- 1Chemical Oceanography and Marine Pollution Department, National Institute for Marine Research and Development “Grigore Antipa”, Constanta, Romania
- 2Physical Oceanography and Coastal Engineering Department, National Institute for Marine Research and Development “Grigore Antipa”, Constanta, Romania
- 3National Institute for Marine Research and Development “Grigore Antipa”, Constanta, Romania
- 4Ecology and Marine Biology Department, National Institute for Marine Research and Development “Grigore Antipa”, Constanta, Romania
- 5Finnish Environment Institute, Helsinki, Finland
This study aims to develop a methodology for identifying predominant pressures on the marine ecosystem, emphasizing the significance of examining these pressures and the necessity for management scenarios. The research focuses on how the Black Sea ecosystem responds to the combined effects of human pressures, climate change, and policies. An in-depth analysis was conducted on environmental pressures affecting the Romanian Black Sea, highlighting dominant pressures such as physical habitat loss, hydrocarbon introduction, and non-indigenous species invasion. The research employs a novel methodological approach to assess the implications of these pressures under different Shared Socioeconomic Pathways (SSPs): SSP1 “Taking the Green Road”, SSP2 “Middle of the Road”, and SSP5 “Taking the Highway”. The findings reveal a complex interplay between economic development and environmental conservation, with each pathway presenting distinct outcomes for marine ecosystems. Recent developments, including beach rehabilitation, maritime transport, and oil and gas exploitation, have overshadowed traditional pressures such as nutrient introduction and fishing. The study identifies the increasing vulnerability of critical habitats to anthropogenic pressures, with the rehabilitation of these ecosystems remaining challenging even under reduced pressures. The results underscore the need for adaptive management strategies to enhance the Black Sea ecosystem’s sustainability and resilience. The study’s insights are important for developing management strategies that address ongoing environmental challenges. This research provides knowledge for policymakers and stakeholders involved in marine management and conservation efforts in the Black Sea region, emphasizing the importance of adaptive strategies to mitigate the adverse effects of human activities and climate change on marine ecosystems.
1 Introduction
In today’s rapidly changing world, the marine environment has faced various threats, emphasising the imperative need for developing a systematic approach to prioritising these pressures to ensure the preservation of its ecological balance and long-term health (Halpern et al, 2008; Halpern et al, 2015; Borgwardt et al, 2019; Sundblad et al., 2021; IPCC, 2022; Smit et al., 2022). Understanding how to effectively assess and rank these mechanisms, through which human activities or natural events have altered the marine ecosystem, is paramount for its protection (Ma et al., 2023; Wu et al., 2023; Borja et al., 2016). This knowledge leads to the development of management strategies that can ultimately control the vitality and resilience of our seas (Cormier et al., 2022; Borja et al., 2020). In the past two decades, there has been a notable shift in marine management practices, emphasising an ecosystem-based approach that considers all elements and challenges affecting the ecosystem, versus solely focusing on a specific issue, activity, or species (Breen et al., 2020; Smith et al., 2016). Policies within this domain have played a significant role in this transformation, particularly the Water Framework Directive (WFD) and the Marine Strategy Framework Directive (MSFD) (EU, 2008). Both directives use the term “pressures,” and the MSFD provides detailed specifications on their nature and demands for evaluating predominant ones, including their synergistic effects, in the European marine regions (Crise et al., 2015). One of the regions is the Black Sea, located at the crossroads of Europe and Asia, serving as a critical maritime region, supporting diverse ecosystems, and playing a pivotal role in regional and global contexts (Black Sea Commission, 2007; Black Sea Commission (BSC), 2019). The pressures derive from various uses and activities, utilising different pathways, like direct discharge, atmospheric deposition, and river systems (Black Sea Commission (BSC), 2019; Slobodnik et al., 2020; Lazar et al., 2021; Strokal et al., 2023). which leads to cumulative impacts on the sea (Willsteed et al., 2017; Stelzenmüller et al., 2018a; Julius et al., 2022). These pressures include the introduction of substances, noise, litter and energy from multiple sources, overfishing, habitat destruction, climate change, and other factors that, considered together, create a more significant and often more complex impact on the sea’s ecosystems and overall health. Therefore, understanding cumulative impacts is crucial for effective marine management and conservation efforts (Ban et al., 2010; Curtin and Prellezo, 2010; Katsanevakis et al., 2020; EPA, 2022).
Cumulative impacts refer to environmental alterations arising from the combined influence of historical and current human activities and natural processes (Foley et al., 2017). According to the EU, cumulative impact is defined as “the impacts (positive or negative, direct, and indirect, long-term, and short-term) arising from a series of activities in an area or region, where each effect may not be significant if taken in isolation. Cumulative impacts include a temporal dimension, as they should calculate the impact on environmental resources resulting from changes brought about by past, present and reasonably foreseeable future actions” (Clark, 1994; Ross, 1998; EU, 1999a; EU, 1999b; Piet et al., 2021). Currently, there are several initiatives in different European sea basins based on the methodology detailed above that investigate how cumulative impact assessments can be conducted and used to support the implementation of maritime policies like Adriplan Cumulative impact tool (currently called Tools4MSP), SYMPHONY - a cumulative assessment tool in Swedish Marine Spatial Planning, SIMCelt - Maritime Spatial Planning: Transboundary Cooperation in the Celtic Seas Cumulative Effects Assessment and Marine Spatial Planning, Cumulative Impact Assessment work from ESaTDOR, Med-IAMER - Interreg Med PANACeA project, Plan4Blue on the cumulative environmental impact is based on the Pressure Index and the Baltic Sea Impact Index developed by HELCOM, the SIMNORAT project - tools for cumulative impact and the ecosystem approach for maritime spatial planning in Portugal. A literature review conducted in 2016 (Korpinen and Andersen, 2016) revealed a significant gap in the Black Sea’s cumulative pressures and impacts on the research landscape, where only one study encompassed the Mediterranean and Black Sea regions (Micheli et al., 2013). Notably, no studies specifically addressed only the Black Sea (Ban et al., 2010). Thus, the research in this area has laid the foundation for comprehensively understanding the pressures’ dynamics in the Black Sea by developing a methodology for hierarchising pressures under different development scenarios.
Black Sea challenges and progress
In the 1980s and early 1990s, the ecological state of the Black Sea was in a severely deteriorated condition (Kideys, 2002; Oguz and Velikova, 2010; Oguz, 2012; Mee et al., 2012). The decline was primarily due to eutrophication and the invasion of the comb jelly Mnemiopsis leidyi, worsened by pollution and overfishing (Gomoiu, 1992; Kideys, 2002). As a result of political willingness and economic decline (Strokal and Kroeze, 2013), the sea region witnessed encouraging outcomes stemming from the implementation of specific actions by the Danube Basin’s countries and those bordering the Black Sea after the ratification of the two conventions aimed at safeguarding both the Black Sea (The Convention on the Protection of the Black Sea Against Pollution – Bucharest Convention, 1992) and the Danube (International Convention for the Protection of the Danube River, 1994, ICPDR). Consequently, since the mid-1990s, mainly due to the reduction of the nutrient input (Lancelot et al., 2002; Friedland et al., 2021), both eutrophication and the Mnemiopsis impact have diminished (Oguz, 2005), leading to positive signs of recovery in the ecosystem. However, the recovery did not lead to the same pristine status as known in the ‘60s (Gomoiu, 1992; Langmead et al., 2009) but to a state dominated by jellies and opportunistic species as an alternative to the fish-dominated healthy state which could not be interpreted as a tendency of improvement and rehabilitation of the North-Western Black Sea shelf (McQuatters-Gollop et al., 2009; Oguz and Velikova, 2010). While the eutrophication status of the North-Western shelf, mainly influenced by significant European rivers (Lazar, 2021) such as the Danube, Dniester, and Dnieper, has shown gradual improvement over the past two decades (Slobodnik et al., 2020), the last assessment (2018) of Romania’s Black Sea waters according to the Marine Strategy Framework Directive (EC, 2017a) (MSFD) still unveiled ecological concerns. This evaluation highlighted non-indigenous species (D2), eutrophication (D5), environmental contaminants (D8), biodiversity issues concerning demersal fish (D1), and benthic habitats (D6) as areas of “non-good” environmental status. However, the “good” environmental status (GES) for contaminants in seafood (D9), marine litter (D10), and coastal fish (D1) was reported (Boicenco et al., 2018). This dichotomy suggests a need for targeted measures to address the identified concerns while maintaining efforts to sustain the positive aspects of the marine environment in the Romanian Black Sea waters’ ecological status.
The paper aims to develop a methodology for identifying predominant marine environment pressures, underscores the importance of studying these pressures, and highlights the need for effective management scenarios in the Black Sea. In order to investigate how ecosystems in the Black Sea respond to the combined effects of increasing human pressures and climate change and to identify and explore potential management options to mitigate and adapt to these impacts, we conducted a comprehensive review of activities and identified associated indicators, assessed the complex linkages between activities and pressures, built a matrix evaluating the ecosystem components sensitivity, and created a mental map and model that integrated the correlations between activities, pressures, and habitats.
2 Material and methods
The methodology outlines a comprehensive approach to identifying the predominant pressures on the marine ecosystem components and analysing different policies under specific scenarios (Figure 1). This approach is both systematic and integrative, starting with the identification of anthropogenic pressures and culminating in strategic scenario development for future planning. Each step is crafted to build upon the previous, creating a cohesive and detailed strategy for understanding and addressing the pressures on the marine environment. The first phase is to conduct an inventory (step 1) of the different activities and uses of the marine environment to lay the foundation for further analysis. The objective is to establish a baseline understanding of how the marine environment is utilized, setting the stage for subsequent analysis. This information is then used to identify potential sources of pollution, associated indicators, and available data. This characterisation (step 2) provides a detailed overview of the different activities, uses, and pollution sources from the Romanian coastal zone aiming to identify associated indicators and compile available data illustrating the direct links between human activities and environmental pressures. The next phase involves assessing the pressures against existing legislation (step 3), consisting of discharges from different point sources in the Black Sea. This assessment allows the identification of potential gaps in regulations and areas where further action is needed. The impact analysis (step 4) then evaluates the status of different marine ecosystem components against targets, limits, and specific legislation to understand the impacts of identified pressures and how they alter the marine environment. The link between pressures and impact is achieved by the habitat’s sensitivity matrix (step 5), built to identify the most critical areas and the most significant drivers of ecosystem change, allowing for prioritising pressures (step 6). Thus, utilizing the insights from the habitat sensitivity matrix and the impact analysis, step 6 is about prioritizing pressures based on their severity and impact. It ensures that management and mitigation efforts are directed where they are most needed. The final phase includes the cumulative pressures assessment (step 7) with the scope to understand the broader environmental challenges that arise from the interplay of various pressures, and scenario development (step 8), which evaluates potential future outcomes based on different intervention strategies and involves a detailed analysis of how various management strategies could influence the marine ecosystem under different future scenarios.
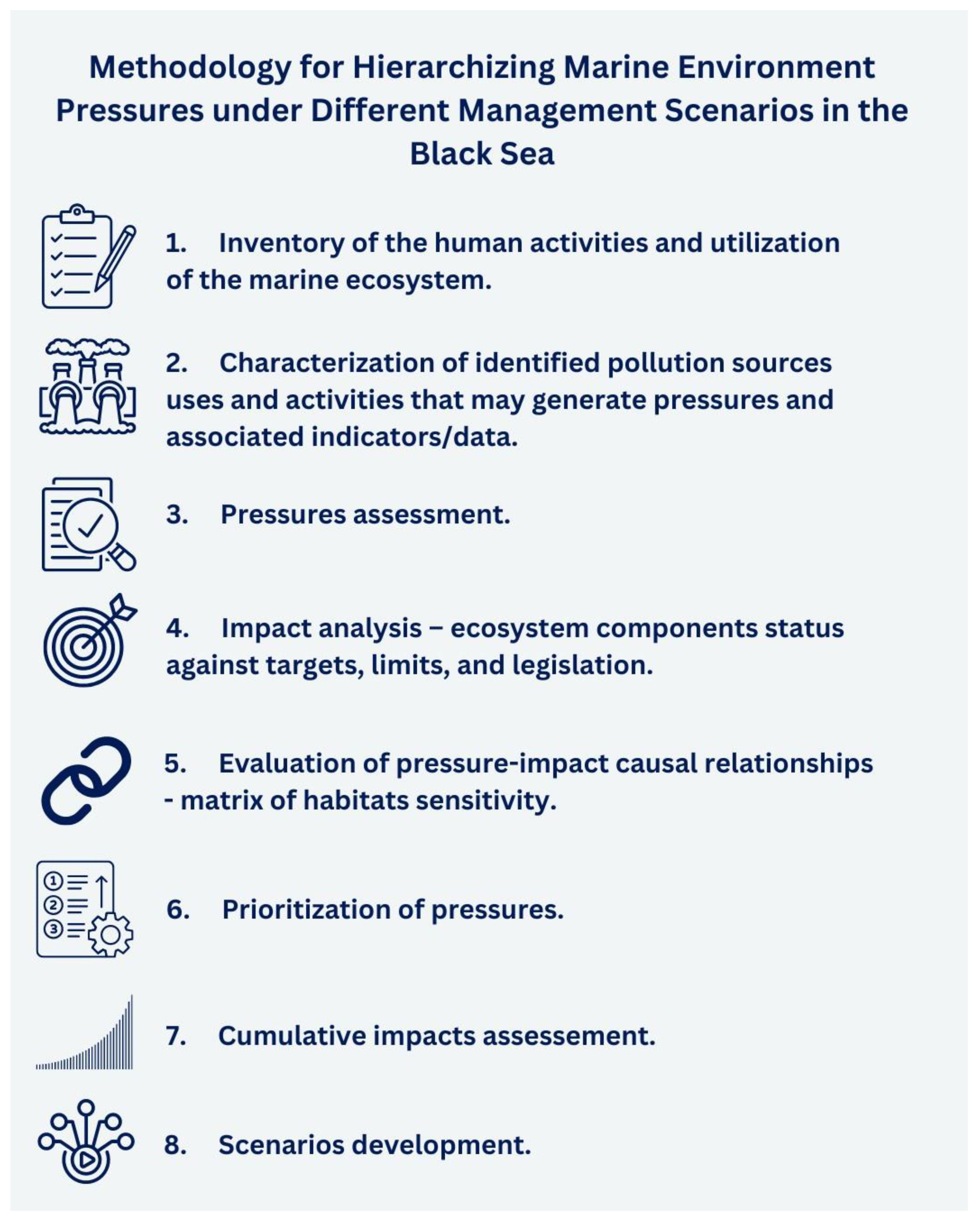
Figure 1 Steps of the methodology for hierarchising marine environment pressures under different management scenarios.
