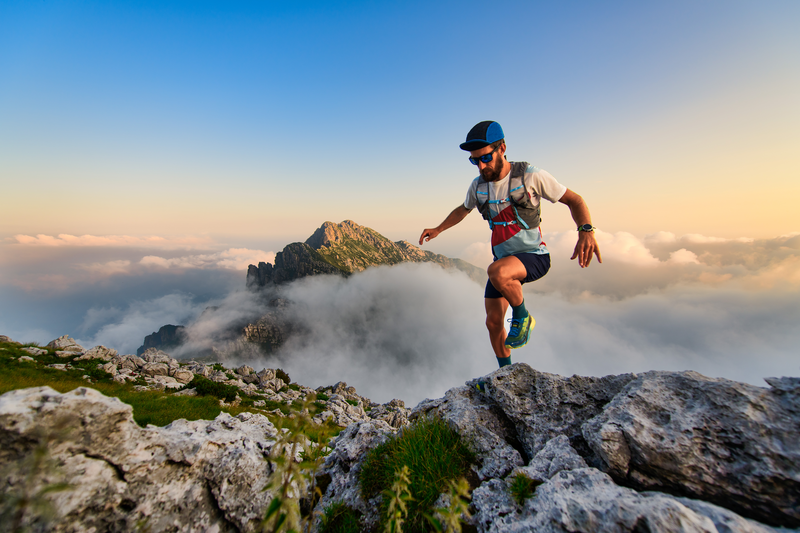
95% of researchers rate our articles as excellent or good
Learn more about the work of our research integrity team to safeguard the quality of each article we publish.
Find out more
PERSPECTIVE article
Front. Mar. Sci. , 23 December 2024
Sec. Marine Fisheries, Aquaculture and Living Resources
Volume 11 - 2024 | https://doi.org/10.3389/fmars.2024.1384083
This article is part of the Research Topic Towards an Expansion of Sustainable Global Marine Aquaculture View all 13 articles
Blue foods from aquaculture are essential in bridging the protein gap to feed the human population in the future. However, for aquaculture production to be sustainable, production must be within planetary boundaries, and sourcing of sustainable raw materials is a key driver in sustainable production. This article explores the role of single-cell proteins (SCPs) derived from microorganisms in aquafeeds. Three main aspects are discussed: sustainability, scalability of fermentation technology, and fish performance. In addition, and through a comprehensive proof-of-concept trial with rainbow trout (Oncorhynchus mykiss), this article demonstrates SCP’s efficacy in replacing traditional feed ingredients without compromising fish growth and health. The trial’s findings demonstrate a high protein digestibility and a balanced amino acid profile, as well as health benefits measured through oxidative burst response. To date, commercial adoption of SCP has been hindered by high production costs and the need for substantial investments to scale fermentation technologies. However, the sustainability landscape is changing as large industry players openly commit to sustainability targets and realize that longer-term and investment thinking into the future is needed. In conclusion, SCP emerges as a promising avenue for sustainable aquafeeds, offering a solution to the protein supply challenge within planetary boundaries. In addition, in terms of environmental benefit, SCP shows clear advantages regarding land use, carbon emissions, biodiversity impact, and water consumption. Ultimately, the successful integration of SCP into aquafeeds could significantly contribute to the industry’s sustainability goals and play an essential role in securing the future supply of raw material proteins.
Aquaculture has become the fastest-growing animal protein industry, and it has been identified as one of the main blue food industries that play a critical role to meet the protein gap of 100 million (dry) MTs annually estimated by 2050 for our growing human population (FAO, 2022). However, this food production must be delivered within planetary boundaries, and one of the drivers of sustainable aquaculture is the use of sustainable raw materials. For many years, the aquaculture industry has been searching for novel or alternative protein-rich raw materials to complement a raw material basket of marine ingredients, animal by-products, and plant ingredients. In addition, heightened prices and supply shortages in plant proteins have been recorded since 2022 due to natural and geopolitical events, which further motivates a drive for alternative protein sources (Aas et al., 2022). Alternative or novel protein candidates include insects, macroalgae, and single-cell organisms or single-cell proteins (SCPs) (Colombo et al., 2023).
