- 1Norwegian Institute for Water Research (NIVA), Oslo, Norway
- 2Shirshov Institute of Oceanology, Russian Academy of Sciences, Moscow, Russia
Little is known about the role of remote and sparsely populated Arctic coastal zones in the microplastic cycle. Distribution of microplastics was studied in the Svalbard fjords in June – July 2022 with the main goal of assessing rivers’ role in the fate of microplastic in Arctic coastal waters. Surface microplastics (0 – 20 cm depth, 500 – 5000 µm size) were sampled with a neuston net in triplicate per study site in parallel with sampling of subsurface microplastics with a pump system (1.5 m depth, 100 – 5000 µm size). The central part of Isfjorden and its several branches covering populated and unpopulated fjords were studied; the sampling was conducted during an intense riverine discharge in all studied sites. Maximum abundance of surface microplastics (71,400 items/km2 or 0.19 iterms/m3, 0.19 mg/m3) was found along the river plume border in the middle of populated Adventfjorden indicating importance of both local sources and surface hydrodynamics in the formation of microplastics accumulation hotspots. All other unpopulated fjords were free of the floating on the sea surface microplastics as river discharge prevented transport of microplastics inside the fjords. The highest concentration of subsurface microplastics was found in the central part of Isfjorden and the lowest – in river plume waters, which also indicates the removal of microplastics from the inner part of fjords during an intensive river discharge. Our results may suggest that Arctic rivers flowing through unpopulated areas bring clean water and thereby reduce level of microplastic pollution in the coastal waters. In contrast to the rest of the world’s ocean, rivers are not the main source of microplastic pollution in the Arctic Ocean.
Introduction
Microplastic pollution of the world’s oceans is a serious environmental problem. According to 2013 data, 4.8 to 12.7 million tons of plastic enter the world’s oceans annually (Cózar et al., 2017; Jambeck et al., 2015). Once in the World Ocean, plastic items break down and are able to spread over long distances with ocean currents, settle on beaches and on the seabed (Woodall et al., 2014), as well as accumulate in the ocean in closed circulation zones (Lusher, 2015). Microplastic particles are found in the entire water column from the surface to the bottom, in ice cores (Kanhai et al., 2020; Obbard et al., 2014), in marine organisms (Fang et al., 2018; Gebruk et al., 2022).
However, there is still no consensus on the main sources of microplastics, distribution mechanisms, and long-term forecasts of impacts on various ecosystems, especially for remote regions such as the Arctic (AMAP, 2021; PAME, 2019). Currently, various studies consider the following sources of microplastic in the Arctic. The most significant contribution is made by the waters of the Atlantic Ocean (Berezina et al., 2023; Cózar et al., 2017; Ross et al., 2021). Other possible sources are the inflow from the Pacific Ocean (Ikenoue et al., 2023b, 2023a), spread of microplastics due to the dynamics of ice formation (Peeken et al., 2018), atmospheric transport (Evangeliou et al., 2020), shipping activities and transport with rivers (Frank et al., 2021; Mani et al., 2019; Yakushev et al., 2021; Zhdanov et al., 2022).
It is believed that most of the plastic in the oceans, 80%, comes from rivers, 0.41–4 million tons of plastic enter the world ocean annually (Lebreton et al., 2017; Lechner et al., 2014; Schmidt et al., 2017). The amount of microplastics supplied from rivers depends on the population density of the catchment area and the volume of river discharge. Rivers flowing into the Arctic Ocean account for 11% of freshwater discharges into the world’s oceans, and most have low population densities in their catchments. The biggest Arctic rivers, Great Siberian Rivers (Ob, Yenisei, Lena) annually discharge more than 2000 km3 of water in total. However, major Siberian cities are located quite far from river mouths, the level of plastic pollution in these rivers decreased downstream (Frank et al., 2021; Vorobiev et al., 2023). Moreover, on the sea shelfs there was found less microplastics in river plumes than in the surrounding seawater (Berezina et al., 2023; Yakushev et al., 2021). This testify that populated Arctic rivers are not the main source of plastic pollution in the Arctic Ocean.
Little is known about microplastic fate in low populated regions in the Arctic including Arctic islands. One of the most studied of them is the Svalbard Archipelago located in the western part of the Arctic Ocean, about 800 km away from mainland Europe. Although studies of microplastic levels in different matrices are conducted regularly in Svalbard (Bao et al., 2022; Carlsson et al., 2021; Choudhary et al., 2022; Granberg et al., 2020; Iannilli et al., 2019; Lloyd-Jones et al., 2023; Ramasamy et al., 2021; Singh et al., 2021; Teichert et al., 2021), there are still significant gaps in understanding the role of Arctic coastal zones in the microplastic cycle. Svalbard is sparsely populated with only a few permanent settlements (Longyearbyen, Hornsund, Sveagruva, Ny Ålesund and Barentsburg). The main sources of pollution in these areas originate from household and industrial activities including shipping, tourism, and fishing (Singh et al., 2021). Additionally, atmospheric transport and ocean currents can introduce microplastics from the remote regions to Svalbard waters (Bergmann et al., 2019; Evangeliou et al., 2020; Ruman et al., 2012). Svalbard fjords with rugged coastline, lots of lagoons and river estuaries act as centres of plastic accumulation (Harris, 2020; Pakhomova et al., 2024). Fjords in Svalbard serve as transition zones between freshwater and marine environments, making them key sites for studying the mechanisms of microplastic transfer and accumulation. This makes Svalbard a unique area for microplastic fate research in Arctic estuaries.
This research aimed to study the fate of microplastics in Arctic coastal waters using Svalbard as an example. Specifically, the goal was to assess the influence of riverine discharge on the distribution and amount of microplastics in fjord waters both on the sea surface and in the subsurface water (1 – 2 m depth). Studies were performed in populated and unpopulated fjords to trace the effect of local sources of pollution. The relevance of this study lies in its harmonized approach to the investigation of microplastics on Svalbard. Unlike previous research, we conducted parallel measurements of both surface and subsurface waters, using a three-replicate net sampling method. Additionally, we focused on the boundary of the river plume, a critical area for understanding the distribution and concentration of microplastics in the fjords.
Materials and methods
Sampling
Sampling was conducted in Svalbard fjords between June 28 – July 2, 2022. Nine polygons were studied in Isfjorden (IF) and its branches (Figure 1). Two polygons were located in Isfjorden main part: IF1 and IF2 at southern and northern sides of the fjord representing incoming and outcoming water in Isfjorden. The only settlement located in studied area is Svalbard’s main town, Longyearbyen, which has about 2450 inhabitants and is located on southwestern shore of Adventfjorden (AF). Adventfjorden is exposed to river runoff by two rivers: Adventelva (unpopulated) and Longyearelva (flows through the city). The wastewater outflow is located close to the northern coast of the fjord at approximately 50 m depth, near the seabed. In Adventfjorden samples were taken at three polygons: AF1 in the inner part of fjord around the river plume border; AF2 and AF3 in the eastern and western sides of outer part of the fjord, respectively. AF3 polygons was close to the city port area.
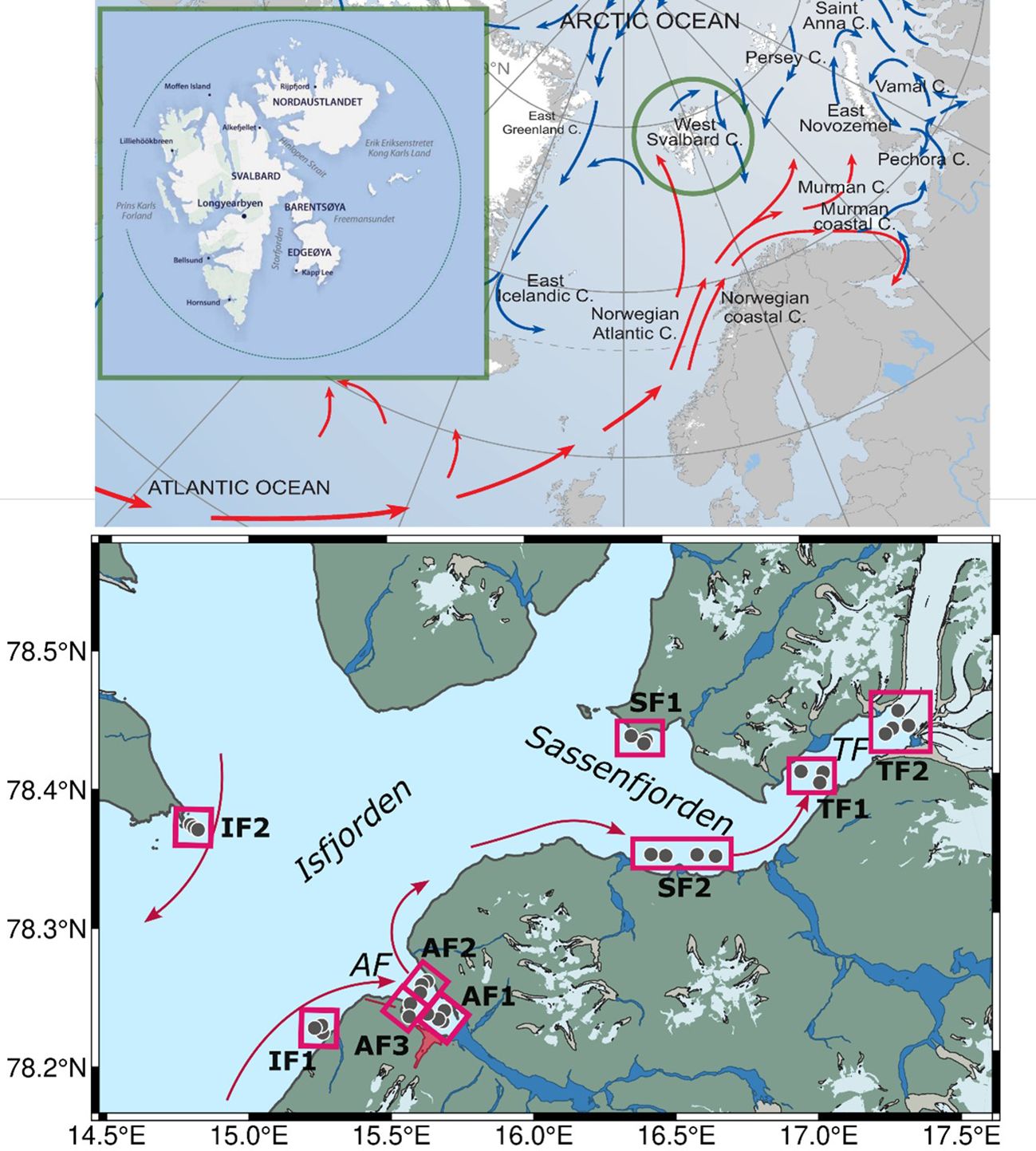
Figure 1. Study site. Upper panel: map of the Arctic Ocean currents (adapted from http://www.arcticportal.org) and a simplified map of Svalbard Archipelago. Lower panel: map of Isfjorden; red squares represent studied polygons that consisted of 2-4 net transects on the sea surface (grey circles) with simultaneous pumping of water from the subsurface. Currents inside Isfjorden are shown by arrows, modified from (Skogseth et al., 2020). Areas of Longyearbyen city and Svalbard airport are marked on the map with red colour. AF, Adventfjorden; TF, Tempelfjorden.