2.1 Data collection
2.1.1 The study area
The Romanian coastline along the Black Sea extends from the northern border with Ukraine to the southern border with Bulgaria, spanning a length of 244 km, representing 6% of the total Black Sea coast. The Black Sea receives a significant influx of freshwater annually from various rivers, including the Danube, Dniester, Dnieper, Don, and Bug. The Danube, Europe’s second-largest river basin after the Volga, contributes the most to the Black Sea, accounting for 55% of the freshwater input. The Danube has a total river basin area of 801,463 km2, covering approximately 33% of the Black Sea basin and traversing the territories of 19 countries ICPDR, 2005). Originating in the Black Forest Mountains of Germany, the Danube flows 2,857 km before reaching the Black Sea, with an average flow of 194 km3/year out of the total freshwater input of 350 km3/year ICPDR, 2005; Mee, 1992) (Panin and Jipa, 2002) This substantial contribution significantly influences the hydrography, chemistry, and biology of the entire north-western region of the Black Sea (Mee, 1992).
The continental shelf in the North-Western sector of the Black Sea has the largest expansion in the entire basin, with the 100 m isobath moving up to 180 km away from the shore. This expansion is due to the substantial sediment brought by the hydrographic network and the basin’s configuration. Near the Romanian shore, the continental shelf narrows from north to south, placing the 100 m isobath at 180–200 km in the northern sector and 100–110 km in the southern sector (ESPON, 2013). Romania’s maritime zones, including territorial waters, contiguous zone, and Exclusive Economic Zone, cover an area of approximately 29,600 km2. These zones overlap with the continental shelf (0–200 m depth) by about 75%. The maximum depth in the Romanian sector reaches 1500–1700 m (Monitorul Oficial, 2023).
The ongoing study incorporated diverse data sources, including information on pressures from activities occurring on land, at sea, and offshore involving the release or emission of pollutants into the atmosphere, water, or soil. While some data sets are accessible to the public (https://sdi.eea.europa.eu/), others were acquired from the National Romanian Water Authority (Table 1; Supplementary Table 1). Discharge data were used for activity characterization (step 2) and pressure assessment (step 3).
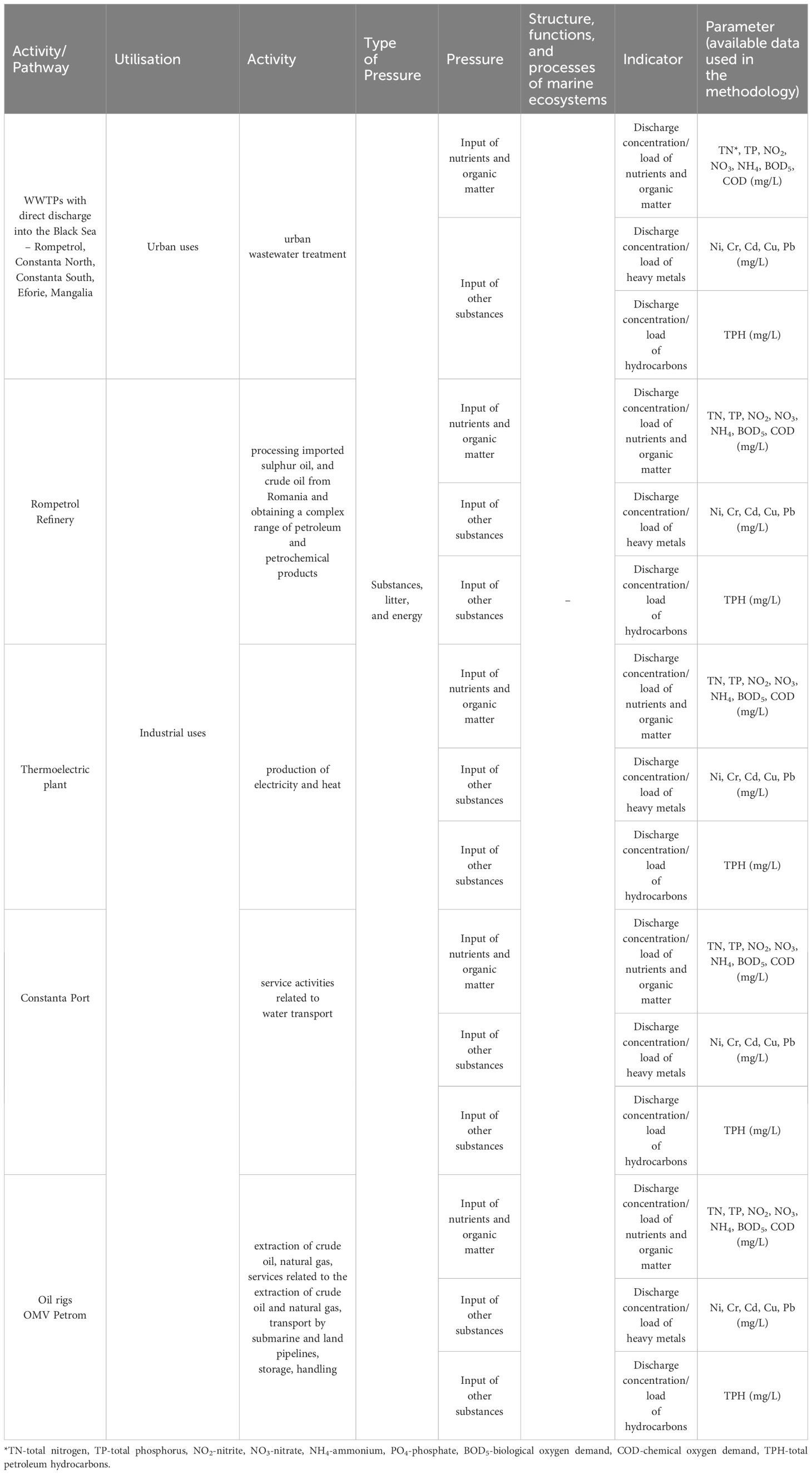
Table 1 Activities and pathways for pressures and ecosystem components, indicators and available data – Black Sea, 2017–2018.
The methodology described in Figure 1 has the following steps:
1. Inventory of the human activities and utilization of marine ecosystem - the inventory process undertaken within the Romanian Exclusive Economic Zone (EEZ) (Figure 2) consisted of identifying activities, identification of significant pressures (according to WFD) and key pressures. The process was significantly enriched by actively involving stakeholders, mainly Romanian Water Authorities. The method ensured a thorough understanding of the Romanian EEZ, laying a solid foundation for the next steps. An Excel spreadsheet that includes all existing activities (N=40) and incorporates spatial data for those with direct discharge into the Black Sea (N=8) was created. Additional information about the coastal defence and flood protection sector - beach nourishment works was collected from the National Romanian Water Authority (https://dobrogea-litoral.rowater.ro/?page_id=551). The format was designed by the project partners (National Institute for Marine Research and Development, National Water Authority, Local Water Authority and Ministry of Environment, Water and Forests throughout three virtual workshops (Zoom) held on April 6, April 29, and May 14, 2020.
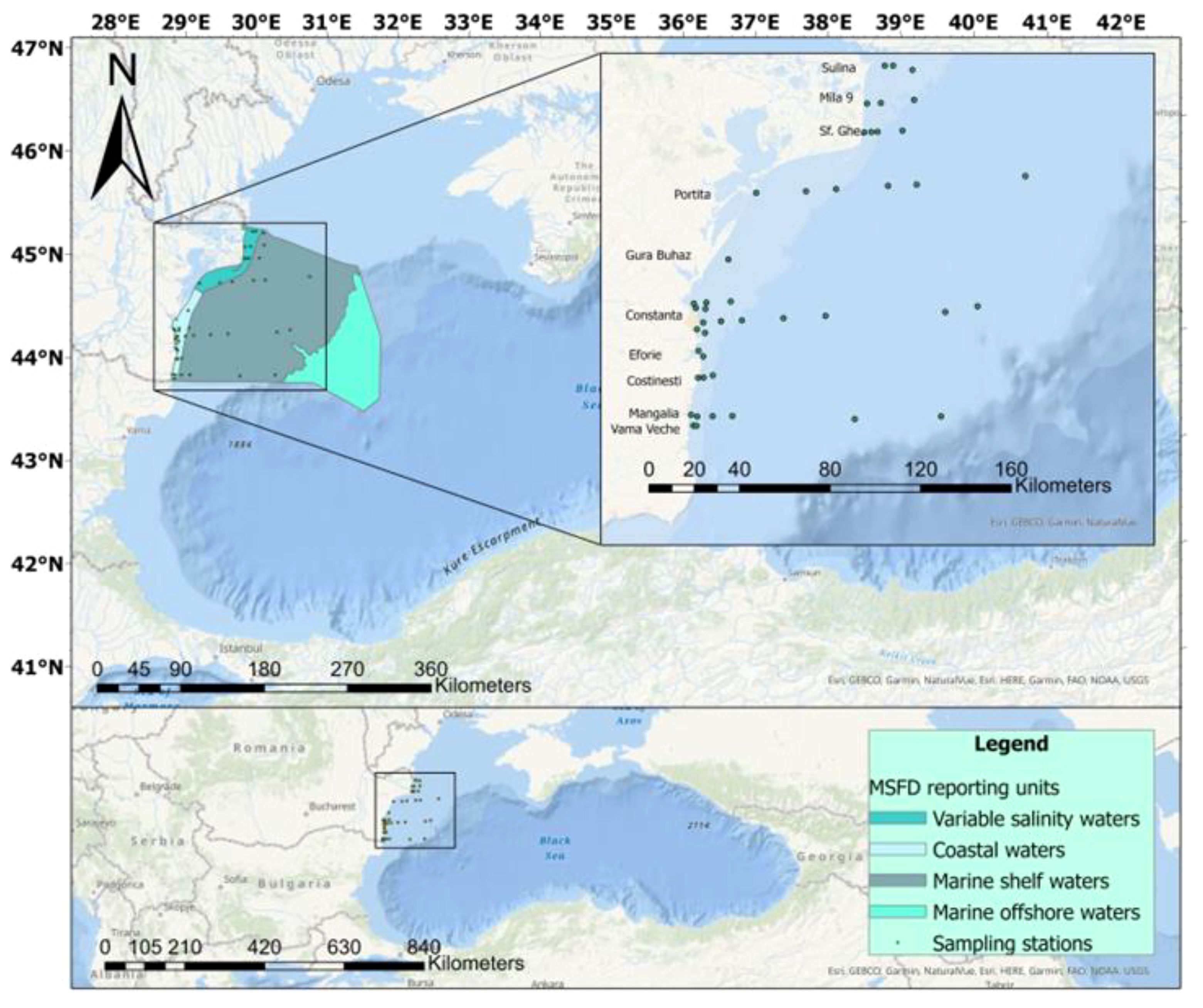
Figure 2 Map of sampling stations for evaluating the ecosystem components status – Romanian Black Sea, 2017–2019.
2. Characterization of identified pollution sources uses and activities that may generate pressures and associated indicators/data – this step was focused on analyzing the types of emissions from identified activities. This step involved quantifying emissions to understand their impact on the marine environment ensuring each activity was accompanied by detailed emissions data, where available (Table 1; Supplementary Table 1). In the inventory, the sources of pollution are classified as potentially significant pressures (by the Local Water Authority) by applying the set of criteria presented below:
A. Point sources:
a. Human settlements > 2000 p.e. that have wastewater collection systems and/or treatment plants discharging into water resources; also, settlements < 2000 p.e. are considered potentially significant sources only if they have a centralized sewage system, as well as human settlements with a combined sewage system that are unable to collect and treat the mix of wastewater and stormwater during periods of heavy rain;
b. Industry (IED installations - including units listed in E-PRTR - water emissions, units discharging hazardous substances and/or priority substances above the limits of current legislation, units not complying with existing environmental water legislation, units causing accidental pollution of water resources, other units that have established a measures program);
c. Agriculture (livestock farms under the IED Directive - including units listed in E-PRTR - water emissions, farms discharging hazardous substances and/or priority substances above the limits of current legislation, other livestock units not complying with existing environmental water legislation, units causing accidental pollution of water resources, non-compliant pesticide storage, other units that have established a measures program);
d. Other point sources that do not comply with existing environmental water legislation.
