- 1National Research Council (CNR), Institute for Biological Resources and Marine Biotechnologies (IRBIM), Ancona, Italy
- 2Faculty of Biology, Fisheries and Economics, The Arctic University of Norway (UIT), Tromsø, Norway
- 3SINTEF Ocean, Fishing Gear Technology, Trondheim, Norway
- 4Technical University of Denmark, National Institute of Aquatic Resources, Hirtshals, Denmark
Pots are traditional fishing gear type largely employed in Mediterranean Small Scale Fisheries (SSFs). Their often higher species selectivity and catch quality compared to other gear types such as passive set nets or trawls are appealing characteristics for reducing the impact on benthic communities and diversifying fishing effort in the region. Despite this, in some SSFs, pots are still rarely used as prevalent gear due to low landings and profits. The present study investigated if an experimental pot design of two different colours could improve the catch efficiency for mantis shrimp (Squilla mantis) in the Adriatic coastal SSF, compared to the traditionally used design. The experimental design in white colour increased the catch efficiency for S. mantis compared to the same design in black colour and the traditional pots, while the catch efficiency for black goby (Gobius niger, another commercial species) was higher in the experimental design regardless of the colour. Furthermore, the species composition in catches was not significantly affected with the use of the experimental design, neither in target nor in bycatch species, therefore not causing negative effect on the species community. Finally, the experimental pot design also led to some improvements in gear durability, ensuring a higher resistance to wear, sea currents and marine fouling. The promising results obtained with this new design could broaden the interest towards increased use of pots as alternative and low impact gear in other Mediterranean areas.
1 Introduction
Mediterranean coastal Small Scale Fisheries (SSFs hereafter) employ a wide range of fishing gear types and fishing strategies to target several species (Bastardie et al., 2017; Tiralongo et al., 2018; Ramieri et al., 2019; Grati et al., 2022). Pots, defined as a sub-type of trap gear (FPO – 08.2) in the FAO catalogue of fishing gears (ISSCFG, 2016), are amongst the most traditional gear employed (Farrugio, 2013). They are passive gears that attract the target species to enter the gear through one or more entrances and retain them by preventing their subsequent escape (Miller, 1990; Pol et al., 2010). Fish, crustaceans and molluscs are attracted to pots by means of bait or pasture, or simply by the shelter or space pots could provide (e.g. cephalopods, Sobrino et al., 2011).
Each pot design is usually species-specific, and each single technical parameter of a pot can potentially affect its entry, retention and selection efficiency (Suuronen et al., 2012; Amengueal-Ramis et al., 2016; Petetta et al., 2021b). Thus, pot design parameters such as volume, shape, netting mesh size and colour, and entrance(s) (i.e. number, dimensions, position) are factors that could affect the catch efficiency (Petetta et al., 2021b).
Potentially high species selectivity and catch quality obtained in pot fisheries could have a potential to reduce the impact on the benthic community and diversify the fishing effort in the region (Petetta et al., 2021b). Specifically, pots could contribute at reducing the high discard rates of unwanted species currently produced by other fisheries using other fishing gear types (e.g. passive set nets, Tiralongo et al., 2021; bottom trawls Morello et al., 2009; Petetta et al., 2021b and dredges, Morello et al., 2005; Vasapollo et al., 2020; Petetta et al., 2021a), and obtaining higher selling prices due to improved catch quality (Brčić et al., 2017). However, despite their growing popularity among fishers, pots are still rarely used as prevalent gear in SSFs, due to low catch yields and associated income. In 2021, the European Mediterranean pot fisheries accounted for approximately 3200 tonnes and a value of 24 million EUR, representing 1% of the total fisheries landings and 1.6% of their revenues, respectively (Scientific Technical and Economic Committee for Fisheries (STECF), 2023). In the Western Adriatic Sea (FAO Geographical Sub-Area 17), SSFs currently employ different pot designs all-year round to target cuttlefish (Sepia officinalis; March-July), mantis shrimp (Squilla mantis; July-November) and changeable nassa (Tritia mutabilis; November-March) (Grati et al., 2018).
S. mantis is the only stomatopod crustacean commercially exploited in the Mediterranean, and it is regularly found in fish markets of Spain, Italy, Egypt, and Morocco (Abelló and Martín, 1993). This species ranks first among the crustaceans landed in the Italian Adriatic ports (around 3300 tonnes in 2021; FAO-GFCM, 2023), being an important component of multispecies bottom and beam trawl fisheries and being targeted by dedicated gillnet and pot fisheries. The latter account for around 8% of the total passive gears S. mantis landings (SAC, and Scientific Advisory Committee on Fisheries, 2022). However, there is no reliable data on the size of the fleet engaged in this fishery that is also not specifically regulated (Scientific Technical and Economic Committee for Fisheries (STECF), 2023). The design of the commercially used pots in the S. mantis fishery has evolved over time following empirical trials and attempts by fishers to optimize the catch efficiency for mantis shrimp, the space required on board, the costs for construction and the durability of the gear. Nowadays, the most commonly employed pot design along the Italian Adriatic coast has a square shape with a volume of around 12 l. This pot design is sold by local fishing enterprises, but several fishers produce them by hand, recycling materials and components intended for other uses. The pots targeting S. mantis usually catch also another species with commercial value, i.e. black goby (Gobius niger), with relevant landings (87 tonnes in 2021 for the Italian fishing fleet in GSA17, FAO-GFCM, 2023), especially in the northern part of the area (Bon et al., 2006).
S. mantis pot fisheries have been conducted in the Northern part of the area since the second half of the last century. The use of these pots has been limited in the past due to a strong competition with other passive gear types and active gears targeting the same species (Froglia, 1996). However, in the last years, pots are being used in a wider area, along the entire Western Adriatic coasts. Within the SSFs, pots are being increasingly more used compared to passive nets because they are not subjected to dolphin depredation which is a considerable challenge in the area (Lauriano et al., 2009; Li Veli et al., 2023).
In this study, we developed and tested a new pot design intended to improve catch efficiency in S. mantis pot fishery. Further, we estimated and compared the impact of the traditional and experimental gear regarding the effect on catch species composition. Specifically, when changing gear or design it is important to evaluate the impact from a species community perspective to assess if the new gear design could have potential negative effects on catch composition considering not only those species having a commercial interest or requiring close protection or management measures, but all the species caught by the specific gear (Petetta et al., 2023). Finally, we assessed the wear resistance and robustness of both pot designs for use in S. mantis fishery.
Therefore, this study was designed to address the following questions:
- Can the new pot design increase catch efficiency of S. mantis and G. niger?
- Is the new pot design affecting the catch efficiency for bycatch species?
- What is the species composition in the catches of the fishery and is it affected by changing the pot design?
- Is the new pot design more robust and wear resistant than the currently used?
2 Materials and methods
2.1 Pot designs
The differences in pot technical parameters between the traditional and experimental designs tested and the reason for their modifications are listed in Table 1. The traditional design was the most commonly used design in the fishery, while the experimental design was developed based on inputs from artisanal fishers of SSFs in the fishing area.
The traditional design (hereafter, green pot) had a square shape with a single entrance on one side and the pot frame was made of galvanized metal with dark green plastic coating to provide resistance to oxidation. It had a rectangular mesh size (10*22 mm) and the frame of the entrance was made of a plastic material of the same colour as the pot. Despite its widespread use in the commercial fishery, this design shows some shortcomings regarding both catch efficiency for S. mantis and gear durability.
1. The square shape does not ensure an optimal guidance towards the single entrance for those individuals approaching to the pot from one of the other three sides without entrances. In fact, they may lose contact with the pot sides before finding the entrance, when moving along them, due to the 90 degrees’ sharp corners (Figure 1). Also, the rectangular mesh size might be too large to effectively retain all commercial sizes of S. mantis and G. niger. Therefore, the experimental pot design was created with a circular shape without sharp corners, to ensure optimal guidance towards the single entrance, and with a smaller netting mesh size (10 mm square mesh).
2. As this fishery is carried out in shallow waters, we focused on pot colour, which may affect the attraction to the targeted species and therefore the entrance efficiency. Based on this, the experimental design was made in two versions only differing by colour, specifically in white and black (Figure 1). These two versions (hereafter, white pot and black pot) were chosen to represent the maximum contrast and thereby the potential maximal effect of changing pot colour on catch efficiency.
3. Traditional pots are usually deployed in fishing grounds for the entire fishing season (approximately four months, from late summer to autumn) and retrieved every 1–2 days or more in the event of adverse sea weather conditions. Therefore, they are subjected to various stressors including: (i) wear- represented by the loss or wear of some components and damage to the gear structure, (ii) storms- which can potentially result in pot loss, and (iii) marine fouling, involving reduction of the gear catch efficiency for S. mantis. This results in a short life span and easy breakage. To increase the duration and resistance to wear and marine fouling, we made structural changes in the experimental design concerning pot frame, netting mesh and funnel materials. Pot weight was also increased to investigate the resistance to sea currents (Table 1).
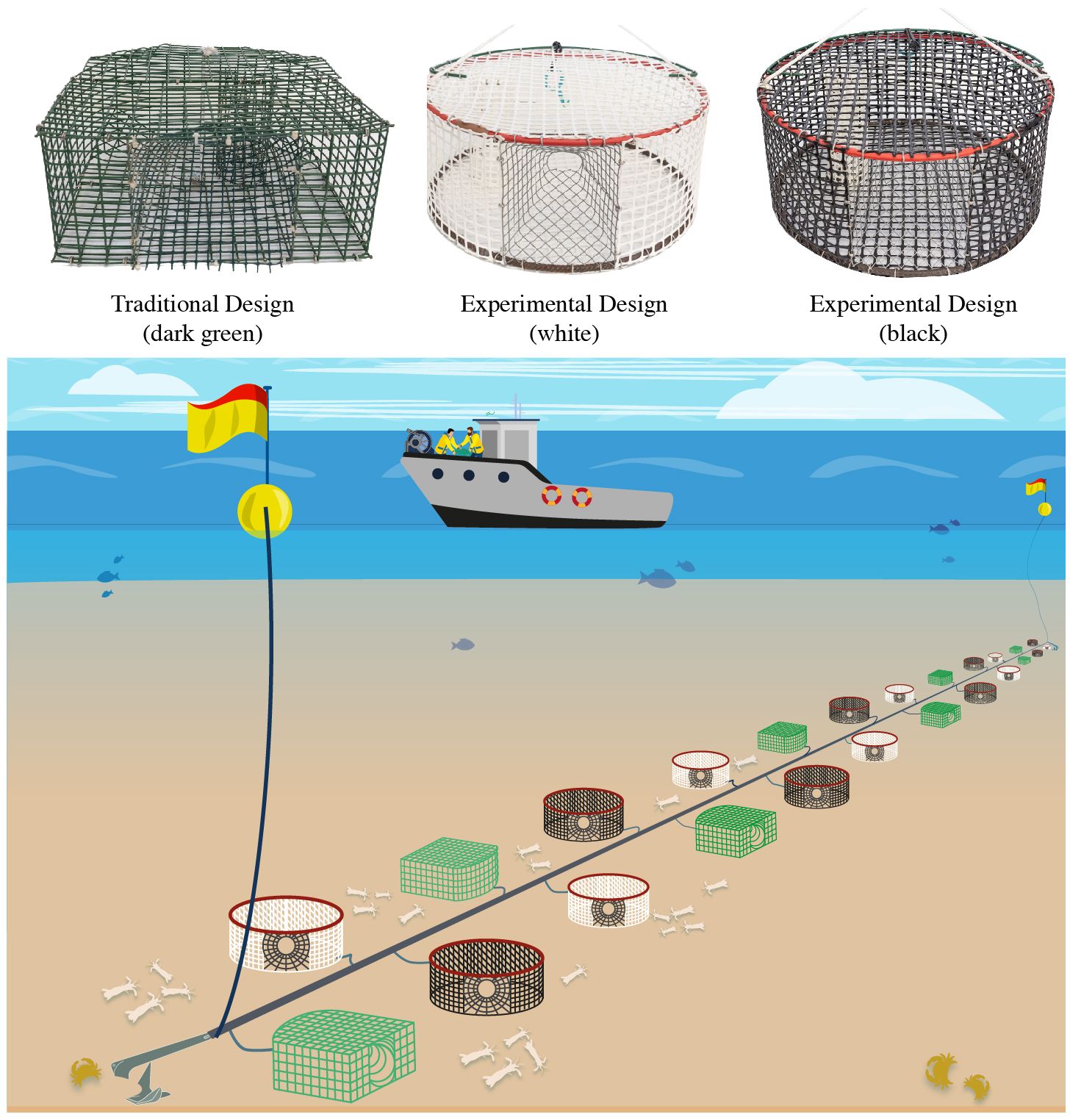
Figure 1 Top: Illustration of the three mantis shrimp pot designs tested in the present study i.e. green (traditional) and white, black (experimental) pots. Down: Experimental design, with the three pot designs alternated on the same string. Pots are usually deployed in strings and attached with branch lines that connect them to a mainline.
