, p < 0.001).
Each transition between disturbance levels (e.g., from level 0 to level 2, from level 2 to level 3, and so on) was assessed to understand how the odds of experiencing a disturbance change as we move from one level to another. The coefficient for transitioning from disturbance level 0 to 2 was estimated at -1.58 (p = 0.006), indicating that the odds of experiencing a disturbance at level 2 versus level 0 decreased by approximately 79% when considering other predictor variables. Transitioning from disturbance level 2 to 3 was associated with a coefficient of 0.33 (p = 0.005), indicating a 61% increase in the odds of experiencing a disturbance at level 3 versus level 2, holding other factors constant. The coefficient for transitioning from disturbance level 3 to 4 was estimated at 1.07 (p = 0.008), suggesting a notable increase in the odds of experiencing a disturbance at level 4 versus level 3. Transitioning from disturbance level 4 to 5 showed a coefficient of 2.57 (p = 0.7), indicating a substantial increase in the odds of experiencing a disturbance at level 5 versus level 4, although this result was not statistically significant.
Noise levels
In Figure 8, the weighted received sound levels are illustrated for the flight altitudes 70-10 m above the microphone, across a frequency span from 20 Hz up to 16.25 kHz, including the “PCA” (Phocid Carnivore in Air) weighting function (Southall et al., 2007). All DJI models had a peak sound level at 25.2-40 Hz, whereas the Autel Evo II RTK peaked at 50 Hz. The maximum weighted received sound level above 50 Hz varied from 63.6-61.4 dB re 20 µPa from 10-70 m altitude for DJI Phantom 4 Pro, 54.2-53.2 dB for Autel EVO II RTK, 55.4-58.5 dB for Mavic 2, and 55.5-55.9 dB for Mavic 3 (Figure 9). All drone models made noise well above the seals’ hearing threshold. Low frequency hearing below 50 Hz are missing for the Phoca audiogram, but drone noise is relatively close to the threshold and noise <100 Hz is therefore not expected to contribute much to the overall noise exposure.
