- 1Key Laboratory of Aquaculture in the South China Sea for Aquatic Economic Animals, Guangdong Ocean University, Fisheries College, Zhanjiang, China
- 2Guangdong Provincial Key Laboratory of Aquatic Animal Disease Control and Healthy Aquaculture, College of Fisheries, Guangdong Ocean University, Zhanjiang, China
Hainan medaka (Oryzias curvinotus) is a small euryhaline fish species native to the northern margin of the South China Sea. Our long-term field observations indicate a concerning decline in its wild resources. Climate change, an uncontrollable factor, has altered the species’ distribution pattern. In this study, we simulated the shifts in the species range of O. curvinotus during the Last Glacial Maximum (LGM), current, and the next one hundred year, and analyzed its habitat attributes. The results demonstrate that bio2 (mean diurnal range of temperature) is a crucial factor in shaping the species range of O. curvinotus. The simulation results reveal that the current habitats are located in the coastal areas of northern Vietnam, the northeastern Hainan Province, the coastal areas of Guangdong Province and Guangxi Zhuang Autonomous Region, and a few areas in Taiwan Province of China, covering a total area of 17.82×104 km2. Highly suitable habitats are mainly concentrated in the coastal areas of Hai Phong, Nam Dinh, and Thanh Hoa in northern Vietnam, the central part of Leizhou Peninsula, and the west coast of the Pearl River Estuary. For the tropical species Hainan medaka, the impact of the LGM was relatively minor, and there were extensive suitable habitats during historical times, including three refugia. Currently, only Refugium 2 near Guanghai Town, Taishan County, Jiangmen City, Guangdong Province in China remains, while the other two refugia have submerged below sea level. Future climate warming under different carbon emission levels is projected to cause a short-term expansion, followed by a relief in expansion. By 2100, the potential habitat area of O. curvinotus is slightly larger than the current scenario. It is noteworthy that under future climate warming scenarios, the highly suitable habitats will not migrate northward but will expand near the 21°N latitude. Overall, Hainan medaka is not expected to be threatened in the future. Our study provides long-term dynamic distribution data, which provides a theoretical basis for the long-term development and conservation management of Hainan medaka.
Introduction
Evidence that the climate is changing is indisputable (Berrang-Ford et al., 2011), and this phenomenon poses a potential threat to the planet’s biodiversity (Kappelle et al., 1999). Over the past 100 years, the Earth’s climate has visibly warmed, precipitation regimes have changed, and biologists have been concerned about the impact of these shifts on species distribution (Araújo and Rahbek, 2006). Under the background of global climate change, the study of species distribution range has become the premise of biodiversity conservation strategy, especially for those endemic species with narrow distribution range. For example, the endangered endemic annonaceae species in East Africa may lose some of their original suitable habitats due to future warming (Mkala et al., 2023). In addition, climate change will drive some species to higher altitudes or latitudes (Hickling et al., 2005; Chen et al., 2011; Liao et al., 2023). Over the past few decades, more than 1,700 species have been confirmed to migrate poleward at a rate of 6.1 km/decade (Parmesan and Yohe, 2003), and the rate of migration has doubled or tripled in recent years to a staggering 16.9 km/decade (Chen et al., 2011). Of the species studied, the current focus is mainly on plants and various insect groups, etc., while relatively little is known about vertebrates, especially these tropical endemic fish.
Hainan medaka (Oryzias curvinotus) is a small euryhaline fish that is confined to the coastal waters of the South China Sea, including mangroves, brackish water confluxes in estuaries, and even freshwater streams extending inland (Dong et al., 2021). It is small in size, usually growing up to 2 inches long. Similar to freshwater Japanese medaka (Oryzias latipes), Hainan medaka is an excellent euryhaline model fish resource to be developed. As a euryhaline fish, O. curvinotus exhibits unique adaptive traits that are crucial not only for its own survival but also serve as indicators of ecological balance and environmental health in the South China Sea region. Changes in the habitat of this species directly reflect subtle variations in environmental factors such as salinity and water temperature in the region, which may further impact the stability and biodiversity of ecosystems including mangrove forests, coral reefs, and seagrass beds. However, recent fieldwork indicates that the habitat of Hainan medaka appears to be under threat, and the population resources are not optimistic. Climate change, a long-term and irreversible factor, needs to be considered in addition to the immediate effects of human activities (Yao et al., 2022). In particular, the fate of Hainan medaka, which is located in a biological hotspot (Oriental region), is a key ecological issue in the context of global climate change. By conducting thorough investigations into the habitat shifts and adaptive mechanisms of O. curvinotus, a more precise understanding of the ecological health of the South China Sea can be gained, thereby providing scientific evidence for the formulation of effective environmental protection and management strategies.
Effectively predicting the species range and their impacts under climate change, as well as understanding their habitat attributes, is crucial for developing appropriate strategies for species conservation (Alabdulhafith et al., 2022). Species distribution models (SDMs), also known as climate envelope-models, habitat models, and (environmental or ecological) niche-models, largely address the urgent need for ecologists to understand the habitat attributes of species and their distribution range shifts. Maximum entropy model (MaxEnt) is the most widely recognized and used SDMs, due to its high prediction accuracy, automatic assessment of important environmental variables, fast operation, and small sample size requirements (Phillips et al., 2006; Ma and Sun, 2018; Li et al., 2019; Xiao et al., 2022; Liao et al., 2023). MaxEnt is therefore widely used to predict the potential distribution of plants, birds, insects, nematodes, corals, bryophytes, and fungi (Sérgio et al., 2007; Phillips and Dudík, 2008; Tognelli et al., 2009; Williams et al., 2009; Young et al., 2009; Alabdulhafith et al., 2022), providing valuable information in biogeography, invasion biology, conservation biology, and ecology.
In this study, we integrated our previous field data with reported occurrence sites and climate data to analyze the historical period (the Last Glacial Maximum, LGM, dating back approximately between 25,000 and 19,000 years ago), current scenarios, and future potential habitats under different warming levels for the euryhaline fish endemic to the coast of the northern South China Sea. The objectives of this study were to (1) comprehend the habitat properties of O. curvinotus, (2) investigate its distribution and refuge locations during historical periods, and (3) assess potential habitats in the present context while considering how future climate change may impact its distribution.
Materials and methods
Field survey and occurrence data collection
We conducted field survey work on O. curvinotus from 2015 to 2018, and successfully recorded a total of 19 recording sites (Table 1), including mangrove tidal channels, estuary with a wide salinity range and some freshwater streams. In addition to our survey data, we have collected occurrence data of O. curvinotus through the Global Biodiversity Information Facility (GBIF database, https://www.gbif.org/, accessed on 18 June 2023) and literature (Hamaguchi and Sakaizumi, 1992; Kondo et al., 2001; Koga et al., 2002; Matsuda et al., 2003; Hamaguchi et al., 2004; Shiga and Suzuki, 2004; Shinomiya et al., 2006; Kamei et al., 2007; Kato et al., 2010, 2011; Masaoka et al., 2012; Wang et al., 2017; Dong et al., 2020, 2021; Li et al., 2022a; Yao et al., 2022; Gao et al., 2023). We have made every effort to gather all relevant published literature and publicly available data. For the occurrence data collected in the three ways, we first eliminated duplicates and outliers. In order to avoid overfitting, we created a 1km × 1km grid in ArcGIS 10.7 (Esri, Redlands, CA, USA), and reserved only the point closest to the center in each grid. Finally, 38 valid GPS sites were obtained and used for input occurrence data to the model (Figure 1; Supplementary Table 1).
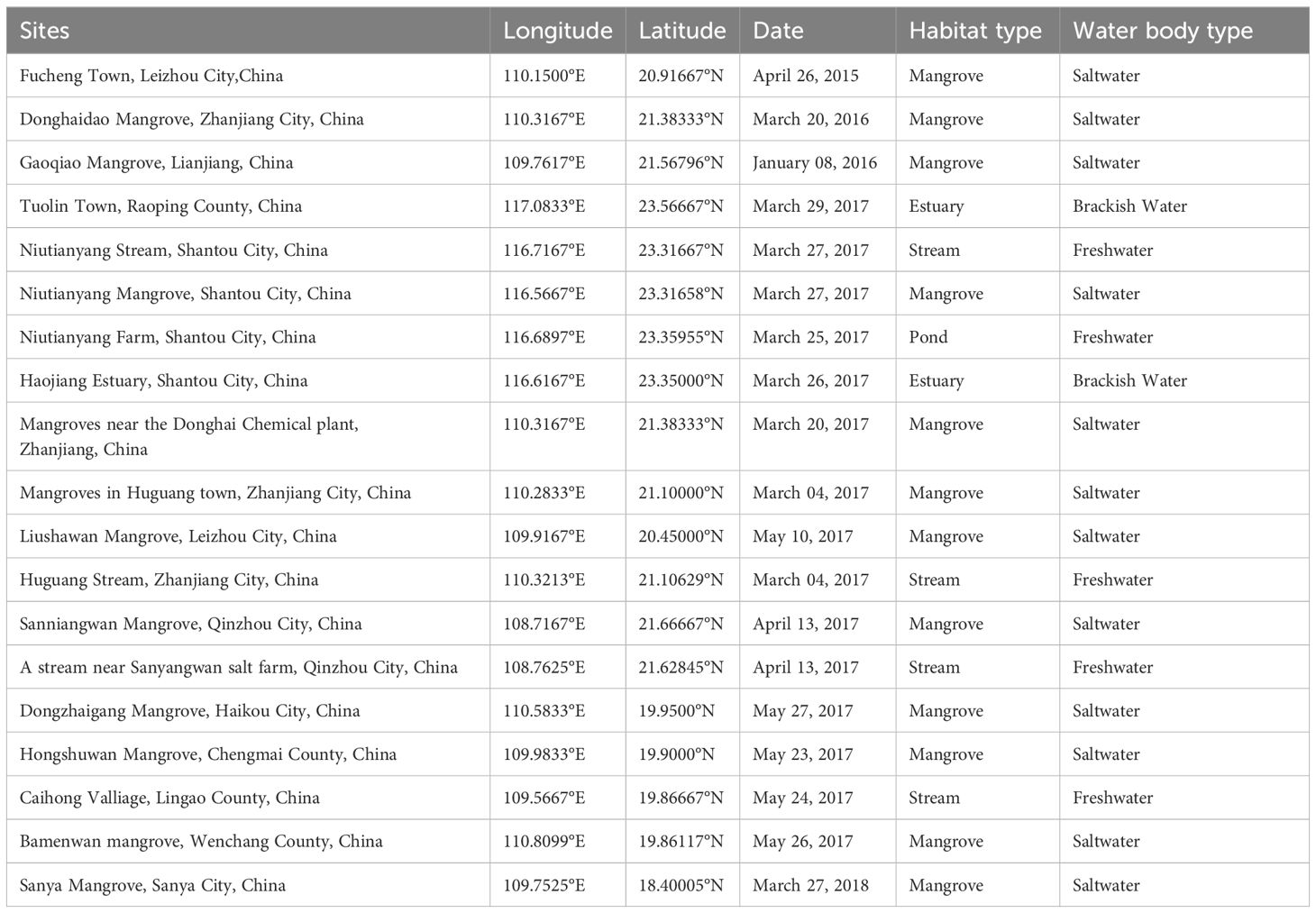
Table 1. Information from our comprehensive field survey conducted between 2015 to 2018 on the spatial distribution of O. curvinotus.
