- 1Universidad Autónoma de Baja California Sur, Departamento Académico de Ciencias Marinas y Costeras, La Paz, BCS, Mexico
- 2Centro para la Biodiversidad Marina y la Conservación, A.C., La Paz, BCS, Mexico
- 3Centro de Investigaciones Biológicas del Noroeste S.C. Departamento de Ecología Pesquera, La Paz, BCS, Mexico
- 4Universidad Autónoma de Baja California, Facultad de Ciencias Marinas, Ensenada, BC, Mexico
- 5Department of Life Sciences, Texas A&M University, Corpus Christi, TX, United States
- 6Centro de Estudios del Medio Ambiente, Eco-Alianza de Loreto, Loreto, BCS, Mexico
- 7Conserva Loreto, The Ocean Foundation, Loreto, BCS, Mexico
- 8Scripps Institution of Oceanography, University of California San Diego, La Jolla, CA, United States
Seagrasses are globally acknowledged as crucial habitats as they provide a variety of ecosystem services. Mexico’s legislation protects most of these marine plants; however, the protection often fails in application. The Gulf of California, despite being a biodiversity hotspot, has scant data on seagrasses. Here, human activity and climate change increasingly threaten these coastal ecosystems, with conservation and research efforts lacking coordination at a regional level. Our manuscript aimed to review and standardize existing data on Gulf of California seagrass species, ensuring open access for data updates; pinpointing conservation deficiencies; and guiding future research. We have added new records to the official public data, but we were able to recapture only 25% of the seagrass locations meaning a potential reduction in their historical distribution of 45.8%. Even though Mexico’s legislation protects some species of seagrasses, it protection often fails in the application. We identified that only 6.1% of the seagrass locations are within protected areas that recognize their presence in their management plans (e.g., the Balandra Flora and Fauna Protected Area and the Upper Gulf of California and Colorado River Delta Biosphere Reserve). At least 55.9% of seagrass records are associated with potentially damaging activities like pollution, coastal modification or biological resources use, while 23% are exposed to higher frequency of marine heatwaves. Given the importance of seagrass meadows under Mexican law and their internationally recognized ecological value, sharing current information and guiding research is essential. Our study seeks to galvanize renewed research initiatives and raise more awareness on the conservation of the Gulf of California’s seagrasses.
1 Introduction
Seagrasses provide essential ecosystem services including primary productivity, nutrient cycling, habitat provision, coastal protection, and carbon sequestration (Costanza et al., 1997; Ondiviela et al., 2014; Unsworth et al., 2019; United Nations Environment Programme, 2020). However, these vital meadows are in global decline due to climate change, overfishing, coastal development, and pollution (Orth et al., 2006; Waycott et al., 2009; Short et al., 2011; Brodie and de Ramon N’yeurt, 2018). The extent of seagrass loss is not fully known, largely because of insufficient historical data on their distribution (Brodie and de Ramon N’yeurt, 2018; McKenzie et al., 2020). This lack of data is pronounced in the Gulf of California, where there has been a historical deficit in mapping and research of seagrass species (López-Calderón et al., 2010; López-Calderón et al., 2016; McKenzie et al., 2020; Dunic et al., 2021).
The Gulf of California, along with the Pacific coast of the Baja California Peninsula, contains significant stretches of seagrasses within the Mexican Pacific (UNEP-WCMC and Short, 2021). Four seagrass species have been documented in the Gulf of California; three are protected under Mexican law (NOM-059-SEMARNAT-2010, DOF, 2019). —Zostera marina L. 1753, Halophila decipiens Ostenfeld 1902 (both under Special Protection), and Halodule wrightii Ascherson 1868 (considered Threatened). The fourth species, Ruppia maritima L. 1753, is not included in this legislation. Although widely distributed, the most prominent seagrass meadows are found in lagoons and bays, which makes them vulnerable to anthropogenic impacts (López-Calderón et al., 2010, López-Calderón et al., 2016). Given the importance of seagrasses for their benefits to human well-being, as blue carbon sinks and indirect contributors to climate change mitigation (Friess, 2023), it is crucial to gain a thorough understanding of their conditions and establish a research baseline. To date, the absence of coordinated regional research has resulted in a fragmented comprehension of seagrass ecosystems, and there is a significant lack of large-scale mapping, monitoring, or analysis of spatial trends. Consequently, the available official data on seagrass distribution in the Gulf of California is incomplete, inconsistent, or not current (e.g., UNEP-WCMC). Since these data are utilized by stakeholders and policymakers both locally and internationally, the need for accuracy is critical.
This review aims to 1) provide a comprehensive, validated, open-source dataset of seagrass locations in the Gulf of California by compiling an extensive collection of published studies, gray literature, herbarium records, and input from experts; 2) identify current research and conservation shortcomings; and 3) establish a baseline to direct future scientific endeavors. This effort is expected to act as a catalyst for new studies and support informed conservation tactics for seagrasses in the Gulf of California.
2 Materials and methods
2.1 Study area
The Gulf of California extends over 1,200 km, encompassing 40 coastal lagoons and 922 islands, and is part of the unique Cortezian marine ecoregion (Spalding et al., 2007). The regional climate is influenced by the El Niño Southern Oscillation (ENSO) and the Pacific Decadal Oscillation (PDO), factors contributing to substantial oceanic changes (Luch-Cota et al., 2013). In recent decades, the Gulf of California has experienced pronounced warming, affecting a multitude of marine species and habitats (Nevárez-Martínez et al., 2001; Velarde et al., 2004; Favoretto et al., 2022; Sánchez-Cabeza et al., 2022). It features distinct latitudinal gradients in sea surface temperature and chlorophyll-a concentrations, which delimit three primary oceanographic regions (Brusca, 2010; Ulate et al., 2016). These regions all experience similar maximum summer sea surface temperatures around 30°C. However, during winter, the northern region records cooler temperatures (16°C), the central region exhibits an intermediate average temperature of around 20°C while the southern region remains warmer (22°C). Also, the northern region has the highest annual average chlorophyll-a concentration at 1.6 mg m–3, compared to the southern region, which has an average of 0.5 mg m–3 (Ulate et al., 2016).
Seagrass meadows, found in the Gulf of California’s bays, lagoons, and inlets, are increasingly vulnerable to human activities. The eastern lagoons are heavily exploited for agriculture, aquaculture, settlement, and fishing. In contrast, the western bays and inlets face pressures from coastal development and mining activities (Álvarez-Borrego, 1983; Nevárez-Martínez et al., 2001; Velarde et al., 2004; González-Abraham et al., 2015; Páez-Osuna et al., 2016; Sánchez-Cabeza et al., 2022). These cumulative pressures threaten the most favorable habitats for seagrass meadows, underscoring the urgency to address current and future anthropogenic threats in the Gulf of California.
2.2 Systematic review protocol
In conducting this review, we undertook a thorough examination of academic and gray literature, national and international herbarium records, and floristic surveys. Our research employed ‘Google Scholar’ for the English search terms ‘Seagrasses’, ‘Gulf of California’ and the name of the species recorded in the region ‘Zostera marina,’ ‘Ruppia maritima,’ ‘Halodule wrightii,’ ‘Halophila decipiens’. Equivalent Spanish terms were used, replacing ‘Seagrasses’ with ‘Pastos marinos’ and ‘Gulf of California’ with ‘Golfo de California.’ The search was conducted from July 2019 to March 2021. Additionally, we classified the studies according to their primary focus and depicted their temporal progression graphically. While our primary emphasis was on peer-reviewed scientific, we included other sources of information such as technical and governmental reports, theses at the undergraduate, master’s, and doctoral levels due to their significant insights into the distribution and condition of the seagrass meadows.
Subsequently, we evaluated seven online herbarium collections from the following institutions: the University of Arizona (ARIZ), Arizona State University (ASU), Autonomous University of Baja California (CMMEX), National Herbarium of Mexico (MEXU) of the National Autonomous University of Mexico, Smithsonian National Museum of Natural History (SI NMNH), Jesús González Ortega Herbarium at the Autonomous University of Sinaloa (UAS), and the University of Sonora (UNISON-USON). From these archives, we extracted details such as species identification, collection dates, locality, and geographical coordinates. Each entry was then organized by location, facilitating the creation of maps that illustrate the historical distribution of each seagrass species throughout the Gulf of California.
2.3 Assessing seagrass distribution and potential stressors
2.3.1 Validation and assessment of distribution decline
Historical distribution records were obtained through the systematic review. On the field, we executed aerial reconnaissance to authenticate the presence or absence of seagrasses at coordinates reported in historical records. Drone flights, with altitudes oscillating between 10m and 60m, were primarily employed for intertidal seagrass detection. In addition, snorkeling expeditions complemented these aerial inspections. We classified records as “present”, when the record was validated at historically reported coordinates, when seagrasses were not found, the record was classified as “absent”. Finally, where the historical record was conspicuously imprecise (e.g., coordinates fell inland) or when pixel classification and drone imagery were unattainable, records were designated as “data deficient”. The Datasheet 1 includes information about the tools used in each location, and specific data about them.
2.3.2 Assessment of protection level
We define the protection level as the existence of conservation measures for each record such as the presence of a protected area. The visualization of this data is available at Datasheet 2. As the presence of a protected area is not indication of active protection on the seagrass ecosystem, we also reviewed management plans to discriminate the ones mentioning seagrasses explicitly or not. We then classified areas not protected as the ones outside any management polygon and separated protected areas according to their category established by the National Commission of Protected Natural Areas (CONANP) (e.g., National Park, Flora and Fauna protection area, Biosphere reserve, or Ramsar site). We used spatial data from government digital repositories (Comisión Nacional de Áreas Naturales Protegidas, 2021) Natural Protected Area locations, utilizing Geographical Information Systems (QGIS, v. 3.18).
