- 1Chair for Fish Diseases and Fisheries Biology, Faculty of Veterinary Medicine, Ludwig-Maximilians-Universität München, Munich, Germany
- 2Department of Veterinary Public Health, Faculty of Veterinary Science, Chulalongkorn University, Bangkok, Thailand
- 3Center of Excellence for Shrimp Molecular Biology and Biotechnology (Centex Shrimp), Faculty of Science, Mahidol University, Bangkok, Thailand
- 4Department of Anatomy, Faculty of Science, Mahidol University, Bangkok, Thailand
Microplastics and glyphosate-based herbicides (GBHs) unavoidably contaminate aquatic environments, yet their combined effect on aquatic organisms has been scarcely investigated. We present a short-term study on individual and combined effects of aged polyethylene (PE) microplastics and GBH exposures at environmentally relevant concentrations on oxidative damage, antioxidant responses, immune parameters, and hepatopancreas histology in shrimp (Litopenaeus vannamei). In addition, acetylcholinesterase (AChE) was evaluated as a biomarker of GBH exposure. After 96 hours of exposure, individual PE microplastic exposure slightly influenced oxidative status, immune parameters, and histology. Decreases in AChE concentration and alterations in oxidative status, immunity, and cell population in hepatopancreas were observed in single GBH-exposed shrimp. The combinations of aged PE microplastics and GBH induced some changes that differed from individual GBH exposures. For instance, some alterations implied that the presence of aged PE microplastics may alter GBH toxicity to L. vannamei (AChE concentration and some expressions of immune-related genes). In conclusion, our results suggest that single exposure of aged PE microplastics at environmental concentration may slightly affect the health of L. vannamei and that aged PE microplastics are likely to modify the toxicity of other co-exposed chemicals.
1 Introduction
Pacific white shrimp (Litopenaeus vannamei) is a major contributor to global shrimp production with an annual global output of almost 5 million metric tons, accounting for over 50% of the total cultured shrimp production (Food and Agriculture Organization of the United Nations, 2023). These significant and continuously growing volumes of industrial-scale production are increasing the risk of shrimp exposure and contamination with microplastics due to the widespread utilization of plastic production in aquaculture (Reunura and Prommi, 2022). Tens of thousands of tons of plastic load is estimated to enter and contaminate marine environments, with the most abundant particle size of 30–500 µm of polyethylene (PE) microplastics detected in sediment (Vianello et al., 2013; Cózar et al., 2014). Microplastic exposure can interfere with the innate immune system, alter oxidative stress status through changes in superoxide dismutase and glutathione peroxidase gene expressions, and influence hepatopancreas damage in affected L. vannamei (Niemcharoen et al., 2022). Moreover, the microplastics undergoing aging processes in seawater can end up with changes in several of their original material characteristics, for example, the occurrence of oxidized groups and microcracks on their surface and a decrease in hydrophobicity (Da Costa et al., 2018; Tu et al., 2020). This phenomenon can lead microplastics to act as vectors for surrounding hydrophilic micropollutants. Exposure to such particle–contaminant complexes can lead to alterations in behavior, chemical fate, and toxicological effects of the sorbed micropollutants in environments and organisms (Menéndez-Pedriza and Jaumot, 2020).
Glyphosate-based herbicides (GBHs; with glyphosate as the active ingredient) are among the most popular non-selective and broad-spectrum herbicides widely used in the control and elimination of weeds (Székács and Darvas, 2018). Soybean, a major protein source of shrimp feed, was reported to be contaminated with up to 100 mg/kg glyphosate—five times above the acceptable maximum residue level of 20 mg/kg soybean proposed by the Food and Agriculture Organization of the UN (FAO) (Then, 2013). Once the glyphosate enters natural seawater, it could persist in the dark at 31°C (similar to shrimp culture conditions) for up to 315 days (Mercurio et al., 2014). Additionally, exposure to GBHs in invertebrates has been shown to influence immune function, oxidative stress, and antioxidant defense, leading to a reduction in survival rate (Hong et al., 2018; Yang et al., 2019).
Taking the above information in a joined context, there is a high likelihood that microplastics and GBHs co-exist in shrimp culture environments. Therefore, microplastics may serve as carriers and possibly influence the behavior and fate of the GBHs in feed as one of the main exposure routes to these micropollutants, resulting in modifications of adverse effects in affected shrimp (Thammatorn and Palić, 2022). However, to our best knowledge, an experimental study about the acute effects of co-exposure of L. vannamei to microplastics and GBHs is not available.
In the current study, PE microplastics were selected since PE is commonly used as a pond liner material in shrimp culture (Ranjan and Boyd, 2018), and it is frequently found in marine environments (Vianello et al., 2013). Shape, size, and concentration of used PE microplastics were based on the environmentally relevant PE microplastic properties detected in marine sediment due to behaviors of shrimp that tend to dwell and feed on the bottom of a pond and limited/missing information about microplastic contamination amounts in shrimp feed. The objectives of this study were 1) to determine oxidative stress, antioxidant, and immune responses and analyze the histology of L. vannamei digestive system after acute (96-hour) exposure to feed contaminated with a GBH at an environmentally relevant concentration found in soybean, or at an unexpectedly high concentration in case of GBH misuse, and mechanically and biologically aged PE microplastics, either alone or in combination with GBH; and 2) to assess whether the co-exposure to PE microplastics can affect acetylcholinesterase activity and biomarker responses provoked by the GBH toxicity in shrimp (Mensah et al., 2012).
2 Materials and methods
2.1 Preparation of aged microplastics and treatment feeds
Mechanically and biologically aged PE microplastic fragments (32–500 µm) with and without sorbed GBHs were generated and coated on basic feed for exposure treatment (further information in Supplementary Figure 1; Supplementary Text 1). The sizes and concentrations of the microplastics were selected based on environmental detection in marine sediment (Vianello et al., 2013). In addition, GBH concentrations were used according to the environmentally relevant glyphosate residue level in soybean and soybean percentage in typical shrimp feed (Then, 2013; Food and Agriculture Organization of the United Nations, 2017).
2.2 Animals and experimental design
A total of 180 juvenile Pacific white shrimp (L. vannamei; 4.5 ± 0.9 g, 8.1 ± 0.6 cm) from Crusta Nova farm (Munich, Germany) were randomly and equally divided into six treatment groups: A) negative control (NC; basic feed without additional contaminants except with background contaminants), B) only microplastic (MP; basic feed contaminated with 408 ± 128 microplastic pieces/g feed, Supplementary Text 2), C) MP+Lo-GBH (MP + 20 mg glyphosate/kg feed), D) Lo-GBH (basic feed contaminated with 20 mg glyphosate/kg feed), E) MP+Hi-GBH (MP + 200 mg glyphosate/kg feed), and F) Hi-GBH (basic feed contaminated with 200 mg glyphosate/kg feed). Each of these groups was further divided into three replicates of 10 shrimp. To mimic the environment of shrimp farming with pond liners, two (longer) side walls of independent aquariums (50 cm long × 26.5 cm wide × 31.5 cm high) were lined with PE pond liner and filled with 33 L of artificially prepared seawater. The shrimp were reared in aquariums at 30°C ± 1°C and on a photoperiod of 12-hour light:12-hour dark. Total artificial saltwater was renewed, and debris, i.e., shrimp exoskeleton and feces, were removed once a day to maintain water quality, as follows: 22 ± 2 ppt salinity, >6 mg/L dissolved oxygen, pH 7.8 ± 0.2, <0.05 ppm total ammonia nitrogen, <0.2 ppm nitrite, and <10 ppm nitrate. The shrimp were manually fed with 3.54% biomass/day three times a day (08:00–09:00, 12:00–13:00, and 16:00–17:00) based on the recommendation of the feed manufacturer for the entire experimental period.
At the end of the 96-hour toxicity test and after cold-induced anesthesia, 100-µL hemolymph was withdrawn from the ventral sinus of each individual using a 1-mL syringe containing 100 µL of an ethylenediaminetetraacetic acid (EDTA)-free anticoagulant. The obtained hemolymph–anticoagulant mixture was used for the determination of total hemocyte count (THC) and phenoloxidase (PO) activity.
After hemolymph collection, the shrimp were euthanized. Three shrimp from each treatment group were fixed with Davidson’s solution and then processed for histological examination (Cervellione et al., 2017). Whole hepatopancreas and a muscle portion were extracted from the rest of the euthanized shrimp and snap-frozen in a bath containing dry ice and denatured absolute ethanol to determine the malondialdehyde (MDA) content, total protein, and mRNA expression in hepatopancreas as well as for measuring the acetylcholinesterase (AChE) activity and concentration, and total protein in muscular tissue. The scheme of the experimental design is presented in Figure 1.
2.3 Acetylcholinesterase
The AChE activity was measured in triplicate in pooled muscle samples in accordance with procedures described by Wilson and Henderson (2007) and Tu et al. (2009). In short, muscular tissue was homogenized in ice-cold solubilization buffer (50 mM KH2PO4/K2HPO4, pH 7.5, and 1× protease inhibitor cocktail) in a ratio of 1 to 7.5 (muscle to buffer). The clear supernatant [post-mitochondrial fraction (PMF)] was obtained by centrifuging the homogenate at 10,000 g for 10 minutes at 4°C. The final 320-µL reaction mixture in each well of a 96-well plate included the following: PMF sample, ChE assay buffer (0.05 M Na2HPO4/NaH2PO4, pH 7.4), 5,5′-dithiobis-2-nitrobenzoic acid (DTNB) color reagent with a final concentration of 0.32 mM, and acetylthiocholine (ATCh substrate) with a final concentration of 1.5 mM. The measurement was performed using a microplate reader (SpectraMax® M5, Molecular Devices, San Jose, CA, USA) at 412 nm for 10 minutes. The AChE activity unit was converted from absorbance/min to nmol hydrolyzed acetylthiocholine·min−1·mL−1 PMF. Additionally, the AChE concentration at minute 10 was calculated in the unit of nmol hydrolyzed acetylthiocholine/mL PMF.
