- Benthic Resources and Processes Group, Institute of Marine Research, Fram Centre, Tromsø, Norway
Wild gadoids are known to have close associations with open-net fish farms, leading to concerns about changes in natural fish distribution and feeding patterns. However, the nature of the relationships between non-pelagic fish and mobile benthic invertebrates and aquaculture facilities remains poorly documented, even though this group contains widespread commercial North Atlantic species. This study utilizes baited remote and towed underwater video surveys to resolve the ecosystem-level effects of open salmon aquaculture cages and organic enrichment on the spatial structure and relative abundance of demersal fish and crustacean communities. Towed camera surveys were carried out 50 to >600 m downstream of three farms producing finfish in Western Finnmark and four in the Frøya region of Norway. Towed camera surveys were paired with baited remote underwater video surveys at the four Frøya farms. The declining enrichment gradient with distance downstream of the farm cages was confirmed by modeled and observed depositional flux measurements and benthic infaunal community composition. The community structure of wild demersal fish and crustaceans in close association with the seafloor varied with the distance from the aquaculture sites. In addition to the established pattern of the attraction of gadoids, the edible crab (Cancer pagurus) and flatfish (Pleuronectes platessa and Microstomus kitt) showed a preference for areas 10–150 m from farm cages where organically enriched sediments contain a rich food source of opportunistic polychaetes. In contrast, the cuckoo wrasse (Labrus mixtus) and the common hermit crab (Pagurus bernhardus) exhibited higher numbers in locations over 600 m from the farms. Findings show how changes in the benthic food web from aquaculture enrichment can affect the spatial distribution of poorly studied but commercially important fish and crustaceans. Such changes in distribution can impact the availability of these species to coastal fisheries, especially in dense aquaculture regions.
1 Introduction
The European aquaculture industry is dominated by Atlantic salmon cultured in northern European nations: Scotland, Iceland, and the Faroe Islands, but most of all Norway (Iversen et al., 2020; European Environmental Agency, 2021). Most fish are cultured within open cages located in fjords or semi-exposed coastal regions, and expansion is thus reliant on available coastal space (Hersoug et al., 2021). Regions in mid and northern Norway, where free space remains available and rising coastal water temperatures are improving environmental conditions for culturing Atlantic salmon, are experiencing regional expansion in salmon aquaculture (Torbjørn, 2008; Bailey et al., 2017). Within existing and new aquaculture sites, the governance of salmon farming in northern Atlantic waters aims to develop an industry that produces a sustainable food source (Aarstad et al., 2023). This requires knowledge of all impacts of salmon farming across biological and physical components of the coastal ecosystem. Implicit in this objective is the expectation that it must avoid impacts on wild fish and crustacean stocks exploited by the coastal fishing industry. Research has shown that the gadoids [saithe (Pollachius virens), Atlantic cod (Gadus morhua), and haddock (Melanogrammus aeglefinus)] are attracted to finfish farms, but less is known about the impacts on the distribution of other coastal fish, especially non-pelagic species that live on or are close to the seafloor and crustaceans.
The coastal fishing industry has a long-standing economic and social role in Europe and is a major food producer for numerous countries. The most important demersal fisheries in European waters target gadoids: Atlantic cod (G. morhua), haddock (M. aeglefinus), saithe (P. virens), and whiting (Merlangius merlangus) and crustaceans such as the Norwegian lobster (Nephrops norvegicus) (ICES Fisheries Overview, 2021). However, non-pelagic fish such as the flatfish (Pleuronectes platessa and Microstomus kitt), spurdog (Squalus acanthias), ling (Molva molva), Atlantic redfish (Sebastes spp.), and tusk (Brosme brosme) are also key coastal species commercially fished in NE Atlantic and Norwegian waters (ICES Fisheries Overview, 2021). Crustaceans such as the northern prawn (Pandalus borealis) and the edible crab (Cancer pagurus) are also abundant on the NE Atlantic coast where they present significant commercial value (Woll et al., 2006; Öndes et al., 2017). Growth of the finfish aquaculture industry in European coastal zones, while important for employment in rural regions, is in conflict for space with coastal commercial and recreational fisheries for these species (Bostock et al., 2016; Olaussen, 2018). Concerns also exist as to the environmental impacts associated with finfish aquaculture, particularly those that can affect coastal fish and crustacean stocks in areas already experiencing declines in fishing resources (Bailey and Eggereide, 2020; Bjørkan and Eilertsen, 2020). Potential impacts include effects from the chemical treatment of caged salmon lice infestations on the mortality of coastal shrimp and the European lobster (Parsons et al., 2020; Samuelsen et al., 2020) and changes to the use of coastal habitats by Atlantic cod (Skjæraasen et al., 2022). Some wild benthopelagic fish visit and congregate around salmon net pens to feed on uneaten food and fish feces that fall through the open pens (Dempster et al., 2009, 2010; Fernandez-Jover et al., 2011). The physical structure of net pens also acts as a fish aggregating device, and wild fish can be chemically attracted to the congregation of farmed fish (Dempster et al., 2002; Tanner and Williams, 2015).
Coastal finfish cages are known to attract wild fish in the Mediterranean Sea, Canary Islands, and Australia, a process known to affect the behavior and distribution of some species (Boyra et al., 2004; Dempster et al., 2004, 2005). Saithe are well known to gather around finfish farms in mid and northern Norway (Bjordal and Skar, 1992; Dempster et al., 2009; Uglem et al., 2009; Dempster et al., 2010) and Scotland (Carss, 1990). Atlantic cod, haddock (M. aeglefinus), and mackerel (Scomber scomber) are also the most common species around farm cages when considering biomass (Dempster et al., 2009, 2010). Atlantic cod from nearby spawning and feeding grounds are attracted to net pens, where some individuals can spend considerable time (months). This increased understanding of cod movements around net pens leads to further questions as to the implications of net pen attraction and aquaculture feed on cod life histories, behavior, health, and reproduction (Skjæraasen et al., 2022). In contrast to attracting fish, boat and machinery noise and activity can also cause fish and other marine life to avoid the area. Additionally, increased chances to encounter predators such as cod and haddock can in theory lead to fewer small fish and crustaceans close to the open-net pens. Through either attraction or aversion, aquaculture pens undoubtedly impact the movement of other marine species in coastal areas. Understanding the complexities of this process across all ecological groups is a necessary part of assessing and managing the ecosystem-level interaction of open-net pens.
Visual counts by snorkeling, trawling, stationary video camera systems, and hydroacoustic surveys have been used to examine wild fish species in association with finfish farms (Machias et al., 2005; Tuya et al., 2006; Dempster et al., 2009; Uglem et al., 2009; Dempster et al., 2010; Goodbrand et al., 2013; Tanner and Williams, 2015). Each has limitations in which compartments of the fish communities they can resolve, and a combination of methods are the most effective means to understand changes in community dynamics. Baited remote underwater video systems (BRUVS) are now a common technique to survey demersal fish and crustacean communities on and above the seafloor (Zintzen et al., 2012; Ebner et al., 2014). The distribution of wild benthic fish and crustaceans around finfish farms in southern Australia was examined using a BRUVS and detected only small-scale changes in numbers around the farm cages (Tanner and Williams, 2015). Towed underwater camera techniques used for mapping and quantifying benthic communities (e.g., Dunlop et al., 2020) can be combined with BRUVS to record densities of conspicuous benthic invertebrates (e.g., the edible crab and potential prey species) and approximate densities of benthic fish. The ability to undertake video tows several hundred meters in length enables relatively high-resolution spatial studies and provides a mechanism for describing changes along the traditional fish farm benthic enrichment gradient, usually spanning hundreds to thousands of meters (Keeley et al., 2013, 2019).
Despite the large-scale expansion of finfish aquaculture in northern Europe and strong interest regarding potential implications for coastal wild fish and crustacean populations, research to date has mostly focused on farm effects on gadoid fish distribution (i.e., saithe, Atlantic cod, and haddock). In particular, the effects of local organic enrichment on the distribution of other demersal fish and crustacean species living in close association with the seafloor have been largely unstudied. This study aims to address this by examining the effects of the presence of salmon aquaculture facilities and a particulate organic material enrichment gradient from farm wastes (uneaten food and feces) on the spatial structure, density, and relative abundance of demersal fish and crustacean communities.
2 Methods
2.1 Towed underwater camera transects and baited remote underwater video surveys
A towed underwater camera (TUC) system was used to record the relative diversity and density of demersal fish and crustaceans in the vicinity of seven study farms in northern and mid-Norway (described in Section 2.2). The TUC consisted of a GoPro Hero4 in an underwater housing, two video lights [Keldan 4X video light (9,000 lm)], and laser scales mounted on a frame that is easily maneuverable over the seabed in the main current direction. The TUC was “flown” approximately 1 m above the seabed, and the position was continually recorded by the vessel’s Global Positioning System (GPS). The TUC and its use as a low-cost and effective tool for coastal ecological mapping is described in detail in Dunlop et al. (2020).
BRUVS surveys were conducted in accordance with the field procedures outlined in Langlois et al. (2020). A single stereo-BRUVS that consisted of two HD GoPro Hero4 video cameras in customized underwater housings (from SeaGIS) mounted 70 cm apart on a steal base bar attached to a weighted aluminum frame was deployed. Camera housings faced toward, but at a slightly inwards angle (7°), a mesh bait bag containing ~1 kg of freshly thawed Atlantic herring (Clupea harengus) suspended 1.5 m from the housings on a pole in the middle of the field of view. The lighting was from a single Keldan 4X video light (9,000 lm). The BRUVS was lowered by rope to the seabed, and the GPS position was recorded and marked by two surface buoys. The BRUVS remained on the seabed for at least 1 hour during which continuous HD video footage was recorded of fish and crustaceans attending the bait.