Climate variables and processing
To investigate the impact of climate change on euryhaline fish distribution in tropical regions, a set of 19 bioclimatic factors (Table 2) with 2.5 arc-minutes spatial resolution were selected as environmental variables for the model, which were downloaded from the WORLDCLIM 2.0 database (https://www.worldclim.org/data/bioclim.html, accessed on June 18, 2023). The downloaded climate variable layer was converted into ASCLL format in ArcGIS 10.7 for MaxEnt model analysis. The climate variables used in this study include historical (the LGM), current and future climates (Fick and Hijmans, 2017). The future climate includes four carbon emission levels (SSP1-2.6: the low-end level, 376 ppm CO2-equivalent level; SSP2-4.5: the low-moderate level, 650 ppm CO2-equivalent level; SSP3-7.0: the medium-high level, 1011 ppm CO2-equivalent level and SSP5-8.5: the high level, 1228 ppm CO2-equivalent level) (Meinshausen et al., 2020; Liao et al., 2022) and different future decades (2040, 2060, 2080, and 2100) (Fick and Hijmans, 2017).
In order to avoid the multicollinearity of climate factors leading to overfitting of the model, we conducted a variance inflation factor analysis (VIF) (Liao et al., 2023). We first used ArcGIS 10.7 to convert the GPS site distribution data (csv format) into raster (shp format), then extracted 19 climate variable values at each site using the extraction function of the spatial analysis tool in the toolbox, and performed VIF analysis on 19 climate factors at all sites. We removed 12 multicollinearity climate variables (VIF > 10) by using USDM version 1.1-18 package in R 4.1.3 (Naimi et al., 2014), and 7 variables were left for modeling: bio2, bio7, bio8, bio9, bio12, bio15 and bio17.
Potential habitat modeling and statistical analysis
The potential suitable habitat of O. curvinotus was simulated using the maximum entropy model by MaxEnt version 3.3.3k (Phillips et al., 2006). The input data included optimized climate variables and occurrence data. Among them, 25 percent of the occurrence data was randomly selected for the test dataset and 75 percent for the training dataset (Yuan et al., 2015; Yi et al., 2016; Liao et al., 2022, 2023; Xiao et al., 2022). The program ran at least 1000 iterations until it converged (threshold 0.00001) (Zhang et al., 2018; Liao et al., 2022). The robustness of the MaxEnt model is assessed by the AUC value of the threshold-independent receiver-operating characteristic (ROC) analysis: above 0.9 is defined as excellent, between 0.8 and 0.9 is defined as good, between 0.7 and 0.8 is considered acceptable, between 0.6 and 0.7 is defined as poor, and below 0.6 is considered inadequate (Phillips et al., 2006; Liao et al., 2022). Simulating the probability of occurrence of O. curvinotus in a certain location as its suitability value to distinguish different levels of potential habitat (Phillips et al., 2006). The potential suitable habitats obtained through modeling were categorized into four levels based on their suitability values: highly suitable habitats, moderately suitable habitats, lowly suitable habitats, and unsuitable habitats. The suitability values ranged from 0.6 to 1 for highly suitable habitats, from 0.4 to 0.6 for moderately suitable habitats, from 0.2 to 0.4 for lowly suitable habitats, and from 0 to 0.2 for unsuitable habitats (Zhang et al., 2018; Liao et al., 2023). The area of each part was counted in the 3D analysis tool in ArcGIS, and then the area change chart was drawn by HIPLOT online server (https://hiplot.cn/) (Li et al., 2022b). During the LGM, habitat suitability exceeding 0.9 was defined as Refugium (Gathorne-Hardy et al., 2002). The contribution rate, permutation importance of climate variables and their response curve were completed by Jackknife test in MaxEnt software (Phillips et al., 2006).
Results
Model performance, variable contribution, and response curves
The AUC values of both the training (0.999) and test (0.998) sets were greater than 0.9, indicating that the simulation results were reliable and even excellent. The results of the Jackknife test revealed relevant information about the contribution and importance of climate variables (Table 3). For contribution, annual precipitation (bio12, 35.32%) explained the current potential range of O. curvinotus to the greatest extent, followed by the mean diurnal range (bio2, 24.91%). Climate factors bio7 (Temperature annual range), bio8 (mean temperature of wettest quarter), bio9 (mean temperature of driest quarter), bio15 (Precipitation seasonality), and bio17 (precipitation of driest quarter) contributed a total of 39.77%. The permutation importance index identified two of the most important climate variables, bio8 (mean temperature of wettest quarter, 46.35%) and bio2 (mean diurnal range, 35.51%). Considering the contribution rate and permutation importance, bio2 is a key climate factor for the potential distribution of O. curvinotus. For the most important variables and those that contributed the most, bio2 was used for further analysis. The mean diurnal temperature range varied from 6.9 to 7.9 degrees Celsius, indicating a habitat of low suitability for O. curvinotus. When the mean diurnal temperature range falls between 6 and 6.9 degrees Celsius, the habitat suitability for O. curvinotus is considered moderate. In areas where the mean diurnal temperature range is below 6 degrees Celsius, O. curvinotus exhibits a high degree of suitability (Figure 2).
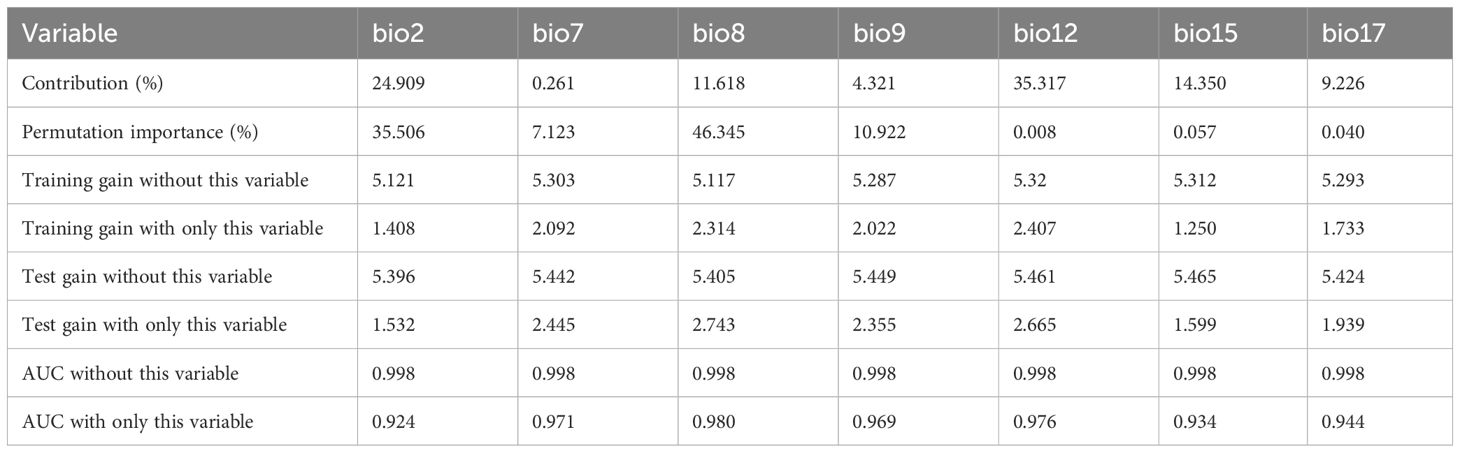
Table 3. The contribution rate, permutation importance of filtered climate factors used in the model prediction, and relevant information of the Jackknife test.
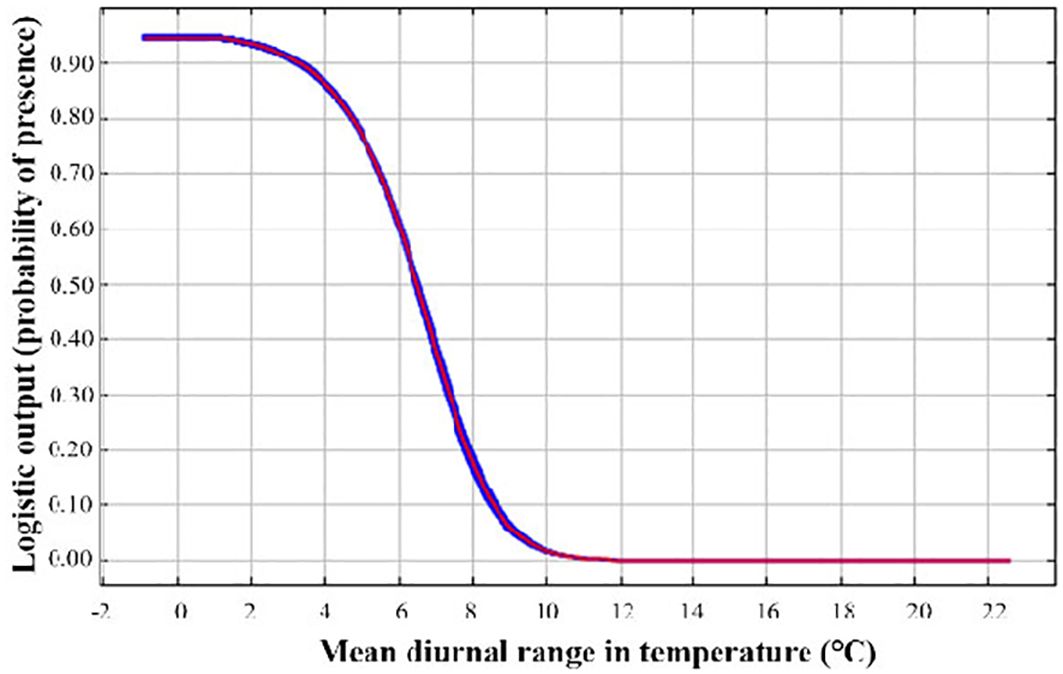
Figure 2. Response curves of climatic suitability of bio2 [Mean diurnal range (mean of monthly max temperature-min temperature)].
