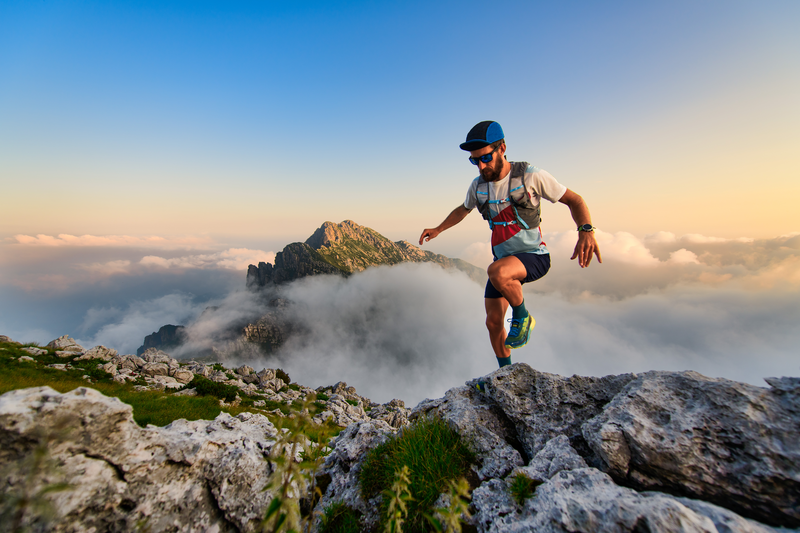
94% of researchers rate our articles as excellent or good
Learn more about the work of our research integrity team to safeguard the quality of each article we publish.
Find out more
ORIGINAL RESEARCH article
Front. Mar. Sci. , 22 April 2024
Sec. Marine Biotechnology and Bioproducts
Volume 11 - 2024 | https://doi.org/10.3389/fmars.2024.1384136
This article is part of the Research Topic Marine Microbial Ecology: Insights from Bioactive Compounds and Bioinformatics View all 4 articles
Harvested Laminaria hyperborea contributes to approximately 3.3 million tonnes of epiphytes discarded as waste despite containing organisms such as red algae and bryozoa, known to produce a range of high-value and bioactive compounds. Our research aim is to valorize epiphyte biomass by exploring the cytotoxic and antimicrobial potential in various extracts and optimized fractionation by flash chromatography. All extracts and fractions were tested for cytotoxicity towards cell lines derived from Acute Myeloid Leukemia (AML), prostate cancer (PC-3), and breast cancer (MCF-7), as well as non-tumorigenic cell lines from the kidney and heart using metabolic activity as a read-out. Antimicrobial activity was assessed using microplate based Alamar Blue assay and minimum inhibitory concentration (MIC) to determine the MIC against various human pathogens. Six extracts demonstrated significant cytotoxicity across all five cell lines (EC50 values below 46 µg/mL), while hexane extract selectively targeted AML cells with EC50 of 1.1 µg/mL. The hexane and ethyl acetate extracts displayed antimycobacterial and antifungal activity (MIC 100 µg/mL). A multistep flash fractionation of the methanolic extract revealed several cytotoxic and antimicrobial activities. The flash fractions displayed a selective activity pattern depending on the elution solvent. Nine fractions were cytotoxic towards all cell lines (EC50 below 72 µg/mL), and four were highly cytotoxic (EC50 below 5.4 µg/mL). Two non-polar fractions were selective for the AML cell line. These results highlight the potential for discovering anticancer and antimicrobial compounds in epiphytes from Laminaria, necessitating further research on their active compounds. Overall, this work underscores the environmental and scientific value of converting marine by-products into a valuable resource, aligning with sustainability and ethical goals.
Throughout human history, natural products (NPs) have been pivotal in treating various diseases and serving as the foundation for drug development. Newman and Cragg’s 2020 study demonstrated that two-thirds of all new small drugs approved by the US FDA from 1981 to 2019 were derived from natural sources (Newman and Cragg, 2020). This underscores the enduring importance of natural products as a rich source of novel compounds in drug discovery. In recent years the interest in NPs from marine environment has increased, partly driven by the blue bioeconomy and the need for the discovery of new sustainable bioresources. However, the vast biodiversity of the less researched marine ecosystems are believed to harbor unique bioactive compounds due to the complex ecological pressures of a harsh and competitive environment (Hay, 1996; Hay and Fenical, 1996; Fogg, 2001); (Kiuru et al., 2014; Liang et al., 2019; Carroll et al., 2023). Every year, new marine molecules with therapeutic potential are discovered (Alves et al., 2018). These discoveries are not only promising for pharmaceutical applications but also pave the way for innovative cosmeceuticals (Pereira, 2018a), nutraceuticals, and functional foods (Plaza et al., 2008; Pereira, 2018b; Ghosh et al., 2022).
While the potential of marine resources for drug discovery is significant, only a few examples have reached the industrial sector (Murray et al., 2013). Traditional bioprospecting methods, which rely on the collection of organisms, present their own set of challenges. These include inconsistent or insufficient yields, which in turn affect the availability of bioactive compounds. This scarcity further hinders chemical characterization and biological screening. In addition to these technical challenges, ethical concerns have emerged regarding the direct harvesting of marine organisms. The absence of regulation and the prevalence of unsustainable practices pose a significant threat to marine ecosystems, disrupting biodiversity and the overall ecological balance. Furthermore, discussions concerning the intellectual property rights over marine resources has become increasingly significant. These issues are relevant not only in the context of fair share of resources and benefits (Atanasov et al., 2021), but also in relation to sample collection in international waters, where a legal framework for the protection of marine life was only recently established (Kachelriess, 2023).
Mariculture has emerged as a promising solution to address bioresource challenges and promote sustainable practices. The escalating interest in harvesting and cultivating algae for food production and other valuable commodities has led to a global production volume of 36 million tonnes (wet weight) of algae in 2020 (The State of World Fisheries and Aquaculture 2022, 2022). Norway has a particular tradition of harvesting and using seaweed (Rudovica et al., 2021), being one of the first countries to enter the seaweed market and explore a coastline of about 100,000 kilometers and an estimated 60 million tonnes of standing kelp biomass (Huse and Bakketeig, 2018). The kelp industry primarily concentrates on extracting alginate and producing agricultural products. Annually, around 150,000 wet tonnes of Laminaria hyperborea are harvested from regulated seaweed fields (World Bank, 2023). This regulated harvesting of Laminaria hyperborea ensures a sustainable and reliable biomass source, consistently supplying valuable natural products for industrial applications and pharmaceutical research.
The epiphytic biomass associated with Laminaria hyperborea represents a distinct macro- and micro-environment containing different red algae and invertebrates, mainly bryozoa and hydrozoa, potentially harboring novel compounds with pharmaceutical potential (Rocha et al., 2011; Tian et al., 2018; Figuerola and Avila, 2019; D’Ambra and Lauritano, 2020; Behera et al., 2022). Despite their prospect, epiphytes are considered byproducts and are typically discarded during seaweed processing. It is estimated that approximately 7,500 tonnes of epiphytic mass are currently disposed of as waste on an annual basis in Norway alone. Previous studies on the epiphytes of Laminaria have primarily focused on their variable colonization under different conditions, such as trawling (Christie et al., 1998), seasons (Christie et al., 2003; Andersen, 2007; Pedersen et al., 2014), wave exposure (Andersen, 2007), farming effluents (Haugland et al., 2021), or canopy light and depth (Whittick, 1983; Vigander, 2007; Pedersen et al., 2014). By investigating the bioactive compounds present in the epiphytic biomass, one can transform this underutilized resource into a valuable asset for marine bioprospecting, offering a zero-waste and sustainable alternative solution adhering to responsible bioprospecting and environmental preservation principles.