SCP products are derived from the fermentation of microorganisms, such as bacteria, yeast, fungi, or microalgae using renewable feedstocks, and provide a readily available protein-rich microbial biomass. While SCP is not a new concept, having been used for human and animal nutrition since the 1960s, biotechnological advances have enabled more efficient and cost-effective production processes (Glencross et al., 2020b; Jones et al., 2020; Sharif et al., 2021). Fermentation technologies are used today to produce food and feed ingredients and additives such as yeast extracts, organic acids, enzymes, and vitamins. Some famous examples of food fermentation products are Spirulina used by NASA for astronaut foods (Soni et al., 2021) and the meat replacement Quorn, which was launched in the 1980s (Gastaldello et al., 2022). However, the industry is still judged with mixed opinions, partly related to the use of synthetic biology concerns and the lack of full understanding related to the environmental, climate, and nature-based benefits of these technologies.
The potentially high protein content (70% or higher) of these microorganisms combined with high growth rates, a balanced amino acid profile that meets the nutritional requirements of various aquaculture species, and the ability to convert a diverse array of platform molecules into protein makes SCP a frontrunner when it comes extending the raw material basket beyond fish meal and plant protein currently used. However, SCP still represents a very low inclusion of protein sources in commercial aquafeeds (Aas et al., 2022). To increase the commercial use of SCP, it is important that production costs decrease, and this requires a substantial increase in investment to produce large volumes in bioreactors located close to aquaculture farming facilities (Sarker, 2023). In this view, investors need reliable and thorough scientific evidence to support decision-making. This article summarizes the landscape for SCP across the three critical levers of (i) sustainability, (ii) scalability and advances in fermentation technology, and (iii) fish nutrition and performance, and presents a recent proof-of-concept trial.
Modern aquafeeds currently rely on relatively high levels of terrestrial plant materials, such as soy protein concentrate, which have their limitations, including high water and land use, pesticide use, biodiversity loss, deforestation risk, greenhouse gas (GHG) emissions, and price volatility (Fry et al., 2016; Glencross et al., 2020a). Despite the fact that the aquaculture industry has become more efficient with improved feed conversion (Glencross et al., 2023a), while also improving its environmental footprint, and reduced dependency on marine proteins from capture fisheries (Aas et al., 2022; Naylor et al., 2021), a large proportion of the environmental or carbon footprint is defined by feed and raw materials (Boyd and McNevin, 2024). In consequence, investing in SCP technologies and optimizing them for aquafeed can help create a more resource-efficient model for aquaculture, promoting a sustainable seafood supply chain that meets growing global demand. The adoption of this alternative needs an extra validation step to show that food and feed safety will not be compromised (Glencross et al., 2020a; Jones et al., 2020).
Figure 1 shows a potential schematic for the process of SCP production. The sustainability of SCPs lies in their ability to address several environmental and resource challenges that potentially enables a near-zero carbon footprint. For example, traditionally fermentation technologies have used sugars as the carbon feedstock, but producing the feedstock molecules from carbon capture and the electrolysis of water to produce hydrogen and oxygen are an emerging possibility. Given that the energy required for electrolysis of water is renewable, then resource use (and GHG emissions) can be minimized when compared to soy protein without land use change (1.8 kg CO2e/kg), Norwegian fish meal (2–3 kg CO2e/kg), and krill (2.8 kg CO2e/kg) and massively reduced with regard to soy protein with land use change (5–7 kg CO2e/kg) (Ulf et al., 2022; Skretting Sustainability report, 2022). In addition, the feedstock production requires very little or no water and land use, compared to proteins derived from agriculture. This feedstock source also limits any reputational and environmental sustainability risks related to deforestation, biodiversity loss, and the protection of marine stocks and biomass. Together with the availability of renewable energy, the choice of microorganism and the management of waste streams can impact the environmental footprint of SCP production. Ongoing research and development are focused on optimizing these processes to enhance sustainability further and full LCA studies are needed to fully understand the impact.