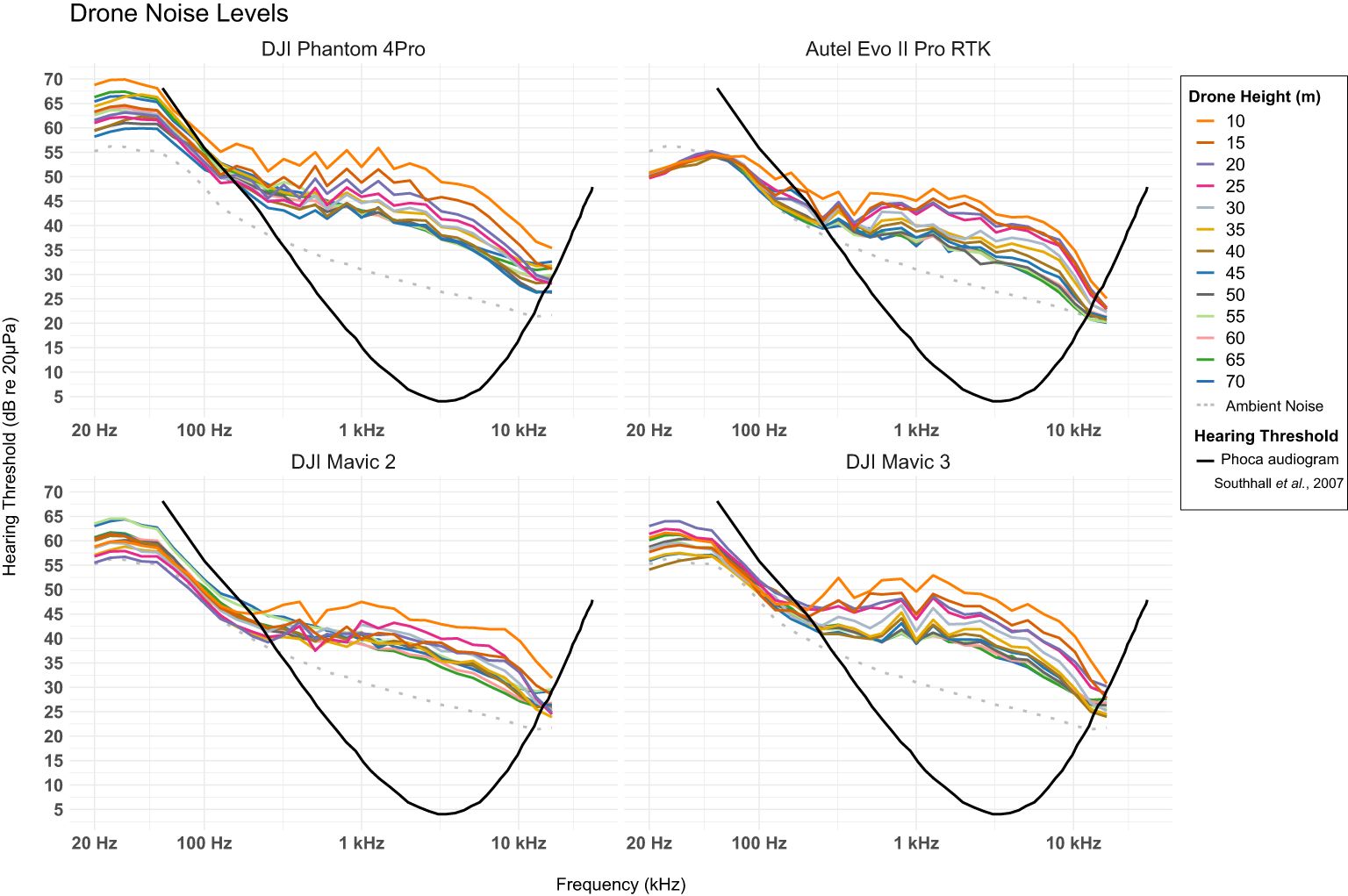
Figure 8. The weighted received sound level (dB) re 20µPa for the hearing threshold for phocids “PCA” (Phocid Carnivore in Air (Southall et al., 2007),) from 20 Hz to 16.25 kHz for four different drone models: DJI Phantom 4 Pro (top left), Autel Evo II RTK (top right), DJI Mavic 2 (bottom left), and DJI Mavic 3 (bottom right), at altitude from 10-70 m.
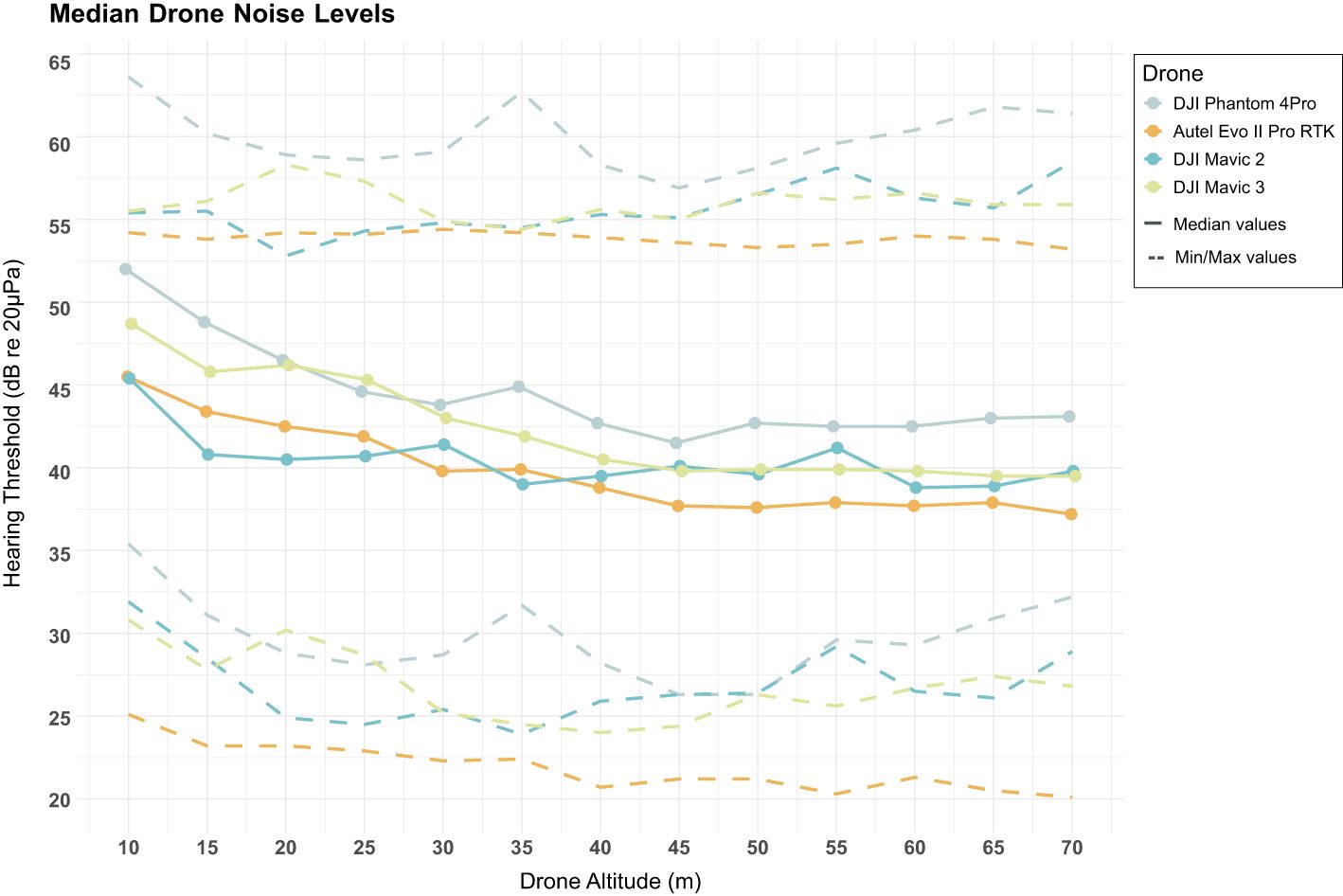
Figure 9. The median weighted received sound level span (dB) re 20µPa (solid lines), with min. and max values (dashed lines) for the hearing threshold for phocids (Southall et al., 2007) from 63.5 Hz to 16.25 kHz for four different drone models: DJI Phantom 4 Pro (grey), Autel Evo II RTK (orange), DJI Mavic 2 (blue), and DJI Mavic 3 (green) at altitude from 10-70 m.
Discussion
This study emphasizes the importance of carefully considering various factors when selecting both the drone model and flight protocol for wildlife monitoring. We observed significant differences in seal behaviour between the two drone models and identified specific flight altitudes where behaviours were minimally affected. These findings provide valuable guidance for establishing optimal flight parameters to minimise disturbance during drone-based wildlife monitoring.
Our observations explore distinct behavioural patterns exhibited by grey seals and harbour seals in response to different flight altitudes and approaches of two commercial drones, DJI Phantom 4 Pro and Autel EVO II RTK. Notably, both species showed reductions in resting behaviour and increased vigilance and flush response with decreasing flight altitude, particularly evident during