In this study, we therefore explore the bioactivity of different extracts of the epiphytic biomass of commercially harvested Laminaria hyperborea’s to evaluate the anti-cancer and anti-microbial potential of its secondary metabolites.
All chemicals used were of analytical grade. Hexane, Chloroform, Ethyl acetate, Acetone, Isopropanol, Methanol (≥99.9%), Ethanol, and DMSO (D5879) were acquired from Sigma-Aldrich Inc. (Sigma-Aldrich, St. Louis, MO, USA). Ultrapure water was either deionized at the University of Bergen or produced by a Millipore Milli-Q Direct 8 water purification system. Hoechst 33342 fluorescent DNA staining reagent was purchased from Thermo Fisher Scientific (Waltham, MA, USA). DMEM (D6429) and RPMI medium (R5886), penicillin/streptomycin (P0781), glutamine (G7513), fetal bovine serum (F7524), formaldehyde, phosphate buffered saline tablets (PBS) were purchased from Sigma-Aldrich (St. Louis, MO, US). WST-1 cell proliferation reagent from Roche Diagnostics GmbH (Mannheim, Germany) and MycoAlert™ was obtained from BioNordika AS (Oslo, Norway).
The epiphyte biomass was acquired from Alginor ASA (Haugesund, Norway). Laminaria and its epiphytes samples were harvested from the coast of Haugesund, Norway (Rogaland field 55E; N 59°11’ E 005°06’) in February 2022. The epiphyte biomass had been collected by the industrial partner through gentle mechanical scraping directly after harvest (within 3 hours) and frozen immediately after separation. After receiving the biomass, the collected epiphyte material was washed thoroughly with water, and the species were identified. Next, the epiphyte mass was immersed in liquid nitrogen, freeze-dried, and milled with a kitchen-type blender. The dried material was stored at −20°C when not used.
The algae and fauna in the epiphytic biomass were carefully examined at macroscopic and microscopic levels using a magnifying glass and a light microscope. The percentage of bryozoan cover on the epiphytic algae was visually estimated. Species identification of the minor constituents of the biomass was challenging due to the preponderance of small, fragmented, or otherwise damaged individuals and the absence of reproductive structures. However, 16 species were found on the stipe of Laminaria hyperborea, dominated by red algae in terms of biomass. Four species, Ptilota gunneri, Palmaria palmata, Membranoptera alata, and Rhodomela lycopodioides, covered by the bryozoa Membranipora membranacea and Electra pilosa, made up approximately 90% of the epiphyte biomass. A list of the present species and their frequency can be found in Supplementary Material Table S1.
Fifteen grams of freeze-dried epiphytic biomass was extracted separately by maceration at room temperature (20°C) with 500mL of each solvent; hexane, chloroform, ethyl acetate, acetone, isopropanol, methanol, aqueous ethanol (70:30 v/v) and deionized water. The extraction was repeated thrice, each for 24 hours, under constant stirring. All the filtrates were collected, filtered again with Whatman No. 1 filter paper and glass wool through a Buchner funnel, and concentrated with a rotary evaporator under reduced pressure at 20–25°C and dried under nitrogen stream or freeze-dried to obtain the crude yields. The resulting pasty extracts were conserved and stored at -20 or 4°C until future use. Stocks of 10 mg/mL were prepared by dissolving the compounds in DMSO (D5879, Sigma-Aldrich) or miliQ water for bioactivity tests.
Fractionation by flash chromatography was performed using a Interchim puriFlash® with a Biotage Sfär C18D column. The fractionation was performed using three solvents, (A) super distilled water, (B) methanol, and (C) ethyl acetate, in a stepwise gradient: 0-6 min: 95% A +5% B, 6-12 min: 75% A + 25% B, 12-18 min: 50% A + 50% B, 18-24 min: 25% A + 75% B, 24-36 min: 0% A + 100% B, 36-42 min: 50% B + 50% C, 42-48 min: 0% B + 100% C. The flow rate was 12 mL/min, and an aliquot of the methanolic extract (1,5 g) was applied. Chromatograms were collected at 220, 280, 330, 450, and 560 nm using a spectral scan between 200 and 800 nm. In total, 24 fractions were collected and regrouped into 21 fractions. To obtain the yields, they were evaporated as described for the crude extracts. The dry fractions were dissolved in DMSO (D5879, Sigma-Aldrich) or miliQ water to a concentration of 10-80 mg/mL and stored at -20°C until further use.
Epiphyte extracts and the flash fractions were tested for their capacity to induce cell death in the human acute myeloid leukemia (AML) cell line MOLM-13 (DSMZ, ACC-554) (Matsuo et al., 1997), the human prostate cancer (PC) cell line PC-3 (ATCC, CRL-1435), and the human breast cancer line MCF-7 (ATCC, HTB-22). They were also tested against the non-cancerous cell lines, normal epithelial rat kidney cell line NRK (ATCC, CRL-6509), and normal rat cardiomyoblast line H9c2 (ATCC, CRL-1446). Further description of the cells is shown in Table 1.
MOLM-13 cells were cultured in RPMI medium, and NRK, H9c2, PC-3, and MCF-7 were cultured in Dulbecco’s modified Eagle’s medium (DMEM), both supplemented with 10% (v/v) fetal bovine serum (FBS), 0.2 mM L-glutamine and 100 IU/mL penicillin and 0.1 mg/L streptomycin. The MOLM-13 cells were suspended and cultured to a density between 10 to 80 × 104 cells/mL and diluted by adding fresh medium. The adherent cells (NRK, H9c2, PC-3, and MCF-7) were cultured until reaching 90% confluence. They were then detached by mild trypsinization, centrifuged at 120 x G for 4 minutes, and reseeded in fresh medium at around 30% confluence. Cells were incubated at 37°C in a humidified atmosphere with 5% CO2. Adherent cells that had undergone more than 14 passages were not used. The cells were tested for mycoplasma infection using MycoAlert™ every two months. No infection was detected during this study.
For cytotoxic testing, MOLM-13 cells were seeded as 20,000 cells/well in 96-well microplates with 100 μL medium/well on the day of the experiment. The adherent cells were seeded the day before the experiment to allow the cells to attach to the substratum. NRK cells were seeded as 1,000 cells/well, H9c2 as 1,500 cells/well, MCF-7 as 2,500-3,000 cells/well, and PC-3 as 2,000 cells/well in 96-well microplates with 100 μL medium/well. The cells were exposed to various concentrations of crude extracts or flash fractions for 72 hours and incubated at the same conditions as described in the section 2.6. The same volume of DMSO was used as solvent control. The cell viability was assessed by WST-1 reagent, following the manufacturer’s instructions. The assay is based on the ability of the mitochondria of the viable cells to convert the WST-1 reagent into a soluble formazan dye, which can be measured spectrophotometrically. This degree of conversion is proportional to the number of viable cells. The plates were further incubated for 2 hours before the signal was recorded at 450 nm with reference at 620 nm using a Wallac EnVision™ _2103 Multilabel reader. The cells were next fixed by adding 100 μL 4% buffered formaldehyde (pH 7.4) containing 0.01 mg/mL of the DNA-specific fluorescent dye, Hoechst 33342 (Polysciences Inc.), and the morphology of the nuclei was visualized by fluorescence microscopy (Nikon Diaphot 300 fitted with a 40× Flu-Phase contrast lens and a DS-Fi3 camera). A minimum of 100 cells from each sample of interest were used to determine cell death microscopically. The combination of WST-1 assay and microscopic evaluation has previously been shown to give reliable results and complement each other, especially if working with colored extracts (Myhren et al., 2014).