Figure 1. Single-cell protein production from air, water, CO2, and renewable energy (Fackler et al., 2021; Mishra et al., 2020; Molitor et al., 2019).
This is of great importance in the current context, where many companies, including large aquaculture companies, have signed up to science-based targets (https://sciencebasedtargets.org/). Targets for Scope 3 emission reductions by 2030, compared to baselines before 2020, start at 30% and higher. To support these targets, SCPs offer a promising avenue for sustainable feed ingredient production. Their lower footprint, potential to reduce land use, carbon emissions, and minimized water use make them an attractive option for addressing the challenges. In addition, there is limited impact on biodiversity, which will be a vital sustainability metric going forward.
SCPs belong to an extensive library of microorganism options that can grow further as fermentation technology improves and evolves to become an industrial, state-of-the-art process. In addition, because microorganisms have extremely short generation times compared to plants and animals, biotechnology research has a key role to play in the selection and improvement of strains with high and digestible protein content and a targeted amino acid profile for precise nutrition.
Companies working in the pathway towards commercial production are in operation. One example is Calysta (www.calysta.com), and through a joint venture with Calysseo, it will produce 20,000 MT per year in its first FeedKind production plant in China. Other ventures, such as UniBio (Uniprotein®, www.unibio.dk) with a unique U-Loop® technology, have also been reported to be commercial. Emerging companies, such as Solar Foods (https://solarfoods.com/) and Deep Branch (www.deepbranch.com), are not yet at commercial scale. Both UniBio and Calysta use gas fermentation platforms of methanotrophic bacteria to produce the protein-rich product, while others use hydrogen-oxidizing bacteria. dsm-firmenich is a world leader in fermentation technologies and is working on the development of SCPs for aquaculture, companion animals, and food products.
Transitioning to commercial scale requires a large capital investment to scale up and reduce the cost of production to deploy the right product specifications at a commercial sales price. Accordingly, there has always been a benchmark in salmon aquafeed, as an example, to compare price/kg with standard raw materials and less appreciation for an investment price needed as companies ramp up production. At the same time, historically there was less emphasis on environmental and biodiversity impacts and a short-term approach to raw material basket availability and feed market conditions. However, today, many companies from different parts of the aquaculture value chain are making commitments on climate and nature (see, for instance, sciencebasedtargets.org). Accordingly, there is a wider perspective on raw materials in aquafeeds with longer-term goals, return on investment, and sustainability targets becoming more relevant as stakeholders take note that conventional protein sources cannot meet the future demand for protein within planetary boundaries.
Success at scale-up is also determined by the potential of the fermentation platform for commercial quantities, and historically, some start-ups with new bioproducts have failed to commercialize because of lack of scale-up knowledge and practical insight or industrial experience. Financing of the ramp up can also be a barrier as large capital is required for fermentation technologies at commercial scale. Government incentives play a role in site selection, where current government policies and growing climate concerns can support the development. A recent example of government focus is the social sustainable feed mission from the Norwegian government (Et samfunnsløft for bærekraftig fôr), recognizing both the urgency around climate change and the low self-sufficiency of Norway in the raw material supply chain (https://www.forskningsradet.no/en/research-policy-strategy/ltp/sustainable-feed/). For example, in 2021, only 8% of the feed raw materials used in the Norwegian salmon industry were sourced from Norway (Aas et al., 2022). In addition, the European Union has over-dependence on protein supply from outside EU and has adopted import restrictions [carbon border adjustment mechanism (CBAM)]. Simultaneously, the EU has also initiated programs to stimulate protein production (examples include EU Protein Strategy and New Green Deal).
The performance of SCP in fish trials is an important screening stage for the development of alternative raw materials for aquaculture. Because previous studies indicated that some SCP products can have adverse effects on protein digestibility with repercussions on fish growth, health, and welfare (Glencross et al., 2020b), the current trial was designed to go beyond the conventional approach of zootechnical indicators, and therefore also tested the effect of SCP sources in protein digestibility and retention, amino acid digestibility, and health. We showcase two SCP products that were included in rainbow trout (Oncorhynchus mykiss) diets up to 20% inclusion over a 12-week trial.