Total protein concentration in PMF samples was determined using a Coomassie (Bradford) protein assay kit (Thermo Scientific®, Rockford, IL, USA) and used to normalize and calculate the AChE activity and concentration as nmol hydrolyzed acetylthiocholine·min−1·mg−1 protein and nmol hydrolyzed acetylthiocholine/mg protein, respectively.
2.4 Malondialdehyde content
Quantification of MDA content was performed as previously described protocol (Tu et al., 2008) with some modifications. Briefly, hepatopancreatic tissue was homogenized in ice-cold solubilization buffer to obtain 10% w/v homogenate. The homogenate was diluted 1:5 with the ice-cold solubilization buffer and incubated in an incubator (Binder GmbH, Tuttlingen, Germany) at 37°C for 1 hour. Afterward, 600 µL of 5% chilled trichloroacetic acid and 600 µL of 0.67% thiobarbituric acid were added to the MDA sample. The filtrates in microcentrifuge tubes with screwed caps were obtained from the filtration of 1-mL syringes loaded with the well-mixed samples through 0.2-µm syringe filters and then placed in a boiling water bath (GFL® 1002–1013, Burgwedel, Germany) for 15 minutes. After boiling, the filtrates were placed in three replicate wells and measured at 535 nm.
Total protein concentration was quantified using a Coomassie-based protein assay kit (Thermo Scientific®, Rockford, IL, USA). The hepatopancreas homogenate was centrifuged at 10,000 g for 10 minutes at 4°C to obtain the supernatant as a PMF sample. Then, the PMF sample was assayed as a total protein sample of hepatopancreas according to the procedure specified by the manufacturer. Final MDA contents normalized with total protein concentrations were expressed as nmol MDA produced/mg protein.
2.5 Total hemocyte count
Thirty microliters of the obtained hemolymph–anticoagulant mixture was immediately mixed with an equal volume of 10% Formal Saline and kept at 4°C. The fixed hemolymph samples were mixed with an equal proportion of 0.25% Rose Bengal Solution and incubated for 10 minutes at room temperature. After incubation, 10 µL of the stained hemolymph sample was placed in a hemacytometer for THC determination using a light microscope (Olympus® CX31, Tokyo, Japan). The THC was represented as hemocyte numbers per milliliter of hemolymph.
2.6 Phenoloxidase activity
The obtained hemolymph–anticoagulant mixture was further centrifuged in a refrigerated centrifuge (4°C) at 800 g for 10 minutes to separate the clear supernatant and freeze at −80°C as a plasma sample for PO activity assay. The PO activity was investigated as previously described, with minor modifications (Huang et al., 2010; Song et al., 2017). Briefly, 6.7 µL of one-time thawed pool of three plasma samples was pipetted directly into a 96-well microtiter plate, followed by 293.3 µL of freshly made 3 mg/mL l-di-hydroxy-phenylalanine (l-DOPA) solution in 0.1 M potassium phosphate (K2HPO4/KH2PO4) buffer, pH 6.6, thoroughly mixed, and incubated at room temperature for 6 minutes. PO activity was measured as OD at 490 nm and expressed as a unit of PO activity per mL of hemolymph. An increase in absorbance of 0.001 per minute was defined as one unit of PO activity.
2.7 qRT-PCR analysis of gene expression
The TRI Reagent® (Sigma-Aldrich Chemie GmbH, Taufkirchen, Germany) was used to extract total RNA from the hepatopancreas. Thereafter, cDNA was generated using the reverse transcription reaction mix (Promega GmBH, Walldorf, Germany). The qPCR was performed on several immune-related genes and run on an Agilent Mx3000P QPCR System (Agilent Technology, Santa Clara, CA, USA) with the following reaction setting: 95°C for 10 minutes, followed by 40 cycles of 95°C for 15 seconds, and 60°C for 1 minute. All samples were amplified in triplicate, and the obtained Ct values were used for relative quantification of the mRNA expression level according to the 2−ΔΔCt method (Livak and Schmittgen, 2001). The detailed method is provided in the Supplementary Material (Supplementary Table 1; Supplementary Text 3).
2.8 Histological examination
A cephalothorax of each representative shrimp preserved in Davidson’s solution for a minimum of 48 hours was cut into four sections with 2–3-mm thickness from anterior to posterior part, placed in a tissue processing cassette, and submitted to the Institute of Veterinary Pathology (Center for Clinical Veterinary Medicine, Ludwig-Maximilians-University, Munich, Germany) for microtome section (4 μm) preparation and hematoxylin and eosin (H&E) staining. The histological interpretation in shrimp hepatopancreas for each section, based on the presentation of hepatopancreatic cell diversity (population), including the hemocyte accumulation, was observed and photographed using an optical microscope (Leica DM750, Wetzlar, Germany) with a digital camera (Leica ICC50 HD).
2.9 Statistical analysis
All the numerical data are presented as the mean ± standard deviation. The data were analyzed and visualized using the RStudio program with Rcpp and dplyr packages (R version 4.2.0). Data were tested for homogeneity of variance (Bartlett’s test) and normality of distribution (Shapiro–Wilk test) prior to the analysis. The data that did not meet the assumptions of equal variance and normality were analyzed by a non-parametric test (Kruskal–Wallis test) followed by the Wilcoxon rank sum test (Mann–Whitney U test) with continuity correction. Data that satisfied the assumptions were analyzed by one-way ANOVA followed by Tukey’s test to identify statistically significant differences between means of treatment groups. Significant differences were considered at a p-value of less than 0.05.
3 Results
3.1 Effect of PE microplastics and GBH exposure on AChE activity and concentration
A significant decrease in AChE concentration was observed in shrimp exposed to GBH alone, where concentrations relative to the NC were significantly reduced by 24.87% and 31.36% after exposure to low and high GBH concentrations, respectively. Following the co-exposure to PE microplastics and GBH, reduction in the AChE concentration showed a similar trend, but less pronounced and not statistically significant, at approximately 13.17% and 19.44% at the same concentrations of GBH. Similar to shrimp exposed to PE microplastics alone, although not significantly different, there was an 18.65% decrease in AChE concentration in the muscle tissue (Figure 2A). Neither single nor combined exposures to PE microplastics and GBH significantly affected the AChE activity in the shrimp muscle (Figure 2B).
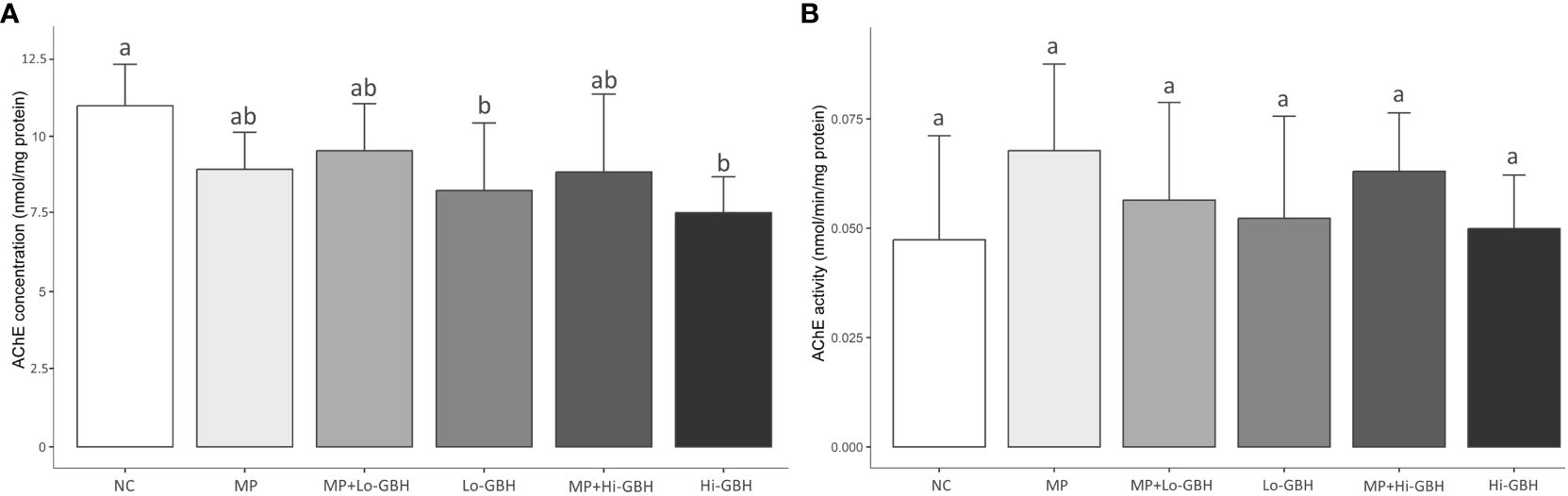
Figure 2 Acetylcholinesterase (AChE) concentration (A) and activity (B) in the muscle of Pacific white shrimp (Litopenaeus vannamei) exposed to polyethylene (PE) microplastics and glyphosate-based herbicide (GBH) alone or in combination for 96 hours. Data are expressed as mean ± SD (n = 9). Different letters denote significant differences (p < 0.05, Tukey’s multiple-comparison test).