B. Diffuse sources:
a. Human settlements > 2000 p.e. that do not have wastewater collection systems and/or have non-compliant municipal waste sites, human settlements (<2000 p.e.) for water bodies that do not meet environmental objectives, etc.;
b. Industry (storage of raw materials, finished products, auxiliary products, storage of non-compliant waste, units causing diffuse accidental pollution, abandoned industrial sites, etc.);
c. Agriculture (animal rearing and cultivation of agricultural lands, especially in cases where there is non-compliance with current legislation, agro-livestock farms that do not have appropriate manure storage systems, units using non-compliant pesticides, storage of non-compliant fertilizers, etc.)
C. Hydromorphological alterations (including water intakes)
3. Pressures assessment – the assessment involved comparing each discharge within the Romanian EEZ against environmental legislation and analyzing the ecological status data from the WISE Freshwater portal. This step aimed to identify discrepancies between emissions and legal standards, finding areas where activities might pose significant environmental risks. The pressures on the marine environment were classified and assessed according to Annex III (Table 2A) of Commission Directive 2017/845 (EC, 2017b).
4. Impact analysis – ecosystem components status against targets, limits, and legislation.– this step involves a detailed analysis of various components of the marine ecosystem status, both from pelagic and benthic habitats – phytoplankton, zooplankton, phytobenthos, macrozoobenthos and abiotic conditions from seawater and marine sediments. The ecosystem components were analysed according to ANNEX III Indicative lists of ecosystem elements, anthropogenic pressures and human activities relevant to the marine waters, Table 1 Structure, functions and processes of marine ecosystems of Commission Directive 2017/845 (EC, 2017b). This step is crucial for bridging the gap between recognising environmental pressures and comprehensively evaluating their actual and potential effects on marine biodiversity, habitat integrity, and ecosystem functions.
5. Evaluation of pressure – impact casual relationship - matrix of habitats sensitivity - The evaluation of the connections between pressures and the ecosystem components relies on the ecosystem components’ sensitivity matrix, which provides a systematic framework for evaluating the sensitivity of various marine ecosystem components to different stressors or disturbances (Figure 3). The matrix takes the form of a table with rows designated for the pressures as outlined in Annex III (Table 2A) of Commission Directive 2017/845 (EC, 2017b) and columns corresponding to different habitat types and species from the studied area. Thus, each cell contains the product of ranks as described in (Halpern et al., 2007) (Supplementary Table 2) for features (surface, frequency of apparition, functional impact, resistance, and recovery) within each cell at the intersection of a specific pressure and ecosystem component (Supplementary Table 3). The values were standardised to fall within a range of 0 to 1.
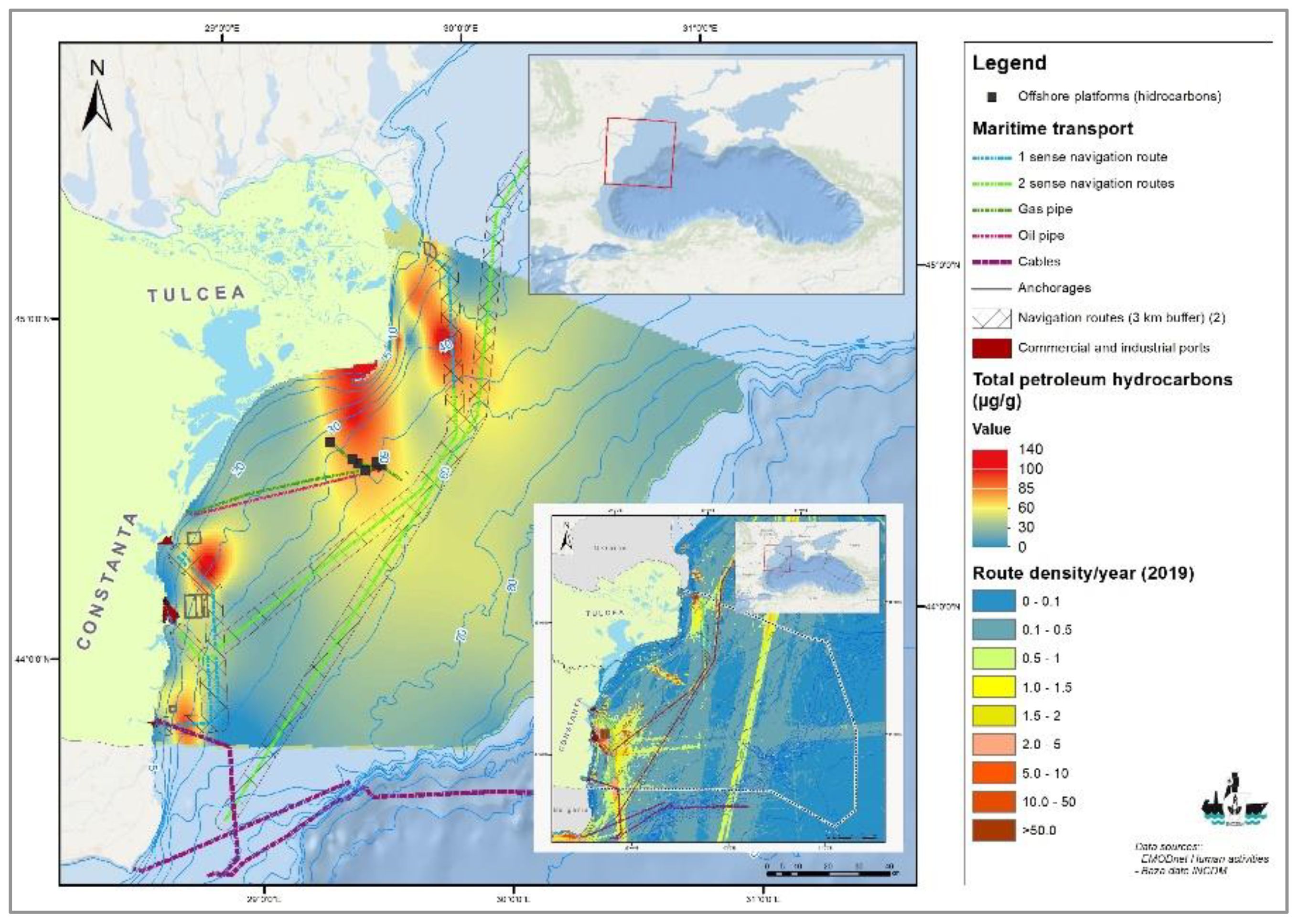
Figure 3 Distribution of total petroleum hydrocarbons (TPH) content in marine sediments (µg/g) and maritime traffic density – Romanian Black Sea.
6. Prioritisation of pressures - Semi-quantitative modelling was done with Mental Modeler software to obtain fuzzy cognitive maps (FCM) and metrics about the correlation between activities, pressures, and ecosystem components. Mental Modeler is a decision support software that helps experts understand the impact associated with environmental change and develop strategies to mitigate unwanted outcomes by capturing, communicating, and representing knowledge (Gray et al., 2014). Fuzzy-Logic Cognitive Mapping (FCM) represents a structured form of concept mapping that allows for the creation of qualitative fixed models, which are then transformed into partially quantitative dynamic models (Gray et al., 2013). By building the FCM, Mental Modeler allowed us to develop the semi-quantitative Activities – Pressures – Impact model that defines essential components and the strength of relationships between them and may run scenarios which determine how the system reacts under certain conditions. FCM was built by graphically representing knowledge by defining three characteristics of the system:
-System components – human uses and activities, anthropogenic pressures and impacts on pelagic habitats, benthic habitats, fish, birds, and marine mammals.
-Positive or negative relationships between components, according to expert judgment.
-The degree of influence that one component can have on another, defined by qualitative weights (for example, for the causal relationship activity - pressure - the source of significant, potentially significant, or insignificant pollution), sensitivity score (for the causal relationship pressure - impact, the score obtained based on the sensitivity matrix).
Thus, weight factors were set for activity-pressure links at 0.8 for “high probabilities”, 0.5 for “moderate probabilities”, and 0.2 for “low probabilities”. In contrast, pressure-impact links were weighted with values calculated in the habitats’ sensitivity matrix.
7. Cumulative impacts assessment - The cumulative impacts assessment process involves the use of a sensitivity matrix to methodically assess the impact of various pressures on marine ecosystem components along the Romanian Black Sea coast. By summing the scores from the sensitivity matrix for each habitat, a cumulative impact score is derived, offering a comprehensive measure of how these pressures collectively affect different marine habitats.
8. Scenarios development - The Shared Socioeconomic Pathways (SSPs) encompass climate change projections outlining anticipated global socioeconomic shifts until 2100 (Riahi et al., 2017). These pathways are the foundation for formulating greenhouse gas emissions scenarios under diverse climate policies (Pinnegar et al., 2021). Utilised prominently in the IPCC Sixth Assessment Report on climate change in 2021, the SSPs offer descriptive narratives depicting alternative socioeconomic trajectories (O’Neill et al., 2020; Rozenberg et al., 2014; Nilsson et al., 2017). These storylines articulate a qualitative understanding of the logical connections among various narrative elements. Employing various Integrated Assessment Models (IAMs), the SSPs can be quantified to investigate potential future trajectories encompassing socioeconomic and climate dimensions (Kok et al., 2019; Nilsson et al., 2017). Out of the initial five scenarios, we investigated three: SSP1, known as “Taking the Green Road”; SSP2, named “Middle of the Road,” and SSP5, “Taking the Highway”. We defined the narratives of each scenario according to the literature. Thus, the story of SSP1, “Taking the Green Road” for the coastal Black Sea region centers on a future in which sustainable development is prioritized. In this scenario, there is a concerted effort towards environmental preservation, renewable energy adoption, and a strong focus on mitigating the impacts of climate change (Riahi et al., 2017; Nilsson et al., 2017), and stringent environmental regulations and policies to preserve the marine ecosystem. It could involve initiatives to drastic pollution reduction and protect biodiversity, and promote eco-friendly tourism practices. Coastal communities might have extended and affordable investments in renewable energy sources, such as wind or solar power, reducing reliance on fossil fuels and minimising carbon emissions. Additionally, SSP1 strongly enhances the international collaboration among Black Sea countries to actively address common environmental challenges, fostering cooperation for marine conservation and sustainable fisheries management. The narrative would likely portray a region where economic growth is balanced with environmental conservation, ensuring a resilient and thriving coastal ecosystem for future generations. The scenario looks like the Black Sea’s riparian countries embrace the Green Deal initiatives – Fit for 55, European climate law, EU strategy on adaptation to climate change, EU biodiversity strategy for 2030, Farm to fork strategy, European industrial strategy, Circular economy action plan, Batteries and waste batteries, A just transition, Clean, affordable, and secure energy, EU chemicals strategy for sustainability and Forest strategy and deforestation (Ciot, 2022; Vela Almeida et al., 2023).
In the narrative of SSP2, “Middle of the Road” (Riahi et al., 2017; Nilsson et al., 2017), the Black Sea region follows a trajectory characterised by moderate changes and a balance between economic progress and environmental concerns. In this scenario, the approach to coastal development might involve a mix of sustainable practices and some degree of conventional methods. Efforts to address environmental concerns could exist, but they might not take centre stage compared to SSP1. There might be moderate regulations on industries and tourism activities along the Black Sea coastline, aiming for a balance between growth and conservation. Coastal communities may see some adoption of renewable energy sources, but the region might still rely significantly on traditional energy sources. This scenario could depict collaborative efforts among Black Sea countries, although they might not be as ambitious or comprehensive as those in SSP1. Overall, the narrative of SSP2 for the coastal Black Sea suggests a path where environmental considerations coexist with economic development, but without the same heightened emphasis on sustainability as in SSP1.
In the narrative of SSP5, “Fossil-fuelled Development” or “Taking the Highway” (Nilsson et al., 2017; Riahi et al., 2017), the coastal Black Sea region undergoes a trajectory marked by prioritising rapid economic growth and energy-intensive development, often at the expense of environmental concerns. This scenario might showcase extensive industrialisation along the Black Sea coastline, heavily relying on fossil fuels for energy generation and industrial activities. Immediate financial benefits may take precedence over environmental laws and conservation initiatives, which can result in more pollution and the deterioration of coastal habitats. Coastal communities may witness significant infrastructure development for industries and transportation, potentially impacting natural habitats and marine biodiversity. Renewable energy adoption might be limited, with the region largely dependent on conventional and less sustainable energy sources. Individual economic and territorial ambitions might overshadow collaboration among Black Sea countries, potentially leading to conflicts over resource utilisation and environmental degradation. The narrative of SSP5 could portray a future where short-term economic gains outweigh long-term sustainability, potentially posing challenges to the health and preservation of the coastal Black Sea environment.
In the context of addressing climate change across all three scenarios (SSP1, SSP2, and SSP5), consider the guiding pathway RCP2.6, aligning with the ambitious objective of limiting global warming to below 2.0°C until 2050, as set forth by the Paris Agreement (IPCC, 2014; UN, 2015).