2.3.3 Assessment of potential human and climate stressors
We identified potential human stressors based on the presence of activities known to harm seagrass health and gauged climate stressors using marine heatwave frequency as a proxy. Due to the varied thermal tolerances of species and a lack of specific quantitative data, we propose that increased marine heatwave frequency, indicative of extreme climate events, suggests elevated ecosystem stress.
For human stressors, we consulted scientific literature, local reports, and protected area management plans from governmental archives. We noted the presence of activities such as agricultural runoff leading to eutrophication, coastal development activities like construction and dredging that destroy habitats, and harmful fishing practices. These stressors often co-occur, amplifying their impact. Spatial correlation to localities was conducted using QGIS.
Regarding climate stressors, we analyzed sea surface temperature (SST) data from the Reynolds’ OISST dataset, spanning 1982-2020, with a resolution of 0.25 x 0.25 degrees. Marine heatwaves, defined as periods where daily SSTs exceeded the 90th percentile threshold for at least five consecutive days, were identified and their frequency was modeled using a GLM with a Poisson distribution in R, utilizing the “heatwaveR” package (Hobday et al., 2016; Schlegel and Smit, 2018). Rates of change in heatwave frequency were calculated for each grid point and used as a proxy for potential future impacts, normalized on a 0-1 scale for compatibility with other criteria. Detailed methodologies and additional statistical treatments are provided in the Datasheet 2.
3 Results
3.1 Seagrass distribution
Our research compiled records of seagrass species from 28 locations in the Gulf of California, with 20 on the eastern coast and six on the Baja California Peninsula (Figure 1). We compared our results with the official data from Ocean Data Viewer where only nine seagrass locations have been reported (UNEP-WCMC & Short, 2021). This data does not provide information about the extension or the name of the locality, only provide the coordinates. Additionally, they focus on only two of the four species of seagrass in the Gulf of California (Zostera marina and Halodule wrightii). Particularly in Los Cabos, this database includes a record of Phillospadix torreyi and Phillospadix scouleri, both species that are not recorded within the Gulf of California.
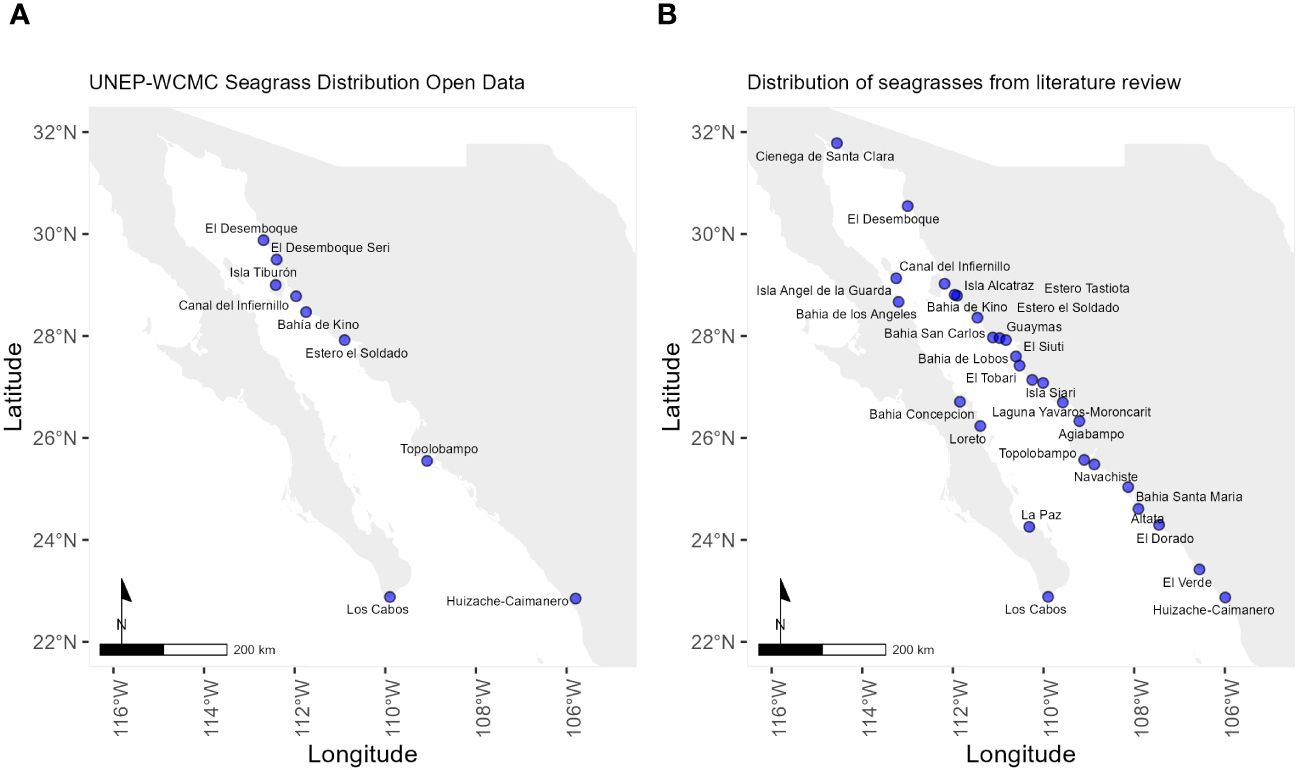
Figure 1 Dual maps compare seagrass distributions along the coast. (A) displays records obtained from UNEP-WCMC (2021), while (B) reflects this study’s updated findings, showcasing expanded and refined seagrass locations. Data information with geographic coordinates is available in Datasheet 1.
We identified primary seagrass localities through record frequency, occurrence rates, and data on their distribution. First, The Canal del Infiernillo, between the Sonora coast and Tiburon Island, includes all bays and estuaries within, then Bahía Concepción, as a second critical seagrass habitat in the Gulf of California.
In this work, we include new records. Records prior to 2018 are observations by the authors that have not been previously published. While the records obtained during our field validation efforts were collected between 2018 and 2022 (Datasheet 1). In 2020, we identified H. wrightii in Ensenada de la Paz channel. Additionally, H. decipiens was in San Gabriel Bay on Isla Espiritu Santo in 2018, and at the Pichilingue research unit of the Autonomous University of Baja California Sur in 2022.
3.2 Human and climate stressors
The analysis of our results shows that several locations are likely facing multiple stress factors. 17 of 28 historical locations are subject to the three main types of human-induced stressors: pollution, coastal modification, and overuse of biological resources (Figure 2A). While climate stress occurs in 12 locations. However, this phenomenon is most pronounced at spots like the Colorado River delta, Bahia Concepción, and El Verde coastal lagoon (Figure 2B).
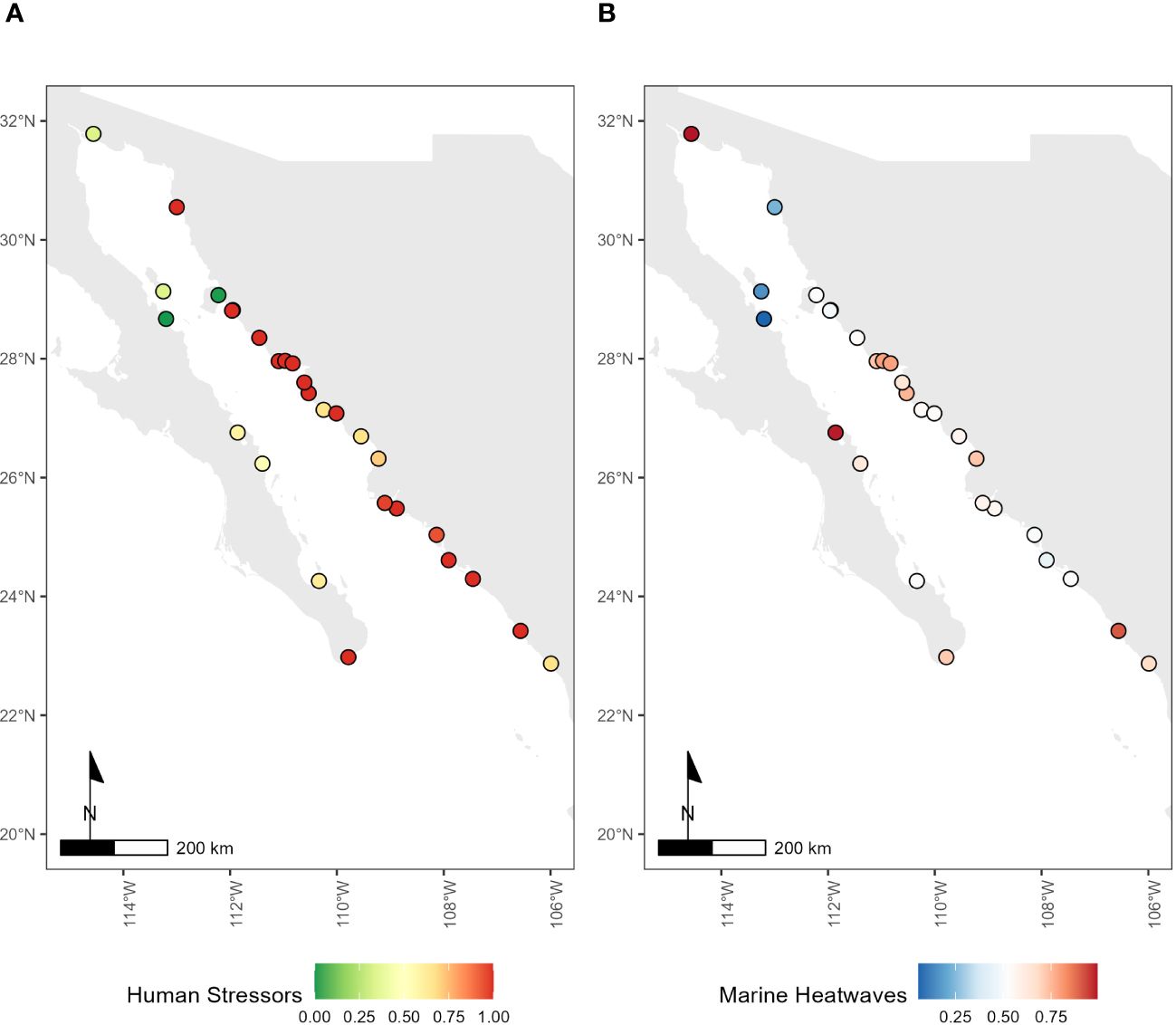
Figure 2 Spatial distribution of human stressors and marine heatwave frequencies over seagrass meadows. (A) illustrates a cumulative score for human-induced stressors impacting seagrass meadows, with values ranging from low (0.00) to high (1.00), depicted as a gradient of colors from green (low) to red (high). (B) represents the slope of marine heatwave (MHW) frequencies, calculated for each seagrass locality, with cooler colors indicating lower frequencies and warmer colors denoting higher frequencies. Both panels are geographically aligned to demonstrate the spatial correlation between human activities and thermal anomalies across the studied seagrass habitats.