3.2 Effect of PE microplastics and GBH exposure on oxidative stress
3.2.1 Oxidative damage: MDA content
After 96 hours of exposure, there were significant increases of 91.41% and 103.93% in MDA content in the hepatopancreas of shrimp in the MP+Lo-GBH and Hi-GBH groups compared to the NC, respectively. The insignificant increases in the MDA content compared with the NC were observed in the MP (31.72%), Lo-GBH (77.86%), and MP+Hi-GBH groups (81.26%). However, these differences between the individual and combined treatments at low and high GBH concentrations were not statistically significant (Figure 3A).
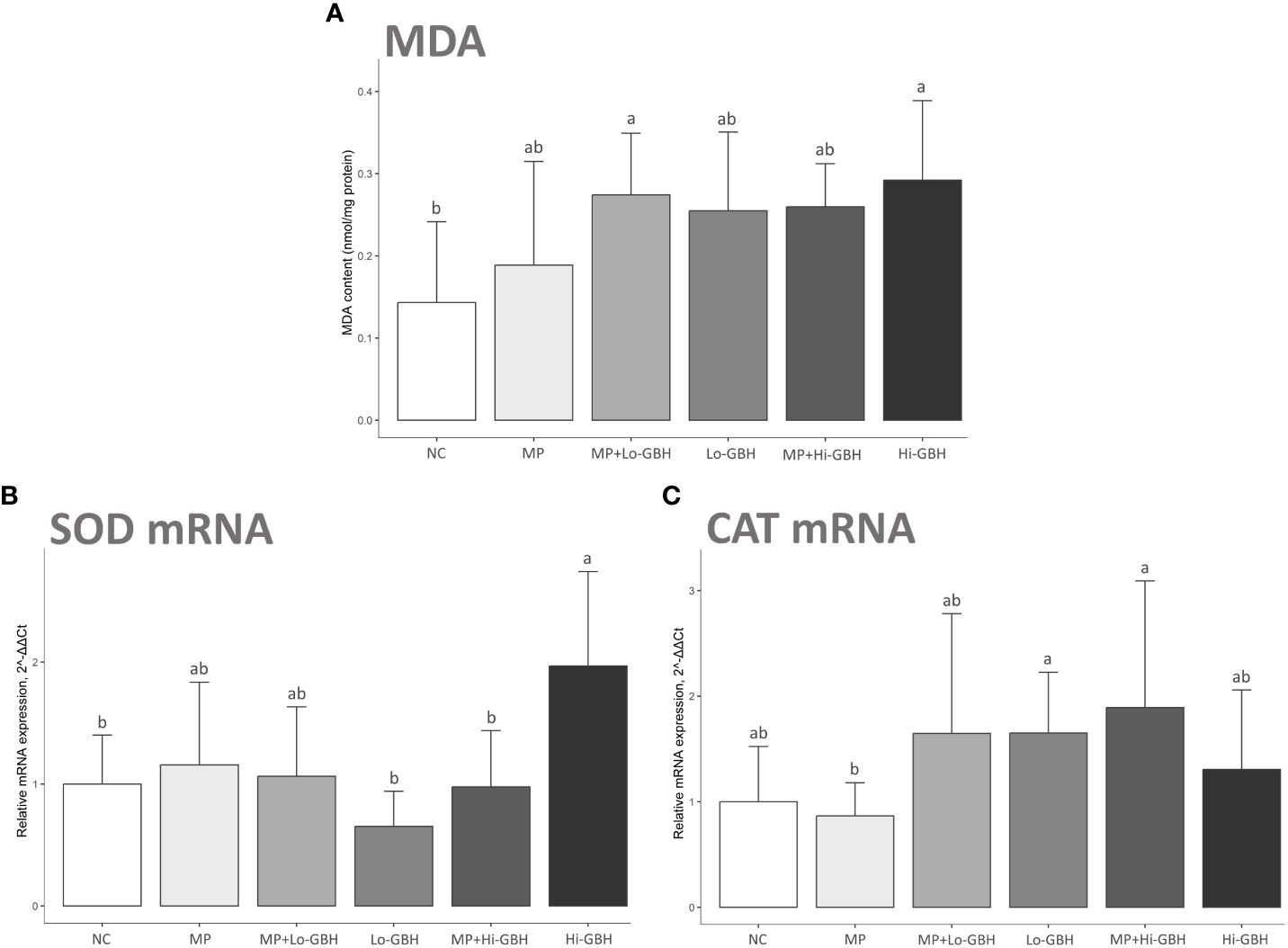
Figure 3 Oxidative stress biomarkers: malondialdehyde (MDA) content (A) and expression of mRNA encoding antioxidant enzymes (B, C) in hepatopancreas of Pacific white shrimp (Litopenaeus vannamei) after 96 hours of exposure to polyethylene (PE) microplastics and glyphosate-based herbicide (GBH). Data are expressed as mean ± SD (n = 9). Different letters denote significant differences [p < 0.05, Tukey’s multiple-comparison test (A) and Wilcoxon rank sum test (B, C)].
3.2.2 Antioxidant system: superoxide dismutase and catalase
The MP group showed a non-significant difference compared to the NC and an inverse trend in transcript expressions between genes encoding superoxide dismutase (SOD) and catalase (CAT). There was a 1.16-fold increase in the gene encoding SOD expression, while a 0.87-fold decrease in the one encoding CAT expression was observed (Figures 3B, C).
The Hi-GBH group displayed a significant increase in transcript encoding SOD (1.97-fold; cytMnSOD) compared to the NC (Figure 3B). Changes in trends for cytMnSOD and CAT expression levels were observed in the Lo-GBH and Hi-GBH groups, without statistical significance. The opposite trend of 0.65-fold decreased cytMnSOD and 1.65-fold increased CAT expressions was observed in the Lo-GBH group, whereas the similarly increasing trend of 1.97-fold cytMnSOD and 1.31-fold CAT expressions was observed in the Hi-GBH group (Figures 3B, C).
Transcript encoding SOD was significantly lower in the MP+Hi-GBH group compared to the Hi-GBH group (Figure 3B). However, no significant differences were recognized for the expressions of cytMnSOD and CAT in the MP+Lo-GBH and MP+Hi-GBH groups when compared to the NC. cytMnSOD upregulation (1.06-fold) and CAT upregulation (1.65-fold) trends were noted in the MP+Lo-GBH group, while the MP+Hi-GBH group showed cytMnSOD downregulation (0.97-fold) and CAT upregulation (1.89-fold) trend compared to the NC (Figures 3B, C).
3.3 Effect of PE microplastics and GBH on immune responses
No mortality during the 96-hour exposure period was observed, and the shrimp in all study groups showed no signs of abnormality in their behavior and general health.
3.3.1 Hemolymph: THC and PO activity
THC and PO activity were not significantly affected after 96-hour dietary exposure to PE microplastics and GBH, either as single or as combined exposure. Nevertheless, the THC was increased across all treatments by 2.40%, 8.15%, 29.00%, 38.74%, and 20.63% in the MP, MP+Lo-GBH, Lo-GBH, MP+Hi-GBH, and Hi-GBH groups, respectively. In the Hi-GBH group, the PO activity was the most decreased by 18.50%, while the PO activity was slightly decreased by 9.59% in the MP group and increased by 2.85%, 6.91%, and 6.07% in the MP+Lo-GBH, Lo-GBH, and MP+Hi-GBH groups, respectively (Figure 4A).