Percent growth inhibition of cells exposed to treatments was calculated as follows:
where A is the absorbance of the treated well with the sample; AC is the absorbance of control. Control wells were prepared under the same experimental conditions by adding only the cells under investigation; Ab is the absorbance of the blank medium. In cases where the extracts had color which could interfere with the WST-1 signal, the absorbance of medium and extract was used as blank subtraction (Ab) instead.
Four-parameter non-linear regression analysis was used to calculate EC50 values from the WST-1 data.
To determine the cytotoxic selectivity of the extracts and fractions tested, the selectivity index (SI) was calculated according to the following equation:
where the EC50 is the concentration that reduces the viability of cells to 50% compared to untreated cells.
Mycobacterium tuberculosis (reference strain H37Rv) was streaked onto 7H10+OADC agar plates and incubated at 37 °C. In OADC-enriched liquid 7H9 medium, pure colonies from agar plates were grown to the middle of the log phase. Subsequently, cultures were exponentially grown and inoculated into 7H9 medium on 96-well plates at progressively higher concentrations of the testing chemicals, with approximately 4 × 105 CFU/mL in 200 µL of each well. Before receiving 32.5 µL of a resazurin-tween mixture (8:5 ratio of 0.6 mM Resazurin in 1× PBS to 20% Tween 80), plates were incubated at 37 °C for one week. The production of fluorescent resorufin assists in determining the minimum inhibitory concentration (MIC) of the compounds tested (Krishna et al., 2019; Koçak Aslan et al., 2022; Sarkar et al., 2023).
Utilizing a broth microdilution technique, susceptibility testing was completed in accordance with the Clinical and Laboratories Institute’s (CLSI) suggested procedure. In order to achieve the final target inoculum of 5x105 colony-forming units (CFU)/mL, fresh overnight Staphylococcus aureus colony growth was resuspended in sterile saline, corrected to a 0.5 McFarland turbidity standard using a spectrophotometer, and then diluted 1:200 into Mueller-Hinton Broth. With a 800 µg/mL in the first well of a microtiter plate, two-fold serial dilutions of the compounds were made. They were then inoculated with 100 µL of bacterial inoculum, sealed in a plastic bag to avoid moisture loss, and incubated for 24 hours at 35 °C. Following incubation, bacterial growth on assay plates was observed visually, and the MIC of the test compounds was reported (Schumacher et al., 2018; Özcan et al., 2023).
The samples were tested for their antifungal activity against Candida albicans (Ca, CCUG32723), Candida glabrata (Cg, ATCC15545), Candida tropicalis (Ct, ATCC13803), Candida krusei (Ck, CCUG35869), Candida parapsilosis (Cp, CCUG32995), Trichosporon mucoides (Tm, ATCC204305). In general, a microtiter plate is employed, which includes an RPMI medium, a 2-fold serial dilution of a test drug, and a preset quantity of test organism inoculum. For the preparation of the inoculum, subculture all organisms at 35°C using an antimicrobial-free medium, such as Sabouraud’s dextrose agar, to ensure purity and organism viability. Approximately five colonies at least 1 mm in diameter should be picked and suspended in sterile saline or water, vortexed, and adjusted using a spectrophotometer to a transmittance that equals a 0.5 McFarland standard at a wavelength of 530 nm. This stock solution is used to make a working solution by preparing a 1:100 dilution, followed by a 1:20 dilution, with RPMI 1640 culture medium. The final resulting inoculum in each well should correspond to be approximately 1.5 X 103 cells per mL. After drug plates are inoculated, they are incubated without agitation at 35 °C for 24 hours before reading. The growth control well is inspected for the presence or absence of growth. Plates for which there is insufficient growth in the control well to make an accurate reading are held for 48 hours. The plates were observed for visual growth (turbidity) in the wells, and MIC values were recorded (Berkow et al., 2020; Wiederhold, 2021).
All data were derived from multiple experiments conducted at least in triplicate for crude extracts, always in independent experiments, and duplicate or triplicate for the flash fractions. Data analysis was performed using GraphPad Prism v9 (GraphPad Software Inc., San Diego, CA, USA) and Office Excel 365 (Microsoft, Redmond, WA, USA). To determine differences between the extracts and between the fractions, one-way ANOVA and Tukey’s test with multiple comparisons, p = 0.01, was performed using GraphPad Prism (GraphPad 10.1.0 Software).
The extraction of epiphytic biomass was carried out using a series of eight solvents, ranging in polarity from hexane to water (Table 2). The extraction yields varied across the solvents, with the highest yields observed for the aqueous extracts (18.5% and 19.3%). Conversely, the non-polar hexane yielded the lowest at 0.44%. It was generally noted that the non-polar solvents (hexane, chloroform) and those ranging from weak polar to polar aprotic (ethyl acetate, acetone) resulted in lower yields (< 1%).
The methanol extraction gave the highest extraction yield among the cytotoxic active crudes and was further fractionated. A multistep purification scheme was followed, including a reversed-phase C-18 silica column and stepwise gradient elution with water, methanol, and ethyl acetate. Twenty-four fractions of a volume of 24 mL were initially collected and regrouped into twenty-one fractions based on DAD-HPLC analysis. Fractions 2 and 15 exhibited phase differentiations evident by a distinct coloration and were thus further divided accordingly (a, lower phase and b, upper phase). All fractions were dried, each redissolved in the same volume of DMSO, and analyzed for their cytotoxic and antimicrobial activity.
The crude extracts of the epiphytes (hexane, chloroform, ethyl acetate, acetone, isopropanol, methanol, aqueous-ethanol, and water) were tested for cytotoxic activity towards three cancerous cell lines (MOLM-13 acute myeloid leukemia, MCF-7 breast cancer, and PC-3 prostate cancer) and two non-cancerous cell lines (NRK kidney epithelial cells, and H9c2 cardiomyoblasts) and the viability was assessed by metabolic conversion of the reporter molecule WST-1. The results are summarized in Table 3.
Table 3 Cytotoxic activity, EC50 in µg/mL of epiphyte crude extracts against cancerous and non-tumorigenic cell lines.
From the EC50 values listed in Table 3, it is evident that the chloroform, ethyl acetate, and acetone extracts were the most potent towards all three cancerous cell lines. Of the cell lines tested, the leukemia MOLM-13 line was the most sensitive, and PC-3 was the most resilient toward the cytotoxic activity in the extracts. The chloroform extract was the most active with EC50 values against MOLM-13, MCF-7, and PC-3, respectively of 1.1 ± 0.34, 8.3 ± 0.94, and ≥ 25 μg/mL, following the ethyl acetate with EC50 1.2 ± 0.37, 9.0 ± 1.1, and ≥ 25 μg/mL. No significant difference in activity was observed between chloroform, ethyl acetate, acetone, and isopropanol extracts (Table 3). The methanolic extract was active towards MOLM-13 (EC50 5.6 ± 0.99 μg/mL) but less efficient against breast and prostate cancer cell lines with EC50 23.6 ± 1.79 μg/mL for MCF-7 and 46.2 ± 5.87 for PC-3. The most hydrophobic extract, the hexane, exhibited AML cell selectivity, with very high activity for the MOLM-13 cells (EC50: 1.9 ± 0.80 μg/mL), but it was non-active towards the other cell lines. The aqueous extract showed weak cytotoxicity towards MOLM-13 and none towards MCF7 or PC-3 cell lines.
To verify that the reduced metabolic activity was due to cell death, and not antiproliferative effect, we studied the nuclear morphology of the cells. Indeed, the cells treated with extracts which reduced metabolic activity (i.e. WST-1 signal) had nuclei with typical apoptotic features, such as hypercondensed and fragmented nuclei, and sometimes pyknotic nuclei (Figure 1), in line with what has been observed for other bioactive extracts (Oftedal et al., 2010). The presence of apoptotic cells occurred at the same concentrations where we noted a drop in the metabolic activity (data not shown).