Sassenfjorden (SF) is a branch of Isfjorden next to Adventfjorden. Several small rivers flow into SF from the south and the north, and the large Sassenelva river from the south-east. Two polygons were studied in SF: the outer part close to the northern coast (SF1) and along the southern coast (SF2). Tempelfjorden (TF) is a branch of the Isfjorden in the inner part of Sassenfjorden (Figure 1). Numerous streams and the Murdochelva River, which originates in the glaciers of Bünsow Land, flow into the fjord. The sampling sites were located in the outer fjord (TF1) and the inner part of the fjord (TF2), between the Tunabreen glacier and Murdochelva River. There are no permanent settlements in Sassenfjorden and Tempelfjorden.
According to typical cyclonic water circulation in the Isfjord system (Skogseth et al., 2020), water will flow in the following sequence for the stations being studied IF1 – AF3 – AF1 – AF2 – SF2 – TF1 – TF2 – SF1 – IF2 (Figure 1). However, wind direction can alter this circulation of the surface layer.
Sampling was carried out on the boat Polarcirkel, equipped with a manual winch. Each studied polygon consisted of 2-4 net transects on the sea surface with simultaneous pumping of water from the subsurface layer (for about 1 hour) and covered a certain area of the fjord.
Microplastics floating on the sea surface were sampled using a neuston net (40 x 60 cm opening, nylon material, mesh size 0.333 mm) trawled across the sea surface at low vessel speed (2–3 knots) for approximately 20-30 minutes. After trawling, the net was washed externally with filtered seawater and the contents of the cod end were rinsed with filtered water (0.45 µm filter) into a clean glass jar with a lid. The jars with the suspensions were transferred to the NIVA laboratory for further analysis. A calibrated flow meter General Oceanics was used to measure the exact volume of filtered water per sample, which varied in range 70–349 m3 (Supplementary Table S1).
Subsurface microplastics were collected by filtering seawater using a flow-through system with a water intake located at a depth of approximately 1.5 m on the left side of the boat. Subsurface seawater was passed under pressure through stainless steel mesh filter (100 µm pore size) within the filtration system. The system consisted of first step water appliance protective systems and food grade PVC pipes. The Decast Metronic flow meter integrated into the system provided an accurate recording of the water volume for each sample, which varied from 0.13 to 0.46 m3 per sample. After sampling, filters were sealed in pre-rinsed 500 mL jars (with pre-filtered 0.45 μm pore size water). The jars were stored until the analysis in the onshore clean laboratory at NIVA.
In addition, during sampling, the water temperature was continuously measured through a flow system at a depth of 1.5 m using PyroScience optical sensor FireSting-PRO.
Sample handling and laboratory analysis
No processing of net samples was performed prior to analysis. The collected suspension was washed with filtered water from jars into a plastic basin for initial visual analysis (Lusher et al., 2020) for the presence of microplastics (500 – 5000 µm) in the sample. After visual sorting was completed, the sample was washed onto a metal sieve with a mesh size of 500 µm for final inspection. For visual sorting, a lamp with an illuminated magnifying glass was used. Potential plastic particles were stored in Eppendorf tubes prior to analysis at NIVA laboratory, where all particles were photographed on graph paper, described (colour, shape), and size was measured using INFINITY ANALYZE and CAPTURE software (length, width and particle area). All particles were individually weighed on a microbalance (Sartorius SE2-F) before the identification step.
Pump samples were processed in the clean NIVA laboratory to remove organic matter with 10% KOH (24hr incubation at 40°C and 100 rpm (Bråte et al., 2018)) in the same jars where the filters were stored after sampling. The processed samples were filtered onto 47 mm GF/A papers with 1.6 μm pore size. The filter with material was immediately transferred to a petri dish and covered for drying and further analysis. Visual sorting for the presence of microplastics (100 – 5000 µm) in the sample was performed for the entire filter area using a dissecting microscope Nikon SMZ745. Potential plastic items were pictured, and size of items was measured using Infinity 1-3C/INFINITY 1 Lumenera camera and INFINITY ANALYZE and CAPTURE software.
Identification of chemical composition of all potential plastic items selected under visual sorting was made using a Fourier Transform Infrared spectroscopy (FT-IR) analysis on PerkinElmer Spotlight 400 FTIR. All particles were measured individually with Frontier ATR – for surface samples and transmission µFTIR with DCC – for subsurface samples. Measurements were obtained at 4 cm-1 spectral resolution for the range 4000 to 600 cm-1. Library matching was performed in the Spectrum 10 software (v. 10.6.2). Each spectrum was compared to several different libraries available at the Norwegian Institute for Water Research (NIVA): PerkinElmer ATR Polymers library, BASEMAN library (Primpke et al., 2018), and several in-house libraries including reference polymers, different textile materials, and potential sources of laboratory contamination. All spectra were manually inspected (expert knowledge) to ensure that all spectra showed characteristic peaks of polymers and the library matches were acceptable (>0.8).
Quality assurance and quality control
To control and minimise external contamination, the following steps were performed. The neuston net was rinsed from outside with seawater before each sampling. All equipment including filtration system, metal filters, glass jars, etc. were rinsed with pre-filtered distilled water (0.45 µm) before use. GF/A filters were checked under a microscope for contamination prior to use. The samples and used equipment were covered where practically possible with aluminium foil or glass to minimise periods of exposure. Personal protective equipment, 100% cotton lab coats, and gloves were worn during the whole processing procedure. All procedures in the laboratory were conducted in a clean airflow cabinet (Labculture LA2-5A1-E). The composition of all plastic materials used during sampling and analysis was identified and considered during analysis (material of the net, filtration system, hoses, boat, laboratory equipment, clothes).
Field blanks for neuston net sampling method were performed in triplicate by washing the net with cod end from outside followed by analysis of cod end content filtered on GF/A filter. More than 50 textile fibres were found in each field blank but not fragments. Therefore, textile fibres were excluded from the analysis of net samples due to the challenges of controlling for this contamination during field sampling. Technical fibres (solid lines, made of PE, PP and PA, i.e. fishing lines or ropes originated from maritime activities) were included in the dataset. For control of airborne contamination during sample processing and analysis, a wetted GF/A filter was exposed in an open glass Petri dish close to the sample during processing and analysed for foreign plastic particles. Given that no contamination was observed within the target size range and morphology, no data correction of net sample results was performed.
To control contamination during sampling and processing of pump samples, four field blanks were taken (one per sampling day). Specifically, field blanks were conducted in between sampling stations using the same procedures as for sampling, with the exception of seawater pumping while the filters were in the filtration system. All blanks were processed and analysed for microplastics in the same way as the samples including visual sorting under microscope and FTIR analysis. A total of 8–10 fibres per field blank were found. No fragments were found in the field blanks. All fibres detected in the blanks were confirmed as cellulose and viscose with the exception of two acrylic and two polyester fibres. Since all cellulose and viscose were excluded from the data set (not presumed to be plastic), no data correction for these items was needed. No similar acrylic and polyester fibres (colour, size) were found in the corresponding samples, so no correction was made. To assess the potential error in microplastic analysis due to contamination, limits of detection and quantification (LOD and LOQ) were calculated from blank samples and compared to the number of items found (Supplementary Figure S1; Alling, 2023).
To control the quality of the analysis, positive procedural controls (recovery tests) were performed in the laboratory. In case of net samples, a standard material of three polymers (polyethylene, polypropylene, and polystyrene, in form of spheres and fragments, size 500 – 3000 µm, 24 items) were added manually to the cod ends containing some marine suspended matter. In case of pump samples, a standard reference material in form of soda tablets (Martínez-Francés et al., 2023) containing three polymers (polyethylene, polyvinylchloride, and polystyrene, 106 items, size 150 – 350 µm) were added to the 1L bottles containing suspended material washed from the pump system filter (Alling, 2023; van Bavel, 2022). This was performed in 3 replicates for each method to ensure method validity. All analysis steps were followed according to the field samples analysis, including visual sorting under microscope and µFTIR measurements. Validation of method used for particle extraction showed recovery rates 100% and 93% for net and pump samples respectively and 100% correct polymer type identification. The methods were deemed appropriate for the investigation.