3.3 Conservation and management
We observed that most of the seagrass records are located outside protected areas or in areas with low levels of protection that lack management strategies and plans, such as Ramsar sites. While 13.2% of the records are found within protected areas (Figure 3). However, its presence and relevance are only recognized in the management plan of the Balandra Flora and Fauna Protection Area (DOF, 2012).
3.4 Current information and knowledge gaps
Our comprehensive review yielded 77 documents related to seagrasses in the Gulf of California, classified into eight categories. 42 of them were “Peer-reviewed” academic articles. The categories of “Books” and “Book Chapters” followed, with 11 and 6 records respectively. Since 1996, ten theses have been registered spanning bachelor’s, master’s, and doctoral degrees, although only three have been published as peer-reviewed papers. Despite relatively limited, we included two outreach papers and five reports of projects of research in our analysis.
Although research into seagrasses began more than 100 years ago, the rise of peer-reviewed publications occurred at the end of the 20th century, in the 1980s, when the peak of research in a decade was reached, with 12 of them produced between 1980 and 1988. However, from 1990 to 2021, this trend reversed, adding a total of 25 peer-reviewed publications in 31 years, i.e., a rate of 0.80, less than one publication per year. Despite this slowdown, starting in 2010, an important moment stands out in the production of research on seagrasses, with the increase in theses and reports, indicating a resurgence of interest in research and the continued study of seagrasses in the region (Figure 4A).
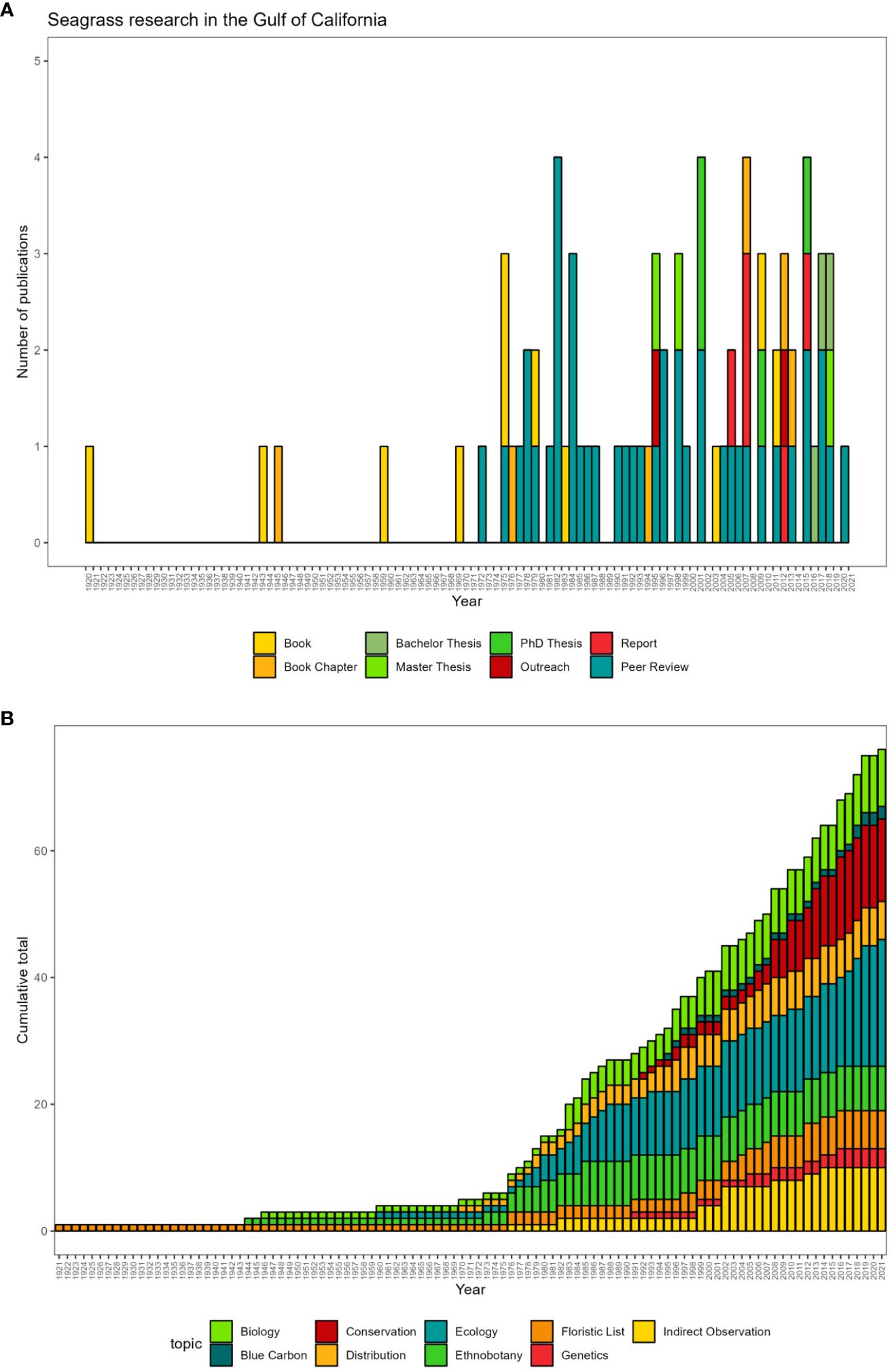
Figure 4 Seagrass research in the Gulf of California by (A) type of document and (B) research topics accumulated over the years.
During the first years, there is a trend towards taxonomic and ethnobotanical studies. This period is characterized by no increase in the number of studies until the 1970s when studies focused on the distribution of seagrasses emerge, and the first research mentioning them indirectly (Indirect observations) appears.
Ecology and conservation are the most studied topics, increased almost constantly since the first publication in 1977 and 1992, respectively. Both topics reaching a maximum number of studies in 2021. The last decade of the 20th century was an important time for research, as new lines of research emerged, such as genetics, blue carbon, in which it is necessary to redouble efforts (Figure 4B).
4 Discussion
4.1 Seagrass distribution
The mapping of seagrass habitats remains a significant challenge, despite recent advances in technology that promise to narrow the information gap. Even the wide extent of seagrasses in the Gulf of California, our validation data results only confirmed the presence of seagrasses at 13 out of the 28 historically noted localities (Datasheet 1). This indicates a potential decline of 46.4% from their known historical distribution, a concerning trend also reported by López-Calderón et al. (2016).
The seagrass meadows of the Canal del Infiernillo are the most important within the Gulf of California, due they cover the largest spatial area and appear to be the most consistent in their distribution over time. It extends between the Sonora coast and Tiburon Island, includes all bays and estuaries therein. The Canal del Infiernillo seagrass meadows, estimated at 7,000 hectares in 2010 (López-Calderón et al., 2016). We confirm the presence of seagrasses in the Canal del Infiernillo; however, we cannot know the species composition. Despite this, the meadows have shown remarkable stability and density, possibly due to Comcaac’s conservation efforts. This area is part of the Comcaac (Seri) community’s territory, known for its historical use of seagrasses, and has been under exclusive fishing concession since 1975 (DOF, 1975; Felger et al., 1980; Felger and Moser, 1985).
Bahía Concepción hosts the only population of Z. marina on the east coast of the Gulf of California. Documented on two beaches, El Requeson and Punta Arenas, these meadows cover roughly 33.74 hectares (López-Calderón et al., 2016). However, during our field work in January 2023, no Zostera marina was found, only Ruppia maritima was present. This finding may be due to the increase in temperatures in the region (Figure 2), due to Z. marina declines at temperatures above 25°C and disappears at 28°C, while R. maritima has a wide range of tolerance to temperature changes (Phillips and Meñez, 1988; Lazar and Dawes, 1991; Meling-Lopez and Ibarra-Obando, 1999; Santamaría-Gallegos et al., 2000). The ecological succession between both species had already been previously reported at the this and another sites, mainly in areas of lower environmental quality (Meling-Lopez and Ibarra-Obando, 1999; Cho et al., 2009; López-Calderón et al., 2010).
The status of seagrass meadows on the eastern Gulf of California coast is uncertain. We can confirm the presence of seagrasses in 13 sites. However, we do not know the composition of the seagrass species, since the key meadows are found predominantly within coastal lagoons, with high levels of eutrophication. For example, Bahía de Kino, where significant anthropogenic activities have been recorded, it is too difficult identified species using remote technologies.
Regarding the several new locations for H. wrightii and H. decipiens in La Paz (Supplementary Table 1), we think that changes in seagrass distribution can be attributed to the reproductive strategies and seasonal dynamics, linked to life cycles, which can result in meadows disappearing for years at a time, especially among annual species (Pérez-Estrada et al., 2021). H. wrightii, a perennial species, primarily propagates vegetatively (Santamaría-Gallegos, 2016; Pérez-Estrada et al, 2021). While H. decipiens, an annual species, germinates from seeds and is visible from April to October, declining in winter in most locations (Dawes and Lawrence, 1980; Dawes et al., 1995; Kuo and Kirkman, 1995; Kenworthy, 1999; Deis, 2000; Santamaría-Gallegos, 2016). These species form transient, scattered patches that are often underreported and difficult to detect due to algal overgrowth. Misidentification with macroalgae or other seagrasses is common, which may have contributed to the overestimation of H. decipiens in the Gulf of California’s marine flora records.