The trial was conducted in CRNA facilities (Village-Neuf, France; Permit no. 26235). Two different non-GM, SCP products (SCP1 and SCP2: DSM Biotechnology Centre, Delft, The Netherlands) were tested. Experimental diets were formulated to be isonitrogenous and isoenergetic. The six experimental diets (SCP1-5, SCP1-10, SCP1-20, SCP2-5, SCP2-10, and SCP2-20) were compared against a control diet formulated with 10% fish meal and 20% soy protein concentrate (Supplementary Table 1). The experimental diets had SCP inclusion of 5% (SCP1 5% and SCP2 5%), 10% (SCP1 10% and SCP2 10%), and 20% (SCP1 20% and SCP2 20%) of both test products (SCP1 and SCP2). In the 5% and 10% SCP feeds, the SCP replaced fish meal, meaning that the 10% SCP feed did not contain any fish meal. In the 20% SCP feeds, all the fish meal was replaced and 10% of the dietary soy protein concentrate was also replaced with the SCP (as % feed inclusion).
Rainbow trout eyed eggs (generation E21SPR; Aqualor, Fénétrange, France) were hatched at CRNA. Fish were individually weighed at the beginning of the trial (IBW 49.7 ± 0.03 g) and allocated to the experimental groups; each group consisted of three replicate tanks with 20 fish per tank. Fish were fed the experimental diet twice a day for 84 days. At the end of the experiment, zootechnical parameters were assessed: survival (%), body weight (FBW, g), weight gain (WG, g), daily weight gain (DWG, g), specific growth rate (SGR, % BW d−1), and feed conversion ratio (FCR). The detailed formulas for calculation of WG, DWG, SGR, and FCR are provided in the Supplementary Materials. Whole fish samples were taken at the beginning and end of the trial to analyze whole-body protein retention. Samples for apparent digestibility coefficient (ADC) calculation and samples from the head kidney for oxidative burst measurements (as an indicator of health status) were also taken (see the Supplementary Materials).
All diets showed similar performance in final weight (267.0 ± 6.56 g; average ± standard deviation) except for the SCP2 20%, which performed significantly lower than the control, although the difference was less than 5% (256.3 ± 4.9 g). The diet with the highest final mean weight was the SCP2 10% (276.5 ± 1.8 g). In addition, no difference in feed intake values was observed across all treatment groups (data not shown). In recent years, there have been many trials assessing the effect of SCP on fish and shrimp performance (Glencross et al., 2020b; Jones et al., 2020; Sharif et al., 2021). However, it is important to note that results are not always consistent and vary with the level of inclusion, trial design, feed design, initial weight, trial duration, and origin of the SCP organism used for the replacement. For example, the final weight of small Atlantic salmon was significantly lower when 40% of crude protein from FM was substituted with SCP from the yeasts Candida utilis (34.5% dietary inclusion) or Kluyveromyces marxianus (30.2% dietary inclusion) (Øverland et al., 2013). However, at lower levels of inclusion, SCP from the bacterium Methylococcus capsulatus induced no changes in growth performance when used at up to 14% inclusion of the feed in spotted seabass (Yu et al., 2023).
In the present trial, protein ADC was significantly improved in relation to the control diet when either SCP was included in the diet at 10% and 20% inclusion (Table 1). Furthermore, multiple comparisons revealed that inclusion levels but not SCP source had a significant effect on protein ADC, with inclusion at 10% producing the highest protein ADC (p < 0.001, Table 1). Control, SCP1 5%, and SCP2 5% groups showed a lower total amino acid digestibility, but differences did not clearly correlate to growth performance. Essential amino acid digestibility did not show significant differences. Interestingly, branched-chain amino acid digestibility showed a dose response with higher digestibility with increased inclusion levels of SCP1 and showed less differences across SCP2 treatments.
Table 1. Effect of experimental diet on protein apparent digestibility coefficient (ADC), protein retention, and total, essential, non-essential, and branched-chain amino acid ADC.