Data analysis
The Python 3 packages for data analysis (scipy, pandas) were used to process the raw field data and calculate main statistics for datasets (mean, standard deviation, median, percentiles). Maps were plotted with QGIS 3.28 geographic information system and were edited with Inkscape graphics software. The Levene test was applied to test the hypothesis that all input samples are from populations with equal variances. The t-test for independent samples was used to quantify the difference between the arithmetic means of the samples in Adventfjorden.
Microplastics concentrations were calculated in different units, in items/km2, items/m3 and mg/m3, so that they can be easily compared with the results of other studies. Area covered with the net transect was calculated in m2 and then converted to km2 with a simple formula: , where L is a transect length and W is the net opening width (60 cm). The volume of the filtered water (V) was then calculated taking that 20 cm (H) of the net opening was submerged: V = LH. Concentrations were also calculated for different size ranges 100 – 300 µm, 300 – 1000 µm, 1000 – 5000 µm (Supplementary Table S2) and separate for fragments and fibres for subsurface samples (Table 1). LOD and LOQ were calculated as the mean number of microplastics in the blanks + 3 and 10 x standard deviation, respectively.
According to (Kukulka et al., 2012) turbulent downward flux of plastic pieces is significant for moderate wind conditions (>5 m/s). During sampling in Isfjorden, the weather was calm with wind speeds of 0 – 7 m/s in the open part and <4 m/s in the inner parts of the fjord. Wave heights were less than 10 – 20 cm and the resulting vertical mixing was expected to be limited. Therefore, no correction of surface microplastic concentration for influence of vertical mixing was made.
Particles from subsurface layer are much lower in size than from surface layer and it is impossible to weight them. Therefore, the mass of subsurface microplastics was estimated based on the polymer density and volume of every particle, assuming that all items are ellipsoid cylinders with measured length and width, and the items thickness was calculated as a function of the width depending on size fraction (Alling, 2023).
Results
Net samples (sea surface)
Microplastics were found in 11 of 29 net samples. Eighteen net samples were free from microplastics. In total, 143 microplastic particles in size range 500 – 5000 µm confirmed by FTIR analysis were found in the net samples. The most of the microplastic items were identified as polyethylene (PE, 67.8%) followed by polypropylene (PP,16.1%), expanded polystyrene (EPS, 14.7%), polyamide (PA, 0.7%) and polyvinyl chloride (PVC, 0.7%) (Figure 2). The majority of found items were fragments (95%), only 7 technical fibres (solid lines) were found in Adventfjorden.
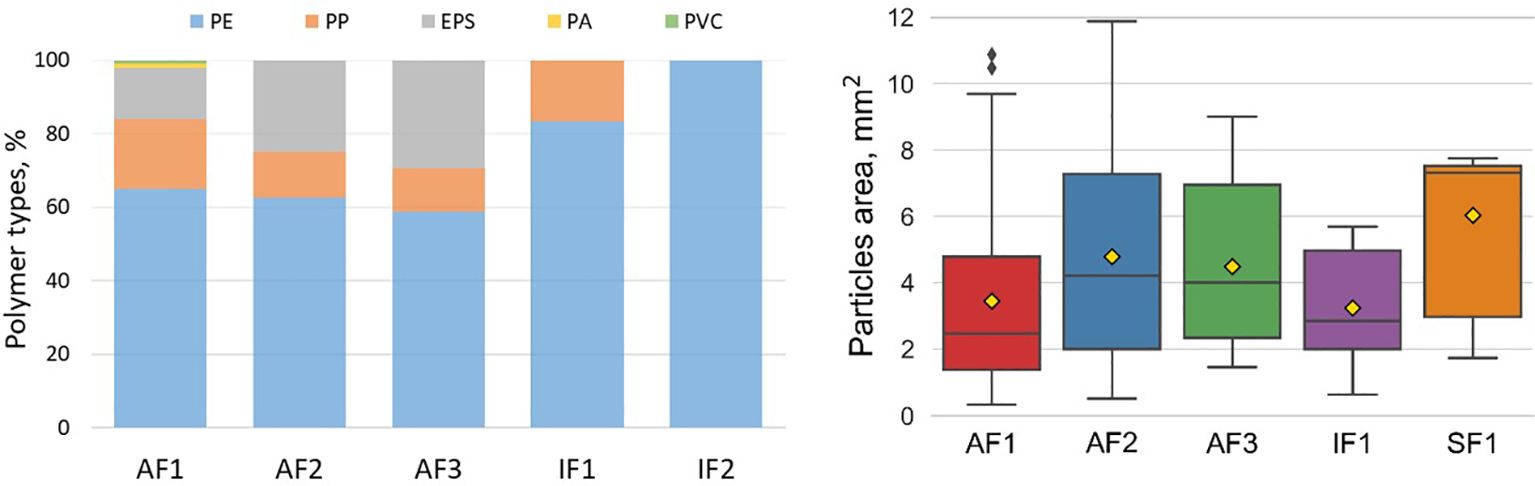
Figure 2. Microplastic polymer composition (left) and size (particles area, right) on the sea surface (net samples) at different polygons (size range 500 – 5000 µm).
Microplastic abundance in the studied area varied from 0 to 71,400 items/km2 per transect (0 – 33 particles, see more details in Supplementary Table S1; see Table 1 for concentration in volume units). No microplastics were found in inner and outer Tempelfjorden, northern Isfjorden and southern Sassenfjorden (TF1, TF2, IF1, SF2). Microplastics were found in one of three transects in northern Sassenfjorden (SF1) and southern Isfjorden (IF1), 4 and 12 particles were found respectively, that resulted in average MPs abundance for three transects 1200 for SF1 and 3300 items/km2 for IF1 polygons. In Adventfjorden (AF) microplastics were found in all transects with a maximum in the inner part of AF at the very defined border of the river plume (16,800 – 71,400 items/km2, Figure 3). This resulted in an average surface microplastics concentration for inner part of Adventfjorden (AF1) 38,300 items/km2. Five different polymer types were found at station AF1: PE, PP, EPS, PA, and PVC that was the highest diversity in the studied area. In the outer part of AF 4 – 8 times less concentrations were found, average was 4800 items/km2 in the eastern part (AF2) and 9400 items/km2 in the western part (AF3).
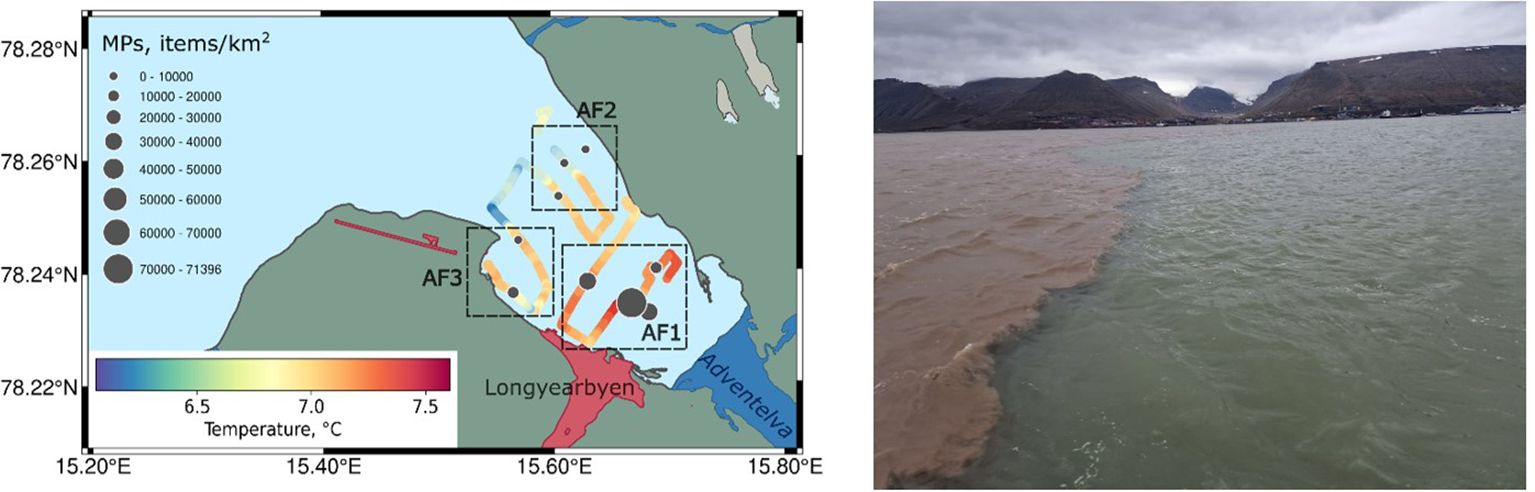
Figure 3. Abundance of surface microplastics (black dots, items/km2) at each net transect (coloured lines representing temperature) in Adventfjorden (left) and river plume border during sampling in Adventfjorden (right, Longyearbyen view).
Distribution of MPs mass concentrations followed the same tendency as for MPs abundance varying between 31.1 – 44.0, 3.8 – 11.7, 9.6 – 19.2, 0 – 10.5 and 0 – 7.0 mg/km2 with average 37.2, 7.6, 14.4, 3.5 and 2.3 mg/km2 for AF1, AF2, AF3, IF1, and SF1, respectively (see Table 1 for concentration in volume units). In most cases the ratio between mass concentration and abundance was above 1 ranging from 1 to 2.5 except for one net transect at AF1 where the ratio dropped down to 0.4 – transect at the river plume border, which had maximum MPs abundance. This was a result of difference in particle size at this transect in comparison with all other transects: 2.1 ± 1.8 mm2 and 4.7 ± 1.3 mm2 for particles area (2D dimension), respectively. However, no significant difference in the average particle area was found for the studied polygons according to t-test (p > 0.05) (Figure 2).