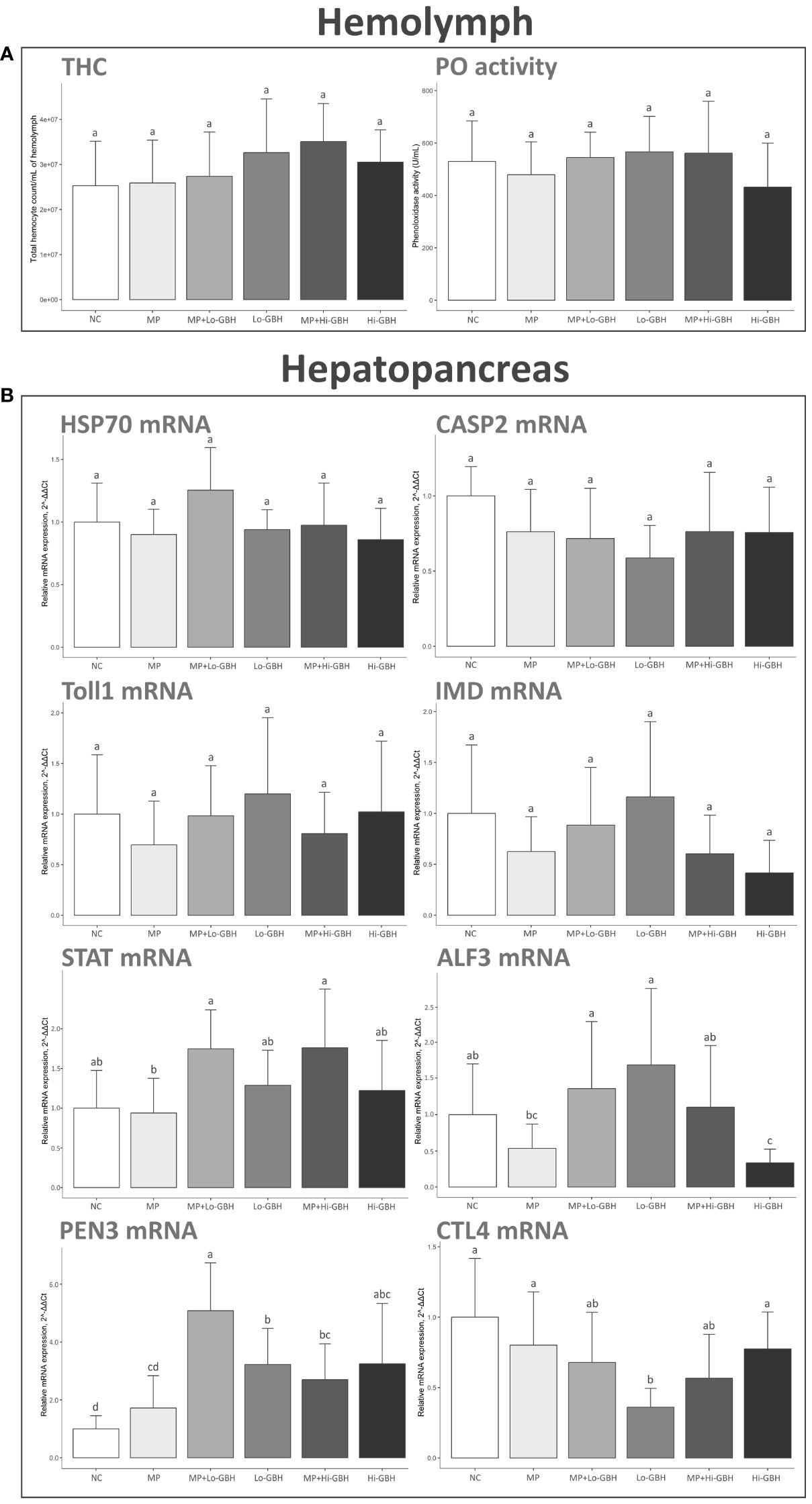
Figure 4 Immunological effects of microplastic (MP)/glyphosate-based herbicide (GBH) on total hemocyte count (THC) and phenoloxidase (PO) activity in hemolymph (A) and immune-related gene expression in hepatopancreas (B). Data are expressed as mean ± SD (THC and PO activity: n = 10, gene expression: n = 9). Different letters denote significant differences {p < 0.05, Tukey’s multiple-comparison tests [(A, B); CASP2 and STAT] and Wilcoxon rank sum test (B); HSP70, Toll1, IMD, ALF3, PEN3, and CTL4}.
3.3.2 Hepatopancreas: immune-related gene expression
Exposure of shrimp to dietary PE microplastics for 96 hours did not significantly alter the transcript profile of immune-related genes of interest compared to the NC. After exposure to GBH, the transcript encoding anti-lipopolysaccharide factor 3 (ALF3) was significantly downregulated in the Hi-GBH group compared to the NC and MP+Hi-GBH groups.
Penaeidin-3 (PEN3) in the Lo-GBH and Hi-GBH groups was significantly upregulated compared to that in the NC. Combination exposure to PE microplastics and GBH also resulted in significant upregulation of PEN3 compared to that in the NC. Furthermore, a statistically significant difference between the MP+Lo-GBH and Lo-GBH groups was seen in the expression level of PEN3.
The transcript expression of CTL4 encoding C-type lectin 4 was significantly downregulated in the Lo-GBH group when compared to the NC. No significant differences were observed in aged PE microplastics combined with low-dose GBH. No statistically significant alterations in the CTL4 expression level were recognized between single GBH exposures and combination exposures at the same GBH concentration.
The expressions of other immune-related genes including hsp70, Casp2, Toll1, IMD, and STAT were not significantly affected by any of the treatment groups compared to the NC (Figure 4B).
3.4 Effect of PE microplastics and GBH on hepatopancreas histology
Histological observation of the hepatopancreatic tissues of shrimp treated with different combinations in feed pallets revealed prominent populations of blister-like cells (B cells) and resorptive cells (R cells) in the tissue sections of the MP group (Figure 5B). The intact organization in cytoarchitecture was similar to the one presented in the NC group (Figure 5B). Furthermore, a number of B cells were observed in the hepatopancreatic tubules, together with the infiltration of hemocytes in the inter-tubular space of hepatopancreas in the Lo-GBH group compared to the NC (Figure 5D). The hepatopancreatic tissues of the Hi-GBH group presented with a dominant R-cell distribution along, and including cross-sections of, the tubules when compared with that of the NC (Figure 5F). Notably, in the histology of hepatopancreas taken from shrimp in the combination groups MP+Lo-GBH and MP+Hi-GBH, numerous populations of ambiguous cells with vacuoles (characterized between B and R cells) were prominent in comparison with those in the NC and MP groups (Figures 5C, E). The existence of possible adverse effects on hepatopancreas histology in shrimp with feed contaminated with PE microplastics and GBH, either alone or in combination, is presented in Figure 5.
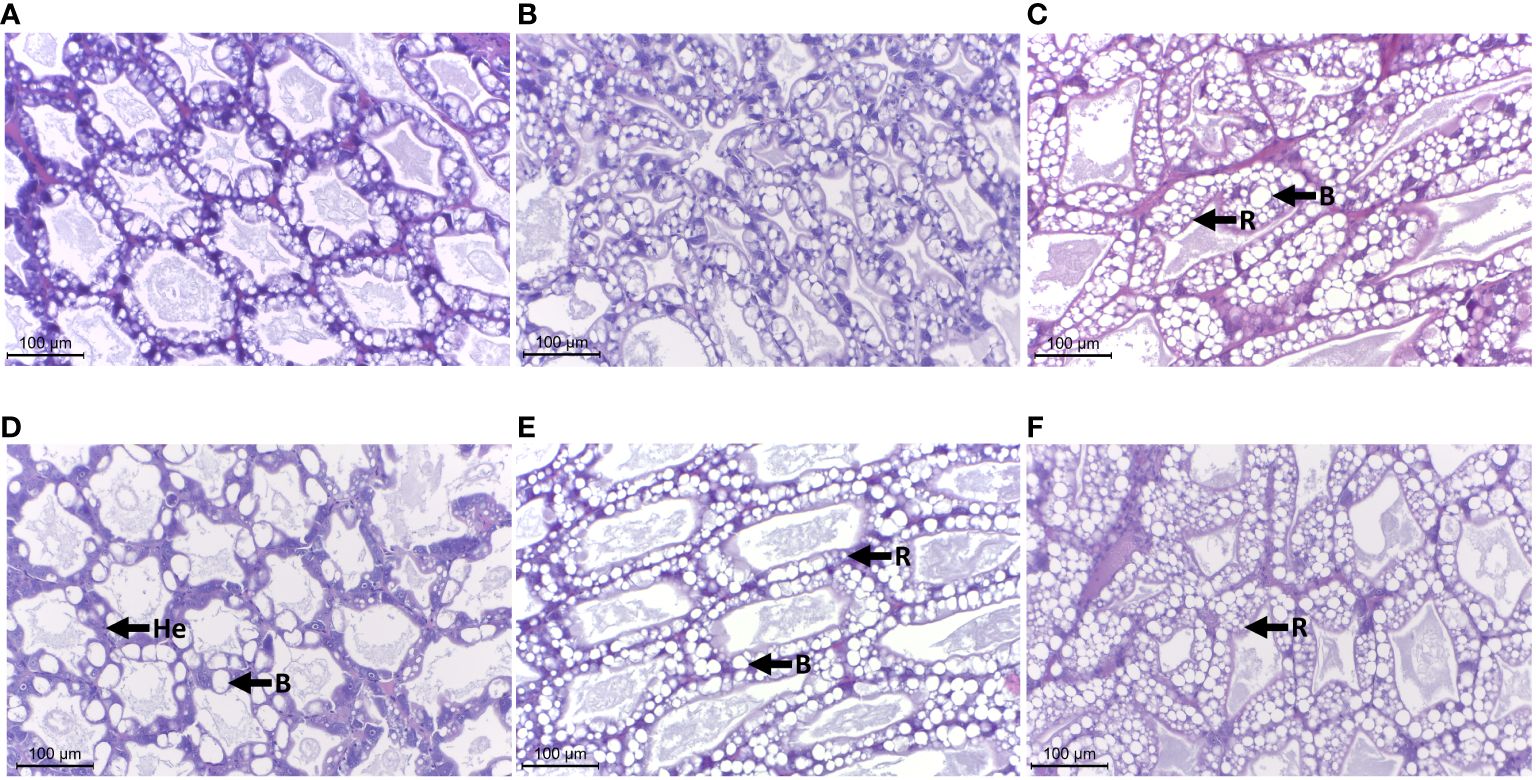
Figure 5 Effects of polyethylene (PE) microplastics and glyphosate-based herbicide (GBH) in single or combination on histology of the hepatopancreas, H&E stain, ×400 magnification, scale bar = 100 µm. He, hemocytes; B, B cells; R, R cells. (A) Negative control (NC). (B) Microplastic (MP). (C) MP+Lo-GBH. (D) Lo-GBH. (E) MP+Hi-GBH. (F) Hi-GBH.