It has been previously suggested that SCPs in aquafeeds might negatively impact performance due to the lower digestibility of the protein ingredient (e.g., cell walls of microorganisms and non-amino acid protein fraction) and have detrimental impacts on gut health (Glencross et al., 2020b; Jones et al., 2020). However, the results here recorded support the use of SCPs tested because the protein ADC was significantly improved relative to that of the control diet, when SCP1 or SCP2 were included at 10% (Table 1). Moreover, the protein ADCs obtained here (>91%) were higher than those previously recorded for salmonids (80% to 90%) fed SCP diets (Glencross et al., 2023b; Hardy et al., 2018; Kaushik and Luquet, 1980; Lee et al., 2020; Romarheim et al., 2011; Storebakken et al., 2004). Concomitantly, the ADC of amino acids was highest at 10% or 20% inclusion, mainly driven by non-essential and branched-chain amino acids (Table 1). These results are particularly notable for branched-chain amino acids (e.g., leucine, isoleucine, and valine), as these cannot be synthesized by fishes and play significant structural and functional roles (Ahmad et al., 2021). Results here recorded for oxidative burst showed a pattern for an improved dose response for either SCP with increased inclusion levels among experimental diets (no significant differences; Supplementary Table 2). The functional benefits of branched-chain amino acids, as well as the functional components usually present in SCPs that are beneficial for fish health, such as nucleic acids, β-glucans, and microbe-associated molecular patterns, among others (Morales-Lange et al., 2024; Øverland and Skrede, 2017; Øvrum Hansen et al., 2019; Rocha et al., 2023; Romarheim et al., 2011), might warrant the health benefits recorded for fish fed SCP-based diets. This probably explains the nominally positive dose-dependent response found here for oxidative burst, which reflects the increase in cell metabolism and oxygen consumption coupled with the release of reactive oxygen species that serve as the first line of defense against microbial infection. Nevertheless, and considering the non-statistical significance of the oxidative burst pattern here recorded, future studies are needed to confirm the functional benefits of SCP1 and SCP2 under challenging more commercial-like conditions.
In conclusion, digestibility of the amino acids in the finished feed with high inclusion of SCPs showed excellent results, suggesting that the high fish performance observed was related to the high digestibility of the AA in the product. At the same time, feed intake was similar across treatments, demonstrating a high palatability of the ingredient, and indicating that the SCP tested in the current trial had limited negative properties, such as the presence of nucleic acids. Ultimately, the present results successfully demonstrate the potential of incorporating two SCP products in rainbow trout aquafeeds up to 20% dietary inclusion, either as full replacers of FM or as partial replacers of soy protein concentrate.
SCP products are considered low carbon-intensive microbial-sourced proteins that can help close the impending protein gap without increasing the carbon footprint of the aquaculture food system, as well as that of terrestrial species. Indeed, SCP products do not require the extensive use of arable land, freshwater, or marine resources, and they can utilize waste streams as substrates. In addition, microbial organisms hold a huge potential to integrate circular bioeconomy processes because different biomass sources often classified as residues can be used as energy sources to produce single-cell organisms. This will ensure the sustainability of blue and green systems, particularly in the food sector, by partially decoupling animal production from conventional inputs and associated challenges, such as fertilizer use, deforestation, biodiversity impact, and elevated water consumption. Ultimately, SCPs can be seen as a potential driver for decarbonization and circular economy, given that such microorganisms can be powered by renewable energy sources (i.e., solar, wind, or biogas) and concomitantly capture and convert carbon dioxide into biomass. Success at scale also relies on partnerships along the value chain. Each member of the value chain has a role to play in enabling the industrial production of SCP products, from research on the biology of microbial organisms to industry players committed to invest in large-scale production, and feed formulators and farmers, who must be ready to adopt and willing to pay an investment price in view of a more sustainable industry with a lower environmental footprint. In conclusion, the growing momentum behind SCP products and evolving technologies with an ambitious scale, coupled with exciting nutritional value, and their benefits in terms of sustainability metrics, will accelerate the use of SCPs in aquaculture in the future.