Current habitats
The distribution of potential habitat in the current situation is shown in Figure 3. The total potential habitat area is 17.82×104 km2, including 1.44×104 km2 of highly suitable habitats, 3.91×104 km2 of moderately suitable habitats, and 12.47×104 km2 of lowly suitable habitats. The potential habitats are mainly distributed in the coastal areas of northern Vietnam, the northeastern Hainan Province, the coastal areas of Guangdong Province and Guangxi Zhuang Autonomous Region, and a small part of Taiwan Province in China. Highly suitable habitats are mainly distributed in Hai Phong, Nam Dinh and Thanh Hoa areas of Vietnam, the central area of Leizhou Peninsula and the west bank of the Pearl River Estuary of China. The moderately suitable habitat extends from the highly suitable habitat to Hanoi of Vietnam, the whole Leizhou Peninsula, the two sides of the Pearl River Delta, and the northeast coast of Hainan Island in China (Figure 3). On the basis of moderately suitable habitat, the lowly suitable habitat extended further to inland areas, especially the southern Guangxi Zhuang Autonomous region has a large area of low potential habitat.
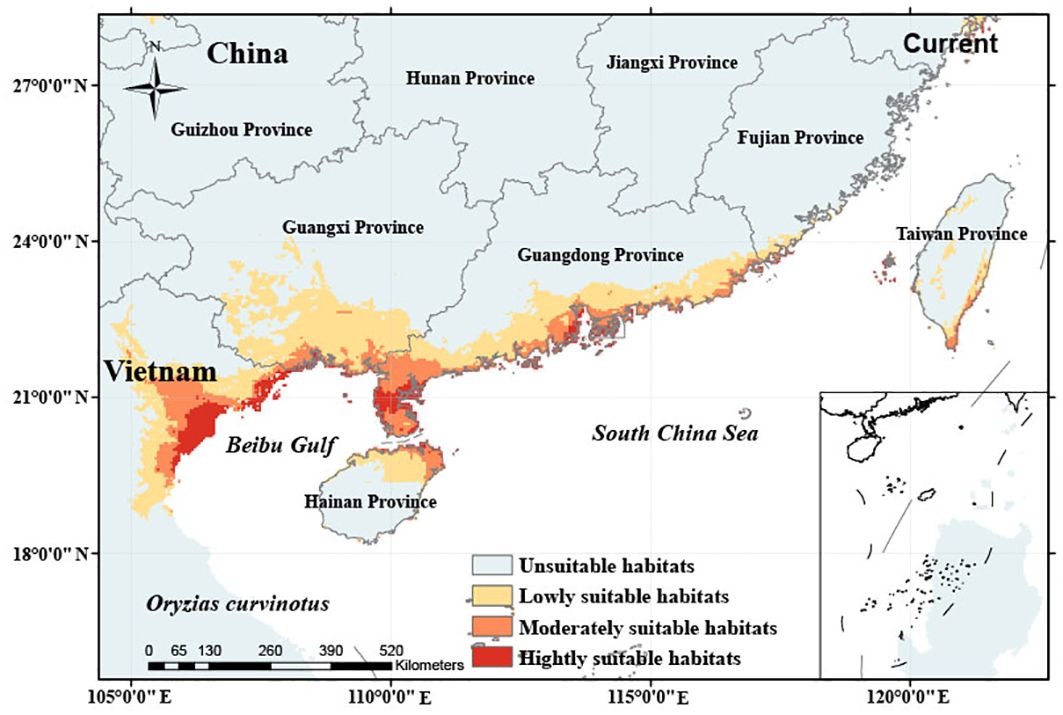
Figure 3. Simulation of potential distribution areas of O. curvinotus with varying suitability in the current climate based on occurrence records.
Habitats in the LGM
Our simulations revealed that during the historical period (the LGM), O. curvinotus had a wide range of habitats, which were located in tropical and subtropical regions and were therefore less affected by glacial periods. The present study reveals that during the LGM, the habitat of O. curvinotus was mainly located in the northern margin of Sundaland, including Guangdong Province, Guangxi Zhuang Autonomous Region and Hainan Province in South China, as well as the northern part of the South China Sea and the Beibu Gulf (Figure 4). The total potential habitat area reached 126.85×104 km2, of which 30.83×104 km2 were highly suitable. In addition, during the LGM, there were three refuges (Refugium 1, Refugium 2 and Refugium 3). Refugium 1 was located in Beibu Gulf, Refugium 2 was located near Guanghai Town, Taishan County, Jiangmen City, Guangdong Province, and Refugium 3 was located in the east of Hong Kong (Figure 5).
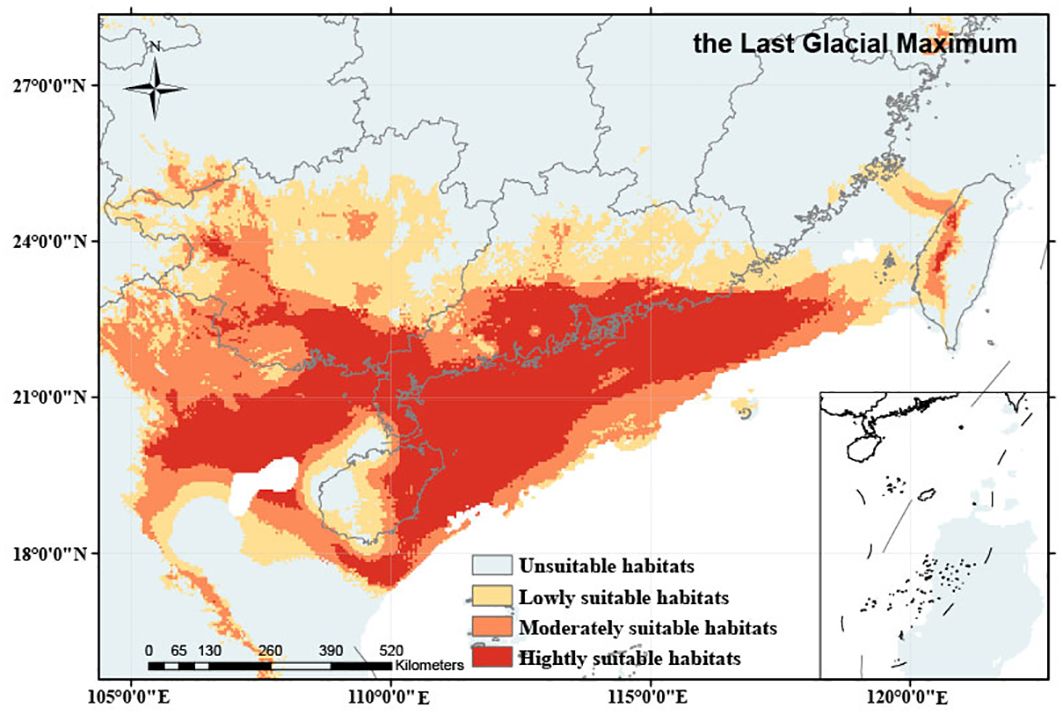
Figure 4. Potential distribution areas of O. curvinotus with different suitability under paleoclimate (the LGM).
Time series analysis in the warming future
We simulated the potential habitat shift process of O. curvinotus under four climate warming scenarios with different carbon emission levels, as shown in Figures 6–9. Under the background of a low-end level carbon emission scenario (SSP1-2.6), the potential habitats will expand from the current to 2080 (for example, the lowly suitable habitats at the border of Guangdong Province and Guangxi Zhuang Autonomous Region in China are obviously expanding northward), but it will shrink by 2100. By 2100, the habitats in the border area between Guangdong Province and Guangxi Zhuang Autonomous Region, east Guangdong region and the coast of Fujian decreased significantly, but the highly suitable habitats in Leizhou Peninsula, northeast Hainan Island and southwest Taiwan Island increased slightly (Figures 6A–D). Under the scenario of low-moderate level carbon emission (SSP2-4.5), there is a trend of habitat expansion from the current to 2040, and then a trend of retreat until 2100 (Figures 7A–D). During this period, the highly suitable habitats in Leizhou Peninsula and northeast Hainan will increase (Figure 7D). Under the scenario of medium-high or high levels of carbon emissions, the potential habitats of O. curvinotus show oscillating changes, expanding from the present to 2040, then slightly shrinking in 2060, recovering in 2080, and shrinking again in 2100 (Figures 8A–D). As in the previous cases, highly suitable habitats in Leizhou Peninsula, northeastern Hainan, and southwestern Taiwan are expected to expand by 2100 (Figures 9A–D). In terms of area change, from the current to 2100, the four warming scenarios under carbon emission levels caused the potentially suitable habitat (including highly, moderately and lowly suitable habitats) of O. curvinotus to first expand, then stabilize, and finally retreat to a slightly higher area than the current area (Figures 10A–D).