The information about the marine ecosystem was collected through six expeditions (in 2017 – March, July, and November; in 2018 – July and September; and in 2019 – August) carried out with R/V “Steaua de Mare”. During these expeditions, we conducted the sampling of water, sediment, and biota from a network comprising 45 stations located on the Romanian shelf and covering three of the marine reporting units (MRU) according to the Marine Strategy Framework Directive (MSFD) (European Union, 2008) (Figure 2). The samples were analysed within NIMRD (National Institute for Marine Research and Development) laboratories, using specific methods for each parameter.
2.2 Data analysis
Data were visualised and analysed using specialised software – MS Excel, Statistica® 14.0.1.25 (TIBCO Software Inc., Palo Alto, CA, USA) TIBCO Software, Inc, 2023, PRIMER v7.0.21 (PRIMER-E Ltd, Plymouth, UK) (Clarke and Gorley, 2015), ArcGIS Desktop 10.7 software (ESRI, 2019), Mental Modeler (Gray et al., 2013).
3 Results
3.1 The results of applying the methodology to the Black Sea context
3.1.1 Inventory of the human activities and utilization of the marine ecosystem
The pollution sources inventory analysis reveals 40 activities regarding the physical restructuring of rivers, coastline, or seabed (water management), extraction of living and non-living resources, production of energy, urban (direct discharging of wastewater from treatment plants) and industrial uses (refinery, ports, shipyards), tourism and leisure. Of these, 12 are coastal villages without sewage and wastewater treatment facilities. All sources are situated along the central-southern Romanian Black Sea coast, primarily in Constanta County. This region boasts roughly 70 kilometres of coastline, with 40 kilometres for tourism (beaches). Approximately 400,000 residents inhabit the broader coastal area covered by protected zones, attracting over 1.3 million visitors annually (Nuno, 2022).
3.1.2 Characterization of identified pollution sources uses and activities that may generate pressures and associated indicators/data
The characterisation of pollution sources relies on a dual assessment: tracking sources by emissions and categorising them as insignificant or significant in terms of their pollution potential, utilising the methodology outlined by the Water Framework Directive (WFD) (EU, 2014). Thus, in this area, there were identified as potential significant sources of pollution seven big human agglomerations (>10,000 p.e.) connected to the sewage system and four wastewater treatment plants (WWTP) with direct discharge into the Black Sea, eight human agglomerations with 2,000–10,000 p.e. from which only four are connected to the sewage system and one WWTP and five industrial sources (ports and shipyards, refinery, oil and gas exploitation rigs). Four agglomerations with 2,000–10,000 p.e. and eight with less than 2,000 p.e. with agriculture as a primary activity are not connected to any sewage system or WWTP (River Basin Management Plan 2016–2011 available at https://dobrogea-litoral.rowater.ro/?page_id=469). Additionally, temporary activities include coastal protection and beach rehabilitation, natural resource exploration (oil and gas), seasonal population increase due to intensified summer tourism (14 resorts), and, more recently, the side effects of the war in Ukraine (e.g., an increase in the number of ships in the Romanian area) (OECD, 2022). This assessment does not take into consideration the Danube’s input.
3.1.3 Pressures assessment
Based on the available emissions data, the primarily monitored pressures encompass substances, litter, and energy themes. These pressures predominantly involve the introduction of nutrients and organic matter, along with releasing substances (such as heavy metals and hydrocarbons) from specific point sources. The most recent WFD assessment within the 2nd River Basin Management Plan indicated moderate to poor status in transitional and coastal waters. Specifically, according to WISE Freshwater information system for Europe, coastal waters deteriorated compared to the previous RBMP cycle).
3.1.3.1 Introduction of nutrients
The Romanian Black Sea coast witnessed a general decline in nutrient intake from wastewater treatment plants. However, 51.4% of discharged wastewater into the Black Sea remains insufficiently treated. Certain areas, particularly neighbouring the biggest WWTP and port areas, exhibit exceedances of permissible discharge concentrations. Although port zones demand a comprehensive assessment of marine environment quality due to diverse activities, only Constanta Port’s treatment plant has currently been evaluated. Neighbouring pollution land sources, Black Sea waters face risks in achieving a “good” environmental status (GES), notably in the biggest WWTP and port areas and seasonally at stations near treatment plant discharges from the southern littoral.
3.1.3.2 Introduction of organic matter
The levels of organic matter (measured as biological oxygen demand – BOD5 and chemical oxygen demand – COD) in the released wastewater surpass the permitted limits outlined in the national legislation near Mangalia WWTP, Constanta Port, and the OMV Petrom platform, suggesting environmental compliance issues in these areas. The amount of undertreated and insufficiently treated water discharged into the Black Sea contributes to the increased load of organic matter and accumulates with the already recognised risk of failing to achieve GES for Descriptor 5 — Eutrophication.
3.1.3.3 Introduction of other substances – heavy metals and TPHs (Total Petroleum Hydrocarbons)
The annual average concentrations of heavy metals (Cu, Cd, Pb, Ni, Cr) in discharged waters notably rise around the WWTPs near Eforie Sud and Mangalia. Surprisingly, even though larger treatment plants like Constanta Sud and Constanta Nord contribute the most to annual flow, they do not show similarly elevated metal levels. The national legislation was not otherwise breached except for mercury, which exceeded the maximum permissible values in 2017 in effluents from Constanta Sud, Eforie, and Rompetrol treatment plants.
The hydrocarbon values found in wastewater discharged into the marine environment between 2017 and 2018 ranged from 0.053 mg/L to 0.049 mg/L, all well below the maximum limit of 5 mg/L set by the prevailing legislation.
3.1.4 Impact analysis – habitats status against targets, limits, and legislation
3.1.4.1 Pelagic habitat
3.1.4.1.1 Phytoplankton
Blooms (abundances surpassing one million cells/L) of five microalgae species were detected on the continental shelf. Notably, in May 2019, Skeletonema subsalsum, Pseudo-nitzschia delicatissima, and Chaetoceros curvisetus peaked at 2.3, 1.8, and 1.1 million cells/L, near the Danube’s mouths. Planktolyngbya circumcreta, a cyanobacterium, achieved its maximum density (1.09 million cells/L) in July 2018 in the same area. The coccolithophore Emiliania huxleyi reached 1.06 million cells/L in marine waters in July 2017. However, biomass-wise, the waters from the Danube’s influence area maintained GES from 2017 to 2019.
Conversely, coastal and marine waters exceeded target values, classifying them as being in non-GES. Thus, the findings underscore the impact of anthropogenic activities on the coastal zone. The recorded proliferation of phytoplankton, notably exceeding target values in coastal and marine waters, points towards ecological stress induced by human-related factors. The increase in densities and deviations from environmental targets in these areas indicate a potential disruption caused by anthropogenic influences. As such, there is a pressing need for comprehensive management strategies to mitigate the adverse effects of human activities on coastal ecosystems and safeguard their ecological integrity.
3.1.4.1.2 Zooplankton
Zooplankton exhibited a prevalent GES, particularly for mesozooplankton biomass, with high percentages in coastal and marine waters. However, the variable salinity waters, being influenced by the riverine input, faced challenges, falling short of achieving GES. In the cold season, we observed all three Marine Reporting Units (MRUs) reaching 100% GES regarding copepod biomass. As an eutrophication indicator, Noctiluca scintillans biomass, while indicating GES in all areas during the warm season, experienced an increase in the cold season, when only the marine waters maintaining a GES. These observations highlight different MRUs’ seasonal dynamics and potential climate change implications on varying environmental statuses, emphasising the need for targeted management strategies to address specific challenges in each context.
3.1.4.2 Abiotic conditions in seawater
The Romanian waters of the Black Sea did not reach GES in the proportion of 51% (stations out of the total number), representing approximately 45% of the monitored area and approximately 27% of the Romanian waters of the Black Sea (exclusive economic zone) due to the nutrient concentrations. Transitional and marine waters in the north are the most eutrophicated. In coastal waters, GES is not reached in the larger WWTP and Constanta port areas. On the southernmost profiles, Mangalia and Vama Veche, as well as stations on the bathymetric strip 70–100m (Portita, Est Constanta and Mangalia), have predominantly a “good” and “very good” status of nutrients, a condition confirmed by biological elements status (benthic communities from that area).
The influence of discharges from the six coastal sources on the Black Sea waters, focusing on the metals Cu, Cd, Pb, Ni, and Cr, exhibits a range from potentially significant to insignificant. During the investigation of water samples from oil and gas platforms, Ni and Cr in bottom waters revealed slightly elevated levels compared to the background values for marine waters. However, they remained within the permissible limits for marine water quality. In the marine waters, the concentrations of total petroleum hydrocarbons in water samples did not exceed the maximum allowable limit of 200 μg/L as outlined by national legislation, both within the coastal zone and the continental shelf area. Therefore, the overall impact of the investigated metals and discharges on the Black Sea waters demonstrates variability, with some elements approaching significant levels but generally remaining within acceptable thresholds. Additionally, the concentrations of total petroleum hydrocarbons in the marine environment, according to regulatory standards, indicate a relatively controlled impact or too permissive standards.
3.1.4.3 Benthic habitat
3.1.4.3.1 Phytobenthos
The coastal waters had the highest quantitative proportion of opportunistic species (51.5 – 75.5%). Constanta industrialised area is mainly dominated by opportunistic species, primarily from the genera Ulva and Cladophora, which experience substantial biomass development, especially during the summer due to increased nutrient levels. Notably, in none of the water bodies did the proportion of opportunistic species biomass fall below the threshold value of 40%. Consequently, none of the three water bodies achieved GES between 2017 and 2019. Applying the “proportion of opportunistic species biomass to total biomass” indicator reveals that only 45% of the monitored coastal stations achieved a good ecological status or potential. Thus, the consistently high proportion of opportunistic species and the failure of water bodies to achieve GES highlight the substantial ecological challenges in the coastal areas, particularly in the Constanta industrialised region. This emphasises the need for targeted and proactive measures to address and mitigate the impact of opportunistic species and promote a healthier ecological balance in the coastal ecosystem. The current accepted scientific name for Cystoseira barbata has been updated to Gongolaria barbata. However, due to the widespread recognition of the species under its former name, the term C. barbata will be utilized throughout this document.
3.1.4.3.2 Macrozoobenthos
The M-AMBI (n) values consistently demonstrated GES for transitional waters, from the northern area, with all levels surpassing the threshold (0.61). Evaluation of the ecological status of coastal water bodies revealed a robust result, with 82% of monitoring stations indicating a good status. On the Romanian continental shelf, the ecological status of benthic macrofauna inhabiting circalittoral soft-bottom habitats revealed that 23% of stations exhibited a poor status (M-AMBI*(n) < 0.68). The M-AMBI values consistently describe good ecological conditions in transitional and coastal water bodies, indicating effective ecosystem health. While there are compartments of poor status of communities from circalittoral soft-bottom habitats, most stations on the Romanian continental shelf exhibit good ecological conditions. This suggests a general environmental state but highlights the importance of addressing specific areas to achieve comprehensive ecosystem health.
3.1.4.4 Abiotic condition in sediments
Increased accumulations of Cd and Ni were noted in marine sediments in the vicinity of industrialised areas, such as refineries and ports. In the biggest WWTP and port Constanta areas, the cumulative impact of port discharges and activities is reflected in increased concentrations of heavy metals, especially Cu, Ni and Cd. In the discharge area of the Eforie WWTP, no increased accumulations of heavy metals were recorded, except for Ni. Thus, the impact of discharges of the investigated metals (Cu, Cd, Pb, Ni, Cr), from the six pollution sources on marine sediments can be characterised as ranging between potentially significant and insignificant. In sediment samples from the oil and gas platforms area, heavy metal concentrations fell within the ranges of variation observed at the Romanian seaside. Still, the average Cu, Cd and Ni values for 2017–2018 slightly exceeded the recommended values for marine sediment quality.
Although no exceedances of total petroleum hydrocarbon levels were reported in discharged and marine waters, the maximum allowable limit (100 μg/g) in sediments was exceeded both in the coastal area, in the Constanta South area, but especially in the continental shelf area where hydrocarbon exploitation activities are carried out for a long time (Figure 3).
3.1.5 Evaluation of pressure-impact causal relationships
The matrix of the ecosystem components’ sensitivity is a valuable tool for conservation, policy development, and scientific research (Figure 4). It aids in making informed decisions about the management and protection of species and habitats, ensuring the sustainability of ecosystems in the face of various environmental challenges (Goodsir et al., 2015; Korpinen et al., 2019; Quemmerais-Amice et al., 2020). Thus, by categorising habitats based on their sensitivity, the matrix helps prioritise management efforts. Consequently, the ecosystem components identified as highly sensitive may require more immediate and targeted conservation measures to prevent degradation or loss. In the development of the Matrix of the ecosystem components’ sensitivity, was adopted the classifications for activities and pressures by referencing previous categorizations established in the Marine Strategy Framework Directive and by the designation of seabed conservation features, as mandated by the Habitats Directive 92/43/EEC. Scores are computed by integrating data from surveys and expert judgment, as highlighted in the degree of certainty feature (Supplementary Tables 2, 3) focusing on criteria such as the extent (surface), the functional impact, the habitat’s ability to regenerate, its tolerance to alterations in environmental conditions, and the reversibility of impact. High scores indicate habitats that are highly sensitive and potentially less capable of recovering from disturbance, signifying a need for prioritized conservation efforts. Conversely, lower scores are assigned to more resilient habitats, indicating a lesser immediate need for intervention. This scoring system enables a ranked prioritization of habitats based on their sensitivity, guiding more focused and effective management and conservation strategies.