2.2 Study area and sampling design
TUC surveys were carried out in the vicinity of three study farms in Western Finnmark, northern Norway, in October 2017 (Figure 1A). Farm A is on the western shore of Øksfjorden (Figures 1B, C) and was established in 2011, and it has a maximum allowed biomass of 3,600 tons of Atlantic salmon. Farms B and C are on the shores of Vargsundet, north of Altafjorden (Figures 1B, D), and were established in 2005 and 2001, respectively. Farm B is at the entrance to Bekkarfjorden and has a maximum allowed biomass of 5,400 tons, while Farm C on the eastern shore of Vargsundet has a maximum allowed biomass of 3,480 tons of salmon (Figure 1D). TUC transects began approximately 50 m from the fish cage and ran parallel to the coastline along the 70- and 100-m depth contours to approximately 800 m downstream of each farm. At Farms A and B, two to three replicate transects were recorded along each depth contour (70 and 100 m), while the shallower waters at Farm C only allowed for a pair of replicate transects along the 70-m depth contour. Details for TUC surveys in Finnmark are presented in Supplementary Table 1A. TUC sections were separated into categories representing distance to the nearest finfish farm: Farm (50 to 149 m to the nearest farm cage), Intermediate (150 to 599 m from the farm), and Reference (>600 m from the farm). A distance of over 600 m from the farm was considered as a reference site, as simulations of the dispersal of organic waste from salmon aquaculture in fjords have predicted that >75% of organic waste is dispersed less than 500 m from the cages (Bannister et al., 2016).
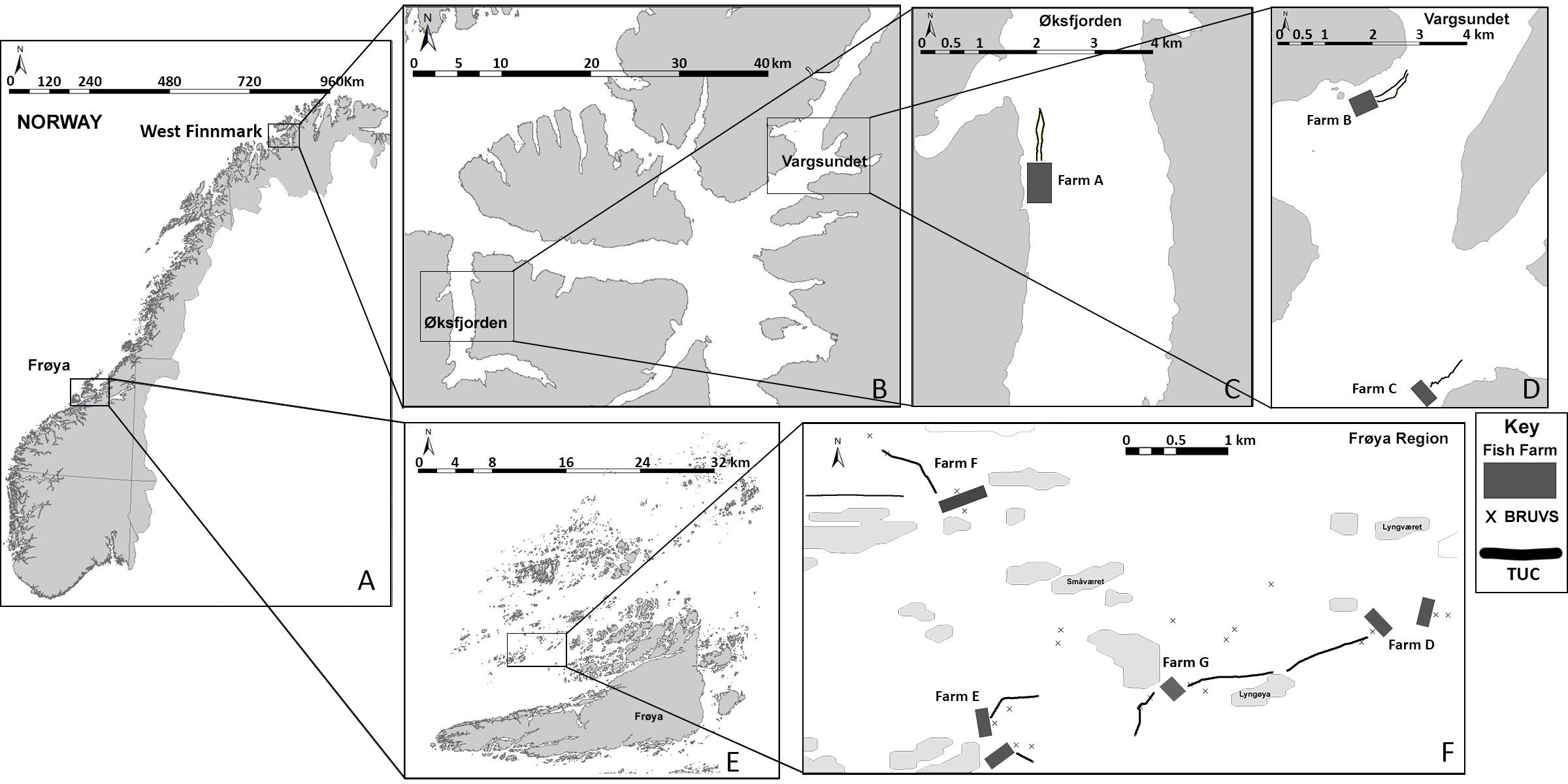
Figure 1 Map of the study locations (A) on the Norwegian coast, (B) Western Finnmark and (E) Frøya. Maps of the positions of towed underwater camera (TUC) transects and baited remote underwater video system (BRUVS) surveys within (C) Øksfjorden, (D) Vargsundet and (F) the Frøya region.
TUC and BRUVS surveys were conducted between October 2019 around four salmon farms (Farms D–G) in the Frøya region of central western Norway. This is a semi-exposed coastal region, populated by many small low-lying islands close to the larger island of Frøya, where many high-capacity Atlantic salmon farms are situated (Figures 1A, E). Two of the study farms (Farms D and E) had a maximum allowed biomass of 9–11,000 tons, and Farms F and G contained between 5,500 and 6,240 tons of salmon. Farm F was established in 1995, Farm E in 2000, Farm D in 2008, and the latest, Farm G, in 2017. The seabed around these farms consists of sandy-soft sediments and scattered rocky reefs at water depths between 20 and 75 m. At Farms E and G, two replicate TUC transects were recorded leading from the farm edge to ~800 m downstream of the farms in the main current direction. A single replicate transect was recorded in the primary current direction leading away from Farms D and F (Figure 1F). At each of the four Frøya farms, two replicate BRUVS surveys were conducted less than 10 m from the cage edge (referred to as the “Farm” location in further analysis), while a further two replicate deployments were positioned 150 m downstream of the farm to coincide with TUC transect tracks (described as “Intermediate”). Intermediate deployments were, however, not achieved at Farm F. For each farm, two reference locations over 600 m downstream of the farm were surveyed with the BRUVS (Figure 1F). BRUVS deployments were upon flat sandy/mud substrate between rocky reef structures. Details of the BRUVS and TUC surveys in the Frøya region are presented in Supplementary Table 1B.
2.3 Enrichment gradient and benthic indicators
The depositional flux rate of suspended particulate matter along the enrichment gradient leading downstream of the farms in the main current direction was measured using sediment traps. Each trap mooring consisted of a pair of cylindrical sediment traps for collecting suspended particulate material attached to a frame suspended approximately 2.5 m above the seafloor. A detailed description of the trap design and sampling procedure is available in Keeley et al. (2019) as is the procedure for the quantification of mean total particulate matter (TPM) dry weight (g m−2 d−1) from trap samples. At the three study farms in Finnmark, trap moorings were deployed beside the farm cages and then at 100–200-m intervals until 650 m downstream of the farm. TPM values were corrected to max production at each farm, and a power function was fitted to the relationship between full production TPM and distance to each farm. Modeled and observational flux measured with sediment traps showed a rapid decline in organic waste deposition with distance from Farms A, B, C, E, and F. Depositional flux information was, however, not available for Farms F and G, but they were assumed to show a general pattern of declining deposition with distance downstream of the farm cages. The flux of organic material was higher at the cages of Farms B and C (44 and 51 g m−2 d−1) than at Farm A (24 g m−2 d−1). At the Frøya Farms E and F, organic material was deposited by the cages at 80 and 33 g m−2 d−1 at peak production but rapidly declined to background levels past 300 m downstream of the cages. For all farms, background fluxes (equivalent to the most distant stations) were achieved at around 650 m from the cages (Supplementary Figures 1A–C).
Benthic infaunal samples were collected at Farms A, B, C, D, and E with a grab from the cage edge up to 1.5 km downstream of each farm in the main current direction. Further infaunal sampling details are presented in Dunlop et al. (2021) and Keeley et al. (2019); Keeley et al. (2020). Infaunal samples collected at the Finnmark farms (A, B, and C) underwent a full taxonomic investigation with all fauna counted and identified to the lowest possible taxonomic group. Community composition was quantified and converted to infauna and AZTI marine biotic index (AMBI) and Norwegian sensitivity index (NSI) following the Norwegian standard NSI6665 (Standards Norway 2013). Infaunal samples were grabbed around two of the Frøya farms (D and E) and used to calculate the total number of species (S), total abundance (N), and the Shannon–Wiener diversity index (H′, base e). Quantitative densities of surface-dwelling fauna or epifauna (echinoderms, crustaceans, and bivalves) not well represented in grab sampling were recorded by a drop video quadrat (1 m2) following the study transects leading in the down-current direction from Farms D and E out to approximately 1,200 m. Details of the video surveys for epifauna and methods for image analysis and density estimates are presented in Keeley et al. (2020).