Pump samples (subsurface)
Totally, 310 potential plastic particles in size range 100 – 5000 µm were found under visual analysis in 9 samples of subsurface water. Only 101 were confirmed to be plastic after FTIR analysis ranging from 4 to 19 particles per sample (see details in Supplementary Table S1). 47% of items were represented by fragments and 53% – by fibres (Figure 4). The majority of the microplastic items were identified as polyethylene terephthalate (PET, 25.7%) followed by polyamide (PA, 19.8%), polyethylene (PE, 16.8%) polypropylene (PP,15.8%), polyacrylic (Acryl, 12.9%), polystyrene (PS, 5.0%), and polyvinyl chloride (PVC, 1%), polytetrafluoroethylene (PTFE, 1%), ethylene propylene (Other, 1%), polymethyl methacrylate (Other, 1%) (Figure 4).
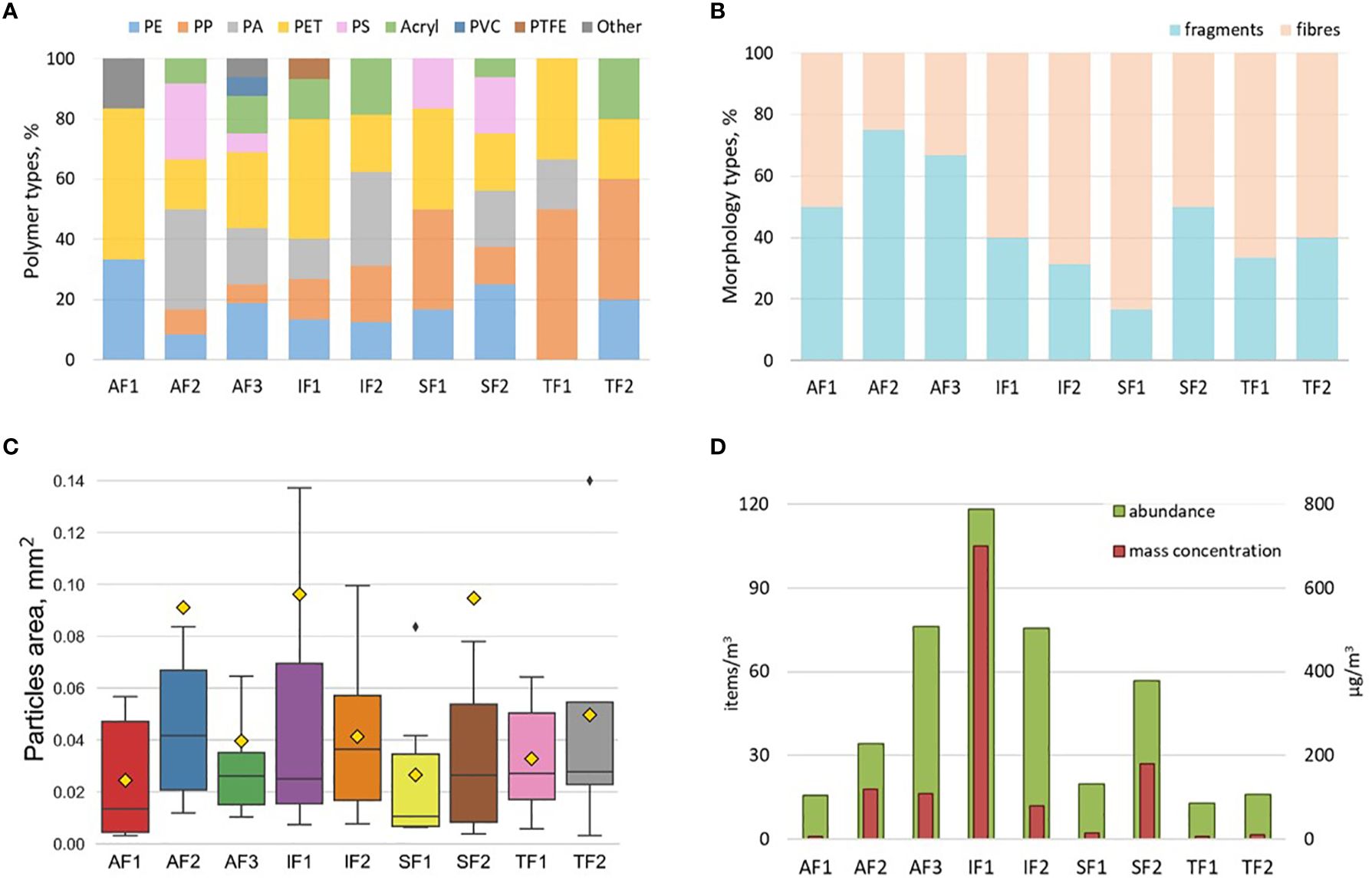
Figure 4. Microplastic’s polymer composition (A), morphology (B), size (C) and concentration (D) in subsurface layer (pump samples) at different stations (size range 100 – 5000 µm).
Microplastics in subsurface layer were observed at all studied sites. The number of items found ranged from 4 to 16 per sample, which was above the LOD value for all samples but below the LOQ for four samples (AF1, SF1, TF1 and TF2). The abundance varied from 12.9 to 118.1 items/m3 and mass concentration – from 0.007 to 0.70 mg/m3 (Table 1; Figure 4). Minimum number of MPs was found in the inner parts of fjords, at AF1, TF1, TF2 and in the northern part of Sassenfjorden at SF1 (13.0 – 19.9 items/m3, 0.006 – 0.016 mg/m3). These stations were characterized by the lowest diversity of polymer types of microplastics in the samples (3 – 4 types). Maximum abundance of MPs was found at two stations in central part of Isfjorden followed by Adventfjorden outer part and southern part of Sassenfjorden, 118.1, 75.5, 76.2, and 56.7 items/m3 at IF1, IF2, AF3, and SF2 respectively. Mass concentration had a maximum at southern station in Isfjorden, IF1, 0.70 mg/m3 and varied between 0.08 – 0.16 mg/m3 at stations IF2, AF2, AF3 and SF2. The highest diversity of polymer types was found in the southern part of outer Adventfjorden, AF3 (8 types, Figure 4). Fragments were most abundant in outer Adventfjorden, 75% and 62.5% for AF2 and AF3 respectively, decreasing to 50% at AF1 and SF2. Fibers dominated at all other stations, IF1, IF2, SF1, TF1, TF2 (Figure 4).
Discussion
Microplastics on the sea surface
During the sampling period, there were two opposing water flows in Adventfjorden: high saline water inflow to the fjord driven by WSW wind, which was preferable in June, and an intensive freshwater discharge from Adventselva and Longyearelva rivers in the inner part of the fjord (Table 2). Looking for the shorter time period, WSW wind was observed during six days before sampling (92% of time and 8% – no wind) that allowed to form well defined river plume border (Table 2; Figure 3). Such borders act as an accumulation spot for all floating items in the ocean (Malli et al., 2022). Sampling for surface microplastics at AF1 was performed along the river plume border and resulted in the highest concentration of microplastics (up to 71,400 items/km2) as well as the greatest diversity of polymer types found in the studied area (t-test, p=0.01). During our studies in summer 2021, carried out using exactly the same methods (Pakhomova et al., 2024), SWS-SW wind was observed 67% of time during six days before sampling while other 33% there was SES wind. As a result, the river plume in Adventfjorden was not well defined, leading to a different spatial distribution of microplastics in Adventfjorden and lower concentrations (Pakhomova et al., 2024). This highlights the significance of small-scale hydrodynamics to microplastic transfer, distribution and to the formation of microplastics accumulation hotspots.
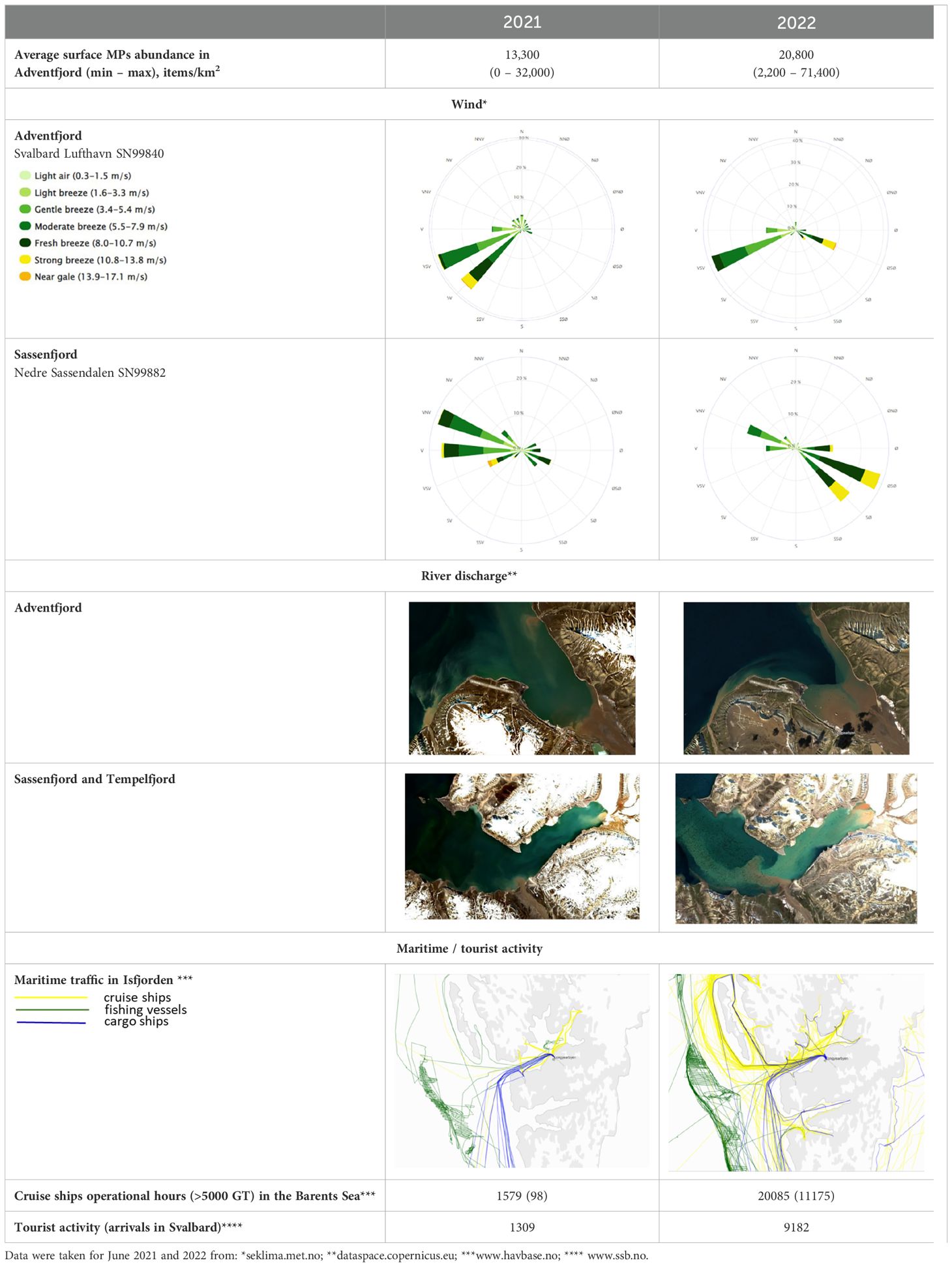
Table 2. Microplastic abundance, hydrodynamical conditions and maritime/tourist activity in June 2021 and 2022 in studied area.