While documented changes in Z. marina meadows exist, other species lack comprehensive distribution data. For example, R. maritima was last officially reported in Los Cabos in the late 1990s (Ramírez-García and Lot, 1994; León de la Luz et al., 1997), with no subsequent sightings. Consequently, seagrass mapping and monitoring efforts should account for their dynamic nature. Regular long-term monitoring, as suggested by Valle et al. (2013), including both remote sensing and fieldwork over periods such as four years, could yield more accurate spatial distribution models for these populations (Bremner et al., 2023). It is crucial to conduct detailed fieldwork to revalidate species distributions and understand the ecological dynamics of seagrass meadows in the Gulf of California.
4.2 Summary of human and climate stressors
Coastal lagoons and estuaries along the Sonora and Sinaloa coastlines are subject to three major human-induced stressors: pollution, coastal modification, and overuse of biological resources Figure 2A. The environmental stress in these regions is largely attributed to population growth and the indirect effects of agricultural and livestock runoff (Montes et al., 2012; Páez-Osuna et al., 2017).
The primary environmental threat comes from agricultural pollutants like fertilizers and pesticides. These substances accumulate in sediments and water, posing risks to the health of ecosystems and leading to significant marine pollution (Carvalho et al., 1996; Galindo-Reyes et al., 1999; Páez-Osuna et al., 2017; Ruíz-Fernández et al., 2002). In Sonora and Sinaloa, the expansion of aquaculture has also been problematic. Related coastal alterations have substantially affected local hydrology and altered coastal processes. Organic discharges and nutrient outflow from aquaculture operations diminish water quality, which in turn adversely affects vegetation cover (Alonso-Pérez et al., 2003; González-Abraham et al., 2015), and may lead to succession events in vegetation, such as those observed between R. maritima and Z. marina (López-Calderón et al., 2010).
In Baja California Peninsula, coastal alterations and tourism are the primary threats to seagrass meadows (Figure 2A). In Los Cabos, tourism infrastructure has led to significant ecological strain, with the San José estuary’s geomorphology reduced by over 40% in 20 years, affecting its ecosystem services (Arizpe et al., 2018; Wurl, 2019). While, tourism development, combined with natural events like hurricanes and climate phenomena, has been detrimental, particularly to H. wrightii in La Paz Bay’s Balandra MPA (Pérez-Estrada et al., 2021).
Environmental stressors, such as pronounced warming and heatwave events, are higher in Loreto and Concepcion Bay (Figure 2B). The seagrass meadows of Bahía Concepción face a challenging environment due to their small size, human threats, nutrient discharges leading to eutrophication, and climate change impacts. Studies indicate a significant decrease in seed banks from 1995 to 2010 (Santamaría-Gallegos et al., 2000; López-Calderón et al., 2016).
The Midriff Islands Region, including Bahía de los Ángeles, Tiburon, and Ángel de la Guarda islands, faces less environmental pressure, making these sites potential climate refugia (Figure 2B). The cooler temperatures and upwelling events here present a research opportunity for understanding future climate impacts on seagrasses (López et al., 2006).
Despite some seagrasses being located within protected areas, their conservation is not guaranteed due to the highly disturbed and fragmented coastal landscape. As shown in Figure 3, few seagrass records fall within fully protected zones with restrictions on extractive activities. Most are in minimally protected or unprotected areas. If current pressures like habitat degradation and population reduction continue, some seagrass populations may risk extinction in the short to medium term. Immediate and proactive conservation measures are critical for seagrass recovery and preservation.
4.3 Conservation and management
Despite increased recognition of the importance of seagrass conservation, often linked with the protection of mangroves and reefs, a significant gap persists between scientific understanding and its integration into effective policy and conservation action (Duarte et al., 2008; López-Calderón et al., 2014; Fortes et al., 2018). For example, despite its recognition as a Priority Marine Area, granted in 1998 by CONABIO, USAID, WWF, FMCN and Packard Foundation (López-Calderón et al., 2016), Bahía Concepción lacks formal protection and a dedicated conservation strategy.
The complex interaction among resource management, public policy, and economic objectives in coastal regions creates a barrier to implementing laws that protect seagrass ecosystems. Developing management plans that safeguard key ecosystems while accommodating local economic interests is a delicate balance, often met with opposition to restrictions (Bennett and Dearden, 2014). Standard conservation strategies may not always be suitable for seagrass habitats due to their unique dynamics and fragility. For instance, the establishment of the Balandra Flora and Fauna Protection Area in November 2012 (DOF, 2012), which aimed to limit certain activities, paradoxically intensified tourism pressure, thereby potentially impacting the local H. wrightii population (Pérez-Estrada et al., 2021). This development highlights the insufficient consideration given to seagrass meadows within management plans. Consequently, despite numerous seagrass locations falling within designated protection areas (refer to Figure 3), there is no assurance that these habitats are effectively safeguarded.
Community engagement is crucial in conserving seagrass ecosystems, which are frequently undervalued and misunderstood. Successful conservation models have leveraged environmental education and citizen science to involve coastal communities in monitoring seagrass populations (Cunha et al., 2013; Yaakub et al., 2014). Identifying and safeguarding potential threat zones, creating micro-protected areas, and conducting water quality assessments could provide vital sanctuaries for seagrass recovery and scientific study. A multidisciplinary approach is essential for seagrass conservation, one that addresses the ecological, societal, and economic facets of these critical habitats.
4.4 Current information and knowledge gaps
Since 1980, the number of peer-reviewed publications and the increase in theses and reports indicate a resurgence of interest in seagrass research, led mainly by local researchers (Figure 4A). However, there are still notable gaps in knowledge.
Seagrasses have been documented since 1645, documenting the cultural and ethnobotanical uses of these plants (Felger and Moser, 1973; Felger, 1976; Felger and Lowe, 1976; Sheridan and Felger, 1977; Valencia et al., 1985; Felger et al., 2013). This type of research has been published as ethnobotanical studies and floristic lists that also provide information on distribution (Den Hartog, 1970; McMillan and Phillips, 1979b; Wilder et al., 2007). On the other hand, in the 1960s emerged biological and ecological studies (Figure 4B). The first topic describes mainly phenological and reproductive aspects of seagrasses (Dawson, 1944; Setchell, 1946; Dawson, 1960; Edwards, 1978; McMillan and Phillips, 1979a; McMillan, 1983; Phillips and Backman, 1983; Phillips et al., 1983).
Meanwhile the ecological issues explore interactions with other species, including algae, fishes, invertebrates (Phillips and McRoy, 1980; Paul & Bowers, 1982; Oliva-Martinez and Ortega, 1983; Phillips, 1984; Ortega et al., 1986; Ezcurra et al., 1988; Flores-Verdugo et al., 1988; Ibarra-Obando and Ríos, 1993). Other aspects addressed in ecological studies include biomass quantification, population changes, and energy flow models (Sánchez-Lizaso and Riosmena Rodríguez, 1997; Torre-Cosio, 2002; López-Calderón et al., 2010, López-Calderón et al., 2014; Pérez-Estrada et al., 2021; Van Dam et al., 2021).
New critical research themes, as conservation and genetics, emerged from the 1990s, providing assessment of biodiversity threats and conservation status of the ecosystem (Glenn et al., 1992; Brusca et al., 2005; Basurto, 2008; Morzaira-Luna and Daneman, 2018; Riosmena-Rodríguez et al., 2013; López-Calderón et al., 2016). Including the evaluation of variability, resilience, and connectivity of Z. marina populations on the Gulf of California and the Pacific coast (Backman, 1991; Muñíz-Salazar et al., 2005; Talbot et al., 2016) (Figure 4B).
Despite this broad spectrum of research, there exist certain areas of study that remain under-researched, representing significant knowledge gaps. For instance, seagrass distribution has been examined since the initial records on the coasts of the Gulf of California (Den Hartog, 1970; McMillan and Phillips, 1979a; Aguilar-Rosas and López-Ruelas, 1985; Ibarra-Obando and Ríos, 1993; Riosmena-Rodríguez and Sánchez-Lizaso, 1996; Santamaría-Gallegos et al., 2006). However, the depth of information varies greatly: some reports merely document species presence, while others include meadow sizes. Estimations of meadow extent are seldom reported, with the only data originating from Canal del Infiernillo, Bahía Concepción and Bahía Balandra (Torre-Cosio, 2002; Hinojosa-Arango et al., 2014; López-Calderón et al., 2014, López-Calderón et al., 2016; Pérez-Estrada et al., 2021).
The subject of blue carbon in seagrass, though well-studied globally (Lavery et al., 2013; Oreska et al., 2017; Friess, 2023), is lacking in the Gulf of California, with only two studies providing information (Flores-Verdugo et al., 1995; Herrera-Silveira et al., 2018). Lastly, fishing and aquaculture research indirectly offer information on seagrass distribution (Paul and Bowers, 1982; Santamaría-Gallegos et al., 1999; Zetina-Rejón, 1999; Bourillón-Moreno, 2002).
4.4.1 Research by species
The species Z. marina has been the primary focus of most of these studies since its initial documentation in 1645 (Figure 4). It has been the subject of at least 44 individual studies (López-Calderón et al., 2010; Riosmena-Rodríguez et al., 2013; López-Calderón et al., 2016), and its presence has been noted in 15 distinct locations (Supplementary Table 2). It is an annual species, which germinates during the fall, reaching its greatest abundance in the winter, mainly when the water temperature ranges between 17-19°C. About 70% of the species’ records have been documented during the fall-winter season. Its decline begins with increasing temperatures, disappearing almost completely during the summer (Phillips and Backman, 1983; Phillips and McRoy, 1980; Santamaría-Gallegos et al., 2006).