the LawnMower approach, that caused significantly stronger disturbance rates compared to the Direct approach. This behavioural change is consistent with previous studies on pinnipeds’ reactions to aerial surveys with various drone models (Pomeroy et al., 2015; Sweeney et al., 2015; McIntosh et al., 2018; Laborie et al., 2021; Palomino-González et al., 2021; Tadeo et al., 2023). While our study quantified the percentage of disturbance using post-processed videos for behavioural analysis, rather than relying solely on on-site evaluations during flights, it is important to acknowledge that the superiority of this approach may vary, depending on factors such as location, species, and research objectives. Further comparative studies between on-site and post-processing behavioural evaluations would be valuable for a comprehensive understanding of the impact of drone-based wildlife monitoring.
To ensure acceptable levels of behavioural alteration during drone monitoring of seals, we conclude that minimum flight altitudes should be set at 45 m for both seal species for the Autel EVO II RTK drone and for the DJI Phantom 4 Pro it should be 55 m over grey seals and 50 m over harbour seals. These altitudes correspond to a disturbance level of 0.2-0.3. The maximum weighted received noise levels at these altitudes were: Autel Evo II RTK 53.6 dB re 20μPa at 45 m and Phantom 4 Pro 59.6 dB re 20μPa at 55 m, 58.1 dB re 20μPa at 50 m. The discrepancy in disturbance levels observed between the Autel Evo II RTK and the Phantom 4 Pro drones, with the latter exhibiting higher noise levels, suggests that the difference in drone noise may be the cause of the stronger disturbance reaction to the Phantom 4 Pro drone. However, the effect of noise cannot be separated from the larger size and thereby potentially greater visibility of the Phantom 4 Pro drone to seals.