The frames of experimental pots were made by using materials from other traditional fishing gears of the SSFs. Specifically, the upper circular plastic frame was made using fyke net targeting S. officinalis and the lower circular iron frame was designed using basket trap for T. mutabilis.
In both traditional and experimental pot designs, an opening was placed on the upper side to allow easy baiting and pot emptying with a quick operation of lifting and closing. In the traditional design, it was regulated by means of an internal elastic rope, while in the experimental design by a hook connected by an elastic band to the upper side of the pot (Figure 1). The shape and dimensions of the entrance was identical in both designs: oval-shaped, positioned in the front side and with a 12 cm long funnel (Table 1). On the opposite side of the entrance, sideways, there was a box serving as a bait container.
2.2 Experimental design and sea trials
A commercial vessel representative of the local fleet (LOA 6.8 m; gross tonnage 1 GT; gasoline-powered engine 100 HP) with a crew of two fishers was used for sea trials. The activities were performed in two consecutive years (2021 and 2022) in late summer/autumn, which corresponds to the main fishing season for S. mantis (Grati et al., 2018).
Fishing operations were carried out in shallow waters (around 15 m of depth) at 1–3 nautical miles off Pedaso coast (Central-western Adriatic Sea, Italy), where fishing grounds are characterized by sandy bottoms and are inhabited by S. mantis. Fresh sardines were used as bait (~ 40 gr per pot). In every trial, 40 pots for each design were deployed (120 in total). They were set in 4 fleets containing 30 pots each by alternating the three pot designs. The distance between pots was 15 m (Figure 1). Each fleet was made of a main propylene line with 1.5 m branch lines where pots were attached through a quick release swivel. This allowed to ensure a correct setup depending on the force and direction of bottom water current.
After each deployment, observers on board sorted the catch, which was kept separate by pot type. The total catch for each pot was then divided into a landed catch (i.e., main target species and other species with commercial value) and discarded catch (species with no commercial value, commercial individuals under the minimum landing size or in poor conditions). The number of individuals of any species caught in each pot was recorded. All individuals were identified to the lowest taxonomic level possible, counted, weighed to the lowest 1 g by a small balance scale with marine compensation. Individuals of G. niger and S. mantis were measured to the nearest 0.5 cm for total length (TL; G. niger) and to the nearest 0.1 mm for carapace length (CL; S. mantis). No subsampling was conducted throughout this study.
In 18 deployments, a small underwater camera (GoPro Hero 4 Black; GoPro® Inc., California) was fixed inside one randomly selected pot, to record the behaviour of S. mantis and other species as they approached the pot, during entry and once inside. The camera was alternately used in green, black and white pots. It was positioned to the opposite side of the entrance, oriented towards the funnel. No torches or lights were used, since i) they could affect behaviour of animals approaching to the pot and ii) the shallow depths allowed to obtain useful footages only with the natural light. The camera battery did not cover the entire duration of the deployments, but only around the first hour of set, which always happened at daylight.
At the end of fishing trials in 2021 and 2022, pots were left at sea for an additional month. In these periods, pots were again baited and hauled several times to assess: i) the pot resistance to a longer soaking period, including bad weather conditions expressed in number of pot lost; ii) the level of damages expressed in number of damaged pots; and iii) the loss of catch efficiency over time in correlation with the degree of marine fouling on the gear (see section 2.2.4).
2.3 Data analysis
To perform the analyses described in sections 2.3.1 and 2.3.2, we first assigned a fixed rank to each single species caught in the sea trials, following the procedure described in (Petetta et al., 2023), by including it into one of the following 3 categories: 1) ‘Target species’, i.e. the main commercial species targeted by S. mantis pots in the study area; 2) ‘Other species of commercial value’, i.e. additional species with a commercial value, that are landed; 3) ‘Other species of no commercial value’, i.e. those species usually discarded by the fishers.
Since the three pot designs were deployed together, attached to the same fleets (paired deployment design), catch data was treated as paired. The data analysis procedures described below were conducted using the software SELNET (Herrmann et al., 2012, 2016, 2017, 2022).
2.3.1 Comparing the experimental and traditional pot catch efficiency
The length measurements collected for the most abundant commercial species caught, i.e. S. mantis and G. niger, were used to investigate the length-dependent effect on the catch efficiency by changing pot design. The analysis was carried out independently for each species following the description below. The comparison of the catch efficiency between pot designs was conducted separately for each of the three combinations (sets): white versus green, black versus green, white versus black. In each of the sets, the first design acted as a test design and the second as a baseline design for the specific comparison. Catch comparisons were first performed separately for the two fishing seasons separately (2021 and 2022) followed by a comparison with aggregating the data over these seasons. We used the method described in Herrmann et al. (2017), which models the length-dependent catch comparison rate (CCl) summed over pot deployments by:
where ntl,j and nbl,j are the numbers of individuals caught in each size class l for the test (t) and baseline (b) pots, respectively, in deployment j. m is the number of paired deployments considered. We estimated the catch comparison rate CC(l,v) of Equation 1 using maximum likelihood estimation by minimizing Equation 2:
where the outer summation is over the length classes l of S. mantis or G. niger, respectively, and the inner summation is over the deployments m. The v parameter describes the catch comparison curve defined by CC(l,v). The experimental CCl was modelled by Equation 3:
where f is a polynomial of order k with coefficients v0 to vk. We considered f of up to an order of 4 with parameters v0, v1, v2, v3, and v4. Leaving out one or more of the parameters v0…v4 led to 31 additional models for the catch comparison function CC(l, v).
The estimations of the catch comparison rate were made, among these models, by using the multi-model inference to obtain a combined model (Burnham and Anderson, 2002; Herrmann et al., 2017). The ability of the combined model to describe the experimental data was evaluated based on the p-value, calculated by the ratio between model deviance and degrees of freedom (DOF; Wileman et al., 1996; Herrmann et al., 2017). With p-value > 0.05, the combined model was assumed to describe the experimental data sufficiently well, while with p-value< 0.05 and deviance/DOF >> 1, the residuals were inspected to determine whether the results were due to structural problems when modelling the experimental data, or to over-dispersion in the data (Wileman et al., 1996).
A catch comparison rate of 0.5 would imply a similar catch efficiency between pot designs, i.e. an individual of length l has the same probability of being retained by either design. However, since CC(l,v) does not provide a direct relative value of the catch efficiency between gears, we further estimated the catch ratio CR(l,v) (Herrmann et al., 2017) as follows:
In Equation 4, a CR(l,v) value of 1.0. would imply a similar catch efficiency between pot designs, while a CR(l,v) value significantly larger than 1.0 would imply that the test pots (depending on the comparison) are catching significantly more individuals with length l compared to baseline pots.
We estimated Efron percentile 95% Confidence Intervals (CIs; Efron, 1982) for CC(l,v) and CR(l,v) using a double bootstrapping method (Herrmann et al., 2017), which accounts for both between-deployments variability and within-deployment variability. However, contrary to the double bootstrapping method in Herrmann et al. (2017), in this study we conducted the outer bootstrapping loop (that accounts for the variability between deployments) in pairs, to take full advantage of the experimental design of deploying the three pot designs simultaneously. By using multi-model inference in each bootstrap iteration, the method also accounted for the uncertainty in model selection. We performed 1000 bootstrap repetitions and calculated the 95% CIs. Significant differences in catch efficiency between designs for specific sizes of each species (S. mantis and G. niger) were identified by checking for length classes in which the 95% CIs for the catch ratio curve did not contain 1.0 (Grimaldo et al., 2019). Specifically, if CR(l,v) for one or more length classes does not include the value 1.0 within the 95% confidence intervals (CIs), it implies that a significant difference in capture efficiency is detected at the 95% confidence level for those size classes.
We applied the exploitation pattern indicator for catch comparison to summarize the relative performance of tested pots on each species caught (Veiga-Malta et al., 2019; Petetta et al., 2023). We estimated the mean percentage of individuals for each species (in both number of individuals nPi and weight wPi) retained by the test compared to the baseline pots (depending on the comparison) as follows (Equations 5 and 6):
where i is the pre-defined species index (species rank) for each species observed in gear catches (i.e., Herrmann et al., 2022). Parameter is the average weight for species i caught in the test pots in deployment j, while is the average weight for species i caught in the baseline pots in deployment j. An indicator value of 100% means that the test pot catches the same number of individuals or weight of the species analysed as the baseline pot, while indicator values of 50% and 150% mean that the test pot caught 50% less and 50% more individuals or weight than the baseline pot, respectively.
2.3.2 Comparing the species composition
We performed a catch composition analysis (Herrmann et al., 2022; Madhu et al., 2023; Petetta et al., 2023) to quantify and compare the species composition in the catches of the three pot designs. This methodology allows to measure how much each species caught dominates in the sample, thus evaluating the impact of a fishing gear from a species community perspective (Warwick et al., 2008). The catch dominance analysis was here performed to evaluate, for each pot design separately and for each species, its relative abundance in both the total catch, the discarded catch and the landed catch. The estimation of the species dominance pattern was averaged over pot deployments.