Data on the expanse of Z. marina meadows, however, is limited and only available for Canal del Infiernillo and Bahía Concepción (Torre-Cosio, 2002; López-Calderón et al., 2016). However, in recent years, Z. marina has not been reported in Bahía Concepción, which can be considered an indicator that the species is at risk, at least in that region. In the Canal del Infiernillo, the populations remain apparently stable and without human stressors (Figure 2) under the management of the Seri culture. These populations maintain genetic connectivity between Bahía Concepción and may be the key to rescuing the populations of the Baja California peninsula (Muñíz-Salazar et al., 2005) through seagrass dispersal aided by water mass exchanges between these key Gulf locations (López-Calderón et al., 2008).
Ruppia maritima, with reports of its presence in 23 different localities (Supplementary Table 2), is the most broadly distributed species within the Gulf of California. Though frequently studied within wetland contexts, R. maritima is often overlooked as a seagrass species (Oliveira et al., 1983; Kuo and den Hartog, 2001). It is a very resistant species that tolerates wide ranges of temperature and salinity; it is commonly present in areas of lower environmental quality (Cho et al., 2009; López-Calderón et al., 2010).
This species is mentioned in 29 studies, either directly or indirectly, however information on its distribution, abundance, or biomass estimation is provided in a mere six studies (Edwards, 1978; Oliva-Martinez and Ortega, 1983; Flores-Verdugo et al., 1988; López-Calderón et al., 2010, López-Calderón et al., 2014; García-Trasviña, 2017). Its rising prominence in recent publications due to increasing biomass, spatial distribution, and significance as a food source for green turtles, is noteworthy (López-Calderón et al., 2010, López-Calderón et al., 2014).
In this study we recorded R. maritima in four new locations in the municipality of Loreto and one more in Mulegé (Supplementary Table 1). In El Juncalito and Puerto Escondido beaches the plants were short (less than 10 cm in height), lacked reproductive structures and showed low density. Green algae of the genus Caulerpa were mixed with R. maritima. Respect to the records of the estuaries, the R. maritima populations were associated to other kinds of vegetation (marshes and mangroves in the estuaries of Loreto and palm trees in Mulegé). All of them presented conditions of isolation, however, they are threatened by human activities. This species remains the only seagrass species in Mexico that is not included in NOM-059.
The species H. wrightii was initially documented in the Canal del Infiernillo in 1979 and has since been reported in seven localities (Supplementary Table 1). Eight studies focused on the species are reported. Preliminary studies concentrated on the presence of this species within the Gulf of California (McMillan and Phillips, 1979b; Aguilar-Rosas and López-Ruelas, 1985; Ramírez-García and Lot, 1994). However, since 2010, new studies have emerged that focus on the seasonal characteristics of H. wrightii, including evaluations of the abundance and morphology of its shoots, as well as biomass quantification (Santamaría-Gallegos, 2016; García-Trasviña, 2017). Notably, Campos-Dávila et al. (2019) and Pérez-Estrada et al. (2021, 2023) have provided descriptions of the species’ phenology, physiological traits, and fauna associated with H. wrightii meadows.
We contribute with new information to the knowledge of the species, reporting new sites within Bahía de La Paz where it had not been reported before (Supplementary Table 1). These new records, indicates an expansion of its distribution range, probably favored by its tolerance to changes in temperature and salinity (Phillips, 1960; Mazzotti et al., 2007). Despite this new information, a decrease in the extent of the patches has also been observed. For example, in Balandra Bay, a reduction of 70% is estimated between 2013 and the present (Pérez-Estrada et al., 2021).
Finally, H. decipiens has been documented exclusively in Bahía de La Paz, including in Ensenada de La Paz and in estuaries on the West side of Isla Espírito Santo (Supplementary Datasheet 3). The three existing studies on this species have described the seasonal variation in its life cycle, its characterization, and biomass quantification (Santamaría-Gallegos et al., 2006; Santamaría-Gallegos, 2016; García-Trasviña, 2017). As far as we know, it remains a species restricted to Bahía de La Paz. However, although in the past it had been reported exclusively on Costa Baja beach (Santamaría-Gallegos et al., 2006), this work adds new records (Supplementary Table 1). Indicating, as in the case of H. wrightii, an expansion in its distribution range, probably driven by its tropical affinity (Santamaría-Gallegos et al., 2006).
5 Conclusions
This review represents an initial comprehensive assessment of the conservation status and knowledge of seagrass species in the Gulf of California, pointing to a significant lag in research in the region and the need to incentivize investment by government institutions. To address knowledge gaps and advance conservation, the following measures are recommended:
● Formation of a collaborative network among seagrass ecosystem researchers to foster data sharing and joint studies.
● Regular updates on mapping the distribution and area of seagrass species, particularly within MPAs, utilizing a combination of remote sensing and fieldwork, including area estimation and use of quadrats for sampling. The differentiation between seagrass patches and meadows must be clear and consistent.
● Implementation of environmental programs to improve assessment of ecosystem services, with a focus on key environmental indicators like temperature, water quality, nutrient levels, and heavy metal contents to gauge ecosystem health.
● Detailed investigation into the phenological and reproductive patterns of seagrass species in the Gulf to comprehend their transient nature, complemented by genetic research to understand population connectivity and gene flow.
● Assessment of seagrass contributions to fisheries, including studies on fauna-associated dispersal and the effects of atmospheric phenomena on these ecosystems.
● In-depth exploration of biogeochemical cycling, carbon storage, and sequestration within seagrass habitats to understand their role in climate mitigation.
● Implementation of environmental educations program that raises awareness among the population about the importance of seagrasses. Forums and workshops can be tools that activate participation through citizen science.
Author contributions
MR-Z: Conceptualization, Data curation, Formal analysis, Investigation, Methodology, Software, Writing – original draft, Writing – review & editing. CP-E: Investigation, Methodology, Resources, Validation, Writing – review & editing. JL-C: Data curation, Investigation, Resources, Writing – review & editing. AC: Data curation, Resources, Writing – review & editing. SV: Data curation, Resources, Validation, Writing – review & editing. FF: Conceptualization, Funding acquisition, Investigation, Project administration, Resources, Supervision, Validation, Writing – original draft, Writing – review & editing.
Funding
The author(s) declare that no financial support was received for the research, authorship, and/or publication of this article.
Acknowledgments
MRZ and FF thank the support of the Institute of the Americas, in particular, Richard Kiy, Tania Miranda and Catalina López Sagastegui.
Conflict of interest
The authors declare that the research was conducted in the absence of any commercial or financial relationships that could be construed as a potential conflict of interest.
Publisher’s note
All claims expressed in this article are solely those of the authors and do not necessarily represent those of their affiliated organizations, or those of the publisher, the editors and the reviewers. Any product that may be evaluated in this article, or claim that may be made by its manufacturer, is not guaranteed or endorsed by the publisher.
Supplementary material
The Supplementary Material for this article can be found online at: https://www.frontiersin.org/articles/10.3389/fmars.2024.1402044/full#supplementary-material
References
Aguilar-Rosas R., López-Ruelas J. (1985). Halodule wrightii aschers (Potamogetonales: cymodoceae) in Topolobampo Bay, Sinaloa, Mexico. Cienc. Mar. 11, 87–91. doi: 10.7773/cm.v11i2.463
Alonso-Pérez F., Ruiz-Luna A., Turner J., Berlanga-Robles C. A., Mitchelson-Jacob G. (2003). Land cover changes and impact of shrimp aquaculture on the landscape in the Ceuta coastal lagoon system, Sinaloa, Mexico. Ocean Coast. Manage. 46, 583–600. doi: 10.1016/S0964-5691(03)00036-X
Álvarez-Borrego S. (1983). “Gulf of California,” in Estuaries and Enclosed Seas. Ecosystems of the World. Ed. Ketchum B. H. (New York: Elsevier Scientific Press), 427–449.
Arizpe O., Juarez J., Cruz P., Torres A. (2018). Evolution of a coastal oasis in a high population growth rate municipality: Los Cabos, Mexico. Sustainability City 13, 605–613. doi: 10.2495/SDP
Backman T. W. (1991). Genotypic and phenotypic variability of Zostera marina on the west coast of North America. Can. J. Bot. 69, 1361–1371. doi: 10.1139/b91-176
Basurto X. (2008). Biological and ecological mechanisms supporting marine self-governance: the Seri Callo de Hacha fishery in Mexico. Ecol. Soc 13, 20. doi: 10.5751/ES-02587-130220
Bennett N. J., Dearden P. (2014). Why local people do not support conservation: Community perceptions of marine protected area livelihood impacts, governance and management in Thailand. Mar. Policy. 44, 107–116. doi: 10.1016/j.marpol.2013.08.017
Bourillón-Moreno L. (2002). Exclusive fishing zone as a strategy for managing fishery resources by the re Indians, Gulf of California, Mexico. The University of Arizona, Arizona (AZ.
Bremner J., Petus C., Dolphin T., Hawes J., Beguet B., Devlin M. J. (2023). A seagrass mapping toolbox for South Pacific environments. Remote Sens. 15, 834. doi: 10.3390/rs15030834
Brodie G., de Ramon N’yeurt A. (2018). Effects of Climate Change on Seagrasses and Seagrass Habitats Relevant to the Pacific Islands. Pacific marine climate change report card. Sci. Rev., 112–131.
Brusca R. C. (2010). The Gulf of California: biodiversity and conservation (Arizona: University of Arizona Press). doi: 10.2307/j.ctt181hwrc
Brusca R. C., Findley L. T., Hastings P. A., Hendrickx M. E., Cosio J. T., van der Heiden A. M. (2005). “Macrofaunal diversity in the gulf of California,” in Biodiversity, ecosystems, and conservation in Northern Mexico. Eds. Cartron J. L. E., Felger R. S. (Oxford University Press, New York), 179–202.