The flight altitudes summarised in Table 1, reported no behavioural impact at altitudes 30-50 m for grey seals (Pomeroy et al., 2015), harbour seals (Pomeroy et al., 2015), Antarctic fur seals (Krause et al., 2021), leopard seals (Krause et al., 2021), Steller’s sealions (Sweeney et al., 2015) and Australian fur seals (McIntosh et al., 2018). Similar drone models were used for these studies, the DJI Phantom 4 Pro (McIntosh et al., 2018) and a APH-22 (Sweeney et al., 2015; Krause et al., 2021), with a received sound level of 31.3–57.8 dB re 20 μPa at 0–90 m distance (Goebel et al., 2015). For grey and harbour seals in Pomeroy et al. (2015), four different drones were used with unknown sound levels, DJI F450 (1.7 kg), Cinestar (2.65 kg), Vulcan 8 (2.65 kg), and Skyjib 8 (weight unknown).
Tadeo et al. (2023) found a significant reduction in resting behaviour and an increase in vigilance among harbour seals, with the most pronounced effects observed when drone altitude decreased from 20 m to 10 m with a DJI Phantom 4Pro. However, significant effects on both behaviours were noted across all drone altitudes from 100 m and below. Before flight, Tadeo et al. (2023) reported an average resting behaviour of ~75% and vigilance of ~20%, but found that these changed to ~25% resting and 55% vigilance at altitudes between 30-100 m. In contrast, our study observed resting and vigilant behaviours ranging from 66-77% and 2-5%, respectively, at altitudes between 50-70 m. In our study, the DJI Phantom 4Pro could reach 20 m before the resting behaviour was reduced to 24-27%, and vigilance increasing to 50-55% at altitudes between 15 m and 20 m.
This variation in findings could be attributed to the methodological differences, as the before and after flight data Tadeo et al. (2023) collected were from scan samplings every 5 minutes from land at distances ranging from 200 to 450 m, which did not provide comparable data to the much higher resolution data from the continuous recordings from the drone. The large behavioural differences could also be due to the mean group size, which was only 7 individuals in Tadeo et al. (2023), whereas the mean group size in the present study was 60 individuals at Galgerev and 170 at Jørgens Lo. This could potentially affect the behaviour of the animals, with greater vigilance in smaller groups (Campos, 2017).
Furthermore, environmental factors and site-specific characteristics may have contributed to the observed differences. For instance, seals at Jørgens Lo exhibited a 45% lower susceptibility to disturbance compared to those at Galgerev. This variation could be attributed to the differing species compositions of seals at each site as there were rarely grey seals hauled out at Jørgens Lo, but almost always grey seals at Galgerev, as well as variations in human presence due to seal watching operators. Habituation or increased sensitization to anthropogenic activities may influence the behavioural responses of seals in these locations.
We also found a difference in the reported noise level for the Phantom 4Pro, where Tadeo et al. (2023) reported an average broadband Sound Pressure Level (SPL) of 47.5-50.0 dB re 20μPa at an altitude of 10 m, we measured the weighted received sound level for phocids according to Southall et al. (2007) which was 63.6 dB re 20μPa at an altitude of 10 m.
The statistical analyses supported the multifaceted nature of disturbance dynamics in our study environment, highlighting the influence of various ecological and anthropogenic factors. Increasing wind speed reduced the disturbance level by approximately 9.4%. This could be due to an increase in background noise from the wind, masking some of the noise from the drone. During low tide there was 31.4% decrease in odds of disturbance which might be due to the increased cost of fleeing, as they need to move further to get into their aquatic buffer zone. During days with full moon the odds of disturbance went up by 91.7%. Cronin et al. (2009) previously documented a consistent predilection among seals: they tend to venture out to sea just after the full moon each month. This behaviour suggests that lunar phases impact their decision-making regarding foraging, rest, and movement. This could be related to fish abundancy near the surface surging, as moonlight draws zooplankton (a primary prey for fish) closer to the surface (Croxall et al., 1985; Thompson et al., 1989). It could also be the large difference in tidal currents, as tagged seals exhibited a predilection for heading to sea on the first rising tide following a full moon (Cronin et al., 2009).
Notably, our analysis of noise levels generated by the drones highlights implications for seal auditory sensitivity. All drone models emitted noise levels at frequencies above the seals’ hearing threshold, suggesting that drones may have acoustic impacts on pinnipeds, particularly at close proximity. We observed an average difference of 7.6 dB re 20µPa in maximum weighted noise levels between the DJI Phantom 4 Pro and Autel EVO II RTK drone at the same flying altitude, underlining the necessity for meticulous measurements and descriptions in both permit applications and publications when choosing a drone. Compared to Laborie et al. (2021), we measured the DJI Mavic 2 to have a weighted received sound level of 54.3 dB re 20μPa at 25 m, and they measured a phocid audiogram weighted received level of 57 dB re 20μPa at 25 m.