2.4 Video annotation methods
Video footage from the BRUVS was viewed in the VLC player, and all fish and crustaceans entering the field of view were identified to the highest taxonomic level. The maximum number of fish and crustaceans observed at the bait at one time (MaxN) during each minute of individual BRUVS deployments was recorded. MaxN is a recognized proxy of relative abundance in BRUVS studies (Langlois et al., 2020). Any distinctive behaviors were noted alongside whether species approached and fed on the bait or just entered the field of view. For analysis purposes, the TUC transect footage from Frøya and Finnmark was subdivided into ~5–10-m swath sections; within each section, all conspicuous fish and crustacean taxon were identified, and the number of individuals from each taxon was recorded. Counts of individual taxa were converted into densities by dividing by the estimated seafloor area covered (m2), scaled using paired lasers.
2.5 Data analysis
The relationships between BRUVS MaxN counts and TUC densities and distance to the nearest farm (Cage, Intermediate, and References), study sites (A, B, C, D, E, F, and G), and their interactions were tested using generalized linear models (GLMs). GLMs had either a Poisson distribution, suitable for the analysis of count data, or a negative binomial distribution for overdispersed data. Zero-inflated GLMs were used when the number of zeros in MaxN counts and densities meant that the data did not fit a Poisson or negative binomial distribution (Zuur et al., 2009; Zuur and Ieno, 2016). The goodness of fit of all GLMs was tested using a chi-squared test based on the residual deviance and degrees of freedom. Univariate statistical analysis was conducted in the R language and environment for statistics (R Core Team, 2022) using the package pscl (Zeileis et al., 2008). Multivariate analyses were conducted on square root-transformed MaxN or density data to reduce the influence of dominant species (Clarke and Gorley, 2015). A PERMANOVA was used on a Bray–Curtis similarity index to evaluate the effect of the distance to farm category (Cage, Intermediate, and References) and study farm on community composition. The same factors were used in a canonical analysis of principal (CAP) coordinates analysis to visually represent farm or distance-to-farm-specific community structures. Key demersal species contributing to the dissimilarity between benthic epifaunal communities at distance categories or farms were overlaid as vector plots upon the CAP ordination. Similarity Percentage (SIMPER) analysis also determined the role of individual species in the dissimilarities between communities observed around different study farms and within distance groups. Multivariate statistical analysis was carried out in PRIMER-e v.7 (Clarke and Gorley, 2015).
3 Results
3.1 Frøya BRUVS and TUC surveys
Thirteen demersal fish and two crustacean taxa were identified in the Frøya BRUVS surveys and 17 fish and five crustacean taxa in TUC surveys in the same region (Figure 2). A significant difference in the fish and crustacean assemblages was resolved between the different Frøya farms in both BRUVS (PERMANOVA; F = 1.9, p = 0.03) and TUC surveys (F = 3.3, p = 0.001) (Table 1). CAP analysis of the assemblages in TUCs showed a clear separation between the four Frøya farm sites (D, E, F, and G). Atlantic cod (G. morhua), saithe (P. virens), cuckoo wrasse (Labrus mixtus), and the edible crab (C. pagurus) characterized assemblages around Farms D and E in both BRUVS and TUC surveys; in addition, BRUVS surveys identified thorny ray (Raja clavata) and poor cod (Trisopterus minutus) to be common. CAP and SIMPER analyses revealed a distinction in assemblages at sites F and G, where haddock (M. aeglefinus) and the hermit crab (Pagurus bernhardus) were characteristic species in both surveys. Spiny dogfish (S. acanthias) and whiting (M. merlangus) were also regular visitors to the BRUVS at these farms (Figures 3A, B). Dissimilarities in the SIMPER analysis were mainly driven by higher average abundances of saithe, poor cod, and the goby Pomatoschistus spp. close to Farms D and E (Supplementary Tables 2A, B).
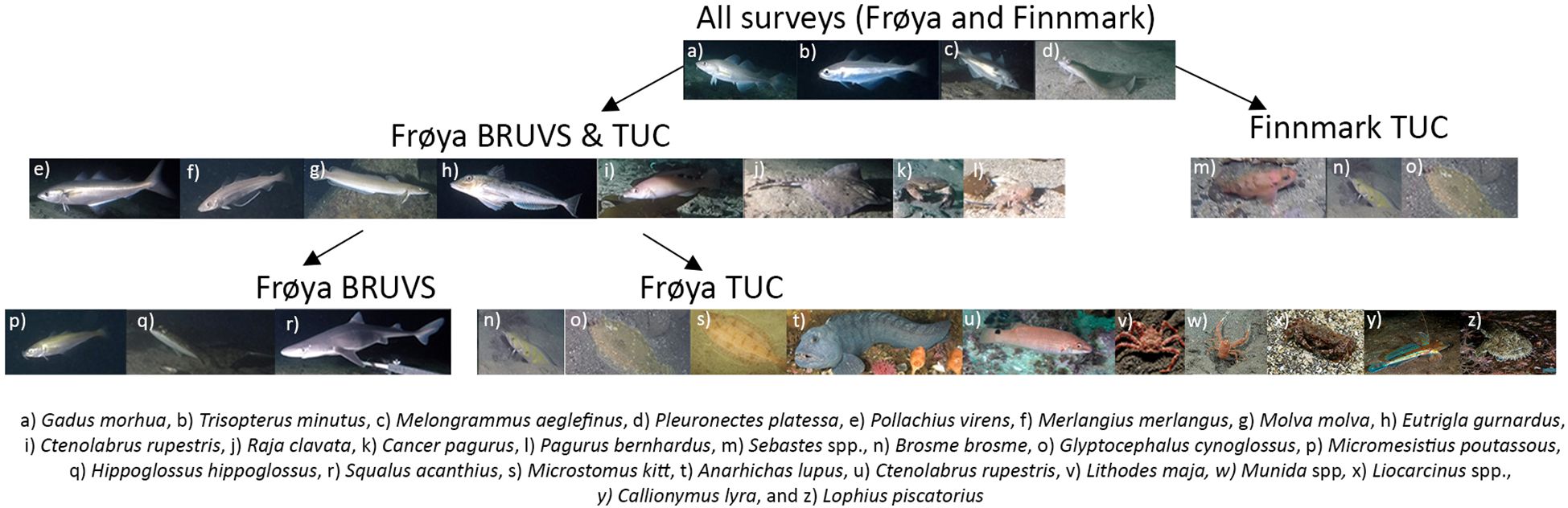
Figure 2 Demersal fish and crustacean taxa observed in baited remote underwater video system (BRUVS) and towed underwater camera (TUC) surveys at farm sites at Frøya and Finnmark. Images extracted from BRUVS and TUC videos.
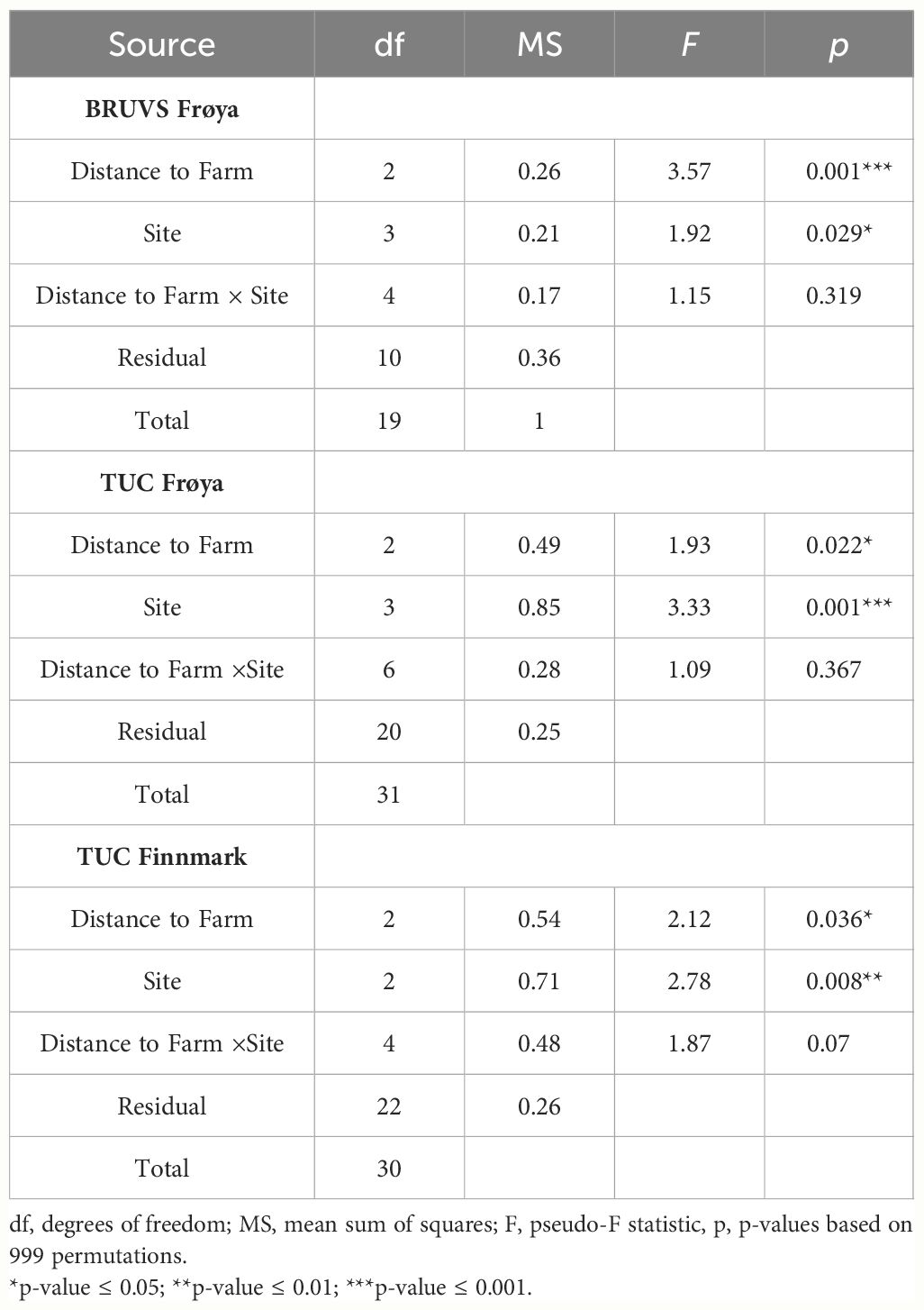
Table 1 Results of multivariate PERMANOVA tests of the relationship between demersal fish and crustacean assemblages with distance to farm, site, and the interaction between distance to farm and site.