4 Discussion
Several studies of glyphosate toxicity on AChE activity in crustaceans reported that glyphosate acts as a concentration-dependent AChE inhibitor. However, in this study, during 96-hour dietary exposure of L. vannamei to individual, aged PE microplastic, GBH, and their combinations, no significant changes in AChE activity were observed when compared to those in the NC. The magnitude and severity of the adverse effects depend on the concentration and bioavailability of glyphosate and adjuvants in target tissues (Hong et al., 2018; Lanzarin et al., 2019), and there is a possibility that environmentally relevant GBH concentrations used in this study were insufficient to trigger the significant alteration in AChE activity in the exposed shrimp, as reported in some other organisms (Lopes et al., 2017; Lanzarin et al., 2019). In addition to glyphosate’s inhibitory effect on the AChE activity, it is possible that a lower concentration of AChE in muscle tissue may cause decreased sensitivity of muscle samples for the purposes of detecting changes of the AChE activity in organisms exposed to GBH for a short period of time. For example, Glusczak et al. (2006) reported that exposing the piava freshwater fish (Leporinus obtusidens) to GBH for 96 hours significantly decreased the AChE activity in the brain, but not in the muscle. Significant decreases in AChE concentration observed in shrimp from the Lo-GBH and Hi-GBH groups suggests, however, that the potential for environmentally relevant concentrations of GBH to cause disruption in mechanisms involved in AChE regulation in L. vannamei cannot be completely ruled out.
The bioavailability and concentration of GBH at the site of its metabolic activity could influence not only AChE activity but also its concentration. In zebrafish (Danio rerio) exposed to glyphosate, alteration in AChE gene expression was reported, yet the AChE activity was not affected during the entire period of the acute toxicity test (Lopes et al., 2017). In contrast, similar but less pronounced inhibition of AChE concentrations of the MP+Lo-GBH and MP+Hi-GBH groups compared to the Lo-GBH and Hi-GBH groups, respectively, implied that aged PE microplastics and/or the aging process may be responsible for the reduction of GBH bioavailability in the shrimp muscle. A possible scenario where microplastics reduce the transfer and bioavailability of particle-mediated micropollutants has been supported by recent studies. Mohamed Nor and Koelmans (2019) reported that PE microplastics could sorb, and reduce the desorption of, polychlorinated biphenyls (PCBs) in contaminated feed, leading to lower transfer of PCBs across the simulated gut model. Similarly, Dolar et al. (2021) suggested that polyester fibers and rubber crumbs with mixed synthetic materials tend to reduce the bioavailability of co-exposed chlorpyrifos in woodlice (Porcellio scaber), based on the increases in hemocyte viability and AChE activity compared to those of P. scaber exposed to chlorpyrifos alone.
A possible explanation for this phenomenon may be that the emergence of new functional groups (hydroxyl and carbonyl groups), as well as biofilm colonization on the PE microplastic surface during the biological aging process, may have increased microplastic surface hydrophilicity (Da Costa et al., 2018; Tu et al., 2020), thereby inducing stronger binding between these aged PE microplastics and hydrophilic GBH. In addition to interactions between aged PE microplastics and GBH, further interactions between feed and PE-GBH microplastics in the gastrointestinal tract of L. vannamei could also play a major role in decreasing desorption and uptake of GBH from the aged microplastics into the intestinal lumen and their transfer to target tissues. One possibility is that aged PE-GBH microplastics could be released during the digestion of feed pellets and that PE may still have the capacity to sorb and trap GBH released in the process (keeping the adsorption reaction equilibrium). As a consequence, less availability of GBH to be transferred into the L. vannamei tissues may subsequently result in a reduced inhibitory effect on AChE concentration in the MP+Lo-GBH and MP+Hi-GBH groups.
In the present study, a set of oxidative stress indicators—MDA content and expression levels of cytMnSOD and CAT—were measured to evaluate the potential oxidative damage induced by single or combined exposures to aged PE microplastics and GBH. We found that the MDA content trend was increased in the hepatopancreas across all treatment groups compared to the NC. Nevertheless, the insignificant increase in MDA content in the MP, Lo-GBH, and MP+Hi-GBH groups may be associated with the observed changes in cytMnSOD and CAT expression levels of each respective group. Even though the cytMnSOD and CAT expression levels of the MP group were not significantly different compared to the NC, the inverse relationship between their expression levels could still indicate the harmonization of SOD and CAT work against cellular oxidative stress, resulting in its insignificantly increased MDA contents and oxidative cellular damage (Wang et al., 2018). This reasoning also provides support for the observed histological result of no differences in organization and morphology of hepatopancreatic cells between the MP and NC groups, suggesting that ingestion of feed contaminated with aged PE microplastics at environmentally relevant concentration for 96 hours does not induce significant oxidative stress in L. vannamei. Similar results were reported in brine shrimp (Artemia salina) after exposure to polystyrene microplastics (1 mg/L) for 48 hours and 14 days, where only 14-day exposure induced a sharp increase in reactive oxygen species (ROS) production (Suman et al., 2020).
Considering the oxidative stress induced by GBH alone and in combination with aged PE microplastics, we found that shrimp from the Hi-GBH and MP+Lo-GBH groups had significantly increased MDA content, while in the Lo-GBH and MP+Hi-GBH groups, the increase in MDA content was trending but not significant when compared to that in the NC. This MDA increase indicated that individual and combined GBH dietary exposure could induce lipid damage. However, the post-hoc comparisons indicated that there were no significant differences in MDA content between groups exposed to single GBH and combined GBH with aged PE microplastics at the same concentrations. This further suggests that the presence of aged PE microplastics did not have a significant effect on MDA induction of GBH in L. vannamei.
Corresponding with the above, histological examination revealed no evidence of significant differences in hepatopancreatic cell damage between single and combination groups. However, the increased presence of B and R cells was observed after dietary exposures to both single and combined GBH, suggesting that B and R cells could have a role in GBH detoxification and maintenance of shrimp homeostasis. A similar result was reported by Sreeram and Menon (2005) when a pronounced increase in B-cell number was associated with exposure to sublethal concentrations of petroleum hydrocarbons in the hepatopancreas of Kadal shrimp (Metapenaeus dobsoni) and related to a detoxification mechanism at a cellular level. An increase in altered R cells of ghost shrimp (Palaemonetes argentinus) exposed to pesticides suggests that R cells may be directly involved in detoxification (Petriella, 2007), although R cells were thought to be only indirectly related to detoxification by providing active energy for this mechanism in response to environmental stress in Macrobrachium borellii Nobili, 1896, and P. argentinus Nobili, 1901 (Collins, 2010).
In addition to the MDA content, the expression of cytMnSOD and CAT genes was studied to determine the oxidative stress potential of dietary GBH exposures. There was no clear antioxidant response trend for the dietary exposures of L. vannamei to single and combined GBH, except for the significantly increased expression of cytMnSOD in the Hi-GBH group when compared to the NC. The increase in cytMnSOD expression after exposure to a high concentration of GBH may have resulted from compensatory mechanisms in hepatopancreas to sustain the homeostasis of cells under conditions of increased GBH bioavailability. Concerning the combination effects in our study, hepatopancreas transcript profiling revealed significant upregulation of cytMnSOD in the Hi-GBH group compared to the MP+Hi-GBH group; further, an inverse regulation trend of CAT between these two groups was observed.
Wang et al. (2021) suggested that biofouling in the biological aging process of PE microplastics may increase the affinity of microplastics to polar chemicals (like GBH). Furthermore, the establishment of biofilms and the presence of microbes on PE microplastic surfaces have the potential to interact with both microplastics and GBH. Another possible microbial source in aged PE microplastic–GBH–microbe interactions is the gut microbiota of the test species. A previous study has demonstrated that glyphosate or GBH at specific concentrations alters the composition of the gut microbiota in Chinese mitten crab (Eriocheir sinensis) (Yang et al., 2019). Such alterations are further associated with oxidative stress and changes in biotransformation capacity or uptake pathways of glyphosate within the gastrointestinal tract. Therefore, toxic concentrations of GBH released from the aged PE microplastics may be altered through interactions with the gut microbes, changing diffusion across the gut barrier, resulting in qualitative or quantitative modifications of GBH-induced oxidative stress in target tissues. This reasoning could also partially explain why both cytMnSOD and CAT expression levels in shrimp exposed to either single or combined GBH were not changed significantly, except in the Hi-GBH group, but allowed for insufficient increase of the MDA content in hepatopancreas to respond to GBH toxicity.
Changes in physiology and the amount of circulating hemocytes are associated with levels of PO activity (Kulkarni et al., 2021). In the present study, there were no statistically significant differences between PO activities between treatment groups, consistent with the corresponding changes in THC. The THC and PO activity results of the MP group were in accordance with a study by Dolar et al. (2021), who demonstrated insignificant alterations in THC and PO-like activity in crustacean P. scaber following 1.5% crumb rubber exposure. Revel et al. (2020) also reported no significant differences in PO activity in the coelomic fluid of estuarine worm (Hediste diversicolor) during 10-day exposure to merely polyethylene and polypropylene microplastic combination at environmentally relevant concentrations (10 and 50 mg/kg sediment, respectively). However, it is interesting to note that, although not statistically significant, a clear decreasing trend in PO activity and THC in shrimp from the Hi-GBH group was observed when compared to the MP+Hi-GBH group, possibly indicating reduced GBH toxicity and/or increase in compensatory response mechanisms following simultaneous exposure of shrimp to microplastics and GBH. Immunotoxicity modification by co-exposure of a toxic compound and microplastics was previously observed in other invertebrate organisms such as blood clam (Tegillarca granosa) (Tang et al., 2020) and mussel Mytilus coruscus (Gu et al., 2020).
Cell death via activation of caspases (enzymes that execute cytotoxic mechanisms in innate immunity) occurred in cells during exposure to microplastics (Hou et al., 2021) or GBH (Hao et al., 2019). Here, analysis of transcript encoding caspase-2 enzyme in hepatopancreas showed no significant differences in expression of Casp2 between the MP group and NC, also in accordance with unchanged MDA content and expression levels of cytMnSOD and CAT in the hepatopancreas.