Campos-Dávila L., Pérez-Estrada C. J., Rodríguez-Estrella R., Morales-Bojórquez E., Brun-Murillo F. G., Balart E. F. (2019). Seagrass Halodule wrightii as a new habitat for the amphioxus Branchiostoma californiense (Cephalochordata, Branchiostomidae) in the southern Gulf of California, Mexico. Zookeys 873, 113–131. doi: 10.3897/zookeys.873.33901
Carvalho F. P., Fowler S. W., Gonzalez-Farias F., Mee L. D., Readman J. W. (1996). Agrochemical residues in the Altata-Ensenada del Pabellon coastal lagoon (Sinaloa, Mexico): A need for integrated coastal zone management. Int. J. Environ. Health Res. 6, 209–220. doi: 10.1080/09603129609356892
Cho H. J., Biber P., Nica C. (2009). “The rise of Ruppia in seagrass beds: changes in coastal environment and research needs,” in Handbook on Environmental Quality. Eds. Drury E. K., Pridgen T. S. (Nova Science Pub Inc, UK), 1–15.
Comisión Nacional de Áreas Naturales Protegidas (2021) Áreas Naturales Protegidas Federales de la República Mexicana. Available online at: file:///C:/Users/diana.leyja/AppData/Local/Temp/arc61CF/tmpCA1D.tmp.htm8/10/2018.
Costanza R., d’Arge R., de Groot R., Farber S., Grasso M., Hannon B., et al. (1997). The value of the world’s services and natural capital. Nature 387, 253–260. doi: 10.1038/387253a0
Cunha A. H., Assis J. F., Serrão E. A. (2013). Seagrasses in Portugal: A most endangered marine habitat. Aquat. Bot. 104, 193–203. doi: 10.1016/j.aquabot.2011.08.007
Dawes C. J., Hanisak D., Kenworthy W. J. (1995). Seagrass biodiversity in the Indian River Lagoon. Bull. Mar. Sci. 57, 59–66.
Dawes C. J., Lawrence J. M. (1980). Seasonal changes in the proximate constituents of the seagrasses Thalassia testudinum, Halodule wrightii. and Syringodium filiforme. Aquat. Bot. 8, 371–380. doi: 10.1016/0304-3770(80)90066-2
Dawson E. Y. (1944). The marine algae of the Gulf of California. Allan Hancock pacific expeditions 3, 189–452.
Dawson E. Y. (1960). A review of the ecology, distribution, and affinities of the benthic flora. Systematic Zoology 9, 93–100. doi: 10.2307/2411958
Deis D. R. (2000). “Monitoring the effects of construction and operation of marina of the seagrass Halophila decipiens in Fort Lauderdale, Florida,” in Seagrasses: Monitoring ecology, physiology, and management. Ed. Bortone S. A. (CRC Press, Boca Ratón. FL), 147–155.
Diario Oficial de la Federación (1975) Decreto por el que se declara que única y exclusivamente los miembros de la tribu Seri y los de la Sociedad Cooperativa de Producción Pesquera S. C. L., podrán realizar actos de pesca en las aguas de los esteros y bahías, situados en el Golfo de California y de los litorales que forman la Isla del Tiburón localizada en el Mar de Cortés. Available online at: https://dof.gob.mx/nota_detalle.php?codigo=4749758andfecha=11/02/1975#gsc.tab=0.
Diario Oficial de la Federación (2012) Decreto por el que se declara área natural protegida, con el carácter de Área de Protección de Flora y Fauna, la región conocida como Balandra, localizada en el municipio La Paz, en el Estado de Baja California Sur, misma que cuenta con una superficie total de 2,512-73-07,50 hectáreas. Available online at: https://www.dof.gob.mx/nota_detalle.php?codigo=5413569andfecha=29/10/2015#gsc.tab=0.
Diario Oficial de la Federación (2019) orma Oficial Mexicana NOM-059-SEMARNAT-2010: Protección ambiental-especies nativas de México de flora y fauna silvestres-categorías de riesgo y especificaciones para su inclusión, exclusión o cambio-lista de especies en. Available online at: https://www.dof.gob.mx/nota_detalle.php?codigo=5578808andfecha=14%2F11%2F2019#gsc.tab=0.
Duarte C. M., Dennison W. C., Orth R. J. W., Carruthers T. J. B. (2008). The charisma of coastal ecosystems: addressing the imbalance. Estuar. Coast. 31, 233–238. doi: 10.1007/s12237-008-9038-7
Dunic J. C., Brown C. J., Connolly R. M., Turschwell M. P., Côté I. M. (2021). Long-term declines and recovery of meadow area across the world’s seagrass bioregions. Glob. Change Biol. 27, 4096–4109. doi: 10.1111/gcb.15684
Edwards R. R. C. (1978). Ecology of a coastal lagoon complex in Mexico. Estuar. Coast. Mar. Sci. 6, 75–92. doi: 10.1016/0302-3524(78)90043-9
Ezcurra E., Felger R. S., Rusell A. D., Equihua M. (1988). Freshwater Islands in a Desert Sand Sea: The hydrology, flora, and phytogeography of the Gran Desierto Oases of Northwestern Mexico. Desert Plants. 9, 35–63.
Favoretto F., Sánchez C., Aburto-Oropeza O. (2022). Warming and marine heatwaves tropicalize rocky reefs communities in the Gulf of California. Prog. Oceanogr. 206, 102838. doi: 10.1016/j.pocean.2022.102838
Felger R. S. (1976). The Gulf of California: an ethno-ecological perspective. Nat. Resour. J. 16, 451–464. https://www.jstor.org/stable/24881037
Felger R. S., Lowe C. H. (1976). “The island and coastal vegetation and flora of the northern part of the Gulf of California,” in Gulf of California Vegetation and Flora (Los Angeles, CA: Natural History Museum of Los Angeles), 1–59. doi: 10.5962/p.241254
Felger R., Moser M. B. (1973). Eelgrass (Zostera marina L.) in the Gulf of California: Discovery of its nutritional value by the Seri Indians. Science 181, 355–356. doi: 10.1126/science.181.4097.355
Felger R. S., Moser M. B. (1985). People of the desert and sea, Ethnobotany of the Seri Indians (Tucson: University of Arizona Press). doi: 10.2307/j.ctv1n6pvq5
Felger R. S., Moser M. B., Moser E. W. (1980). “Seagrasses in Seri Indian culture,” in Handbook of seagrass biology: an ecosystem perspective (Garland STPM Press, New York).
Felger R. S., Wilder B. T., Romero-Morales H. (2013). Plant life of a Desert Archipelago: Flora of the Sonoran Islands in the Gulf of California (Arizona: University of Arizona Press).
Flores-Verdugo F. J., Briceño-Dueñas R., González-Farias F., Calvario-Martínez O. (1995). “Balance de Carbono en un ecosistema lagunar-estuarino de boca efímera en la Costa Noroccidental de México (Estero El Verde, Sinaloa),” in Temas de Oceanografía Biológica en México Vol II. Eds. Rosa-Vélez J. d. l., González-Farias F. F. (UABC Press, Ensenada), 137–160.
Flores-Verdugo F., Day J. W., Mee L., Brisei R. (1988). Phytoplankton production and seasonal biomass variation of seagrass, Ruppia maritima L., in a tropical Mexican lagoon with an ephemeral inlet. Estuar 11, 51–56. doi: 10.2307/1351717
Fortes M. D., Ooi J. L. S., Tan Y. M., Prathep A., Bujang J. S., Yaakub S. M. (2018). Seagrass in Southeast Asia: A review of status and knowledge gaps, and a road map for conservation. In Bot. Mar. 61, 269–288. doi: 10.1515/bot-2018-0008
Friess D. A. (2023). The potential for mangrove and seagrass blue carbon in Small Island States. Curr. Opin. Environ. Sustainability 64, 101324. doi: 10.1016/j.cosust.2023.101324
Galindo-Reyes J. G., Fossato V. U., Villagrana-Lizarraga C., Dolci F. (1999). Pesticides in water, sediments, and shrimp from a coastal lagoon off the Gulf of California. Mar. pollut. Bull. 38, 837–841. doi: 10.1016/S0025-326X(99)00086-7
García-Trasviña J. A. (2017). Caracterización y cuantificación de biomasa de tres especies de pastos marinos en Punta Roca caimancito, Baja California Sur. Universidad Autónoma de Baja California Sur, La Paz, (BCS.
Glenn E. P., Felger R. S., Burquez A., Turner D. S. (1992). Ciénega de Santa Clara: Endangered wetland in the Colorado river delta, Sonora, Mexico. Nat. Resour. 32, 817–824. https://www.jstor.org/stable/24884507
González-Abraham C., Ezcurra E., Garcillán P. P., Ortega-Rubio A., Kolb M., Bezaury Creel J. E. (2015). The human footprint in Mexico: physical geography and historical legacies. PloS One 10, e0121203. doi: 10.1371/journal.pone.0121203
Herrera-Silveira J. A., Mendoza-Martínez J. A., Morales-Ojeda S. M., Camacho-Rico A., Medina-Gómez I., Ramírez-Ramírez J., et al. (2018). Database of carbon stocks in seagrasses of Mexico. Elementos para Políticas Públicas 2, 45–52. doi: 10.7717/peerj.8790
Hinojosa-Arango G., Rioja-Nieto R., Suárez-Castillo Á.N., Riosmena-Rodríguez R. (2014). Using GIS methods to evaluate rhodolith and sargassum beds as critical habitats for commercially important marine species in Bahía Concepción, B.C.S., México. Cryptogam. Algol. 35, 49–65. doi: 10.7872/crya.v35.iss1.2014.49
Hobday A. J., Alexander L. v., Perkins S. E., Smale D. A., Straub S. C., Oliver E. C. J., et al. (2016). A hierarchical approach to defining marine heatwaves. Prog. Oceanogr. 141, 227–238. doi: 10.1016/j.pocean.2015.12.014
Ibarra-Obando S. E., Ríos R. (1993). “Ecosistema de Fanerógamas marinas,” in Biodiversidad Marina y Costera. Eds. Salazar-Vallejos y S. I., González N. E. (CONABIO CIQRO, México), 54–65.