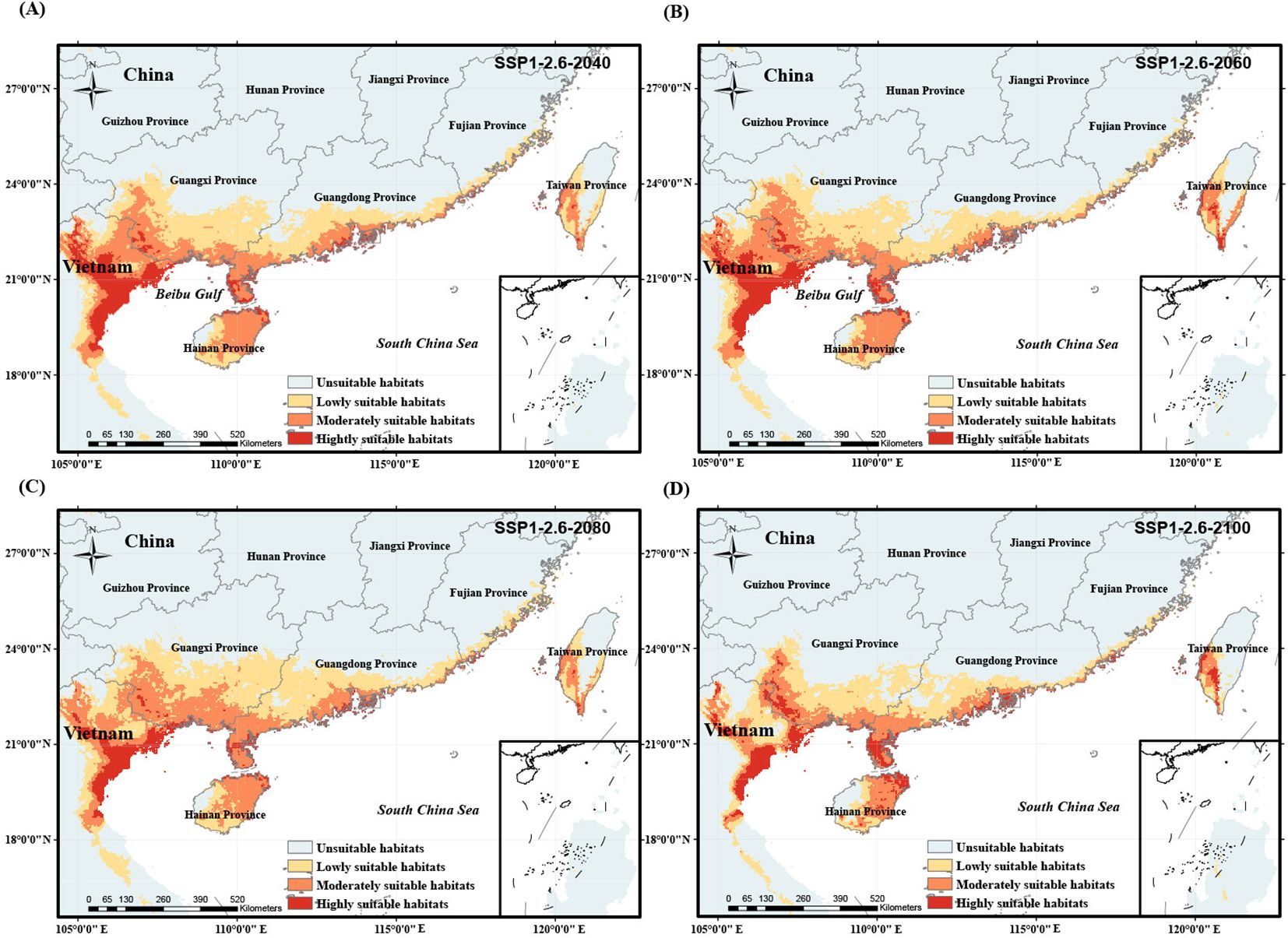
Figure 6. Potentially suitable habitat of O. curvinotus under future climate warming scenario with the low-end level carbon emission from 2040 to 2100. (A) SSP1-2.6-2040; (B) SSP1-2.6-2060; (C) SSP1-2.6-2080; (D) SSP1-2.6-2100.
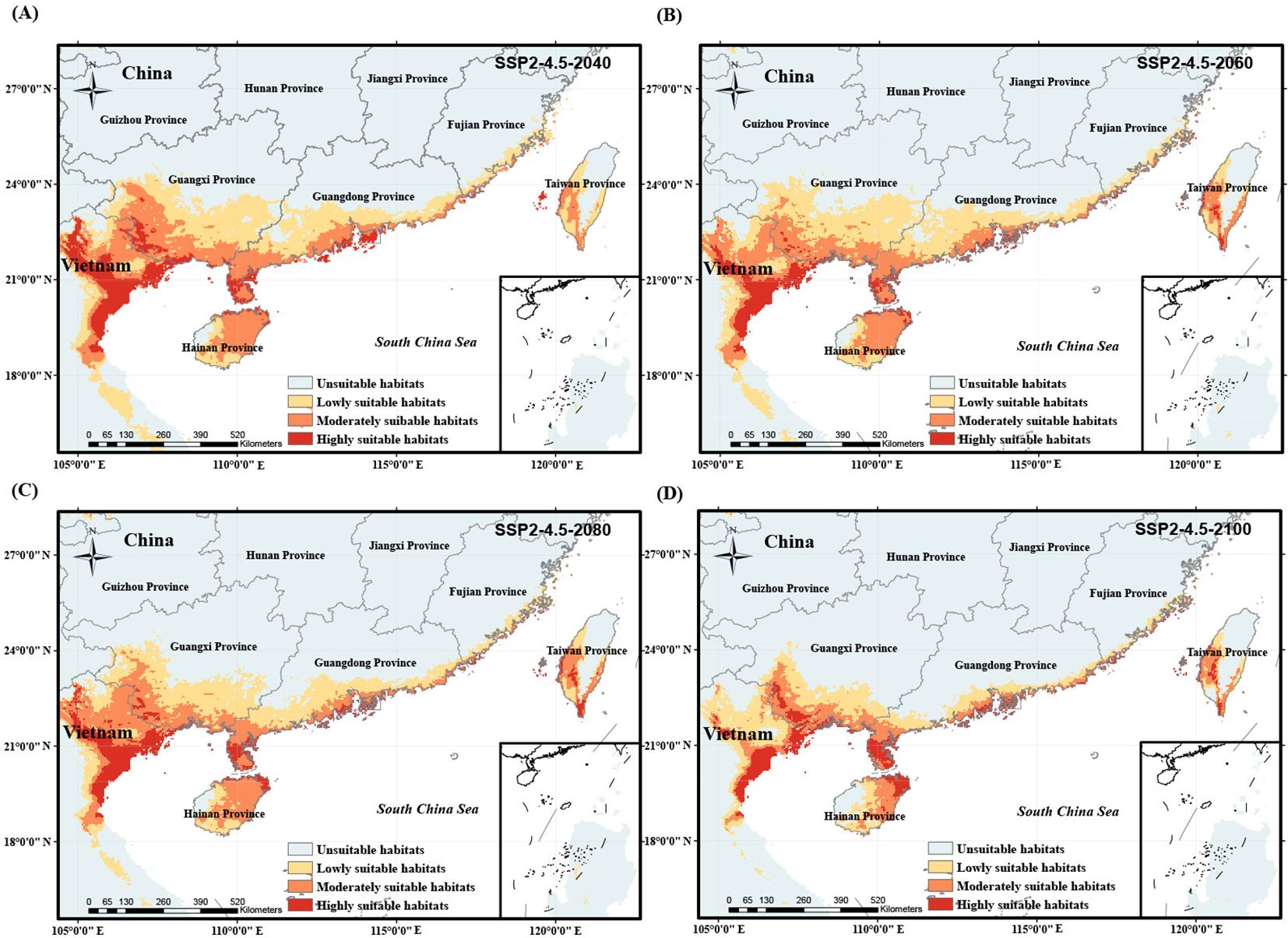
Figure 7. Potentially suitable habitat of O. curvinotus under future climate warming scenario with the low-moderate level carbon emission from 2040 to 2100. (A) SSP2-4.5-2040; (B) SSP2-4.5-2060; (C) SSP2-4.5-2080; (D) SSP2-4.5-2100.