Previous studies (Redza-Dutordoir and Averill-Bates, 2016; Fei et al., 2020) have shown that the imbalance between ROS and the antioxidant system could cause oxidative stress, resulting in the increase of cellular lipid peroxidation level and disruption of membrane fluidity and integrity, activation of caspases, and eventually cell death. However, microplastic sizes (32–500 µm) used in our experiment may be an important factor for an explanation of negligible induction of cell death (Beijer et al., 2022). This is also supported by Tang et al. (2020), who reported the significant downregulation of Caspase-3 in hemocytes of T. granosa exposed to 500 nm compared to the 30-μm polystyrene particle size.
Considering the cytotoxicity effect of GBH alone and in combination, there were no significant differences in the hepatopancreatic expression of Casp2 in all groups exposed to GBH at environmentally high concentrations via feed, suggesting that caspase-induced hepatopancreatic cell death is unlikely to be affected by GBH. Corresponding with this, the expressions of cytMnSOD and CAT in hepatopancreas were generally altered, thereby responding to elevated MDA contents induced by GBH exposure, in an effort to maintain cellular redox homeostasis and prevent hepatopancreatic cells from damage and death. Additionally, this effect was consistent with no apparent changes in the expression of transcript encoding HSP70 across all treatment groups, supporting the suggestion that changes in antioxidant enzyme level and activity may be sufficient to modulate oxidative stress, resulting in no disturbance in the expression of hsp70.
Another possibility resulting in the lack of effects on hsp70 expression in hepatopancreas may be that several families of heat shock proteins (HSPs; HSP60, HSP70, and HSP90) are triggered by a different cascade of events and changed in different patterns depending on exposed chemical pollutants. Magni et al. (2019) presented some evidence that zebra mussel (Dreissena polymorpha) exposed to a combination of 1- and 10-μm virgin polystyrene microbeads at 2 × 106 microplastic particles/L for 6 days exhibited induction of oxidative stress, whereas the inactivation of HSPs in gills was observed, inferring that HSPs may be disrupted by a different mechanism.
To explore the toxicological effects of aged PE microplastics and GBH, either alone or in combination, on humoral immunity, we investigated the expression of a number of transcripts encoding humoral immune-related molecules in the hepatopancreas. We found no significant differences across expressions of investigated immune-related genes between the MP group and NC, indicating that dietary exposure to aged PE microplastics at an environmentally relevant concentration may not significantly affect the humoral innate immunity of L. vannamei. A previous study (Zhang et al., 2022) also recorded no changes in the expression of immune-related genes interleukin-8 (IL-8) and NF-κB p65 in the intestine of hybrid snakehead larvae (Channa maculata × Channa argus) exposed to virgin fluorescent polystyrene microplastics with 0.5-µm and 5-µm diameters at concentrations of 0.2 mg/L, 2 mg/L, and 20 mg/L for 48 hours.
To some extent, the reported results are consistent with our findings. Although our investigated transcript expressions showed relatively high inter-individual variations, the obtained data are still sufficient to demonstrate significant changes in transcripts encoding humoral immune-related molecules in response to single or combined GBH exposures. Such variation may be related to the gender-specific and molting status-specific differences in responses to harm in crustaceans (Liu et al., 2004; de Melo et al., 2020). The C-type lectin 4 (CTL4) and anti-lipopolysaccharide factor 3 (ALF3) genes, encoding probably an effector molecule and antimicrobial peptide (AMP) in L. vannamei, were significantly downregulated in the Lo-GBH and Hi-GBH groups, respectively, whereas the PEN3 gene, encoding AMP, was significantly upregulated in L. vannamei exposed to GBH alone or in combination. These results suggest that bioavailable GBH may cross the intestinal membrane and is distributed to other tissues with effects on humoral immunity in the hepatopancreas of L. vannamei. Unlike ALF3 and CTL4, which mostly could be detected in hepatopancreas and hemocytes (Li et al., 2015; Yin et al., 2023), PEN3 is mainly expressed in hemocytes, but rarely in the hepatopancreas of shrimp (Muñoz et al., 2002).
As we investigated the expressions of ALF3, CTL4, and PEN3 in hepatopancreas only, it is possible that the significant upregulation of PEN3 in hepatopancreas across GBH exposure groups could originate from hemocyte infiltration and accumulation in this organ, corresponding to histological observation of increased hemocyte presence in GBH-treated shrimp. However, not all shrimp samples from the GBH-treated group showed an obvious increase in hemocyte infiltration, possibly as a consequence of histology sampling and processing. Another possibility is that such upregulation of PEN3 may be a direct response of the hepatopancreatic cells to GBH exposure. Therefore, putative sources of PEN3 gene expression remain unknown and could be further investigated. Interestingly, statistically significant differences in expressions of ALF3 between the MP+Hi-GBH and Hi-GBH groups as well as of PEN3 between the MP+Lo-GBH and Lo-GBH groups were revealed, implying that aged PE microplastics may modify the immunotoxicity of GBH.
Moreover, the expression patterns of the other immune-related molecules profiles (Toll1, IMD, and STAT) appeared to exhibit no significant alterations and generally do not follow similar expression patterns to ALF3, CTL4, or PEN3. The non-uniform pattern of differential expressions between each of the genes of interest is therefore more likely due to the crosstalk of different signaling pathways in a coordinated manner to fine-tune an effective humoral immune response. For instance, the expression pattern of ALF3 inversely compared to that of CTL4 and PEN3 across GBH treatment groups in our study was in accordance with a study by Liu et al. (2016), who demonstrated that there was significantly increased expression of ALF, while the expression of PEN3 was significantly decreased in hemocytes of L. vannamei following Vibrio anguillarum infection. Furthermore, RNAi silencing of Toll, which encodes a receptor in the Toll pathway, resulted in a pronounced increase of transcripts involved in the IMD pathway in L. vannamei, and vice versa (Liu et al., 2016). Guanzon and Maningas (2018) also showed that Toll3 in Toll pathway links with the regulation of another pathway (IRF Vago-JAK/STAT), suggesting that humoral immune-mediated pathways may be inter-dependent and implicate a variety of signal-transduction pathways in L. vannamei that are not fully understood.
The crustacean humoral innate immune response, mainly mediated by Toll, immune deficiency (IMD), and JAK/STAT pathways, is triggered by binding of pattern recognition receptors (PRRs) with pathogen-associated molecular patterns (PAMPs), damage-associated molecular patterns (DAMPs), or cytokine-like molecules, which elicit the expression of gene-encoded defense molecules, such as receptors, transcriptional factors, AMPs, or effectors, to protect the shrimp from an exogenous or endogenous threat (Yan et al., 2022). Nonetheless, with insignificant changes in Casp2 and hsp70 expressions, as well as no observed histological alteration linked to cellular death in the hepatopancreas, the significant alterations in the expression of ALF3, CTL4, and PEN3 in GBH treatment groups may be modulated with a mechanism following a different trigger than DAMP release from injured cells.
Plausible alternatives to DAMP release include microbial dysbiosis or disruptions of neuroendocrine–immune (NEI) regulation due to the mechanism of action and toxicity of GBH per se. Given that glyphosate is a derivative of glycine and is similar to glutamate structure, there is a potential for it to act as a neurotransmitter, and exposure to glyphosate may cause interference in glycine-mediated signaling and glutamate modulation (Myers et al., 2016). A recent study (Wang et al., 2019) also elucidated that the neurotransmitters glycine and glutamate may be involved in the NEI regulation network in L. vannamei. Apart from being an analog of glycine, the mechanism of action of glyphosate in GBH is to inhibit the enzyme 5-enolpyruvylshikimate-3-phosphate synthase (EPSPS) in the shikimic acid pathway of undesired plants, which is also present and is inhibited in microbiota, leading to microbial dysbiosis in the GBH-affected host.
A recent study on Chinese mitten crab (E. sinensis) investigated the diversity of microbial communities in the intestines of the crab exposed to glyphosate solution at half of the LC50 concentration for 96 hours, depicting that glyphosate may perturb gut microbial diversity involving the immune regulation and negatively affect the survival rate of E. sinensis (Yang et al., 2019). Studies of the gut and whole body of honey bees (Apis mellifera) exposed to glyphosate solutions showed a decrease in abundance of gut microbiota, possibly related to downregulation of the AMP-related genes, i.e., apidaecin, defensin, and hymenoptaecin (Motta et al., 2022). Similarly, findings in Mediterranean mussel (Mytilus galloprovincialis) suggest that glyphosate and its metabolite, AMPA, may be involved in changes in the transcription of immune-related genes by disturbance of host microbiota communities (Iori et al., 2020). Hence, we believe that alterations in some immune-related gene expressions in shrimp exposed to GBH, either alone or in combination, may be the consequences of microbial dysbiosis or dysregulation of the NEI system rather than DAMPs released from damaged cells that trigger immune pathways.
5 Conclusion
This study explored the toxicity of aged PE microplastics and GBH, either alone or in combination, orally exposed to shrimp L. vannamei and provided considerations for the potential of aged PE microplastics to modify the toxicity of GBH. We found evidence that 96 hours of exposure to an environmental concentration of aged PE microplastics through feed slightly affected the oxidant/antioxidant balance, immune processes, and hepatopancreas histology of shrimp. GBH alone can alter AChE, some parameters of antioxidant status and immunity, and the proportion of hepatopancreatic cells. The combined exposure of GBH and microplastics is likely to alter the toxicity of GBH in some endpoints; however, the mechanism and pattern of such modification are still unclear. Therefore, this study indicates the need for further research into the long-term toxicology mechanisms to broadly capture the risk related to microplastics and GBH on shrimp.