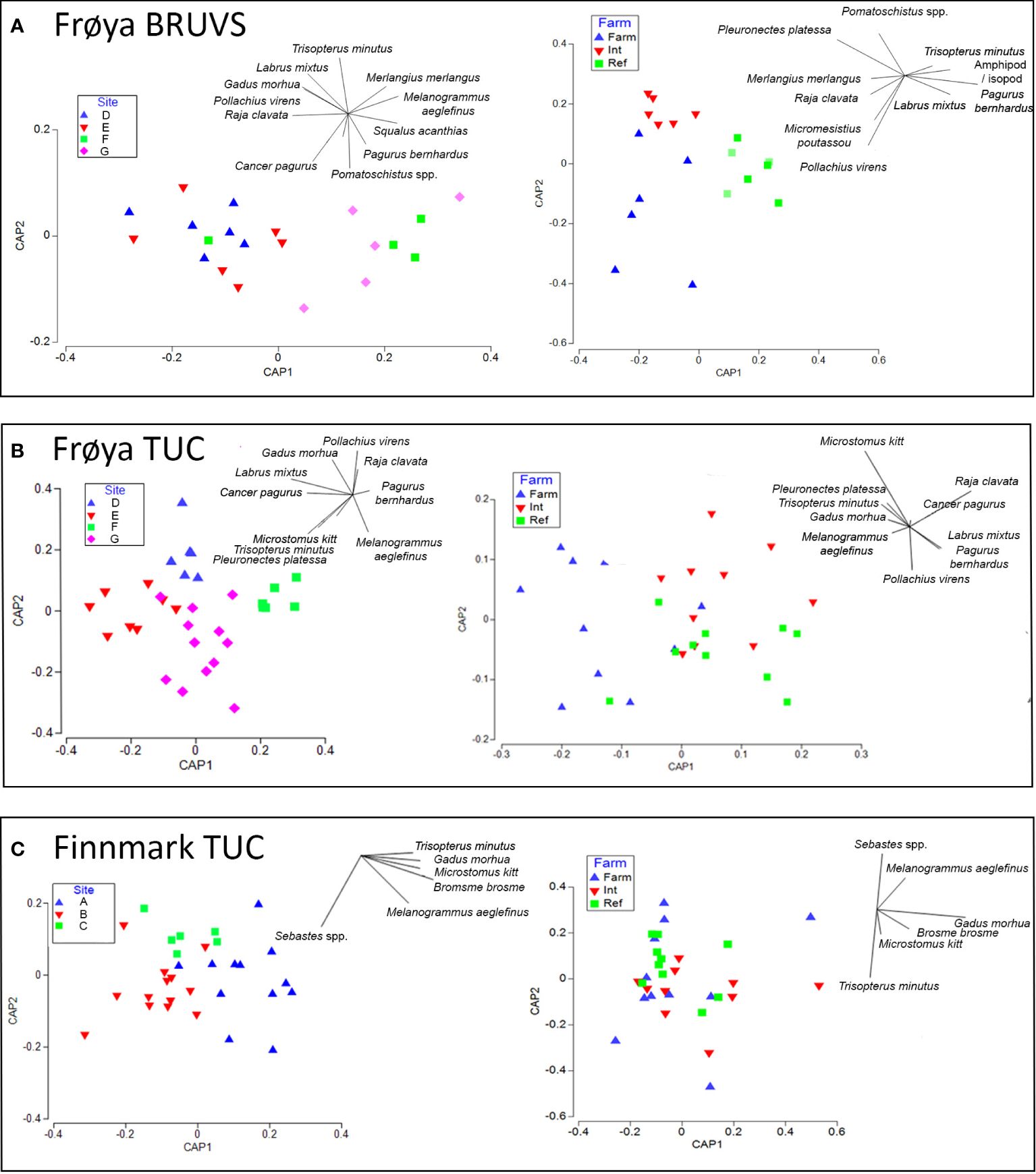
Figure 3 Ordination diagram of the canonical analysis of principal components (CAP) of demersal fish and crustacean communities recorded in (A) Frøya baited remote underwater video system (BRUVS), (B) Frøya towed underwater camera and (C) Finnmark towed underwater camera surveys. The community structure sampled in each BRUVS deployment or transect are displayed at different farm sites and at Farm, Intermediate (Int) and Reference (Ref) locations in relation to the farm cages. Overlay represents the key taxa involved in structuring the community composition.
PERMANOVA analysis using MaxN from BRUVS and density from TUC surveys as measures of abundance indicated a significant difference in assemblages between areas close to the farm cages, at an intermediate distance and reference sites (F = 3.6, p = 0.001, F = 1.9, p = 0.02, Table 1). Results revealed no significant interaction between the distance to farm and site factors on assemblage structure (Table 1). Plaice (P. platessa) and skate (R. clavata) were common in farm and intermediate zones by both survey methods. However, only BRUVS showed that high numbers of saithe (P. virens) characterized farm assemblages. In contrast, a higher density of the lemon sole (M. kitt) in farm cages was only captured in TUC surveys. T. minutus characterized assemblages close to the farm in TUC surveys, but contrastingly in BRUVS, the species was most associated with reference sites. Both survey types found L. mixtus and the hermit crab to be primary species at reference sites (CAP plots, Figures 3A, B). SIMPER analysis revealed that the greatest dissimilarity in community structure was between farm and reference transect regions. In BRUVS surveys, higher average abundances of P. virens, P. platessa, and Pomatoschistus spp. at the farm sites and T. minutus and P. bernhardus within reference locations were responsible for the majority (53.4%) of the dissimilarity. In TUC surveys, higher abundances of poor cod, the goby (Gobiusculus flavescens), the flatfishes (plaice and lemon sole), saithe, and the edible crab at the farm accounted for over 50% of the dissimilarly in community structure (Supplementary Tables 2C, D).
Differences in fish and crustacean assemblage structure between farm, intermediate, and reference areas were supported by species-specific spatial responses. P. virens MaxN and density were significantly higher at cages in Frøya for both survey methods (Z = −6.5, p < 0.0001 and Z = −4.23, p < 0.0001). A mean MaxN of 20 saithe was observed at one time in BRUVS surveys around the farm cages, compared to only a few individuals at Intermediate or Reference sites. However, this relationship was only at cages in sites D and E where most saithe were observed in BRUVS surveys, made apparent by a significant farm and site interaction (GLM; Z = 3.7, p < 0.001) (Figure 4A). Significantly more plaice (P. platessa) were observed close to the farm or at an intermediate distance in both survey types at sites D and E where plaice were prevalent (GLM; Z = −4.9, p < 0.0001 and Z = −4.0, p < 0.0001). A mean MaxN of 1.67 plaice was recorded at both Farm and Intermediate sites compared to 0.125 individuals at reference sites (Figure 4A). On average, three plaice were recorded every 100 m2 in transect sections 50–149 m from the cages, while a mean of 1.5 individuals per 100 m2 and a mean of less than 1 were observed in intermediate and reference transect sections (Figure 4B). The flatfish M. kitt was not present in BRUVS surveys, but a significantly higher density of individuals was found around farm cages in TUCs (Z = −4.6, p < 0.0001). R. clavata MaxN recorded in BRUVS deployments was slightly higher in the area around the cages where a mean maximum of three individuals (±0.5) were observed at once; however, this relative difference was not statistically significant (Figure 4A). C. pagurus MaxN and density were higher at farm and intermediate sites; however, only densities from TUC surveys were significantly higher (Z = 2.5, p < 0.05), and a significant interaction with site shows that this only occurred at sites D and E where the species was common (Figures 4A, B). L. mixtus and P. bernhardus MaxN and density were consistently higher in reference sites in both BRUVS (Z = 2.2, p < 0.05, and Z = 3.1, p < 0.005) and TUC surveys (Z = 2.6, p < 0.05, and Z = 1.9, p = 0.05). T. minutus MaxN was significantly higher in reference sites in the BRUVS (Z = 4.3, p < 0.0001), while conversely more individuals (mean one individual per 100 m2) were at reference locations in TUC surveys (Z = 6.4, p < 0.0001) (Figures 4A, B). A summary of the relationship of demersal fish and crustacean taxa to distance to farm resolved by GLMs is presented in Table 2, while full GLM results for the Frøya BRUVS and TUC surveys are presented in Supplementary Tables 3A, B.