In this study, we employed a method where flight altitudes were systematically reduced within a single flight session, resulting in a cumulative exposure rather than entirely independent exposures at each altitude. While this method introduced a potential confounding factor with total flight time being associated with flight altitude, it was preferred for several critical reasons.
Firstly, previous studies have indicated that harbour seals are less disturbed by indirect approaches from the sea (Allen et al., 1984; Suryan and Harvey., 1999; Johnson and Gutiérrez, 2007) compared to direct approaches (Henry and Hammill, 2001). By starting at a higher altitude and gradually descending, we aimed to minimise the initial disturbance and allow seals to acclimate to the presence of the drone. However, we acknowledge that this method confounds the altitude variable with flight time, as the seals were exposed to progressively lower altitudes over the course of a single flight. This means that any significant results observed in our ordinal logistic regression model could be attributed to either altitude or the duration of exposure, or potentially both. If we had conducted independent flights with a single fly-over at randomised test altitudes between 10 and 70 m, we could have isolated the effects of altitude more definitively. However, it is important to consider the potential trade-offs of the independent flight approach. Independent flights at randomised altitudes might elicit stronger responses at higher altitudes because seals could react more strongly to the initial presence of the drone. Our cumulative exposure approach was intended to mitigate this immediate response, enabling us to explore a range of altitudes in a more controlled manner and observe how seals’ responses evolved over time.
Furthermore, this method reduced the number of flight exposures over each haul-out site. By minimising the number of flights, we aimed to decrease the overall disturbance to the seals, ensuring that our study did not unduly impact their natural behaviour and habitat. This consideration is crucial in wildlife research, where the ethical implications of repeated disturbances must be carefully managed.
In conclusion, while our chosen method introduces certain limitations, it also offers valuable insights into the seals’ responses to varying altitudes over time. Future studies could benefit from combining both approaches: conducting initial independent flights to establish baseline altitude-specific responses and then employing cumulative exposure methods to understand the effects of prolonged exposure and habituation. This combined approach would provide a more comprehensive understanding of how drones impact wildlife and inform guidelines for minimising disturbance in conservation efforts.
While previous research has documented diverse behavioural responses to drones among marine mammals (Fettermann et al., 2019; Duporge et al., 2021), our focus on hauled-out pinnipeds provides valuable insights into species-specific reactions in relation to altitude, noise and environmental parameters. Understanding these nuances is critical for determining optimal flight parameters balancing minimised disturbance while maximizing resolution of data quality. However, it is imperative to acknowledge that intraspecific differences in behavioural responses may arise due to various environmental factors and anthropogenic disturbances. Moreover, the potential disturbance from drones extends beyond seals, with implications for other marine fauna such as birds (see supplements). Recognising these complexities, we advocate for a holistic approach to wildlife monitoring that considers the broader ecological context and aims to mitigate adverse impacts on marine ecosystems.
Data availability statement
The raw data supporting the conclusions of this article will be made available by the authors, without undue reservation.
Ethics statement
Ethical approval was not required for the study involving animals in accordance with the local legislation and institutional requirements because it was assumed that the study method would not inflict pain, suffering, anxiety or permanent damage to the animals, equivalent to or stronger than an injection with a needle.
Author contributions
ES: Conceptualization, Data curation, Formal analysis, Funding acquisition, Investigation, Methodology, Visualization, Writing – original draft, Writing – review & editing. JK: Data curation, Software, Writing – review & editing. AG: Conceptualization, Funding acquisition, Project administration, Supervision, Writing – review & editing. JT: Conceptualization, Funding acquisition, Methodology, Project administration, Supervision, Writing – review & editing.
Funding
The author(s) declare financial support was received for the research, authorship, and/or publication of this article. The Danish Environment Protection Agency, The Danish Nature Agency, Wadden Sea National Park, Vadehavscentret, and The Fisheries and Maritime Museum, Esbjerg have provided financial support for this study.
Acknowledgments
We would like to thank Jeppe Dalgaard Balle for support, and acknowledge The Danish Environment Protection Agency, The Danish Nature Agency, Wadden Sea National Park, Vadehavscentret, and The Fisheries and Maritime Museum, Esbjerg for financial support.
Conflict of interest
The authors declare that the research was conducted in the absence of any commercial or financial relationships that could be construed as a potential conflict of interest.