3.1.6 Prioritisation of pressures
Obtaining the semi-quantitative model and assessing as objectively as possible the causal relationships in which pressures on the marine ecosystem are involved allowed the establishment of the hierarchy of pressures based on the centrality score calculated by Mental Modeler software, considering all causal relationships (activities-pressures and pressures-impacts) and their intensity from the sensitivity matrix (Figure 5). The hierarchy of pressures was achieved by the descending order of “centrality”, which represents the absolute value of the influence of pressure in the model. For calculating centrality, Mental Modeler measures the importance or influence of a node within a network by analysing its connections, pathways, and relationships with other nodes, aiding in understanding their impact or prominence in the overall system (Figure 6). Thus, the higher this value, the greater the importance of pressure in the model. The model has 29 components – 7 drivers, 13 receivers, 9 ordinary connections, and 64 total connections with a density of 0.08, representing 2.21 connections/components (Supplementary Figure 1).
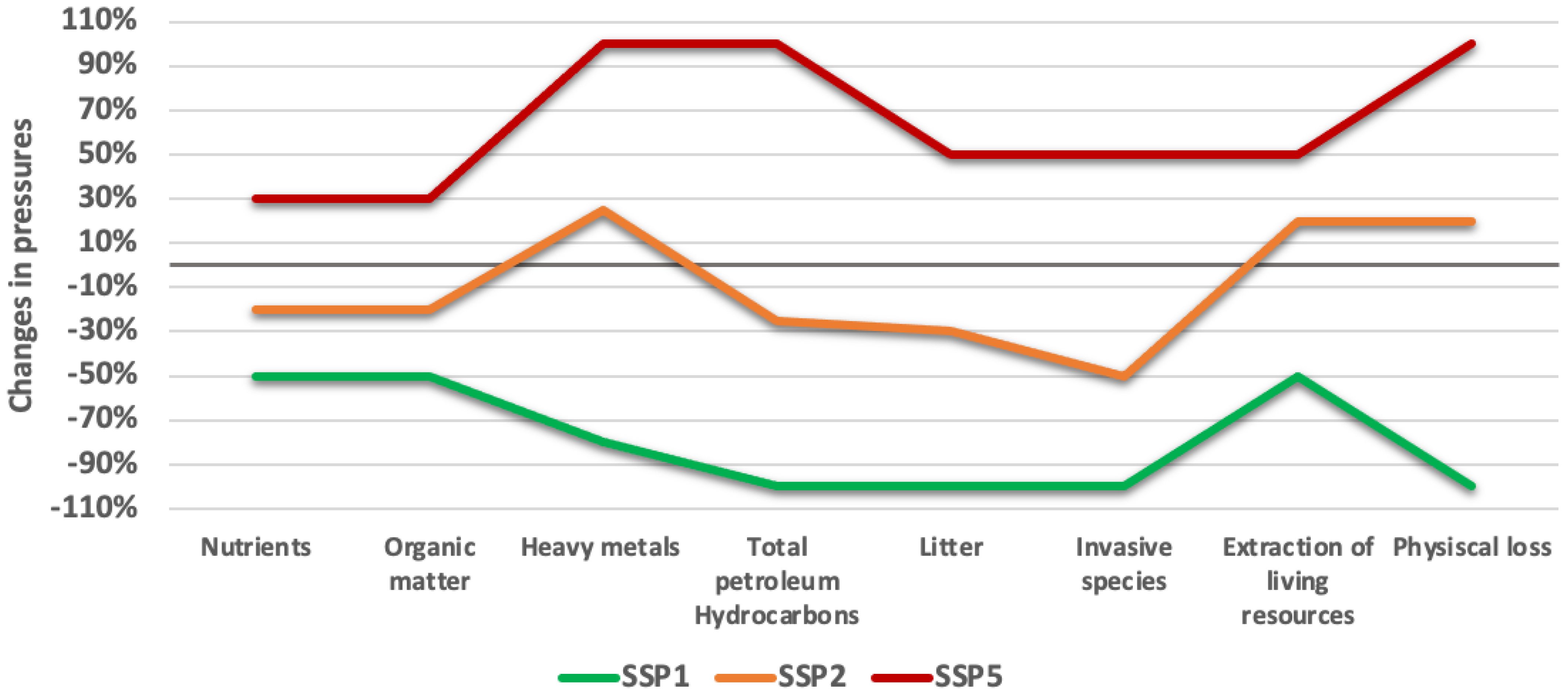
Figure 5 Changes in components according to each scenario narrative (negative values stand for decreasing and positive for pressure increasing).
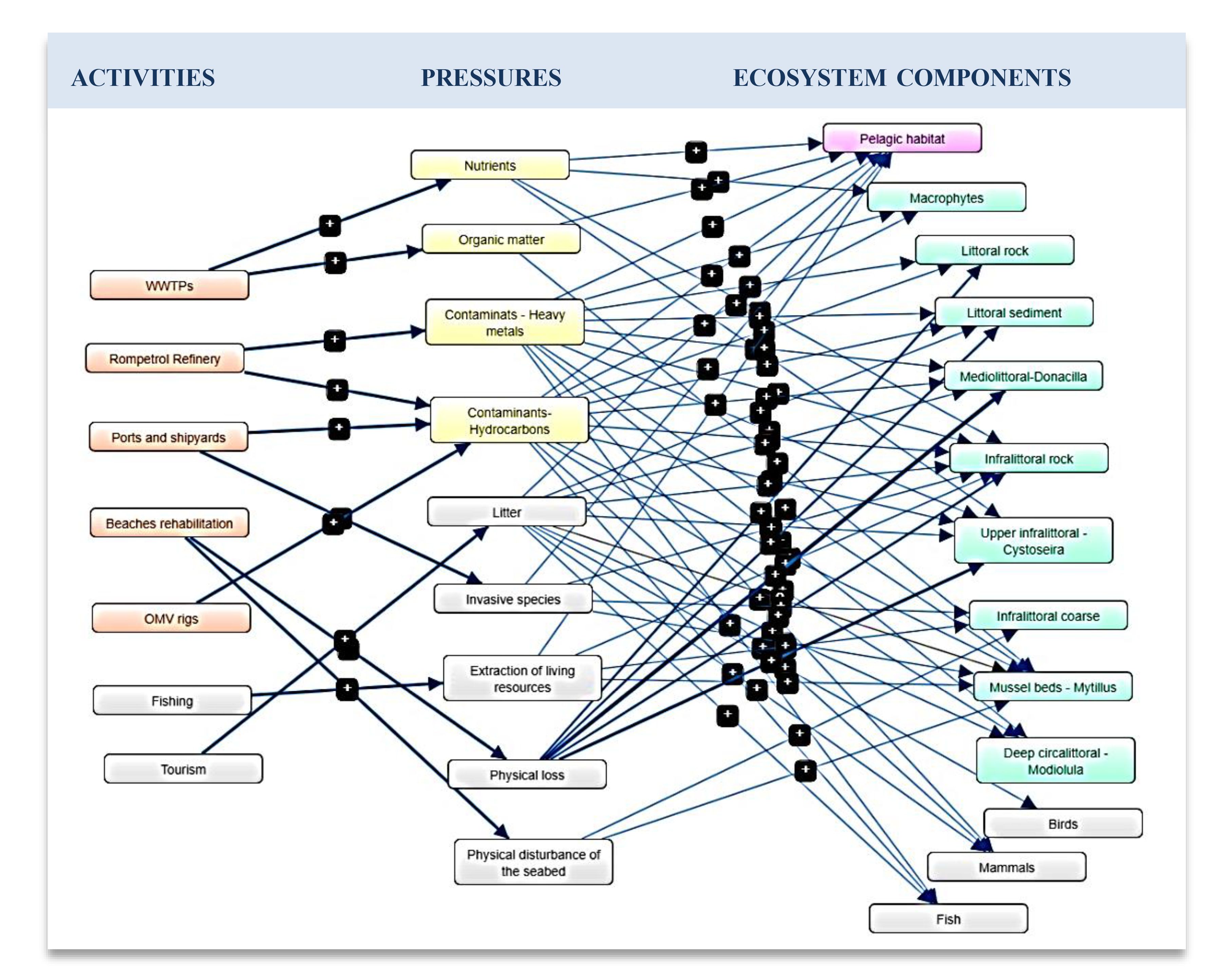
Figure 6 Fuzzy Cognitive Map (FCM) of activities (orange)-pressures (yellow) -impacts on the ecosystem components (magenta – pelagic habitat consisting of phytoplankton and zooplankton; green – benthic habitats; grey – species, assessed only by expert judgement-.
3.1.7 Cumulative impacts
The pelagic ecosystem is influenced by multiple pressures, with the introduction of invasive planktonic species constituting a 40.7% influence. Nutrient loading and the addition of other substances each have a 20.5% impact. The accumulation of litter and organic debris further exacerbates contamination levels, indicating a significant threat of biological invasions. Macrophytes face substantial threats from the addition of substances (80%) and nutrients (20%). Coastal environments, including the littoral rock (0–1m), littoral sediment (0–1m), mediolittoral coarse or medium clean sand (0–1m), and upper infralittoral rock (3–10m) habitats, experience pronounced effects (99%, 96%, 97%, and 92% respectively) from physical loss due to beach nourishment activities. The infralittoral rock habitat accumulates impacts from various stressors, predominantly from physical loss (67.2%), followed by the introduction of non-native species (14.9%) and impacts from bottom trawling fisheries (14.9%). These fisheries also affect the infralittoral coarse, mixed, sand, and mud sediment (1–20m) habitats, where they contribute to a 27.5% impact, alongside physical seabed disturbances (4.9%) and a significant threat of physical loss (67.6%). In the Mytilus galloprovincialis beds located in circalittoral mud and mixed sediments (30–60m), a combination of pressures significantly impacts the environment. Here, substance input is the predominant issue (35.6%), followed by the introduction of the non-indigenous Rapana whelk (23.7%), and equal inputs of litter and organic matter (11.9% each). Although the intensity of bottom trawling decreases offshore, its impact, including the extraction of wild species (8.5%) and physical seabed disturbances (8.5%), remains notable. The deep circalittoral shelly mud habitat, inhabited by Modiolula phaseolina (60–120m), primarily suffers from substance input (66.7%) and litter (33.3%). Bird populations are predominantly disturbed (93.3%) by various anthropogenic activities, which disrupt natural behaviours, coupled with a 6.67% impact from litter. Mammals are notably affected by accidental captures in fisheries (79.8%), along with substance input (6.7%) and litter (13.5%), highlighting the consequences of human exploitation and incidental harm. Similarly, fish populations are significantly impacted by direct extractions or mortality/injury (92.3%), with additional pressures from litter (3.3%) and substance input (3.3%), indicating the extent of anthropogenic stressors on these species (Figure 7).
3.1.8 Scenarios development
Knowing the sources, pressures and impacts on prevalent ecosystem components and their cumulative effect facilitates the development of scenarios, allowing researchers and policymakers to assess potential future outcomes based on different intervention strategies according to each narrative (OECD, 2016). This foresight is crucial for informed decision-making and long-term planning. In response to these scenarios, we have identified specific variables for each respective Key Performance Indicator (KPIs), which serve as guiding metrics to assess and track environmental impact and sustainability within each scenario (Table 2). The percentage values associated with each scenario typically reflect the likelihood or desirability of that future pathway based on narratives, expert judgment and Romania’s profile (https://ssp-extensions.apps.ece.iiasa.ac.at/profile/Romania/development). Thus, KPIs in SSP1 are strongly reduced based on concerted efforts toward environmental preservation and climate change mitigation, alongside stringent policies, drive significant pollution reduction and biodiversity protection in the marine ecosystem. KPIs in SSP2 reflect a scenario where environmental considerations and economic development proceed together, though without the intense focus on sustainability found in SSP1. Finally, for SSP5, KPIs are aligned with prioritizing short-term economic gains over long-term sustainability, which could challenge the health and conservation of the coastal Black Sea environment.