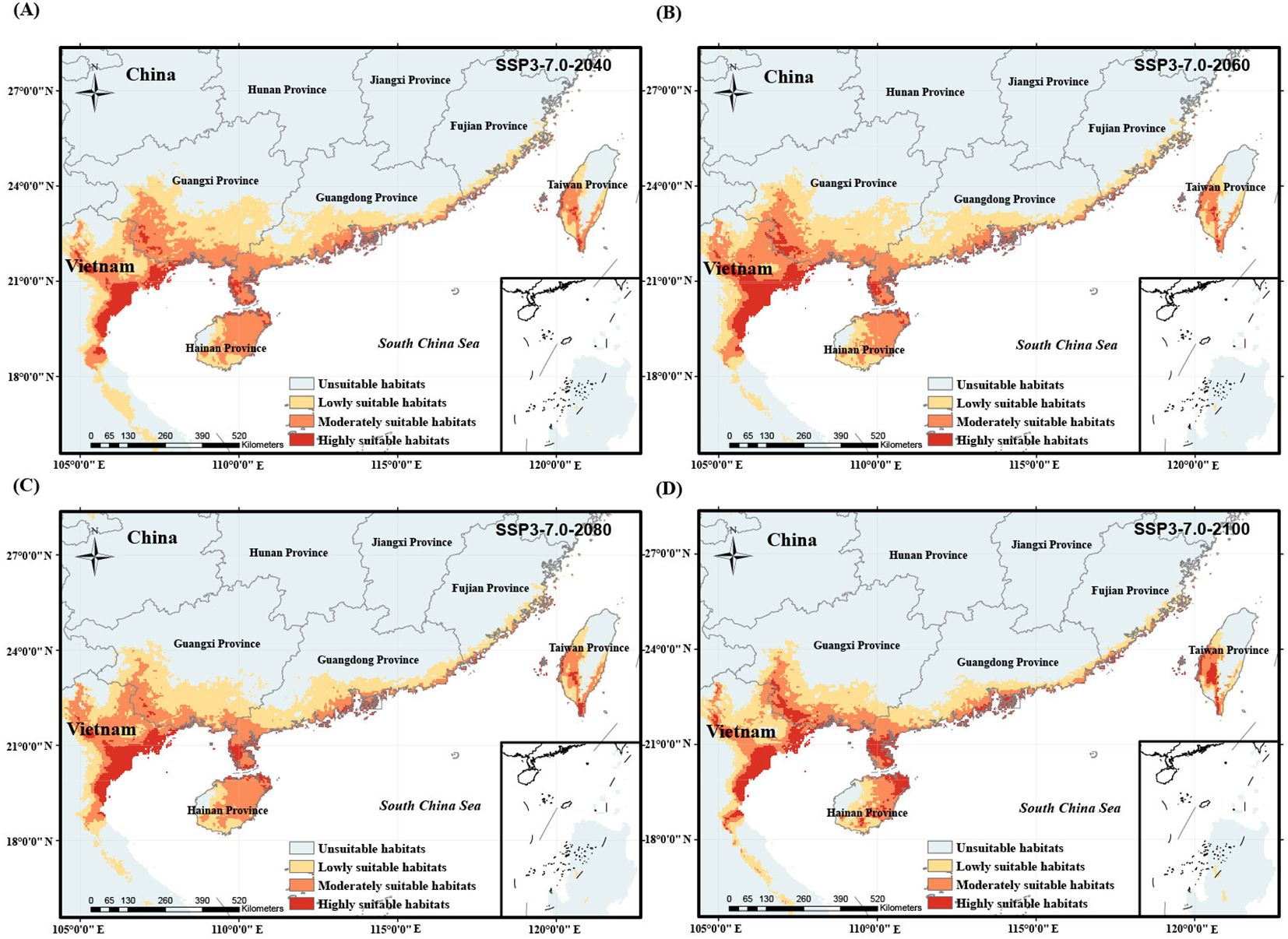
Figure 8. Potentially suitable habitat of O. curvinotus under future climate warming scenario with the medium-high level carbon emission from 2024 to 2100. (A) SSP3-7.0-2040; (B) SSP3-7.0-2060; (C) SSP3-7.0-2080; (D) SSP3-7.0-2100.
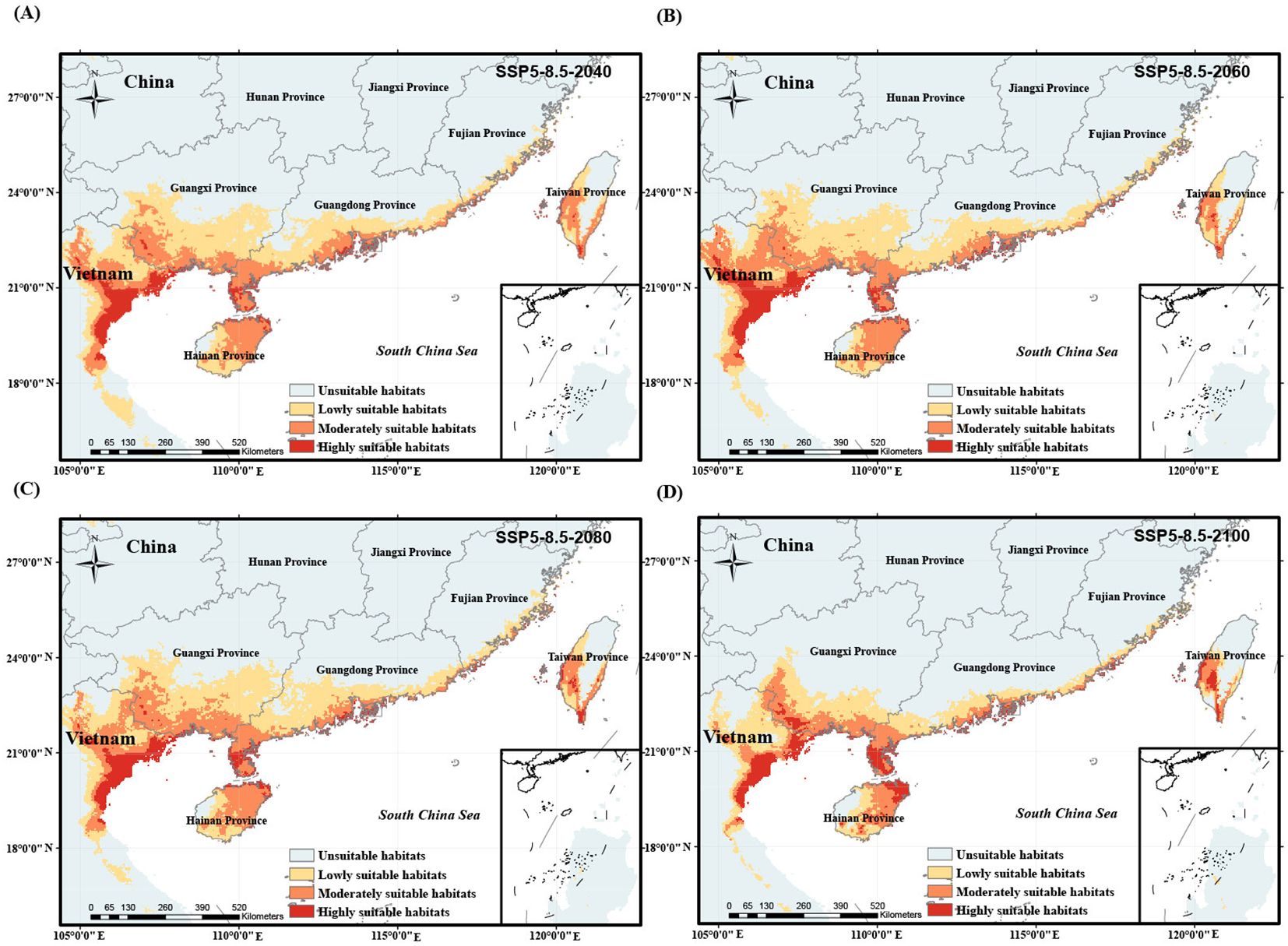
Figure 9. Potentially suitable habitat of O. curvinotus under future climate warming scenario with the high level carbon emission from 2040 to 2100. (A) SSP5-8.5-2040; (B) SSP5-8.5-2060; (C) SSP5-8.5-2080; (D) SSP5-8.5-2100.
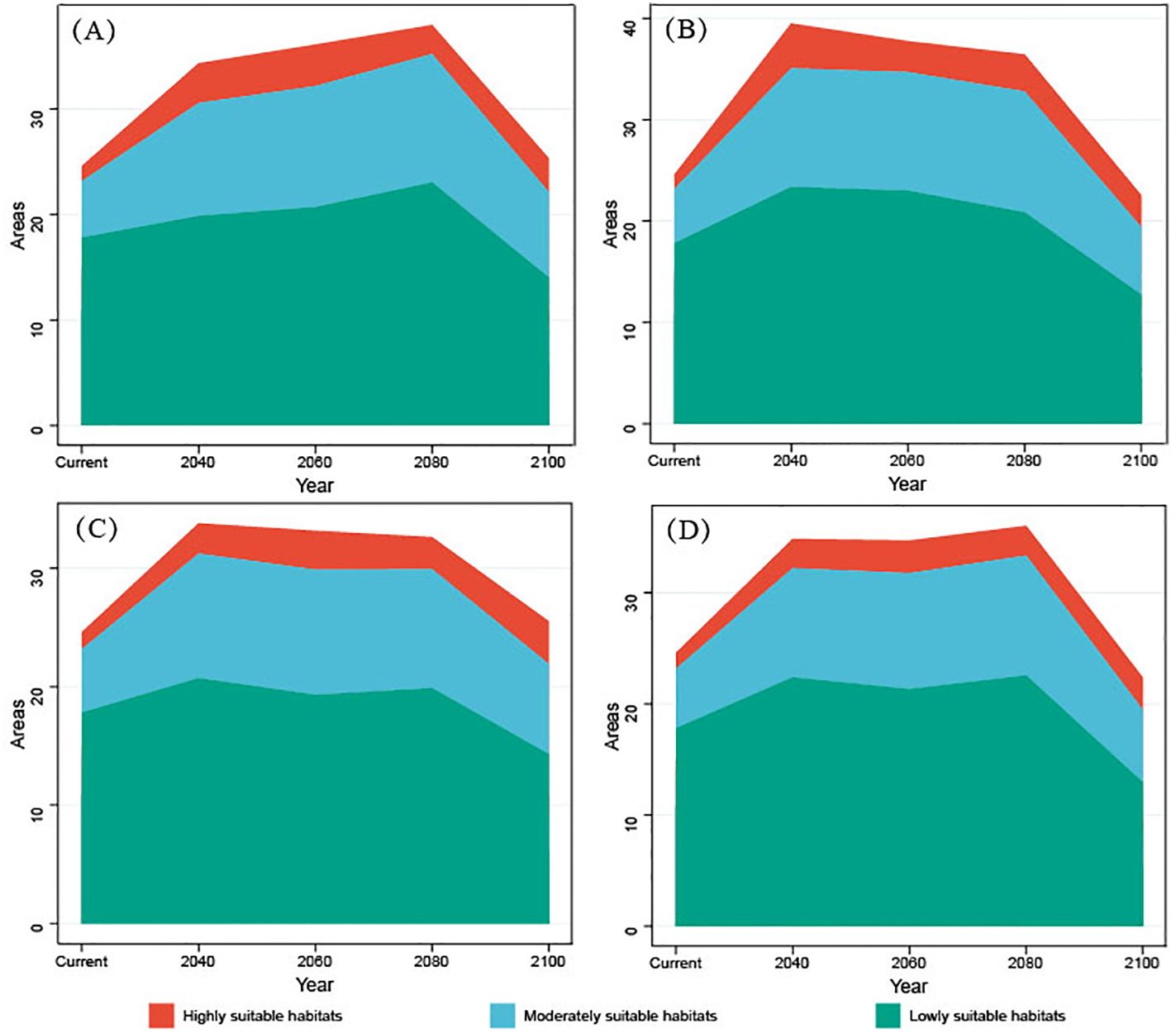
Figure 10. Time series analysis of potential habitat areas (×104 km2) of O. curvinotus from current to future 2100. (A) SSP5-8.5-2040; (B) SSP5-8.5-2060; (C) SSP5-8.5-2080; (D) SSP5-8.5-2100.