The original contributions presented in the study are included in the article/Supplementary Material. Further inquiries can be directed to the corresponding author.
The animal study was approved by project authorization using animals for scientific purposes N°26235 obtained by the Centre of Animal Nutrition and Health (Village-neuf, France) following the legal EU legislation in force Directive 2010/63. The study was conducted in accordance with the local legislation and institutional requirements.
LB: Writing – original draft, Writing – review & editing. HN: Writing – review & editing; CRE: Writing – review & editing. ES: Conceptualization, Methodology, Data curation, Formal analysis, Writing – review & editing.
The author(s) declare that financial support was received for the research, authorship, and/or publication of this article. dsm-firmenich entirely funded the production of the test products and the fish study. The funder was not involved in the study design, collection, analysis, interpretation of data, the writing of this article, or the decision to submit it for publication.
The authors would like to thank P. Cabo-Valcarce, C. Chatelle, P. Jenn, J. Schmeisser, and E. Bacou for conducting the fish trial.
Authors LB, ES, HM, and CE were employed by company dsm-firmenich.
All claims expressed in this article are solely those of the authors and do not necessarily represent those of their affiliated organizations, or those of the publisher, the editors and the reviewers. Any product that may be evaluated in this article, or claim that may be made by its manufacturer, is not guaranteed or endorsed by the publisher.
The Supplementary Material for this article can be found online at: https://www.frontiersin.org/articles/10.3389/fmars.2024.1384083/full#supplementary-material
Aas T. S., Åsgård T., Ytrestøyl T. (2022). Utilization of feed resources in the production of Atlantic salmon (Salmo salar) in Norway: An update for 2020. Aquacult. Rep. 26, 101316. doi: 10.1016/j.aqrep.2022.101316
Ahmad I., Ahmed I., Fatma S., Peres H. (2021). Role of branched-chain amino acids on growth, physiology and metabolism of different fish species: A review. Aquac. Nutr. 27, 1270–1289. doi: 10.1111/anu.13267
Boyd C. E., McNevin A. A. (2024). Resource use and pollution potential in feed-based aquaculture. Rev. Fish. Sci. Aquac. 32, 306–33. doi: 10.1080/23308249.2023.2258226
Colombo S. M., Roy K., Mraz J., Wan A. H. L., Davies S. J., Tibbetts S. M., et al. (2023). Towards achieving circularity and sustainability in feeds for farmed blue foods. Rev. Aquac. 15, 1115–1141. doi: 10.1111/raq.12766
Fackler N., Heijstra B. D., Rasor B. J., Brown H., Martin J., Ni Z., et al. (2021). Stepping on the gas to a circular economy: Accelerating development of carbon-negative chemical production from gas fermentation. Annu. Rev. Chem. Biomol. Eng. 12, 439–470. doi: 10.1146/annurev-chembioeng-120120-021122
FAO. (2022). The state of World Fisheries and Aquaculture 2022. Towards Blue Transformation (Rome: FAO). doi: 10.4060/cc0461en
Fry J. P., Love D. C., MacDonald G. K., West P. C., Engstrom P. M., Nachman K. E., et al. (2016). Environmental health impacts of feeding crops to farmed fish. Environ. Int. 91, 201–214. doi: 10.1016/j.envint.2016.02.022
Gastaldello A., Giampieri F., De Giuseppe R., Grosso G., Baroni L., Battino M. (2022). The rise of processed meat alternatives: A narrative review of the manufacturing, composition, nutritional profile and health effects of newer sources of protein, and their place in healthier diets. Trends Food Sci. Technol. 127, 263–271. doi: 10.1016/j.tifs.2022.07.005