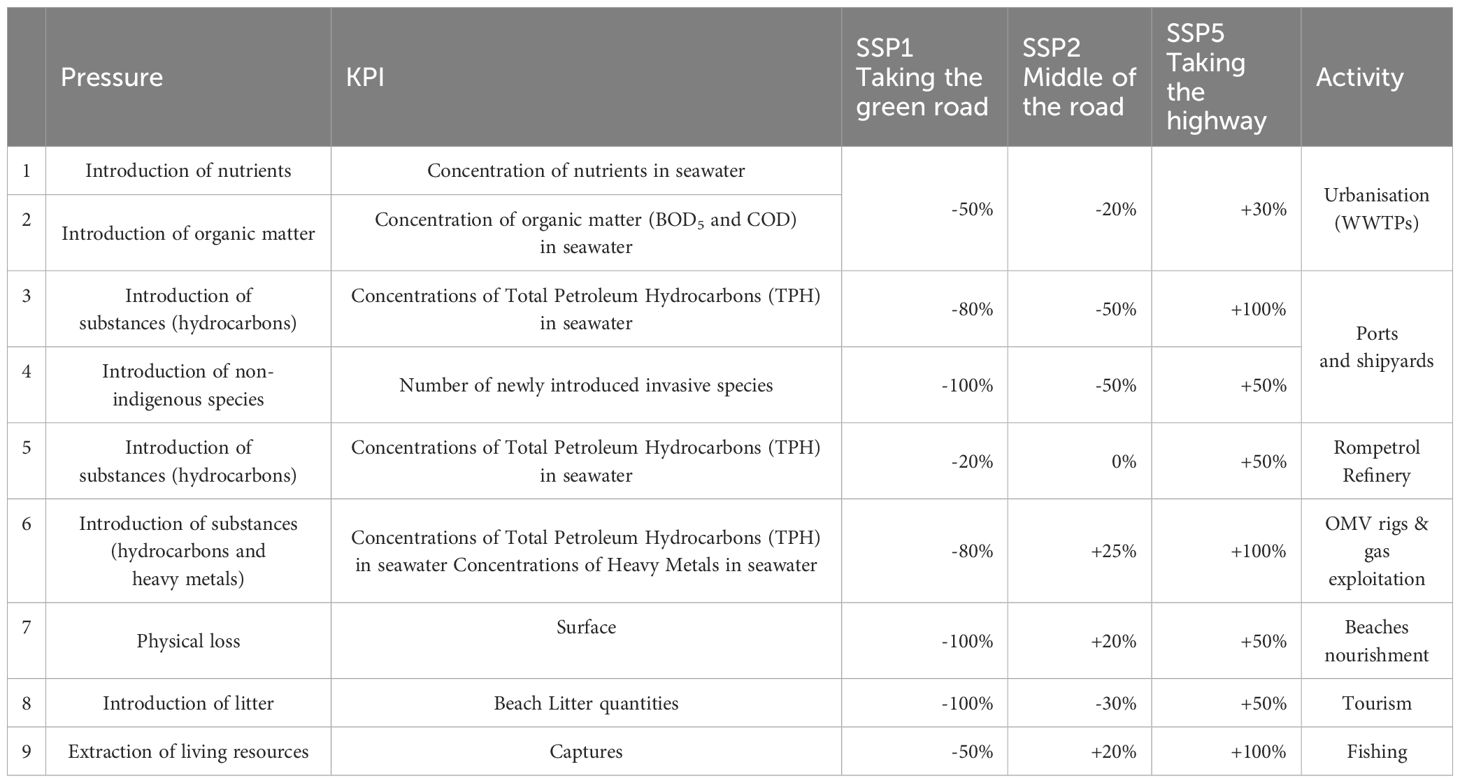
Table 2 Pressure variability (associated activities) according to SSPs and estimated associated Key Performance indicators (KPI).
Changes were made individually to each pressure (Table 2) (Figure 5), and the Mental Modeler produced corresponding values (Figure 8) based on the initial model. The Mental Modeler scenario interface (Supplementary Figures 2, 4) shows the relative change in the components included in the model, influenced by the defined relationships in the FCM for each selected scenario. Running scenarios involve changing variables from −1 (significant negative change) to +1 (significant positive change). As a result, a bar graph visually represented the relative system change, displaying how components could respond within the specified scenario (Supplementary Figures 2, 4) (Gray et al., 2013).
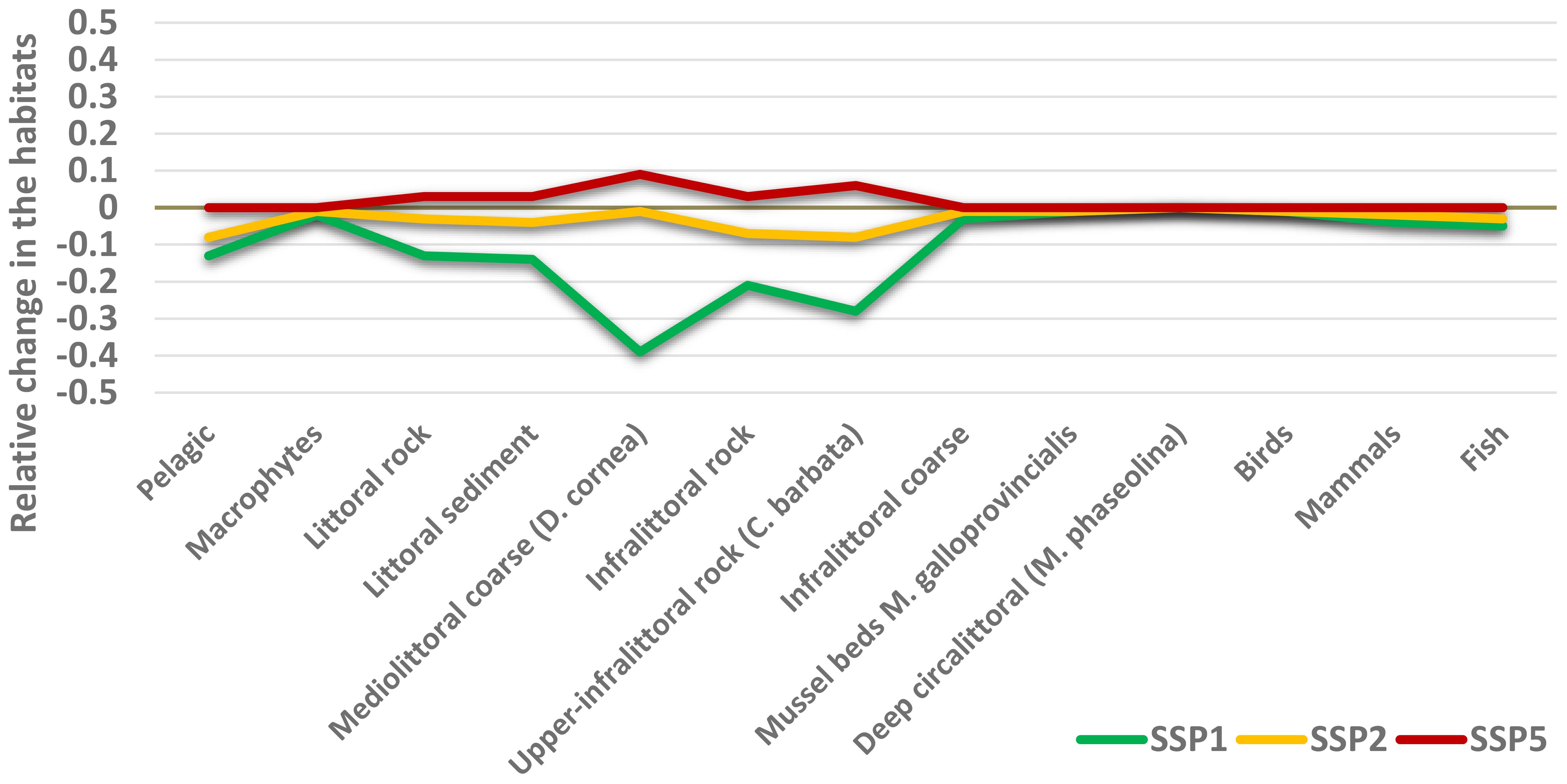
Figure 8 Relative changes in the ecosystem components in three scenarios (negative values stand for improvement and positive for degradation) (SSP1 – Taking the green road, SSP2-Middle of the road, SSP5-Taking the highway).
4 Discussion
The application of the methodology revealed that the primary pressure is physical loss, with subsequent pressures including the introduction of hydrocarbons and non-indigenous species (Figure 9). The activities associated with these pressures include beach nourishment, maritime transport, port infrastructure, and oil exploitation in the Romanian Black Sea. It is noteworthy to observe that traditional pressures in the region, such as nutrient introduction and fishing (Oguz and Velikova, 2010; Oguz, 2017), have been surpassed by recent beach nourishment projects, maritime transport and infrastructure and oil and gas exploitations. The need for prompt mitigation measures is urgent, as these activities can severely disrupt the marine ecosystem. Additionally, the ongoing conflict in Ukraine, which has increased maritime traffic and opened new corridors for grain exports, together with climate change-induced alterations such as changes in river hydrology, elevated surface temperatures, and altered wave, wind, and current patterns, could further destabilize the ecosystem.
Significant habitat loss has been observed in areas with mediolittoral coarse or medium clean sand, particularly impacting species like Donacilla cornea, which only recently reappeared (Micu and Micu, 2006), after nearing extinction between the 1970s and 1980s. Despite attempts at species relocation in 2022 (AON S.R.L, 2022), adverse weather conditions led to poor outcomes. The upper-infralittoral rock habitats dominated by Cystoseira barbata have also been heavily impacted by beach rehabilitation works. The habitat of Cystoseira barbata is linked to zoobenthos communities characterised by a well-organised structure, diversity, and productivity (Filimon et al., 2016). The loss of these habitats risks the complex balance of marine life and could result in irreversible ecological damages, as these species play critical roles in maintaining ecosystem stability (Duffy, 2006).
The introduction of hydrocarbons from port activities and oil and gas exploitation poses significant threats to pelagic habitats, potentially disrupting ecological balances and affecting species distribution and abundance (Perhar and Arhonditsis, 2014). Therefore, it is essential to enforce strict regulations on hydrocarbon discharges to maintain environmental standards and apply penalties for non-compliance.
Marine ecosystems, once significantly impacted, are often difficult to restore even after reducing pressures due to their complex dynamics and variable resilience. Hence, pressure assessments need to be dynamic and straightforward to aid decision-makers (Sinclair et al., 2017). Employing different management scenarios against a baseline provides a practical approach for ecosystem-based management.
Management decisions must aim for substantial reductions in pollutants and stressors, aligning with the “Taking the Green Road” narrative. Achieving a 50% reduction in nutrients and organic matter requires strong political will, stringent legislation, and strict enforcement of regulations against agricultural runoff and industrial discharge. This involves mitigating nutrient pollution, encouraging sustainable farming, investing in advanced wastewater treatment, and monitoring industrial processes to prevent heavy metal discharge. Moreover, a 25% reduction in hydrocarbons and a 50% reduction in heavy metals necessitate comprehensive strategies including strict industrial emissions controls and proper waste disposal. Such measures are crucial to protecting the marine environment, requiring a concerted effort to monitor and regulate industrial activities. While stringent environmental policies can enhance certain habitats and improve ecosystem services, they may also pose challenges to industries and communities reliant on these areas, potentially affecting economic activities and coastal erosion management. On the other hand, the level of awareness and education is remarkably high, and people follow the rules without too much constraint. In this case (SSP1), due to the policy implementation, the most significant decrease in pressure is experienced in the habitat of Mediolittoral coarse or medium clean sand with Donacilla cornea (and Ophelia bicornis) (0–1m), mainly because the decision to forego beach nourishment and other activities resulting in no more physical loss. Similarly, implementing a strict environmental protection policy could bring improvement in the Upper-infralittoral rock dominated by Cystoseira barbata (3–10m) and the Infralittoral rock (1–18m) habitats, leading to ecosystem services improvement by enhanced biodiversity and ecosystem health, carbon sequestration, nutrient cycling, or bioremediation (Farghali et al., 2023) of the coastal waters. However, there might also be negative consequences or challenges associated with these actions. Halting beach nourishment efforts might affect coastal erosion control or impact certain human activities that depend on wider beaches. Additionally, while the strict environmental policy can provide significant advantages for particular habitats, it might pose challenges for industries or communities reliant on these areas for economic activities. Overall, while there are positive aspects to these decisions regarding habitat preservation and environmental protection, there might also be trade-offs or challenges that need to be addressed for a comprehensive understanding of their impacts.
In a moderate scenario (SSP2), management strategies will balance economic growth with environmental protection, imposing moderate restrictions on pollutants and development. This approach aims to reduce nutrient levels, control non-indigenous species, and decrease marine litter, though less effectively than more stringent policies. Encouraging a shift towards renewable energy while maintaining some reliance on traditional sources is also part of this strategy. Moderate increases in habitat disruption from beach rehabilitation and fishing highlight the need for habitat restoration and sustainable land-use to mitigate adverse impacts and promote long-term ecological resilience. Notably, there is no observed reduction in total petroleum hydrocarbons in seawater due to industrial activities, and the contribution from oil and gas development projects is on the rise. Moderate increases have been noted in physical loss from beach rehabilitation efforts and fishing activities (Table 2). In this instance, there is still a positive trend in the condition of the pelagic habitat, along with enhancements observed in habitats like the Infralittoral rock and Upper-infralittoral rock dominated by Cystoseira barbata. The pelagic environment also undergoes improvement due to the reduction in nutrient input, although to a lesser extent than in SSP1. The already significant physical loss of habitats should be addressed through various measures, including habitat restoration initiatives, conservation efforts, and sustainable land-use planning. These measures should aim to counteract the adverse impacts of habitat loss by restoring degraded areas, establishing protected zones, and implementing practices that promote ecosystem resilience. Additionally, community involvement, education, and the promotion of responsible resource management plays crucial roles in mitigating habitat loss and fostering long-term ecological sustainability.
Conversely, a rapid growth-focused scenario (SSP5) prioritizes economic expansion over stringent environmental regulations, allowing increased pollution and reliance on fossil fuels. This approach leads to extensive infrastructure development along the coastline, potentially harming natural habitats and biodiversity. A lack of coordinated action may worsen environmental challenges across borders, emphasizing the need for collaborative strategies to address transboundary issues.