The estimation of the catch dominance, in both number of individuals (dni) and weight (dwi) was done separately by design and for each fraction of the catch (total, discarded, landed), by Equation 7 (Cerbule et al., 2022; Herrmann et al., 2022; Madhu et al., 2023; Petetta et al., 2023):
where j represents the deployment and i is the species rank previously defined. nij is the count numbers for each species in each pot type, respectively. Parameter ρ is the average weight of species i in deployment j in a given fraction of the catch and is obtained from the total weight and number of individuals. Q stands for the total number of species observed. m is the total number of paired deployments considered in the analysis.
Further, the cumulative dominance curves were estimated, in both number of individuals (DnI) and weight (DwI), to represent the cumulative proportional abundances of the species (Cerbule et al., 2022; Herrmann et al., 2022; Madhu et al., 2023; Petetta et al., 2023):
In Equation 8, I is the species index summed up in the nominator. The fixed species ranking in all catches in the cumulative dominance curves allows the comparison of the steepness of the cumulative dominance curves. Specifically, steeper sections in dominance curves will imply that some species are being more dominant than other species in the pot design, while horizontal parts show that the particular species are not abundant (Cerbule et al., 2022; Herrmann et al., 2022; Petetta et al., 2023).
The Efron 95% CIs were used to provide the uncertainty of the values of dominance patterns obtained following the procedure described in (Herrmann et al., 2022). This procedure enables the estimation of the uncertainty around the dominance values at species level induced by the limited sample sizes at single deployment as well as for the between deployment variation in species dominance.
Furthermore, the difference Δd in species dominance d between pots (green pot, gr; white pot, wh; black pot, bl) pots was estimated, for both number of individuals and weight by (Herrmann et al., 2022; Petetta et al., 2023):
The CIs for Equation 9 were obtained based on separate bootstrap populations for dg, dw and db, as described in (Cerbule et al., 2022; Herrmann et al., 2022). The significance was detected by inspecting the CIs for the difference: if the 0.0 value was within the CIs, no significant difference was detected.
2.3.3 Underwater video observations
The recordings of underwater footages from the cameras used in 18 deployments were used for identifying the species approaching the pot entrance and studying their behaviour both outside and inside the pot. VLC media player was used to observe the footages, and data was collected on i) the timing of entrance by species caught during the first hour of deployment, to study how soon after the deployment each species approach the gear; ii) the behaviour of pot-approaching-animals (in a qualitative manner), to study eventual differences in behaviour between species regarding pot designs.
2.3.4 Assessment of catch efficiency reduction due to marine fouling
To evaluate the Catch Efficiency Reduction (CER) related to fouling, we performed a numerical regression of the number of pots catching S. mantis on fouling (expressed as percentage of fouled pots). Since the trend was clearly not linear (see Supplementary Material, Supplementary Figure S8), we employed a Generalized Linear Model (GLM) to fit the data. The choice of the distribution was based on the evaluation of which distribution better suited the data; in our case, a negative binomial distribution was selected. According to the different fouling locations observed, we considered the entrance fouling value for commercial design, and the netting frame fouling value for the experimental design (white and black pots). To evaluate the statistical difference in the GLM coefficients, which represents CER rate per unit of fishing operation, between the two designs, we utilized a bootstrap technique. From two reference datasets, one for the commercial design and the other for experimental design, 1000 samples were extracted with replacement from each dataset. Subsequently, each set was fitted with the respective GLM. From the 1000 values of difference between the coefficient relative to the commercial design and the experimental one, we derived the Efron 95% CIs for the difference.
Statistical analyses were performed using the R statistical software (R Core Team, 2023). For the GLM with a negative binomial family distribution, we utilized the glm.nb function from the R package MASS (version 7.3–60.0.1).
3 Results
A total of 30 valid fishing operations were performed in the two years (Supplementary Material; Supplementary Table S1). The soak time varied from 1 to 95 hours (average range of 14–48 hours), depending on the fisher’s choice and on the weather conditions.
A total of 24 species were caught over all deployments (Table 2). S. mantis was the only “Target species”, while 8 species were included in the “Other species with commercial value” category and were mostly fish, except for the octopus (Octopus vulgaris) and T. mutabilis. Finally, 15 species were included in the “Species without commercial value” category and were mostly fish (10 species) followed by molluscs and crustaceans (3 and 2 species, respectively).
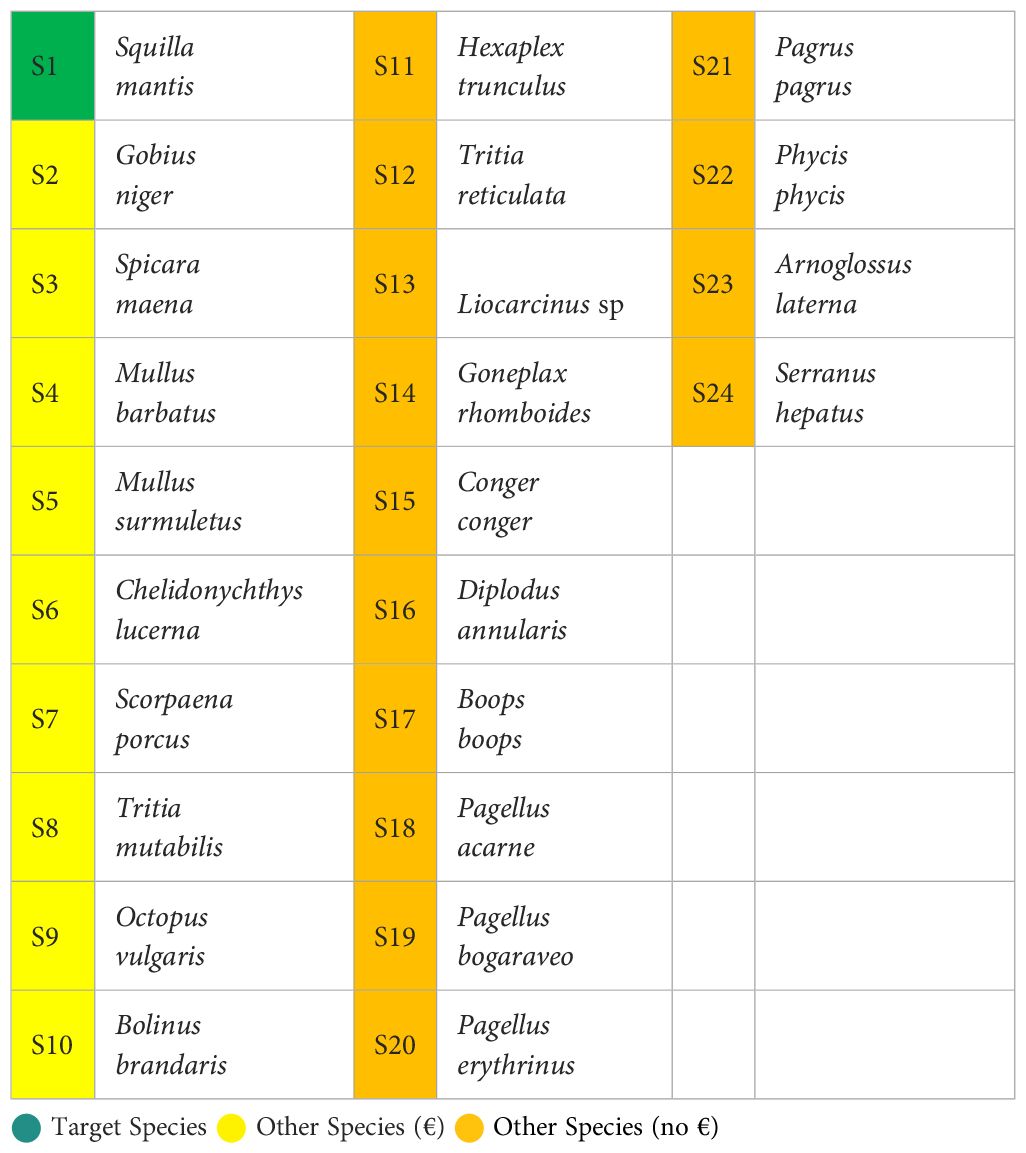
Table 2 Assigned ranking (S) of the species caught during the sea trials and divided by category, i.e. target species, other species with commercial value (€) and other species without commercial value (no €).
3.1 Comparing the experimental and traditional pot catch efficiency
The catch comparison and catch ratio results obtained by comparing two pot designs at a time (white vs green, black vs green, white vs black) for S. mantis and G. niger are shown in Figures 2 and 3, respectively. Additional results are reported in Supplementary Material. Regarding S. mantis, white pots were significantly more efficient than green pots, for almost all the length classes (13 to 32.5 mm; Figure 2, top), and black pots (from 22 to 32.5 mm; Figure 2, bottom). On the contrary, black pots were significantly more efficient than green pots only at catching small specimens (from 13 to 23 mm; Figure 2, middle). Regarding G. niger, both experimental pots had a significantly higher catch efficiency than green pots for wide length ranges, i.e. 9.5 to 14.5 cm (white vs green; Figure 3, top) and 10.5 to 15 cm (black vs green; Figure 3, middle). This is also evident from the length frequency distributions obtained with the different pot designs (shown in the catch ratio graphs; Figure 3, right). Pot colour did not affect the catch efficiency for G. niger, since the catch comparison between black and white pots did not reveal any significant differences (Figure 3, bottom).