Figure 1 Nuclear morphology of cells treated with various crude extracts. The cells were treated with the given extracts after 24 h of incubation and then fixed and stained with the DNA-dye Hoechst 33342 to visualize the nuclei. Images were acquired by fluorescence microscopy. The arrowheads indicate typical apoptotic nuclei, with hypercondensed chromatin, shrinkage, and in the case of MOLM-13 cells treated with hexane extract, pyknosis. The bars represent 20 µm in all images.
The chloroform, ethyl acetate, and acetone extracts demonstrated high activity towards H9c2 and NRK, while the isopropanol and methanol extracts showed moderate activity. The aqueous, 70% ethanol, and hexane extract showed low or no toxicity against the normal cell line.
The selectivity index (SI, Equation 2) was calculated to reveal which extract contained activity that could be further investigated for anti-cancer purposes. The SI was highest for the MOLM-13 cells for all extracts (Table 4). For the two other cancerous cell lines, the SI indices were lower. The hexane extract exhibited the highest SI for MOLM-13 cells (SI>53) compared with either of the non-cancerous cells. The chloroform, ethyl-acetate, acetone, and methanolic crudes exhibited intermediate selectivity, with SI ranging from 2.2 to 2.8 for NRK and 3.0 to 4.4 for H9c2, respectively.
A total of 21 fractions from the methanol extract were prepared and screened for anticancer activity (Table 5). In the first ten fractions, eluted with a higher water content (95 to 50% water), only fraction 2B exhibited high activity against all tested cell lines, with EC50 values at or below 5 μg/mL (Table 5, Figure 2). Fraction 8 showed moderate but selective activity against MOLM-13 (EC50 > 25 μg/mL). The cytotoxicity of the remaining fractions was significantly higher with increasing proportions of methanol in the gradient, and the fractions eluted with 100% methanol (fractions 11-18) were potent against all tested cell lines (Figure 2). Fraction 15B and fraction 16 exhibited the highest toxicity against leukemia cells, with EC50 values at 0.07 ± 0.02 μg/mL and 0.09 ± 0.02 μg/mL, respectively (Table 5). Fraction 16 was also efficient against MCF-7 and PC-3 cell lines, with EC50 values below 1 μg/mL, while fraction 15B displayed strong toxicity against MCF-7 cells but lower toxicity against PC-3 cells. A change of the solvent gradient to methanol and ethyl acetate (50:50) resulted in active fractions toward all three cancer cell lines (Figure 2). Fraction 23, eluted with 100% ethyl acetate, displayed selective toxicity exclusively toward leukemia cells (EC50 18.2 ± 2.14 μg/mL for MOLM-13 and >100 for the rest cell lines).
Table 5 Cytotoxic activity, EC50 in µg/mL of flash fractions against cancerous and non-tumorigenic cell lines.
Figure 2 Distribution of the cytotoxic activity across the fractionation. EC50 of flash fractions of the methanolic extract towards all three cancer lines MOLM-13, MCF-7, and PC-3 after 72h treatment. Values are presented as logEC50 values, reversed y-axis, mean ± SEM, n=3 or 4 for active fractions and n=2 for inactive fractions, independent experiments.
In line with our observations from the tests on the crude extracts, the fractions from the methanolic extract that were active toward cancer cells were also, to some extent, active toward non-cancerous cells (Table 5). In addition, the fractions containing cytotoxic activity showed the highest SI for MOLM-13 cells compared with non-cancerous cells (Table 6). Fraction 2b, the most polar active fraction, had high activity towards the NRK and H9c2 cell lines. Fractions 15 to 20 were also cytotoxic against the normal cell lines, holding moderate selectivity towards MOLM-13 with a maximum SI of around 3. Fractions 21 to 24 exhibited no detectable cytotoxicity towards either NRK or H9c2 cells, and fraction 23, which was eluted using ethyl acetate, displayed high selectivity towards MOLM-13 compared to non-cancerous cell lines.
The epiphyte extracts under study were evaluated for effects against eight common human microbial pathogens using the Microplate Alamar Blue Assay (MABA) or E-test to define the Minimum inhibitory concentration (MIC). MIC values of the algal extracts against Mycobacterium tuberculosis, Staphylococcus aureus, Candida albicans, Candida glabrata, Candida tropicalis, Candida krusei, Candida parapsilosis, Trichosporon mucoides are presented in Table 7. The most susceptible strain tested was M. tuberculosis, while the most resistant microorganisms assessed were S. aureus, C. albicans, C. tropicalis, C. krusei, and C. parapsilosis, which all were unaffected by the highest concentration tested (MIC values > 200 μg/mL). The hexane, ethyl acetate, chloroform, and aqueous extracts exhibited moderate to good antimycobacterial activity with MIC values ranging between 100 to 200 μg/mL. The hexane extract was the most active against the fungal strains showing activity against C. glabrata and T. mucoides at concentrations of 100 μg/mL and 200 μg/mL, respectively. In addition, the ethyl acetate extract inhibited the T. mucoides with a MIC of 100 μg/mL. At the same time, the ethanol extract, despite being inactive to most of the microorganisms tested, exhibited moderate action against T. mucoides (MIC = 200 μg/mL). The methanol extract showed no inhibitory effects against any of microorganisms tested.
Table 7 Minimum inhibitory concentration (MIC) in µg/mL of the epiphytic extracts. Mt (Mycobacterium tuberculosis), Sa (Staphylococcus aureus), Ca (Candida albicans), Cg (Candida glabrata), Ct (Candida tropicalis), Ck (Candida krusei), Cp (Candida parapsilosis), Tm (Trichosporon mucoides).
The 21 flash fractions were also screened for their antimicrobial activity. The MIC values against the two pathogenic bacteria, M. tuberculosis and S. aureus, and one fungal strain, C. albicans, are presented in Table 8. The fractions eluted with higher water content (95% to 50% A) did not exhibit any antimicrobial activity. The antimicrobial effect was then significantly improved by decreasing the water content of the mobile phase (75% to 100% methanol). The fractions obtained with 100% methanol (fractions 11 to 18) demonstrated potent activity against M. tuberculosis with MIC values ranging from 50 to 200 μg/mL. Fractions 16 and 17 displayed the highest anti-tuberculosis activity (MIC = 50 μg/mL). As the hydrophobicity of the elution solvent was increased by using a mixture of 50% methanol and 50% ethyl acetate, the anti-microbial activity disappeared, and fractions 19-24 had no detectable activity towards either of the microorganisms tested.
Table 8 Minimum inhibitory concentration (MIC) in µg/mL for the flash fractions. Mt (Mycobacterium tuberculosis), Sa (Staphylococcus aureus), Ca (Candida albicans).
Numerous studies have focused on the potential of macroalgae as a source of new anticancer and antimicrobial compounds, with multiple crude extracts screened over the past four decades to discover new compounds (Noda et al., 1990; Harada and Kamei, 1997; Harada et al., 1997; Shoeib et al., 2004; Xu et al., 2004; Zubia et al., 2009; Allmendinger et al., 2010; Spavieri et al., 2010a; Martins et al., 2018, 2010b). However, to the best of our knowledge, there are no reports on the presence of bioactivities in epiphytes from Laminaria hyperborea. As such, the present study represents the first systematic investigation of this epiphytic biomasses’ potential in producing bioactive secondary metabolites with cytotoxic and antimicrobial potential.