Glencross B. D., Baily J., Berntssen M. H. G., Hardy R., MacKenzie S., Tocher D. R. (2020a). Risk assessment of the use of alternative animal and plant raw material resources in aquaculture feeds. Rev. Aquacult. 12, 703–758. doi: 10.1111/raq.12347
Glencross B. D., Fracalossi D. M., Hua K., Izquierdo M., Mai K., Øverland M., et al. (2023a). Harvesting the benefits of nutritional research to address global challenges in the 21st century. J. World Aquacult. Soc. 54, 343–363. doi: 10.1111/jwas.12948
Glencross B. D., Huyben D., Schrama J. W. (2020b). The application of single-cell ingredients in aquaculture feeds—A review. Fish. Sahul. 5, 22. doi: 10.3390/fishes5030022
Glencross B., Muñoz-Lopez P., Matthew C., MacKenzie S., Powell A., Longshaw M., et al. (2023b). Digestibility of bacterial protein by Atlantic salmon (Salmo salar) is affected by both inclusion level and acclimation time. Aquaculture 565, 739137. doi: 10.1016/j.aquaculture.2022.739137
Hardy R. W., Patro B., Pujol-Baxley C., Marx C. J., Feinberg L. (2018). Partial replacement of soybean meal with Methylobacterium extorquens single-cell protein in feeds for rainbow trout (Oncorhynchus mykiss Walbaum). Aquac. Res. 49, 2218–2224. doi: 10.1111/are.2018.49.issue-6
Jones S. W., Karpol A., Friedman S., Maru B. T., Tracy B. P. (2020). Recent advances in single cell protein use as a feed ingredient in aquaculture. Curr. Opin. Biotechnol. 61, 189–197. doi: 10.1016/j.copbio.2019.12.026
Kaushik S. J., Luquet P. (1980). Influence of bacterial protein incorporation and of sulphur amino acid supplementation to such diets on growth of rainbow trout, Salmo gairdnerii Richardson. Aquaculture 19, 163–175. doi: 10.1016/0044-8486(80)90017-4
Lee S., Chowdhury M. A. K., Hardy R. W., Small B. C. (2020). Apparent digestibility of protein, amino acids and gross energy in rainbow trout fed various feed ingredients with or without protease. Aquaculture 524, 735270. doi: 10.1016/j.aquaculture.2020.735270
Mishra A., Ntihuga J. N., Molitor B., Angenent L. T. (2020). Power-to-protein: Carbon fixation with renewable electric power to feed the world. Joule 4, 1142–1147. doi: 10.1016/j.joule.2020.04.008
Molitor B., Mishra A., Angenent L. T. (2019). Power-to-protein: converting renewable electric power and carbon dioxide into single cell protein with a two-stage bioprocess. Energy Environ. Sci. 12, 3515–3521. doi: 10.1039/C9EE02381J
Morales-Lange B., Hansen J.Ø., Djordjevic B., Mydland L. T., Castex M., Mercado L., et al. (2024). Immunomodulatory effects of hydrolyzed Debaryomyces hansenii in Atlantic salmon (Salmo salar L): From the in vitro model to a natural pathogen challenge after seawater transfer. Aquaculture 578, 740035. doi: 10.1016/j.aquaculture.2023.740035
Naylor R. L., Hardy R. W., Buschmann A. H., Bush S. R., Cao L., Klinger D. H., et al. (2021). A 20-year retrospective review of global aquaculture. Nature 591, 551–563. doi: 10.1038/s41586-021-03308-6
Øverland M., Karlsson A., Mydland L. T., Romarheim O. H., Skrede A. (2013). Evaluation of Candida utilis, Kluyveromyces marxianus and Saccharomyces cerevisiae yeasts as protein sources in diets for Atlantic salmon (Salmo salar). Aquaculture 402–403, 1–7. doi: 10.1016/j.aquaculture.2013.03.016
Øverland M., Skrede A. (2017). Yeast derived from lignocellulosic biomass as a sustainable feed resource for use in aquaculture. J. Sci. Food Agric. 97, 733–742. doi: 10.1002/jsfa.2017.97.issue-3
Øvrum Hansen J., Hofossæter M., Sahlmann C., Ånestad R., Reveco-Urzua F. E., Press C. M., et al. (2019). Effect of Candida utilis on growth and intestinal health of Atlantic salmon (Salmo salar) parr. Aquaculture 511, 734239. doi: 10.1016/j.aquaculture.2019.734239