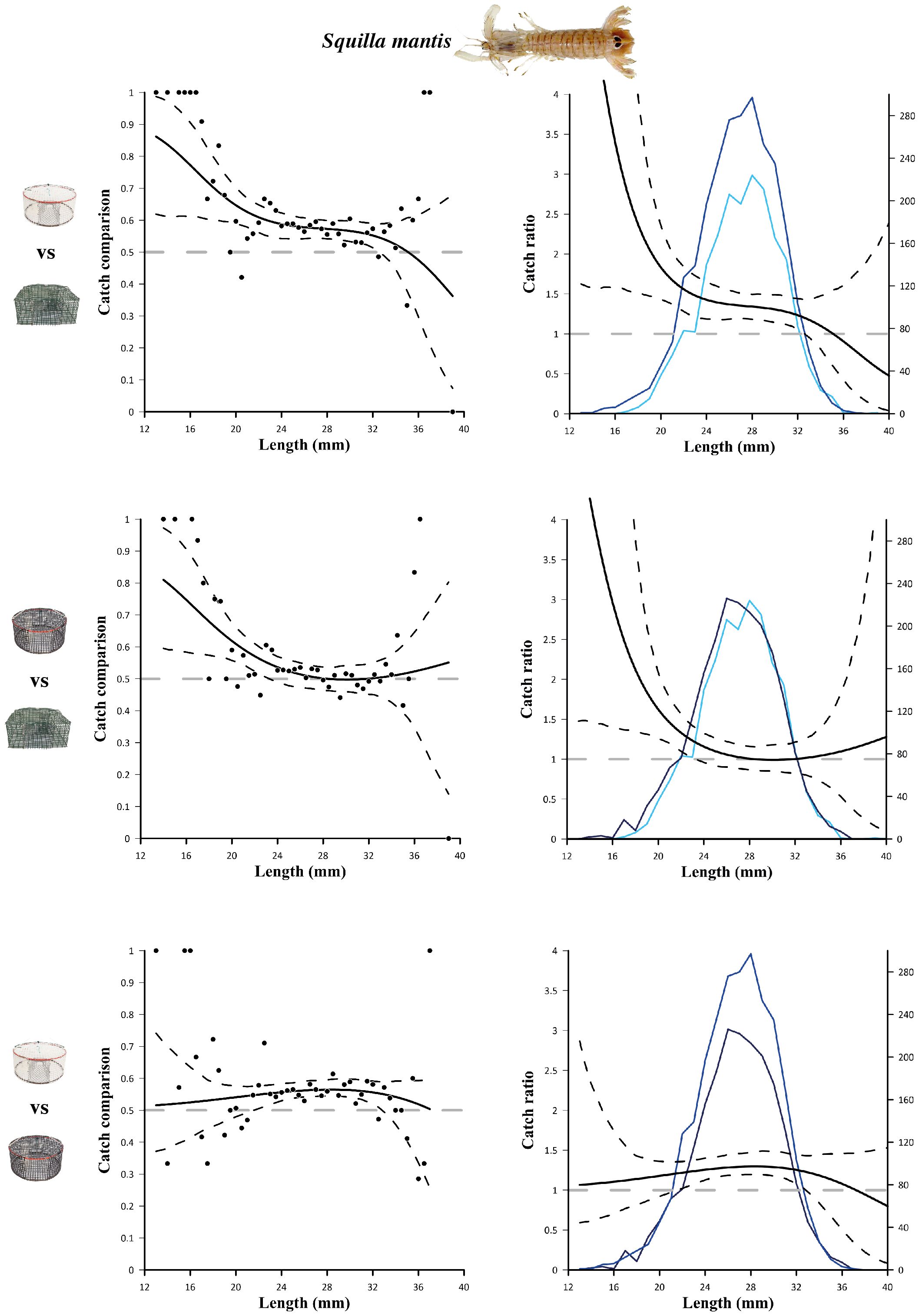
Figure 2 Results of the catch comparison analyses obtained for mantis shrimp (Squilla mantis) between the three different pot designs, traditional design (green) and experimental design (white and black). The graph on the left shows the modelled catch comparison rate (black line) with 95% CIs (black stippled curves); black circles represent the experimental rate; the grey horizontal line at 0.5 represents the baseline at which both gears have equal catch rates. Graphs on the right show the catch ratio (black line) with 95% CI (black stippled curves); blue lines represent the length frequency distributions obtained with the two gears (blue: white pots; light blue: green pots; dark blue: black pots); the grey horizontal line at 1.0 represents the baseline at which both gears have equal catch rates.
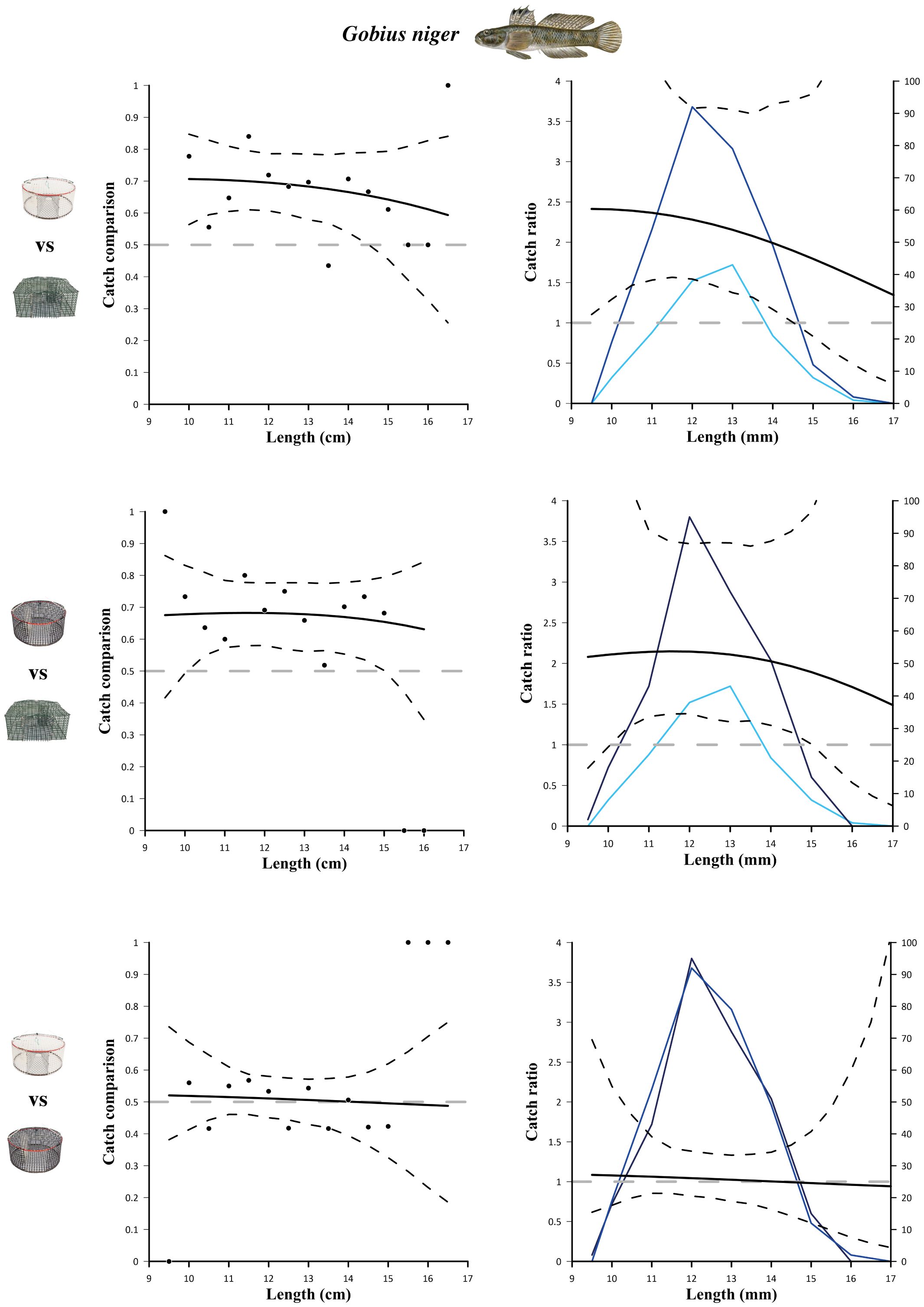
Figure 3 Results of the catch comparison analyses obtained for black goby (Gobius niger) between the three different pot designs, traditional design (green) and experimental design (white and black). The graph on the left shows the modelled catch comparison rate (black line) with 95% CIs (black stippled curves); black circles represent the experimental rate; the grey horizontal line at 0.5 represents the baseline at which both gears have equal catch rates. Graphs on the right show the catch ratio (black line) with 95% CI (black stippled curves); blue lines represent the length frequency distributions obtained with the two gears (blue: white pots; light blue: green pots; dark blue: black pots); the grey horizontal line at 1.0 represents the baseline at which both gears have equal catch rates.
Figure 4 and Supplementary Table S3 show the results obtained from the exploitation pattern indicator analysis. The results for S. mantis and G. niger confirm the observations made in the catch comparison analysis. White pots caught significantly more S. mantis than both green and black pots in both number of individuals (on average, 37 and 27% more, respectively) and weight (on average, 34 and 30% more, respectively), while no significant differences were detected between black and green pots. Both white and black pots caught, on average, 122–140% (in number of individuals) and 129–136% (in weight) more G. niger than green pots, while no significant differences were observed between the two experimental pot designs (Figure 4, Supplementary Table S3). Also, white pots were significantly more efficient at catching Spicara maena (species ID 3) than black pots and green pots in both number of individuals (on average, 150 and 347% more, respectively) and weight (on average, 218 and 367% more, respectively). No other significant differences between pots were observed in the catches of the other species.
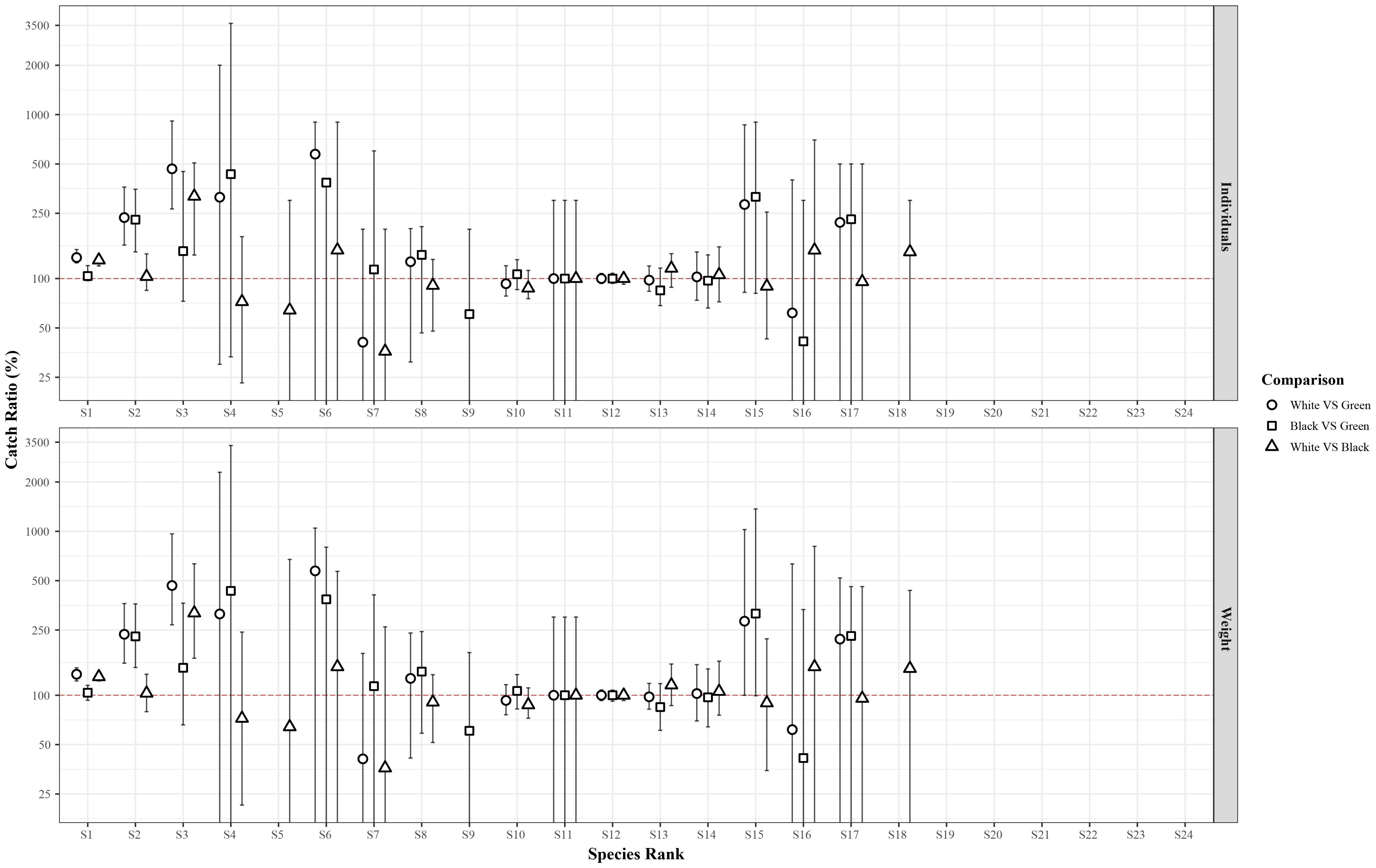
Figure 4 Results of the exploitation pattern indicator analysis for the 24 species caught in the sea trials. For each species, the three box plots indicate the ratio (in average percentages with 95% confidence intervals) of the total number of individuals (upper graph) and weight (lower graph) retained by the test pot compared to the baseline pot, for each comparison performed, i.e. white vs green, white vs black or black vs green pots. The horizontal red line indicates similar exploitation pattern between the two pot designs compared.