Data availability statement
The raw data supporting the conclusions of this article will be made available by the authors, without undue reservation.
Ethics statement
The animal study was approved by Regierung von Oberbayern ROB-55.2-2532.Vet_03-22-2. The study was conducted in accordance with the local legislation and institutional requirements.
Author contributions
WT: Conceptualization, Data curation, Formal analysis, Investigation, Methodology, Software, Validation, Visualization, Writing – original draft, Writing – review & editing. PC: Investigation, Methodology, Validation, Writing – review & editing. TK: Investigation, Writing – review & editing. DP: Conceptualization, Project administration, Resources, Supervision, Writing – review & editing.
Funding
The author(s) declare that no financial support was received for the research, authorship, and/or publication of this article.
Acknowledgments
The authors are grateful to Crusta Nova GmbH for providing the Pacific white shrimp (L. vannamei) for this study. The research was supported by a doctoral scholarship from the Anandamahidol Foundation and by the Ministry of Education, Youth and Sports of the Czech Republic, project “CENAKVA” (LM2023038).
Conflict of interest
The authors declare that the research was conducted in the absence of any commercial or financial relationships that could be construed as a potential conflict of interest.
Publisher’s note
All claims expressed in this article are solely those of the authors and do not necessarily represent those of their affiliated organizations, or those of the publisher, the editors and the reviewers. Any product that may be evaluated in this article, or claim that may be made by its manufacturer, is not guaranteed or endorsed by the publisher.
Supplementary material
The Supplementary Material for this article can be found online at: https://www.frontiersin.org/articles/10.3389/fmars.2024.1384487/full#supplementary-material
References
Beijer N. R., Dehaut A., Carlier M. P., Wolter H., Versteegen R. M., Pennings J. L., et al. (2022). Relationship between particle properties and immunotoxicological effects of environmentally-sourced microplastics. Front. Water 4, 866732. doi: 10.3389/frwa.2022.866732
Cervellione F., Mcgurk C., Silva P., Owen M., Van Den Broeck W. (2017). Optimization of fixation methods for image analysis of the hepatopancreas in whiteleg shrimp, penaeus vannamei (Boone). J. Fish Dis. 40, 517–527. doi: 10.1111/jfd.12531
Collins P. (2010). Environmental stress upon hepatopancreatic cells of freshwater prawns (Decapoda: Caridea) from the floodplain of paraná River. Natural Sci. 2, 748–759. doi: 10.4236/ns.2010.27094
Cózar A., Echevarría F., González-Gordillo J. I., Irigoien X., Úbeda B., Hernández-León S., et al. (2014). Plastic debris in the open ocean. Proc. Natl. Acad. Sci. 111, 10239–10244. doi: 10.1073/pnas.1314705111
Da Costa J. P., Nunes A. R., Santos P. S. M., Girão A. V., Duarte A. C., Rocha-Santos T. (2018). Degradation of polyethylene microplastics in seawater: insights into the environmental degradation of polymers. J. Environ. Sci. Health Tox Hazard Subst Environ. Eng. 53, 866–875. doi: 10.1080/10934529.2018.1455381
de Melo M. S., Nazari E. M., Müller Y. M. R., Gismondi E. (2020). Modulation of antioxidant gene expressions by roundup® Exposure in the decapod macrobrachium potiuna. Ecotoxicology Environ. Saf. 190, 110086. doi: 10.1016/j.ecoenv.2019.110086
Dolar A., Selonen S., Van Gestel C. A. M., Perc V., Drobne D., Jemec Kokalj A. (2021). Microplastics, chlorpyrifos and their mixtures modulate immune processes in the terrestrial crustacean porcellio scaber. Sci. Total Environ. 772, 144900. doi: 10.1016/j.scitotenv.2020.144900
Fei F., Gao X., Wang X., Liu Y., Bin H., Liu B. (2020). Effect of spectral composition on growth, oxidative stress responses, and apoptosis-related gene expression of the shrimp, penaeus vannamei. Aquaculture Rep. 16, 100267. doi: 10.1016/j.aqrep.2019.100267
Food and Agriculture Organization of the United Nations (2017). Aquaculture Feed And Fertilizer Resources Information System (Rome: Food And Agriculture Organization).
Food and Agriculture Organization of the United Nations (2023) Information And Analysis On Markets And Trade Of Fisheries And Aquaculture Products. Available online at: https://www.fao.org/in-action/globefish/news-events/trade-and-market-news/q1–2023-jan-mar/en/ (Accessed 27/05/2023).
Glusczak L., Dos Santos Miron D., Crestani M., Braga Da Fonseca M., Araújo Pedron F. D., Duarte M. F., et al. (2006). Effect of glyphosate herbicide on acetylcholinesterase activity and metabolic and hematological parameters in Piava (Leporinus obtusidens). Ecotoxicology Environ. Saf. 65, 237–241. doi: 10.1016/j.ecoenv.2005.07.017
Gu H., Wei S., Hu M., Wei H., Wang X., Shang Y., et al. (2020). Microplastics aggravate the adverse effects of BDE-47 on physiological and defense performance in mussels. J. Hazardous Materials 398, 122909. doi: 10.1016/j.jhazmat.2020.122909
Guanzon D. A. V., Maningas M. B. B. (2018). Functional elucidation of LvToll 3 receptor from P. Vannamei through Rna interference and its potential role in the shrimp antiviral response. Dev. Comp. Immunol. 84, 172–180. doi: 10.1016/j.dci.2018.01.020
Hao Y., Chen H., Xu W., Gao J., Yang Y., Zhang Y., et al. (2019). Roundup® Confers cytotoxicity through DNA damage and mitochondria-associated apoptosis induction. Environ. pollut. 252, 917–923. doi: 10.1016/j.envpol.2019.05.128
Hong Y., Yang X., Huang Y., Yan G., Cheng Y. (2018). Assessment of the oxidative and genotoxic effects of the glyphosate-based herbicide roundup on the freshwater shrimp, macrobrachium nipponensis. Chemosphere 210, 896–906. doi: 10.1016/j.chemosphere.2018.07.069
Hou J., Lei Z., Cui L., Hou Y., Yang L., An R., et al. (2021). Polystyrene microplastics lead to pyroptosis and apoptosis of ovarian granulosa cells via NLRP3/caspase-1 signaling pathway in rats. Ecotoxicology Environ. Saf. 212, 112012. doi: 10.1016/j.ecoenv.2021.112012
Huang J., Yang Y., Wang A. (2010). Reconsideration of phenoloxidase activity determination in white shrimp litopenaeus vannamei. Fish Shellfish Immunol. 28, 240–244. doi: 10.1016/j.fsi.2009.10.010
Iori S., Rovere G. D., Ezzat L., Smits M., Ferraresso S. S., Babbucci M., et al. (2020). The effects of glyphosate and ampa on the mediterranean mussel mytilus galloprovincialis and its microbiota. Environ. Res. 182, 108984. doi: 10.1016/j.envres.2019.108984
Kulkarni A., Krishnan S., Anand D., Kokkattunivarthil Uthaman S., Otta S. K., Karunasagar I., et al. (2021). Immune responses and immunoprotection in crustaceans with special reference to shrimp. Rev. Aquaculture 13, 431–459. doi: 10.1111/raq.12482
Lanzarin G. A. B., Félix L. M., Santos D., Venâncio C. A. S., Monteiro S. M. (2019). Dose-dependent effects of A glyphosate commercial formulation – roundup® Ultramax - on the early zebrafish embryogenesis. Chemosphere 223, 514–522. doi: 10.1016/j.chemosphere.2019.02.071
Li H., Chen Y., Li M., Wang S., Zuo H., Xu X., et al. (2015). A C-type lectin (LvCTL4) from litopenaeus vannamei is A downstream molecule of the NF-κb signaling pathway and participates in antibacterial immune response. Fish Shellfish Immunol. 43, 257–263. doi: 10.1016/j.fsi.2014.12.024
Liu Y., Song L., Sun Y., Liu T., Hou F., Liu X. (2016). Comparison of immune response in pacific white shrimp, litopenaeus vannamei, after knock down of toll and IMD gene in vivo. Dev. Comp. Immunol. 60, 41–52. doi: 10.1016/j.dci.2016.02.004
Liu C.-H., Yeh S.-T., Cheng S.-Y., Chen J.-C. (2004). The immune response of the white shrimp litopenaeus vannamei and its susceptibility to vibrio infection in relation with the moult cycle. Fish Shellfish Immunol. 16, 151–161. doi: 10.1016/S1050-4648(03)00058-5
Livak K. J., Schmittgen T. D. (2001). Analysis of relative gene expression data using real-time quantitative Pcr and the 2– Δδct method. Methods 25, 402–408. doi: 10.1006/meth.2001.1262
Lopes F. M., Caldas S. S., Primel E. G., Da Rosa C. E. (2017). Glyphosate adversely affects danio rerio males: acetylcholinesterase modulation and oxidative stress. Zebrafish 14, 97–105. doi: 10.1089/zeb.2016.1341
Magni S., Della Torre C., Garrone G., D’amato A., Parenti C. C., Binelli A. (2019). First evidence of protein modulation by polystyrene microplastics in A freshwater biological model. Environ. pollut. 250, 407–415. doi: 10.1016/j.envpol.2019.04.088
Menéndez-Pedriza A., Jaumot J. (2020). Interaction of environmental pollutants with microplastics: A critical review of sorption factors, bioaccumulation and ecotoxicological effects. Toxics 8, 40. doi: 10.3390/toxics8020040
Mensah P. K., Muller W. J., Palmer C. G. (2012). Acetylcholinesterase activity in the freshwater shrimp caridina nilotica as A biomarker of roundup® Herbicide pollution of freshwater systems in South Africa. Water Sci. Technol. 66, 402–408. doi: 10.2166/wst.2012.206
Mercurio P., Flores F., Mueller J. F., Carter S., Negri A. P. (2014). Glyphosate persistence in seawater. Mar. pollut. Bull. 85, 385–390. doi: 10.1016/j.marpolbul.2014.01.021
Mohamed Nor N. H., Koelmans A. A. (2019). Transfer of pcbs from microplastics under simulated gut fluid conditions is biphasic and reversible. Environ. Sci. Technol. 53, 1874–1883. doi: 10.1021/acs.est.8b05143
Motta E. V. S., Powell J. E., Moran N. A. (2022). Glyphosate induces immune dysregulation in honey bees. Anim. Microbiome 4, 16. doi: 10.1186/s42523-022-00165-0
Muñoz M., Vandenbulcke F., Saulnier D., Bachère E. (2002). Expression and distribution of penaeidin antimicrobial peptides are regulated by haemocyte reactions in microbial challenged shrimp. Eur. J. Biochem. 269, 2678–2689. doi: 10.1046/j.1432-1033.2002.02934.x
Myers J. P., Antoniou M. N., Blumberg B., Carroll L., Colborn T., Everett L. G., et al. (2016). Concerns over use of glyphosate-based herbicides and risks associated with exposures: A consensus statement. Environ. Health 15, 19. doi: 10.1186/s12940-016-0117-0
Niemcharoen S., Haetrakul T., Palić D., Chansue N. (2022). Microplastic-contaminated feed interferes with antioxidant enzyme and lysozyme gene expression of pacific white shrimp (Litopenaeus vannamei) leading to hepatopancreas damage and increased mortality. Animals 12, 3308. doi: 10.3390/ani12233308
Petriella L. S.-A. (2007). Functional morphology of the hepatopancreas of palaemonetes argentinus (Crustacea: decapoda): influence of environmental pollution. Rev. Biología Trop. 55, 79–86. doi: 10.15517/rbt.v55i0.5808
Ranjan A., Boyd C. (2018). Appraising pond liners for shrimp culture. Available online at: https://www.globalseafood.org/advocate/appraising-pond-liners-shrimp-culture/ (Accessed 27/05/2023).