We found a high degree of both cytotoxic and antibacterial activity in several of our extracts. In general, despite giving the highest yield (Table 2), the two aqueous extracts had the lowest cytotoxic potential (Table 3). At the same time, no such trend related to the extraction solvent used was found for the antimicrobial activity (Table 7). An explanation for the low activity in our aqueous extracts could be that they mostly contain polysaccharides and proteins - as generally observed for aqueous alga extracts (Galland-Irmouli et al., 1999; Lafarga et al., 2020; Olsson et al., 2020; Castejón et al., 2021). The organic extracts, however, were more potent, in line with other reports (Sastry and Rao, 1994; Val et al., 2001; El-Shaibany et al., 2020). The chloroform and ethyl acetate extracts displayed the highest overall cytotoxicity in cancer and non-tumorigenic cell lines (Table 3) while the hexane and ethyl acetate were most potent towards the sensitive microbial strains (Table 7).
Due to the high activity in the organic extracts, we further fractionated the methanolic extract with a high extraction yield. Methanol has a broad extraction capacity, and by purifying the crude extract with flash chromatography with a decreasing polarity gradient, the activity of this extract could be further investigated. A similar activity pattern as seen for the crude extracts, was observed: the highly polar flash fractions had no significant activity, whereas the organic fractions revealed high cytotoxicity (Figure 2). An exception was fraction 2A, which, despite its high polarity, exhibited potent cytotoxicity against all the cell lines, indicating the presence of highly active polar compounds different from the following fractions (Table 4). An important finding was that some of the purified flash fractions showed an improvement in the cytotoxic potency (EC50 ranging from 0.07 μg/ml) (Table 6), possibly due to increased concentrations of active compound(s), or removal of substances that mask cytotoxic effects.
In the potent fractions (15-17, eluted with methanol), fucoxanthin was identified with DAD-HPLC analysis compared with a commercial standard (data not shown), which could be responsible for the activities (Mumu et al., 2022). Fucoxanthin and its metabolites, fucoxanthinol, amarouciaxanthin (Asai et al., 2004), and isomers such as 13-cis or 13’-cis fucoxanthin (Nakazawa et al., 2009), are found to reduce cell viability when tested on prostate cancer cell lines DU145, PC-3, and LNCaP (Kotake-Nara et al., 2001, 2005; Satomi and Nishino, 2009; Satomi, 2012). However, pure fucoxanthin was tested on the cell lines in our study - but did not produce substantial cytotoxicity (Table S2). This suggests that the observed bioactivity in fractions 15-17 might not be related to the presence of fucoxanthin – even though synergistic effects between fucoxanthin and other bioactive constituents cannot be ruled out. The type of constituents and their relative contributions to the observed activities are yet to be elucidated, but it is likely that the vast array of halogenated terpenoids (Cabrita et al., 2010; Jesus et al., 2019), phenolic compounds (Cotas et al., 2020), mycosporine-like amino acids (Yuan et al., 2009; Athukorala et al., 2016; Lalegerie et al., 2019; Nishida et al., 2020), carotenoids and pigments (Schubert et al., 2006; Lalegerie et al., 2020) found in red algae could be involved. Particularly halogenated compounds such as bromophenols found in this type of extracts have proved to be characteristic compounds in red seaweeds and have diverse biological effects, including antibacterial, antifungal, anti-inflammatory, and cytotoxic effects (Shoeib et al., 2004; Jesus et al., 2019). However, we cannot exclude the presence of bioactive compounds produced by other species like invertebrates present in our samples.
This study aimed partly to investigate whether the epiphytic biomass contained compounds that could be promising leads in cancer treatment or infections. For cancer therapy, a certain selectivity towards cancer cells compared to non-tumorigenic cells is needed, whereas a low MIC must be fulfilled for anti-microbial compounds. For our cytotoxicity data, we calculated the selectivity index (SI) from the difference in EC50 values between the cancer and non-tumorigenic cells. It is proposed that the SI needs to be higher than at least 3 (Mahavorasirikul et al., 2010; Weerapreeyakul et al., 2012) or even 10 (Peña-Morán et al., 2016) for a sample to be eligible for further investigation. In several of our extracts and fractions we found an SI higher than 3 (Table 4 and 6). For instance, the hexane crude exhibited high activity towards MOLM-13 cells exclusively with SI greater than 53 compared to the non-carcinogenic cell lines and merits further investigations to find if there are novel leads for cancer therapy. Although our extracts were tested on a small panel of cells (two non-tumorigenic and three cancer cell lines), the results are promising in light of the high potency and selectivity. As further fractionation and isolation of this biomass progress, tests on more cell lines should be performed to identify their selectivity toward certain cancer types. However, from the data presented herein, it appears that the leukemia cell line MOLM-13 is particularly susceptible, especially in the activities present in the hexane extracts or the most organic flash fractions from the methanolic extract (Tables 3, 4, 5, and 6). The lowest MIC value in our experiments with the crude extracts was 100 μg/mL, which was achieved with the strongest organic solvents (Table 7). However, after fractionation of the methanolic extract, we achieved MIC-values of 50 μg/mL against Mycobacterium tuberculosis (Table 8), again demonstrating the importance of purification to evaluate the activity potential of complex samples.
Overall, this study provides valuable insights into the potential of epiphyte crudes and the subsequential fractionation as a novel source of new anticancer and antimicrobial agents. The observed cytotoxic and antimicrobial activities suggest the presence of compounds with the potential to selectively target cancer cells and inhibit human pathogens. Further research is needed to isolate and identify the specific compounds responsible for these effects and to elucidate the underlying mechanisms of action. Thus, in line with the challenges and ethical concerns of bioprospecting, epiphytes represent a great opportunity for valorization, transforming an abundant waste material into a major opportunity.
The original contributions presented in the study are included in the article/Supplementary Material. Further inquiries can be directed to the corresponding author. Replication data is deposited in UoB Open Research Data (https://doi.org/10.18710/WQIZAX). The deposited data contains information on the cell assays results and analysis.
Ethical approval was not required for the studies on humans in accordance with the local legislation and institutional requirements because only commercially available established cell lines were used.
AB: Conceptualization, Data curation, Formal analysis, Investigation, Methodology, Validation, Visualization, Writing – original draft, Writing – review & editing. LH: Conceptualization, Formal analysis, Funding acquisition, Investigation, Methodology, Project administration, Resources, Supervision, Validation, Visualization, Writing – original draft, Writing – review & editing. SV: Data curation, Formal analysis, Investigation, Methodology, Writing – original draft, Writing – review & editing. HH: Data curation, Formal analysis, Investigation, Writing – original draft, Writing – review & editing. TT: Methodology, Resources, Supervision, Writing – original draft, Writing – review & editing, Formal analysis. GK: Funding acquisition, Resources, Writing – original draft, Writing – review & editing. MJ: Conceptualization, Formal analysis, Funding acquisition, Investigation, Methodology, Project administration, Resources, Supervision, Validation, Visualization, Writing – original draft, Writing – review & editing.
The author(s) declare financial support was received for the research, authorship, and/or publication of this article. This research was funded by the Research Council of Norway (RCN project numbers # 234506, #261669, and #309592) and the European Council JPIAMR (RCN project #298410) to TT. This work was supported by funding from the NordForsk NCoE program NordAqua (project no.: 82845) to LH.
We thank Professor Inga Kjersti Sjøtun (Faculty Mat. Nat., Department of Biological Science) for assistance with species identification and researcher Reidun Æsøy (Centre for Pharmacy, Department of Clinical Science) for her technical support with the cell assay work.
Author GK was employed by company Alginor ASA.
The remaining authors declare that the research was conducted in the absence of any commercial or financial relationships that could be construed as a potential conflict of interest.
All claims expressed in this article are solely those of the authors and do not necessarily represent those of their affiliated organizations, or those of the publisher, the editors and the reviewers. Any product that may be evaluated in this article, or claim that may be made by its manufacturer, is not guaranteed or endorsed by the publisher.