3.2 Comparing the species composition
Figure 5 represents, for each design, the cumulative species dominance (in percentages), in both number of individuals and weight, of the three catch fractions. Additional results are reported in Supplementary Material. Regardless of the pot design, the species having a commercial value represented, on average, more than 66% in number of individuals and 88% in weight. The catch composition in weight was dominated by the target species S. mantis, with a contribution of more than 75% and 85% of the total and landed catch, respectively (with no significant differences between pot designs; Supplementary Table S4), while no contribution was given to the discarded fraction. In number of individuals, this species almost saturates the cumulative curve of the landed fraction while its contribution to the total catch was around 50% on average. G. niger (species ID 2) was the second most abundant landed species, with contributions ranging, on average, from 5% to 9% in number of individuals and from 3% to 5.5% in weight. Small contributions to the landed fraction (<3% in number of individuals and<1.5% in weight) were given by blotched picarel (S. maena, species ID 3), red mullet (Mullus barbatus, species ID 4) and changeable nassa (species ID 8). Nevertheless, the latter species had a high proportion in the discarded catch (on average, 24–27% in numbers and 14–17% in weight). Two other mollusc species had high proportions in the discarded fraction, i.e. purple dye murex, (Bolinus brandaris, species ID 10; on average 22–25% in numbers and 24–39% in weight) and netted dog whelk (Tritia reticulata, species ID 12; on average 29–32% in numbers and 9–14% in weight). Smaller proportions were observed for two always discarded crab species, i.e. angular crab (Goneplax rhomboides species ID 13) with less than 9.5% and 4.5% in the discarded and total fractions, respectively, and swimming crab (Liocarcinus sp., species ID 14), with less than 3.5% and 1.5% in the discarded and total fractions, respectively. The species with species IDs 15–24 were small fish that are only occasionally caught and discarded because of being too few, too small or below the legal size; therefore, their proportion in the catches were very low, especially in weight.
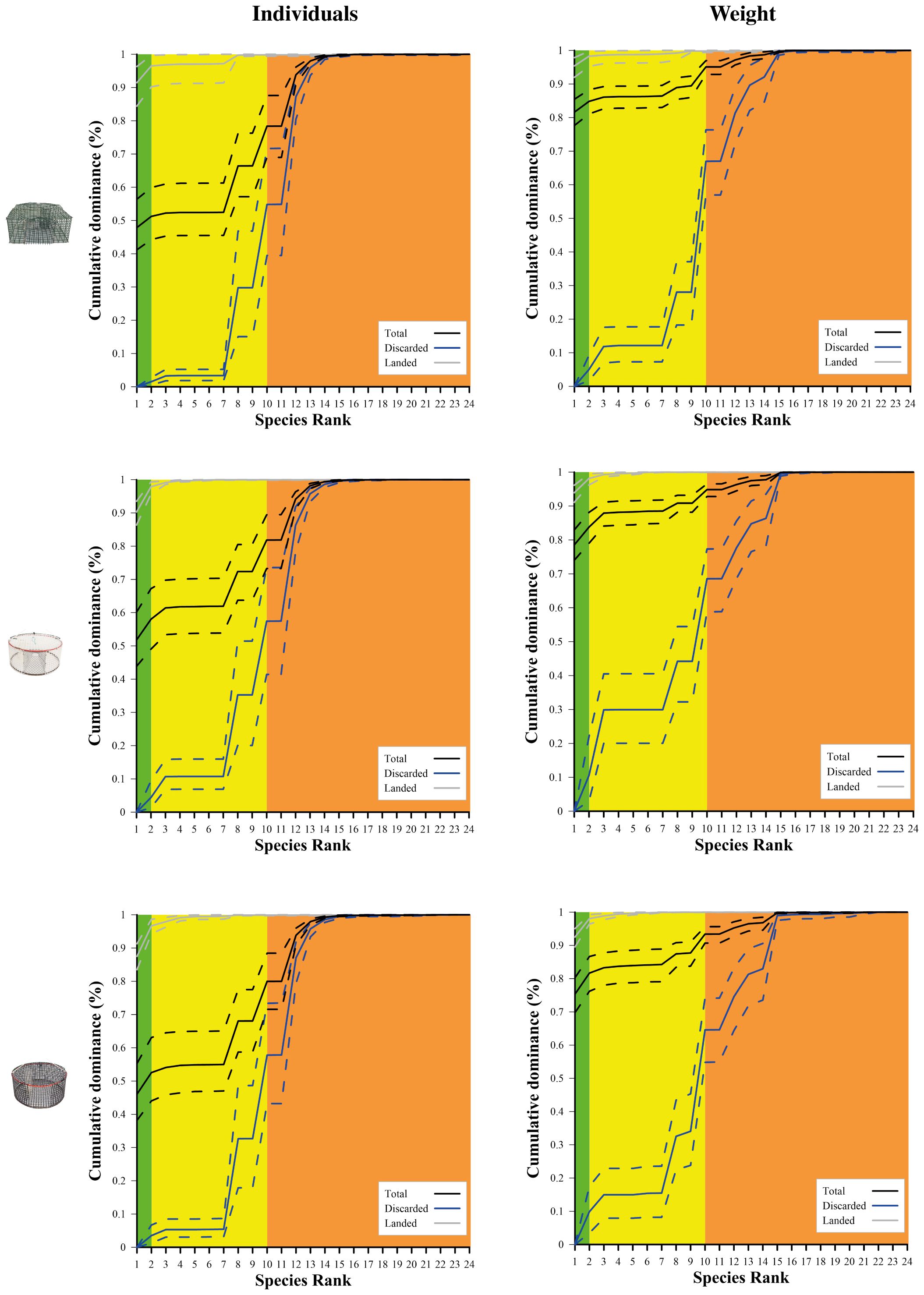
Figure 5 Cumulative species dominance in the catch of traditionally used green pots (top) and experimental white (middle) and black pots (down). The curves (solid lines) with 95% CIs (dotted lines) represent the cumulative species dominance for the catches in both number of individuals (left) and weight (right). The green, yellow and orange areas represent target species, other species with commercial value and species without commercial value, respectively.
Figure 6 shows the comparison (delta plots) between the catch dominance curves, for the total catch of the pots tested, i.e. white vs green, black vs green and white vs black. This figure provides a detailed insight at each single species level, considering the number of individuals (left) and weight (right). White pots had a significantly larger dominance of S. maena (species ID 3), when compared to both green and black pots, in both number of individuals and weight. Also, G. niger (species ID 2) was significantly more dominant in the total catch of white and black pots, when compared to the total catch of green pots, in both number and weight. In the comparison between black and green pots, another significant difference (only in weight) was detected for S. mantis (species ID 1), for which a larger dominance was observed in the total catch of green pots (Figure 5).
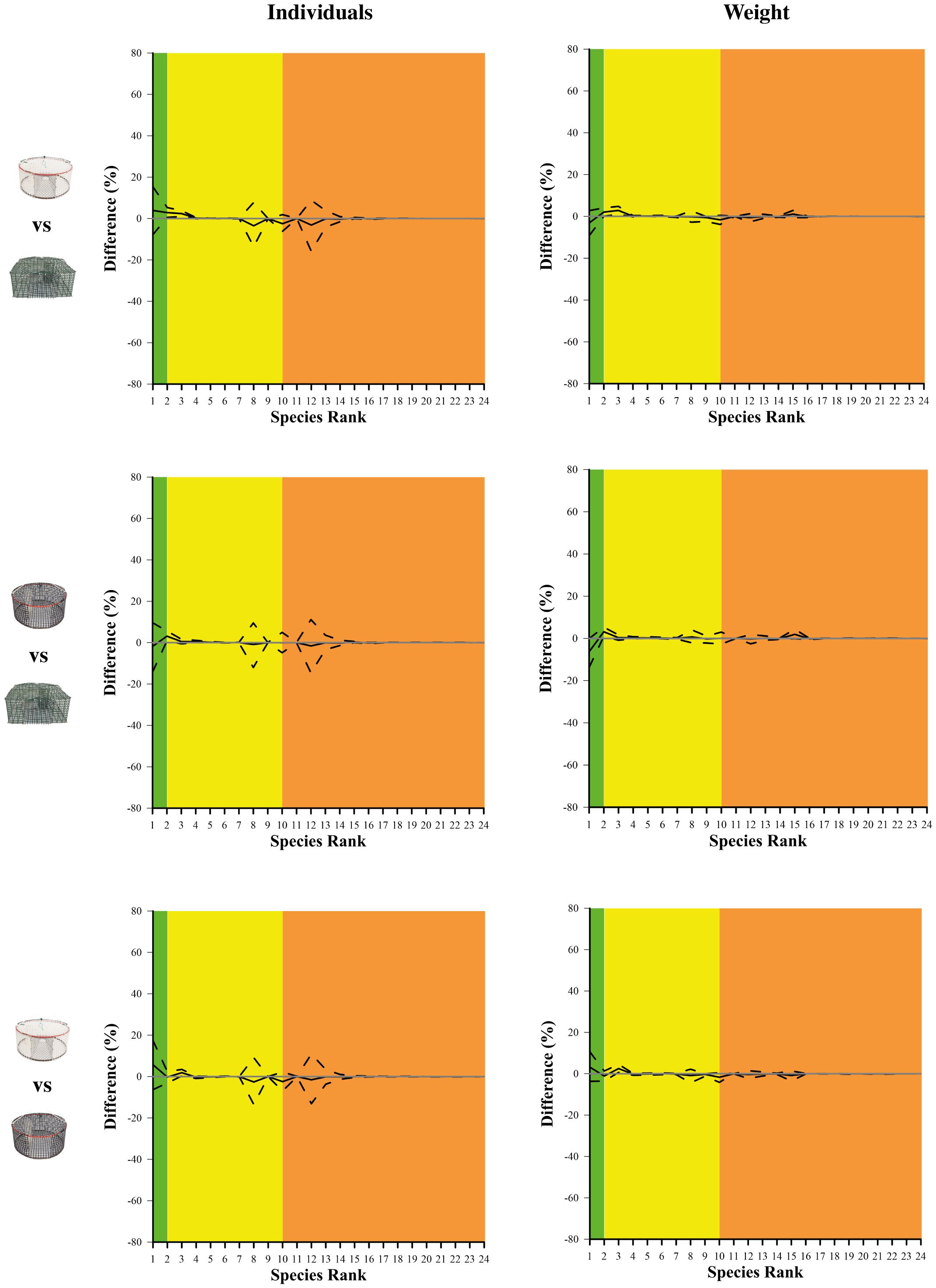
Figure 6 Delta plots resulting from the comparison of single species dominance in the total catch between white and green pots (top), black and green pots (middle) and white and black pots (down) in both number of individuals (left column) and weight (right column). The curves (solid lines) with 95% CIs (dotted lines) represent the comparison between gears at single species level. The grey horizontal line at 0 represents an equal proportion between the two gears. The green, yellow and orange areas represent target species, other species with commercial value and species without commercial value, respectively.