Redza-Dutordoir M., Averill-Bates D. A. (2016). Activation of apoptosis signalling pathways by reactive oxygen species. Biochim. Et Biophys. Acta (Bba) - Mol. Cell Res. 1863, 2977–2992. doi: 10.1016/j.bbamcr.2016.09.012
Reunura T., Prommi T. O. (2022). Detection of microplastics in litopenaeus vannamei (Penaeidae) and macrobrachium rosenbergii (Palaemonidae) in cultured pond. Peerj 10, E12916. doi: 10.7717/peerj.12916
Revel M., Yakovenko N., Caley T., Guillet C., Châtel A., Mouneyrac C. (2020). Accumulation and immunotoxicity of microplastics in the estuarine worm hediste diversicolor in environmentally relevant conditions of exposure. Environ. Sci. pollut. Res. 27, 3574–3583. doi: 10.1007/s11356-018-3497-6
Song H. L., Tan B. P., Chi S. Y., Liu Y., Chowdhury M. K., Dong X. H. (2017). The effects of A dietary protease-complex on performance, digestive and immune enzyme activity, and disease resistance of litopenaeus vannamei fed high plant protein diets. Aquaculture Res. 48, 2550–2560. doi: 10.1111/are.13091
Sreeram M. P., Menon N. (2005). Histopathological changes in the hepatopancreas of the penaeid shrimp metapenaeus dobsoni exposed to petroleum hydrocarbons. J. Mar. Biol. Assoc. India 47, 160–168.
Suman T. Y., Jia P.-P., Li W.-G., Junaid M., Xin G.-Y., Wang Y., et al. (2020). Acute and chronic effects of polystyrene microplastics on brine shrimp: first evidence highlighting the molecular mechanism through transcriptome analysis. J. Hazardous Materials 400, 123220. doi: 10.1016/j.jhazmat.2020.123220
Székács A., Darvas B. (2018). Re-registration challenges of glyphosate in the european union. Front. Environ. Sci. 6, 78. doi: 10.3389/fenvs.2018.00078
Tang Y., Rong J., Guan X., Zha S., Shi W., Han Y., et al. (2020). Immunotoxicity of microplastics and two persistent organic pollutants alone or in combination to A bivalve species. Environ. pollut. 258, 113845. doi: 10.1016/j.envpol.2019.113845
Thammatorn W., Palić D. (2022). Potential risks of microplastic fomites to aquatic organisms with special emphasis on polyethylene-microplastic-glyphosate exposure case in aquacultured shrimp. Appl. Sci. 12, 5135. doi: 10.3390/app12105135
Then C. (2013). High levels of residues from spraying with glyphosate found in soybeans in Argentina. Testbiotech Background Rep. 22, 1–14.
Tu C., Chen T., Zhou Q., Liu Y., Wei J., Waniek J. J., et al. (2020). Biofilm formation and its influences on the properties of microplastics as affected by exposure time and depth in the seawater. Sci. Total Environ. 734, 139237. doi: 10.1016/j.scitotenv.2020.139237
Tu H. T., Silvestre F., Bernard A., Douny C., Phuong N. T., Tao C. T., et al. (2008). Oxidative stress response of black tiger shrimp (Penaeus monodon) to enrofloxacin and to culture system. Aquaculture 285, 244–248. doi: 10.1016/j.aquaculture.2008.08.032
Tu H. T., Silvestre F., Scippo M.-L., Thome J.-P., Phuong N. T., Kestemont P. (2009). Acetylcholinesterase activity as A biomarker of exposure to antibiotics and pesticides in the black tiger shrimp (Penaeus monodon). Ecotoxicology Environ. Saf. 72, 1463–1470. doi: 10.1016/j.ecoenv.2009.04.008
Vianello A., Boldrin A., Guerriero P., Moschino V., Rella R., Sturaro A., et al. (2013). Microplastic particles in sediments of lagoon of venice, Italy: first observations on occurrence, spatial patterns and identification. Estuarine Coast. And Shelf Sci. 130, 54–61. doi: 10.1016/j.ecss.2013.03.022
Wang Y., Branicky R., Noë A., Hekimi S. (2018). Superoxide dismutases: dual roles in controlling ros damage and regulating Ros signaling. J. Cell Biol. 217, 1915–1928. doi: 10.1083/jcb.201708007
Wang J., Guo X., Xue J. (2021). Biofilm-developed microplastics as vectors of pollutants in aquatic environments. Environ. Sci. Technol. 55, 12780–12790. doi: 10.1021/acs.est.1c04466
Wang F., Li S., Xiang J., Li F. (2019). Transcriptome analysis reveals the activation of neuroendocrine-immune system in shrimp hemocytes at the early stage of Wssv infection. BMC Genomics 20, 247. doi: 10.1186/s12864-019-5614-4
Wilson B. W., Henderson J. D. (2007). Determination of cholinesterase in blood and tissue. Curr. Protoc. Toxicol. 34, 12.13. doi: 10.1002/0471140856.tx1213s34
Yan P., Lin C., He M., Zhang Z., Zhao Q., Li E. (2022). Immune regulation mediated by Jak/Stat signaling pathway in hemocytes of pacific white shrimps, litopenaeus vannamei stimulated by lipopolysaccharide. Fish Shellfish Immunol. 130, 141–154. doi: 10.1016/j.fsi.2022.07.048
Yang X., Song Y., Zhang C., Pang Y., Song X., Wu M., et al. (2019). Effects of the glyphosate-based herbicide roundup on the survival, immune response, digestive activities and gut microbiota of the chinese mitten crab, eriocheir sinensis. Aquat. Toxicol. 214, 105243. doi: 10.1016/j.aquatox.2019.105243
Yin C., Shen X., Wang Y., Hu J., Bao Z., Wang M. (2023). Comparative study of five anti-lipopolysaccharide factor genes in litopenaeus vannamei. Dev. Comp. Immunol. 139, 104557. doi: 10.1016/j.dci.2022.104557
Keywords: aged microplastics, glyphosate, combined toxicity, immune response, oxidative stress
Citation: Thammatorn W, Cholewińska P, Kruangkum T and Palić D (2024) Aged polyethylene microplastics and glyphosate-based herbicide co-exposure toxicity in Pacific white shrimp (Litopenaeus vannamei). Front. Mar. Sci. 11:1384487. doi: 10.3389/fmars.2024.1384487
Received: 09 February 2024; Accepted: 15 May 2024;
Published: 07 June 2024.
Edited by:
Monique Mancuso, National Research Council (CNR), ItalyReviewed by:
Carola Murano, Anton Dohrn Zoological Station Naples, ItalyYafei Duan, South China Sea Fisheries Research Institute, China
Copyright © 2024 Thammatorn, Cholewińska, Kruangkum and Palić. This is an open-access article distributed under the terms of the Creative Commons Attribution License (CC BY). The use, distribution or reproduction in other forums is permitted, provided the original author(s) and the copyright owner(s) are credited and that the original publication in this journal is cited, in accordance with accepted academic practice. No use, distribution or reproduction is permitted which does not comply with these terms.
*Correspondence: Dušan Palić, ZC5wYWxpY0BsbXUuZGU=