The Supplementary Material for this article can be found online at: https://www.frontiersin.org/articles/10.3389/fmars.2024.1384136/full#supplementary-material
Allmendinger A., Spavieri J., Kaiser M., Casey R., Hingley-Wilson S., Lalvani A., et al. (2010). Antiprotozoal, antimycobacterial and cytotoxic potential of twenty-three British and Irish red algae. Phytother. Res. 24, 1099–1103. doi: 10.1002/ptr.3094
Alves C., Silva J., Pinteus S., Gaspar H., Alpoim M. C., Botana L. M., et al. (2018). From marine origin to therapeutics: the antitumor potential of marine algae-derived compounds. Front. Pharmacol. 9. doi: 10.3389/fphar.2018.00777
Andersen G. S. (2007) Kelp associated floral epiphytes : Productivity and community structure in relation to wave exposure and season. Available online at: https://www.duo.uio.no/handle/10852/11655 (Accessed August 2, 2023).
Asai A., Sugawara T., Ono H., Nagao A. (2004). Biotransformation of fucoxanthinol into amarouciaxanthin a in mice and hepg2 cells: formation and cytotoxicity of fucoxanthin metabolites. Drug Metab. Dispos 32, 205–211. doi: 10.1124/dmd.32.2.205
Atanasov A. G., Zotchev S. B., Dirsch V. M., Orhan I. E., Banach M., Rollinger J. M., et al. (2021). Natural products in drug discovery: advances and opportunities. Nat. Rev. Drug Discovery 20, 200–216. doi: 10.1038/s41573-020-00114-z
Athukorala Y., Trang S., Kwok C., Yuan Y. (2016). Antiproliferative and antioxidant activities and mycosporine-like amino acid profiles of wild-harvested and cultivated edible canadian marine red macroalgae. Molecules 21, 119. doi: 10.3390/molecules21010119
Behera D. P., Ingle K. N., Mathew D. E., Dhimmar A., Sahastrabudhe H., Sahu S. K., et al. (2022). Epiphytism, diseases and grazing in seaweed aquaculture: A comprehensive review. Rev. Aquaculture 14, 1345–1370. doi: 10.1111/raq.12653
Berkow E. L., Lockhart S. R., Ostrosky-Zeichner L. (2020). Antifungal susceptibility testing: current approaches. Clin. Microbiol. Rev. 33, e00069–e00019. doi: 10.1128/CMR.00069-19
Cabrita M. T., Vale C., Rauter A. P. (2010). Halogenated compounds from marine algae. Mar. Drugs 8, 2301–2317. doi: 10.3390/md8082301
Carroll A. R., Copp B. R., Davis R. A., Keyzers R. A., Prinsep M. R. (2023). Marine natural products. Nat. Prod. Rep. 40, 275–325. doi: 10.1039/D2NP00083K
Castejón N., Thorarinsdottir K. A., Einarsdóttir R., Kristbergsson K., Marteinsdóttir G. (2021). Exploring the potential of Icelandic seaweeds extracts produced by aqueous pulsed electric fields-assisted extraction for cosmetic applications. Mar. Drugs 19, 662. doi: 10.3390/md19120662
Christie H., Fredriksen S., Rinde E. (1998). “Regrowth of kelp and colonization of epiphyte and fauna community after kelp trawling at the coast of Norway,” in Recruitment, colonization and physical-chemical forcing in marine biological systems. Eds. Baden S., Phil L., Rosenberg R., Strömberg J.-O., Svane I., Tiselius P. (Springer Netherlands, Dordrecht), 49–58. doi: 10.1007/978-94-017-2864-5_4
Christie H., Jørgensen N. M., Norderhaug K. M., Waage-Nielsen E. (2003). Species distribution and habitat exploitation of fauna associated with kelp ( Laminaria Hyperborea ) along the Norwegian Coast. J. Mar. Biol. Ass. 83, 687–699. doi: 10.1017/S0025315403007653h
Cotas J., Leandro A., Monteiro P., Pacheco D., Figueirinha A., Gonçalves A. M. M., et al. (2020). Seaweed phenolics: from extraction to applications. Mar. Drugs 18, 384. doi: 10.3390/md18080384
D’Ambra I., Lauritano C. (2020). A review of toxins from cnidaria. Mar. Drugs 18, 507. doi: 10.3390/md18100507
El-Shaibany A., AL-Habori M., Al-Maqtari T., Al-Mahbashi H. (2020). The Yemeni brown algae dictyota dichotoma exhibit high in vitro anticancer activity independent of its antioxidant capability. BioMed. Res. Int. 2020, 1–9. doi: 10.1155/2020/2425693
FAO, Organization: Food and Agriculture Organization of the United Nations (2022). The state of world fisheries and aquaculture 2022 (FAO). doi: 10.4060/cc0461en
Figuerola B., Avila C. (2019). The phylum bryozoa as a promising source of anticancer drugs. Mar. Drugs 17, 477. doi: 10.3390/md17080477
Fogg G. E. (2001). “Algal adaptation to stress — Some general remarks,” in Algal adaptation to environmental stresses. Eds. Rai L. C., Gaur J. P. (Springer Berlin Heidelberg, Berlin, Heidelberg), 1–19. doi: 10.1007/978-3-642-59491-5_1
Galland-Irmouli A.-V., Fleurence J., Lamghari R., Luçon M., Rouxel C., Barbaroux O., et al. (1999). Nutritional value of proteins from edible seaweed Palmaria palmata (dulse). J. Nutr. Biochem. 10, 353–359. doi: 10.1016/S0955-2863(99)00014-5
Ghosh S., Sarkar T., Pati S., Kari Z. A., Edinur H. A., Chakraborty R. (2022). Novel bioactive compounds from marine sources as a tool for functional food development. Front. Mar. Sci. 9. doi: 10.3389/fmars.2022.832957
Harada H., Kamei Y. (1997). Selective cytotoxicity of marine algae extracts to several human leukemic cell lines. Cytotechnology 25, 213–219. doi: 10.1023/A:1007987010840
Harada H., Noro T., Kamei Y. (1997). Selective antitumor activity in vitro from marine algae from Japan coasts. Biol. Pharm. Bull. 20, 541–546. doi: 10.1248/bpb.20.541
Haugland B., Armitage C., Kutti T., Husa V., Skogen M., Bekkby T., et al. (2021). Large-scale salmon farming in Norway impacts the epiphytic community of Laminaria hyperborea. Aquaculture Environ. Interact. 13, 81-100. doi: 10.3354/aei00392
Hay M., Fenical W. (1996). Chemical ecology and marine biodiversity: insights and products from the sea. oceanog 9, 10–20. doi: 10.5670/oceanog.1996.21
Hay M. E. (1996). Marine chemical ecology: what’s known and what’s next? J. Exp. Mar. Biol. Ecol. 200, 103–134. doi: 10.1016/S0022-0981(96)02659-7
Huse G., Bakketeig E. (2018). Ressursoversikten (Havforskningsinstituttet, Nordnes, Bergen: Havforskningsinstituttet). Available at: https://www.hi.no/hi/nettrapporter/fisken-og-havet/2018/ressursoversikten_2018_ny_til_web_1.
Jesus A., Correia-da-Silva M., Afonso C., Pinto M., Cidade H. (2019). Isolation and potential biological applications of haloaryl secondary metabolites from macroalgae. Mar. Drugs 17, 73. doi: 10.3390/md17020073
Kachelriess D. (2023). The High Seas Biodiversity Treaty: An Introduction to the Agreement under the United Nations Convention on the Law of the Sea on the conservation and sustainable use of marine biological diversity of areas beyond national jurisdiction (IUCN). Available at: https://www.iucn.org/resources/grey-literature/high-seas-biodiversity-treaty-policy-brief.