Microplastic concentrations in Adventfjorden in 2022 (average 20,800 items/km2) are for the second year the highest observed in our previous studies using the same methods for the open ocean in the Eurasian Arctic, the open Central Atlantic and Northwest Pacific (average 1000–8800 items/km2; Supplementary Table S3 (Berezina et al., 2023; Pakhomova, 2023; Yakushev et al., 2021; Zhdanov et al., 2023; Zhdanov and Polivanova, 2024)). This may indicate a possible accumulation of microplastics in the fjord. Microplastics from different sources can be accumulated at plume border in Adventfjorden: transport from the North Atlantic, port and tourist activities, coastal and river discharge with Longyearelva, which flows through the city. The existing data set does not allow to make any conclusion about the main source of microplastics in Adventfjorden, while presence of EPS only in samples from Advenfjorden can justify that it is of local origin.
According to the typical water circulation in Adventfjorden, station AF3 reflects microplastics inflow into Adventsfjorden, but may also be affected by local port activity. Station AF2 represents the outflow of microplastics from Adventfjorden. Concentration of microplastics at station AF3 was twice higher than at station AF2. The result supposes that under observed hydrodynamical conditions the main part of microplastics could remain inside Adventfjorden. Particles after some time may settle to the bottom or wash ashore on the east coast of the fjord under the influence of the SWS wind, which prevails in the fjord and is perpendicular to the east coast (Table 2). In case of more southern wind or variable wind direction in Adventfjorden, particles can be transported further to Sassenfjorden and Tempelfjorden and accumulate there under W-WNW wind, as was observed in 2021 (Table 2; Pakhomova et al., 2024).
Higher concentration of microplastics in Adventfjorden, as well as more frequent occurrence (100% of stations against 66%) in 2022 compared to 2021 can be a result of higher ships and tourist activities in 2022 (Table 2). In 2021 restrictions after the Covid –19 were still valid and cruise ships were not allowed to enter Isfjorden. This resulted in a smaller number of tourists in Longyearbyen and a smaller number of ships, cruise and fishing, in the Barents Sea and near Svalbard in 2021 (Table 2). This difference in tourism/shipping activity in 2021 and 2022 was very noticeable (10–100 times), while the difference in surface microplastic concentrations was less pronounced (average 1.6 times in abundance and 1.9 in mass). This may suggest the higher importance of local sources of surface microplastics in Adventfjorden than maritime activity and long-range transport.
Floating microplastics were found only at two stations outside Advenfjorden – southern station in Isfjorden and northern station in Sassenfjorden. Occurrence of microplastics at these sites was lower than in Adventfjorden, (33%, 1 of 3 transects), and the concentration was also lower (Table 1). Microplastics found at station IF1 can indicate that there is long-range transport of microplastics to the Isfjorden from North Atlantic as Isfjorden has cyclonic circulation and waters at this station are not affected by local sources of MPs from Longyearbyen city. Presence of microplastics at station SF1 can be the result of both local sources (Longyearbyen) and long-range transport. Obtained data for polymer composition of microplastics at SF1 do not allow to make more precise conclusion here as found particles were made of PE only, which was found at all studied locations.
Absence of surface microplastics in Tempelfjorden can be explained by two factors working simultaneously: SE-ESE wind and intensive river discharge. ESE wind promoted the spreading of rivers plumes for the whole area of Sassenfjorden and Tempelfjorden, preventing any particles from floating into the fjord from the outside (Table 2). All these rivers float through unpopulated areas and seem to be free of microplastics. The same situation was observed for the southern part of Sassenfjorden, at station SF2 – inflow of many rivers into Isfjorden between Andventfjorden and Sassenfjorden worked as a barrier for the transfer of the floating particles into the southern part of Sassenfjorden (Table 2).
No floating microplastics were found at station IF2, in waters flowing out from Isfjorden, which could mean that the majority of microplastics remain in the Isford system.
Microplastics in the subsurface layer
Spatial distribution, concentrations, and characteristics of microplastics found on the sea surface and in subsurface waters differed significantly (p < 0.01 for abundance and particle area). Maximum concentration of surface microplastics was found in the inner Adventfjorden (AF1) while there was observed minimum concentration for the subsurface microplastics there. The lowest concentrations of subsurface microplastics were also found in the northern Sassenfjorden (SF1) and in Tempelfjorden (TF1 and TF2). These four stations were also characterized by the lowest diversity of found polymer types. Sampling at all these locations was carried out in the river plume waters (Figure 1; Supplementary Table S1). Given that the amount of microplastics detected at these four stations was below the limit of quantification (LOQ), it is possible that the detected microplastics were artifacts of contamination during sampling and analysis. Therefore, it can be inferred that the river water was transporting microplastic-free water into the fjords.
The highest diversity of polymers, together with the highest proportion of fragments, was observed at station AF3, closest to the port area, which appears to be a significant source of microplastics here. Abundance of microplastics at AF2 was twice as small as at AF3 that can indicate that some part of subsurface microplastics remains in Adventfjorden as it was found for surface microplastics. The polymer composition of subsurface microplastics detected at stations AF2 and SF2 was identical; however, the concentration at SF2 was twice as high as in Adventfjorden. This suggests that subsurface microplastics were transported from Adventfjorden to the southern part of Sassenfjorden. Wastewater discharge point that located close to AF2 may be the primary source of microplastics at SF2, as currents transport them along the coast from Adventfjorden to Sassenfjorden (Herzke et al., 2021), where they encounter the opposing current of the Sassenelva River. The continuous input of microplastics from wastewater discharge may explain why station SF2 is the only river plume station with microplastic concentrations above the LOQ.
The highest concentration of subsurface microplastics was found in the central part of Isfjorden. The observed distribution of subsurface microplastics indicates that Adventfjorden is not the main source of this type of microplastics in the Isfjorden system and that it is located outside Isfjorden – the water from North Atlantic. It was shown that microplastics in the subsurface layer could be transported easily over long distances (Pakhomova et al., 2022; van Sebille et al., 2020). Cruise ships may play a significant role in introducing high concentrations of microplastics into Isfjorden as they can discharge a lot of microplastics with grey waters (Peng et al., 2022). It is forbidden to discharge grey waters in the fjords, and it must be done in open waters. But if grey water discharge occurs to the south of Svalbard, the currents can bring these waters to the fjords. The suggestion that the main source of subsurface microplastics is shipping activities may be confirmed by comparison of results of studies in 2021 and 2022. Abundance of subsurface microplastics in 2022 is 5 times higher than in 2021, that is in line with intensity of shipping activity in these years (Table 2).
Comparison of subsurface microplastics at stations IF1 and IF2 representing incoming and outcoming microplastics into the Isfjord system shows that 36% of microplastics by abundance and 89% by mass concentration may remain inside the Isfjord system.
Comparison of microplastics on the sea surface and in subsurface layer
Since the surface and subsurface samples were collected using different methods, the same size fraction and morphology of the items will be considered in this section for correct comparison in details: 500 – 5000 µm size fraction, without textile fibers in the dataset, concentration in volume units (Figure 5). The spatial distribution of surface and subsurface microplastics clearly shows that the mechanism of transport of these two types of microplastics differs. Movement of surface microplastics is driven mainly by wind while subsurface microplastics – by currents. Formation of accumulation zones is typical for surface microplastics but not for subsurface (Pakhomova et al., 2022; Zhdanov et al., 2023). Abundance of surface microplastics is lower than subsurface by two orders of magnitude (p < 0.005). However, mass concentration on the surface is higher by one order of magnitude than for subsurface microplastics. The items found in the subsurface layer are smaller than those floating on the surface even in the same size fraction. Polymer composition also differs as mainly items with positive buoyancy are found on the sea surface and with both positive and negative buoyancy – in the upper water column. The found features of surface and subsurface microplastics are summarized in Table 3. These differences have also been found for the open sea waters of the Eurasian Arctic and Central Atlantic (Yakushev et al., 2021; Zhdanov et al., 2023).