3.3 Underwater video observations
The recording duration varied from 55 to 65 minutes for each deployment. A total of 1044 minutes of video recordings were collected and analysed. The majority of individuals entering the pots in the first hour of deployment were mantis shrimps; only a few black gobies and blotched picarel were observed. The timing of first entrance for S. mantis was around 16 ± 3 (se) minutes (range: 3–46 minutes). Moreover, in the monitored pots around 70% of S. mantis catches occurred in the first hour of deployment (Supplementary Table S6).
Individuals of S. mantis tended to quickly climb along the slope of the entrance, and once entered, showed a stress response probably due to confinement, but no escape events through the entrance or meshes were recorded. No cases of aggressive behaviour were recorded between S. mantis individuals or other species inside the pot. Other fish species (e.g., S. maena and tub gurnard, Chelidonichthys lucernus) stopped for some time in front of the entrance, often without entering the pot. In several deployments, specimens of S. maena entered and exited the pots several times through pot entrance. Individuals of G. niger, once entered, tended to lie at the bottom of the pot in close contact with the seabed.
3.4 Wear resistance and influence of marine fouling
The main results are synthetized in Table 3. No pots were lost during our experiments, although percentages of damaged pots increased over time. Experimental design proved more resistant to long soak times and marine currents, as commercial pots wear out in half the time and needed to be repaired. Images of marine fouling on pots are reported in Supplementary Material. We observed that fouling affects almost exclusively the plastic material. Therefore, it affected the entrance in commercial design and the netting frame in the experimental design that were made of plastic materials. Specifically, after every fishing season, the plastic entrances of traditional pots were massively covered by fouling, whereas in experimental design, no fouling on the entrances was observed. The GLM regression analysis revealed a significant CER rate for traditional design with the increase of entrance fouling (p-value< 0.001). The coefficient was -0.072, showing a CER rate of approximately 7% (exp(-0.072) = 0.930) per fishing operation. Similarly, the GLM regression analysis for the novel design demonstrated a significant CER rate with the increase of netting frame fouling (p-value< 0.001). The coefficient was -0.0439, corresponding to a CER rate of about 4% (exp(-0.0439) = 0.957) per fishing operation. The observed difference between the two designs was statistically significant, as the 95% confidence interval (95% CI) was [-0.050, -0.012]. Therefore, the CER rate of commercial design should be considered significantly higher than novel design.
4 Discussion
The aim of the present study was to improve the performance of pots targeting mantis shrimp in a specific coastal SSF, by developing a new pot design. The adoption of pots as a low environmental impact gear encourages the SSF fleet diversification and thus reduces the fishing effort of other multi-species gear that often can catch unwanted species (e.g., gillnets and trammel nets; Yıldız and Karakulak, 2016; Lucchetti et al., 2020; Tzanatos et al., 2020).
The increase in pot volume from traditional to experimental design was made with an aim to increase the catch. Although in this study we cannot rule out that pot volume increase alone had an effect on the catch, since we tested a combination of different technical parameters together (e.g. also pot shape and mesh size), the mean number of S. mantis caught in each experimental pot (regardless of the colour) was slightly higher than the mean number in the traditional pot, suggesting that the volume increase could improve catches. Specifically, a larger pot volume might delay the saturation of the pot, i.e. when more animals are prevented to enter due to high densities of other preys inside the pot. Pot saturation has been widely observed in crustaceans (Miller, 1979; Castro and DeAlteris, 1990) and fish (Ovegård et al., 2011). Despite the volume increased by 50% compared to the traditional pot, the new design still remains small and easy to handle on board the Mediterranean SSF vessels.
Regarding the change of the pot shape, similar studies with pots targeting crabs in other areas have shown contrasting results on fishing efficiency (Vazquez Archdale and Kuwahara, 2005; Olsen et al., 2019). The observation of underwater videos made in the present study revealed that mantis shrimps in the vicinity of the pot reached the entrance more quickly in the circular design rather than in the traditional square design.
Both experimental pots caught a significantly larger amount of smaller S. mantis than traditional pots. This can be related to smaller mesh size used in experimental pot design that did not allow the escapement of individuals of smaller length classes of S. mantis. The same was observed for G. niger, since the experimental design was significantly more efficient than the traditional design at preventing small fish from escaping. We are confident in making this assumption since all pots were deployed in the same fishing ground at the same time; therefore, S. mantis and G. niger populations exploited by different pots was the same. Also, in a few cases we found small individuals of S. mantis stuck in the meshes, suggesting escape attempts through the pot netting, as observed in Croatia by Brčić et al. (2019). Even though in this fishery S. mantis do not have a minimum landing size (Regulation (EU), 2019) and all individuals caught are landed, the higher catch efficiency of experimental pots for the smallest length classes may pose a concern regarding sustainability of this fishery. However, it is worth considering that S. mantis is a fast-growing species with life-span from settlement estimated at around 18 months (Abelló and Martín, 1993). Also, this species exhibits strong scavenger habits when conditions permit. For example, in the Adriatic Sea, which is highly exploited in coastal areas particularly by dredging (Petetta et al., 2021a), fishing leads to large amounts of discarded organisms exploitable by scavengers (Carlucci et al., 2024). Hence, in this area, all the environmental conditions required for S. mantis to thrive are present, encompassing those influenced by human activities, thus securing their potential for future expansion. The most recent assessments estimated that S. mantis in this area is sustainably exploited; however, it was recommended not to increase the fishing mortality (SAC, and Scientific Advisory Committee on Fisheries, 2022). Therefore, the mesh size of experimental pots could be increased to reduce captures of small S. mantis and ensure a higher sustainability of the fishery. In this regard, further studies about pot selectivity are encouraged to estimate the optimal mesh size, taking into account that this species has a length at first sexual maturity at 25.5 mm CL (Colella et al., 2016). Also, since this species shows high survival rates (Barragán-Méndez et al., 2020), especially if harvested with pots, it would be appropriate to release the smaller specimens directly into the sea.
Pot colour is a technical parameter that depending on fishery and target species could influence catch efficiency (Petetta et al., 2021b). In the present study, the new pot design, in white colour, was more efficient at capturing S. mantis than the same design in black colour and the traditional green pot design. This colour preference could likely be related to the complex colour vision in stomatopod crustaceans, which has been widely investigated (Marshall, 1999; Thoen et al., 2014; Streets et al., 2022). Mantis shrimps have both colour vision, that allows them to easily distinguish several colours, and polarization vision for visual tasks such as finding prey (Zhong et al., 2020). The effect of pot colour in the experimental design was not evident for the smallest individuals, suggesting that they are not subjected to a white/black attraction/deterrence. The blotched picarel was also caught in significantly larger amounts in the white pots, compared to black pots and traditional green pots. This is an interesting insight for a species of the Sparidae family, which is highly exploited by several SSFs of the Mediterranean (Grati et al., 2022). Its colour preference for white pots is in contrast with what described in Cetinic et al. (2002) for a coastal fishery of the Eastern Adriatic (called “Tramata”) specifically targeting sparids, where the ropes of the gear are usually equipped with white plastic lines or other material of white colour as an additional method for scaring the fish. We can hypothesize that colour of the gear is not affecting catch efficiency for G. niger, since no differences in the catches between the black and white experimental pots were observed for this species, which represents an important additional catch, especially in the northern part of the Adriatic Sea (Bon et al., 2006). In the same area, Grati et al. (2010) studied the behaviour of T. mutabilis towards basket traps, also stating that this species did not show any preference for black compared to white netting.
In the present study, the majority of species caught (15 out of 24) were always discarded, as it was observed in other fisheries in the area, such as bottom trawl fishery (Petetta et al., 2022, 2023). However, in our experiments, the amount of specimens caught within the discarded species were low compared to the high rates of discards produced by, for instance, bottom trawls in the area (Petetta et al., 2023). Specifically, species with commercial value represented, in weight and numbers, the majority of total catch in pots, while the unwanted species had a minor proportion, with no Protected, Endangered and Threatened (PET) species caught. By contrast, passive nets (gillnet and trammel net) locally used in the coastal SSFs of the area often catch several individuals of species without commercial interest and PET species, such as elasmobranchs and sea turtles (Lucchetti et al., 2017, 2019; Virgili et al., 2018, 2024).
As regards to the material of construction, we observed that the use of a plastic netting ensured a higher resistance to wear of the gear (and therefore less maintenance) in the new design. By contrast, the use of plastic in the entrance funnel in traditional design over time led to a reduction in catch efficiency due to a greater susceptibility to fouling of this material. Marine fouling is also an issue in other trap fisheries as in the case of fyke net targeting cuttlefish, reducing fishing performance over time (Belcari et al., 2002).
In our study, rigging of the gear ensured that no pot was lost, resisting to bad weather conditions and underwater currents. Also, the increased weight of the experimental pot can contribute to a higher stability of the gear. Given a low likelihood of pot loss, the expected plastic pollution and ghost fishing is supposed to be very low. In some crustacean pot fisheries, ghost fishing pots were observed to capture from 8 to 16% crab compared to actively fished pots (Cerbule et al., 2023; Yu et al., 2024), while, in other fisheries, pots’ fishing potential has been found to be low after bait consumption, with a high chance of escape for non-target species (Adey et al., 2008; Ungfors et al., 2013).
The improved catch efficiency observed when using the new pot design could broaden the interest towards this fishery, also in other Mediterranean areas where S. mantis is abundant. The experiment we conducted, if replicated, will help understanding whether the encouraging results here obtained can be extrapolated in the whole mantis shrimp fishery. In conclusion, we demonstrated that gear modification in the context of a coastal SSF can lead to both, a reduced environmental impact and improved fishing performance in a more sustainable way.