Rocha S. D. C., Morales-Lange B., Montero R., Teklay Okbayohanese D., Kathiresan P., Press C. M., et al. (2023). Norway spruce extracts (NSEs) as bioactive compounds in novel feeds: Effect on intestinal immune-related biomarkers, morphometry and microbiota in Atlantic salmon pre-smolts. J. Funct. Foods. 111, 105888. doi: 10.1016/j.jff.2023.105888
Romarheim O. H., Øverland M., Mydland L. T., Skrede A., Landsverk T. (2011). Bacteria grown on natural gas prevent soybean meal-induced enteritis in Atlantic salmon. J. Nutr. 141, 124–130. doi: 10.3945/jn.110.128900
Sarker P. K. (2023). Microorganisms in fish feeds, technological innovations, and key strategies for sustainable aquaculture. Microorganisms 11, 439. doi: 10.3390/microorganisms11020439
Sharif M., Zafar M. H., Aqib A. I., Saeed M., Farag M. R., Alagawany M. (2021). Single cell protein: Sources, mechanism of production, nutritional value and its uses in aquaculture nutrition. Aquaculture 531, 735885. doi: 10.1016/j.aquaculture.2020.735885
Soni R. A., Sudhakar K., Rana R. S., Baredar P. (2021). Food supplements formulated with Spirulina, in: Algae. Springer. Singapore. Singapore. pp, 201–226. doi: 10.1007/978-981-15-7518-1
Storebakken T., Baeverfjord G., Skrede A., Olli J. J., Berge G. M. (2004). Bacterial protein grown on natural gas in diets for Atlantic salmon, Salmo salar, in freshwater. Aquaculture 241, 413–425. doi: 10.1016/j.aquaculture.2004.07.024
Ulf H., Nistad A. ,. A., Aiegler F., Shraddha M., Wocken Y., Hognes E. S. (2022). Greenhouse gas emissions of Norwegian Salmon products, SINTEF rapport; 2022:01198 A, SINTEF Ocean As. (Norway: SINTEF Ocean AS).
Yu M.-H., Li X.-S., Wang J., Longshaw M., Song K., Wang L., et al. (2023). Substituting fish meal with a bacteria protein (Methylococcus capsulatus, Bath) grown on natural gas: Effects on growth non-specific immunity and gut health of spotted seabass (Lateolabrax maculatus). Anim. Feed. Sci. Technol. 296, 115556. doi: 10.1016/j.anifeedsci.2022.115556
Keywords: novel, bacteria, yeast, fungi, protein, salmonids, digestibility, aquaculture
Citation: Buttle L, Noorman H, Roa Engel C and Santigosa E (2024) Bridging the protein gap with single-cell protein use in aquafeeds. Front. Mar. Sci. 11:1384083. doi: 10.3389/fmars.2024.1384083
Received: 08 February 2024; Accepted: 03 December 2024;
Published: 23 December 2024.
Edited by:
Xinxin Wang, Akvaplan niva AS, NorwayReviewed by:
Pavan Kumar, Guru Angad Dev Veterinary and Animal Sciences University, IndiaCopyright © 2024 Buttle, Noorman, Roa Engel and Santigosa. This is an open-access article distributed under the terms of the Creative Commons Attribution License (CC BY). The use, distribution or reproduction in other forums is permitted, provided the original author(s) and the copyright owner(s) are credited and that the original publication in this journal is cited, in accordance with accepted academic practice. No use, distribution or reproduction is permitted which does not comply with these terms.
*Correspondence: Louise Buttle, bG91aXNlLmJ1dHRsZUBkc20tZmlybWVuaWNoLmNvbQ==
Disclaimer: All claims expressed in this article are solely those of the authors and do not necessarily represent those of their affiliated organizations, or those of the publisher, the editors and the reviewers. Any product that may be evaluated in this article or claim that may be made by its manufacturer is not guaranteed or endorsed by the publisher.
Research integrity at Frontiers
Learn more about the work of our research integrity team to safeguard the quality of each article we publish.