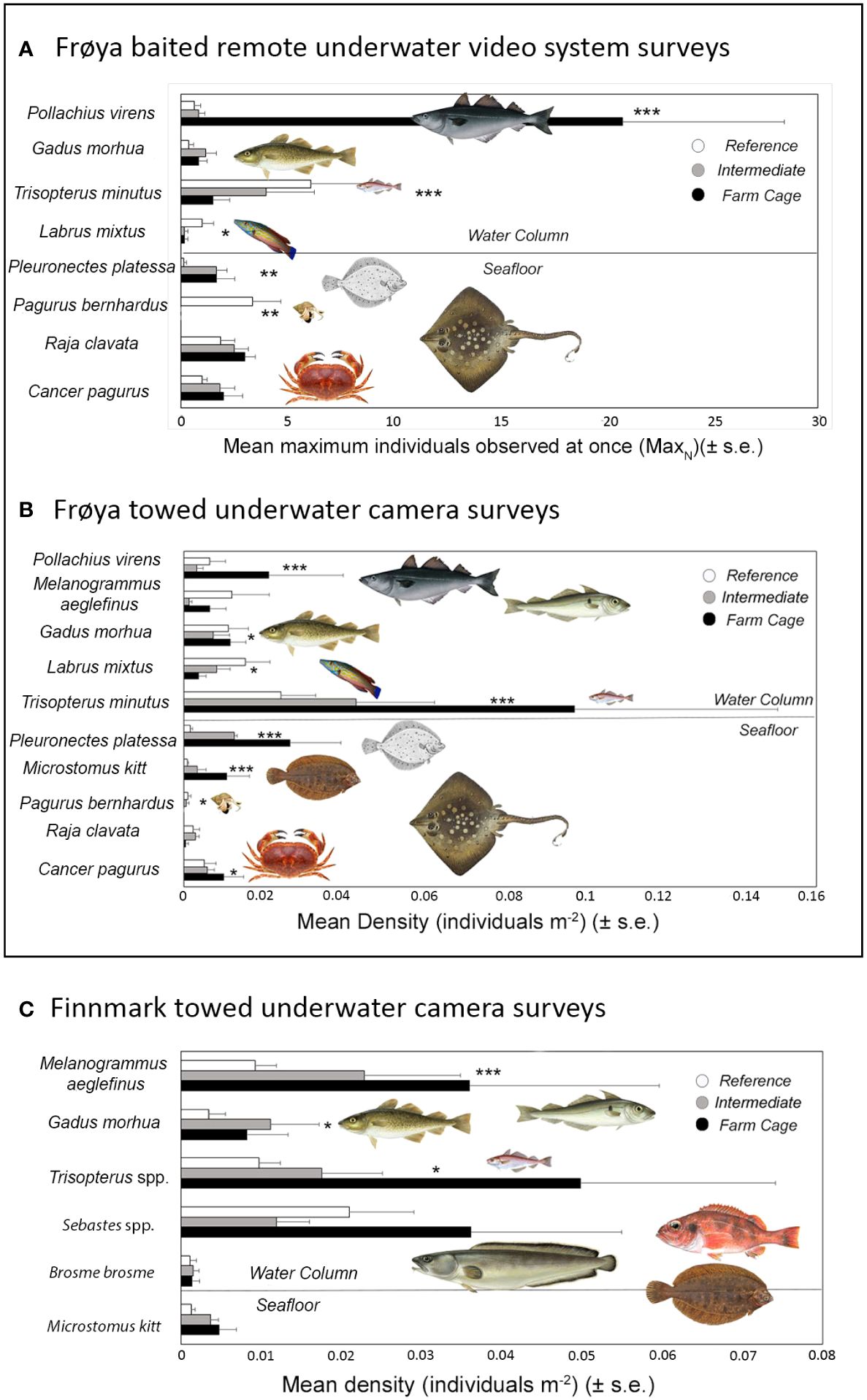
Figure 4 Bar plots of the mean (± s.e.) MaxN or density of dominant fish and crustacean species in (A) Frøya BRUVS (each bar represent n = 6) and (B) TUC transects (each bar represent n = 12) in each distance to farm category. Plot (C) represent the mean (± s.e.) density of dominant fish and crustaceans in each distance to farm category in Finnmark TUC transects. The significance level of relationships between fish and crustacean MaxN and density and distance to farm modelled using GLMs are marked. *: p-value ≤ .05; ** p-value ≤ .01; p-value ≤ .001.
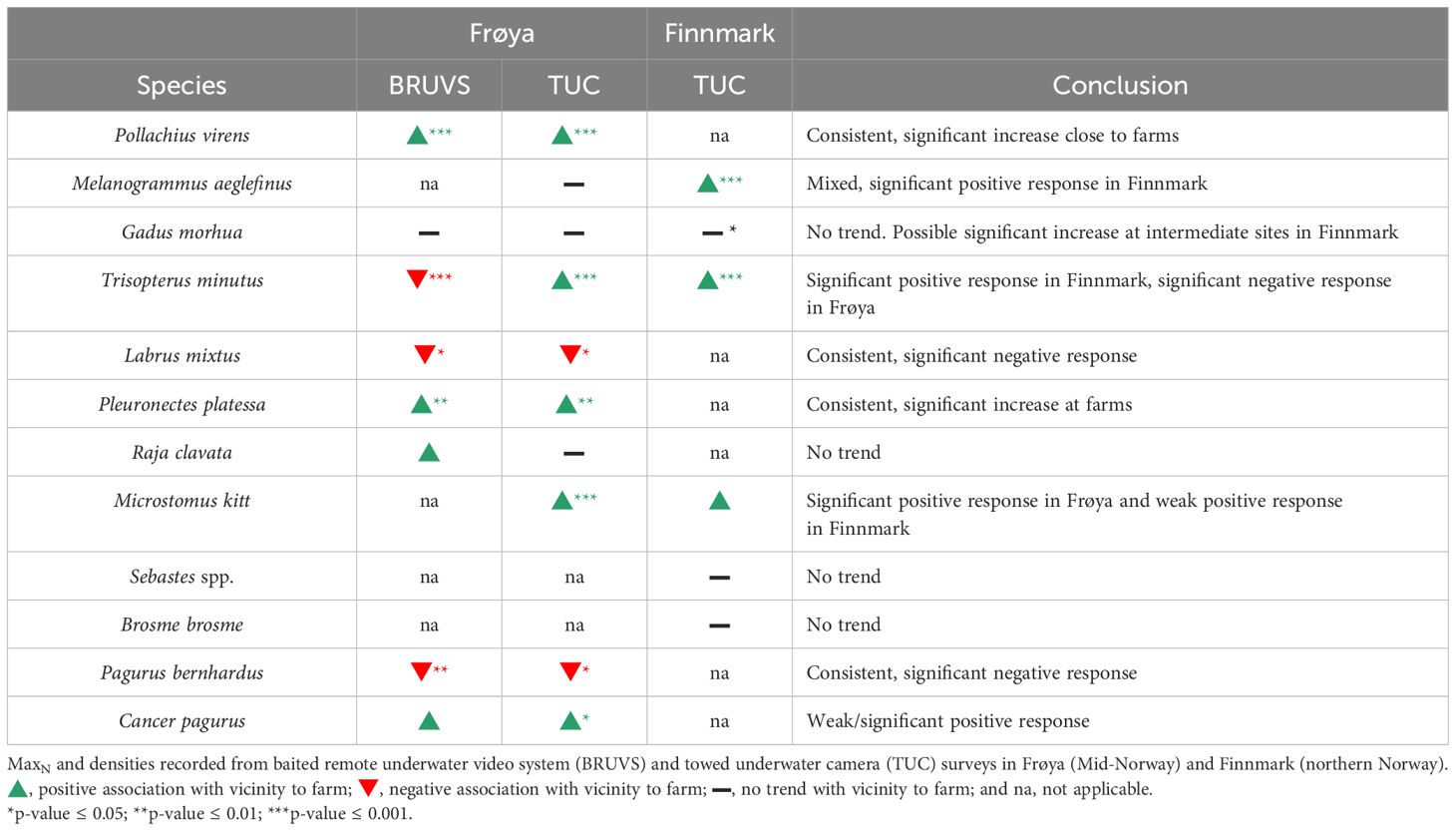
Table 2 Summary table of the results of generalized linear model (GLM) examining the relationship between fish and crustacean MaxN and densities and distance to the nearest farm.
3.2 Finnmark TUC surveys
At Finnmark farm sites, some of the same common demersal fish species in Frøya were also observed in TUC surveys (Atlantic cod, haddock, poor cod, and lemon sole); in addition, the rocky reef-associated species, rockfish (Sebastes spp.), and tusk (B. brosme) were recorded. However, only a few individual crustaceans were found in transects at Finnmark sites and not enough for statistical analysis (Figure 2). PERMANOVA results revealed a significant difference in demersal fish assemblages between the three farm sites (F = 2.78, p = 0.008) (Table 1). Cod, tusk, poor cod, haddock, and lemon sole were characteristic of Farm A, while rockfish particularly characterized assemblages at site B. Few demersal fish or crustaceans at all were seen in TUC transects at site C (Figure 3C; Supplementary Table 2E). There was limited separation between assemblages between distance to farm categories in the CAP plots (Figure 3C). However, SIMPER analysis revealed that higher average abundances of poor cod, rockfish, and haddock close to the farm were responsible for the majority (64% and 71%) of the dissimilarity in community composition between farm and reference and intermediate sites (Supplementary Table 2F). PERMANOVA results using density from TUC surveys as a measure of abundance indicated a significant difference in the demersal and crustacean assemblage composition between farm, intermediate, and reference areas (F = 2.12, p = 0.036; Table 1). In terms of species-specific responses with respect to proximity to the farm, densities of haddock (Z = −3.5, p < 0.0004) and poor cod (Z = −2.5, p < 0.05) were significantly higher in areas close to the farm; however, both species were only prevalent at site A as revealed by a significant interaction between site and distance to farm category in the model. G. morhua densities were higher at farm and intermediate sites (Z = 1.9, p = 0.06) compared to reference sites. For Sebastes spp., B. brosme, and M. kitt, there was no significant farm or site effect on fish density; however, the density of M. kitt was slightly higher at farm and intermediate areas compared to reference locations (Figure 4C, Table 2; Supplementary Table 3C).
3.3 Behavioral observations
The bait of the BRUVS was directly fed upon by S. acanthias, R. clavata, C. pagurus, and ling cod (M. molva), and in many cases, S. acanthias individuals tore open the bait bag and removed its contents. Aggressive conflict between these species for access to the bait was observed on numerous occasions. Other fish such as G. morhua, M. merlangus, M. aeglefinus, and P. platessa occasionally fed at the bait but only when more aggressive species such as S. acanthias, R. clavata, and M. molva, which regularly chased other fish species from the bait and out the camera field of view, were absent. P. virens and T. minutus were seen congregating in the vicinity of the bait but mainly remained in the background of the camera’s field of view.