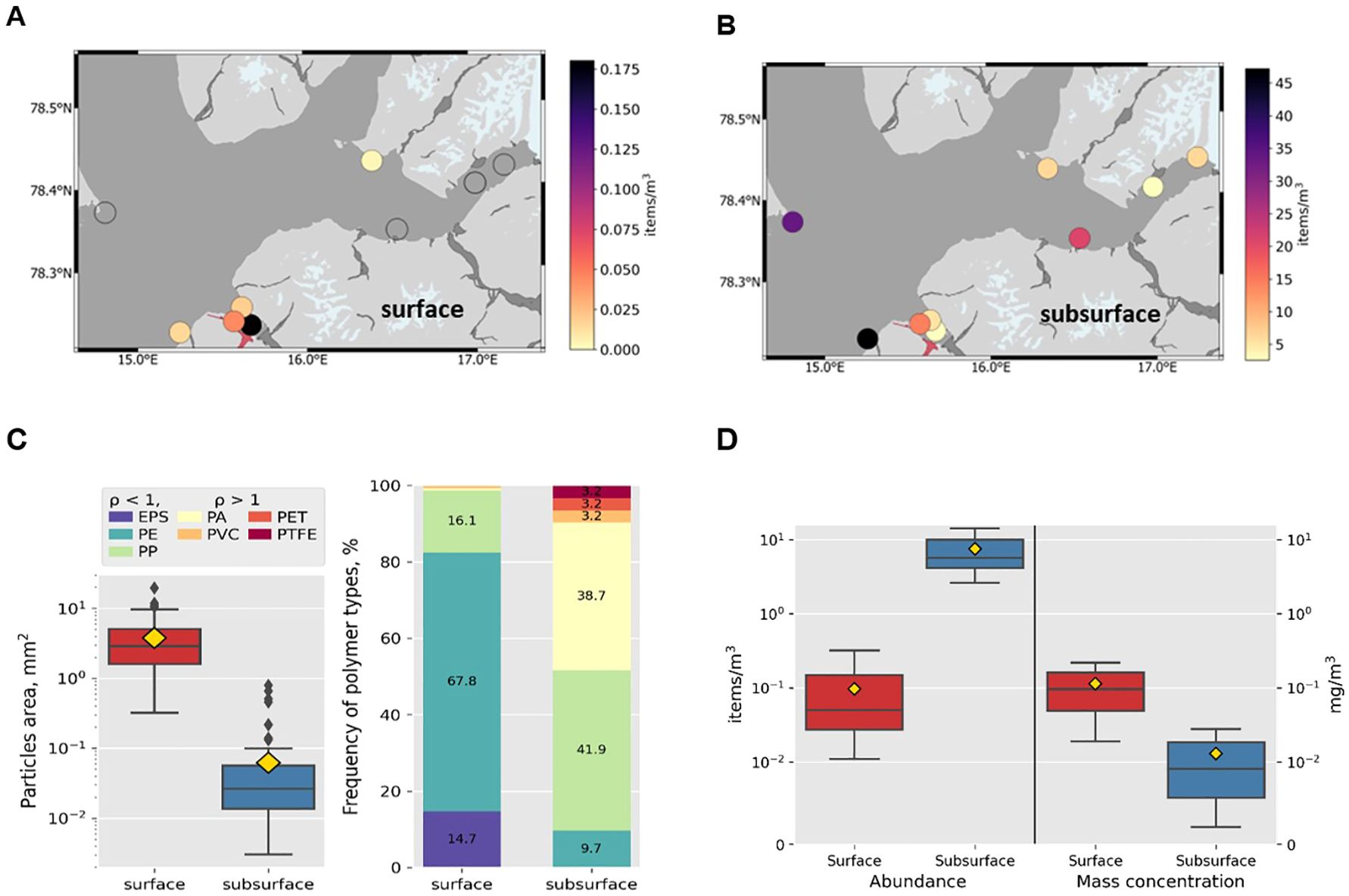
Figure 5. Characteristics of surface and subsurface microplastics (size range 500 – 5000 µm, no textile fibres): spatial distribution in Isfjorden (A; B); particle’s area and polymer composition (C); abundance and mass concentration in Adventfjorden (D).
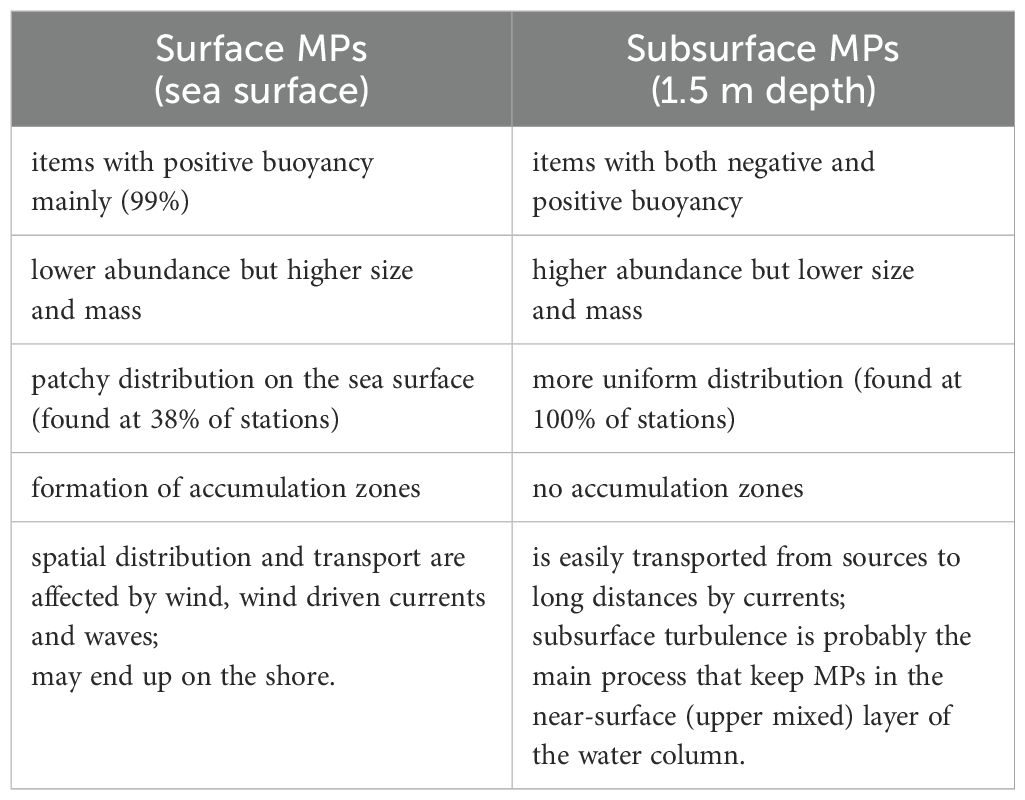
Table 3. Comparison of the characteristics of microplastics floating on sea surface and in subsurface layer.
Therefore, surface and subsurface microplastics differ significantly in a number of properties and, apparently, they should be considered as two independent groups of microplastics that may have different sources, and their distribution is driven by different hydrophysical processes.
Despite the difference in characteristics of these two types of microplastics in the Isfjord system, there were some similarities for the Isfjord system. It was found that less microplastics flow out of fjords than enters. River plumes affect the distribution of both types of microplastics by redistribution and decreasing their concentration.
Role of Arctic coastal zone in microplastic cycle in the ocean
The absence of surface microplastic in river plume water in Isfjorden suggest that rivers in the studied regions are free of microplastics. The results may suggest that Arctic rivers flowing through unpopulated areas bring clean water and thereby reducing level of microplastic pollution in coastal waters. This can be true for big size fraction of microplastics (>100 – 300 µm), while concentration of small sized microplastics that can be transferred by the air can be high in Arctic rivers as for some other contaminants (Evangeliou et al., 2020).
Taking into account that high amount of plastic litter was found on many islands in the Arctic (Bergmann et al., 2022; Ershova et al., 2024; Vesman et al., 2020), the fact that fjords accumulate floating/suspended items (Bianchi et al., 2020; Harris, 2020) and the findings of this study that more microplastics is coming into fjords than it comes out, we can suppose that Arctic islands are a dead end for floating plastics.
In this way, the coastal zone of the Arctic, including on the islands, is an important site for the global cycle of microplastics in the ocean, as it is both a recipient of microplastics transported here from distant sources and a supplier of clean water with Arctic rivers. Further studies are needed to understand the fate of microplastics in Arctic coastal sediments and beaches, and to quantify the Arctic’s role in clearing microplastics from the World Oceans.
Conclusions
The present study of the fate of microplastics in Svalbard coastal waters can be considered as a model study for understanding microplastic behaviour across the other Arctic islands. Svalbard, like much of the Arctic coast, is characterized by rivers largely free of anthropogenic pollution near their mouths and small, scarce settlements primarily engaged in port activities and shipping. At the same time Atlantic waters bring microplastics through long distances to both Svalbard and other Arctic islands. Based on these observations, it is reasonable to suggest that the findings from Svalbard might apply broadly across the Arctic. Specifically:
● Arctic rivers flowing through unpopulated areas bring clean water and thereby reduce level of microplastic pollution in the coastal waters;
● Coastal areas, especially fjords, in the Arctic may act as final repositories for floating plastics;
● Both long-range transport of microplastics and local sources are important in the Arctic;
● There are clear differences in sources and behaviour of surface and subsurface microplastics;
● Hydrophysical conditions varying in time and space play a crucial role in the formation of microplastic hotspots, which may limit the robustness of sporadic observations in assessing microplastic pollution in the Arctic.