Publisher’s note
All claims expressed in this article are solely those of the authors and do not necessarily represent those of their affiliated organizations, or those of the publisher, the editors and the reviewers. Any product that may be evaluated in this article, or claim that may be made by its manufacturer, is not guaranteed or endorsed by the publisher.
Supplementary material
The Supplementary Material for this article can be found online at: https://www.frontiersin.org/articles/10.3389/fmars.2024.1411292/full#supplementary-material
References
Adame K., Pardo M. A., Salvadeo C., Beier E., Elorriaga-Verplancken F. R. (2017). Detectability and categorization of California sea lions using an unmanned aerial vehicle. Mar. Mammal Sci. 33, 913–925. doi: 10.1111/mms.12403
Allen S. G., Ainley D. G., Page G. W., Ribic C. A. (1984). The effect of disturbance on harbor seal haul out patterns at Bolinas Lagoon, California. Fishery Bull. 82, 493–500.
Álvarez-González M., Suarez-Bregua P., Pierce G. J., Saavedra C. (2023). Unmanned aerial vehicles (UAVs) in marine mammal research: A review of current applications and challenges. Drones 7, 667. doi: 10.3390/drones7110667
Campos F. A. (2017). “Vigilance, sentinels, and alarms,” in Encyclopedia of evolutionary psychological science. Eds. Shackelford T. K., Weekes-Shackelford V. A. (Springer International Publishing, Cham), 1–3.
Castro J., Borges F. O., Cid A., Laborde M. I., Rosa R., Pearson H. C. (2021). Assessing the behavioural responses of small cetaceans to unmanned aerial vehicles. Remote Sens. 13, 156. doi: 10.3390/rs13010156
Christiansen F., Nielsen M. L. K., Charlton C., Bejder L., Madsen P. T. (2020). Southern right whales show no behavioral response to low noise levels from a nearby unmanned aerial vehicle. Mar. Mammal Sci. 36, 953–963. doi: 10.1111/mms.12699
Cronin M. A., Zuur A. F., Rogan E., McConnell B. J. (2009). Using mobile phone telemetry to investigate the haul-out behaviour of harbour seals Phoca vitulina vitulina. Endangered Species Res. 10, 255–267. doi: 10.3354/esr00170
Croxall J. P., Everson I., Kooyman G. L., Ricketts C., Davis R. W. (1985). Fur seal diving behaviour in relation to vertical distribution of krill. J. Anim. Ecology. 54, 1–8. doi: 10.2307/4616
Domínguez-Sánchez C. A., Acevedo-Whitehouse K. A., Gendron D. (2018). Effect of drone-based blow sampling on blue whale (Balaenoptera musculus) behavior. Mar. Mammal Science. 34, 841–850. doi: 10.1111/mms.12482
Duporge I., Spiegel M. P., Thomson E. R., Chapman T., Lamberth C., Pond C., et al. (2021). Determination of optimal flight altitude to minimise acoustic drone disturbance to wildlife using species audiograms. Met. Ecol. Evol. 12, 2196–2207. doi: 10.1111/2041-210X.13691
Erbe C., Hawkins, ANPaAD (2012). “The effects of underwater noise on marine mammals. The effects of noise on aquatic life,” in Advances in experimental medicine and biology (Springer Verlag, New York), 17–22.
Erbe C., Parsons M., Duncan A., Osterrieder S. K., Allen K. (2017). Aerial and underwater sound of unmanned aerial vehicles (UAV). J. Unmanned Vehicle Systems. 5, 92–101. doi: 10.1139/juvs-2016-0018
Fettermann T., Fiori L., Bader M., Doshi A., Breen D., Stockin K. A., et al. (2019). Behaviour reactions of bottlenose dolphins (Tursiops truncatus) to multirotor Unmanned Aerial Vehicles (UAVs). Sci. Rep. 9, 8558. doi: 10.1038/s41598-019-44976-9
Fiori L. M., Bader M. K. F., Orams M. B., Bollard B. (2020). Insights into the use of an unmanned aerial vehicle (UAV) to investigate the behavior of humpback whales (Megaptera novaeangliae) in Vava’u, Kingdom of Tonga. Mar. Mammal Sci. 36, 209–223. doi: 10.1111/mms.12637
Giles A. B., Butcher P. A., Colefax A. P., Pagendam D. E., Mayjor M., Kelaher B. P. (2021). Responses of bottlenose dolphins (Tursiops spp.) to small drones. Aquatic Conservation. Mar. Freshw. Ecosyst. 31, 677–684. doi: 10.1002/aqc.3440
Goebel M. E., Perryman W. L., Hinke J. T., Krause D. J., Hann N. A., Gardner S., et al. (2015). A small unmanned aerial system for estimating abundance and size of Antarctic predators. Polar Biol. 38, 619–630. doi: 10.1007/s00300-014-1625-4
Gonzalez L. F., Montes G. A., Puig E., Johnson S., Mengersen K., Gaston K. J. (2016). Unmanned aerial vehicles (UAVs) and artificial intelligence revolutionizing wildlife monitoring and conservation. Sensors 16, 97. doi: 10.3390/s16010097
Henry E., Hammill M. O. (2001). Impact of small boats on the haul-out activity of harbour seals (Phoca vitulina) in Métis Baym Saint Lawrence Estuary, Québec, Canada. Aquat. Mammals 27, 140–148.