Kenworthy W. J. (1999). The role of sexual reproduction in maintaining populations of Halophila decipiens: implications for the biodiversity and conservation of tropical seagrass ecosystems. Pac. Conserv. Biol. 5, 260–268. doi: 10.1071/PC000260
Kuo J., den Hartog C. (2001). “Seagrass taxonomy and identification key,” in Global Seagrass Research Methods. Eds. Short F. T., Coles R. G. (Elsevier, Amsterdam), 31–58. doi: 10.1016/B978-044450891-1/50003-7
Kuo J., Kirkman H. (1995). Halophila decipiens Ostenfeld in estuaries of southwestern Australia. Aquat. Bot. 51, 335–340. doi: 10.1016/0304-3770(95)00468-F
Lavery P. S., Mateo M.Á., Serrano O., Rozaimi M. (2013). Variability in the carbon storage of seagrass habitats and its implications for global estimates of blue carbon ecosystem service. PloS One 8, e73748. doi: 10.1371/journal.pone.0073748
Lazar A. C., Dawes C. J. (1991). A seasonal study of the seagrass Ruppia maritima L. in Tampa Bay, Florida. Organic constituents and tolerances to salinity and temperature. Bot. Mar. 34, 265–269. doi: 10.1515/botm.1991.34.3.265
León de la Luz J. L., Domínguez-Cadena R., Domínguez-León M., Navarro-Pérez J. J. (1997). Floristic composition of the San José del Cabo oasis, Baja California Sur. SIDA Contrib. Bot. 17, 599–614. https://www.jstor.org/stable/41967252
López M., Candela J., Argote M. L. (2006). Why does the Ballenas Channel have the coldest SST in the Gulf of California? Geophys. Res. Lett. 33. doi: 10.1029/2006GL025908
López-Calderón J., Martínez A., González-Silvera A., Santamaría del Ángel E., Millán-Núñez E. (2008). Mesoscale eddies and wind variability in the northern Gulf of California. Geophys Res 113, C10001. doi: 10.1029/2007JC004630
López-Calderón J. M., Riosmena-Rodríguez R., Rodríguez-Baron J. M., Carrión-Cortez J., Torre J., Meling-López A., et al. (2010). Outstanding appearance of Ruppia maritima along Baja California Sur, Mexico and its influence in trophic networks. Mar. Biodivers. 40, 293–300. doi: 10.1007/s12526-010-0050-3
López-Calderón J. M., Riosmena-Rodríguez R., Rodriguez-Barrón J. M., Hinojosa-Arango G. (2014). “La planta acuática Ruppia maritima en el noroeste de México: Aumento de su presencia y efectos en la cadena trófica,” in Especies invasoras acuáticas: casos de estudio en ecosistemas de México. Eds. Low-Pfeng A. M., Quijón P. A., Peters-Recagno E. M. (Secretaría de Medio Ambiente y Recursos Naturales (Semarnat, México), 471–492.
López-Calderón J. M., Riosmena-Rodríguez R., Torre J., Meling-López A., Basurto X. (2016). Zostera marina meadows from the Gulf of California: conservation status. Biodivers. Conserv. 25, 261–273. doi: 10.1007/s10531-016-1045-6
Luch-Cota S. E., Tripp-Valdez M., Lluch-Cota D. B., Lluch-Belda D., Verbesselt J., Herrera-Cervantes H., et al. (2013). Recent trends in sea surface temperature off Mexico. Atmósfera 26, 537–546. doi: 10.1016/S0187-6236(13)71094-4
Mazzotti F. J., Pearlstine L. G., Chamberlain R., Barnes T., Chartier K., DeAngelis D. (2007). “Stressor Response Models for Seagrasses, Halodule wrightii and Thalassia testudnium,” in JEM Technical Report. Final Report to the South Florida water Management District and the U.S. Geological Survey (University of Florida, Florida Lauderdale Research and Education Center, Fort Lauderdale, Florida), 19.
McKenzie L. J., Nordlund L. M., Jones B. L., Cullen-Unsworth L. C., Roelfsema C., Unsworth R. K. F. (2020). The global distribution of seagrass meadows. Environ. Res. Lett. 15, 074041. doi: 10.1088/1748-9326/ab7d06
McMillan C. (1983). Seed germination for an annual form of Zostera marina from the Sea of Cortez, Mexico. Aquat. Bot. 16, 105–110. doi: 10.1016/0304-3770(83)90055-4
McMillan C., Phillips R. C. (1979a). Differentiation in habitat response among populations of New World seagrass. Aquat. Bot. 7, 185–196. doi: 10.1016/0304-3770(79)90021-4
McMillan C., Phillips R. C. (1979b). Halodule wrightii aschers. in the sea of Cortez, Mexico. Aquat. Bot. 6, 393–396. doi: 10.1016/0304-3770(79)90077-9
Meling-Lopez A. E., Ibarra-Obando S. E. (1999). Annual life cycles of two Zostera marina L. populations in the Gulf of California: contrasts in seasonality and reproductive effort. Aquat. Bot. 65, 59–69. doi: 10.1016/S0304-3770(99)00031-5
Montes A. M., González-Farias F. A., Botello A. V. (2012). Pollution by organochlorine pesticides in Navachiste-Macapule, Sinaloa, Mexico. Environ. Monit. Assess. 184, 1359–1369. doi: 10.1007/s10661-011-2046-2
Morzaira-Luna H., Daneman G. (2018). “Humedales,” in Bahía de Los Ángeles: recursos naturales y comunidad. Línea base 2007. Eds. Danemann G. D., Ezcurra E. (Instituto Nacional de Ecología, México), 243–289.
Muñíz-Salazar R., Talbot S. L., Sage G. K., Ward D. H., Cabello-Pasini A. (2005). Population genetic structure of annual and perennial populations of Zostera marina L. along the Pacific coast of Baja California and the Gulf of California. Mol. Ecol. 14, 711–722. doi: 10.1111/j.1365-294X.2005.02454.x
Nevárez-Martínez M. O., Lluch-Belda D., Cisneros-Mata M. A., Pablo Santos-Molina J., de los Angeles Martínez-Zavala M., Lluch-Cota S. E. (2001). Distribution and abundance of the Pacific sardine (Sardinops sagax) in the Gulf of California and their relation with the environment. Prog. Oceanogr. 49, 565–580. doi: 10.1016/S0079-6611(01)00041-6
Oliva-Martinez G., Ortega M. M. (1983). Estudio preliminar de la vegetación sumergida en la Laguna Caimanero y marisma de Huizache, Sinaloa. An. Inst. Biol. Mex. 54, 113–151.
Oliveira F., Pirani E. C., José R., Giulietti A. M. (1983). The Brazilian seagrasses. Aquat. Bot. 16, 251–267. doi: 10.1016/0304-3770(83)90036-0
Ondiviela B., Losada I. J., Lara J. L., Maza M., Galván C., Bouma T. J., et al. (2014). The role of seagrasses in coastal protection in a changing climate. Coas. Eng. 87, 158–168. doi: 10.1016/j.coastaleng.2013.11.005
Oreska M. P. J., McGlathery K. J., Porter J. H. (2017). Seagrass blue carbon spatial patterns at the meadow-scale. PloS One 12, e0176630. doi: 10.1371/journal.pone.0176630
Ortega M. M., Ruiz-Cardenas J., Oliva-Martinez M. G. (1986). La vegetación sumergida en la Laguna Agiabampo. An. Inst. Biol. Mex. 57, 59–108.
Orth R. J., Carruthers T. J., Dennison W., Duarte C. M. (2006). A global crisis for seagrass ecosystems. Bioscience 56, 987–996. doi: 10.1641/0006-3568(2006)56[987:AGCFSE]2.0.CO;2
Páez-Osuna F., Álvarez-Borrego S., Ruiz-Fernández A. C., García-Hernández J., Jara-Marini M. E., Bergés-Tiznado M. E., et al. (2017). Environmental status of the Gulf of California: a pollution review. Earth-Science Rev. 166, 181–205. doi: 10.1016/j.earscirev.2017.01.014
Páez-Osuna F., Sánchez-Cabeza J. A., Ruiz-Fernández A. C., Alonso-Rodríguez R., Piñón-Gimate A., Cardoso-Mohedano J. G., et al. (2016). Environmental status of the Gulf of California: A review of responses to climate change and climate variability. Earth-Sci. Rev. 162, 253–268. doi: 10.1016/j.earscirev.2016.09.015
Paul R. K. G., Bowers A. B. (1982). The ecology and Penaeus fishery of a coastal lagoon system in West Mexico. In Oceanol. Acta 383-, 388.
Pérez-Estrada C. J., Falcón-Brindis A., Rodríguez-Estrella R., Morales-Bojórquez E., Crespo-Domínguez J. M., Brun-Murillo F. G. (2021). Seasonal shifts in morphology, physiology and population traits in the seagrass Halodule wrightii (Cymodoceaceae) in a subtropical arid area. Aquat. Bot. 172, 103381. doi: 10.1016/j.aquabot.2021.103381
Pérez-Estrada C. J., Rodríguez-Estrella R., Brun-Murillo F. G., Gurgo-Salice P., Valles-Jiménez R., Morales-Bojórquez E., et al. (2023). Diversity and seasonal variation of the molluscan community associated with the seagrass Halodule wrightii in a marine protected area in the southern Gulf of California. Aquat. Ecol., 1–21. doi: 10.1007/s10452-023-10011-3
Phillips R. C. (1960). Observations on the Ecology and Distribution of the Florida seagrasses. Prof. Pap. Ser. Fla. Board Conserv. (2), 1–72.
Phillips R. C. (1984). The Ecology of Eelgrass Meadows in the Pacific Northwest: A Community Profile (U.S: Fish Wildl.Serv).