Data availability statement
The raw data supporting the conclusions of this article will be made available by the authors, without undue reservation.
Ethics statement
Ethical approval was not required for the study involving animals in accordance with the local legislation and institutional requirements since the fishing trials have been authorised by the Italian Ministry of Agriculture, Food Sovereignty and Forests and subsequently by the Italian Coastguard. No other authorization or ethics board approval was required. No information on animal welfare or on steps taken to mitigate fish suffering and methods of sacrifice is provided since the animals were not exposed to any additional stress other than that involved in commercial fishing practices.
Author contributions
MV: Conceptualization, Data curation, Investigation, Methodology, Supervision, Validation, Visualization, Writing – original draft, Writing – review & editing. AP: Conceptualization, Data curation, Formal analysis, Investigation, Methodology, Supervision, Validation, Visualization, Writing – original draft, Writing – review & editing. BH: Conceptualization, Data curation, Formal analysis, Methodology, Visualization, Writing – review & editing. KC: Methodology, Visualization, Writing – review & editing. SG: Formal analysis, Writing – review & editing. DLV: Methodology, Writing – review & editing. GB: Data curation, Writing – review & editing. AL: Funding acquisition, Project administration, Resources, Validation, Visualization, Writing – review & editing.
Funding
The author(s) declare financial support was received for the research, authorship, and/or publication of this article. This study was conducted with the contribution of the LIFE financial instrument of the European Community, DELFI Project-Dolphin Experience: Lowering Fishing Interactions (LIFE18NAT/IT/000942). It does not necessarily reflect the European Commission’s views and in no way anticipates future policy.
Acknowledgments
The deepest appreciation goes to the crew of MP Giammario GB and his family (Pedaso, Italy) for their effort in the fieldwork and data collection. We are grateful to Monia Bonfili (Bonfili Design; www.bonfilidesign.it) for the figures illustration.
Conflict of interest
The authors declare that the research was conducted in the absence of any commercial or financial relationships that could be construed as a potential conflict of interest.
Publisher’s note
All claims expressed in this article are solely those of the authors and do not necessarily represent those of their affiliated organizations, or those of the publisher, the editors and the reviewers. Any product that may be evaluated in this article, or claim that may be made by its manufacturer, is not guaranteed or endorsed by the publisher.
Supplementary material
The Supplementary Material for this article can be found online at: https://www.frontiersin.org/articles/10.3389/fmars.2024.1408036/full#supplementary-material.
References
Abelló P., Martín P. (1993). Fishery dynamics of the mantis shrimp Squilla mantis (Crustacea: Stomatopoda) population off the Ebro delta (northwestern Mediterranean). Fish. Res. 16, 131–145. doi: 10.1016/0165-7836(93)90048-C
Adey J. M., Smith I. P., Atkinson R. J. A., Tuck I. D., Taylor A. C. (2008). “Ghost fishing” of target and non-target species by Norway lobster Nephrops norvegicus creels. Mar. Ecol. Prog. Ser. 366, 119–127. doi: 10.3354/meps07520
Amengueal-Ramis J. F., Vázquez-Archdale M., Cánovas-Pèrez C., Morales-Nin B. (2016). The artisanal fishery of the spiny lobster Palinurus elephas in Cabrera National Park, Spain: Comparative study on traditional and modern traps with trammel nets. Fish. Res. 179, 23–32. doi: 10.1016/j.fishres.2016.01.022
Barragán-Méndez C., González-Duarte M. M., Sobrino I., Vila Y., Mancera J. M., Ruiz-Jarabo I. (2020). Physiological recovery after bottom trawling as a method to manage discards: The case study of Nephrops norvegicus and Squilla mantis. Mar. Policy 116, 103895. doi: 10.1016/j.marpol.2020.103895
Bastardie F., Angelini S., Bolognini L., Fuga F., Manfredi C., Martinelli M., et al. (2017). Spatial planning for fisheries in the Northern Adriatic: working toward viable and sustainable fishing. Ecosphere 8, e01696. doi: 10.1002/ecs2.1696
Belcari P., Sartor P., Sanchez P., Demestre M., Tsangridis A., Leondarakis P., et al. (2002). Exploitation patterns of the cuttlefish, Sepia officinalis (Cephalopoda, Sepiidae), in the Mediterranean Sea. Bull. Mar. Sci. 71, 187–196.
Bon G., Feliziani L., Doz M., Barbieri G., Kutin S. (2006). Innovative methods of analysis of the trap fisheries for Squilla mantis and Gobius Niger. Biol. Mar. Mediterr. 13, 809–813.
Brčić J., Herrmann B., Masanovic M., Krstulovic Sifner S., Baranovic M., Škeljo F. (2019). Mesh sticking probability in fishing gear selectivity: Methodology and case study on Norway lobster (Nephrops norvegicus) and mantis shrimp (Squilla mantis) in the Mediterranean Sea creel fishery. Mediterr. Mar. Sci. 0, 487–495. doi: 10.12681/mms.19018
Brčić J., Herrmann B., Mašanović M., Šifner S. K., Škeljo F. (2017). Influence of soak time on catch performance of commercial creels targeting Norway lobster (Nephrops norvegicus) in the Mediterranean Sea. Aquat. Living Resour. 30, 36. doi: 10.1051/alr/2017035
Burnham K. P., Anderson D. R. (2002). Model selection and multimodel inference. A practical information-theoretic approach. 2nd ed (New York: Springer).
Carlucci R., Cipriano G., Cascione D., Ingrosso M., Barbone E., Ungaro N., et al. (2024). Influence of hydraulic clam dredging and seasonal environmental changes on macro-benthic communities in the Southern Adriatic (Central Mediterranean Sea). BMC Ecol. Evol. 24, 1–19. doi: 10.1186/s12862-023-02197-9
Castro K. M., DeAlteris J. T. (1990). Effects of trap saturation and species interaction on the capture of Callinectes Spp. crabs in the Guayas Estuary. Fish. Res. 8, 223–232. doi: 10.1016/0165-7836(90)90023-O
Cerbule K., Herrmann B., Grimaldo E., Brinkhof J., Sistiaga M., Larsen R. B., et al. (2023). Ghost fishing efficiency by lost, abandoned or discarded pots in snow crab (Chionoecetes opilio) fishery. Mar. pollut. Bull. 193, 115249. doi: 10.1016/j.marpolbul.2023.115249
Cerbule K., Savina E., Herrmann B., Larsen R. B., Feekings J. P., Krag L. A., et al. (2022). Quantification of catch composition in fisheries: A methodology and its application to compare biodegradable and nylon gillnets. J. Nat. Conserv. 70, 126298. doi: 10.1016/j.jnc.2022.126298
Cetinic P., Soldo A., Dulcic J., Pallaoro A. (2002). Specific method of fishing for Sparidae species in the eastern Adriatic. Fish. Res. 55, 131–139. doi: 10.1016/S0165-7836(01)00299-5
Colella S., Donato F., Panfili M., Santojanni A. (2016). Reproductive parameters and sexual maturity scale of Squilla mantis L. 1758 (crustacea: stomatopoda) in the North- Central Adriatic Sea (GSA17). Biol. Mar. Mediterr. 23, 258–259. Available at: https://hdl.handle.net/20.500.14243/375084
Efron B. (1982). The jackknife, the bootstrap and other resampling plans (Society for Industrial and Applied Mathematics (SIAM). doi: 10.1137/1.9781611970319
FAO-GFCM (2023). FAO FishstatJ. @ in Fishery and Aquaculture Statistics. GFCM capture production 1970–2021 (FishstatJ) (Rome: FAO Fisheries and Aquaculture Department). Available at: www.fao.org/fishery/statistics/software/fishstatj/en.
Farrugio H. (2013). “Current situation of small-scale fisheries in the Mediterranean and Black Sea: strategies and methodologies for an effective analysis of the sector,” in Report of the first regional symposium on sustainable small-scale fisheries in the mediterranean and black sea, (General Fisheries Commission for the Mediterranean (GFCM)). 27–30.
Froglia C. (1996). Growth and behaviour of Squilla mantis (mantis shrimp) in the Adriatic Sea, EU Study DG XIV/MED/93/016, Final Report.
Grati F., Aladžuz A., Azzurro E., Bolognini L., Carbonara P., Çobani M., et al. (2018). Seasonal dynamics of small-scale fisheries in the Adriatic Sea. Mediterr. Mar. Sci. 19, 21–35. doi: 10.12681/mms.2153
Grati F., Azzurro E., Scanu M., Tassetti A. N., Bolognini L., Guicciardi S., et al. (2022). Mapping small-scale fisheries through a coordinated participatory strategy. Fish Fish. 23, 773–785. doi: 10.1111/faf.12644
Grati F., Polidori P., Scarcella G., Fabi G. (2010). Estimation of basket trap selectivity for changeable nassa (Nassarius mutabilis) in the Adriatic Sea. Fish. Res. 101, 100–107. doi: 10.1016/j.fishres.2009.09.012
Grimaldo E., Herrmann B., Su B., Føre H. M., Vollstad J., Olsen L., et al. (2019). Comparison of fishing efficiency between biodegradable gillnets and conventional nylon gillnets. Fish. Res. 213, 67–74. doi: 10.1016/j.fishres.2019.01.003
Herrmann B., Cerbule K., Brčić J., Grimaldo E., Geoffroy M., Daase M., et al. (2022). Accounting for uncertainties in biodiversity estimations: A new methodology and its application to the mesopelagic sound scattering layer of the high arctic. Front. Ecol. Evol. 10. doi: 10.3389/fevo.2022.775759
Herrmann B., Sistiaga M., Nielsen K. N., Larsen R. B. (2012). Understanding the size selectivity of redfish (Sebastes spp.) in North Atlantic trawl codends. J. Northwest Atl. Fish. Sci. 44, 1–13. doi: 10.2960/J.v44.m680
Herrmann B., Sistiaga M., Rindahl L., Tatone I. (2017). Estimation of the effect of gear design changes on catch efficiency: Methodology and a case study for a Spanish longline fishery targeting hake (Merluccius merluccius). Fish. Res. 185, 153–160. doi: 10.1016/j.fishres.2016.09.013
Herrmann B., Sistiaga M., Santos J., Sala A. (2016). How many fish need to be measured to effectively evaluate trawl selectivity? PloS One 11, 1–22. doi: 10.1371/journal.pone.0161512
ISSCFG (2016). “International standard statistical classification of fishing gear,” in Handbook of fishery statistics, (Rome: FAO). vol. 2.