Johnson A., Gutiérrez A. A. (2007). Regulation compliance by vessels and disturbance of harbour seals (Phoca vitulina). Can. J. Zoology 85, 290–294. doi: 10.1139/Z06-213
Krause D. J., Hinke J. T., Goebel M. E., Perryman W. L. (2021). Drones minimize antarctic predator responses relative to ground survey methods: an appeal for context in policy advice. Front. Mar. Sci. 8, 648772. doi: 10.3389/fmars.2021.648772
Krause D. J., Hinke J. T., Perryman W. L., Goebel M. E., LeRoi D. J. (2017). An accurate and adaptable photogrammetric approach for estimating the mass and body condition of pinnipeds using an unmanned aerial system. PloS One 12, e0187465. doi: 10.1371/journal.pone.0187465
Laborie J., Christiansen F., Beedholm K., Madsen P. T. (2021). Behavioural impact assessment of unmanned aerial vehicles on Weddell seals (Leptonychotes weddellii). J. Exp. Mar. Biol. Ecol. 536 (151509). doi: 10.1016/j.jembe.2020.151509
Landeo-Yauri S. S., Castelblanco-Martínez D. N., Hénaut Y., Arreola M. R., Ramos E. A. (2022). Behavioural and physiological responses of captive Antillean manatees to small aerial drones. Wildlife Res. 49, 24–33. doi: 10.1071/WR20159
McIntosh R. R., Holmberg R., Dann P. (2018). Looking without landing-using remote piloted aircraft to monitor fur seal populations without disturbance. Front. Mar. Sci. 5, 1–13. doi: 10.3389/fmars.2018.00202
Mulero-Pázmány M., Jenni-Eiermann S., Strebel N., Sattler T., Negro J. J., Tablado Z. (2017). Unmanned aircraft systems as a new source of disturbance for wildlife: A systematic review. PloS One 12, e0178448. doi: 10.1371/journal.pone.0178448
Mustafa O., Barbosa A., Krause D. J., Peter H.-U., Vieira G. (2018). State of knowledge: antarctic wildlife response to unmanned aerial systems. Polar Biol. 41, 2387–2398. doi: 10.1007/s00300-018-2363-9
Palomino-González A., Kovacs K. M., Lydersen C., Ims R. A., Lowther A. D. (2021). Drones and marine mammals in Svalbard, Norway. Mar. Mammal Science. 37, 1212–1229. doi: 10.1111/mms.12802
Perryman W., Goebel M. E., Ash N., LeRoi D., Gardner S. (2010). Small unmanned aerial systems for estimating abundance of krill-dependent predators: A feasibility study with preliminary results. AMLR. 2011, 64–72.