The main limitations of the methodology are linked to lack of data and exclusion of socioeconomic data. Addressing these limitations involves a combination of improving data quality, integrating socioeconomic considerations, acknowledging and managing uncertainties, and adopting a more dynamic approach to accommodate temporal variability. These enhancements will contribute to a more robust and applicable methodology for assessing and hierarchising pressures on marine ecosystems for policy-makers decisions.
5 Conclusion
This methodology offers a structured framework to assess and prioritize the impacts of various pressures on the marine environment, aiding decision-makers in developing targeted strategies. It highlights the complex nature of environmental pressures in the Romanian Black Sea, primarily driven by human activities such as beach nourishment, maritime transport, and oil and gas exploitation. The re-emergence and subsequent threat to species like Donacilla cornea illustrate the delicate balance of marine ecosystems and the significant impact of human activities.
The adaptability of this approach under various scenarios enhances its utility in resource allocation and promoting sustainable marine practices. It evaluates potential impacts across different development trajectories, aiding in informed policy-making and sustainable development. Scenarios ranging from strong environmental focus (SSP1) to economic prioritization (SSP5) provide insights into potential future ecosystem management outcomes.
Improving the methodology for hierarchising marine environment pressures involves systematically refining and enhancing its effectiveness by considering diverse perspectives to ensure a comprehensive understanding of the marine environment and its related pressures through stakeholder engagement. The results of the methodology should be included in the dynamic modelling capabilities that account for temporal and spatial variations of marine pressures. Scenario-based modelling integrating socioeconomic issues to capture the complex interactions influencing marine pressures should be implemented to predict potential future impacts under different conditions
Future enhancements should include refining the methodology by incorporating diverse stakeholder perspectives and improving dynamic modelling to account for temporal and spatial variations. This could involve advanced modelling techniques like Ecopat with Ecosim (EwE) to simulate ecological dynamics more accurately, offering a comprehensive view of marine ecosystem pressures and facilitating effective management and cooperation among Black Sea countries.
Data availability statement
The data analyzed in this study is subject to the following licenses/restrictions: Data from sources were directly supplied by the Romanian Water Authority as a partner of the project. The data from marine cruises are restricted under different projects policies. Requests to access these datasets should be directed to https://www.nodc.ro/.
Author contributions
LL: Conceptualization, Methodology, Software, Visualization, Writing – original draft, Writing – review & editing. AS: Resources, Writing – review & editing, Writing – original draft. LB: Resources, Writing – original draft, Writing – review & editing. AO: Resources, Writing – review & editing, Writing – original draft. ND: Resources, Writing – original draft, Writing – review & editing. EB: Resources, Writing – review & editing, Writing – original draft. VA: Resources, Writing – original draft, Writing – review & editing. AF: Resources, Writing – review & editing, Writing – original draft. GH: Resources, Writing – original draft, Writing – review & editing. OM: Resources, Writing – review & editing, Writing – original draft. EP: Resources, Writing – original draft, Writing – review & editing. FT: Resources, Writing – original draft, Writing – review & editing. OV: Resources, Writing – review & editing, Writing – original draft. SK: Supervision, Writing – review & editing, Writing – original draft.
Funding
The author(s) declare financial support was received for the research, authorship, and/or publication of this article. This research was supported by the Administrative Capacity Operational Program 2014-2020, priority axis IP12/2018 under project code MySMIS 127598/SIPOCA 608 “Improving the capacity of the central public authority in the field of marine environment protection in terms of monitoring, evaluation, planning, implementation and reporting of requirements set out in the Framework Directive Marine Strategy and for integrated coastal zone management”. The writing time of the article was supported by Nucleu Programme 2023-2026 - SMART-BLUE, PN23230101, grant agreement 33N/2023 and the European Union’s Horizon 2020 research and innovation program HORIZON-CL6-2021-BIODIV-01-04 under grant agreement No. 101059877 “GES4SEAS -Achieving Good Environmental Status for maintaining ecosystem SErvices, by Assessing integrated impacts of cumulative pressures” and HORIZON-CL6-2022-BIODIV-01-01 under grant agreement No. 101081642 “OBAMA-NEXT - Observing and mapping biodiversity and ecosystems, with particular focus on coastal and marine ecosystems” planning, implementation and reporting of requirements set out in the Framework Directive Marine Strategy and for integrated coastal zone management”.
Conflict of interest
The authors declare that the research was conducted in the absence of any commercial or financial relationships that could be construed as a potential conflict of interest.
Publisher’s note
All claims expressed in this article are solely those of the authors and do not necessarily represent those of their affiliated organizations, or those of the publisher, the editors and the reviewers. Any product that may be evaluated in this article, or claim that may be made by its manufacturer, is not guaranteed or endorsed by the publisher.
Supplementary material
The Supplementary Material for this article can be found online at: https://www.frontiersin.org/articles/10.3389/fmars.2024.1388877/full#supplementary-material
References
AON S.R.L (2022). Revizuire studiu de evaluare adecvata pentru proiect "Reducerea eroziunii costiere faza II lot-5 -Protectia si reabilitarea litoralului romanesc al Merii negre in zona Eforie -celula de plaja ECnBS2. Constanta: Societatea de Cercetare a Biodiversitatii si Ingineria Mediului AON S.R.L.
Ban N. C., Alidina H. M., Ardron J. A. (2010). Cumulative impact mapping: Advances, relevance and limitations to marine management and conservation, using Canada's Pacific waters as a case study. Mar. Policy 34, 876–886. doi: 10.1016/j.marpol.2010.01.010
Black Sea Commission. (2007). Black sea transboundary diagnostic analysis. Istanbul, Turkey: Black Sea Commission Publications.
Black Sea Commission (BSC). (2019). State of the environment of the black sea, (2009–2014/5). ed. Krutov A. (Istanbul, Turkey: Publications of the Commission on the Protection of the Black Sea Against Pollution (BSC)), 811.
Boicenco L., Abaza V., Anton E., Bisinicu E., Buga L., Coatu V., et al. (2018) Studiu privind elaborarea raportului privind starea ecologică a ecosistemului marin Marea Neagră conform cerinţelor art. 17 ale Directivei Cadru Strategia pentru mediul marin, (2008/56/EC). Available at: http://www.mmediu.gov.ro/app/webroot/uploads/files/STUDIU%20MSFD%20V1.9.pdf.
Borgwardt F., Robinson L., Trauner D., Teixeira H., Nogueira A. J. A., Lillebø A. I., et al. (2019). Exploring variability in environmental impact risk from human activities across aquatic ecosystems. Sci. Total Environ. 652, 1396–1408. doi: 10.1016/j.scitotenv.2018.10.339
Borja A., Elliott M., Andersen J. H., Berg T., Carstensen J., Halpern B. S., et al. (2016). Overview of integrative assessment of marine systems: The ecosystem approach in practice. Front. Mar. Sci. 3. doi: 10.3389/fmars.2016.00020
Borja A., White M. P., Berdalet E., Bock N., Eatock C., Kristensen P., et al. (2020). Moving toward an agenda on ocean health and human health in europe. Front. Mar. Sci. 7. doi: 10.3389/fmars.2020.00037
Breen P., Tully O., Hynes S., Loughlin C., Reecht Y., Morley T. (2020). An integrated methodology for assessing ecological and economic impacts for marine management: A case study for abrasion and mobile fishing gear effects. Ocean Coast. Manage. 198. doi: 10.1016/j.ocecoaman.2020.105351
Ciot M. G. (2022). Implementation perspectives for the european green deal in central and eastern europe. Sustainability (Switzerland) 14. doi: 10.3390/su14073947
Clark R. (1994). Cumulative effects assessment: A tool for sustainable development. Impact Assess. 12, 319–331. doi: 10.1080/07349165.1994.9725869
Clarke K. R., Gorley R. N. (2015). PRIMER v7: user manual/tutorial. Plymouth routines in multivariate ecological research (PRIMER-690 E) (Plymouth, UK: PRIMER-E).
Cormier R., Elliott M., Borja Á. (2022). Managing marine resources sustainably – the 'Management response-footprint pyramid' Covering policy, plans and technical measures. Front. Mar. Sci. 9. doi: 10.3389/fmars.2022.869992
Crise A., Kaberi H., Ruiz J., Zatsepin A., Arashkevich E., Giani M., et al. (2015). A MSFD complementary approach for the assessment of pressures, knowledge and data gaps in Southern European Seas: The PERSEUS experience. Mar. pollut. Bull. 95, 28–39. doi: 10.1016/j.marpolbul.2015.03.024
Curtin R., Prellezo R. (2010). Understanding marine ecosystem based management: A literature review. Mar. Policy 34, 821–830. doi: 10.1016/j.marpol.2010.01.003
Duffy J. ,. E. (2006). Biodiversity and the functioning of seagrass ecosystems. Mar. Ecol. Prog. Ser. 311, 233–250. doi: 10.3354/meps311233
EC. (2017b). Commission decision (EU) 2017/848 of 17 May 2017 laying down criteria and methodological standards on good environmental status of marine waters and specifications and standardised methods for monitoring and assessment, and repealing Decision 2010/477/EU. Luxembourg: Official Journal of the European Union.
EC (2017a). COMMISSION DIRECTIVE (EU) 2017/845 of 17 May 2017 amending Directive 2008/56/EC of the European Parliament and of the Council as regards the indicative lists of elements to be taken into account for the preparation of marine strategies. Luxembourg: Official Journal of the European Union.
EPA. (2022). Cumulative impacts recommendations for ORD research.ESRI, arcGIS desktop: release 10.7 (Redlands, CA, USA: Environmental Systems Research Institute).
ESPON. (2013). European seas and territorial development, opportunities and risks ANNEX 5 to the final report: Black sea regional profile.
EU (1999a). Integrating environmental concerns into development and economic cooperation. Draft version 1.0 (Brussels: European Comision). Available at: https://www.eea.europa.eu/help/glossary/eea-glossary/cumulative-impacts.
EU (1999b) Guidelines for the Assessment of Indirect and Cumulative Impacts as well as Impact Interactions. Available at: http://europa.eu.int.
EU (2008). Directive 2008/56/EC of the European Parliament and of the Council of 17 June 2008 establishing a framework for community action in the field of marine environmental policy (Marine Strategy Framework Directive). Luxembourg: Official Journal of the European Union.
EU (2014). DIRECTIVE 2000/60/EC OF THE EUROPEAN PARLIAMENT AND OF THE COUNCIL of 23 October 2000 establishing a framework for Community action in the field of water policy. Luxembourg: Official Journal of the European Union.
Farghali M., Mohamed I. M. A., Osman A. I., Rooney D. W. (2023). “Seaweed for climate mitigation, wastewater treatment, bioenergy, bioplastic, biochar, food, pharmaceuticals, and cosmetics: a review,” in In environmental chemistry letters, vol. 21. (Germany: Springer Science and Business Media Deutschland GmbH), 97–152). doi: 10.1007/s10311–022-01520-y
Filimon A., Abaza V., Marin O., Dumitrache C. (2016) Community structure of zoobenthos associated with Cystoseira barbata facies from the Southern Romanian Black Sea coast. Available at: https://www.researchgate.net/publication/335834374.
Foley M. M., Mease L. A., Martone R. G., Prahler E. E., Morrison T. H., Murray C. C., et al. (2017). The challenges and opportunities in cumulative effects assessment. Environ. Impact Assess. Rev. 62, 122–134. doi: 10.1016/j.eiar.2016.06.008
Friedland R., Macias D., Cossarini G., Daewel U., Estournel C., Garcia-Gorriz E., et al. (2021). Effects of nutrient management scenarios on marine eutrophication indicators: A pan-european, multi-model assessment in support of the marine strategy framework directive. Front. Mar. Sci. 8. doi: 10.3389/fmars.2021.596126
Gomoiu M.-T. (1992). Marine eutrophication syndrome in the north-western part of the Black Sea. Sci. Total Environ. 683–692. doi: 10.1016/B978–0-444–89990-3.50059–6
Goodsir F., Bloomfield H. J., Judd A. D., Kral F., Robinson L. A., Knights A. M. (2015). A spatially resolved pressure-based approach to evaluate combined effects of human activities and management in marine ecosystems. ICES J. Mar. Sci. 72, 2245–2256. doi: 10.1093/icesjms/fsv080
Gray S. R. J., Gagnon A. S., Gray S. A., O'Dwyer B., O'Mahony C., Muir D., et al. (2014). Are coastal managers detecting the problem? Assessing stakeholder perception of climate vulnerability using Fuzzy Cognitive Mapping. Ocean Coast. Manage. 94, 74–89. doi: 10.1016/j.ocecoaman.2013.11.008
Gray S. A., Gray S., Cox L. J., Henly-Shepard S. (2013). “Mental Modeler: A fuzzy-logic cognitive mapping modeling tool for adaptive environmental management,” in 2013 46th Hawaii international conference on system sciences. (IEEE), 965–973. doi: 10.1109/HICSS.2013.399
Halpern B. S., Frazier M., Potapenko J., Casey K. S., Koenig K., Longo C., et al. (2015). Spatial and temporal changes in cumulative human impacts on the world's ocean. Nat. Commun. 6, 1–7. doi: 10.1038/ncomms8615
Halpern B. S., Selkoe K. A., Micheli F., Kappel C. V. (2007). Evaluating and ranking the vulnerability of global marine ecosystems to anthropogenic threats. Conserv. Biol. 21, 1301–1315. doi: 10.1111/j.1523–1739.2007.00752.x
Halpern B. S., Walbridge S., Selkoe K. A., Kappel C. V., Micheli F., D’Agrosa C., et al. (2008). A global map of human impact on marine ecosystems. Science 319, 948–952. doi: 10.1126/science.1151084
ICPDR. (2005) The danube river basin district. Available at: http://www.icpdr.org/DANUBIS.