4 Discussion
4.1 Result summary
Baited remote underwater video system and towed underwater camera surveys resolved local and regional scale differences in the associations of demersal fish and large mobile crustacean communities with finfish aquaculture cages. In addition to confirming existing theories that gadoids are attracted to farms, the two-pronged approach taken in this study revealed that locally abundant and commercially valuable flatfish and edible crabs also prefer areas in close proximity (10–150 m) to farm cages. However, the cuckoo wrasse and the common hermit crab were mainly found over 600 m from the farms in both camera surveys. Contrasting results on the relative distribution of poor cod to farms from BRUVS and TUC surveys highlight potential effects associated with the sampling methodology for this species.
4.2 Relative abundance and density of demersal gadoids in relation to finfish cages
The tight congregation of saithe feeding on waste salmon feed around Scottish and Norwegian fish farms is well reported (Carss, 1990; Mente et al., 2008; Dempster et al., 2009, 2010). Pelagic camera counts saw Atlantic cod aggregating within 100 m of two farms in Øksfjord, Finnmark, where they fed on waste feed along with benthic invertebrates and smaller fish (Dempster et al., 2010). BRUVS and TUC results confirmed the tight congregation of saithe around the Frøya study farms but also some indication that cod abundance increased within the intermediate zone of Finnmark farms. Such results are in agreement with recent findings from Skjæraasen et al. (2022) that some Atlantic cod can spend considerable time around cages.
Dempster et al. (2010) found no overall specific pattern in haddock aggregation around farms, except at one farm in Finnmark where four times more haddock were observed within 100 m of the farm compared to 200 m away. TUC surveys in Finnmark also confirmed an increase in haddock within the vicinity of some farm cages. Haddock is a demersal fish that also uses the midwater environment, feeding on a wide range of benthic and pelagic invertebrates and smaller fish (Albert, 1994). The species is therefore detectable in the water column cameras used by Dempster et al. (2010) as well as the more demersal-focused BRUVS and TUC surveys. Haddock are opportunistic feeders, and their diet reflects the local abundance of benthic and pelagic prey (Jiang and Jørgensen, 1996); therefore, accumulations of benthic invertebrates and smaller gadoids (young saithe and poor cod) closer to the farm cage provide an attractive food source.
The abundance of poor cod in relation to the study farms varied between BRUVS and TUC surveys. BRUVS surveys recorded much fewer poor cod beside or within 150 m of the cages than over 600 m away, which contrasted with TUC surveys. In both Frøya and Finnmark TUC surveys, significantly more poor cod were found in association with cages. The latter result is in accordance with a previous finding that poor cod abundances were elevated around one farm in mid-Norway (Dempster et al., 2010). Poor cod mostly consume benthic prey such as polychaetes, crustaceans, and small fish such as gobies (Salvanes and Nordeide, 1993), which means that an accumulation of opportunistic polychaetes as observed in association at these sites can act as a food source to attract poor cod to the farm area. The use of BRUVS assembles competitors or predators around the bait, which can lead to some fish being discouraged from approaching and entering the camera field of view (Jones et al., 2003; Dunlop et al., 2015). Small gadoids such as poor cod are the prey of large fish, such as skates (Skjæraasen and Bergstad, 2000) and other gadoids (Salvanes and Nordeide, 1993; Lambert et al., 2009). The aggregation of potential predators around BRUVS deployed close to and at an intermediate distance from the farm is likely to reduce the number of poor cod approaching the BRUVS and being recorded. This is supported by behavioral observations of poor cod rarely approaching the bait in Frøya BRUVS deployments when skate and gadoids were present.
4.3 Elevated relative abundance and density of flatfish around finfish aquaculture
Flatfish, the European plaice, and lemon sole mainly feed upon polychaetes, mollusks, and crustaceans living on or within the sediment (Rijnsdorp and Vingerhoed, 2001; Allen et al., 2004). Lemon sole are particularly reliant upon polychaetes in northern Atlantic waters (Rae, 1956; Allen et al., 2004). Increased organic enrichment from finfish farms is well known to enhance the biomass of opportunistic infauna alongside a reduction in species diversity (Brown et al., 1987; Kutti et al., 2007). Reductions in the organic enrichment of sediments with distance from Farm B and C cages coincided with a clear decline in macrofaunal abundance and enhanced macrofauna relative diversity. In addition to the full results on associated infaunal biotic indices at Farms A–C in Dunlop et al. (2021), infaunal assessments are presented here in number of individuals per main biotic group observed in each grab with distance to farm (Supplementary Figures 1A–C). Polychaetes dominated the infaunal communities at all three Finnmark farms, but ophiuroids were also well represented in communities at Farm B over 360 m from the cages. Polychaete abundances were at their highest generally being 50 and 250 m from the farm cages where abundances reached between 300 and 1,500 individuals per grab. After approximately 250 m from farm cages, polychaete numbers were generally below 200 individuals per grab and gradually declined with increasing distance, with the exception of one grab close to 1 km from Farm B. Previous drop video transects from Keeley et al. (2020), leading from Frøya Farms D and E, showed elevated densities of the large tube-dwelling polychaete, Arenicola marina, within 100 and 200 m of the cages. Densities of the opportunistic polychaete of the genus Capitella, an effective consumer of organic waste, were also highly elevated but between 100 and 400 m from the farms. A full assessment of infaunal communities along the enrichment gradient from the Frøya Farms D and E is available in Keeley et al. (2019); Keeley et al. (2020).
The total abundance of macrofauna in sediments was the highest under cages at Frøya Farms D and E and remained elevated approximately 600 m from the farm. Communities close to the farm were dominated by the polychaetes Capitella sp. and Ophryotrocha sp. and the large burrowing polychaete A. marina (Keeley et al., 2019, 2020). Sediment grab sampling collected around the Finnmark Farms A, B, and C in February 2019 also showed elevated total macrofaunal abundances, dominated by Capitella sp. Here, macrofaunal community indices indicative of enrichment were generally detected out to approximately 600 m. Results demonstrate that highly abundant macrofaunal communities, comprised mostly of opportunistic polychaete complexes, were present beneath and near to (<300 m) the study farms. These accumulations present a food source to plaice and lemon sole and are a likely reason why flatfish could be observed in higher abundances close to the farm cage or at intermediate distances. Video quadrats (1 m2) surveys undertaken in 2016 to quantify benthic epifauna densities around Farms D and E also observed that plaice were present close to the cages and suggested an attraction to elevated macrofaunal biomass (Keeley et al., 2021).
Edible crabs showed some attraction to the area surrounding the farm cages in Frøya. This observation is consistent with a previous finding that edible crabs collected close to Frøya Farms D and E had isotopic signatures in their tissues that suggested they had been feeding on waste fish feed, associated waste products, and/or other invertebrates that feed on the waste (Woodcock et al., 2018). The elevated relative abundance and densities of the edible crab at Frøya farms in both BRUVS and TUC surveys here, therefore, further reinforce the theory that the local edible crab population receives a trophic subside from farm waste products. The common hermit crab, the other crustacean commonly observed in the sandy seafloors around Frøya, was only observed over 150 m from the farm. P. bernhardus individuals are omnivores and rely upon deposit feeding for organic content contained in sandy sediments and filter feeding to collect zooplankton as well as scavenging (Gerlach et al., 1976; Ramsay et al., 1996). The higher flux of particulate material within 150 m of the farms at Frøya can potentially clog the hermit crab filtering apparatus. The benthic geochemical state and habitat characteristics around the Frøya farms are altered by enrichment, and sediments closer to the farms were of finer particle size (Keeley et al., 2020). Changes in sedimentary conditions around the farm could disturb deposit-feeding conditions for hermit crabs, leading to populations favoring areas outside of the primary farm footprint.
Cuckoo wrasse were recorded at higher numbers in areas more than 600 m from the Frøya farms by both survey methods. Despite little knowledge of the population ecology of wrasse on the Norwegian coast, several wrasse species, including cuckoo wrasse, are intensively fished to act as cleaner fish to control sea lice infestations in finfish farms (Treasurer and Feledi, 2014; Skiftesvik et al., 2015). Our results indicate a negative response in cuckoo wrasse numbers to the presence of finfish farms in the Frøya regions through either a change in habitat condition or a reduction in sessile epifauna prey within the farm far-field area. This highlights a potential additional pressure from the aquaculture industry, aside from widespread harvesting for cleaner fish purposes, on the cuckoo wrasse population dynamics in regions of high farming intensity.
5 Conclusion
The potential impacts of finfish aquaculture on the coastal ecosystem are wide ranging and complex, and as a result, it is increasingly recognized that an ecosystem-based approach to understanding aquaculture impacts and the inclusion of such knowledge into integrated coastal management plan is required (Barton and Fløysand, 2010; Gentry et al., 2020). An ecosystem-based management approach considers all components of the ecosystem and their interactions with aquaculture (Aguilar-Manjarrez et al., 2010). This involves the construction of food webs through an understanding of trophic connections and the inclusion of knowledge on how aquaculture influences components and connections. The novel results put forward here provide fundamental information on aquaculture-related effects on demersal fish and crustaceans’ spatial dynamics and potential food web changes. These groups are key to coastal ecosystems as well as coastal fisheries across northern Europe. The development of coastal aquaculture has also led to increasing conflict for access to coastal marine resources from both the aquaculture and fisheries industries. The current results clearly demonstrate how finfish farms can impact the local distribution of commercially valuable demersal fish and crustaceans. Changes in spatial distribution will impact the availability of fish and crustaceans to coastal fishers, especially in dense aquaculture regions. It is, however, unclear from these local scale results how finfish aquaculture will impact the overall abundance and distribution of demersal fish and crustaceans in northern European coastal waters.