Kiuru P., D’Auria M. V., Muller C. D., Tammela P., Vuorela H., Yli-Kauhaluoma J. (2014). Exploring marine resources for bioactive compounds. Planta Med. 80, 1234–1246. doi: 10.1055/s-0034-1383001
Koçak Aslan E., Han M.İ., Krishna V. S., Tamhaev R., Dengiz C., Doğan Ş.D., et al. (2022). Isoniazid linked to sulfonate esters via hydrazone functionality: design, synthesis, and evaluation of antitubercular activity. Pharmaceuticals 15, 1301. doi: 10.3390/ph15101301
Kotake-Nara E., Asai A., Nagao A. (2005). Neoxanthin and fucoxanthin induce apoptosis in PC-3 human prostate cancer cells. Cancer Lett. 220, 75–84. doi: 10.1016/j.canlet.2004.07.048
Kotake-Nara E., Miyashita K., Nagao A., Kushiro M., Zhang H., Sugawara T. (2001). Carotenoids affect proliferation of human prostate cancer cells. J. Nutr. 131, 3303–3306. doi: 10.1093/jn/131.12.3303
Krishna V. S., Zheng S., Rekha E. M., Guddat L. W., Sriram D. (2019). Discovery and evaluation of novel Mycobacterium tuberculosis ketol-acid reductoisomerase inhibitors as therapeutic drug leads. J. Comput. Aided Mol. Des. 33, 357–366. doi: 10.1007/s10822-019-00184-1
Lafarga T., Acién-Fernández F. G., Garcia-Vaquero M. (2020). Bioactive peptides and carbohydrates from seaweed for food applications: Natural occurrence, isolation, purification, and identification. Algal Res. 48, 101909. doi: 10.1016/j.algal.2020.101909
Lalegerie F., Lajili S., Bedoux G., Taupin L., Stiger-Pouvreau V., Connan S. (2019). Photo-protective compounds in red macroalgae from Brittany: Considerable diversity in mycosporine-like amino acids (MAAs). Mar. Environ. Res. 147, 37–48. doi: 10.1016/j.marenvres.2019.04.001
Lalegerie F., Stiger-Pouvreau V., Connan S. (2020). Temporal variation in pigment and mycosporine-like amino acid composition of the red macroalga Palmaria palmata from Brittany (France): hypothesis on the MAA biosynthesis pathway under high irradiance. J. Appl. Phycol 32, 2641–2656. doi: 10.1007/s10811-020-02075-7
Liang X., Luo D., Luesch H. (2019). Advances in exploring the therapeutic potential of marine natural products. Pharmacol. Res. 147, 104373. doi: 10.1016/j.phrs.2019.104373
Mahavorasirikul W., Viyanant V., Chaijaroenkul W., Itharat A., Na-Bangchang K. (2010). Cytotoxic activity of Thai medicinal plants against human cholangiocarcinoma, laryngeal and hepatocarcinoma cells in vitro. BMC Complement Altern. Med. 10, 55. doi: 10.1186/1472-6882-10-55
Martins R. M., Nedel F., Guimarães V. B. S., da Silva A. F., Colepicolo P., de Pereira C. M. P., et al. (2018). Macroalgae extracts from Antarctica have antimicrobial and anticancer potential. Front. Microbiol. 9. doi: 10.3389/fmicb.2018.00412
Matsuo Y., MacLeod R., Uphoff C., Drexler H., Nishizaki C., Katayama Y., et al. (1997). Two acute monocytic leukemia (AML-M5a) cell lines (MOLM-13 and MOLM-14) with interclonal phenotypic heterogeneity showing MLL-AF9 fusion resulting from an occult chromosome insertion, ins(11;9)(q23;p22p23). Leukemia 11, 1469–1477. doi: 10.1038/sj.leu.2400768
Mumu M., Das A., Emran T. B., Mitra S., Islam F., Roy A., et al. (2022). Fucoxanthin: A promising phytochemical on diverse pharmacological targets. Front. Pharmacol. 13. doi: 10.3389/fphar.2022.929442
Murray P. M., Moane S., Collins C., Beletskaya T., Thomas O. P., Duarte A. W. F., et al. (2013). Sustainable production of biologically active molecules of marine based origin. New Biotechnol. 30, 839–850. doi: 10.1016/j.nbt.2013.03.006
Myhren L., Nilssen I. M., Nicolas V., Døskeland S. O., Barratt G., Herfindal L. (2014). Efficacy of multi-functional liposomes containing daunorubicin and emetine for treatment of acute myeloid leukaemia. Eur. J. Pharmaceutics Biopharmaceutics 88, 186–193. doi: 10.1016/j.ejpb.2014.04.002
Nakazawa Y., Sashima T., Hosokawa M., Miyashita K. (2009). Comparative evaluation of growth inhibitory effect of stereoisomers of fucoxanthin in human cancer cell lines. J. Funct. Foods 1, 88–97. doi: 10.1016/j.jff.2008.09.015
Newman D. J., Cragg G. M. (2020). Natural products as sources of new drugs over the nearly four decades from 01/1981 to 09/2019. J. Natural Products 83, 770–803. doi: 10.1021/acs.jnatprod.9b01285
Nishida Y., Kumagai Y., Michiba S., Yasui H., Kishimura H. (2020). Efficient extraction and antioxidant capacity of mycosporine-like amino acids from red alga dulse palmaria palmata in Japan. Mar. Drugs 18, 502. doi: 10.3390/md18100502
Noda H., Amano H., Arashima K., Nisizawa K. (1990). Antitumor activity of marine algae. Hydrobiologia 204, 577–584. doi: 10.1007/BF00040290
Oftedal L., Selheim F., Wahlsten M., Sivonen K., Døskeland S. O., Herfindal L. (2010). Marine Benthic Cyanobacteria Contain Apoptosis-Inducing Activity Synergizing with Daunorubicin to Kill Leukemia Cells, but not Cardiomyocytes. Mar. Drugs 8, 2659–2672. doi: 10.3390/md8102659
Olsson J., Toth G. B., Albers E. (2020). Biochemical composition of red, green and brown seaweeds on the Swedish west coast. J. Appl. Phycol 32, 3305–3317. doi: 10.1007/s10811-020-02145-w
Özcan E., Vagolu S. K., Gündüz M. G., Stevanovic M., Kökbudak Z., Tønjum T., et al. (2023). Novel quinoline-based thiosemicarbazide derivatives: synthesis, DFT calculations, and investigation of antitubercular, antibacterial, and antifungal activities. ACS Omega 8, 40140–40152. doi: 10.1021/acsomega.3c03018
Pedersen M., Nejrup L., Pedersen T., Fredriksen S. (2014). Sub-canopy light conditions only allow low annual net productivity of epiphytic algae on kelp Laminaria hyperborea. Mar. Ecol. Prog. Ser. 516, 163–176. doi: 10.3354/meps11019
Peña-Morán O., Villarreal M., Álvarez-Berber L., Meneses-Acosta A., Rodríguez-López V. (2016). Cytotoxicity, Post-Treatment Recovery, and Selectivity Analysis of Naturally Occurring Podophyllotoxins from Bursera fagaroides var. fagaroides on Breast Cancer Cell Lines. Molecules 21, 1013. doi: 10.3390/molecules21081013
Pereira L. (2018a). Seaweeds as source of bioactive substances and skin care therapy—Cosmeceuticals, algotheraphy, and thalassotherapy. Cosmetics 5, 68. doi: 10.3390/cosmetics5040068
Pereira L. (2018b). Therapeutic and nutritional uses of algae (Boca Raton: CRC Press/Taylor & Francis Group).