Discussion
O. curvinotus is a typical small tropical fish mainly found in the northern coast of the South China Sea. Generally, species with limited distributions tend to have narrower ecological niches, such as smaller temperature variations within their range, making them more susceptible to climate change (Liao et al., 2022). The habitats of species in tropical regions are typically characterized by high humidity and warmth (Skendžić et al., 2021). For instance, Neurobasis chinensis inhabits the hot and humid tropical regions of East Asia and is projected to expand its range northward under future warming climates (Skendžić et al., 2021; Liao et al., 2022). However, humidity is not a significant factor for underwater species throughout their lives. In this study, we determined that a mean diurnal temperature range of 8 °C is the critical point for the distribution range of O. curvinotus. In areas where the mean diurnal temperature exceeds 8 °C, O. curvinotus has virtually no suitable habitat. This is consistent with its status as a tropical species. Tropical species, especially tropical plants, are affected more by climate change than by topographic gradients (Toledo et al., 2012). Climate is a strong driver of species distribution, with 72% of tropical species affected by temperature (Toledo et al., 2012). In this study, the habitat of O. curvinotus has typical characteristics of tropical species, and only inhabits within the range of mean diurnal temperature of 8°C, especially within 6°C, which is a highly suitable habitat for it. This reveals that one of the habitat characteristics of O. curvinotus is a small range of the mean diurnal temperature. Another relatively important climatic factor shaping the distribution of O. curvinotus is the mean temperature of wettest quarter (bio8, contribution 11.62%, permutation importance 46.34%). The mean temperature of wettest quarter in the habitat area of O. curvinotus is more than 25 °C, with the highly suitable habitat exceeding 28 °C. These findings again confirm the habitat properties of O. curvinotus as a tropical species.
Our results revealed that the current distribution area is mainly concentrated in northern Vietnam, northeastern Hainan Province, the coast of Guangdong Province and Guangxi Zhuang Autonomous Region, and a small part of Taiwan Province, in total, covered ca. 17.82×104 km2. Within these ranges, a small number of simulated habitats exist in Taiwan Province of China, which is unexpected and requires further field work to confirm. In addition, it is worth noting that our field work experience confirmed the existence of a large population of O. curvinotus in Sanya, Hainan, and we brought the population of O. curvinotus in this area back to the laboratory for morphological and DNA barcoding identification, confirming the identity of O. curvinotus (Yao et al., 2022). However, the simulation results of this study suggest that Sanya in Hainan Province is not a suitable habitat for O. curvinotus. We speculate that the Sanya population may have undergone adaptive evolution in order to meet the changing climate environment, but the evolution has not yet reached the species level. Our earlier study corroborated these results across various dimensions (Dong et al., 2021; Yao et al., 2022). In our previous research, we discovered that the Sanya population lacked the sex determination gene “dmy” when compared to other populations (Dong et al., 2021). Additionally, Yao et al. (2022) revealed a significant level of genetic differentiation between the Sanya population and others, without indicating species diversification. Currently, the Sanya population is experiencing a bottleneck phase (characterized by a small population size), confirming that it has undergone intense environmental selection (Yao et al., 2022). All of these evidences indicate that the Sanya population is a unique group, exhibiting genetic variations that have emerged as adaptations to the challenging environment of Sanya, located in the China’s Hainan province. Regarding the relationship between the unique physiological response mechanism of O. curvinotus populations in Sanya and climate change, we have conducted a thorough analysis of the species’ resilience or vulnerability in the face of climate change. Firstly, as a euryhaline fish, O. curvinotus possesses physiological characteristics that endow it with remarkable tolerance to salinity fluctuations. Against the backdrop of climate change, the rise in sea level and fluctuations in freshwater input may lead to drastic changes in salinity levels in mangrove tidal creeks and streams. Nevertheless, through a series of unique physiological response mechanisms, such as the fine-tuned regulation of ion transport proteins, O. curvinotus effectively maintains the balance of salts within its body, ensuring the continuation of normal physiological functions even in varying salinity environments. This genetic adaptability provides significant resilience to O. curvinotus in the face of salinity fluctuations, facilitating its survival and reproduction in diverse environments.
However, the impact of climate change on O. curvinotus is not entirely positive. With the persistent increase in global temperatures and the frequent occurrence of extreme climate events, these environmental factors may exceed the adaptive range of O. curvinotus. For instance, high-temperature environments may significantly increase the metabolic rate of O. curvinotus, subsequently escalating its demand for food and oxygen. Under resource-limited conditions, such an elevation in metabolic rate could pose a threat to the survival of O. curvinotus. Furthermore, extreme climate events like heavy rainfall or drought can directly destroy the habitats of O. curvinotus, leading to severe degradation of its living environment. In such scenarios, the genetic adaptability of O. curvinotus may be insufficient to fully cope with these drastic changes, thus exhibiting a degree of vulnerability. However, the adaptation of O. curvinotus to this sudden climate change requires at least several generations of natural selection. Therefore, we believe that the genetic adaptability of O. curvinotus exhibits both resilience and vulnerability in response to climate change. To gain a more comprehensive understanding of this relationship, further research is needed to explore the interaction between genetic variations in O. curvinotus and climate change, as well as to delve deeper into its adaptive mechanisms. This will aid us in providing a more scientific basis for the conservation and utilization of O. curvinotus, while also serving as an important reference for addressing the biodiversity challenges posed by climate change.
The historical species distribution pattern in the Quaternary largely determines their current species range. The conservatism of climatic niches, combined with the cycles of glacial and interglacial periods, has forced many species to reduce their ranges in order to survive under the advance of Pleistocene ice sheets. Refugia provide geographical opportunities for species to retreat, survive, and later recolonize under favorable environmental conditions (Morales-Barbero et al., 2018). Our research has revealed three refugia, all located in the northern part of Sundaland (the northern edge of the South China Sea). Among them, the largest one, Refugium 1, is speculated to be the starting point of the dispersal of highly suitable habitats in the northern part of Vietnam and Leizhou Peninsula. While Refugium 2 and Refugium 3 are speculated to be the sources of species migration for the highly suitable habitats in the Pearl River Delta region. Typically, species range may expand or contract based on the suitability of climate change for its ecological niche (Morales-Barbero et al., 2018). Migrant species may be geographically restricted to a particular location, or continue their colonization route through a habitat matrix, depending on the suitability of climatic conditions (Hewitt, 2000; Petit et al., 2003; Barnosky, 2005). Due to the disappearance of Sundaland after the glacial period, many habitats of O. curvinotus sank into the sea, and highly suitable habitats migrated to neighboring northern Vietnam, Leizhou Peninsula, and a small part of the Pearl River Delta region. Overall, the habitats of O. curvinotus have been in a stage of retraction from the Quaternary to the present day.
Future warming is an undeniable reality. Over the past century, the global temperature has risen by 0.6°C and is continuing to change at an accelerated pace. Projections indicate that by the end of the 21st century, the minimum temperature increase is expected to be between 0.3°C and 1.7°C, with a maximum increase of 2.6°C to 4.8°C (Root et al., 2003; Kumar and Rawat, 2022; Liao et al., 2022, 2023). Climate warming is inevitable, and it will undoubtedly alter the current distribution patterns of various species. The impacts of warming have already been observed in numerous organisms, including plants, dragonflies, butterflies, grasshoppers, lacewings, spiders, reptiles, woodlice, ground beetles, longhorn beetles, soldier beetles, harvestmen, millipedes, aquatic bugs, freshwater fish, birds, and mammals (Parmesan and Yohe, 2003; Root et al., 2003; Hickling et al., 2005, 2006; Lenoir and Svenning, 2015). Tropical species with narrow ecological niche are theoretically vulnerable to the stress of climate warming and migrate northward like most species. However, the subjects studied in this research did not follow this typical pattern. In our study, climate warming under four different carbon emission levels caused the current habitat of O. curvinotus to expand within a short timeframe (by 2040). After a period of adaptation (by 2100), the expansion trend was mitigated, and the expansion area retreated to slightly larger than the current climate scenario. The increase of habitats primarily occurred in northern Vietnam, southern Guangxi, Hainan Island and Taiwan Island, with a notable increase in highly suitable habitats, such as the Red River Delta in Vietnam and the central part of Leizhou Peninsula. Contrary to the typical northward migration pattern observed in other species under the influence of warming (Hickling et al., 2006), the highly suitable habitats for O. curvinotus did not shift latitudinally but instead increased their area around 21°N. East Asia, at 21 degrees north latitude, is a global biodiversity hotspot, and the region’s geological history has shaped a complex climate and geological environment in this area (Hekinian and Walker, 1987; Marchese, 2015), resulting in a complex network of biological and abiotic factors. Numerous species have come to rely on this hotspot habitat, making it a crucial ecosystem for various organisms (Gathorne-Hardy et al., 2002; Gorog et al., 2004). Our subject, O. curvinotus, despite its adaptability, is currently found only in a small area along the northern coast of the South China Sea, including coastal freshwater extending inland. Hayakawa et al. (2015) discovered this species in mangrove plantations and inland paddy fields in Bang La, Do Son, Vietnam, and conducted population genetics studies, recognizing it as a promising experimental model species in tropical Asia (Hayakawa et al., 2015). These provide valuable insight into the dispersal of O. curvinotus into inland freshwater habitats at the same latitude. Indeed, our simulations of O. curvinotus habitats in the context of future climate warming support this notion and underscore the need for further investigation into the species’ ecological niche and potential adaptability to changing environmental conditions.