Data availability statement
The datasets presented in this study can be found in online repositories. The names of the repository/repositories and accession number(s) can be found in the article/Supplementary Material.
Ethics statement
Ethical approval was not required for the study involving animals in accordance with the local legislation and institutional requirements because study took images of wild fish and crustaceans - no removal or contact.
Author contributions
KD: Visualization, Writing – review & editing, Writing – original draft, Supervision, Methodology, Investigation, Formal analysis, Conceptualization. NK: Writing – review & editing, Writing – original draft, Project administration, Methodology, Investigation, Funding acquisition, Conceptualization. IS: Writing – review & editing, Writing – original draft, Visualization, Investigation, Formal analysis.
Funding
The author(s) declare financial support was received for the research, authorship, and/or publication of this article. This work was funded by the Norwegian Research Council under the projects ERA (grant number 228871) and SustainAqua (grant number 267829).
Acknowledgments
This work was received the on-site support of Cermaq Norway AS, Grieg Seafood Finnmark AS, and Mowi Norway AS. The student internship was awarded to IS through the Institute of Marine Research to support her involvement in the project. The Fjord I Nord program at IMR provided funds for the purchase of the BRUVS camera equipment. We would like to thank Raymond Bannister for his role as initial project manager and Astrid Harendza (Akvaplan-niva AS) for contributions to project conceptualization, implementation, and fieldwork. We are grateful to Nils Fanghol for skippering RV Fangst upon which the fieldwork was conducted and the following colleagues at IMR: Erika Gonzales Casal for video annotation, Mona Fuhrmann for assistance in the field, and Terje van der Meeren for help with fish identification.
Conflict of interest
The authors declare that the research was conducted in the absence of any commercial or financial relationships that could be construed as a potential conflict of interest.
Publisher’s note
All claims expressed in this article are solely those of the authors and do not necessarily represent those of their affiliated organizations, or those of the publisher, the editors and the reviewers. Any product that may be evaluated in this article, or claim that may be made by its manufacturer, is not guaranteed or endorsed by the publisher.
Supplementary material
The Supplementary Material for this article can be found online at: https://www.frontiersin.org/articles/10.3389/fmars.2024.1400195/full#supplementary-material
References
Aarstad J., Jakobsen S. E., Fløysand A., Kvitastein O. A. (2023). How Norwegian aquaculture firms across the value chain were affected by and responded to COVID-19. Aquaculture Economics Manage. 28, 1–11. doi: 10.1080/13657305.2023.2251920
Aguilar-Manjarrez J., Kapetsky J. M., Soto D. (2010). The potential of spatial planning tools to support the ecosystem approach to aquaculture (Rome, Italy: FAO/Rome Expert Workshop), 19–21.
Albert O. T. (1994). Ecology of haddock (Melanogrammus aeglefinus L.) in the Norwegian Deep. ICES J. Mar. Sci. 51, 31–44. doi: 10.1006/jmsc.1994.1004
Allen B. M., King P. A., Fives J. M. (2004). Diet of lemon sole Microstomus kitt (Walbaun 1792) in Galway Bay, on the West Coast of Ireland. Irish Naturalists' J. 27, 329–334.
Bailey J. L., Eggereide S. S. (2020). Indicating sustainable salmon farming: The case of the new Norwegian aquaculture management scheme. Mar. Policy 117, 103925. doi: 10.1016/j.marpol.2020.103925
Bailey J., Eggereide S. S., Ellingsgaard K. (2017). Climate change, aquaculture and conflicts of interests in Northern Norway: research results and comparative methodologies (La Paz, California, Mexico: Conference North American Association of Fisheries Economists).
Bannister R. J., Johnsen I. A., Hansen P. K., Kutti T., Asplin L. (2016). Near- and far-field dispersal modelling of organic waste from Atlantic salmon aquaculture in fjord systems. ICES J. Mar. Sci. 73, 2408–2419. doi: 10.1093/icesjms/fsw027
Barton J. R., Fløysand A. (2010). The political ecology of Chilean salmon aquaculture 1982–2010: A trajectory from economic development to global sustainability. Global Environ. Change 20, 739–752. doi: 10.1016/j.gloenvcha.2010.04.001
Bjordal Å, Skar A. B. (1992). Tagging of saithe (Pollachius virens L.) at a Norwegian fish farm: preliminary results on migration (ICES). Bergen: Demersal Fish Committee C.M. 1992/G:35.
Bjørkan M., Eilertsen S. M. (2020). Local perceptions of aquaculture: A case study on legitimacy from northern Norway. Ocean Coast. Manage. 195, 105276. doi: 10.1016/j.ocecoaman.2020.105276
Bostock J., Lane A., Hough C., Yamamoto K. (2016). An assessment of the economic contribution of EU aquaculture production and the influence of policies for its sustainable development. Aquaculture Int. 24, 699–733. doi: 10.1007/s10499-016-9992-1
Boyra A., Sanchez-Jerez P., Tuya F., Espino F., Haroun R. (2004). Attraction of wild coastal fishes to an Atlantic subtropical cage fish farms, Gran Canaria, Canary Islands. Environ. Biol. Fishes 70, 393–401. doi: 10.1023/B:EBFI.0000035435.51530.c8
Brown J. R., Gowen R. J., McLusky D. S. (1987). The effect of salmon farming on the benthos of a Scottish sea loch. J. Exp. Mar. Biol. Ecol. 109, 39–51. doi: 10.1016/0022-0981(87)90184-5
Carss D. N. (1990). Concentrations of wild and escaped fishes immediately adjacent to fish farm cages. Aquaculture 90, 29–40. doi: 10.1016/0044-8486(90)90280-Z
Clarke K. R., Gorley R. N. (2015). Primer v7: User manual/tutorial 3rd edition. Plymouth, United Kingdom: Primer-E Ltd. 1-296.
Dempster T., Fernandez-Jover D., Sanchez-Jerez P., Tuya F., Bayle-Sempere J., Boyra A., et al. (2005). Vertical variability of wild fish assemblages around sea-cage fish farms: implications for management. Mar. Ecol. Prog. Ser. 304, 15–29. doi: 10.3354/meps304015
Dempster T., Sanchez-Jerez P., Bayle-Sempere J. T., Giménez-Casalduero F., Valle C. (2002). Attraction of wild fish to sea-cage fish farms in the south-western Mediterranean Sea: spatial and short-term temporal variability. Mar. Ecol. Prog. Ser. 242, 237–252. doi: 10.3354/meps242237
Dempster T., Sanchez-Jerez P., Bayle-Sempere J., Kingsford M. (2004). Extensive aggregations of wild fish at coastal sea-cage fish farms. Hydrobiologia 525, 245–248. doi: 10.1023/B:HYDR.0000038870.13985.0f
Dempster T., Sanchez-Jerez P., Uglem I., Bjørn P. A. (2010). Species-specific patterns of aggregation of wild fish around fish farms. Estuarine Coast. Shelf Sci. 86, 271–275. doi: 10.1016/j.ecss.2009.11.007
Dempster T., Uglem I., Sanchez-Jerez P., Fernandez-Jover D., Bayle-Sempere J., Nilsen R., et al. (2009). Coastal salmon farms attract large and persistent aggregations of wild fish: an ecosystem effect. Mar. Ecol. Prog. Ser. 385, 1–14. doi: 10.3354/meps08050
Dunlop K. M., Harendza A., Bannister R., Keeley N. (2021). Spatial response of hard and mixed bottom epifauna to organic enrichment from salmon aquaculture in northern Norway. Aquaculture Environ. Interact. 13, 455–475. doi: 10.3354/aei00419
Dunlop K., Harendza A., Plassen L., Keeley N. (2020). Epifaunal habitat association with mixed and hard bottom substrates in coastal waters of northern Norway. Front. Mar. Sci. 7. doi: 10.3389/fmars.2020.568802
Dunlop K. M., Scott E. M., Parsons D., Bailey D. M. (2015). Do agonistic behaviours bias baited remote underwater video surveys of fish? Mar. Ecol. 36, 810–818. doi: 10.1111/maec.12185
Ebner B. C., Starrs D., Morgan D. L. (2014). Emergence of field-based underwater video for understanding the ecology of freshwater fishes and crustaceans in Australia. J. R. Soc. Western Aust. 97, 287–296.
European Environmental Agency. (2021). Aquaculture production in Europe. Available online at: https://www.eea.europa.eu/data-and-maps/indicators/aquaculture-production-4.