Plaza M., Cifuentes A., Ibanez E. (2008). In the search of new functional food ingredients from algae. Trends Food Sci. Technol. 19, 31–39. doi: 10.1016/j.tifs.2007.07.012
Rocha J., Peixe L., Gomes N. C. M., Calado R. (2011). Cnidarians as a source of new marine bioactive compounds—An overview of the last decade and future steps for bioprospecting. Mar. Drugs 9, 1860–1886. doi: 10.3390/md9101860
Rudovica V., Rotter A., Gaudêncio S. P., Novoveská L., Akgül F., Akslen-Hoel L. K., et al. (2021). Valorization of marine waste: use of industrial by-products and beach wrack towards the production of high added-value products. Front. Mar. Sci. 8. doi: 10.3389/fmars.2021.723333
Sarkar S., Mayer Bridwell A. E., Good J. A. D., Wang E. R., McKee S. R., Valenta J., et al. (2023). Design, synthesis, and evaluation of novel Δ2-thiazolino 2-pyridone derivatives that potentiate isoniazid activity in an isoniazid-resistant mycobacterium tuberculosis mutant. J. Med. Chem. 66, 11056–11077. doi: 10.1021/acs.jmedchem.3c00358
Sastry V. M. V. S., Rao G. R. K. (1994). Antibacterial substances from marine algae: successive extraction using benzene, chloroform and methanol. Botanica Marina 37, 357-360. doi: 10.1515/botm.1994.37.4.357
Satomi Y. (2012). Fucoxanthin induces GADD45A expression and G1 arrest with SAPK/JNK activation in LNCap human prostate cancer cells. Anticancer Res. 32, 807–813.
Satomi Y., Nishino H. (2009). Implication of mitogen-activated protein kinase in the induction of G1 cell cycle arrest and gadd45 expression by the carotenoid fucoxanthin in human cancer cells. Biochim. Biophys. Acta 1790, 260–266. doi: 10.1016/j.bbagen.2009.01.003
Schubert N., García-Mendoza E., Pacheco-Ruiz I. (2006). CAROTENOID COMPOSITION OF MARINE RED ALGAE 1. J. Phycology 42, 1208–1216. doi: 10.1111/j.1529-8817.2006.00274.x
Schumacher A., Vranken T., Malhotra A., Arts J. J. C., Habibovic P. (2018). In vitro antimicrobial susceptibility testing methods: agar dilution to 3D tissue-engineered models. Eur. J. Clin. Microbiol. Infect. Dis. 37, 187–208. doi: 10.1007/s10096-017-3089-2
Shoeib N. A., Bibby M. C., Blunden G., Linley P. A., Swaine D. J., Wheelhouse R. T., et al. (2004). In - vitro Cytotoxic Activities of the Major Bromophenols of the Red Alga Polysiphonia l anosa and Some Novel Synthetic Isomers. J. Nat. Prod. 67, 1445–1449. doi: 10.1021/np0305268
Spavieri J., Allmendinger A., Kaiser M., Casey R., Hingley-Wilson S., Lalvani A., et al. (2010a). Antimycobacterial, antiprotozoal and cytotoxic potential of twenty-one brown algae (Phaeophyceae) from British and Irish waters. Phytother. Res. 24, 1724–1729. doi: 10.1002/ptr.3208
Spavieri J., Kaiser M., Casey R., Hingley-Wilson S., Lalvani A., Blunden G., et al. (2010b). Antiprotozoal, antimycobacterial and cytotoxic potential of some british green algae. Phytother. Res. 24, 1095–1098. doi: 10.1002/ptr.3072
Tian X. R., Tang H. F., Tian X. L., Hu J. J., Huang L. L., Gustafson K. R. (2018). Review of bioactive secondary metabolites from marine bryozoans in the progress of new drugs discovery. Future Medicinal Chem. 10, 1497–1514. doi: 10.4155/fmc-2018-0012
Val A., Platas G., Basilio A., Cabello A., Gorrochategui J., Suay I., et al. (2001). Screening of antimicrobial activities in red, green and brown macroalgae from Gran Canaria (Canary Islands, Spain). Int. Microbiol. 4, 35–40. doi: 10.1007/s101230100006
Vigander H. (2007) Epifyttiske alger på Laminaria hyperborea (Gunn.) Foslie kartlagt langs en dybdegradient fra Skipsholmen, mørekysten. Available online at: https://www.duo.uio.no/handle/10852/11650 (Accessed October 9, 2023).
Weerapreeyakul N., Nonpunya A., Barusrux S., Thitimetharoch T., Sripanidkulchai B. (2012). Evaluation of the anticancer potential of six herbs against a hepatoma cell line. Chin. Med. 7, 15. doi: 10.1186/1749-8546-7-15
Whittick A. (1983). Spatial and temporal distributions of dominant epiphytes on the stipes of Laminaria hyperborea (Gunn.) Fosl. (Phaeophyta:Laminariales) in S.E. Scotland. J. Exp. Mar. Biol. Ecol. 73, 1–10. doi: 10.1016/0022-0981(83)90002-3
Wiederhold N. P. (2021). Antifungal susceptibility testing: A primer for clinicians. Open Forum Infect. Dis. 8, ofab444. doi: 10.1093/ofid/ofab444
World Bank (2023). Global seaweed: new and emerging markets report (Washington, DC: World Bank Publications). doi: 10.1596/40187
Xu N., Fan X., Yan X., Tseng C. K. (2004). Screening marine algae from China for their antitumor activities. J. Appl. Phycol 16, 451–456. doi: 10.1007/s10811-004-5508-x
Yuan Y. V., Westcott N. D., Hu C., Kitts D. D. (2009). Mycosporine-like amino acid composition of the edible red alga, Palmaria palmata (dulse) harvested from the west and east coasts of Grand Manan Island, New Brunswick. Food Chem. 112, 321–328. doi: 10.1016/j.foodchem.2008.05.066
Keywords: seaweed, Laminaria, epiphyte biomass, anticancer activity, antimicrobial activity, Mycobacterium tuberculosis, marine by-products, zero-waste
Citation: Barouti A, Herfindal L, Vagolu SK, Homberset H, Tønjum T, Kopplin G and Jordheim M (2024) Evaluation of the cytotoxic and antimicrobial potential of epiphytic biomass obtained from Laminaria hyperborea biorefinery side-streams. Front. Mar. Sci. 11:1384136. doi: 10.3389/fmars.2024.1384136
Received: 08 February 2024; Accepted: 02 April 2024;
Published: 22 April 2024.
Edited by:
Santhiyagu Prakash, Tamil Nadu Fisheries University, IndiaReviewed by:
Ramasamy Ramasubburayan, Manonmaniam Sundaranar University, IndiaCopyright © 2024 Barouti, Herfindal, Vagolu, Homberset, Tønjum, Kopplin and Jordheim. This is an open-access article distributed under the terms of the Creative Commons Attribution License (CC BY). The use, distribution or reproduction in other forums is permitted, provided the original author(s) and the copyright owner(s) are credited and that the original publication in this journal is cited, in accordance with accepted academic practice. No use, distribution or reproduction is permitted which does not comply with these terms.
*Correspondence: Monica Jordheim, bW9uaWNhLmpvcmRoZWltQHVpYi5ubw==; Lars Herfindal, bGFycy5oZXJmaW5kYWxAdWliLm5v
Disclaimer: All claims expressed in this article are solely those of the authors and do not necessarily represent those of their affiliated organizations, or those of the publisher, the editors and the reviewers. Any product that may be evaluated in this article or claim that may be made by its manufacturer is not guaranteed or endorsed by the publisher.
Research integrity at Frontiers
Learn more about the work of our research integrity team to safeguard the quality of each article we publish.