Lauriano G., Caramanna L., Scarnó M., Andaloro F. (2009). An overview of dolphin depredation in Italian artisanal fisheries. J. Mar. Biol. Assoc. United Kingdom 89, 921–929. doi: 10.1017/S0025315409000393
Li Veli D., Petetta A., Barone G., Ceciarini I., Franchi E., Marsili L., et al. (2023). Fishers’ perception on the interaction between dolphins and fishing activities in italian and Croatian waters. Diversity 15 (2), 133. doi: 10.3390/d15020133
Lucchetti A., Bargione G., Petetta A., Vasapollo C., Virgili M. (2019). Reducing sea turtle bycatch in the Mediterranean mixed demersal fisheries. Front. Mar. Sci. 6. doi: 10.3389/fmars.2019.00387
Lucchetti A., Vasapollo C., Virgili M. (2017). Sea turtles bycatch in the Adriatic Sea set net fisheries and possible hot - spot identification. Aquat. Conserv. Mar. Freshw. Ecosyst. 27, 1176–1185. doi: 10.1002/aqc.2787
Lucchetti A., Virgili M., Petetta A., Sartor P. (2020). An overview of gill net and trammel net size selectivity in the Mediterranean Sea. Fish. Res. 230, 105677. doi: 10.1016/j.fishres.2020.105677
Madhu V. R., Anand S. B., Cerbule K., Herrmann B. (2023). Simple pot modification improves catch efficiency and species composition in a tropical estuary mud crab (Scylla serrata) fishery. Estuar. Coast. Shelf Sci. 288, 108369. doi: 10.1016/j.ecss.2023.108369
Marshall J. (1999). The colorfull world of the mantis shrimp. Nat. Commun. 401, 873–874. doi: 10.1038/44751
Miller R. J. (1979). Saturation of crab traps: reduced entry and escapement. ICES J. Mar. Sci. 38, 338–345. doi: 10.1093/icesjms/38.3.338
Miller R. J. (1990). Effectiveness of crab and lobster traps. Can. J. Fish. Aquat. Sci. 47, 1228–1251. doi: 10.1139/f90-143
Morello E. B., Antolini B., Gramitto M. E., Atkinson R. J. A., Froglia C. (2009). The fishery for Nephrops norvegicus (Linnaeus 1758) in the central Adriatic Sea (Italy): Preliminary observations comparing bottom trawl and baited creels. Fish. Res. 95, 325–331. doi: 10.1016/j.fishres.2008.10.002
Morello E. B., Froglia C., Atkinson R. J. A., Moore P. G. (2005). Hydraulic dredge discards of the clam (Chamelea gallina) fishery in the western Adriatic Sea, Italy. Fish. Res. 76, 430–444. doi: 10.1016/j.fishres.2005.07.002
Olsen L., Herrmann B., Grimaldo E., Sistiaga M. (2019). Effect of pot design on the catch efficiency of snow crabs (Chionoecetes opilio) in the Barents Sea fishery. PloS One 14, e0219858. doi: 10.1371/journal.pone.0219858
Ovegård M., Königson S., Persson A., Lunneryd S. G. (2011). Size selective capture of Atlantic cod (Gadus morhua) in floating pots. Fish. Res. 107, 239–244. doi: 10.1016/j.fishres.2010.10.023
Petetta A., Herrmann B., Li Veli D., Virgili M., De Marco R., Lucchetti A. (2023). Every animal matters! Evaluating the selectivity of a Mediterranean bottom trawl fishery from a species community perspective. PloS One 18, e0283362. doi: 10.1371/journal.pone.0283362
Petetta A., Herrmann B., Virgili M., Bargione G., Vasapollo C., Lucchetti A. (2021a). Dredge selectivity in a Mediterranean striped venus clam (Chamelea gallina) fishery. Fish. Res. 238, 105895. doi: 10.1016/j.fishres.2021.105895
Petetta A., Herrmann B., Virgili M., Li Veli D., Brinkhof J., Lucchetti A. (2022). Effect of extension piece design on catch patterns in a mediterranean bottom trawl fishery. Front. Mar. Sci. 9. doi: 10.3389/fmars.2022.876569
Petetta A., Virgili M., Guicciardi S., Lucchetti A. (2021b). Pots as alternative and sustainable fishing gears in the Mediterranean Sea: an overview. Rev. Fish Biol. Fish. 31, 773–795. doi: 10.1007/s11160-021-09676-6
Pol M. V., He P., Winger P. D. (2010). Proceedings of the international technical workshop on gadoid capture by pots (GACAPOT). Massachusetts Div. Mar. Fish. Tech. Rep. 40, 107.
Ramieri E., Bocci M., Markovic M. (2019). “Linking integrated coastal zone management to maritime spatial planning: the mediterranean experience BT,” in Maritime Spatial Planning: past, present, future. Eds. Zaucha J., Gee K. (Springer International Publishing, Cham), 271–294. doi: 10.1007/978–3-319–98696-8_12
R Core Team (2023). R: A language and environment for statistical computing (R Found. Stat. Comput).
Regulation (EU) (2019). Regulation (EU) 2019/1241 of the European Parliament and of the Council of 20 June 2019 on the conservation of fisheries resources and the protection of marine ecosystems through technical measures. 105–201.
SAC, Scientific Advisory Committee on Fisheries (2022). Report of working group on stock assessment of demersal species (WGSAD) (Rome: FAO), 12–17.
Scientific Technical and Economic Committee for Fisheries (STECF) (2023). Fisheries dependent information FDI. Eds. Motova-Surmava Z., Zanzi A. (Luxembourg: Hekim Publications Office of the European Union). JRC136194.
Sobrino I., Juarez A., Rey J., Romero Z., Baro J. (2011). Description of the clay pot fishery in the Gulf of Cadiz (SW Spain) for Octopus vulgaris : Selectivity and exploitation pattern. Fish. Res. 108, 283–290. doi: 10.1016/j.fishres.2010.12.022
Streets A., England H., Marshall J. (2022). Colour vision in stomatopod crustaceans: more questions than answers. J. Exp. Biol. 225 (6), jeb243699. doi: 10.1242/jeb.243699
Suuronen P., Chopin F., Glass C., Løkkeborg S., Matsushita Y., Queirolo D., et al. (2012). Low impact and fuel efficient fishing-Looking beyond the horizon. Fish. Res. 119–120, 135–146. doi: 10.1016/j.fishres.2011.12.009
Thoen H. H., How M. J., Chiou T. H., Marshall J. (2014). A different form of color vision in mantis shrimp. Sci. (80-.). 343, 411–413. doi: 10.1126/science.1245824
Tiralongo N., Mancini E., Ventura D., De malerbe S., Paladini De Mendoza F., Sardone M., et al. (2021). Commercial catches and discards composition in the central Tyrrhenian Sea: a multispecies quantitative and qualitative analysis from shallow and deep bottom trawling. Mediterr. Mar. Sci. 22, 521–531. doi: 10.12681/mms.25753
Tiralongo F., Messina G., Lombardo B. M. (2018). Discards of elasmobranchs in a trammel net fishery targeting cuttlefish, Sepia officinalis Linnaeus 1758 along the coast of Sicily (central Mediterranean Sea). Reg. Stud. Mar. Sci. 20, 60–63. doi: 10.1016/j.rsma.2018.04.002
Tzanatos E., Georgiadis M., Peristeraki P. (2020). “Small-scale fisheries in Greece: status, problems, and management” in small-scale fisheries in Europe: status, resilience and governance. Eds. Pascual-Fernández J. J., Pita C., Bavinck M. (Springer International Publishing, Cham), 125–150. doi: 10.1007/978–3-030–37371-9_7
Ungfors A., Bell E., Johnson M. L., Cowing D., Dobson N. C., Bublitz R., et al. (2013). Nephrops fisheries in european waters. 1st ed (Elsevier Ltd). doi: 10.1016/B978-0-12-410466-2.00007-8
Vasapollo C., Virgili M., Bargione G., Petetta A., De Marco R., Punzo E., et al. (2020). Impact on macro-benthic communities of hydraulic dredging for razor clam ensis minor in the tyrrhenian sea. Front. Mar. Sci. 7, 14. doi: 10.3389/fmars.2020.00014
Vazquez Archdale M., Kuwahara O. (2005). Comparative fishing trials for Charybdis japonica using collapsible box-shaped and dome-shaped pots. Fish. Sci. 71, 1229–1235. doi: 10.1111/j.1444-2906.2005.01087.x
Veiga-Malta T., Feekings J., Herrmann B., Krag L. A. (2019). Industry-led fishing gear development: Can it facilitate the process? Ocean Coast. Manage. 177, 148–155. doi: 10.1016/j.ocecoaman.2019.05.009
Virgili M., Petetta A., Barone G., Li Veli D., Bargione G., Lucchetti A. (2024). Engaging fishers in sea turtle conservation in the Mediterranean Sea. Mar. Policy 160, 105981. doi: 10.1016/j.marpol.2023.105981
Virgili M., Vasapollo C., Lucchetti A. (2018). Can ultraviolet illumination reduce sea turtle bycatch in Mediterranean set net fisheries? Fish. Res. 199, 1–7. doi: 10.1016/j.fishres.2017.11.012
Warwick R. M., Clarke K. R., Somerfield P. J. (2008). “k-dominance curves,” in Encyclopedia of ecology (Elsevier BV Burlington, Massachusetts), 2055–2057. doi: 10.1016/B978-008045405-4.00114-2
Wileman D., Ferro R., Fonteyne R., Millar R. (1996). Manual of methods of measuring the selectivity of towed fishing gears. ICES Coop. Res. Rep. 215, 132.
Yıldız T., Karakulak F. S. (2016). Types and extent of fishing gear losses and their causes in the artisanal fisheries of Istanbul, Turkey. J. Appl. Ichthyol. 32, 432–438. doi: 10.1111/jai.13046
Yu M., Herrmann B., Cerbule K., Liu C., Dou Y., Zhang L., et al. (2024). Ghost fishing efficiency in swimming crab (Portunus trituberculatus) pot fishery. Mar. pollut. Bull. 201, 116192. doi: 10.1016/j.marpolbul.2024.116192
Keywords: sustainable fisheries, alternative fishing gear, small-scale coastal fisheries, Squilla mantis, pot fisheries, Mediterranean fisheries
Citation: Virgili M, Petetta A, Herrmann B, Cerbule K, Guicciardi S, Li Veli D, Barone G and Lucchetti A (2024) Efficient and sustainable: innovative pot design for a Mediterranean small-scale fishery. Front. Mar. Sci. 11:1408036. doi: 10.3389/fmars.2024.1408036
Received: 27 March 2024; Accepted: 10 June 2024;
Published: 28 June 2024.
Edited by:
Donald Behringer, University of Florida, United StatesReviewed by:
Francesco Tiralongo, University of Catania, ItalyFikret Öndes, Izmir Kâtip Çelebi University, Türkiye
Copyright © 2024 Virgili, Petetta, Herrmann, Cerbule, Guicciardi, Li Veli, Barone and Lucchetti. This is an open-access article distributed under the terms of the Creative Commons Attribution License (CC BY). The use, distribution or reproduction in other forums is permitted, provided the original author(s) and the copyright owner(s) are credited and that the original publication in this journal is cited, in accordance with accepted academic practice. No use, distribution or reproduction is permitted which does not comply with these terms.
*Correspondence: Massimo Virgili, massimo.virgili@cnr.it
†These authors have contributed equally to this work