Furthermore, the data collected during these studies showed that processes in the Arctic coastal zone are of great importance for the microplastic cycle and need to be taken into account in mathematical models to correctly predict the future dynamics of plastic pollution in the Arctic.
Data availability statement
The datasets presented in this study can be found in online repositories. The names of the repository/repositories and accession number(s) can be found in the article/Supplementary Material.
Author contributions
SP: Conceptualization, Data curation, Formal analysis, Investigation, Methodology, Validation, Writing – original draft, Writing – review & editing. AB: Formal analysis, Investigation, Software, Visualization, Writing – review & editing. IZ: Data curation, Formal analysis, Investigation, Writing – review & editing. EY: Conceptualization, Funding acquisition, Investigation, Methodology, Project administration, Resources, Supervision, Writing – review & editing.
Funding
The author(s) declare financial support was received for the research, authorship, and/or publication of this article. This work was partly funded by Svalbard’s environmental protection fund, project 22/43 EISVAL (EY, SP); by the Norwegian Ministry of Climate and Environment, projects RUS-19/0001 MAMBA (EY, AB) and 22087186 MIKRONOR2 (SP); by the Research Council of Norway, Arctic Field Grant, projects 333208 SCOPOM (IZ) and 342628/L10 (SP, AB). IZ’s grant from Research Council of Norway was a scheme supporting students, a mechanism for collaboration amongst individual scientists and not institutions.
Acknowledgments
The authors are grateful to the touristic company Hurtigruten Svalbard for help during the field studies, to Jake Martin and Matwey Novikov for help with sampling, to Cecilie Singdahl-Larsen, Madeline Jefroy and Vilde Kloster Snekkevik for help with µFTIR analysis and Tatiana Polivanova for help with the data analysis.
Conflict of interest
The authors declare that the research was conducted in the absence of any commercial or financial relationships that could be construed as a potential conflict of interest.
Publisher’s note
All claims expressed in this article are solely those of the authors and do not necessarily represent those of their affiliated organizations, or those of the publisher, the editors and the reviewers. Any product that may be evaluated in this article, or claim that may be made by its manufacturer, is not guaranteed or endorsed by the publisher.
Supplementary material
The Supplementary Material for this article can be found online at: https://www.frontiersin.org/articles/10.3389/fmars.2024.1392680/full#supplementary-material
References
Alling V. (2023). Monitoring of microplastics in the Norwegian environment (MIKRONOR) (No. 7922–2023), Monitoring (Norway: Norwegian Institute for Water Research and the Norwegian Environment Agency).
AMAP (2021). “AMAP litter and microplastics monitoring guidelines. Version 1.0,” (Tromsø, Norway: Arctic Monitoring and Assessment Programme (AMAP)).
Bao M., Huang Q., Lu Z., Collard F., Cai M., Huang P., et al. (2022). Investigation of microplastic pollution in Arctic fjord water: a case study of Rijpfjorden, Northern Svalbard. Environ. Sci. Pollut. Res. 29, 56525–56534. doi: 10.1007/s11356-022-19826-3
Berezina A., Pakhomova S., Zhdanov I., Mekhova O., Polivanova T., Novikov M., et al. (2023). Surface microplastics in the kara sea: from the kara gate to the 83°N. Front. Mar. Sci. 10. doi: 10.3389/fmars.2023.1268879
Bergmann M., Collard F., Fabres J., Gabrielsen G. W., Provencher J. F., Rochman C. M., et al. (2022). Plastic pollution in the arctic. Nat. Rev. Earth Environ. 3, 323–337. doi: 10.1038/s43017-022-00279-8
Bergmann M., Mützel S., Primpke S., Tekman M. B., Trachsel J., Gerdts G. (2019). White and wonderful? Microplastics prevail in snow from the Alps to the Arctic. Sci. Adv. 5, eaax1157. doi: 10.1126/sciadv.aax1157
Bianchi T. S., Arndt S., Austin W. E. N., Benn D. I., Bertrand S., Cui X., et al. (2020). Fjords as aquatic critical zones (ACZs). Earth-Science Rev. 203, 103145. doi: 10.1016/j.earscirev.2020.103145
Bråte I. L. N., Hurley R., Iversen K., Beyer J., Thomas K. V., Steindal C. C., et al. (2018). Mytilus spp. as sentinels for monitoring microplastic pollution in Norwegian coastal waters: A qualitative and quantitative study. Environ. Pollut. 243, 383–393. doi: 10.1016/j.envpol.2018.08.077
Carlsson P., Singdahl-Larsen C., Lusher A. L. (2021). Understanding the occurrence and fate of microplastics in coastal Arctic ecosystems: The case of surface waters, sediments and walrus (Odobenus rosmarus). Sci. Total Environ. 792, 148308. doi: 10.1016/j.scitotenv.2021.148308
Choudhary S., Neelavanan K., Saalim S. M. (2022). Microplastics in the surface sediments of Krossfjord-Kongsfjord system, Svalbard, Arctic. Mar. Pollut. Bull. 176, 113452. doi: 10.1016/j.marpolbul.2022.113452
Cózar A., Martí E., Duarte C. M., García-de-Lomas J., van Sebille E., Ballatore T. J., et al. (2017). The Arctic Ocean as a dead end for floating plastics in the North Atlantic branch of the Thermohaline Circulation. Sci. Adv. 3, e1600582. doi: 10.1126/sciadv.1600582
Ershova A., Vorotnichenko E., Gordeeva S., Ruzhnikova N., Trofimova A. (2024). Beach litter composition, distribution patterns and annual budgets on Novaya Zemlya archipelago, Russian Arctic. Mar. Pollut. Bull. 204, 116517. doi: 10.1016/j.marpolbul.2024.116517
Evangeliou N., Grythe H., Klimont Z., Heyes C., Eckhardt S., Lopez-Aparicio S., et al. (2020). Atmospheric transport is a major pathway of microplastics to remote regions. Nat. Commun. 11, 3381. doi: 10.1038/s41467-020-17201-9
Fang C., Zheng R., Zhang Y., Hong F., Mu J., Chen M., et al. (2018). Microplastic contamination in benthic organisms from the Arctic and sub-Arctic regions. Chemosphere 209, 298–306. doi: 10.1016/j.chemosphere.2018.06.101
Frank Y. A., Vorobiev D. S., Kayler O. A., Vorobiev E. D., Kulinicheva K. S., Trifonov A. A., et al. (2021). Evidence for microplastics contamination of the remote tributary of the yenisei river, siberia—The pilot study results. Water 13, 3248. doi: 10.3390/w13223248
Gebruk A., Yulia E., Lea-Anne H., Henley Sian F., Vassily S., Nikolay S., et al. (2022). “(Research): microplastics in the arctic benthic fauna: A case study of the snow crab in the pechora sea, Russia,” in Building common interests in the arctic ocean with global inclusion, informed decisionmaking for sustainability. Eds. Berkman P. A., Vylegzhanin A. N., Young O. R., Balton D. A., Øvretveit O. R. (Springer International Publishing, Cham), 85–102. doi: 10.1007/978-3-030-89312-5_6
Granberg M., Friesen L. W. V., Granberg M. E., Pavlova O., Magnusson K. (2020). Summer sea ice melt and wastewater are important local sources of microlitter to Svalbard waters Summer sea ice melt and wastewater are important local sources of microlitter to Svalbard waters. Environ. Int. 139, 105511. doi: 10.1016/j.envint.2020.105511
Harris P. T. (2020). The fate of microplastic in marine sedimentary environments: A review and synthesis. Mar. Pollut. Bull. 158, 111398. doi: 10.1016/j.marpolbul.2020.111398
Herzke D., Ghaffari P., Sundet J. H., Tranang C. A., Halsband C. (2021). Microplastic fiber emissions from wastewater effluents: abundance, transport behavior and exposure risk for biota in an arctic fjord. Front. Environ. Sci. 9. doi: 10.3389/fenvs.2021.662168
Iannilli V., Pasquali V., Setini A., Corami F. (2019). First evidence of microplastics ingestion in benthic amphipods from Svalbard. Environ. Res. 179, 108811. doi: 10.1016/j.envres.2019.108811
Ikenoue T., Nakajima R., Fujiwara A., Onodera J., Itoh M., Toyoshima J., et al. (2023a). Horizontal distribution of surface microplastic concentrations and water-column microplastic inventories in the Chukchi Sea, western Arctic Ocean. Sci. Total Environ. 855, 159564. doi: 10.1016/j.scitotenv.2022.159564
Ikenoue T., Nakajima R., Mishra P., Ramasamy E. V., Fujiwara A., Nishino S., et al. (2023b). Floating microplastic inventories in the southern Beaufort Sea, Arctic Ocean. Front. Mar. Sci. 10. doi: 10.3389/fmars.2023.1288301
Jambeck J. R., Geyer R., Wilcox C., Siegler T. R., Perryman M., Andrady A., et al. (2015). Plastic waste inputs from land into the ocean. Science 347, 768–771. doi: 10.1126/science.1260352
Kanhai L. D. K., Gardfeldt K., Krumpen T., Thompson R. C., O’Connor I. (2020). Microplastics in sea ice and seawater beneath ice floes from the Arctic Ocean. Sci. Rep. 10, 5004. doi: 10.1038/s41598-020-61948-6
Kukulka T., Proskurowski G., Morét-Ferguson S., Meyer D. W., Law K. L. (2012). The effect of wind mixing on the vertical distribution of buoyant plastic debris. Geophysical Res. Lett. 39, 1–6. doi: 10.1029/2012GL051116