Fernandez-Jover D., Arechavala-Lopez P., Martinez-Rubio L., Tocher D. R. (2011). Monitoring the influence of marine aquaculture on wild fish communities: benefits and limitations of fatty acid profiles. Aquaculture Environ. Interact. 2, 39–47. doi: 10.3354/aei00029
Gentry R. R., Alleway H. K., Bishop M. J., Gillies C. L., Waters T., Jones R. (2020). Exploring the potential for marine aquaculture to contribute to ecosystem services. Rev. Aquaculture 12, 499–512. doi: 10.1111/raq.12328
Gerlach S. A., Ekstrøm D. K., Eckardt P. B. (1976). Filter feeding in the hermit crab. Oecologia 24, 257–264. doi: 10.1007/BF00345477
Goodbrand L., Abrahams M. V., Rose G. A. (2013). Sea cage aquaculture affects distribution of wild fish at large spatial scales. Can. J. Fisheries Aquat. Sci. 70, 1289–1295. doi: 10.1139/cjfas-2012-0317
Hersoug B., Mikkelsen E., Osmundsen T. C. (2021). What's the clue; better planning, new technology or just more money? The area challenge in Norwegian salmon farming. Ocean Coast. Manage. 199, 105415. doi: 10.1016/j.ocecoaman.2020.105415
Iversen A., Asche F., Hermansen Ø., Nystøyl R. (2020). Production cost and competitiveness in major salmon farming countries 2003–2018. Aquaculture 522, 735089. doi: 10.1016/j.aquaculture.2020.735089
Jiang W., Jørgensen T. (1996). The diet of haddock (Melanogrammus aeglefinus L.) in the Barents Sea during the period 1984–1991. ICES J. Mar. Sci. 53, 11–21. doi: 10.1006/jmsc.1996.0002
Jones E. G., Tselepides A., Bagley P. M., Collins M. A., Priede I. G. (2003). Bathymetric distribution of some benthic and benthopelagic species attracted to baited cameras and traps in the deep eastern Mediterranean. Mar. Ecol. Prog. Ser. 251, 75–86. doi: 10.3354/meps251075
Keeley N. B., Forrest B. M., Macleod C. K. (2013). Novel observations of benthic enrichment in contrasting flow regimes with implications for marine farm monitoring and management. Mar. pollut. Bull. 66, 105–116. doi: 10.1016/j.marpolbul.2012.10.024
Keeley N., Laroche O., Birch M., Pochon X. (2021). A substrate-independent benthic sampler (SIBS) for hard and mixed-bottom marine habitats: A proof-of-concept study. Front. Mar. Sci. 8. doi: 10.3389/fmars.2021.627687
Keeley N., Valdemarsen T., Strohmeier T., Pochon X., Dahlgren T., Bannister R. (2020). Mixed-habitat assimilation of organic waste in coastal environments–It's all about synergy! Sci. Total Environ. 699, 134281. doi: 10.1016/j.scitotenv.2019.134281
Keeley N., Valdemarsen T., Woodcock S., Holmer M., Husa V., Bannister R. (2019). Resilience of dynamic coastal benthic ecosystems in response to large-scale finfish farming. Aquaculture Environ. Interact. 11, 161–179. doi: 10.3354/aei00301
Kutti T., Hansen P. K., Ervik A., Høisæter T., Johannessen P. (2007). Effects of organic effluents from a salmon farm on a fjord system. II. Temporal and spatial patterns in infauna community composition. Aquaculture 262, 355–366. doi: 10.1016/j.aquaculture.2006.10.008
Lambert G., Nielsen J. R., Larsen L. I., Sparholt H. (2009). Maturity and growth population dynamics of Norway pout (Trisopterus esmarkii) in the North Sea, Skagerrak, and Kattegat. ICES J. Mar. Sci. 66, 1899–1914. doi: 10.1093/icesjms/fsp153
Langlois T., Goetze J., Bond T. (2020). A field and video annotation guide for baited remote underwater stereo-video surveys of demersal fish assemblages. Methods Ecol. Evol. 11, 1401–1409. doi: 10.1111/2041-210X.13470
Machias A., Karakassis I., Giannoulaki M. (2005). Response of demersal fish communities to the presence of fish farms. Mar. Ecol. Prog. Ser. 288, 241–250. doi: 10.3354/meps288241
Mente E., Pierce G. J., Spencer N. J. (2008). Diet of demersal fish species in relation to aquaculture development in Scottish sea lochs. Aquaculture 277, 263–274. doi: 10.1016/j.aquaculture.2008.02.022
Olaussen J. O. (2018). Environmental problems and regulation in the aquaculture industry. Insights from Norway. Mar. Policy 98, 158–163. doi: 10.1016/j.marpol.2018.08.005
Öndes F., Kaiser M. J., Murray L. G. (2017). Relative growth and size at onset of sexual maturity of the brown crab, Cancer pagurus in the Isle of Man, Irish Sea. Mar. Biol. Res. 13, 237–245. doi: 10.1080/17451000.2016.1248849
Parsons A. E., Escobar-Lux R. H., Sævik P. N., Samuelsen O. B., Agnalt A. L. (2020). The impact of anti-sea lice pesticides, azamethiphos and deltamethrin, on European lobster (Homarus gammarus) larvae in the Norwegian marine environment. Environ. pollut. 264, 114725. doi: 10.1016/j.envpol.2020.114725
R Core Team. (2022). R: A language and environment for statistical computing. (Vienna, Austria: R Foundation for Statistical Computing). Available at: https://www.R-project.org/.
Ramsay K., Kaiser M. J., Hughes R. N. (1996). Changes in hermit crab feeding patterns in response to trawling disturbance. Mar. Ecol. Prog. Ser. 144, 63–72. doi: 10.3354/meps144063
Rijnsdorp A. D., Vingerhoed B. V. (2001). Feeding of plaice Pleuronectes platessa L. and sole Solea solea (L.) in relation to the effects of bottom trawling. J. Sea Res. 45, 219–229. doi: 10.1016/S1385-1101(01)00047-8
Salvanes A. G. V., Nordeide J. T. (1993). Dominating sublittoral fish species in a west Norwegian fjord and their trophic links to cod (Gadus morhua L.). Sarsia 78, 221–234. doi: 10.1080/00364827.1993.10413536
Samuelsen O. B., Parsons A. E., Agnalt A. L., Tjensvoll T., Lunestad B. T., Hannisdal R. (2020). Mortality in the rockpool shrimp Palaemon elegans following long-term exposure to low doses of the anti-parasitic drug teflubenzuron. Aquaculture Environ. Interact. 12, 23–29. doi: 10.3354/aei00343
Skiftesvik A. B., Durif C. M., Bjelland R. M., Browman H. I. (2015). Distribution and habitat preferences of five species of wrasse (Family Labridae) in a Norwegian fjord. ICES J. Mar. Sci. 72, 890–899. doi: 10.1093/icesjms/fsu211
Skjæraasen J. E., Bergstad O. A. (2000). Distribution and feeding ecology of Raja radiata in the northeastern North Sea and Skagerrak (Norwegian Deep). ICES J. Mar. Sci. 57, 1249–1260. doi: 10.1006/jmsc.2000.0811
Skjæraasen J. E., Karlsen Ø., Langangen Ø., Meier S., Dunlop K. M., van der Meeren T., et al. (2022). Attraction of cod Gadus morhua from coastal spawning grounds to salmon farms. Aquaculture Environ. Interact. 14, 229–242. doi: 10.3354/aei00440
Standards Norway. (2013). Water quality:guidelines for quantitative sampling and sample processing of marine soft-bottom macrofauna. NS 16665: 2014. Oslo: Standard Norge.
Tanner J. E., Williams K. (2015). The influence of finfish aquaculture on benthic fish and crustacean assemblages in Fitzgerald Bay, South Australia. PeerJ 3, e1238. doi: 10.7717/peerj.1238
Torbjørn L. (2008). Modeling climate change and the effect on the Norwegian salmon farming industry. Natural Resource Modeling 21, 416–435. doi: 10.1111/j.1939-7445.2008.00018.x
Treasurer J., Feledi T. (2014). The physical condition and welfare of five species of wild-caught wrasse stocked under aquaculture conditions and when stocked in Atlantic salmon, Salmo salar, production cages. J. World Aquaculture Soc. 45, 213–219. doi: 10.1111/jwas.12099
Tuya F., Sanchez-Jerez P., Dempster T., Boyra A., Haroun R. J. (2006). Changes in demersal wild fish aggregations beneath a sea-cage fish farm after the cessation of farming. J. Fish Biol. 69, 682–697. doi: 10.1111/j.1095-8649.2006.01139.x
Uglem I., Dempster T., Bjørn P. A., Sanchez-Jerez P., Økland F. (2009). High connectivity of salmon farms revealed by aggregation, residence and repeated movements of wild fish among farms. Mar. Ecol. Prog. Ser. 384, 251–260. doi: 10.3354/meps08001
Woll A. K., van der Meeren G. I., Fossen I. (2006). Spatial variation in abundance and catch composition of Cancer pagurus in Norwegian waters: biological reasoning and implications for assessment. ICES J. Mar. Sci. 63, 421–433. doi: 10.1016/j.icesjms.2005.10.004
Woodcock S. H., Strohmeier T., Strand Ø., Olsen S. A., Bannister R. J. (2018). Mobile epibenthic fauna consume organic waste from coastal fin-fish aquaculture. Mar. Environ. Res. 137, 16–23. doi: 10.1016/j.marenvres.2018.02.017
Zeileis A., Kleiber C., Jackman S. (2008). Regression models for count data in R. J. Stat. Software 27, 1–25. doi: 10.18637/jss.v027.i08
Zintzen V., Anderson M. J., Roberts C. D., Harvey E. S., Stewart A. L., Struthers C. D. (2012). Diversity and composition of demersal fishes along a depth gradient assessed by baited remote underwater stereo-video. PloS One 7, e48522. doi: 10.1371/journal.pone.0048522
Zuur A. F., Ieno E. N. (2016). A protocol for conducting and presenting results of regression-type analyses. Methods Ecol. Evol. 7, 636–645. doi: 10.1111/2041-210X.12577
Keywords: demersal fish, marine crustaceans, aquaculture, baited underwater cameras, coastal zone management
Citation: Dunlop K, Strammer I and Keeley N (2024) Attraction and avoidance of wild demersal fish and crustaceans to open-net aquaculture pens resolved by baited and towed underwater camera surveys. Front. Mar. Sci. 11:1400195. doi: 10.3389/fmars.2024.1400195
Received: 13 March 2024; Accepted: 11 June 2024;
Published: 01 August 2024.
Edited by:
Jie Cao, North Carolina State University, United StatesReviewed by:
José Pedro Andrade, University of Algarve, PortugalJosé Lino Vieira De Oliveira Costa, University of Lisbon, Portugal
Copyright © 2024 Dunlop, Strammer and Keeley. This is an open-access article distributed under the terms of the Creative Commons Attribution License (CC BY). The use, distribution or reproduction in other forums is permitted, provided the original author(s) and the copyright owner(s) are credited and that the original publication in this journal is cited, in accordance with accepted academic practice. No use, distribution or reproduction is permitted which does not comply with these terms.
*Correspondence: Katherine Dunlop, a2F0aGVyaW5lLm1hcnkuZHVubG9wQGhpLm5v