Pomeroy P., O’Connor L., Davies P. (2015). Assessing use of and reaction to unmanned aerial systems in gray and harbor seals during breeding and molt in the UK. J. Unmanned Vehicle Systems. 3, 102–113. doi: 10.1139/juvs-2015-0013
Ramos E. A., Maloney B., Magnasco M. O., Reiss D. (2018). Bottlenose dolphins and Antillean manatees respond to small multi-rotor unmanned aerial systems. Front. Mar. Science. 5, 316. doi: 10.3389/fmars.2018.00316
Seganfreddo S., Teilmann J., van Beest F. M., Galatius A. (2023). Phenology of harbor seal pupping and the influence of weather on pup counts, investigated by UAV. Mar. Mammal Science. 39, 906–917. doi: 10.1111/mms.13020
Slabbekoorn H., Bouton N., van Opzeeland I., Coers A., ten Cate C., Popper A. N. (2010). A noisy spring: the impact of globally rising underwater sound levels on fish. Trends Ecol. Evol. 25, 419–427. doi: 10.1016/j.tree.2010.04.005
Smith C. E., Sykora-Bodie S. T., Bloodworth B., Pack S. M., Spradlin T. R., LeBoeuf N. R. (2016). Assessment of known impacts of unmanned aerial systems (UAS) on marine mammals: data gaps and recommendations for researchers in the United States. J. Unmanned Vehicle Syst. 1, 31–44. doi: 10.1139/juvs-2015-0017
Southall B. L., Bowles A. E., Ellison W. T., Finneran J. J., Gentry R. L., Greene C. R. Jr., et al. (2007). Structure of the noise exposure criteria. Aquat. mammals. 33, 427. doi: 10.1578/AM.33.4.2007.427
Speakman C. N., Johnstone C. P., Robb K. (2019). Increased alertness behavior in Australian fur seals (Arctocephalus pusillus doriferus) at a high vessel traffic haul-out site. Mar. Mammal Science. 36, 486–499. doi: 10.1111/mms.12654
Stankowich T., Blumstein D. T. (2005). Fear in animals: a meta-analysis and review of risk assessment. Proc. R. Soc. 272, 2627–2634. doi: 10.1098/rspb.2005.3251
Suryan R., Harvey. J. (1999). Variability in reactions of Pacific harbor seals, Phoca vitulina richardsi, to disturbance. Fishery Bull. 97, 332–339.
Sweeney K. L., Helker V. T., Perryman W. L., LeRoi D. J., Fritz L. W., Gelatt T. S., et al. (2015). Flying beneath the clouds at the edge of the world: using a hexacopter to supplement abundance surveys of Steller sea lions (Eumetopias jubatus) in Alaska. J. Unmanned Vehicle Syst. 4, 70–81. doi: 10.1139/juvs-2015-0010
Tablado Z., Jenni L. (2015). Determinants of uncertainty in wildlife responses to human disturbance. Biol. Rev. 92, 216–233. doi: 10.1111/brv.12224
Tadeo M. P., Gammell M., O’Callaghan S. A., O’Connor I., O’Brien J. (2023). Disturbances due to unmanned aerial vehicles (UAVs) on harbor seal (Phoca vitulina) colonies: Recommendations on best practices. Mar. Mammal Sci. 39, 757–779. doi: 10.1111/mms.13002
Team R (2022). RStudio: integrated development environment for R (Version 2022.07.2) (Boston, MA: RStudio, PBC).
Thompson P. M., Fedak M., McConnell B., Nicholas K. S. (1989). Seasonal and sex-related variation in the activity patterns of common seals (Phoca vitulina). J. Appl. Ecol. 26, 521–535. doi: 10.2307/2404078
Torres L. G., Nieukirk S. L., Lemos L., Chandler T. E. (2018). Drone up! Quantifying whale behavior from a new perspective improves observational capacity. Front. Mar. Sci. 5, 319. doi: 10.3389/fmars.2018.00319
Keywords: Phoca vitulina, Halichoerus grypus, UAV, Wadden Sea, flight altitude, disturbance, behavioural response, wildlife monitoring
Citation: Stepien EN, Khan J, Galatius A and Teilmann J (2024) How low can you go? Exploring impact of drones on haul out behaviour of harbour - and grey seals. Front. Mar. Sci. 11:1411292. doi: 10.3389/fmars.2024.1411292
Received: 02 April 2024; Accepted: 13 June 2024;
Published: 30 August 2024.
Edited by:
Todd Atwood, U.S. Geological Survey, Alaska, United StatesReviewed by:
David Ainley, H.T. Harvey & Associates, United StatesRebecca Ruth McIntosh, Phillip Island Nature Parks, Australia
Copyright © 2024 Stepien, Khan, Galatius and Teilmann. This is an open-access article distributed under the terms of the Creative Commons Attribution License (CC BY). The use, distribution or reproduction in other forums is permitted, provided the original author(s) and the copyright owner(s) are credited and that the original publication in this journal is cited, in accordance with accepted academic practice. No use, distribution or reproduction is permitted which does not comply with these terms.
*Correspondence: Emilie Nicoline Stepien, emilie.stepien@gmail.com
†ORCID: Anders Galatius, orcid.org/0000-0003-1237-2066