Despite our optimistic findings regarding the recent survival status of this species, proactive conservation measures must still be implemented to secure its long-term survival. To this end, we propose the following conservation strategies: Firstly, monitoring and surveillance: We plan to establish a regular monitoring program to closely track the species’ population dynamics, habitat utilization, and any emerging threats. This will involve conducting surveys, monitoring breeding habitats, and collecting crucial data using remote sensing techniques. These data will not only aid in evaluating the effectiveness of our conservation efforts but also guide future actions. Secondly, habitat restoration and enhancement: We prioritize the restoration and enhancement of the species’ existing habitats, including contaminant removal, controlling invasive species, and promoting native vegetation growth. Additionally, we aim to establish new habitats in suitable areas to mitigate the potential negative impacts of predicted habitat shifts.
Conclusions
This study summarizes the habitat attributes, potential distribution areas and its long-term temporal changes of a small euryhaline fish species. Results show that O. curvinotus is currently mainly distributed along the coastal areas of northern Vietnam, northeastern Hainan, Guangdong and Guangxi Zhuang Autonomous Region, as well as in a few areas of Taiwan Province, China, covering a total area of 17.82 ×104 km2. Highly suitable habitats are mainly found in northern Vietnam, central Leizhou Peninsula, and the western coast of the Pearl River Delta. Three refugia existed in historical times, with the largest one, Refugium 1, speculated to be responsible for the dispersal and establishment of highly suitable habitats in northern Vietnam and central Leizhou Peninsula. Refugium 2 and Refugium 3 are hypothesized to be the sources of immigrant individuals with highly suitable habitats in the Pearl River Delta, with only Refugium 2 remaining to current. Climate warming in the future is not expected to pose a threat to the species’ distribution. Instead, it may lead to a rapid expansion of its habitat in a short period of time, followed by a stabilization and gradual decrease to slightly above the current habitat area. The expansion of its habitat will not shift northward as most species do, but will expand around the 21°N latitude. This study provides valuable insights for the conservation of such small euryhaline fish species and the development of model species suitable for ecological environmental research in the region.
Data availability statement
The original contributions presented in the study are included in the article/Supplementary Material. Further inquiries can be directed to the corresponding author.
Author contributions
JL: Data curation, Investigation, Formal analysis, Conceptualization, Methodology, Software, Writing – original draft. HC: Data curation, Investigation, Writing – review & editing. J-YL: Writing – review & editing. G-CL: Writing – review & editing. XG: Writing – review & editing. C-FL: Data curation, Writing – review & editing. Y-SG: Investigation, Methodology, Writing – review & editing. Z-DD: Writing – review & editing. Z-DW: Conceptualization, Investigation, Data curation, Supervision, Writing – review & editing.
Funding
The author(s) declare financial support was received for the research, authorship, and/or publication of this article. This study was funded by the Guangdong Ocean University Scientific Research Startup Funding Project (Grant No. 060302022312), the Guangdong Provincial Ordinary University Youth Innovative Talent Project in 2024 (Grant No. 2024KQNCX134), the Guangdong Provincial Special Fund Project for Talent Development Strategy in 2024 (Grant No. 2024R3005), and the Science and Technology Planning Projects of Guangdong Province (Grant No.2021B1212050025). National key research and development program of China (2024YFD2401803).
Acknowledgments
We are deeply grateful to our esteemed colleagues, Shuisheng Long, Shun Zhang, Hairui Zhang, Chengqin Huang, Chun Wang, and Shunkai Huang, for their invaluable assistance during the field investigation.
Conflict of interest
The authors declare that the research was conducted in the absence of any commercial or financial relationships that could be construed as a potential conflict of interest.
Publisher’s note
All claims expressed in this article are solely those of the authors and do not necessarily represent those of their affiliated organizations, or those of the publisher, the editors and the reviewers. Any product that may be evaluated in this article, or claim that may be made by its manufacturer, is not guaranteed or endorsed by the publisher.
Supplementary material
The Supplementary Material for this article can be found online at: https://www.frontiersin.org/articles/10.3389/fmars.2024.1391800/full#supplementary-material
References
Alabdulhafith B., Binothman A., Alwahiby A., Haig S. M., Prommer M., Leonardi G. (2022). Predicting the potential distribution of a near-extinct avian predator on the Arabian Peninsula: implications for its conservation management. Environ. Monit. Assess. 194, 535. doi: 10.1007/s10661-022-10225-2
Araújo M. B., Rahbek C. (2006). How does climate change affect biodiversity? Science 313, 1396–1397. doi: 10.1126/science.1131758
Barnosky A. D. (2005). Effects of quaternary climatic change on speciation in mammals. J. Mamm. Evol. 12, 247–264. doi: 10.1007/s10914-005-4858-8
Berrang-Ford L., Ford J. D., Paterson J. (2011). Are we adapting to climate change? Glob. Environ. Change-Human. Policy Dimens. 21, 25–33. doi: 10.1016/j.gloenvcha.2010.09.012
Chen I.-C., Hill J. K., Ohlemüller R., Roy D. B., Thomas C. D. (2011). Rapid range shifts of species associated with high levels of climate warming. Science 333, 1024–1026. doi: 10.1126/science.1206432
Dong Z., Li X., Huang S., Zhang N., Guo Y., Wang Z. (2020). Vitellogenins and choriogenins are biomarkers for monitoring Oryzias curvinotus juveniles exposed to 17 β - estradiol. Comp. Biochem. Physiol. C-Toxicol. Pharmacol. 236, 108800. doi: 10.1016/j.cbpc.2020.108800
Dong Z., Li X., Yao Z., Wang C., Guo Y., Wang Q., et al. (2021). Oryzias curvinotus in Sanya Does Not Contain the Male Sex-Determining Gene dmy. Animals 11, 1327. doi: 10.3390/ani11051327
Fick S. E., Hijmans R. J. (2017). WorldClim 2: new 1-km spatial resolution climate surfaces for global land areas. Int. J. Climatol. 37, 4302–4315. doi: 10.1002/joc.5086
Gao D., Kong C., Liao H., Junaid M., Pan T., Chen X., et al. (2023). Interactive effects of polystyrene nanoplastics and 6:2 chlorinated polyfluorinated ether sulfonates on the histomorphology, oxidative stress and gut microbiota in Hainan Medaka (Oryzias curvinotus). Sci. Total. Environ. 880, 163307. doi: 10.1016/j.scitotenv.2023.163307
Gathorne-Hardy F. J., Syaukani, Davies R. G., Eggleton P., Jones D. T. (2002). Quaternary rainforest refugia in south-east Asia: using termites (Isoptera) as indicators. Biol. J. Linn. Soc 75, 453–466. doi: 10.1046/j.1095-8312.2002.00031.x
Gorog A. J., Sinaga M. H., Engstrom M. D. (2004). Vicariance or dispersal? Historical biogeography of three Sunda shelf murine rodents (Maxomys surifer, Leopoldamys sabanus and Maxomys whiteheadi). Biol. J. Linn. Soc 81, 91–109. doi: 10.1111/j.1095-8312.2004.00281.x
Hamaguchi S., Sakaizumi M. (1992). Sexually differentiated mechanisms of sterility in interspecific hybrids between Oryzias latipes and O. curvinotus. J. Exp. Zool. 263, 323–329. doi: 10.1002/jez.1402630312
Hamaguchi S., Toyazaki Y., Shinomiya A., Sakaizumi M. (2004). The XX-XY Sex-determination System in Oryzias luzonensis and O. mekongensis Revealed by the Sex Ratio of the Progeny of Sex-reversed Fish. Zool. Sci. 21, 1015–1018. doi: 10.2108/zsj.21.1015
Hayakawa H., Le Q. D., Kinoshita M., Takehana Y., Sakuma K., Takeshima H., et al. (2015). Genetic similarity of the Hainan medaka populations collected from hyper- and hypo-osmotic environments in northern Vietnam. Ocean. Sci. J. 50, 231–235. doi: 10.1007/s12601-015-0020-6
Hekinian R., Walker D. (1987). Diversity and spatial zonation of volcanic rocks from the East Pacific Rise near 21° N. Contrib. Mineral. Petrol. 96, 265–280. doi: 10.1007/BF00371248
Hewitt G. (2000). The genetic legacy of the Quaternary ice ages. Nature 405, 907–913. doi: 10.1038/35016000
Hickling R., Roy D. B., Hill J. K., Fox R., Thomas C. D. (2006). The distributions of a wide range of taxonomic groups are expanding polewards. Glob. Change Biol. 12, 450–455. doi: 10.1111/j.1365-2486.2006.01116.x
Hickling R., Roy D. B., Hill J. K., Thomas C. D. (2005). A northward shift of range margins in British Odonata. Glob. Change Biol. 11, 502–506. doi: 10.1111/j.1365-2486.2005.00904.x
Kamei Y., Itou J., Oda S., Masui M., Kim J., Ishikawa T., et al. (2007). Development of a convenient in vitro fertilization method using interspecific hybrids between Oryzias latipes and Oryzias curvinotus. Dev. Growth Differ. 49, 721–730. doi: 10.1111/j.1440-169X.2007.00966.x
Kappelle M., Vuuren M. M. I. V., Pieter Baas (1999). Effects of climate change on biodiversity: a review and identification of key research issues. Biodivers. Conserv. 8, 1383–1397. doi: 10.1023/A:1008934324223