Phillips R. C., Backman T. W. (1983). Phenology and reproductive biology of eelgrass (Zostera marina L.) at Bahia Kino, Sea of Cortez, Mexico. Aquat. Bot. 17, 85–90. doi: 10.1016/0304-3770(83)90020-7
Phillips R. C., Grant W. S., McRoy C. P. (1983). Reproductive Strategies of Eelgrass (Zostera marina L.). Aquat. Bot. 16, 1–20. doi: 10.1016/0304-3770(83)90047-5
Phillips R. C., McRoy C. P. (1980). Handbook of seagrass biology: an ecosystem perspective (New York: Garland STPM).
Phillips R. C., Meñez E. G. (1988). Seagrasses. Smithson. Contrib. Mar. Sci. 34, 1–104. doi: 10.5479/si.01960768.34
Ramírez-García P., Lot A. (1994). La distribución del manglar y los pastos marinos en el Golfo de California, México. An. Inst. Biol. Mex. 65, 63–72.
Riosmena-Rodríguez R., Muñiz-Salazar R., López-Calderón J. M., Torre J. (2013). “Conservation status of Zostera marina populations at Mexican Pacific,” in Advances in Environmental Research. Ed. Daniels J. A. (Hauppauge, NY: Nova Science Pub Inc), 35–63.
Riosmena-Rodríguez R., Sánchez-Lizaso J. L. (1996). El lımite sur de distribución de Zostera marina L. y Phyllospadix torreyi Watson para el noroeste mexicano. Oceánides 11, 45–48.
Ruíz-Fernández A. C., Hillaire-Marcel C., Ghaleb B., Soto-Jiménez M., Páez-Osuna F. (2002). Recent sedimentary history of anthropogenic impacts on the Culiacan River Estuary, northwestern Mexico: geochemical evidence from organic matter and nutrients. Environ. pollut. 118, 365–377. doi: 10.1016/S0269-7491(01)00287-1
Sánchez-Cabeza J.-A., Herrera-Becerril C. A., Carballo J. L., Yáñez B., Álvarez-Sánchez L. F., Cardoso-Mohedano J.-G., et al. (2022). Rapid surface water warming and impact of the recent, (2013–2016) temperature anomaly in shallow coastal waters at the eastern entrance of the Gulf of California. Prog. Oceanogr. 202, 102746. doi: 10.1016/j.pocean.2022.102746
Sánchez-Lizaso J. L., Riosmena Rodríguez R. (1997). Macroalgas epífitas de Zostera marina L. en Bahía Concepción (México: BCS).
Santamaría-Gallegos N. A. (2016). Estudio de las praderas de fanerógamas marinas en Baja California Sur (México): Ciclo de vida y estrategias reproductivas de Zostera marina, Halodule wrightii y Halophila decipiens. Universidad de Alicante, Alicante, (AL.
Santamaría-Gallegos N. A., Félix Pico E., Sánchez Lizaso J. L., Palomares García R. (1999). Temporal coincidence of the annual eelgrass Zostera marina and juvenile scallops Argopecten ventricosus (Sowerby II 1842) in Bahia Concepcion, Mexico. J. Shellfish Res. 18, 415–418.
Santamaría-Gallegos N. A., Riosmena-Rodríguez R., Sánchez-Lizaso J. L. (2006). Occurrence and seasonality of Halophila decipiens Ostenfeld in the Gulf of California. Aquat. Bot. 84, 363–366. doi: 10.1016/j.aquabot.2005.12.001
Santamaría-Gallegos N. A., Sánchez-Lizaso J. L., Félix-Pico E. F. (2000). Phenology and growth cycle of annual subtidal eelgrass in a subtropical locality. Aquat. Bot. 66, 329–339. doi: 10.1016/S0304-3770(99)00082-0
Schlegel R. W., Smit A. J. (2018). heatwaveR: A central algorithm for the detection of heatwaves and cold-spells. Open Source Software 3, 821. doi: 10.21105/joss.00821
Sheridan T. E., Felger R. S. (1977). Indian utilization of eelgrass (Zostera marina L.) in Northwestern Mexico: The Spanish colonial record. Kiva 43, 89–92. doi: 10.1080/00231940.1977.11757893
Short F. T., Polidoro B., Livingstone S. R., Carpenter K. E., Bandeira S., Bujang J. S., et al. (2011). Extinction risk assessment of the world’s seagrass species. Biol. Conserv. 144, 1961–1971. doi: 10.1016/j.biocon.2011.04.010
Spalding M. D., Fox H. E., Allen G. R., Davidson N., Ferdaña Z. A., Finlayson M., et al. (2007). Marine ecoregions of the world: A bioregionalization of coastal and shelf areas. BioScience 57, 573–583. doi: 10.1641/B570707
Talbot S. L., Sage G. K., Rearick J. R., Fowler M. C., Muñiz-Salazar R., Baibak B., et al. (2016). The structure of genetic diversity in eelgrass (Zostera marina L.) along the north Pacific and Bering Sea coasts of Alaska. PloS One 11, e0152701. doi: 10.1371/journal.pone.0152701
Torre-Cosio J. (2002). Inventory, monitoring and impact assessment of marine biodiversity in the Seri Indian territory, Gulf of California, Mexico. University of Arizona, Arizona, (AZ.
Ulate K., Sánchez C., Sánchez-Rodríguez A., Alonso D., Aburto-Oropeza O., Huato-Soberanis L. (2016). Latitudinal regionalization of epibenthic macroinvertebrate communities on rocky reefs in the Gulf of California. Mar. Biol. Res. 12, 389–401. doi: 10.1080/17451000.2016.1143105
UNEP-WCMC, Short F. T. (2021). Global distribution of seagrasses (version 7.1). Seventh update to the data layer used in Green and Short, (2003) (Cambridge (UK: UN Environment Programme World Conservation Monitoring Centre). doi: 10.34892/x6r3-d211
United Nations Environment Programme (2020). Out of the Blue. The value of seagrasses to the environment and to people (Nairobi, UNEP). Available at: www.un.org/Depts/Cartographic/english/htmain.htm.
Unsworth R. K. F., McKenzie L. J., Collier C. J., Cullen-Unsworth L. C., Duarte C. M., Eklöf J. S., et al. (2019). Global challenges for seagrass conservation. Ambio 48, 801–815. doi: 10.1007/s13280-018-1115-y
Valencia M. E., Atondo J. L., Hernández G. (1985). Nutritive value of Zostera marina and cardon (Pachycereus pringlei) as consumed by the Seri Indians in Sonora Mexico. Ecol. Food Nutr. 17, 165–174. doi: 10.1080/03670244.1985.9990890
Valle M., van Katwijk M. M., de Jong D. J., Bouma T. J., Schipper A. M., Chust G., et al. (2013). Comparing the performance of species distribution models of Zostera marina: implications for conservation. Sea. Res. 83, 56–64. doi: 10.1016/j.seares.2013.03.002
Van Dam B., Polsenaere P., Barreras-Apodaca A., Lopes C., Sanchez-Mejia Z., Tokoro T., et al. (2021). Global trends in air-water CO2 exchange over seagrass meadows revealed by atmospheric eddy covariance. Glob. Biogeochem. Cycles 35. doi: 10.1029/2020GB006848
Velarde E., Ezcurra E., Cisneros-Mata M. A., LavÍn M. F. (2004). Seabird ecology, El Niño anomalies and prediction of sardine fisheries in the Gulf of California. Ecol. Appl. 14, 607–615. doi: 10.1890/02-5320
Waycott M., Duarte C. M., Carruthers T. J. B., Orth R. J., Dennison W. C., Olyarnik S., et al. (2009). Accelerating loss of seagrasses across the globe threatens coastal ecosystems. Proc. Natl. Acad. Sci. 106, 12377–12381. doi: 10.1073/pnas.0905620106
Wilder B. T., Felger R. S., Romero-Morales H., Quijada-Mascareñas A. (2007). New plant discoveries for Sonoran islands, Gulf of California, Mexico. J. Botanical Res. Institute Texas 1, 1203–1227. https://www.jstor.org/stable/41971548.
Wurl J. (2019). Competition for water: Consumption of golf courses in the tourist corridor of Los Cabos, BCS, Mexico. Environ. Earth Sci. 78, 674. doi: 10.1007/s12665-019-8689-y
Yaakub S. M., McKenzie L. J., Erftemeijer P. L. A., Bouma T., Todd P. A. (2014). Courage under fire: Seagrass persistence adjacent to a highly urbanised city–state. Mar. pollut. Bull. 83, 417–424. doi: 10.1016/j.marpolbul.2014.01.012
Keywords: critical habitat, blue carbon, spatial monitoring, habitat conservation, Mexico, Eastern Pacific
Citation: Ramírez-Zúñiga MA, Pérez-Estrada CJ, López-Calderón JM, Cannon AL, Vanderplank S and Favoretto F (2024) Updating and validating seagrass ecosystem knowledge in the gulf of California: a comprehensive review. Front. Mar. Sci. 11:1402044. doi: 10.3389/fmars.2024.1402044
Received: 16 March 2024; Accepted: 24 April 2024;
Published: 17 May 2024.
Edited by:
Lorenzo Mari, Polytechnic University of Milan, ItalyReviewed by:
Tania Cota, Center for Research and Advanced Studies - Mérida Unit, MexicoSusan Bell, University of South Florida, United States
Copyright © 2024 Ramírez-Zúñiga, Pérez-Estrada, López-Calderón, Cannon, Vanderplank and Favoretto. This is an open-access article distributed under the terms of the Creative Commons Attribution License (CC BY). The use, distribution or reproduction in other forums is permitted, provided the original author(s) and the copyright owner(s) are credited and that the original publication in this journal is cited, in accordance with accepted academic practice. No use, distribution or reproduction is permitted which does not comply with these terms.
*Correspondence: Fabio Favoretto, ZmZhdm9yZXR0b0B1Y3NkLmVkdQ==