IPCC. (2014). Climate change 2014: synthesis report. Contribution of working groups I, II and III to the fifth assessment report of the intergovernmental panel on climate change. Geneva, Switzerland: IPCC.
IPCC. (2022). “Changing ocean, marine ecosystems, and dependent communities,” in The ocean and cryosphere in a changing climate (Cambridge University Press), 447–588. doi: 10.1017/9781009157964.007
Julius S., Mazur S., Tulve N., Paul S., Loschin N., Barzyk T., et al. (2022). Cumulative impacts: recommendations for ord research. (Washington, DC: U.S. Environmental Protection Agency).
Katsanevakis S., Coll M., Fraschetti S., Giakoumi S., Goldsborough D., Mačić V., et al. (2020). Twelve recommendations for advancing marine conservation in european and contiguous seas. Front. Mar. Sci. 7. doi: 10.3389/fmars.2020.565968
Kideys A. E. (2002). Ecology: Fall and rise of the Black Sea ecosystem. Science 297, 1482–1484. doi: 10.1126/science.1073002
Kok K., Pedde S., Gramberger M., Harrison P. A., Holman I. P. (2019). New European socioeconomic scenarios for climate change research: operationalising concepts to extend the shared socioeconomic pathways. Regional Environ. Change 19, 643–654. doi: 10.1007/s10113–018-1400–0
Korpinen S., Andersen J. H. (2016). A global review of cumulative pressure and impact assessments in marine environments. Front Mar Sci. 3. doi: 10.3389/fmars.2016.00153
Korpinen S., Klančnik K., Peterlin M., Nurmi M., Laamanen L., Zupančič G., et al. (2019) Multiple pressures and their combined effects in Europe's seas. ETC/ICM Technical Report 4/2019: European Topic Centre on Inland, Coastal and Marine waters. Available at: http://europa.eu.
Lancelot C., Martin J. M., Panin N., Zaitsev Y. (2002). The north-western Black Sea: A pilot site to understand the complex interaction between human activities and the coastal environment. Estuarine Coast. Shelf Sci. 54, 279–283. doi: 10.1006/ecss.2000.0647
Langmead O., McQuatters-Gollop A., Mee L. D., Friedrich J., Gilbert A. J., Gomoiu M. T., et al. (2009). Recovery or decline of the north-western Black Sea: A societal choice revealed by socio-ecological modelling. Ecol. Model. 220, 2927–2939. doi: 10.1016/j.ecolmodel.2008.09.011
Lazar L. (2021). Anthropogenic pressures and impacts on the black sea coastal ecosystem (Constanta, Romania: CD PRESS), 167.
Lazar A., Boicenco L, Moncheva S, Denga Y, Atabay H, Abaza V, et al. (2021). Impact of the rivers on the Black Sea ecosystem (Constanta: CD Press), 225.
Ma C., Stelzenmüller V., Rehren J., Yu J., Zhang Z., Zheng H., et al. (2023). A risk-based approach to cumulative effects assessment for large marine ecosystems to support transboundary marine spatial planning: A case study of the yellow sea. J. Environ. Manage. 342. doi: 10.1016/j.jenvman.2023.118165
McQuatters-Gollop A., Gilbert A. J., Mee L. D., Vermaat J. E., Artioli Y., Humborg C., et al. (2009). How well do ecosystem indicators communicate the effects of anthropogenic eutrophication? Estuarine Coast. Shelf Sci. 82, 583–596. doi: 10.1016/j.ecss.2009.02.017
Mee L. D. (1992). The black sea in crisis: A need for concerted international action the black sea. Source: Ambio 21, 278–286.
Mee L., Friedrich J., Gomoiu M. (2012). Restoring the black sea in times of uncertainty. Oceanography 18, 100–111. doi: 10.5670/oceanog.2005.45
Micheli F., Halpern B. S., Walbridge S., Ciriaco S., Ferretti F., Fraschetti S., et al. (2013). Cumulative human impacts on Mediterranean and Black Sea marine ecosystems: Assessing current pressures and opportunities. PloS One 8. doi: 10.1371/journal.pone.0079889
Micu D., Micu S. (2006). Recent records, growth and prosed IUCN status of Donacilla cornea (Poli 1795) from the Romanian Black Sea. Cercetari Mar. 36, 117–132.
Monitorul Oficial. (2023). Ordonanţa de urgenţă nr. 97/2023 pentru aprobarea Planului de amenajare a spaţiului maritim. Available at: https://lege5.ro/gratuit/ge2dcojrge3da/ordonanta-de-urgenta-nr-97–2023-pentru-aprobarea-planului-de-amenajare-a-spatiului-maritim.
Nilsson A. E., Bay-Larsen I., Carlsen H., van Oort B., Bjørkan M., Jylhä K., et al. (2017). Towards extended shared socioeconomic pathways: A combined participatory bottom-up and top-down methodology with results from the Barents region. Global Environ. Change 45, 124–132. doi: 10.1016/j.gloenvcha.2017.06.001
Nuno A. (2022) Shoring up against erosion. Available at: https://www.eib.org/en/stories/coastal-erosion-Romania.
OECD. (2022). Environmental impacts of the war in ukraine and prospects for a green reconstruction. Available at: https://voxUkraine.org/en/economic-reasons-for-a-green-reconstruction-programme-for-Ukraine/.
Oguz T. (2005). Black sea ecosystem response to climatic teleconnections. Oceanography 18 (2), 122–133. doi: 10.5670/oceanog.2005.47
Oguz T. (2017). Controls of multiple stressors on the black sea fishery. Front. Mar. Sci. 4, 1–12. (April). doi: 10.3389/fmars.2017.00110
Oguz T. (2012). Long-term impacts of anthropogenic forcing on the black sea ecosystem. Oceanography 18, 112–121. doi: 10.5670/oceanog.2005.46
Oguz T., Velikova V. (2010). Abrupt transition of the north-western Black Sea shelf ecosystem from a eutrophic to an alternative pristine state. Mar. Ecol. Prog. Ser. 405, 231–242. doi: 10.3354/meps08538
O’Neill B. C., Carter T. R., Ebi K., Harrison P. A., Kemp-Benedict E., Kok K., et al. (2020). Achievements and needs for the climate change scenario framework. Nat. Climate Change 10, 1074–1084. doi: 10.1038/s41558–020-00952–0
Panin N., Jipa D. (2002). Danube river sediment input and its interaction with the north-western Black Sea. Estuarine Coast. Shelf Sci. 54, 551–562. doi: 10.1006/ecss.2000.0664
Perhar G., Arhonditsis G. B. (2014). Aquatic ecosystem dynamics following petroleum hydrocarbon perturbations: A review of the current state of knowledge. In J. Great Lakes Res. 40, 56–72). doi: 10.1016/j.jglr.2014.05.013
Piet G. J., Tamis J. E., Volwater J., de Vries P., van der Wal J. T., Jongbloed R. H. (2021). A roadmap towards quantitative cumulative impact assessments: Every step of the way. Sci. Total Environ. 784. doi: 10.1016/j.scitotenv.2021.146847
Pinnegar J. K., Hamon K. G., Kreiss C. M., Tabeau A., Rybicki S., Papathanasopoulou E., et al. (2021). Future socio-political scenarios for aquatic resources in europe: A common framework based on shared-socioeconomic-pathways (SSPs). Front. Mar. Sci. 7. doi: 10.3389/fmars.2020.568219
Quemmerais-Amice F., Barrere J., La Rivière M., Contin G., Bailly D. (2020). A methodology and tool for mapping the risk of cumulative effects on benthic habitats. Front. Mar. Sci. 7. doi: 10.3389/fmars.2020.569205
Riahi K., van Vuuren D. P., Kriegler E., Edmonds J., O’Neill B. C., Fujimori S., et al. (2017). The Shared Socioeconomic Pathways and their energy, land use, and greenhouse gas emissions implications: An overview. Global Environ. Change 42, 153–168. doi: 10.1016/j.gloenvcha.2016.05.009
Ross W. A. (1998). Cumulative effects assessment: Learning from canadian case studies. Impact Assess. Project Appraisal 16, 267–276. doi: 10.1080/14615517.1998.10600137
Rozenberg J., Guivarch C., Lempert R., Hallegatte S. (2014). Building SSPs for climate policy analysis: a scenario elicitation methodology to map the space of possible future challenges to mitigation and adaptation. Climatic Change 122, 509–522. doi: 10.1007/s10584–013-0904–3ï
Sinclair A. J., Doelle M., Duinker P. N. (2017). Looking up, down, and sideways: Reconceiving cumulative effects assessment as a mindset. Environ. Impact Assess. Rev. 62, 183–194. doi: 10.1016/j.eiar.2016.04.007
Slobodnik J., Arabidze M., Mgeladze M., Korshenko A., Mikaelyan A., Komorin V., et al. (2020) EMBLAS final scientific report. Available at: https://emblasproject.org/wp-content/uploads/2022/03/EMBLAS_Scientific-Report_ISBN-978–617-8111–01-4-web.pdf.
Smit K. P., Van Niekerk L., Harris L. R., McQuatters-Gollop A., Shannon L. J., Sink K. J. (2022). A roadmap to advance marine and coastal monitoring, biodiversity assessment, and international reporting: A developing nation perspective. Front. Mar. Sci. 9. doi: 10.3389/fmars.2022.886373
Smith C. J., Papadopoulou K. N., Barnard S., Mazik K., Elliott M., Patrício J., et al. (2016). Managing the marine environment, conceptual models and assessment considerations for the European marine strategy framework directive. Front. Mar. Sci. 3. doi: 10.3389/fmars.2016.00144
Stelzenmüller V., Coll M., Mazaris A. D., Giakoumi S., Katsanevakis S., Portman M. E., et al. (2018a). A risk-based approach to cumulative effect assessments for marine management. In Sci. Total Environ. 612, 1132–1140). doi: 10.1016/j.scitotenv.2017.08.289
Strokal M., Kroeze C. (2013). Nitrogen and phosphorus inputs to the Black Sea in 1970–2050. Regional Environ. Change 13, 179–192. doi: 10.1007/s10113–012-0328-z
Strokal M., Strokal V., Kroeze C. (2023). The future of the Black Sea: More pollution in over half of the rivers. Ambio 52, 339–356. doi: 10.1007/s13280–022-01780–6
Sundblad E.-L., Grimvall A., Zweifell U. L. (2021) Societal drivers behind pressures on the marine environment swedish institute for the marine environment report no 2021:4 eva-lotta sundblad, anders grimvall, ulla li zweifel. Available at: www.havsmiljoinstitutet.se.
TIBCO Software, Inc (2023). TIBCO statistica; version 14.0.1.25 (Palo Alto, CA, USA: TIBCO Software, Inc).
UN. (2015). Paris agreement. Available at: https://unfccc.int/process-and-meetings/the-paris-agreement.
Vela Almeida D., Kolinjivadi V., Ferrando T., Roy B., Herrera H., Vecchione Gonçalves M., et al. (2023). The "Greening" of Empire: The European Green Deal as the EU first agenda. (Miami, Florida: Political Geography). vol. 105. doi: 10.1016/j.polgeo.2023.102925
Willsteed E., Gill A. B., Birchenough S. N. R., Jude S. (2017). Assessing the cumulative environmental effects of marine renewable energy developments: Establishing common ground. In Sci. Total Environ. 577, 19–32). doi: 10.1016/j.scitotenv.2016.10.152
Keywords: Black Sea, human pressures, climate change, biodiversity, sustainability, environmental pressures, management scenarios
Citation: Lazar L, Spanu A, Boicenco L, Oros A, Damir N, Bisinicu E, Abaza V, Filimon A, Harcota G, Marin O, Pantea E, Timofte F, Vlas O and Korpinen S (2024) Methodology for prioritizing marine environmental pressures under various management scenarios in the Black Sea. Front. Mar. Sci. 11:1388877. doi: 10.3389/fmars.2024.1388877
Received: 20 February 2024; Accepted: 29 May 2024;
Published: 24 June 2024.
Edited by:
Heliana Teixeira, University of Aveiro, PortugalReviewed by:
Stefania A. Ciliberti, Nologin Oceanic Weather Systems, SpainMaria Flavia Gravina, University of Rome Tor Vergata, Italy
Copyright © 2024 Lazar, Spanu, Boicenco, Oros, Damir, Bisinicu, Abaza, Filimon, Harcota, Marin, Pantea, Timofte, Vlas and Korpinen. This is an open-access article distributed under the terms of the Creative Commons Attribution License (CC BY). The use, distribution or reproduction in other forums is permitted, provided the original author(s) and the copyright owner(s) are credited and that the original publication in this journal is cited, in accordance with accepted academic practice. No use, distribution or reproduction is permitted which does not comply with these terms.
*Correspondence: Luminita Lazar, bGxhemFyQGFscGhhLnJtcmkucm8=; Alina Spanu, YXNwaW51QGFscGhhLnJtcmkucm8=