Kato M., Takehana Y., Fukuda Y., Naruse K., Sakaizumi M., Hamaguchi S. (2011). An autosomal locus controls sex reversal in interspecific XY hybrids of the medaka fishes. Heredity 107, 523–529. doi: 10.1038/hdy.2011.38
Kato M., Takehana Y., Sakaizumi M., Hamaguchi S. (2010). A sex-determining region on the Y chromosome controls the sex-reversal ratio in interspecific hybrids between Oryzias curvinotus females and Oryzias latipes males. Heredity 104, 191–195. doi: 10.1038/hdy.2009.114
Koga A., Hori H., Ishikawa Y. (2002). Gamera, a family of LINE-like repetitive sequences widely distributed in medaka and related fishes. Heredity 89, 446–452. doi: 10.1038/sj.hdy.6800162
Kondo M., Nagao E., Mitani H., Shima A. (2001). Differences in recombination frequencies during female and male meioses of the sex chromosomes of the medaka, Oryzias latipes. Genet. Res. 78, 23–30. doi: 10.1017/S0016672301005109
Kumar D., Rawat S. (2022). Modeling the effect of climate change on the distribution of threatened medicinal orchid Satyrium Nepalense D. Don in India. Environ. Sci. pollut. Res. 29, 72431–72444. doi: 10.1007/s11356-022-20412-w
Lenoir J., Svenning J.-C. (2015). Climate-related range shifts – a global multidimensional synthesis and new research directions. Ecography 38, 15–28. doi: 10.1111/ecog.00967
Li J., Du J., Deng A., Chen Z., Guo Y., Wang Z. (2022a). Comparative analysis of central corneal thickness in four fish models. Zebrafish 19, 224–228. doi: 10.1089/zeb.2022.0016
Li X., Mao F., Du H., Zhou G., Xing L., Liu T., et al. (2019). Spatiotemporal evolution and impacts of climate change on bamboo distribution in China. J. Environ. Manage. 248, 109265. doi: 10.1016/j.jenvman.2019.109265
Li J., Miao B., Wang S., Dong W., Xu H., Si C., et al. (2022b). Hiplot: a comprehensive and easy-to-use web service boosting publication-ready biomedical data visualization. Bioinformatics. 23, bbac261. doi: 10.1101/2022.03.16.484681
Liao J., Wang H., Xiao S., Guan Z., Zhang H., Dumont H. J., et al. (2022). Modeling and Prediction of the Species’ Range of Neurobasis chinensis (Linnaeus 1758) under Climate Change. Biology 11, 868. doi: 10.3390/biology11060868
Liao J., Wu Z., Wang H., Xiao S., Mo P., Cui X. (2023). Projected effects of climate change on species range of pantala flavescens, a wandering glider dragonfly. Biology 12, 226. doi: 10.3390/biology12020226
Ma B., Sun J. (2018). Predicting the distribution of Stipa purpurea across the Tibetan Plateau via the MaxEnt model. BMC Ecol. 18, 10. doi: 10.1186/s12898-018-0165-0
Marchese C. (2015). Biodiversity hotspots: A shortcut for a more complicated concept. Glob. Ecol. Conserv. 3, 297–309. doi: 10.1016/j.gecco.2014.12.008
Masaoka T., Okamoto H., Araki K., Nagoya H., Fujiwara A., Kobayashi T. (2012). Identification of the hybrid between Oryzias latipes and Oryzias curvinotus using nuclear genes and mitochondrial gene region. Mar. Genom. 7, 37–41. doi: 10.1016/j.margen.2012.06.002
Matsuda M., Sato T., Toyazaki Y., Nagahama Y., Hamaguchi S., Sakaizumi M. (2003). Oryzias curvinotus Has DMY, a Gene That Is Required for Male Development in the Medaka, O. latipes. Zool. Sci. 20, 159–161. doi: 10.2108/zsj.20.159
Meinshausen M., Nicholls Z. R. J., Lewis J., Gidden M. J., Vogel E., Freund M., et al. (2020). The shared socio-economic pathway (SSP) greenhouse gas concentrations and their extensions to 2500. Geosci. Model. Dev. 13, 3571–3605. doi: 10.5194/gmd-13-3571-2020
Mkala E. M., Mwanzia V., Nzei J., Oluoch W. A., Ngarega B. K., Wanga V. O., et al. (2023). Predicting the potential impacts of climate change on the endangered endemic annonaceae species in east africa. Heliyon 9, e17405. doi: 10.1016/j.heliyon.2023.e17405
Morales-Barbero J., Martinez P. A., Ferrer-Castán D., Olalla-Tárraga M. Á. (2018). Quaternary refugia are associated with higher speciation rates in mammalian faunas of the Western Palaearctic. Ecography 41, 607–621. doi: 10.1111/ecog.02647
Naimi B., Hamm N. A. S., Groen T. A., Skidmore A. K., Toxopeus A. G. (2014). Where is positional uncertainty a problem for species distribution modelling? Ecography 37, 191–203. doi: 10.1111/j.1600-0587.2013.00205.x
Parmesan C., Yohe G. (2003). A globally coherent fingerprint of climate change impacts across natural systems. Nature 421, 37–42. doi: 10.1038/nature01286
Petit R. J., Aguinagalde I., De Beaulieu J.-L., Bittkau C., Brewer S., Cheddadi R., et al. (2003). Glacial refugia: hotspots but not melting pots of genetic diversity. Science 300, 1563–1565. doi: 10.1126/science.1083264
Phillips S. J., Anderson R. P., Schapire R. E. (2006). Maximum entropy modeling of species geographic distributions. Ecol. Model. 190, 231–259. doi: 10.1016/j.ecolmodel.2005.03.026
Phillips S. J., Dudík M. (2008). Modeling of species distributions with Maxent: new extensions and a comprehensive evaluation. Ecography 31, 161–175. doi: 10.1111/j.0906-7590.2008.5203.x
Root T. L., Price J. T., Hall K. R., Schneider S. H., Rosenzweig C., Pounds J. A. (2003). Fingerprints of global warming on wild animals and plants. Nature 421, 57–60. doi: 10.1038/nature01333
Sérgio C., Figueira R., Draper D., Menezes R., Sousa A. J. (2007). Modelling bryophyte distribution based on ecological information for extent of occurrence assessment. Biol. Conserv. 135, 341–351. doi: 10.1016/j.biocon.2006.10.018
Shiga T., Suzuki N. (2004). Expression of guanylyl cyclase genes in medaka hybrids (Oryzias curvinotus×Oryzias latipes). Comp. Biochem. Physiol. B-Biochem. Mol. Biol. 139, 281–286. doi: 10.1016/j.cbpc.2004.08.001
Shinomiya A., Kato M., Yaezawa M., Sakaizumi M., Hamaguchi S. (2006). Interspecific hybridization between Oryzias latipes and Oryzias curvinotus causes XY sex reversal. J. Exp. Zool. 305A, 890–896. doi: 10.1002/jez.a.330
Skendžić S., Zovko M., Živković I. P., Lešić V., Lemić D. (2021). The impact of climate change on agricultural insect pests. Insects 12, 440. doi: 10.3390/insects12050440
Tognelli M. F., Roig-Juñent S. A., Marvaldi A. E., Flores G. E., Lobo J. M. (2009). An evaluation of methods for modelling distribution of Patagonian insects. Rev. Chil. Hist. Nat. 82, 347–360. doi: 10.4067/S0716-078X2009000300003
Toledo M., Peña-Claros M., Bongers F., Alarcón A., Balcázar J., Chuviña J., et al. (2012). Distribution patterns of tropical woody species in response to climatic and edaphic gradients. J. Ecol. 100, 253–263. doi: 10.1111/j.1365-2745.2011.01890.x
Wang Z., Long S., Liao J., Huang C., Zhang H., Huang S., et al. (2017). Complete mitogenome of Hainan medaka Oryzias curvinotus (Teleostei: Beloniformes) and transcriptional differences between male and female liver. Mitochondrial. DNA Part B-Resour. 2, 157–158. doi: 10.1080/23802359.2017.1303340
Williams J. N., Seo C., Thorne J., Nelson J. K., Erwin S., O’Brien J. M., et al. (2009). Using species distribution models to predict new occurrences for rare plants. Divers. Distrib. 15, 565–576. doi: 10.1111/j.1472-4642.2009.00567.x
Xiao S., Li S., Wang X., Chen L., Su T. (2022). Cedrus distribution change: past, present, and future. Ecol. Indic. 142, 109159. doi: 10.1016/j.ecolind.2022.109159
Yao Z., Long S., Wang C., Huang C., Zhang H., Jian L., et al. (2022). Population genetic characteristics of Hainan medaka with whole-genome resequencing. Front. Genet. 13. doi: 10.3389/fgene.2022.946006
Yi Y., Cheng X., Yang Z.-F., Zhang S.-H. (2016). Maxent modeling for predicting the potential distribution of endangered medicinal plant (H. riparia Lour) in Yunnan, China. Ecol. Eng. 92, 260–269. doi: 10.1016/j.ecoleng.2016.04.010
Young B. E., Franke I., Hernandez P. A., Herzog S. K., Paniagua L., Tovar C., et al. (2009). Using spatial models to predict areas of endemism and gaps in the protection of andean slope birds. Auk. 126, 554–565. doi: 10.1525/auk.2009.08155
Yuan H.-S., Wei Y.-L., Wang X.-G. (2015). Maxent modeling for predicting the potential distribution of Sanghuang, an important group of medicinal fungi in China. Fungal Ecol. 17, 140–145. doi: 10.1016/j.funeco.2015.06.001
Keywords: Oryzias curvinotus, climate change, habitats, tropical species, distribution
Citation: Liao J, Chen H, Li J-Y, Li G-C, Guan X, Liang C-F, Guo Y-S, Dong D-Z and Wang Z-D (2024) Habitat changes of a small endemic euryhaline fish species in the northern margin of the South China Sea under the background of global warming. Front. Mar. Sci. 11:1391800. doi: 10.3389/fmars.2024.1391800
Received: 26 February 2024; Accepted: 26 November 2024;
Published: 23 December 2024.
Edited by:
Angel Borja, Marine Research Division, SpainReviewed by:
Lei Xu, Chinese Academy of Fishery Sciences (CAFS), ChinaMario Lepage, INRAE Nouvelle-Aquitaine Bordeaux, France
Copyright © 2024 Liao, Chen, Li, Li, Guan, Liang, Guo, Dong and Wang. This is an open-access article distributed under the terms of the Creative Commons Attribution License (CC BY). The use, distribution or reproduction in other forums is permitted, provided the original author(s) and the copyright owner(s) are credited and that the original publication in this journal is cited, in accordance with accepted academic practice. No use, distribution or reproduction is permitted which does not comply with these terms.
*Correspondence: Zhong-Duo Wang, d2FuZ3pkQGdkb3UuZWR1LmNu
†ORCID: Jian Liao, orcid.org/0009-0005-0459-2060