Lebreton L. C. M., van der Zwet J., Damsteeg J. W., Slat B., Andrady A., Reisser J. (2017). River plastic emissions to the world’s oceans. Nat. Commun. 8, 1–10. doi: 10.1038/ncomms15611
Lechner A., Keckeis H., Lumesberger-Loisl F., Zens B., Krusch R., Tritthart M., et al. (2014). The Danube so colourful: A potpourri of plastic litter outnumbers fish larvae in Europe’s second largest river. Environ. Pollut. 188, 177–181. doi: 10.1016/j.envpol.2014.02.006
Lloyd-Jones T., Dick J. J., Lane T. P., Cunningham E. M., Kiriakoulakis K. (2023). Occurrence and sources of microplastics on Arctic beaches: Svalbard. Mar. Pollut. Bull. 196, 115586. doi: 10.1016/j.marpolbul.2023.115586
Lusher A. (2015). Microplastics in the marine environment: distribution, interactions and effects, in: marine anthropogenic litter. Springer Int. Publishing Cham pp, 245–307. doi: 10.1007/978-3-319-16510-3_10
Lusher A. L., Munno K., Hermabessiere L., Carr S. (2020). Isolation and extraction of microplastics from environmental samples: an evaluation of practical approaches and recommendations for further harmonization. Appl. Spectrosc. 74, 1049–1065. doi: 10.1177/0003702820938993
Malli A., Corella-Puertas E., Hajjar C., Boulay A.-M. (2022). Transport mechanisms and fate of microplastics in estuarine compartments: A review. Mar. Pollut. Bull. 177, 113553. doi: 10.1016/j.marpolbul.2022.113553
Mani T., Primpke S., Lorenz C., Gerdts G., Burkhardt-Holm P. (2019). Microplastic pollution in benthic midstream sediments of the rhine river. Environ. Sci. Technol. 53, 6053–6062. doi: 10.1021/acs.est.9b01363
Martínez-Francés E., van Bavel B., Hurley R., Nizzetto L., Pakhomova S., Buenaventura N. T., et al. (2023). Innovative reference materials for method validation in microplastic analysis including interlaboratory comparison exercises. Anal. Bioanal Chem. 415, 2907–2919. doi: 10.1007/s00216-023-04636-4
Obbard R. W., Sadri S., Wong Y. Q., Khitun A. A., Baker I., Richard C. (2014). Global warming releases microplastic legacy frozen in Arctic Sea ice. Earth’s Future. 2, 315–320. doi: 10.1002/2014EF000240
Pakhomova S. (2023). “Floating microplastics in the Eurasian Arctic: spatial and temporal trends,” Presented Presented at the second international symposium on plastics in the arctic and sub-arctic region(Reykjavík, Iceland). Available at: https://www.arcticplastics.is/program
Pakhomova S., Berezina A., Lusher A. L., Zhdanov I., Silvestrova K., Zavialov P., et al. (2022). Microplastic variability in subsurface water from the Arctic to Antarctica. Environ. Pollut. 298, 118808. doi: 10.1016/j.envpol.2022.118808
Pakhomova S., Berezina A., Zhdanov I., Mekhova O., Ilinskaya A., Golyakov A., et al. (2024). Floating microplastics in Svalbard fjords: High spatial variability requires methodological consistency in estuarine systems. Mar. Pollut. Bull. 207, 116803. doi: 10.1016/j.marpolbul.2024.116803
PAME (2019). Desktop study on marine litter including microplastics in the arctic. Protection of the Arctic Marine Environment (PAME). Available at: http://hdl.handle.net/11374/2389
Peeken I., Primpke S., Beyer B., Gütermann J., Katlein C., Krumpen T., et al. (2018). Arctic sea ice is an important temporal sink and means of transport for microplastic. Nat. Commun. 9, 1505. doi: 10.1038/s41467-018-03825-5
Peng G., Xu B., Li D. (2022). Gray water from ships: A significant sea-based source of microplastics? Environ. Sci. Technol. 56, 4–7. doi: 10.1021/acs.est.1c05446
Primpke S., Wirth M., Lorenz C., Gerdts G. (2018). Reference database design for the automated analysis of microplastic samples based on Fourier transform infrared (FTIR) spectroscopy. Anal. Bioanal Chem. 410, 5131–5141. doi: 10.1007/s00216-018-1156-x
Ramasamy E. V., Sruthy S., Harit A. K., Mohan M., Binish M. B. (2021). Microplastic pollution in the surface sediment of Kongsfjorden, Svalbard, Arctic. Mar. Pollut. Bull. 173, 112986. doi: 10.1016/j.marpolbul.2021.112986
Ross P. S., Chastain S., Vassilenko E., Etemadifar A., Zimmermann S., Quesnel S.-A., et al. (2021). Pervasive distribution of polyester fibres in the Arctic Ocean is driven by Atlantic inputs. Nat. Commun. 12, 106. doi: 10.1038/s41467-020-20347-1
Ruman M., Kozak K., Lehmann S., Kozioł K., Polkowska Ż. (2012). Pollutants present in different components of the Svalbard archipelago environment / Zanieczyszczenia obecne w różnych komponentach środowiska archipelagu Svalbard. Ecol. Chem. Eng. S 19, 571–584. doi: 10.2478/v10216-011-0040-9
Schmidt C., Krauth T., Wagner S. (2017). Export of plastic debris by rivers into the sea. Environ. Sci. Technol. 51, 12246–12253. doi: 10.1021/acs.est.7b02368
Singh N., Granberg M., Collard F., Caruso G., Lu Z., Kögel T., et al. (2021). Microplastics in the realm of Svalbard: current knowledge and future perspective (MIRES). I SESS report 2020 - The State of Environmental Science in Svalbard - an annual report (s. 118–141). Svalbard Integrated Arctic Earth Observing System. doi: 10.5281/zenodo.4293836
Skogseth R., Olivier L. L. A., Nilsen F., Falck E., Fraser N., Tverberg V., et al. (2020). Variability and decadal trends in the Isfjorden (Svalbard) ocean climate and circulation – An indicator for climate change in the European Arctic. Prog. Oceanography 187, 102394. doi: 10.1016/j.pocean.2020.102394
Teichert S., Löder M. G. J., Pyko I., Mordek M., Schulbert C., Wisshak M., et al. (2021). Microplastic contamination of the drilling bivalve Hiatella arctica in Arctic rhodolith beds. Sci. Rep. 11, 14574. doi: 10.1038/s41598-021-93668-w
van Bavel B. (2022). Microplastics in Norwegian coastal areas, rivers, lakes and air (MIKRONOR1) (No. 7811–2023), Environmental monitoring (Norway: Norsk institutt for vannforskning/Norwegian Institute for Water Research & Norwegian Environment Agency.).
van Sebille E., Aliani S., Law K. L., Maximenko N., Alsina J. M., Bagaev A., et al. (2020). The physical oceanography of the transport of floating marine debris. Environ. Res. Lett. 15, 023003. doi: 10.1088/1748-9326/ab6d7d
Vesman A., Moulin E., Egorova A., Zaikov K. (2020). Marine litter pollution on the Northern Island of the Novaya Zemlya archipelago. Mar. Pollut. Bull. 150, 110671. doi: 10.1016/j.marpolbul.2019.110671
Vorobiev E. D., Rakhmatullina S. N., Vorobiev D. S., Frank Y. A. (2023). Assessment of surface water pollution by microplastics in the Yenisei River. Abstracts of the Conference “Microplastics in Polymer Science (Velikiy Novgorod, Russia: Presented at Microplastics in Polymer Science) 85–86.
Woodall L. C., Sanchez-Vidal A., Canals M., Paterson G. L. J., Coppock R., Sleight V., et al. (2014). The deep sea is a major sink for microplastic debris. R. Soc Open Sci. 1, 140317. doi: 10.1098/rsos.140317
Yakushev E., Gebruk A., Osadchiev A., Pakhomova S., Lusher A., Berezina A., et al. (2021). Microplastics distribution in the Eurasian Arctic is affected by Atlantic waters and Siberian rivers. Commun. Earth Environ. 2, 23. doi: 10.1038/s43247-021-00091-0
Zhdanov I., Lokhov A., Belesov A., Kozhevnikov A., Pakhomova S., Berezina A., et al. (2022). Assessment of seasonal variability of input of microplastics from the Northern Dvina River to the Arctic Ocean. Mar. Pollut. Bull. 175, 113370. doi: 10.1016/j.marpolbul.2022.113370
Zhdanov I., Pakhomova S., Berezina A., Silvestrova K., Stepanova N., Yakushev E. (2023). Differences in the fate of surface and subsurface microplastics: A case study in the central atlantic. J. Mar. Sci. Eng 11, 210. doi: 10.3390/jmse11010210
Keywords: plastic pollution, Arctic Ocean, Arctic rivers, hydrodynamics, fjords
Citation: Pakhomova S, Berezina A, Zhdanov I and Yakushev E (2024) Microplastic fate in Arctic coastal waters: accumulation hotspots and role of rivers in Svalbard. Front. Mar. Sci. 11:1392680. doi: 10.3389/fmars.2024.1392680
Received: 27 February 2024; Accepted: 16 September 2024;
Published: 31 October 2024.
Edited by:
Fabiana Corami, National Research Council (CNR), ItalyReviewed by:
Sandra Ramos, University of Porto, PortugalBeatrice Rosso, National Research Council (CNR), Italy
Giuseppe Suaria, National Research Council, Italy
Takahito Ikenoue, Japan Agency for Marine-Earth Science and Technology (JAMSTEC), Japan
Copyright © 2024 Pakhomova, Berezina, Zhdanov and Yakushev. This is an open-access article distributed under the terms of the Creative Commons Attribution License (CC BY). The use, distribution or reproduction in other forums is permitted, provided the original author(s) and the copyright owner(s) are credited and that the original publication in this journal is cited, in accordance with accepted academic practice. No use, distribution or reproduction is permitted which does not comply with these terms.
*Correspondence: Svetlana Pakhomova, c3ZwQG5pdmEubm8=